- 1Department of Neurobiology, Poznań University of Physical Education, Poznań, Poland
- 2Department of Physiology and Biochemistry, Poznań University of Physical Education, Poznań, Poland
Introduction: The effect of brain-derived neurotrophic factor (BDNF) on the modulation of metabolic processes in the liver is poorly understood. Therefore, the aim of this study was to investigate whether hepatic concentrations or activities of metabolic biomarkers depend on altered BDNF/TrkB content in the liver, resulting from different BDNF genotypes of rats. In addition, it was assessed whether 5-week moderate endurance training modifies the levels of BDNF/Trk-B signaling and studied hepatic markers.
Methods: Experiments were performed on wild-type and heterozygous BDNF knockout (HET, SD-Bdnf) rats, which were divided into four groups: control with normal genotype (Bdnf+/+), control with BDNF knockout genotype (Bdnf+/−), trained with normal genotype (Bdnf+/+T) and trained with BDNF knockout genotype (Bdnf +/−T). BDNF/TrkB concentrations as well as selected metabolic biomarkers including lipids—total cholesterol (CHOL), low-density lipoprotein (LDL), triglycerides (TG); enzymes—alanine aminotransferase (ALAT), aspartate aminotransferase (ASAT), gamma-glutamyl transferase (GGT), lactate dehydrogenase (LDH), alkaline phosphatase (ALP); hormones—insulin (INS) and leptin (LEPT) as well as interleukin-6 (IL-6) as regeneration indicator were measured directly in liver homogenates.
Results and Discussion: The study showed that Bdnf+/− rats exhibited reduced BDNF/TrkB signaling (BDNF, p < 0.0001; Trk-B, p = 0.0005), altered lipid levels (CHOL, p < 0.0001; LDL, p < 0.0001; TG, p = 0.0006) and reduced hepatic ALAT (p = 0.0004) and GGT (p < 0.0001) activity, which may contribute to hepatic steatosis and obesity, as well as indicate impairment of specific metabolic pathways in the liver. Interestingly, endurance training did not alter hepatic BDNF and TrkB content, but improved ALAT (p = 0.0366) and ASAT (p = 0.0191) activities and increased hepatic IL-6 (p = 0.0422) levels in Bdnf +/− rats, suggesting enhanced liver regeneration in animals with BDNF allele loss.
Introduction
Brain-derived neurotrophic factor (BDNF) is a protein involved in the modulation of numerous biological processes occurring in the central and peripheral nervous systems in mammals (Li et al., 2022). BDNF has been shown to have the ability to activate transmembrane tyrosine kinase B (Trk-B) receptors in neurons (Guo et al., 2014; Jin, 2020), which triggers various intracellular signaling pathways to alter specific neuronal functions (Yoshii and Constantine-Paton, 2010). However, BDNF and its high-affinity receptors have also been found in skeletal (Mousavi and Jasmin, 2006) and smooth muscles (Aravamudan et al., 2016), the endocrine system (Podyma et al., 2021), adipose tissue (Nakagomi et al., 2015) as well as some inner organs such as the heart (Pius-Sadowska and Machaliński, 2017), pancreas (Fulgenzi et al., 2020) and liver (Genzer et al., 2017), which plays a vital role in many biological processes, including metabolism (Han et al., 2016), detoxification (Grant, 1991), and the production of various hormones and proteins (Canbay et al., 2007).
In the liver, the sources of several neurotrophins and their receptors (including BDNF and Trk-B) are hepatic stellate cells (Cassiman et al., 2001) and hepatocytes (Genzer et al., 2017), which are the main cell types in this organ. The hepatic BDNF/Trk-B axis has been shown to play a key role in maintaining liver innervation and haematopoietic function (García-Suárez et al., 2006) and biliary remodelling during cholestasis (Vivacqua et al., 2014). In addition, it has been shown that hepatic BDNF levels can be useful for diagnosing liver function in patients with cirrhosis (Shu et al., 2019), and associated with liver fibrosis in alcohol use disorders (Girard et al., 2021). Studies in rodents and humans (Chaldakov et al., 2004; Motamedi et al., 2017) have shown that low levels of circulating BDNF are associated with metabolic dysfunctions such as obesity, metabolic syndrome and related disorders (e.g., diabetes, cardiovascular disease). Experiments on mice lacking the gene encoding BDNF (Lyons et al., 1999; Kernie et al., 2000) have shown that exogenous administration of BDNF can reverse the effects of metabolic syndrome. Furthermore, Genzer et al. (2017) showed that BDNF binding to specific receptors in rat liver hepatocytes activates catabolic pathways, such as fatty acid oxidation, in parallel with inhibition of gluconeogenesis and increased glycogen storage. All these reports indicate an important regulatory role for BDNF/TrkB axis in liver tissue (Hang et al., 2021).
It is well known that physical activity, especially of endurance nature, can modulate serum/plasma concentration of BDNF (Szuhany et al., 2015; Dinoff et al., 2016). The results of most human studies suggest considerable elevation of BDNF concentration in blood serum after acute endurance training lasting several weeks (Tang et al., 2008; Rasmussen et al., 2009; Arazi et al., 2021; Ribeiro et al., 2021). Moreover, Belviranlı and Okudan (2018) showed that voluntary wheel training in rats increased not only BDNF levels in plasma but also in liver tissue. In addition, another study in rats showed that the application of moderate to high-intensity training leads to both increased plasma BDNF levels and insulin tolerance, which are suppressed by daily intraperitoneal administration of the Trk-B blocker (Jiménez-Maldonado et al., 2014).
Hence, existing evidence suggests that there may be a strong association between BDNF/Trk-B axis and metabolic products in the liver and physical activity. The purpose of this study was to investigate the potential link between changes in the hepatic BDNF/TrkB axis induced by different BDNF genotype (normal vs. knockout rats) and endurance training (trained vs. untrained rats) and the activity and concentration of metabolic biomarkers in the liver. Knockout rats were selected due to their reduced serum BDNF levels ranging from 20% (Grzelak et al., 2023) to 73% (Harris et al., 2016), while 5-week endurance training was used to increase BDNF levels in the rat liver.
For this reason, two types of rats were used in this study: wild-type and BDNF heterozygous knockout (HET, SD-Bdnf), some of which were subjected to an endurance training procedure. The following four groups of rats were studied in the experiments conducted: 1) with typical hepatic BDNF levels (control with a normal genotype, Bdnf+/+), 2) with altered hepatic BDNF levels [control with BDNF knockout genotype (HET, SD-BDNF, Bdnf+/−)], and (3, 4) trained with normal (Bdnf+/+T) and knockout genotype (Bdnf+/-T) that underwent 5-week endurance training procedure.
In liver homogenates of these rats levels of BDNF, Trk-B as well as concentration/activity of some lipids: total cholesterol (CHOL), low-density lipoprotein (LDL) and triglycerides (TG), enzymes: lactate dehydrogenase (LDH), alanine aminotransferase (ALAT), aspartate aminotransferase (ASAT), gamma-glutamyl transferase (GGT) and alkaline phosphatase (ALP) and hormones: insulin (INS) and leptin (LEPT) were measured. In addition, levels of hepatic interleukin-6 (IL-6) which is an essential factor indicating liver homeostasis, regeneration, infection defence and fine tuning of metabolic functions (Schmidt-Arras and Rose-John, 2016) were also measured in all four studied groups of animals.
Relationships between hepatic amounts of both BDNF/Trk-B and the aforementioned specific metabolic factors were determined separately for each group and then compared among all studied populations. We hypothesised that this approach would allow us to demonstrate how genotype and physical activity affect the association between BDNF/Trk-B axis and metabolic factors in the rat liver.
Materials and methods
Animals
Experiments were carried out in 41 Sprague-Dawley male rats aged 4–5 months, obtained from a breeding colony at SAGE Labs (St. Louis, MO, United States). 21 animals were wild-type and had the typical BDNF genotype (Bdnf-wild type), while the remaining 20 animals were heterozygotes, in which Bdnf was knocked out using targeted zinc finger nuclease technology (Bdnf-knockout, SD-BDNF). The respective liver biomarkers were measured separately in rats from four different randomly selected groups: control normal genotype rats (Bdnf+/+, n = 11, average weight before experiment—524 g), control knockout genotype rats (Bdnf+/−, n = 11, average weight before experiment—681 g), trained normal genotype rats (Bdnf+/+T, n = 10, average weight before experiment—484 g) and trained knockout rats (Bdnf+/−T, n = 9, average weight before experiment—588 g). All animals were kept in standard laboratory cages (2 rats of the same genotype per cage) with ad libitum access to food and water. The room in which the animals were housed had controlled environmental conditions (a reverse 12 h:12 h light/dark cycle, 55% ± 1% humidity, and 22°C ± 2°C). The animals were euthanized with an overdose of sodium pentobarbital (180 mg kg−1) prior to liver collection. The experiments were conducted in accordance with the Polish Animal Protection Act, European Union regulations and ARRIVE guidelines. The experimental procedures were approved by the Local Ethics Committee for Animal Research in Poznań (approval number 58/2018).
Endurance training protocol
In order to examine the effect of endurance training on BDNF/Trk-B signaling and metabolic biomarkers in the liver, moderate intensity training on an electric treadmill for small rodents (Exer-6M, Columbus Instruments) was used in groups of Bdnf+/+T and Bdnf+/-T rats. The training regimen consisted of 1 week of adaptation and 5 weeks of core training (45 min per day, 5 days per week of continuous running with gradually increasing speed up to 25 m/min at the last week of the training session (Grzelak et al., 2023).
Liver collection and homogenization
After anaesthesia, livers of experimental animals were collected, weighed and then placed in cryogenic vials (Yancheng Huida Medical Instruments, China), which were frozen by immersion in liquid nitrogen at–80°C and stored for further measurements. Small distal portions of each lobe of the collected livers were cut off for homogenization. The obtained tissue sections were weighed, and depending on their weight, an appropriate amount (at a ratio of 1:9) of Phosphate Buffered Saline (PBS) with Halt Protease Inhibitor Cocktail EDTA-free (100X) (Thermo Fischer Scientific, United States) was added. Tissue samples were homogenised with the prepared buffer using a dispersion homogenizer (VWR VDI 12, Singapore) in four cycles (each 30 s, with 1 min cooling in ice between cycles) to 30.000 revolutions per minute (rpm). The homogenate was centrifuged at 5,000 rpm by 5 min and the obtained supernatant was kept at—80°C.
Enzyme-linked immunosorbent assay
Levels of BDNF (sensitivity test: 0.035 ng ml−1, cat. number: SRB-T-81493), TrkB (sensitivity test: 12.337 pg/mL; cat. number: 201-11-0426), LEPT (sensitivity test: 7.054 pg/mL; cat number: 201-11-0456), IL-6 (sensitivity test: 1.822 pg/mL; cat number: 201-11-0136) and activity of INS (sensitivity test: 0.102 mIU/L; cat number: 201-11-0708) were determined in liver by commercial ELISA kits according to descriptions of producent (Sunredbio, China). A multi-mode microplate reader (Synergy 2 SIAFRT, BioTek, United States) was used for read of absorbance at 450 nm.
Biochemistry analyses
Levels of LDL (sensitivity test: 3.9 mg/dL; cat. number: 7–280 [Cormay, Poland]), CHOL (sensitivity test: 1.95 mg/dL; cat. number: 7–204 [Cormay, Poland]), TG (sensitivity test: 1.4 mg/dL; cat. number 7–253 [Cormay, Poland]), activities of ALAT (sensitivity test: 8 U/L; cat. number 7–216 [Cormay, Poland]), ASAT (sensitivity test: 7 U/L; cat. number 7–214 [Cormay, Poland]), LDH (sensitivity test: 6.6 U/L; cat number. 7–239 [Cormay, Poland]), GGT (sensitivity test: 8 U/L; cat number 7–224, [Cormay, Poland]), and ALP (sensitivity test: 7.8 U/L; cat number 7–212 [Cormay, Poland]) were measured with clinical chemistry analyzer (Accent 220S, Cormay, Poland).
Statistical analysis
Statistical analyses were carried out with Statistica 13 software (Statsoft, Poland, Cracow). Data were presented as the mean ± standard deviation (SD) for all obtained results. Two-way analysis of variance (ANOVA) was used in order to perform inter-group comparison of molecules concentrations as well as enzymes and hormone activities. Tukey’s HSD post hoc test was employed for determination of statistical significance between compared groups when the interaction between effects was statistically significant.
Results
Liver and body weights
The study showed that the average liver weight of knockout rats was 19% greater than rats with the normal genotype. However, the applied endurance training on treadmill led to a loss of liver weight only in normal genotype rats (by an average of 12%) and did not cause a reduction in the weight of this organ in the group with BDNF knockout genotype. Moreover, our previous study, performed on the same groups of animals (Grzelak et al., 2023), showed that knockout rats had a higher body mass than the normal genotype rats and also indicated that moderate-intensity running training caused weight loss in both trained normal (Bdnf+/+T) and knockout (Bdnf+/−T) rats. A detailed comparison of both body weight and liver weight in the four groups of rats tested (Bdnf+/+, Bdnf+/−, Bdnf+/+T, Bdnf+/−T) is shown in Figure 1.
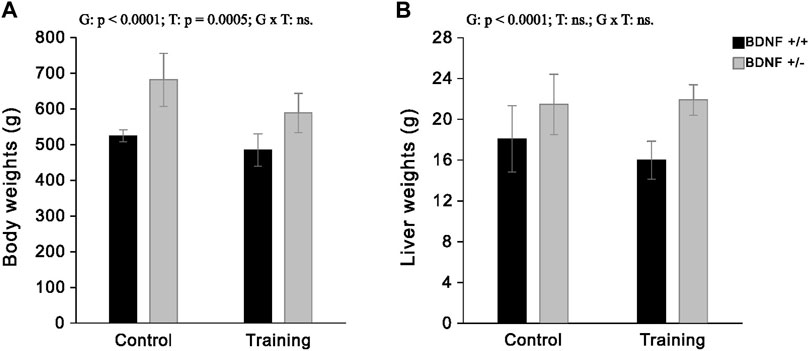
FIGURE 1. The mean values (±SD) of body weights (A) and liver weights (B) of rats from control (left side) and trained (right side) groups. Bdnf+/+ rats are indicated by black, while Bdnf+/− rats are indicated by grey. Comparisons between groups were made by two-way ANOVA. (G) genotype (Bdnf+/+ genotype vs. Bdnf+/− genotype); T—training (control vs. training); G x T—the interaction between genotype and training. For significant interactions Tukey’s post hoc test was performed. Significant results are denoted by p, while non-significant results are denoted by ns. Effects for body weights: Genotype—F = 56.84, p < 0.0001, ηp2 = 0.63; Training—F = 14.76, p = 0.0005, ηp2 = 0.31; Genotype x Training—F = 2.35, p = 0.1351, ηp2 = 0.07. Effects for liver weights: Genotype - F = 29.30, p < 0.0001, ηp2 = 0.47; Training—F = 0.92, p = 0.0005, ηp2 = 0.03; Genotype x Training - F = 2.18, p = 0.1494, ηp2 = 0.06.
Genotype and training effects
BDNF and Trk-B There was a statistically significant reduction in liver levels of both BDNF and Trk-B in rats from the knockout population (Bdnf+/− and Bdnf+/−T) when compared to rats from normal genotype populations (Bdnf+/+ and Bdnf+/+T) (Figures 2A, B; Table 1). Significantly lower level of Trk-B was found in Bdnf+/− group of rats in relation to either Bdnf+/+ or Bdnf+/+T groups of rats (Figure 2B; Table 1). However, no changes in hepatic BDNF and TrkB were found when populations of control (Bdnf+/+ and Bdnf+/−) and trained (Bdnf+/+T and Bdnf+/−T) rats were compared (Figures 2A,B; Table 1).
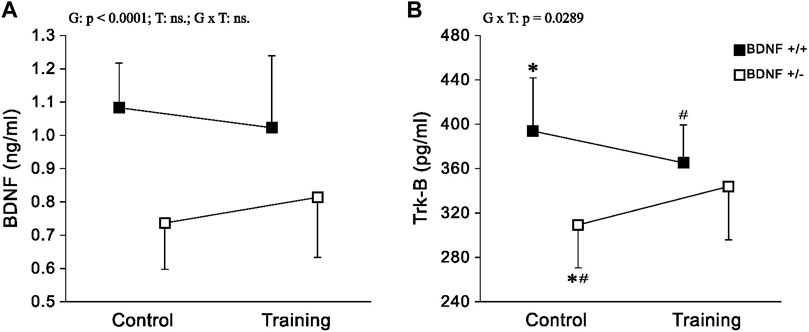
FIGURE 2. The mean values (±SD) of BDNF (A) and Trk-B (B) concentrations in the liver of rats from control (left side) and trained (right side) groups. Control and trained Bdnf+/+ groups are indicated by black square, while control and trained Bdnf+/− groups are indicated by white square. Comparisons between groups were made by two-way ANOVA. (G) genotype (Bdnf+/+ genotype vs. Bdnf+/− genotype); T—training (control vs. training); G x T, the interaction between genotype and training. For significant interactions Tukey’s post hoc test was performed. Post-hoc results: * = significant difference between control rats with Bdnf+/+ genotype and control rats with Bdnf+/− genotype (p = 0.0006); # = significant difference between trained rats with Bdnf+/+ genotype and control rats with Bdnf+/− genotype (p = 0.0351). Detailed results of performed tests are given in Table 1.
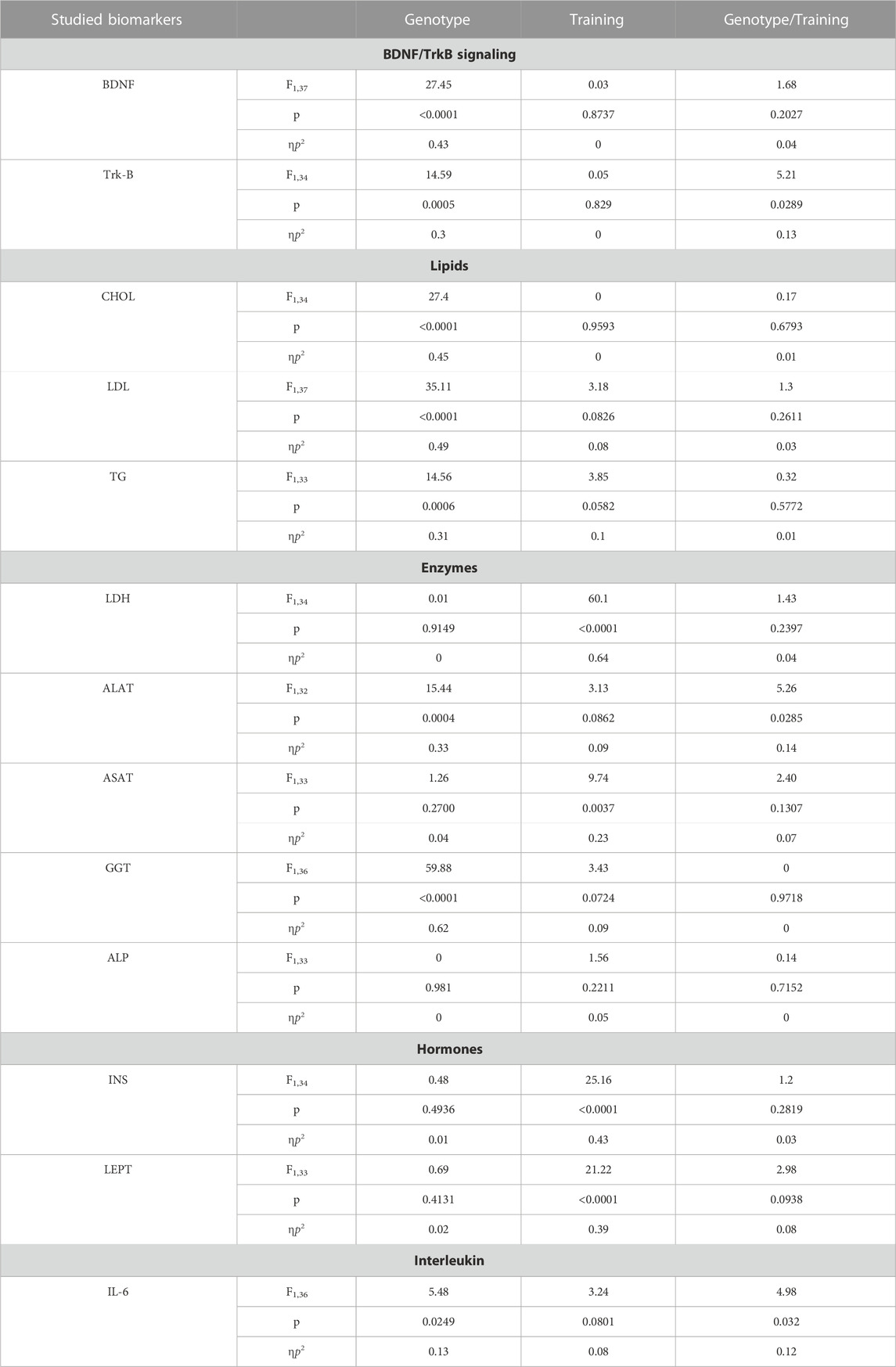
TABLE 1. Results of significance (p), effect size (ηp2) and test power (F) for particular effects and interactions regarding studied biomarkers such as: BDNF and Trk-B (Figures 2A,B), lipids–CHOL, LDL and TG (Figures 3A–C), enzymes—ALAT, ASAT, ALP, GGT, LDH (Figures 4A–E), hormones–INS, LEP (Figures 5A,B) and IL-6 (Figure 6A). Two-way analysis of variance (ANOVA) was performed. Genotype (Bdnf+/+ genotype vs. Bdnf+/− genotype); Training (control group vs. trained group); Genotype/Training (the interaction between genotype and training).
Lipids CHOL and LDL levels were significantly lower, (but significantly higher for TG) in the population of knockout rats (Bdnf+/− and Bdnf+/−T) compared to the population of rats with typical genotype (Bdnf+/+ and Bdnf+/+T) (Figure 3; Table 1). Endurance training did not change the liver contents of CHOL, LDL and TG. Their concentrations were similar in control (Bdnf+/+ and Bdnf+/−) and trained (Bdnf+/+T and Bdnf+/−T) populations (Figure 3; Table 1). However, there was a clear downward trend in TG concentration in trained rats (Bdnf+/+T and Bdnf+/−T).
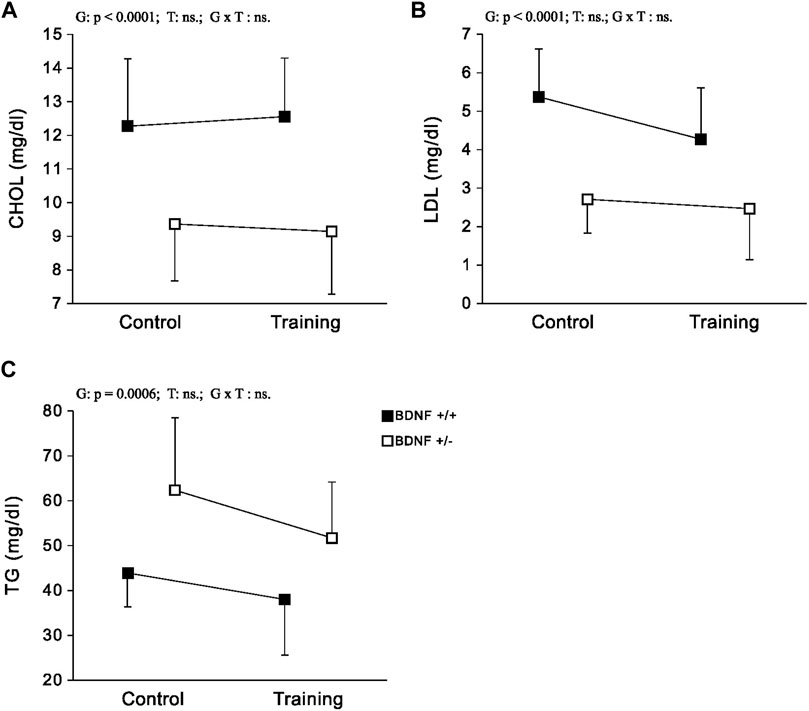
FIGURE 3. The mean values (±SD) of CHOL (A), LDL (B) and TG (C) concentrations in the liver of rats from control (left side) and trained (right side) groups. Control and trained Bdnf+/+ groups are indicated by black square, while control and trained Bdnf+/− groups are indicated by white square. Comparisons between groups were made by two-way ANOVA. (G) genotype (Bdnf+/+ genotype vs. Bdnf+/− genotype); T—training (control vs. training); G x T, the interaction between genotype and training. Significant results are denoted by p, while non-significant results are denoted by ns. Detailed results of performed tests are given in Table 1.
Enzymes Significantly lower activities of ALAT and GGT were observed in the population of knockout rats (Bdnf+/− and Bdnf+/−T) compared to the corresponding population of normal genotype rats (Bdnf+/+ and Bdnf+/+T) (Figures 4A,D; Table 1). However, ALAT activity was lower in the control group of knockout rats compared to the other groups (Figures 4B,C,E; Table 1). In contrast, there was no statistically significant difference in hepatic ASAT, LDH, and ALP activities between normal genotype rats (Bdnf+/+ and Bdnf+/+T) and knockout genotype rats (Bdnf+/− and Bdnf+/−T). There were no differences in hepatic ALP and GGT activities between the control (Bdnf+/+ and Bdnf+/−) and trained (Bdnf+/+T and Bdnf+/−T) populations of rats (Figures 4A,C,D; Table 1). On the other hand, there was increase in both ALAT (not significant) and ASAT activities (Figure 2B; Table 1) as well a statistically significant decrease in LDH activity (Figure 4E; Table 1) in the liver of trained (Bdnf+/+T and Bdnf+/−T) in compare to control rat population (Bdnf+/+ and Bdnf+/−). However, this post-training elevation of ALAT and ASAT activities resulted from an increase of these enzymes activities the only in trained group of rats with BDNF knockout genotype (Figures 4A,B; Table 1).
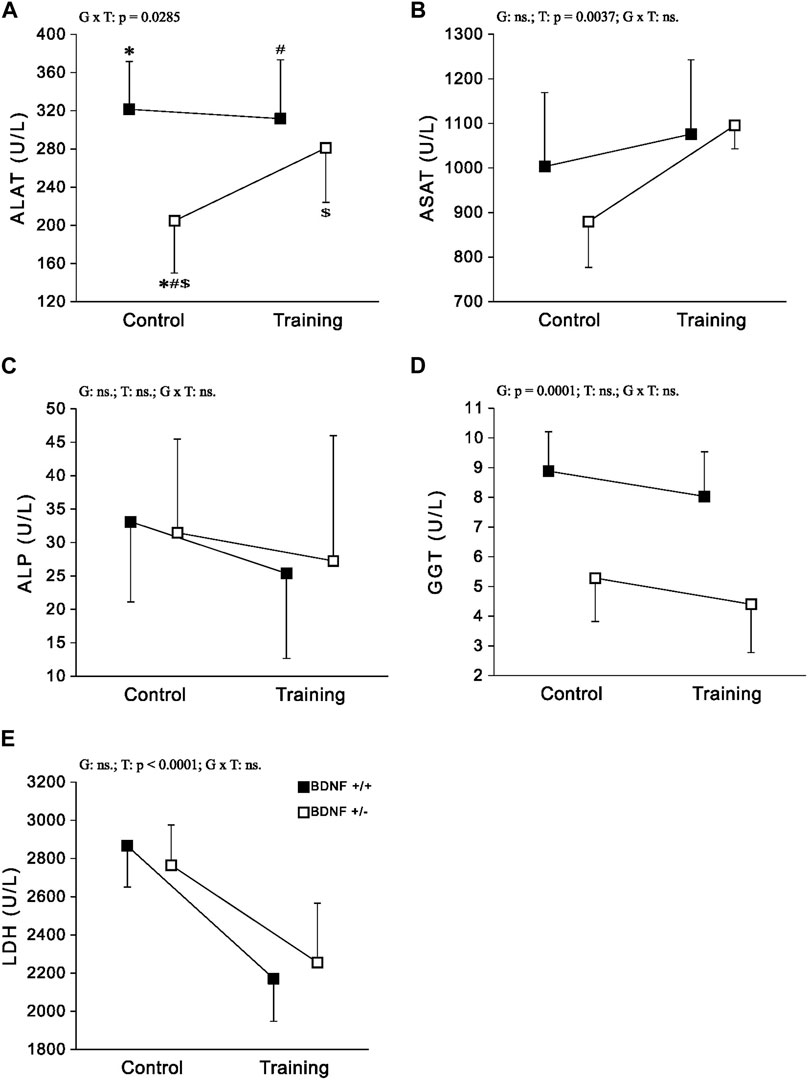
FIGURE 4. The mean values (±SD) of ALAT (A), ASAT (B), ALP (C), GGT (D) and LDH (E) activities in the liver of rats from control (left side) and trained (right side) groups. Control and trained Bdnf+/+ groups are indicated by black square, while control and trained Bdnf+/− groups are indicated by white square. Comparisons between groups were made by two-way ANOVA. (G) genotype (Bdnf+/+ genotype vs. Bdnf+/− genotype); T—training (control vs. training); G x T, the interaction between genotype and training. significant results are denoted by p, while non-significant results are denoted by ns. For significant interactions Tukey’s post hoc test was performed. Post-hoc results: * = significant difference between control rats with Bdnf+/+ genotype and control rats with Bdnf+/− genotype (p = 0.0003); # = significant difference between trained rats with Bdnf+/+ genotype and control rats with Bdnf+/− genotype (p = 0.0014); $ = significant difference between trained rats with Bdnf+/− genotype and control rats with Bdnf+/− (p = 0.0366). Detailed results of performed tests are given in Table 1.
Hormones The concentrations and activities of INS and LEPT in the liver did not differ when the population of typical genotype rats (Bdnf+/+ and Bdnf+/+T) was compared with the corresponding population of knockout genotype rats Bdnf+/− Bdnf+/−T) (Figure 5; Table 1). Liver concentrations of INS and LEPT were significantly higher in the population of trained rats (Bdnf+/+T and Bdnf+/−T) compared to the corresponding population of control rats (Bdnf+/+ and Bdnf+/−) (Figure 5; Table 1).
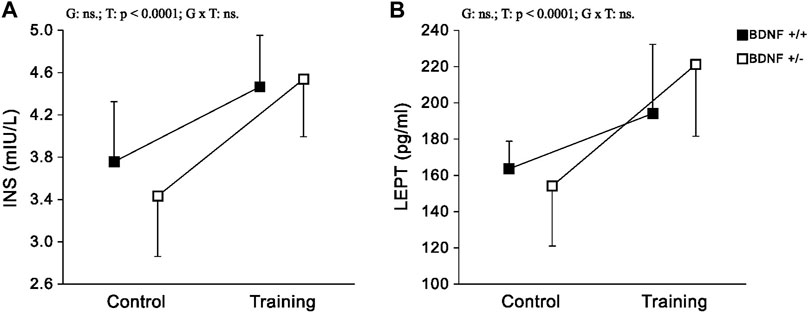
FIGURE 5. The mean values (±SD) of INS (A) and LEPT (B) concentrations in the liver of rats from control (left side) and trained (right side) groups. Control and trained Bdnf+/+ groups are indicated by black square, while control and trained Bdnf+/− groups are indicated by white square. Comparisons between groups were made by two-way ANOVA. (G) genotype (Bdnf+/+ genotype vs. Bdnf+/− genotype); T - training (control vs. training); G x T, the interaction between genotype and training. Significant results are denoted by p, while non-significant results are denoted by ns. Detailed results of performed tests are given in Table 1.
IL-6 The concentration of IL-6 in the liver of rats from the knockout population (Bdnf+/− and Bdnf+/−T) was demonstrated to be statistically higher than that of rats from the normal genotype population (Bdnf+/+ and Bdnf+/+T) (Figure 6; Table 1). However, this difference in IL-6 levels shown between rat populations with different genotypes was caused by a significant increase in IL-6 concentration in the group of trained animals with BDNF gene knockout (see Figure 6 and Table 1) Comparing IL-6 levels in the liver between the control (Bdnf+/+ and Bdnf+/−) and trained (Bdnf+/+T and Bdnf+/−T) populations, no significant difference in IL-6 levels was observed. However, the Bdnf+/−T group showed a significant increase in IL-6 levels compared to the other groups (Figure 6; Table 1).
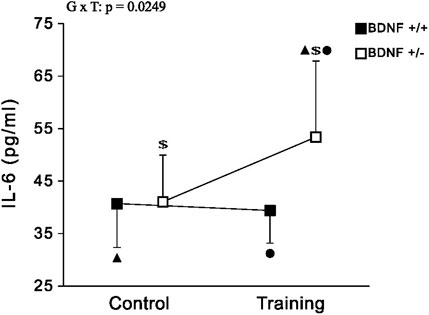
FIGURE 6. The mean values (±SD) of IL-6 (A) concentration in the liver of rats from control (left side) and trained (right side) groups. Control and trained Bdnf+/+ groups are indicated by black square, while control and trained Bdnf+/− groups are indicated by white square. Comparisons between groups were made by two-way ANOVA. G—genotype (Bdnf+/+ genotype vs. Bdnf+/− genotype); T - training (control vs. training); G x T, the interaction between genotype and training. For significant interactions Tukey’s post hoc test was performed. Post-hoc results: ▲ = significant difference between trained rats with Bdnf+/− genotype and control rats with Bdnf+/+ genotype (p = 0.0354); ● = significant difference between trained rats with Bdnf+/− genotype and trained rats with Bdnf+/+ genotype (p = 0.0201); $ = significant difference between trained rats with Bdnf+/− genotype and control rats with Bdnf+/− (p = 0.0422). Detailed results of performed tests are given in Table 1.
Discussion
In the current study, there was an increase in body and liver weight, as well as a decrease in liver BDNF and Trk-B concentrations in BDNF knockout rats (Bdnf+/− and Bdnf+/−T) compared to normal genotype rats (Bdnf+/+ and Bdnf+/+T) (Figures 1A,B; Table 1). Moreover, our previous research (Grzelak et al., 2023) demonstrated that BDNF knockout rats had significantly lower serum BDNF levels than rats with normal genotype. Taken together, these results showed that rats with hemizygous deletion of the BDNF gene exhibited reduced serum BDNF levels and impaired hepatic BDNF/TrkB signaling in the liver. These both findings provide evidence to support the use of these rats as a genetic model to study metabolic syndrome. Moreover, this seems to be consistent with the report by Belviranli and Okudan (2018), which suggests a strong connection between BDNF levels in the liver and circulating blood.
Additionally, our study indicated that reduced BDNF and Trk-B signaling in the liver, resulting from the absence of one BDNF allele, has a particularly important impact on lipids metabolism in hepatocytes. Differences in lipid concentrations, including CHOL, LDL and TG, were evident when comparing the knockout with normal genotype rat populations. In particular, hepatic CHOL and LDL levels were significantly reduced in BDNF knockout rats (Bdnf+/− and Bdnf+/−T) compared to those with normal genotypes (Bdnf+/+ and Bdnf+/+T). CHOL is synthesised directly by hepatocytes in the liver (Zhou and Sun, 2021), while LDL is formed in the liver from particles of very low-density lipoproteins (Feingold, 2022). LDL plays a key role in transporting cholesterol from the liver to peripheral cells, according to previous research (Eckardstein, 2020). Moreover, several studies have demonstrated an inverse relationship between the concentration of CHOL (Abdel-Gayoum and Ahmida, 2017) and LDL (Ogunmoyole et al., 2019) in the liver and their corresponding levels in serum. Thus, our results suggest that CHOL accumulation and storage in the liver may be impaired in rats with hemizygous BDNF deletion. This also indicates that knockout rats may be subject to CHOL accumulation in the arteries, which may lead to the formation of atherosclerotic plaques—a major contributor to atherosclerosis (Subczynski and Pasenkiewicz-Gierula, 2020). It is therefore reasonable that knockout rats may have an increased risk of developing cardiovascular. Such a suggestion is in line with previous human studies that have linked reduced serum or plasma BDNF levels to a higher incidence of various cardiovascular diseases (Chaldakov et al., 2004; Sustar et al., 2019).
We also found that liver TGs levels were significantly higher in knockout rats compared to normal genotype rats. TGs are obtained from dietary sources as well as synthesised in the liver as part of the process of lipid metabolism, where they are stored in hepatocytes (Alves-Bezerra and Cohen, 2017). Elevated levels of TGs in the liver caused by increase food intake, as observed in knockout rats, can lead to increase liver weight and eventually induce fatty liver (Garnol et al., 2016). This may explain the considerable increase in liver weight observed in knockout rats. Furthermore, it can be supposed that elevated hepatic TGs levels may be responsible for increased body weight in these rats, as excess TGs are stored in adipose tissue, leading to obesity (Ahmadian et al., 2009). Based on all the aforementioned observations, it can be strongly inferred that the significant reduction in BDNF/Trk-B signaling in the liver, observed in genetically modified rats, plays a key role in inducing substantial changes in hepatic lipid metabolism. This finding is also supported by the study conducted by Tsuchida et al. (2002), which demonstrated that subcutaneous administration of BDNF could lead to significant reductions in liver weight, TG concentrations, and the development of fatty liver in obese diabetic mice. Therefore, it can be concluded that a decrease in BDNF/Trk-B signaling in the liver is a crucial factor that contributes to the onset of metabolic syndrome symptoms.
The moderate-intensity endurance training applied in our research did not alter either the BDNF and Trk-B content (Figure 1 A and B; Table 1) or the CHOL and LDL concentrations in the liver of the studied rats. Long-term aerobic exercise, particularly at high intensity, is recommended as an effective therapy for improving lipid profiles (Mann et al., 2014) or to counteract metabolic syndrome (Mrówczyński, 2019). However, 5-weeks endurance training of graduate increasing intensity, utilized in the present study, did not result in elevated levels of BDNF and Trk-B in the liver, suggesting that it may have been insufficient to induce significant improvement in the lipid profile. It is important to emphasise that our previous research has shown that training at such intensity can lead to an increase in both BDNF and Trk-B levels in some fast hindlimb muscles (Grzelak et al., 2023). However, there is no a significant effect on circulating levels of BDNF what seems to be in line with results of Gaitan et al. (2021) who found no increase of BDNF level in the blood of adult humans even after 26 weeks of aerobic exercise. However, it cannot be ruled out that the implementation of high-intensity or prolonged endurance activity may evoke increase in BDNF levels in both the serum and the liver which could improve of the lipid profile. It has been reported that high-intensity interval training has a particularly desirable effect on lipid metabolism by reducing their concentrations in the blood, including levels of CHOL, TGs and others (Cao et al., 2022).
Knockout rats (Bdnf+/− and Bdnf+/−T) showed a decrease in liver metabolic enzyme activities, specifically ALAT and GGT compared to rats with a normal genotype (Bdnf+/+ and Bdnf+/+T). ALAT is a specific liver enzyme crucial for amino acid metabolism (Glinghammar et al., 2009) while GGT is a key enzyme in the metabolism of glutathione (Hanigan et al., 2015). Higher activities of these enzymes in the liver indicate more efficient enzyme function, as their levels in the liver are inversely proportional to those in the blood. However, liver disorders can cause the release of these enzymes from hepatocyte cytoplasm into the blood, where they serve as indicators of impaired metabolic function in liver disorders (Vermeulen et al., 1992; Deoras et al., 1997; Ogunmoyole et al., 2019). ALAT and GGT levels are routinely measured in serum or plasma to investigate liver disease (Green and Flamm, 2002; Carobene et al., 2013) due to these both markers are particularly relevant in the context of pathogenesis of various liver diseases related to fat accumulation in liver such as obesity, metabolic syndrome and the cardiovascular disease (Sookoian and Pirola, 2015). For example, elevated levels of these markers have been observed in children as a consequence of liver damage associated with overweight and obesity (Johansen et al., 2020). Therefore, it is reasonable to speculate that knockout rats would exhibit increased activities of ALAT and GGT in circulation, indicating disruptions in metabolic processes within the liver.
Our results demonstrated that endurance training led to an increase in hepatic ALAT activity and a significant elevation in ASAT activity in knockout rats (Bdnf+/− and Bdnf+/−T). However, this effect was mainly observed in the group of trained knockout rats (Figures 5B,C,E; Table 1). It is well-established that endurance training can improve liver function and reduce liver enzyme levels in the bloodstream, such as ALAT and ASAT, particularly in individuals with obesity or metabolic syndrome (Haukeland et al., 2008; Skrypnik et al., 2016). Therefore, our findings demonstrate an increase in ALAT and ASAT levels in the liver of knockout rats, confirmed previous observations. The implemented training regimen led to a significant reduction in hepatic LDH activity in the trained rat population (Bdnf+/+T and Bdnf+/−T), an enzyme crucial for cellular metabolism, particularly anaerobic glycolysis (Farhana and Lappin, 2023). Studies by Lupa et al. (1994), have previously demonstrated a decrease in hepatic LDH activity in response to endurance training in young rats. This finding suggests that endurance training may have a positive impact on liver health and metabolic function, potentially reducing the risk of liver-related disorders. Importantly, our results indicate that the decrease in hepatic LDH activity observed after endurance training is independent of the BDNF genotype.
No significant differences were observed in INS and LEPT hormone concentrations in the livers of rats with knockout (Bdnf+/− and Bdnf+/−T) and normal (Bdnf+/+ and Bdnf+/+T) genotypes. These findings indicate that liver functions, including the regulation of glucose metabolism (Chadt and Al-Hasani, 2020) and maintenance of energy balance (Martínez-Uña et al., 2020) remain unchanged in knockout rats. This can be attributed to the fact that this liver function is closely related to hormones produced by other organs and transported to the liver via the circulation. However, it is worth noting that liver INS and LEPT levels were significantly higher after endurance training in our study. This observation is therefore consistent with previous reports emphasising the importance of regular exercise in regulating body weight and blood sugar levels in humans (Karacabey, 2009). Interestingly, our results indicate that these training effects are not dependent on BDNF genotypes, as observed for LDH activity.
It is important to note that a significant reduction in BDNF and Trk-B levels in liver of knockout rats (Bdnf+/− and Bdnf+/−T) seems to be also associated with a higher concentration of hepatic IL-6 compared to rats with the normal genotype (Bdnf+/+ and Bdnf+/+T). However, such difference is caused by significant increase in IL-6 concentration in the group of trained animals with BDNF gene deficiency (see Figure 2 and Table 1). Cressman et al. (1996) discovered that even a single dose of IL-6 can restore gene expression in mice lacking IL-6 gene, resulting in hepatocyte proliferation and inhibition of liver damage. Garnol et al. (2016) observed heightened levels of IL-6 in the liver after partial hepatectomy, considering it as an essential factor for initiating liver regeneration. Therefore, elevated levels of IL-6 play a crucial role in promoting hepatocyte proliferation and are commonly regarded as a key factor in driving liver regeneration. Accordingly, our findings have demonstrated a significant increase in hepatic IL-6 levels, particularly in the trained knockout rat group (Figure 2; Table 1). This suggests that endurance training has the potential to enhance hepatocyte proliferation and initiate liver regeneration processes, especially in animals with reduced levels of BDNF and Trk-B in the liver. Additionally, it is well-established that muscle cells are a major source of IL-6 production in response to exercise. Steinbacher and Eckl (2015) proposed that IL-6 produced by muscles during exercise exerts an anti-inflammatory effect, leading to a reduction in pro-inflammatory cytokine levels. Moreover, it has been observed that muscles release a substantial amount of IL-6 into the bloodstream even after a single exercise session (Fischer, 2006), and this IL-6 can be captured by the liver (Febbraio et al., 2003). Therefore, our results suggest that the production of IL-6 during training plays a crucial role in promoting liver regeneration, particularly in animals with metabolic liver disorders like knockout rats. Furthermore, the elevated IL-6 levels in BDNF-deficient rats may be a potential factor contributing to the increase in ALAT and ASAT activity. This suggests that IL-6 might play a regenerative role by enhancing the efficiency of some metabolic pathways.
Conclusion
The study revealed that BDNF knockout rats loss exhibited a significant reduction in BDNF/Trk-B content within the liver. This reduction was accompanied by lower levels of lipids, such as CHOL and LDL, and a simultaneous increase in TGs levels. In addition, there was a reduction in the activity of two liver enzymes—ALAT and GGT. These alterations in lipid metabolism and enzyme activity contributed to increase in obesity, as evidenced by elevated liver weight and body weight in the rats. Our findings suggest that a reduction in BDNF/Trk-B signaling contributes to changes in lipid metabolism that lead to hepatic steatosis and obesity, which are symptoms of the metabolic syndrome.
Moderate endurance training induced an increase in hepatic ALAT and ASAT activity in knockout rats, suggesting improved metabolic pathway efficiency in the liver. Furthermore, a significant increase in hepatic IL-6 levels was demonstrated in trained animals with the BDNF knockout genotype, suggesting that the initiation of liver regeneration is mediated by IL-6 produced by contracting skeletal muscle. It cannot be excluded that it is responsible for improving the efficiency of metabolic pathways by increasing ALAT and ASAT activity. On the other hand, the effect of endurance exercise on LEPT and INS concentrations, as well as LDH activity, was not dependent on a specific BDNF genotype.
Future studies should focus on investigating the effects of the BDNF/Trk-B axis on the pathways of the above-mentioned enzymes, which play a key role in liver metabolic functions. Furthermore, upcoming studies should aim to provide a more complex analysis of the role of IL-6 in liver regeneration.
Limitations
Measurement of the biomarkers tested in blood was not possible due to the fact that blood samples were collected to assess neurotrophin levels, as shown in our previous work performed on the same group of animals (Grzelak et al., 2023). The volume of blood samples collected was also limited due to the in vivo electrophysiology experiment being conducted in parallel. In addition, we did not conducted immunochemistry research in liver.
Data availability statement
The original contributions presented in the study are included in the article/Supplementary Material, further inquiries can be directed to the corresponding author.
Ethics statement
The animal study was approved by the Local Ethics Committee for Animal Research in Poznań. The study was conducted in accordance with the local legislation and institutional requirements.
Author contributions
NG: Data curation, Formal Analysis, Investigation, Methodology, Software, Writing–original draft, Writing–review and editing. DK: Formal Analysis, Investigation, Methodology, Writing–original draft, Writing–review and editing. WM: Conceptualization, Formal Analysis, Funding acquisition, Investigation, Methodology, Project administration, Supervision, Writing–original draft, Writing–review and editing.
Funding
The author(s) declare financial support was received for the research, authorship, and/or publication of this article. This study was funded by the Polish National Science Center Grant No. 2017/27/B/NZ7/01113.
Acknowledgments
The authors thank Dr. Tomasz Podgórski and Dr. Joanna Kamińska for technical support and meaningful discussion.
Conflict of interest
The authors declare that the research was conducted in the absence of any commercial or financial relationships that could be construed as a potential conflict of interest.
Publisher’s note
All claims expressed in this article are solely those of the authors and do not necessarily represent those of their affiliated organizations, or those of the publisher, the editors and the reviewers. Any product that may be evaluated in this article, or claim that may be made by its manufacturer, is not guaranteed or endorsed by the publisher.
References
Abdel-Gayoum A. A., Ahmida M. H. S. (2017). Changes in the serum, liver, and renal cortical lipids and electrolytesin rabbits with cisplatin-induced nephrotoxicity. Turk J. Med. Sci. 47, 1019–1027. doi:10.3906/sag-1602-136
Ahmadian M., Duncan R. E., Sul H. S. (2009). The skinny on fat: lipolysis and fatty acid utilization in adipocytes. Trends Endocrinol. Metabolism 20, 424–428. doi:10.1016/j.tem.2009.06.002
Alves-Bezerra M., Cohen D. E. (2017). Triglyceride metabolism in the liver. Compr. Physiol. 8, 1–8. doi:10.1002/cphy.c170012
Aravamudan B., Thompson M. A., Pabelick C. M., Prakash Y. S. (2016). Mechanisms of BDNF regulation in asthmatic airway smooth muscle. Am. J. Physiol. Lung Cell Mol. Physiol. 311, L270–L279. doi:10.1152/ajplung.00414.2015
Arazi H., Babaei P., Moghimi M., Asadi A. (2021). Acute effects of strength and endurance exercise on serum BDNF and IGF-1 levels in older men. BMC Geriatr. 21, 50. doi:10.1186/s12877-020-01937-6
Belviranlı M., Okudan N. (2018). Exercise training increases cardiac, hepatic and circulating levels of brain-derived neurotrophic factor and irisin in young and aged rats. Horm. Mol. Biol. Clin. Investig. 36. doi:10.1515/hmbci-2018-0053
Canbay A., Bechmann L., Gerken G. (2007). Lipid metabolism in the liver. Z Gastroenterol. 45, 35–41. doi:10.1055/s-2006-927368
Cao M., Li S., Tang Y., Zou Y. (2022). A meta-analysis of high-intensity interval training on glycolipid metabolism in children with metabolic disorders. Front. Pediatr. 10, 887852. doi:10.3389/fped.2022.887852
Carobene A., Braga F., Roraas T., Sandberg S., Bartlett W. A. (2013). A systematic review of data on biological variation for alanine aminotransferase, aspartate aminotransferase and γ-glutamyl transferase. Clin. Chem. Lab. Med. 51, 1997–2007. doi:10.1515/cclm-2013-0096
Cassiman D., Denef C., Desmet V. J., Roskams T. (2001). Human and rat hepatic stellate cells express neurotrophins and neurotrophin receptors. Hepatology 33, 148–158. doi:10.1053/jhep.2001.20793
Chadt A., Al-Hasani H. (2020). Glucose transporters in adipose tissue, liver, and skeletal muscle in metabolic health and disease. Pflugers Arch. 472, 1273–1298. doi:10.1007/s00424-020-02417-x
Chaldakov G. N., Fiore M., Stankulov I. S., Manni L., Hristova M. G., Antonelli A., et al. (2004). Neurotrophin presence in human coronary atherosclerosis and metabolic syndrome: a role for NGF and BDNF in cardiovascular disease? Prog. Brain Res. 146, 279–289. doi:10.1016/S0079-6123(03)46018-4
Cressman D. E., Greenbaum L. E., DeAngelis R. A., Ciliberto G., Furth E. E., Poli V., et al. (1996). Liver failure and defective hepatocyte regeneration in interleukin-6-deficient mice. Science 274, 1379–1383. doi:10.1126/science.274.5291.1379
Deoras D. P., Young-Curtis P., Dalvi R. R., Tippett F. E. (1997). Effect of gossypol on hepatic and serum gamma-glutamyltransferase activity in rats. Vet. Res. Commun. 21, 317–323. doi:10.1023/a:1005856119553
Dinoff A., Herrmann N., Swardfager W., Liu C. S., Sherman C., Chan S., et al. (2016). The effect of exercise training on resting concentrations of peripheral brain-derived neurotrophic factor (BDNF): a meta-analysis. PLoS One 11, e0163037. doi:10.1371/journal.pone.0163037
Eckardstein A. von (2020). CME-laboratory 61: new European consensus recommendations on dyslipidemia. Prax. (Bern 1994) 109, 215–220. doi:10.1024/1661-8157/a003437
Farhana A., Lappin S. L. (2023). “Biochemistry, lactate dehydrogenase,” in StatPearls (Treasure Island (FL): StatPearls Publishing). Available at: http://www.ncbi.nlm.nih.gov/books/NBK557536/ (Accessed May 30, 2023).
Febbraio M. A., Ott P., Nielsen H. B., Steensberg A., Keller C., Krustrup P., et al. (2003). Hepatosplanchnic clearance of interleukin-6 in humans during exercise. Am. J. Physiol. Endocrinol. Metab. 285, E397–E402. doi:10.1152/ajpendo.00134.2003
Feingold K. R. (2022). Lipid and lipoprotein metabolism. Endocrinol. Metab. Clin. North Am. 51, 437–458. doi:10.1016/j.ecl.2022.02.008
Fischer C. P. (2006). Interleukin-6 in acute exercise and training: what is the biological relevance? Exerc Immunol. Rev. 12, 6–33.
Fulgenzi G., Hong Z., Tomassoni-Ardori F., Barella L. F., Becker J., Barrick C., et al. (2020). Novel metabolic role for BDNF in pancreatic β-cell insulin secretion. Nat. Commun. 11, 1950. doi:10.1038/s41467-020-15833-5
Gaitán J. M., Moon H. Y., Stremlau M., Dubal D. B., Cook D. B., Okonkwo O. C., et al. (2021). Effects of aerobic exercise training on systemic biomarkers and cognition in late middle-aged adults at risk for alzheimer’s disease. Front. Endocrinol. 12, 660181. doi:10.3389/fendo.2021.660181
García-Suárez O., González-Martínez T., Perez-Perez M., Germana A., Blanco-Gélaz M. A., Monjil D. F., et al. (2006). Expression of the neurotrophin receptor TrkB in the mouse liver. Anat. Embryol. Berl. 211, 465–473. doi:10.1007/s00429-006-0098-9
Garnol T., Kučera O., Staňková P., Lotková H., Červinková Z. (2016). Does simple steatosis affect liver regeneration after partial hepatectomy in rats? Acta Medica (Hradec Kralove) 59, 35–42. doi:10.14712/18059694.2016.87
Genzer Y., Chapnik N., Froy O. (2017). Effect of brain-derived neurotrophic factor (BDNF) on hepatocyte metabolism. Int. J. Biochem. Cell Biol. 88, 69–74. doi:10.1016/j.biocel.2017.05.008
Girard M., Carrier P., Loustaud-Ratti V., Nubukpo P. (2021). BDNF levels and liver stiffness in subjects with alcohol use disorder: evaluation after alcohol withdrawal. Am. J. Drug Alcohol Abuse 47, 191–198. doi:10.1080/00952990.2020.1833211
Glinghammar B., Rafter I., Lindström A.-K., Hedberg J. J., Andersson H. B., Lindblom P., et al. (2009). Detection of the mitochondrial and catalytically active alanine aminotransferase in human tissues and plasma. Int. J. Mol. Med. 23, 621–631. doi:10.3892/ijmm_00000173
Grant D. M. (1991). Detoxification pathways in the liver. J Inher Metab Disea 14, 421–430. doi:10.1007/BF01797915
Green R. M., Flamm S. (2002). AGA technical review on the evaluation of liver chemistry tests. Gastroenterology 123, 1367–1384. doi:10.1053/gast.2002.36061
Grzelak N., Krutki P., Bączyk M., Kaczmarek D., Mrówczyński W. (2023). Influence of altered serum and muscle concentrations of BDNF on electrophysiological properties of spinal motoneurons in wild-type and BDNF-knockout rats. Sci. Rep. 13, 4571. doi:10.1038/s41598-023-31703-8
Guo W., Ji Y., Wang S., Sun Y., Lu B. (2014). Neuronal activity alters BDNF-TrkB signaling kinetics and downstream functions. J. Cell Sci. 127, 2249–2260. doi:10.1242/jcs.139964
Han H.-S., Kang G., Kim J. S., Choi B. H., Koo S.-H. (2016). Regulation of glucose metabolism from a liver-centric perspective. Exp. Mol. Med. 48, e218. doi:10.1038/emm.2015.122
Hang P.-Z., Zhu H., Li P.-F., Liu J., Ge F.-Q., Zhao J., et al. (2021). The emerging role of BDNF/TrkB signaling in cardiovascular diseases. Life 11, 70. doi:10.3390/life11010070
Hanigan M. H., Gillies E. M., Wickham S., Wakeham N., Wirsig-Wiechmann C. R. (2015). Immunolabeling of gamma-glutamyl transferase 5 in normal human tissues reveals that expression and localization differ from gamma-glutamyl transferase 1. Histochem Cell Biol. 143, 505–515. doi:10.1007/s00418-014-1295-x
Harris A. P., Lennen R. J., Brydges N. M., Jansen M. A., Pernet C. R., Whalley H. C., et al. (2016). The role of brain-derived neurotrophic factor in learned fear processing: an awake rat fMRI study. Genes Brain Behav. 15, 221–230. doi:10.1111/gbb.12277
Haukeland J. W., Schreiner L. T., Lorgen I., Frigstad S.-O., Bang C., Raknerud N., et al. (2008). ASAT/ALAT ratio provides prognostic information independently of Child-Pugh class, gender and age in non-alcoholic cirrhosis. Scand. J. Gastroenterology 43, 1241–1248. doi:10.1080/00365520802158614
Jiménez-Maldonado A., de Álvarez-Buylla E. R., Montero S., Melnikov V., Castro-Rodríguez E., Gamboa-Domínguez A., et al. (2014). Chronic exercise increases plasma brain-derived neurotrophic factor levels, pancreatic islet size, and insulin tolerance in a TrkB-dependent manner. PLoS One 9, e115177. doi:10.1371/journal.pone.0115177
Jin W. (2020). Regulation of BDNF-TrkB signaling and potential therapeutic strategies for Parkinson’s disease. J. Clin. Med. 9, 257. doi:10.3390/jcm9010257
Johansen M. J., Gade J., Stender S., Frithioff-Bøjsøe C., Lund M. A. V., Chabanova E., et al. (2020). The effect of overweight and obesity on liver biochemical markers in children and adolescents. J. Clin. Endocrinol. Metab. 105, dgz010. doi:10.1210/clinem/dgz010
Karacabey K. (2009). The effect of exercise on leptin, insulin, cortisol and lipid profiles in obese children. J. Int. Med. Res. 37, 1472–1478. doi:10.1177/147323000903700523
Kernie S. G., Liebl D. J., Parada L. F. (2000). BDNF regulates eating behavior and locomotor activity in mice. EMBO J. 19, 1290–1300. doi:10.1093/emboj/19.6.1290
Li Y., Li F., Qin D., Chen H., Wang J., Wang J., et al. (2022). The role of brain derived neurotrophic factor in central nervous system. Front. Aging Neurosci. 14, 986443. doi:10.3389/fnagi.2022.986443
Lupa V. A., Podolin D. A., Roth D. A., Mazzeo R. S. (1994). Influence of aging and endurance training on lactate dehydrogenase in liver and skeletal muscle. Mech. Ageing Dev. 75, 191–204. doi:10.1016/0047-6374(94)90009-4
Lyons W. E., Mamounas L. A., Ricaurte G. A., Coppola V., Reid S. W., Bora S. H., et al. (1999). Brain-derived neurotrophic factor-deficient mice develop aggressiveness and hyperphagia in conjunction with brain serotonergic abnormalities. Proc. Natl. Acad. Sci. U. S. A. 96, 15239–15244. doi:10.1073/pnas.96.26.15239
Mann S., Beedie C., Jimenez A. (2014). Differential effects of aerobic exercise, resistance training and combined exercise modalities on cholesterol and the lipid profile: review, synthesis and recommendations. Sports Med. 44, 211–221. doi:10.1007/s40279-013-0110-5
Martínez-Uña M., López-Mancheño Y., Diéguez C., Fernández-Rojo M. A., Novelle M. G. (2020). Unraveling the role of leptin in liver function and its relationship with liver diseases. IJMS 21, 9368. doi:10.3390/ijms21249368
Motamedi S., Karimi I., Jafari F. (2017). The interrelationship of metabolic syndrome and neurodegenerative diseases with focus on brain-derived neurotrophic factor (BDNF): kill two birds with one stone. Metab. Brain Dis. 32, 651–665. doi:10.1007/s11011-017-9997-0
Mousavi K., Jasmin B. J. (2006). BDNF is expressed in skeletal muscle satellite cells and inhibits myogenic differentiation. J. Neurosci. 26, 5739–5749. doi:10.1523/JNEUROSCI.5398-05.2006
Mrówczyński W. (2019). Health benefits of endurance training: implications of the brain-derived neurotrophic factor-A systematic review. Neural Plast. 2019, 5413067. doi:10.1155/2019/5413067
Nakagomi A., Okada S., Yokoyama M., Yoshida Y., Shimizu I., Miki T., et al. (2015). Role of the central nervous system and adipose tissue BDNF/TrkB axes in metabolic regulation. NPJ Aging Mech. Dis. 1, 15009. doi:10.1038/npjamd.2015.9
Ogunmoyole T., Oladele F. C., Aderibigbe A., Johnson O. D. (2019). Hepatotoxicity of Telfaria occidentalis root extracts on wistar albino rat. Heliyon 5, e01617. doi:10.1016/j.heliyon.2019.e01617
Pius-Sadowska E., Machaliński B. (2017). BDNF - a key player in cardiovascular system. J. Mol. Cell Cardiol. 110, 54–60. doi:10.1016/j.yjmcc.2017.07.007
Podyma B., Parekh K., Güler A. D., Deppmann C. D. (2021). Metabolic homeostasis via BDNF and its receptors. Trends Endocrinol. Metab. 32, 488–499. doi:10.1016/j.tem.2021.04.005
Rasmussen P., Brassard P., Adser H., Pedersen M. V., Leick L., Hart E., et al. (2009). Evidence for a release of brain-derived neurotrophic factor from the brain during exercise. Exp. Physiol. 94, 1062–1069. doi:10.1113/expphysiol.2009.048512
Ribeiro D., Petrigna L., Pereira F. C., Muscella A., Bianco A., Tavares P. (2021). The impact of physical exercise on the circulating levels of BDNF and nt 4/5: a review. IJMS 22, 8814. doi:10.3390/ijms22168814
Schmidt-Arras D., Rose-John S. (2016). IL-6 pathway in the liver: from physiopathology to therapy. J. Hepatol. 64, 1403–1415. doi:10.1016/j.jhep.2016.02.004
Shu H.-C., Hu J., Jiang X.-B., Deng H.-Q., Zhang K.-H. (2019). BDNF gene polymorphism and serum level correlate with liver function in patients with hepatitis B-induced cirrhosis. Int. J. Clin. Exp. Pathol. 12, 2368–2380.
Skrypnik D., Ratajczak M., Karolkiewicz J., Mądry E., Pupek-Musialik D., Hansdorfer-Korzon R., et al. (2016). Effects of endurance and endurance-strength exercise on biochemical parameters of liver function in women with abdominal obesity. Biomed. Pharmacother. 80, 1–7. doi:10.1016/j.biopha.2016.02.017
Sookoian S., Pirola C. J. (2015). Liver enzymes, metabolomics and genome-wide association studies: from systems biology to the personalized medicine. WJG 21, 711–725. doi:10.3748/wjg.v21.i3.711
Steinbacher P., Eckl P. (2015). Impact of oxidative stress on exercising skeletal muscle. Biomolecules 5, 356–377. doi:10.3390/biom5020356
Subczynski W. K., Pasenkiewicz-Gierula M. (2020). Hypothetical pathway for formation of cholesterol microcrystals initiating the atherosclerotic process. Cell Biochem. Biophys. 78, 241–247. doi:10.1007/s12013-020-00925-2
Sustar A., Perkovic M. N., Erjavec G. N., Strac D. S., Pivac N. (2019). Association between reduced brain-derived neurotrophic factor concentration and coronary heart disease. Indian J. Med. Res. 150, 43–49. doi:10.4103/ijmr.IJMR_1566_17
Szuhany K. L., Bugatti M., Otto M. W. (2015). A meta-analytic review of the effects of exercise on brain-derived neurotrophic factor. J. Psychiatr. Res. 60, 56–64. doi:10.1016/j.jpsychires.2014.10.003
Tang S. W., Chu E., Hui T., Helmeste D., Law C. (2008). Influence of exercise on serum brain-derived neurotrophic factor concentrations in healthy human subjects. Neurosci. Lett. 431, 62–65. doi:10.1016/j.neulet.2007.11.019
Tsuchida A., Nonomura T., Nakagawa T., Itakura Y., Ono-Kishino M., Yamanaka M., et al. (2002). Brain-derived neurotrophic factor ameliorates lipid metabolism in diabetic mice. Diabetes Obes. Metab. 4, 262–269. doi:10.1046/j.1463-1326.2002.00206.x
Vermeulen N. P. E., Bessems J. G. M., Van De Straat R. (1992). Molecular aspects of paracetamol-induced hepatotoxicity and its mechanism-based prevention. Drug Metab. Rev. 24, 367–407. doi:10.3109/03602539208996298
Vivacqua G., Renzi A., Carpino G., Franchitto A., Gaudio E. (2014). Expression of brain derivated neurotrophic factor and of its receptors: TrKB and p75NT in normal and bile duct ligated rat liver. Ital. J. Anat. Embryol. 119, 111–129. doi:10.13128/IJAE-15138
Yoshii A., Constantine-Paton M. (2010). Postsynaptic BDNF-TrkB signaling in synapse maturation, plasticity, and disease. Dev. Neurobiol. 70, 304–322. doi:10.1002/dneu.20765
Keywords: BDNF, knockout genotype, endurance training, liver, rats
Citation: Grzelak N, Kaczmarek D and Mrówczyński W (2023) Comparison of the effects of BDNF/TRKB signalling on metabolic biomarkers in the liver of sedentary and trained rats with normal and knockout BDNF genotypes. Front. Physiol. 14:1268648. doi: 10.3389/fphys.2023.1268648
Received: 28 July 2023; Accepted: 04 December 2023;
Published: 13 December 2023.
Edited by:
Ganesh Kolumam, Calico Life Sciences LLC, United StatesReviewed by:
Siresha Bathina, Baylor College of Medicine, United StatesMuaz Belviranli, Selçuk University, Türkiye
Hyo Youl Moon, Seoul National University, Republic of Korea
Copyright © 2023 Grzelak, Kaczmarek and Mrówczyński. This is an open-access article distributed under the terms of the Creative Commons Attribution License (CC BY). The use, distribution or reproduction in other forums is permitted, provided the original author(s) and the copyright owner(s) are credited and that the original publication in this journal is cited, in accordance with accepted academic practice. No use, distribution or reproduction is permitted which does not comply with these terms.
*Correspondence: Włodzimierz Mrówczyński, bXJvd2N6eW5za2lAYXdmLnBvem5hbi5wbA==