- 1School of Karst Science, Guizhou Normal University, Guiyang, China
- 2State Engineering Technology Institute for Karst Desertification Control, Guiyang, China
- 3Huaxi District Plant Protection Station of Guiyang City, Guiyang, China
- 4Guizhou Provincial Key Laboratory for Rare Animal and Economic Insect of the Mountainous Region, Guiyang University, Guiyang, China
Introduction: The tea green leafhopper, Empoasca (Matsumurasca) onukii Matsuda, R., 1952 (Hemiptera: Cicadellidae), is currently one of the most devastating pests in the Chinese tea industry. The long-term use of chemical pesticides has a negative impact on human health, impeding the healthy and sustainable development of the tea industry in this region. Therefore, there is a need for non-chemical insecticides to control E. onukii in tea plants. The essential oils from plants have been identified for their potential insecticidal ability; however, there is a lack of knowledge regarding the effect of plant essential oils on E. onukii and its gene expression.
Methods: In order to address these knowledge gaps, the components of Pogostemon cablin and Cinnamomum camphora essential oils were analyzed in the present study using gas chromatography-mass spectrometry. The fumigation toxicity of two essential oils on E. onukii was tested using sealed conical flasks. In addition, We performed comparative transcriptome analyses of E. onukii treated with or without P. cablin essential oil.
Results: The 36-h lethal concentration (LC50) values for E. onukii treated with P. cablin and C. camphora essential oils were 0.474 and 1.204 μL mL−1 respectively. Both essential oils exhibited the potential to control E. onukii, but the fumigation activity of P. cablin essential oil was more effective. A total of 2,309 differentially expressed genes were obtained by transcriptome sequencing of E. onukii treated with P. cablin essential oil.
Conclusion: Many of differentially expressed genes were found to contain detoxifification genes, indicating that these families may have played an important role when E. onukii was exposed to essential oil stress. We also found differential expression of genes related to redox-related gene families, suggesting the upregulation of genes associated with possible development of drug and stress resistance. This work offers new insights for the prevention and management of E. onukii in the future.
1 Introduction
Empoasca (Matsumurasca) onukii Matsuda, R., 1952 is an important agricultural pest that damages tea plants. By sucking on the young sections of tea plant tips, adults and nymphs deplete the chlorophyll and sap in buds and leaves. Females also place their eggs on the young tips, blocking the usual nutrient passage and causing cessation of bud and leaf growth. The leaf border and leaf tip turn reddish brown and wither when the damage is severe (Jin et al., 2012). Damaged tea leaves are fire-like and easily broken during processing, which negatively impacts tea quality and output and can result in yield reductions by 15%–20%, and up to 50% in extreme cases (Chen et al., 2019; Zhang, 2019; Zhang and Chen, 2015). These insects are small, tend to hide, have overlapping generations, and cause serious damage; hence, their control is difficult. In recent decades, chemical pesticides have been mainly relied on to prevent and control this pest; however, this is a high-cost method that induces strong resistance in the insects and produces pesticide residues that are harmful to human health. The improvement of pesticide residue limits in foreign countries has considerably hindered the export of tea products from China (Fan, 2021); hence, traditional chemical pesticide control can no longer meet current requirements.
Plant essential oils, also known as volatile plant secondary metabolites, are volatile oils with a significant aromatic component that gives aromatic plants a particular odor, flavor, or smell; they are commonly referred to as metabolic by-products of plants (Pavela, 2015). Plant essential oils have recently gained popularity due to their environmental friendliness and safety for non-target organisms when used to control food storage pests, phytophagous pests, and mosquitos (Filomeno et al., 2017; Muturi et al., 2017; Visakh et al., 2022). Fumigation or exposure to plant essential oils may have toxic effects on phytophagous insects; in addition, plant essential oils can affect the behavioral activities of insects, including egg laying, reproduction, feeding behavior, growth inhibition, etc. (Su et al., 2018; Ikbal and Pavela, 2019). The mechanism of action of various essential oils varies as a result of the complexity of the essential oil composition. One theory suggests that plant essential oils affect normal growth, development, metamorphosis, and reproduction by acting directly on the nervous system (acetylcholinesterase, ionotropic GABA receptors, and metabolic octopamine receptors) such as octopamine and tyramine receptors that regulate insect metabolism and behavior, thus affecting normal growth, development, metamorphosis, and reproduction (Blenau et al., 2012; Oboh et al., 2017). However, because invertebrates only have octopamine receptors, plant essential oils used for pest control do not pose a risk to mammals (Jankowska et al., 2018). Additionally, plant essential oils affect insect respiration, for example, by inhibiting mitochondrial membrane respiratory enzymes and controlling oxygen uptake and carbon dioxide output (Gao et al., 2020). One mechanism of plant essential oils is thought to be inhibition of the oxidative system of insects, which causes generation of reactive oxygen species and induces oxidative stress (Chen et al., 2021a). Recent transcriptome research has demonstrated that insects upregulate the expression of their detoxifying enzyme genes in response to plant secondary compounds, maintaining their ability to function normally (Sierra et al., 2021; Ding et al., 2022).
A more comprehensive understanding of agricultural pest biology has been enabled by the development of transcriptomics. The use of dsRNA to silence resistance genes in pests to improve insecticide efficiency has gradually become a hot issue. Genes associated with E. onukii resistance to pesticides have been reported. For example, Zhang (2019) investigated thiamethoxam resistance in E. onukii and discovered that numerous detoxifying genes in E. onukii were involved in the drug resistance process. According to Chen (2021), the P450 gene (CYP307A) was associated with imidacloprid metabolism and was crucial for nymph molting behavior. However, there are few studies on the effect of plant essential oils on the gene expression profile of E. onukii. Therefore, the initial stage of the present study involved identifying the differentially expressed genes through P. cablin essential oil stress on E. onukii to provide a foundation for its subsequent prevention and control.
2 Materials and methods
2.1 Plant essential oil and tested insects
The two steam-distilled essential oils used in this study (P. cablin and C. camphora) were purchased from Ji’an Zhongxiang Natural Plant Co., Ltd. (Jiangxi, China) in June, 2022. E. onukii were collected from Yuhuang Ancient Tea Factory in Huaxi District, Guiyang City, China (106°75′E, 26°32′N) in June, 2022. The captured insects were placed in an insect cage (75 × 75 × 75 cm) with fresh tea branches and leaves. One day later, adults exhibiting strong activity and consistent morphology were selected for use in the fumigation test.
2.2 Fumigation test
The fumigation activity of essential oils against E. onukii was tested using sealed conical flasks (Zhang et al., 2020). Plant essential oils were diluted with acetone to five concentrations: 1, 0.5, 0.25, 0.125, and 0.0625 μL mL−1. Twenty adult E. onukiis were inoculated in a 250 mL triangular flask, and fresh tea leaves were added. Using a pipette, 30 μL of treatment solution was absorbed onto the filter paper. To keep the filter paper suspended in the bottle and away from the bottle wall, it was placed in the center of the sealing film. An equivalent volume of acetone solution was then added as a blank control after sealing using the sealing film. Each treatment was repeated four times, and E. onukii mortality was recorded after 12, 24, and 36 h.
Data analysis was performed using SPSS (IBM, version 26.0). Fumigation data were analyzed by probit analysis. The probit-log (concentration) regression model was used to calculate lethal concentration 50% (LC50) values and 95% confidence limits.
2.3 Analysis of essential oil components
Gas chromatography‒mass spectrometry (GC‒MS) (HP6890/5975C, Agilent, United States) was used to analyze the essential oil components. The chromatographic column was a HP-5MS (60 m × 0.25 mm × 0.25 μm) elastic quartz capillary column. The injection volume was 0.2 µL, and the initial temperature of the chromatographic column was 70°C (2 min retention); the temperature was increased to 208°C at 3.5°C/min and then to 310°C at 6°C/min. The running time was 58.43 min; the vaporization chamber temperature was 250°C; and the carrier gas was high purity He (99.999%). The pre-column pressure was 18.43 psi, the carrier gas flow rate was 1.0 mL/min, the split ratio was 50:1, and the solvent delay time was 6 min. The ion source was electron ionization, the ion source temperature was 230°C, the quadrupole temperature was 150°C, the electron energy was 70 eV, the emission current was 34.6 μA, the multiplier voltage was 1494 V, the interface temperature was 280°C, and the mass range was 29–500 amu. The peaks in the total ion chromatogram were searched using a mass spectrometry computer data system, and the Nist17 and Wiley275 standard mass spectra were checked to determine the volatile chemical components. The relative mass fraction of each chemical component was determined using the peak area normalization method.
2.4 Transcriptome samples
The fumigation test method for insects was the same as that described in Section 2.2. The LC50 value of P. cablin essential oil was used as the treatment concentration. Treatment (TM1, TM2, TM3) and control groups (CK1, CK2, CK3) were set up, each with 50 individuals. After 12 h of fumigation, the adults were collected and placed under dry ice for rapid freezing and stored at −80°C. They were then transported to Shanghai Ouyi Biomedical Technology Co., Ltd. for RNA extraction, library sequencing, and data analysis.
2.5 RNA extraction and library construction
Total RNA was extracted from E. onukii using Trizol reagent (Invitrogen, United States) and DNase I (Promega, Madison, WI, United States) to remove residual DNA according to the manufacturer’s instructions. The quality of extracted RNA samples was verified by 1% agarose gel electrophoresis, and the extracted total RNA integrity (RIN value) was digitally evaluated again using an Agilent 2,100 Bioanalyzer (Agilent Technologies, Santa Clara, CA, United States). The library was constructed using the TruSeq Stranded mRNA LTSample Prep Kit (Illumina, San Diego, CA, United States) according to the manufacturer’s instructions. The constructed library was subjected to quality inspection using an Agilent 2,100 Bioanalyzer (Agilent Technologies, Santa Clara, CA, United States), and then sequenced on the Illumina HiSeqTM 2,500 platform (Illumina, San Diego, CA, United States) to produce 125 or 150 bp double-ended data.
2.6 Data preprocessing, quality control, and assembly
Sequencing and data analysis were completed by Shanghai OE Biotech Co., Ltd. (Shanghai, China). The raw reads generated in high-throughput sequencing were fastq format sequences. Raw reads were filtered to obtain high-quality reads for subsequent analysis. First, Trimmomatic (version: 0.36) software was used for quality control and removal of joints. On this basis, low-quality and N bases were filtered out and high-quality clean reads were finally obtained (Bolger et al., 2014). We used Trinity (version: 2.4) software to de novo assemble the data (Grabherr et al., 2011). CD-HIT (version: 4.6) software was used to cluster and remove redundancy, and the obtained Unigene was used as a reference sequence for subsequent analysis (Li et al., 2001).
Diamond software was used to align the Unigene to Non-Redundant Protein Sequence Database (NR) (https://www.ncbi.nlm.nih.gov/), clusters of euKaryotic Orthologous Groups (KOG) (ftp://ftp.ncbi.nih.gov/pub/COG/KOG/kyva), Gene Ontology (GO) (http://www.geneontol-ogy.org/), Swiss-Prot (http://www.uniprot.org/), Evolutionary Genealogy of Genes: Non-supervised Orthologous Groups (eggNOG) (http://eggnog.embl.de/), and Kyoto Encyclopedia of Genes and Genomes (KEGG) (http://www.genome.jp/kegg/pathway.html) databases (Buchfink et al., 2015). HMMER software was used to compare the Pfam (http://pfam.xfam.org/) database for functional analysis of the Unigene (Mistry et al., 2013).
2.7 Differentially expressed gene screening and identification
Bowtie 2 software (version: 2.3.3.1) was used to obtain the number of reads aligned to the Unigene in each sample, and eXpress software (version: 1.5.1) was used to calculate the Unigene expression (fragments per kilobase of transcript per million mapped reads [FPKM] value) (Langmead and Salzberg, 2012; Roberts, 2013). After obtaining the counts, the protein-coding genes required filtering, and genes with mean counts >2 were retained for further analysis. DESeq2 software (version: 1.20.0) was used to standardize the counts number of each sample gene (using the BaseMean value to estimate the expression level), calculate the fold change, and for the Negative Binomial distribution test (NB) for the difference significance test (p-value) (Love et al., 2014). Differentially expressed genes with a p-value <0.05 and fold change >2 were selected, and GO and KEGG enrichment analysis were performed to determine the biological functions or pathways mainly affected by the differential Unigenes.
Unigene sequences were sequentially compared in the order of priority of NR, SwissProt, and KOG databases, and the protein with the highest score was selected to determine its coding region sequence, which was translated into the amino acid sequence according to the standard codon table to obtain the nucleic acid sequence (sequence direction 5'→3′) and amino acid sequence of this Unigene coding region. Using annotation data from seven major databases, potential detoxification-related genes were identified, and the BLASTp function of the National Center for Biotechnology Information (NCBI) was then used to confirm the protein that the candidate gene encoded to verify whether it was a detoxification-related gene.
2.8 Quantitative real-time‒polymerase chain reaction (qRT-PCR) real-time fluorescence quantitative verification
RNA integrity was evaluated using a NanoDrop 2000 spectrophotometer (Thermo Scientific, United States) and agarose gel electrophoresis. The RNA to be tested was reverse transcribed into cDNA using a TransScript All-in-One First-Strand cDNA Synthesis SuperMIX for qPCR (ransgen biotech, Beijing, China) kit according to the manufacturer’s instructions. Using β-actin (KJ476139.1) as an internal reference gene, Shanghai Ouyi Biomedical Technology Co., Ltd. designed primers for seven randomly selected genes and internal reference genes using the Primer-BLAST online program (https://www.ncbi.nlm.nih.gov/tools/primer-blast/), which were synthesized by Beijing Qingke Xinye Biotechnology Co., Ltd. The PerfectStartTM Green qPCR SuperMix (transgen biotech, Beijing, China) kit was used to react on a LightCycler® 480 II fluorescence quantitative PCR instrument (Roche, Swiss). The amount of gene expression was calculated using the 2−ΔΔCt method (Livak and Schmittgen, 2001). The primer information of genes is shown in Table 1.
3 Results
3.1 Fumigation activity
Table 2 displays the fumigation toxicity of essential oils from P. cablin and C. camphora to E. onukii. The 12-, 24-, and 36-h LC50 values of E. onukii treated with P. cablin essential oil were 1.041, 0.659, and 0.474 μL mL−1, respectively (Table 2; Supplementary Table S13). The 12-, 24-, and 36-h LC50 values of E. onukii treated with C. camphora essential oil were 2.21, 1.581, and 1.204 μL mL−1, respectively. The LC50 value decreased gradually with the increase in exposure time. The 12-h LC50 value of P. cablin oil was used as the subsequent transcriptome treatment concentration.
A total of 26 compounds were identified in P. cablin essential oil. Table 3 shows that patchouli alcohol, δ-guaiene, α-guaiene, cembrene, α-patchoulene, aciphyllene, caryophyllene, and β-patchoulene were the main components of P. cablin essential oil, accounting for 85.124% of the total essential oils (Supplementary Figure S1B). The highest content was that of patchouli alcohol (30.811%), and the lowest was that of D-ramie (0.012%).
3.2 De novo assembly
After quality control, we assembled the transcriptome data (Supplementary Figure S1A). The transcript sequence was obtained using Trinity software (version 2.4), and the longest sequence was selected as the Unigene. The Unigene was obtained by clustering and removing redundancy using CD-HIT software (version 4.6). Finally, 46,071 Unigenes were obtained, with a total length of 48,202,476 bp and a length distribution ranging from 301 to 23,439 bp (Supplementary Date Sheet S1). Among the obtained Unigenes, 16,979 had a length from 300 to 500 bp, accounting for 36.85% of the total Unigenes. A total of 14,067 were located from 500 to 1,000 bp, accounting for 30.53% of the total Unigenes. There were 9,217 Unigenes located from 1,000 to 2000 bp, accounting for 20% of the total Unigenes. The N50 length of Unigenes was 1,554 bp, which was greater than the average length (1,046.27 bp), indicating high integrity of the gene samples (Figure 1A; Supplementary Table S9A).
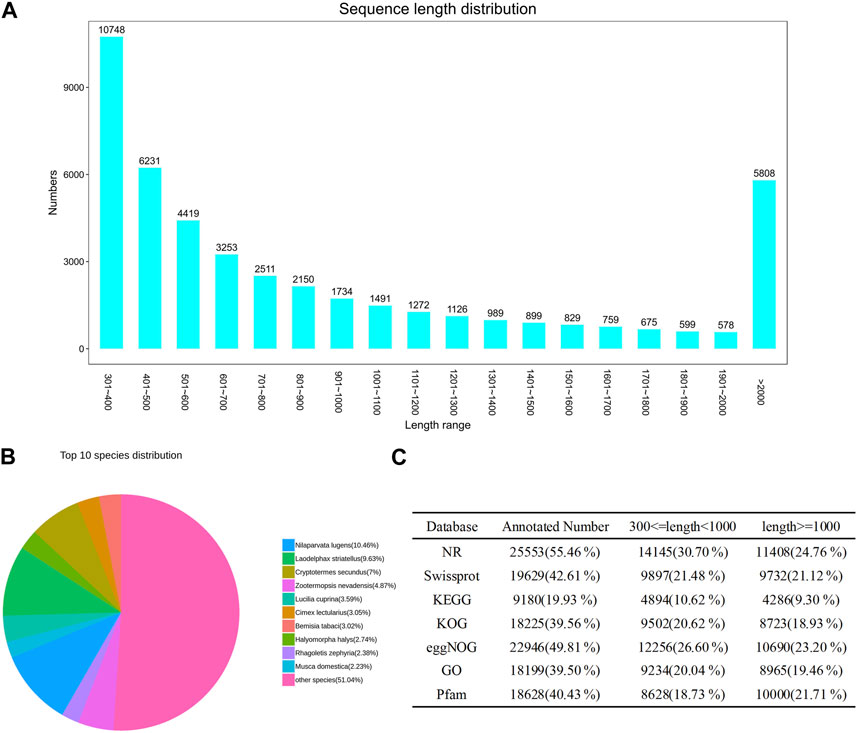
FIGURE 1. (A) Unigene length distribution diagram showing the length of the assembled transcripts generated in the de novo assembly using Trinity after combining all RNA-seq replicates. (B) Annotated top 10 species distribution in NR database, fan shapes of different colors indicate the proportion of annotated species. (C) Number of unigenes annotated to different databases (NR, Swissprot, KEGG, KOG, eggNOG, GO, Pfam).
3.3 Functional annotation of all unigenes
We annotated the obtained Unigenes in NR, Swissprot, KEGG, KOG, eggNOG, GO, and Pfam, from which the numbers of annotated genes were 25,553 (55.46%), 19,629 (42.61%), 9,180 (19.93%), 18,225 (39.56%), 22,946 (49.81%), 18,199 (39.50%), and 18,628 (40.43%), respectively (Figure 1C; Supplementary Table S8). There were 6,421 Unigenes annotated in all seven databases, accounting for 13.94% of the total Unigenes. The NR database had the highest annotation rate, with 25,553 Unigenes annotated, accounting for 55.46% of the total; of these, 14,145 (30.70%) were between 300 and 1,000 bases, and 11,408 (24.76%) were >1,000 bases. The gene sequences of known species in the NR database were compared to the Unigene constructed for E. onukii. Nilaparvata lugens (10.46%), Laodelphax striatellus (9.63%), and Cryptotermes secundus (7%) had the highest alignment rates; i.e., 2,672, 2,462, and 1,789 genes were aligned, respectively (Figure 1B; Supplementary Table S9B). The genes annotated into the GO database could be divided into three categories: biological process, cell component, and molecular function, and could be further divided into 60 subcategories according to their specific functions (Figure 2A; Supplementary Table S10A); the terms “cellular process,” “cell,” and “cell part” were the most dominant, respectively. The assembled Unigenes were annotated in the KOG database and the annotated genes could be divided into 25 functional categories (Figure 2B; Supplementary Table S10B). The most annotated genes were general function prediction only (3,345), followed by signal transduction mechanisms (2,448) and posttranslational modification, protein turnover, and chaperones (1,829).
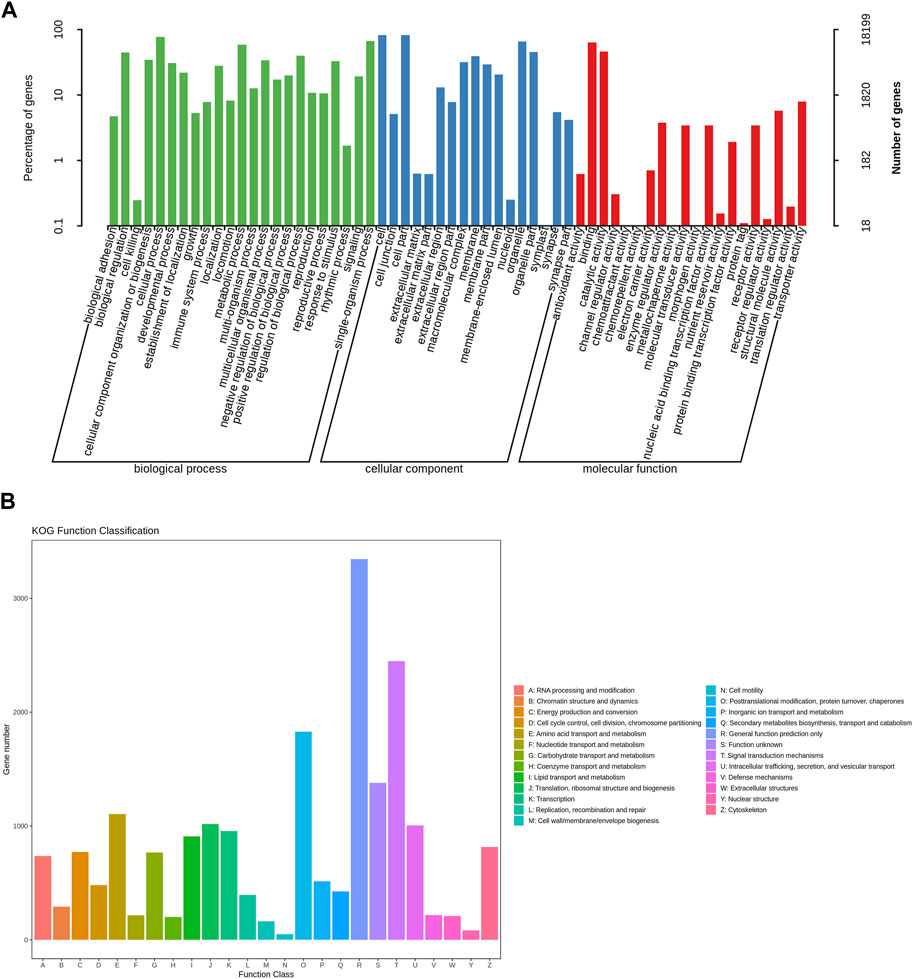
FIGURE 2. The functional annotation of assembled unigenes of E. onukii. (A) GO classifications of assembled unigenes. (B) KOG categories of assembled unigenes.
3.4 Screening and functional analyses of differentially expressed genes
After obtaining the FPKM values of all genes, differentially expressed genes were screened (Supplementary Tables S5–S7). A total of 2,309 differentially expressed genes were found between the control and treatment groups, of which 1,597 showed upregulation and 712 showed downregulation (Supplementary Table S2). The differential expression volcano map is shown in Figure 3A; Supplementary Table S11A. The greater the difference multiple and the higher the -log10 p-value, the stronger the significance of the gene difference and the further the abscissa was from the zero scale.
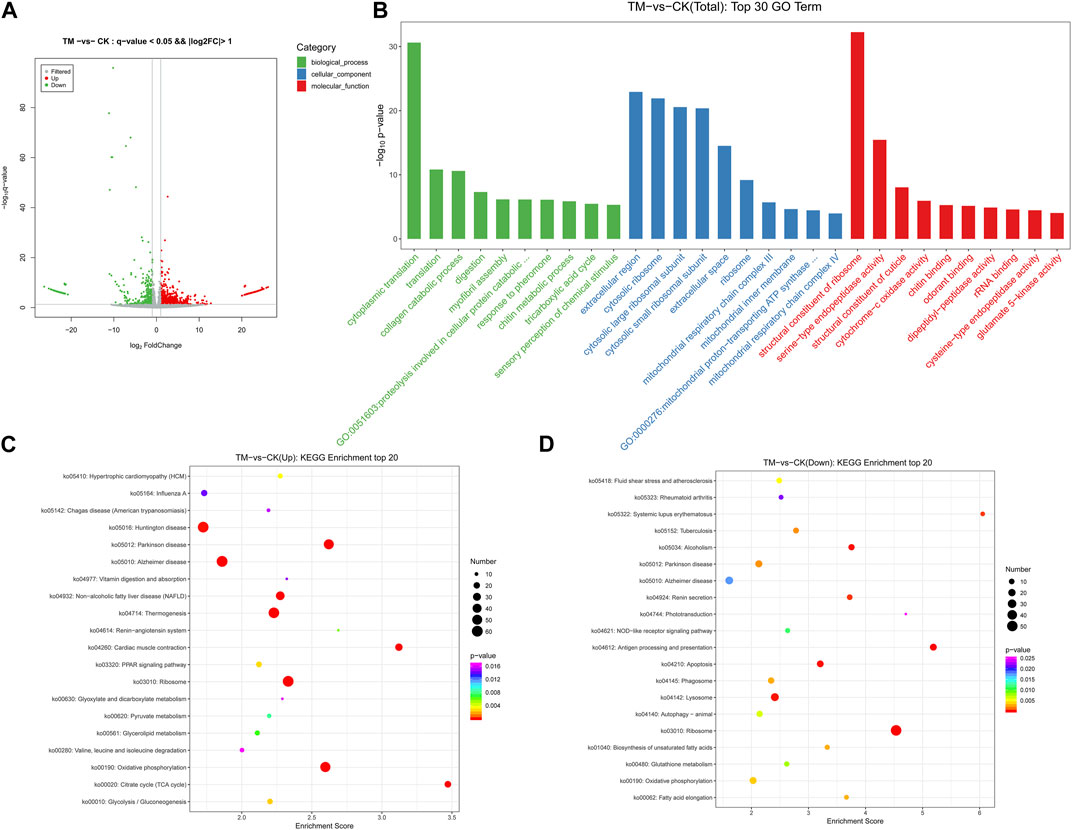
FIGURE 3. (A) Volcano map of differentially expressed genes (DEGs) of E. onukii after exposure of adult to the LC50 value of P. cablin essential oil. (B) Gene Ontology (GO) top 30 enriched terms of differentially expressed genes (DEGs) of E. onukii after exposure of adult to the LC50 value of P. cablin essential oil. (C) Bubble map of the upregulated gene KEGG enrichment pathway and (D) the downregulated gene KEGG enrichment pathway of E. onukii after exposure of adult to the LC50 value of P. cablin essential oil. Pathway significance is shown together with p-value (color), rich factor (vertical ordinate), and a number of involved genes (size of circles).
From the 2,309 differentially expressed genes, a total of 1,211 genes were annotated to the GO functional database, and the top 10 significantly enriched items in three categories were selected (Figure 3B; Supplementary Table S11B). The most significantly enriched biological processes were cytoplasmic translation, translation, and the collagen catabolic process, with 62, 64, and 34 genes, respectively, accounting for 5.12, 5.28, and 2.81%, respectively, of the total. The extracellular region, cytosolic ribosome, and cytosolic large ribosomal subunit were the three most significantly enriched cell components, with 172 (14.2%), 42 (3.47%), and 52 (4.29%) genes, respectively. Among the molecular functions, the structural constituent of ribosome, serine-type endopeptidase activity, and structural constituent of cuticle were the most significantly enriched, with 120 (9.91%), 82 (6.77%), and 23 (1.9%) genes, respectively.
KEGG enrichment analysis showed that a total of 488 differentially expressed genes were categorized into 292 pathways (Supplementary Table S11C). Figure 3C presents the most significant enrichment of the top 20 pathways. Oxidative phosphorylation, Parkinson disease, ribosome, thermogenesis, cardiac muscle contraction, the tricarboxylic acid cycle, Alzheimer disease, non-alcoholic fatty liver disease, and other pathways, were significantly enriched, and the numbers of differentially expressed genes were 52, 50, 59, 57, 26, 21, 60, and 37, respectively. A total of 217 differentially expressed genes were classified into 199 pathways, according to KEGG enrichment analysis of downregulated genes. Ribosome, antigen processing and presentation, apoptosis, alcoholism, lysosome, renin secretion, systemic lupus erythematosus, and tuberculosis had 51, 17, 16, 132, 24, 10, 6, and 11 differential genes, respectively (Figure 3D; Supplementary Table S11D).
3.5 qRT-PCR verification
To verify the accuracy of RNA-seq sequencing results, we used β-actin as an internal reference gene, and randomly selected seven genes among the differentially expressed genes for verification (Supplementary Figures S2A–P). Figure 4A shows that the qRT-PCR sequencing results were consistent with the RNA-seq results, indicating that the results of the above transcriptome sequencing were accurate and reliable (Supplementary Table S12).
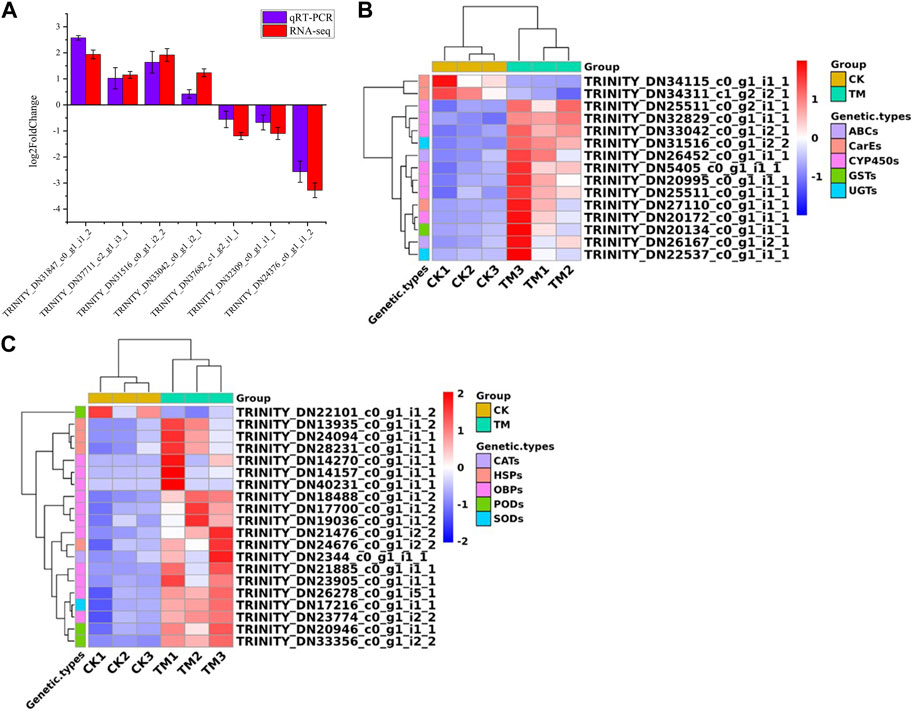
FIGURE 4. (A) Quantitative Real-time PCR validation of transcriptome sequencing results. (B) Differentially expressed genes related to detoxification among differential genes. (C) Differentially expressed genes related to redox and stress resistance. The gene expression (mean ± SD) quantified as a relative fold change was carried outusing the 2−ΔΔCT method.
3.6 Analysis of detoxification-related genes in differentially expressed genes
Through identification and screening of differential genes, we found 14 detoxification genes (Figure 4B; Supplementary Table S3). Seven CYP450 genes were identified, all of which showed upregulation and had log2FoldChange values ranging from 1.96 to 4.37. Two carboxylesterase (CarE) genes showed downregulation; whereas, one GST gene, two ABC genes, the UGT gene, and one CarE gene showed upregulation.
Among the differentially expressed genes, we identified redox genes, including one upregulated superoxide dismutase (SOD) gene; one upregulated catalase (CAT) gene; two upregulated peroxidase (POD) genes; and one downregulated POD gene (Figure 4C; Supplementary Table S4). Furthermore, 11 OBP genes that may be involved in drug tolerance, and four heat shock protein (HSP) genes associated with insect stress resistance, showed upregulation.
4 Discussion
P. cablin essential oil and its related compounds have been demonstrated to have some insecticidal efficacy against a variety of pests in numerous studies. Depending on where it is obtained from, P. cablin essential oil may be categorized as transitional, patchouli alcohol, or pogostone type (Luo et al., 2003; Hu et al., 2006). According to the GC‒MS results, the first six main components of the tested essential oil were patchouli alcohol (30.811%), δ-guaiene (17.085%), α-guaiene (13.711%), caryophyllene (7.252%), α-patchoulene (5.095%), and pogostone (1.272%); hence, the essential oil was patchouli alcohol type. The most commonly reported bioactive substance found in P. cablin is pogostone. Zeng et al. (2006) found that P. cablin essential oil and its compound pogostone had an anti-feedant effect on Pieris rapae and Plutella xylostella larvae and certain contact activity against P. rapae. In addition, patchouli alcohol has also been reported to have strong insecticidal activity against Aedes aegypti (Muturi et al., 2019).
Essential oil from C. camphora and its compounds have insecticidal properties against a variety of insects. The first seven primary components of oil from C. camphora were determined by GC‒MS to be (+)-2-camphor (25.817%), safrole (13.597%), eucalyptol (11.269%), linalool (6.343%), nerolidol (5.852%), D-limonene (5.359%), and α-terpineol (4.618%). Cinnamomum camphora essential oil and its main component eucalyptol are effective against Anopheles stephensi larva and P. xylostella (Xu et al., 2020; Huang et al., 2021). Linalool is toxic to Aphis gossypii and Anticarsia gemmatalis (Jiang et al., 2016; Vicenco et al., 2021). Safrole is toxic to Sitophilus zeamais and A. aegypti (Carmen et al., 2015; Liu et al., 2015). D-limonene has strong fumigation, repellent, and ovicidal effects on Callosobruchus maculatus, Tribolium castaneum, Lasioderma serricorne, and Loposcelis bostrychophila (Qi et al., 2021; Rodrigues et al., 2022). The fumigation activity of essential oils from P. cablin and C. camphora against E. onukii was evaluated using sealed conical flasks, but tea green leafhoppers damage the tea in the open field. Therefore, this should be considered a limitation in the study and future research would address contact toxicity assays to validate our results both in the laboratory and in the field.
In our study, the 36-h semi-lethal concentrations of P. cablin and C. camphora essential oils against E. onukii were 0.474 and 1.204 μL mL−1, respectively. The fumigation toxicity exhibited certain time- and concentration-dependent effects. With increases in time and concentration, the insecticidal activity was further improved, which was consistent with the fumigation results of P. cablin essential oil against Tetranychus cinnabarinus (Cheng et al., 2020). The fact that many of the compounds in the essential oil exhibit a wide range of biological activities, including contact killing, repulsiveness, growth and development inhibition, and fumigation against other insects, suggests that the essential oil may also be effective against E. onukii.
It is generally believed that detoxification of exogenous substances by insects is mainly divided into three stages. Stage I mainly involves oxidation, reduction, and hydrolysis of exogenous substances involved in P450 enzymes and esterases, which makes toxic molecules introduce polar groups (Schama et al., 2016). Cytochrome P450 monooxygenases (P450s) catalyze multiple oxidative transformations of endogenous and exogenous substrates (Li et al., 2007; Nelson, 2017), which plays an important role in plant‒host interactions and metabolism of different insecticides (Philippou et al., 2010; Alptekin et al., 2016). Insecticides and plant secondary compounds are highly metabolized by several CYP450 family genes (Li et al., 2004; Sasabe et al., 2004; Wang et al., 2017). In our study, a total of seven CYP450 genes were upregulated in the essential oil treatment group, indicating that CYP450 genes may have been involved in the detoxification and metabolism of P. cablin essential oil. One of the most common varieties of esterases is CarE, which performs a wide range of physiological functions in organisms, participating in the metabolism of several endogenous compounds (including hormones, pheromones, and neurotransmitters) as well as the detoxification of various exogenous substances (Wei et al., 2019; Hilliou et al., 2021). CarEs play a key role in the defense of various plant secondary metabolites related to plants and insecticides (Oakeshott et al., 2005). In the present study, two CarE genes were downregulated, indicating that P. cablin essential oil may have inhibited the role of CarE in phase I detoxification metabolism by reducing expression of CarE genes in E. onukii. This is consistent with the reported inhibition of plant essential oil on CarE activity of S. zeamais, P. xylostella larvae, and Haemaphysalis longicornis (Liao et al., 2016; Qiao et al., 2021).
The process of binding reaction products in the second stage, which is mostly performed by GSTs and UGTs, causes the reaction products from stage I to join with a number of endogenous substances (such as glutathione [GSH] and glucuronic acid) to generate water-soluble molecules (Glisic et al., 2015; Lushchak et al., 2018). GSTs are extremely varied detoxifying phase II enzymes. In addition to their roles in intracellular transport and oxidative stress reduction, GSTs facilitate excretion of harmful substances out of the cell by increasing the binding of reduced GSH to toxic substances (Lushchak, 2012; Zhu and Luttrell, 2015). In our study, one GST gene was upregulated and may have been involved in the process of catalyzing the binding of GSH to toxic substances, thereby reducing the toxicity of plant essential oils to the insects (Liao et al., 2016; Gao et al., 2020). Many different organisms contain UDP glucuronosyltransferase families (UGTs), the mechanisms of action of which resemble those of GSTs. Two UGT genes were upregulated in our study, suggesting that E. onukii may hasten the hydrolysis rate of the compound by highly expressing UGTs.
ATP-binding cassette transporters are crucial in stage III, which primarily delivers the reaction products from stage II to the extracellular area (Vieira-Brock et al., 2013). ABC transporters either isolate harmful molecules into tissues that are not metabolically active, rendering them inert, or move some toxic compounds away from their targets (Medina et al., 2002; Gott et al., 2017). The detoxification mediated by ABC transporters in many insects has attracted attention (You et al., 2013; Mastrantonio et al., 2017). Based on the aforementioned circumstance, we hypothesize that the ABC transporter in E. onukii may have accelerated the excretion efficiency of essential oils and indirectly participated in the detoxification process.
One of the mechanisms of insecticides is thought to be their ability to cause oxidative stress in insects (Akbar et al., 2012; Lazarevic et al., 2020; Czerniewicz and Chrzanowski, 2021). In our study, we found significant enrichment of superoxide dismutase activity (GO: 0004784), response to oxidative stress (GO: 0006979), oxidation-reduction process (GO: 0055114) and response to ethanol (GO: 0045471) pathways. Two SOD genes, one CAT gene, and two POD genes were upregulated, and one POD gene was downregulated. Other insects also exhibit an increase in the transcriptional expression level of antioxidant enzymes induced by toxic compounds; two SOD genes (MnSOD and ecCuZnSOD1) in Oxya chinensis had higher expression levels after exposure to the insecticide malathion; when these two genes were knocked out, the level of reactive oxygen species in the body increased considerably (Wu et al., 2017). Therefore, we infer that after fumigation with P. cablin essential oil, E. onukii may have produced a certain amount of reactive oxygen species, which increased the transcription level of antioxidant enzymes in the body so as to eliminate the excess reactive oxygen species in the cells to maintain the oxidative balance.
Nine OBPs genes were found in the two enriched pathways of odorant binding (GO: 0005549) and sensory perception of chemical stimulus (GO: 0007606). OBPs are extremely small water-soluble secretory proteins that are found in the lymph of insect olfactory receptors. They play an important role in the selective binding and transportation of fat-soluble odor molecules and have other functions such as anti-inflammatory effects, regeneration and development, visual pigment carrier, and nutritional effects (Leal, 2013; Pelosi et al., 2014; Pelosi et al., 2018). Recent studies have shown that the high expression of these genes is related to the drug resistance of various insects such as Diaphorina citri, N. lugens, Culex quinquefasciatus, and T. castaneum (Liu et al., 2020; Gao et al., 2021; Shen et al., 2022; Zhang et al., 2022). Expressions of nine OBPs were upregulated in this study, and we theorized that this increased the tolerance of E. onukii to essential oil. Insect HSPs play an important role under heat stress, cold stress, and insecticide treatment (Koo et al., 2015; Dumas et al., 2019). We found that four HSPs were upregulated between the differential genes. Interestingly, similar phenomena also occur when other insects are exposed to insecticide stress (Dong et al., 2022). The HSP90, sHSP, and HSP70 gene families of Anopheles sinensis are considered to be involved in pyrethroid resistance (Si et al., 2019). We hypothesized that the upregulated HSP may have enhanced stress resistance in E. onukii.
GO enrichment analysis showed that items related to mitochondrial respiration were significantly enriched (p < 0.05), including cytochrome c oxidase activity (GO: 0004129), mitochondrial respiratory chain complex III (GO: 0005750), mitochondrial electron transport, ubiquinol to cytochrome c (GO: 0006122), mitochondrial respiratory chain complex IV (GO: 0005751), and NADH dehydrogenase (ubiquinone) activity (GO: 0008137). The genes involved in the above items were significantly upregulated, indicating that plant essential oils affected respiration. In addition, in the KEGG pathway, we found that the transcripts in the respiration-related oxidative phosphorylation (ko00190) glycolysis/gluconeogenesis (ko00010) citrate cycle (tricarboxylic acid cycle) (ko00020) pathway were significantly upregulated, indicating enhanced respiration after essential oil exposure. Most insecticides have some effect in inhibiting insect respiration, for example, pyrethroids, which stop the oxidative phosphorylation chain and lower the respiratory rate in Anopheles gambiae (Ingham et al., 2021). It appeared that inhibiting E. onukii respiration was not the target of the essential oil in the present study. Excessive energy used for respiration produces may be used to achieve detoxification (Mu et al., 2005).
5 Conclusion
P. cablin essential oil demonstrated strong insecticidal action, according to the examination of the constituent parts of two essential oils. P. cablin essential oil-stressed transcriptome sequencing of E. onukii revealed 2,309 differentially expressed genes between the treatment and control groups. These genes belonged to five gene families involved in detoxification, as well as redox, HSP, and OBP genes. The identification of these genes offers a theoretical framework for further investigation and development of effective, low-toxicity insecticides for managing E. onukii. In addition, due to the complex composition of the essential oils used in this study, the toxicity of the single compound of the essential oil and the mixture of various compounds can be further compared in future studies to determine the main compounds that exert biological activity and the mixing modes to achieve the optimal control effect.
Data availability statement
The datasets presented in this study can be found in online repositories. The names of the repository/repositories and accession number(s) can be found below: https://www.ncbi.nlm.nih.gov/bioproject/PRJNA974347.
Ethics statement
The manuscript presents research on animals that do not require ethical approval for their study.
Author contributions
All authors contributed to the study conception and design. Material preparation, data collection and analysis were performed by WT, NZ, JW, TP, JH, CL, and YS. The first draft of the manuscript was written by WT and all authors commented on previous versions of the manuscript. All authors contributed to the article and approved the submitted version.
Funding
This research was funded by the World Top Discipline Program of Guizhou Province: Karst Ecoenvironment Sciences (No. 125 2019 Qianjiao Keyan Fa), the Innovation Group Project of Education Department of Guizhou Province ((2021)013), the National Natural Science Foundation of China (32260120), the Natural Science Foundation of Guizhou Province (Qiankehejichu-ZK (2023) General 257) and the Science and Technology Project of Guiyang City ((2020)7-18).
Conflict of interest
The authors declare that the research was conducted in the absence of any commercial or financial relationships that could be construed as a potential conflict of interest.
Publisher’s note
All claims expressed in this article are solely those of the authors and do not necessarily represent those of their affiliated organizations, or those of the publisher, the editors and the reviewers. Any product that may be evaluated in this article, or claim that may be made by its manufacturer, is not guaranteed or endorsed by the publisher.
Supplementary material
The Supplementary Material for this article can be found online at: https://www.frontiersin.org/articles/10.3389/fphys.2023.1217608/full#supplementary-material
References
Akbar S. M., Sharma H. C., Jayalakshmi S. K., Sreeramulu K. (2012). Methylparathion- and carbofuran-induced mitochondrial dysfunction and oxidative stress in Helicoverpa armigera (noctuidae: lepidoptera). Pest. Biochem. Physiol. 103 (1), 31–37. doi:10.1016/j.pestbp.2012.02.005
Alptekin S., Bass C., Nicholls C., Paine M. J. I., Clark S. J., Field L., et al. (2016). Induced thiacloprid insensitivity in honeybees (Apis mellifera L.) is associated with up-regulation of detoxification genes. Insect Mol. Biol. 25 (2), 171–180. doi:10.1111/imb.12211
Blenau W., Rademacher E., Baumann A. (2012). Plant essential oils and formamidines as insecticides/acaricides: what are the molecular targets? Apidologie 43 (3), 334–347. doi:10.1007/s13592-011-0108-7
Bolger A. M., Lohse M., Usadel B. (2014). Trimmomatic: a flexible trimmer for Illumina sequence data. Bioinformatics 30 (15), 2114–2120. doi:10.1093/bioinformatics/btu170
Buchfink B., Xie C., Huson D. H. (2015). Fast and sensitive protein alignment using diamond. Nat. Methods 12 (1), 59–60. doi:10.1038/nmeth.3176
Carmen H. R., Carolina R. M., Itzel B. M., Gonzalo S. A., Angélica U. P., Inés F. C., et al. (2015). Bioactivity of peumus boldus molina, laurelia sempervirens (ruiz & pav.) tul. and laureliopsis philippiana (looser) schodde (monimiacea) essential oils against Sitophilus zeamais motschulsky. Chil. J. Agric. Res. 75 (3), 334–340. doi:10.4067/S0718-58392015000400010
Chen H. (2021). “Study on the function of Cytochrome P450 genes in tee green leafhopper (Empoasca flavescens F.) in response to sublethal concentrations of imidacloprid,” ([Hangzhou]: China Jiliang University). [master's thesis]. doi:10.27819/d.cnki.gzgjl.2021.000609
Chen L. L., Yuan P., Pozsgai G., Chen P., Zhu H., You M. S. (2019). The impact of cover crops on the predatory mite Anystis baccarum (Acari, Anystidae) and the leafhopper pest Empoasca onukii (Hemiptera, Cicadellidae) in a tea plantation. Pest Manag. Sci. 75 (12), 3371–3380. doi:10.1002/ps.5489
Chen Y. Z., Zhang B. W., Yang J., Zou C. S., Li T., Zhang G. C., et al. (2021a). Detoxification, antioxidant, and digestive enzyme activities and gene expression analysis of Lymantria dispar larvae under carvacrol. J. Asia-Pac. Entomol. 24 (1), 208–216. doi:10.1016/j.aspen.2020.12.014
Cheng Z., Duan H., Zhu X., Fan F., Li R., Li S., et al. (2020). Effects of patchouli and wormwood oils on the bioassays and behaviors of Tetranychus cinnabarinus (boisduval) (Acari: tetranychidae). Int. J. Pest Manage. 66 (3), 271–278. doi:10.1080/09670874.2019.1636155
Czerniewicz P., Chrzanowski G. (2021). The Effect of Santolina chamaecyparissus and Tagetes patula essential oils on biochemical markers of oxidative stress in aphids. Insects 12 (4), 360. doi:10.3390/insects12040360
Ding C. Y., Ma Y. M., Li B., Wang Y., Zhao L., Peng J. N., et al. (2022). Identification and functional analysis of differentially expressed genes in Myzus persicae (Hemiptera: aphididae) in response to trans-anethole. J. Insect Sci. 22 (1), 3. doi:10.1093/jisesa/ieab094
Dong B., Liu X. Y., Li B., Li M. Y., Li S. G., Liu S. (2022). A heat shock protein protects against oxidative stress induced by lambda-cyhalothrin in the green peach aphid Myzus persicae. Pest. Biochem. Physiol. 181, 104995. doi:10.1016/j.pestbp.2021.104995
Dumas P., Morin M. D., Boquel S., Moffat C. E., Morin P. J. (2019). Expression status of heat shock proteins in response to cold, heat, or insecticide exposure in the Colorado potato beetle Leptinotarsa decemlineata. Cell Stress Chaperone 24 (3), 539–547. doi:10.1007/s12192-019-00983-3
Fan Y. P. (2021). Research on China's tea export countermeasures under the background of the belt and road initiatives. China J. Commer. (05), 1–3. doi:10.19699/j.cnki.issn2096-0298.2021.05.001
Filomeno C. A., Barbosa L., Teixeira R. R., Pinheiro A. L., Farias E. D., Silva E., et al. (2017). Corymbia spp. and Eucalyptus spp. essential oils have insecticidal activity against Plutella xylostella. Ind. Crop. Prod. 109, 374–383. doi:10.1016/j.indcrop.2017.08.033
Gao S. S., Lu R. X., Zhang Y. L., Sun H. D., Li S. Y., Zhang K. P., et al. (2021). Odorant binding protein C12 is involved in the defense against eugenol in Tribolium castaneum. Pest. Biochem. Physiol. 179, 104968. doi:10.1016/j.pestbp.2021.104968
Gao S. S., Zhang K. P., Wei L. T., Wei G. Y., Xiong W. F., Lu Y. Y., et al. (2020). Insecticidal activity of Artemisia vulgaris Essential Oil and transcriptome analysis of Tribolium castaneum in response to oil exposure. Front. Genet. 11, 589. doi:10.3389/fgene.2020.00589
Glisic B., Mihaljevic I., Popovic M., Zaja R., Loncar J., Fent K., et al. (2015). Characterization of glutathione-S-transferases in zebrafish (Danio rerio). Aquat. Toxicol. 158, 50–62. doi:10.1016/j.aquatox.2014.10.013
Gott R. C., Kunkel G. R., Zobel E. S., Lovett B. R., Hawthorne D. J. (2017). Implicating ABC transporters in insecticide resistance: research strategies and a decision framework. J. Econ. Entomol. 110 (2), 667–677. doi:10.1093/jee/tox041
Grabherr M. G., Haas B. J., Yassour M., Levin J. Z., Thompson D. A., Amit I., et al. (2011). Full-length transcriptome assembly from RNA-Seq data without a reference genome. Nat. Biotechnol. 29 (7), 644–652. doi:10.1038/nbt.1883
Hilliou F., Chertemps T., Maibeche M., Le Goff G. (2021). Resistance in the genus spodoptera: key insect detoxification genes. Insects 12 (6), 544. doi:10.3390/insects12060544
Hu L. F., Li S. P., Cao H., Liu J. J., Gao J. L., Yang F. Q., et al. (2006). GC-MS fingerprint of Pogostemon cablin in China. J. Pharm. Biomed. Anal. 42 (2), 200–206. doi:10.1016/j.jpba.2005.09.015
Huang X., Huang Y. L., Yang C. Y., Liu T. T., Liu X., Yuan H. B. (2021). Isolation and insecticidal activity of essential oil from Artemisia lavandulaefolia DC. against Plutella xylostella. Toxins 13 (12), 842. doi:10.3390/toxins13120842
Ikbal C., Pavela R. (2019). Essential oils as active ingredients of botanical insecticides against aphids. J. Pest Sci. 92 (3), 971–986. doi:10.1007/s10340-019-01089-6
Ingham V. A., Brown F., Ranson H. (2021). Transcriptomic analysis reveals pronounced changes in gene expression due to sub-lethal pyrethroid exposure and ageing in insecticide resistance Anopheles coluzzii. BMC Genomics 22 (1), 337. doi:10.1186/s12864-021-07646-7
Jankowska M., Rogalska J., Wyszkowska J., Stankiewicz M. (2018). Molecular targets for components of essential oils in the insect nervous system—a review. Molecules 23 (1), 34. doi:10.3390/molecules23010034
Jiang H., Wang J., Song L., Cao X., Yao X., Tang F., et al. (2016). GC×GC-TOFMS analysis of essential oils composition from leaves, twigs and seeds of Cinnamomum camphora L. Presl and their insecticidal and repellent activities. Molecules 21 (4), 423. doi:10.3390/molecules21040423
Jin S., Chen Z. M., Backus E. A., Sun X. L., Xiao B. (2012). Characterization of EPG waveforms for the tea green leafhopper, Empoasca vitis gothe (Hemiptera: cicadellidae), on tea plants and their correlation with stylet activities. J. Insect Physiol. 58 (9), 1235–1244. doi:10.1016/j.jinsphys.2012.06.008
Koo J., Son T. G., Kim S. Y., Lee K. Y. (2015). Differential responses of Apis mellifera heat shock protein genes to heat shock, flower-thinning formulations, and imidacloprid. J. Asia-Pac. Entomol. 18 (3), 583–589. doi:10.1016/j.aspen.2015.06.011
Langmead B., Salzberg S. L. (2012). Fast gapped-read alignment with Bowtie 2. Nat. Methods 9 (4), 357–359. doi:10.1038/nmeth.1923
Lazarevic J., Jevremovic S., Kostic I., Kostic M., Vuleta A., Manitasevic J. S., et al. (2020). Toxic, oviposition deterrent and oxidative stress effects of Thymus vulgaris essential oil against Acanthoscelides obtectus. Insects 11 (9), 563. doi:10.3390/insects11090563
Leal W. S. (2013). Odorant reception in insects: roles of receptors, binding proteins, and degrading enzymes. Annu. Rev. Entomol. 58, 373–391. doi:10.1146/annurev-ento-120811-153635
Liu X. Q., Jiang H. B., Liu Y., Fan J. Y., Ma Y. J., Yuan C. Y., et al. (2020). Odorant binding protein 2 reduces imidacloprid susceptibility of Diaphorina citri. Pest. Biochem. Physiol. 168, 104642. doi:10.1016/j.pestbp.2020.104642
Li W., Jaroszewski L., Godzik A. (2001). Clustering of highly homologous sequences to reduce the size of large protein databases. Bioinformatics 17 (3), 282–283. doi:10.1093/bioinformatics/17.3.282
Li X., Baudry J., Berenbaum M. R., Schuler M. A. (2004). Structural and functional divergence of insect CYP6B proteins: from specialist to generalist cytochrome P450. Proc. Natl. Acad. Sci. U. S. A. 101 (9), 2939–2944. doi:10.1073/pnas.0308691101
Li X., Schuler M. A., Berenbaum M. R. (2007). Molecular mechanisms of metabolic resistance to synthetic and natural xenobiotics. Annu. Rev. Entomol. 52, 231–253. doi:10.1146/annurev.ento.51.110104.151104
Liao M., Xiao J. J., Zhou L. J., Liu Y., Wu X. W., Hua R. M., et al. (2016). Insecticidal activity of Melaleuca alternifolia essential oil and RNA-Seq analysis of Sitophilus zeamais transcriptome in response to oil fumigation. PLoS One 11 (12), e0167748. doi:10.1371/journal.pone.0167748
Liu Y., Liu X. C., Liu Q. Y., Niu C., Liu Z. L. (2015). Larvicidal activity of illicium difengpi BN chang (schisandraceae) stem bark and its constituent compounds against Aedes aegypti L. Trop. J. Pharm. Res. 14 (1), 103–109. doi:10.4314/tjpr.v14i1.15
Livak K. J., Schmittgen T. D. (2001). Analysis of relative gene expression data using real-time quantitative PCR and the 2−ΔΔCT method. Methods 25 (4), 402–408. doi:10.1006/meth.2001.1262
Love M. I., Huber W., Anders S. (2014). Moderated estimation of fold change and dispersion for RNA-seq data with DESeq2. Genome Biol. 15 (12), 550. doi:10.1186/s13059-014-0550-8
Luo J. P., Liu Y. P., Feng Y. F., Guo X. L., Hui C. (2003). Two chemotypes of Pogostemon cablin and influence of region of cultivation and harvesting time on volatile oil composition. Acta Pharm. Sin. 38 (04), 307–313. doi:10.16438/j.0513-4870.2003.04.017
Lushchak V. I. (2012). Glutathione homeostasis and functions: potential targets for medical interventions. J. Amino Acids 2012, 736837. doi:10.1155/2012/736837
Lushchak V. I., Matviishyn T. M., Husak V. V., Storey J. M., Storey K. B. (2018). Pesticide toxicity: A mechanistic approach. Excli J. 17, 1101–1136. doi:10.17179/excli2018-1710
Mastrantonio V., Ferrari M., Epis S., Negri A., Scuccimarra G., Montagna M., et al. (2017). Gene expression modulation of ABC transporter genes in response to permethrin in adults of the mosquito malaria vector Anopheles stephensi. Acta Trop. 171, 37–43. doi:10.1016/j.actatropica.2017.03.012
Matsuda R. (1952). A new green Empoasca leafhopper injurious to the tea shrub in Japan. Oyo-Kontyu Tokyo 8, 19–21.
Medina P., Smagghe G., Budia F., Del E. P., Tirry L., Vinuela E. (2002). Significance of penetration, excretion, and transovarial uptake to toxicity of three insect growth regulators in predatory lacewing adults. Arch. Insect Biochem. Physiol. 51 (2), 91–101. doi:10.1002/arch.10053
Mistry J., Finn R. D., Eddy S. R., Bateman A., Punta M. (2013). Challenges in homology search: HMMER3 and convergent evolution of coiled-coil regions. Nucleic Acids Res. 41 (12), e121. doi:10.1093/nar/gkt263
Mu W., Wu K. M., Guo Y. Y., Zhang W. J. (2005). Comparison of the respiratory rate among different resistant strains of the beet armyworm, Spodoptera exigua (hubner) (Lepidoptera: noctuidae) to lambda-cyhalothrin. Acta Entomol. Sin. (06), 881–885. doi:10.16380/j.kcxb.2005.06.011
Muturi E. J., Doll K., Berhow M., Flor-Weiler L. B., Rooney A. P. (2019). Honeysuckle essential oil as a potential source of ecofriendly larvicides for mosquito control. Pest Manag. Sci. 75 (7), 2043–2048. doi:10.1002/ps.5327
Muturi E. J., Ramirez J. L., Doll K. M., Bowman M. J. (2017). Combined toxicity of three essential oils against Aedes aegypti (Diptera: culicidae) larvae. J. Med. Entomol. 54 (6), 1684–1691. doi:10.1093/jme/tjx168
Nelson D. R. (2017). Cytochrome P450 diversity in the tree of life. BBA-Proteins Proteomics 1866 (1), 141–154. doi:10.1016/j.bbapap.2017.05.003
Oakeshott J. G., Devonshire A. L., Claudianos C., Sutherland T. D., Horne I., Campbell P. M., et al. (2005). Comparing the organophosphorus and carbamate insecticide resistance mutations in cholin- and carboxyl-esterases. Chem.-Biol. Interact. 157, 269–275. doi:10.1016/j.cbi.2005.10.041
Oboh G., Ademosun A. O., Olumuyiwa T. A., Olasehinde T. A., Ademiluyi A. O., Adeyemo A. C. (2017). Insecticidal activity of essential oil from orange peels (Citrus sinensis) against Tribolium confusum, Callosobruchus maculatus and Sitophilus oryzae and its inhibitory effects on acetylcholinesterase and Na+/K+-ATPase activities. Phytoparasitica 45 (4), 501–508. doi:10.1007/s12600-017-0620-z
Pavela R. (2015). Essential oils for the development of eco-friendly mosquito larvicides: A review. Ind. Crop. Prod. 76, 174–187. doi:10.1016/j.indcrop.2015.06.050
Pelosi P., Iovinella I., Felicioli A., Dani F. R. (2014). Soluble proteins of chemical communication: an overview across arthropods. Front. Physiol. 5, 320. doi:10.3389/fphys.2014.00320
Pelosi P., Iovinella I., Zhu J., Wang G., Dani F. R. (2018). Beyond chemoreception: diverse tasks of soluble olfactory proteins in insects. Biol. Rev. 93 (1), 184–200. doi:10.1111/brv.12339
Philippou D., Field L., Moores G. (2010). Metabolic enzyme(s) confer imidacloprid resistance in a clone of Myzus persicae (sulzer) (Hemiptera: aphididae) from Greece. Pest Manag. Sci. 66 (4), 390–395. doi:10.1002/ps.1888
Qi X. J., Feng Y. X., Pang X., Du S. S. (2021). Insecticidal and repellent activities of essential oils from seed and root of Celery (Apium graveolens L.) against three stored product insects. J. Essent. Oil Bear. Plants. 24 (5), 1169–1179. doi:10.1080/0972060X.2021.1981159
Qiao Y., Yu Z., Bai L., Li H., Zhang S., Liu J., et al. (2021). Chemical composition of essential oils from Thymus mongolicus, Cinnamomum verum, and Origanum vulgare and their acaricidal effects on Haemaphysalis longicornis (Acari: ixodidae). Ecotox. Environ. Safe. 224, 112672. doi:10.1016/j.ecoenv.2021.112672
Roberts A. (2013). “Ambiguous fragment assignment for high-throughput sequencing experiments,” ([Berkeley]: University of California). [dissertation thesis].
Rodrigues R., Fontes L. D., Brito R. D., Barbosa D., Cito A., Do Carmo I. S., et al. (2022). A sustainable approach in the management of Callosobruchus maculatus: essential oil of protium heptaphyllum and its major compound d-limonene as biopesticides. J. Plant Dis. Prot. 129 (4), 831–841. doi:10.1007/s41348-022-00617-4
Sasabe M., Wen Z., Berenbaum M. R., Schuler M. A. (2004). Molecular analysis of CYP321A1, a novel cytochrome P450 involved in metabolism of plant allelochemicals (furanocoumarins) and insecticides (cypermethrin) in Helicoverpa zea. Gene 338 (2), 163–175. doi:10.1016/j.gene.2004.04.028
Schama R., Pedrini N., Juarez M. P., Nelson D. R., Torres A. Q., Valle D., et al. (2016). Rhodnius prolixus supergene families of enzymes potentially associated with insecticide resistance. Insect biochem. Mol. Biol. 69, 91–104. doi:10.1016/j.ibmb.2015.06.005
Shen R. X., Wang Y. T., Wu J. H., Zhang N., Zhang H. D., Xing D., et al. (2022). Deltamethrin interacts with Culex quinquefasciatus odorant-binding protein: a novel potential resistance mechanism. Parasites Vectors 15, 2. doi:10.1186/s13071-021-05041-5
Si F. L., Qiao L., He Q. Y., Zhou Y., Yan Z. T., Chen B. (2019). HSP superfamily of genes in the malaria vector Anopheles sinensis: diversity, phylogenetics and association with pyrethroid resistance. Malar. J. 18, 132. doi:10.1186/s12936-019-2770-6
Sierra I., Latorre-Estivalis J. M., Traverso L., Gonzalez P. V., Aptekmann A., Nadra A. D., et al. (2021). Transcriptomic analysis and molecular docking reveal genes involved in the response of Aedes aegypti larvae to an essential oil extracted from Eucalyptus. Plos Negl. Trop. Dis. 15 (7), e0009587. doi:10.1371/journal.pntd.0009587
Su L., Qian W. Y., Guo J. Y., Zhang L. (2018). Research progress on pest prevention and control by plant essential oil and its mechanism. J. Inn. Mong. For. Sci. Technol. 44 (01), 61–64.
Vicenco C. B., Silvestre W. P., Lima T. S., Pauletti G. F. (2021). Insecticidal activity of Cinnamomum camphora Ness and Eberm var. linaloolifera Fujita leaf essential oil and linalool against Anticarsia gemmatalis. J. Essent. Oil Res. 33 (6), 601–609. doi:10.1080/10412905.2021.1937353
Vieira-Brock P. L., Andrenyak D. M., Nielsen S. M., Fleckenstein A. E., Wilkins D. G. (2013). Age-related differences in the disposition of nicotine and metabolites in rat brain and plasma. Nicotine Tob. Res. 15 (11), 1839–1848. doi:10.1093/ntr/ntt067
Visakh N. U., Pathrose B., Chellappan M., Ranjith M. T., Sindhu P. V., Mathew D. (2022). Chemical characterisation, insecticidal and antioxidant activities of essential oils from four Citrus spp. fruit peel waste. Food Biosci. 50, 102163. doi:10.1016/j.fbio.2022.102163
Wang R. L., He Y. N., Staehelin C., Liu S. W., Su Y. J., Zhang J. E. (2017). Identification of two cytochrome monooxygenase P450 genes, CYP321A7 and CYP321A9, from the Tobacco Cutworm Moth (Spodoptera Litura) and their expression in response to plant allelochemicals. Int. J. Mol. Sci. 18 (11), 2278. doi:10.3390/ijms18112278
Wei P., Chen M., Nan C., Feng K., Shen G., Cheng J., et al. (2019). Downregulation of carboxylesterase contributes to cyflumetofen resistance in Tetranychus cinnabarinus (Boisduval). Pest Manag. Sci. 75 (8), 2166–2173. doi:10.1002/ps.5339
Wu H., Zhang Y., Shi X., Zhang J., Ma E. (2017). Overexpression of Mn-superoxide dismutase in Oxya chinensis mediates increased malathion tolerance. Chemosphere 181, 352–359. doi:10.1016/j.chemosphere.2017.04.087
Xu Y. T., Qin J., Wang P., Li Q. X., Yu S. S., Zhang Y. L., et al. (2020). Chemical composition and larvicidal activities of essential oil of Cinnamomum camphora (L.) leaf against Anopheles stephensi. Rev. Soc. Bras. Med. Trop. 53, e20190211. doi:10.1590/0037-8682-0211-2019
You M., Yue Z., He W., Yang X., Yang G., Xie M., et al. (2013). A heterozygous moth genome provides insights into herbivory and detoxification. Nat. Genet. 45 (2), 220–225. doi:10.1038/ng.2524
Zeng Q. Q., Cai Y., Yan Z., Wang X., Wang Y. (2006). Studies on insecticidal activity and toxic component of essential oil from Pogostemon cablin. J. Plant Resour. Environ. (03), 21–25.
Zhang H., Li H. L., Wang D. F., Wu G. Y., Wang Q. S. (2020). Toxicity activity of Artemisia lavandulaefola DC essential oil on Empoasca onukii Matsuda. Acta Tea sin. 61 (4), 183–186.
Zhang J., Mao K., Ren Z., Jin R., Zhang Y., Cai T., et al. (2022). Odorant binding protein 3 is associated with nitenpyram and sulfoxaflor resistance in Nilaparvata lugens. Int. J. Biol. Macromol. 209, 1352–1358. doi:10.1016/j.ijbiomac.2022.04.100
Zhang Y. J. (2019). “Resistance mechanism of Empoasca onukii to thiamethoxam,” ([Guiyang]: Guizhou University). [dissertation thesis]. doi:10.27047/d.cnki.ggudu.2019.000070
Zhang Z., Chen Z. (2015). Non-host plant essential oil volatiles with potential for a “push-pull” strategy to control the tea green leafhopper, Empoasca vitis. Entomol. Exp. Appl. 156 (1), 77–87. doi:10.1111/eea.12310
Keywords: Empoasca onukii, plant essential oil, fumigant activity, transcriptome, detoxification genes
Citation: Tan W, Zhang N, Wang J, Pu T, Hu J, Li C and Song Y (2023) Fumigant activity and transcriptomic analysis of two plant essential oils against the tea green leafhopper, Empoasca onukii Matsuda. Front. Physiol. 14:1217608. doi: 10.3389/fphys.2023.1217608
Received: 05 May 2023; Accepted: 04 September 2023;
Published: 19 September 2023.
Edited by:
Joel Vizueta, University of Copenhagen, DenmarkReviewed by:
Youssef Dewer, Agricultural Research Center, EgyptHongbo Jiang, Southwest University, China
Dixit Sharma, Central University of Himachal Pradesh, India
Copyright © 2023 Tan, Zhang, Wang, Pu, Hu, Li and Song. This is an open-access article distributed under the terms of the Creative Commons Attribution License (CC BY). The use, distribution or reproduction in other forums is permitted, provided the original author(s) and the copyright owner(s) are credited and that the original publication in this journal is cited, in accordance with accepted academic practice. No use, distribution or reproduction is permitted which does not comply with these terms.
*Correspondence: Yuehua Song, songyuehua@163.com
†These authors have contributed equally to this work and share first authorship