- Department of Pediatric Dentistry, Nanjing Stomatological Hospital, Affiliated Hospital of Medical School, Nanjing University, Nanjing, China
Dental pulp stem cells (DPSCs) are a type of mesenchymal stem cells that can differentiate into odontoblast-like cells and protect the pulp. The differentiation of DPSCs can be influenced by biomaterials or growth factors that activate different signaling pathways in vitro or in vivo. In this review, we summarized six major pathways involved in the odontogenic differentiation of DPSCs, Wnt signaling pathways, Smad signaling pathways, MAPK signaling pathways, NF-kB signaling pathways, PI3K/AKT/mTOR signaling pathways, and Notch signaling pathways. Various factors can influence the odontogenic differentiation of DPSCs through one or more signaling pathways. By understanding the interactions between these signaling pathways, we can expand our knowledge of the mechanisms underlying the regeneration of the pulp–dentin complex.
1 Introduction
Dental pulp stem cells (DPSCs) are a type of mesenchymal stem cells (MSCs) that express mesenchymal markers, including CD29, CD44, CD59, CD90, CD105, CD146, and CD166 (Liu et al., 2015). Since their discovery and characterization by Gronthos et al. (2000), DPSCs have attracted great interest in the field of regenerative medicine. This is due to their ability to self-renew, their high proliferation rate, their immunomodulatory properties, and their versatile potential to differentiate into different cell types. Furthermore, DPSCs can be easily obtained from extracted third molars or primary teeth. Dental tissues contain various types of MSC sources, namely, pulp, follicle, and papilla, which can differentiate into the desirable lineages when induced under appropriate conditions (Gronthos et al., 2000). DPSCs are used in the treatment of Alzheimer’s disease, diabetes, and immunological diseases and have shown the ability to differentiate into angioblasts and neural cells (Marchionni et al., 2009; Jahanbin et al., 2016; Nuti et al., 2016), smooth muscle cells (Song et al., 2016; Jiang et al., 2019; Ha et al., 2021), chondroblasts (Ba et al., 2017), or hepatocytes (Lei et al., 2021; Li et al., 2021). Most importantly, DPSCs have the unique capability to replace damaged odontoblasts and generate reparative dentin. When odontoblasts are stimulated by dental caries or trauma, DPSCs migrate to the lesion site and differentiate into odontoblast-like cells that contribute to the formation of dentin and pulp-like structures (Arana-Chavez and Massa, 2004; Janebodin et al., 2011; Ma et al., 2012). Therefore, DPSCs play a crucial role in the regeneration of the pulp–dentin complex (Xuan et al., 2018). Pulp disease is a common cause of tooth loss in young individuals (Hull et al., 1997). Although root canal treatment has been proven effective in the treatment of pulp diseases (Oviir, 2005), it does have some limitations, such as tooth discoloration or thick dentinal walls (Jeeruphan et al., 2012). To solve this problem, it is important to focus on regenerating the pulp–dentin complex. However, the odontogenic differentiation of DPSCs is extremely complex and involves multiple signaling pathways. Currently, a large number of studies have shown that activation of associated signaling pathways can promote the odontogenic differentiation of DPSCs. However, there is little comprehensive literature summarizing the signaling pathways related to the odontogenic differentiation of DPSCs (Liang et al., 2021). To provide insight into the better utilization of DPSCs as “seed cells” for pulp–dentin complex regeneration, this article aims to summarize the known signaling pathways associated with the odontogenic differentiation of DPSCs.
2 Results
2.1 Wnt signaling pathways
Wnt signaling pathways play a critical role in promoting tissue repair and regeneration. These pathways can be divided into two types: canonical and non-canonical pathways (Rao and Kuhl, 2010). In canonical pathways (Figure 1), the absence of Wnts leads to the formation of a protein complex consisting of adenomatous polyposis coli (APC), glycogen synthase kinase-3β (GSK-3β), Axin, and casein kinase 1 (Ck1). This complex then phosphorylates β-catenin and leads to its degradation. This inhibits signal transduction in the Wnt signaling pathway. When Wnts are present, they interact with the frizzled receptor and the low-density lipoprotein receptor-related proteins 5 and 6 (LRP5/6). This interaction leads to the destruction of the protein complex. This allows cytoplasmic β-catenin to accumulate and translocate into the nucleus. Once in the nucleus, β-catenin activates target genes and proteins that are involved in odontogenic differentiation, such as Runx2, ALP, DSPP, and OCN. In addition to the canonical pathways, there are also non-canonical pathways that are mediated by Wnt5a. The protein β-catenin is not involved in these pathways. Instead, non-canonical pathways are divided into two subtypes: the planar cell polarity (PCP) pathways and the Wnt/Ca2+ pathways. These non-canonical pathways play a role in tissue repair and regeneration as well (Monroe et al., 2012; He et al., 2014).
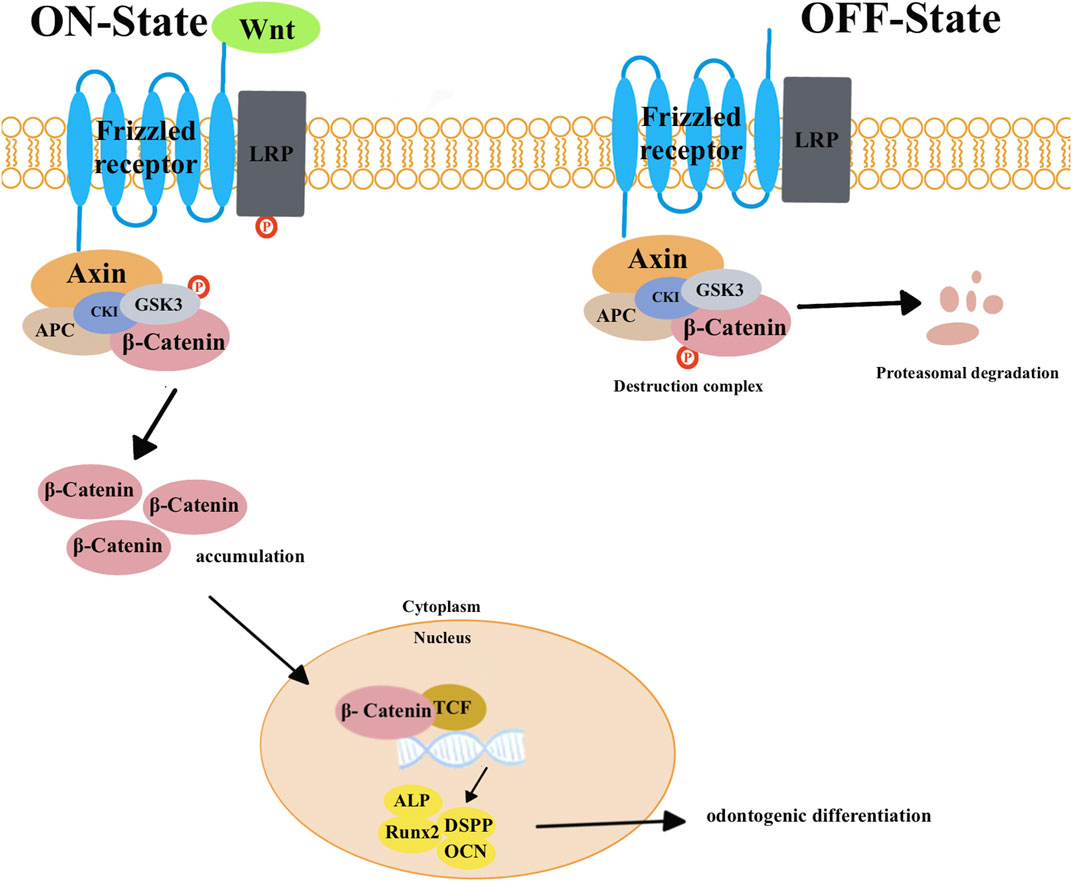
FIGURE 1. In canonical pathways, the presence of Wnt triggers a series of events. This begins with the binding of the frizzled receptor and the low-density lipoprotein receptor-related proteins 5 and 6 (LRP5/6). This binding transmits signals that lead to the degradation of the complex consisting of adenomatous polyposis coli (APC), glycogen synthase kinase-3β (GSK-3β), Axin, and casein kinase 1 (Ck1). This causes β-catenin to be released from this complex, resulting in an increase in its level. The increased β-catenin then moves into the nucleus, displaces co-repressors from transcription factors (TCFs), and activates target genes and proteins involved in odontogenic differentiation, such as Runx2, ALP, DSPP, and OCN. When Wnts are absent, β-catenin remains associated with a protein complex that includes APC, GSK3β, Axin, and Ck1. Within this complex, GSK-3β phosphorylates β-catenin to degrade it. This degradation prevents the occurrence of signal transduction in the Wnt signaling pathway.
Numerous studies have been conducted to investigate how Wnt signaling pathways affect the odontogenic differentiation of DPSCs. However, differences in results are noted. Some researchers have found that β-catenin can activate Runt-related transcription factor 2, (Runx2) and the accumulation of β-catenin can enhance the odontogenic differentiation of DPSCs (Kim et al., 2013; Han et al., 2014; Yoshida et al., 2016; Rahman et al., 2018). In another study, Xin et al. transfected wild-type and mutant special AT-rich sequence-binding protein 2 (SATB2) into DPSCs to induce odontogenic differentiation. The results showed that DPSCs transfected with wild-type SATB2 increased the expression of Runx2, osteopontin (OPN), and alkaline phosphatase (ALP) and also improved calcium nodule formation ability compared to those transfected with mutant SATB2. Dickkopf-1 (DKK1) is considered to be an inhibitor of the Wnt/β-catenin signaling pathways. Compared with wild-type ones, DPSCs that were transfected with mutant SATB2 increased the expression of DKK1 and decreased the expression of active β-catenin. Treated with XAV939 (inhibitor of Wnt signaling pathways), the expression of ALP and Runx2 was decreased both in wild-type and mutant SATB2 transfection groups. This indicates that wild-type SATB2 enhanced the odontogenesis of DPSCs through the Wnt/β-catenin signaling pathways (Xin et al., 2021). Chen et al. revealed that overexpression of differentiation antagonizing non-protein coding RNA (DANCR) suppressed the formation of mineralized nodules and the expression of dentin sialophosphoprotein (DSPP) and dentin matrix protein 1 (DMP-1) in DPSCs after 14 days of odontogenic induction. Meanwhile, the expression of β-catenin and the phosphorylation level of GSK-3β were significantly decreased. Therefore, DANCR inhibits the differentiation of DPSCs into odontoblast-like cells by blocking the canonical Wnt/β-catenin signaling pathways (Chen et al., 2016). Han et al. found that during the odontoblastic differentiation of DPSCs, the expression of β-catenin was upregulated. Knocking down β-catenin accumulation in DPSCs using lentivirus resulted in a significant reduction in matrix mineralization and calcium nodule formation and the expression of DSPP, DMP-1, and ALP. Conversely, stimulating β-catenin accumulation in DPSCs using LiCl led to a significant enhancement in calcium nodule formation and matrix mineralization and the expression of DSPP, DMP-1, ALP, and osteocalcin (OCN) (Han et al., 2014).
These findings suggested that when a special factor promotes the odontogenic differentiation of DPSCs, it can enhance the activity of proteins related to the Wnt signaling pathways. Therefore, these results supported the idea that Wnt signaling pathways can promote the differentiation of DPSCs (Yamashiro et al., 2007; Yokose and Naka, 2010; Lim et al., 2014; Rahman et al., 2018; Lu et al., 2019). Activation of the Wnt/β-catenin signaling pathways in DPSC cultures leads to a significant increase in the expression of important core pluripotency factors, such as SOX2, SSEA1, LIN28, NANOG, and REX1. As a result, the ability of DPSCs to proliferate, self-renew, and generate mature cell types becomes more efficient (Uribe-Etxebarria et al., 2017). The analysis of the metabolic and epigenetic changes revealed that activation of Wnt signaling pathways upregulated cellular consumption of glucose and glutamate/glutamine by DPSCs along with a higher mitochondrial activity (Uribe-Etxebarria et al., 2019). In addition, this transient “hyper-energetic” state appears to be crucial for maintaining the stemness of the cells.
On the contrary, some studies have shown that the Wnt/β-catenin signaling pathway could inhibit the odontogenic differentiation of DPSCs. They have found that a specific protein associated with the Wnt signaling pathway can suppress the odontogenic differentiation of DPSCs (Amri et al., 2016). ScheHer et al. demonstrated that Wnt-1 and β-catenin inhibited the odontogenic differentiation of DPSCs. They observed that DPSCs overexpressing Wnt1 and β-catenin showed reduced ALP activity after undergoing odontoblastic differentiation compared to control cells (Scheller et al., 2008). Zhang et al. found that overexpression of Wnt10A promotes the proliferation of DPSCs but hinders their odontoblastic differentiation. After 6-day odontogenic differentiation, Wnt10A-overexpressing DPSCs downregulated the expression of DSPP, DMP-1, ALP, and collagen-type I-alpha1 (COL1A1) (Zhang et al., 2014).
It seems that different molecules that control Wnt signaling pathways can have varying effects. ScheHer et al. enhanced the expression of β-catenin by retrovirus infection, while others activated β-catenin by exogenous application. This suggests that transient activation of β-catenin can induce odontogenic differentiation of DPSCs. Differences in the level of Wnt activity, experimental conditions, timing, or duration of activation could contribute to these variations (Vijaykumar et al., 2021). Vijaykumar et al. also discovered that activating Wnt/β-catenin signaling could enhance the number of BSP-GFP+ osteoblasts in dental pulp cultures, which aligns with other research findings (Kim et al., 2013; Teufel and Hartmann, 2019). Despite the variations in results, it still indicates that the Wnt/β-catenin signaling pathways are involved in regulating the differentiation of DPSCs, and the molecular mechanisms still need to be further studied in in vivo testing.
2.2 BMP/Smad signaling pathways
The transforming growth factor-β (TGF-β) superfamily transduces intracellular signals via Smad proteins (Figure 2). The bone morphogenetic protein (BMP), which belongs to the TGF-β superfamily, is most closely related to the Smad proteins (Figure 3) (Wu et al., 2016). BMP activates the Smad proteins, allowing signals to be transported to the nucleus for regulation of gene expression (Wu et al., 2016). BMP plays an important role in the process of tooth development, and inhibiting its function can lead to tooth development disorders (Fraser et al., 2004; Salazar et al., 2016; Lowery and Rosen, 2018). Previous studies have shown that BMP/Smad signaling pathways regulate DSPP both in vitro and in vivo (Chen et al., 2008; Chen et al., 2009; Cho et al., 2010). BMP2 can induce DPSCs to differentiate into odontoblasts, thereby achieving dentin regeneration (Woo et al., 2016; Hu et al., 2019; Ho et al., 2021).
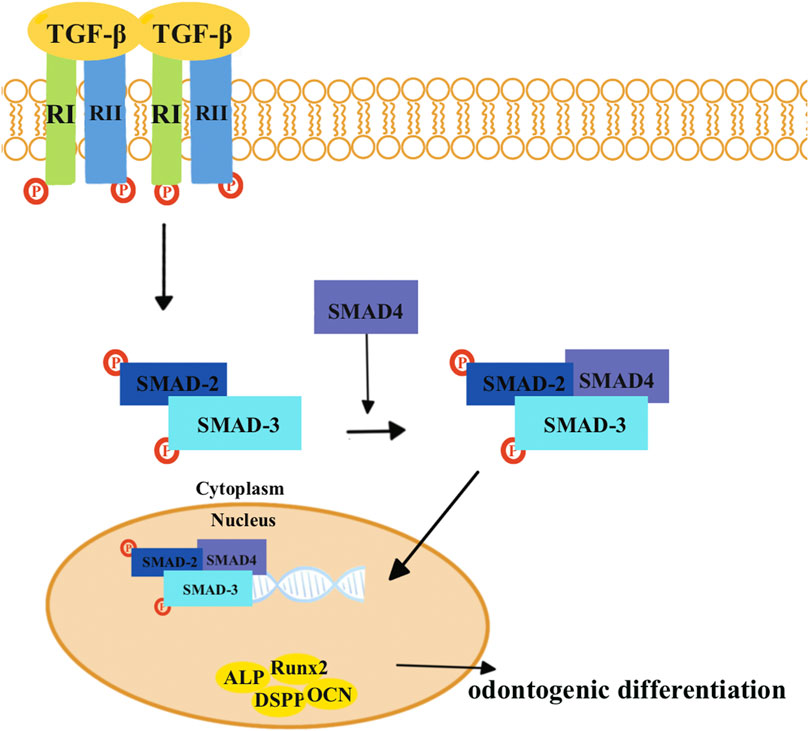
FIGURE 2. TGF-β combines with the TGF-β type II receptors (RIIs), which leads to the recruitment of TGF-β type I receptors (RIs). Then, two RIIs and two RIs form a heterotetrameric complex. The RI then recruits and phosphorylates SMAD2 and SMAD3, which can form heteromeric complexes with SMAD4. Eventually, these complexes translocate into the nucleus where they activate specific genes and proteins that are involved in odontogenic differentiation, including Runx2, ALP, DSPP, and OCN.
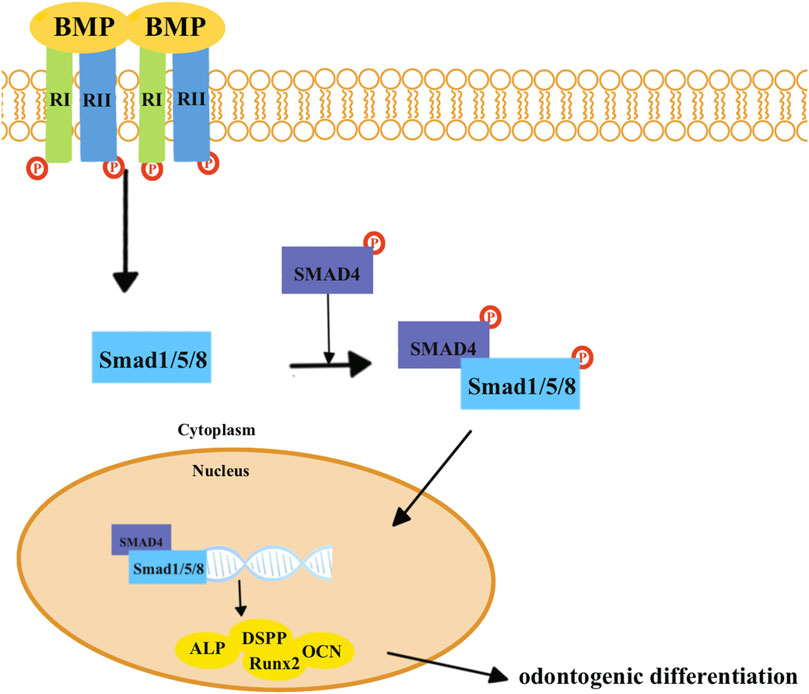
FIGURE 3. BMP combines with the BMP type I receptors (RIs) and TGF-β type II receptors (RIIs). Smad1, Smad5, and Smad8 are activated by BMP receptors, which can form heteromeric complexes with SMAD4. Eventually, these complexes translocate into the nucleus to activate target genes and proteins related to odontogenic differentiation, including Runx2, ALP, DSPP, and OCN.
Zhu et al. discovered that BMP-7 induced odontogenic differentiation of DPSCs. After 7-day and 14-day odontogenic differentiation, DPSCs that were treated with BMP-7 showed an increase in the expression of DSPP, DMP-1, ALP, and OCN. Meanwhile, Western blot results revealed that BMP-7 induction increased the expression of Smad5 and p-Smad5 proteins (Zhu et al., 2018). Qin et al. concluded that BMP2-induced odontoblastic differentiation of DPSCs was mediated by the activated Smad1/5 signaling pathways. DPSCs exposed to BMP-2 showed an enhancement in the phosphorylation of Smad1/5 and an increase in the expression of DSPP and DMP-1. However, these increased expressions were suppressed by noggin, which was an inhibitor of BMP/Smad signaling pathways. The results also revealed that the phosphorylation and nuclear translocation of Smad1/5 were partially inhibited by noggin (Qin et al., 2012b).
These studies have shown that BMP can stimulate the differentiation of DPSCs into odontoblasts. The protein associated with the Smad signaling pathways is also enhanced, which is consistent with the finding of other research studies (Nakashima and Reddi, 2003; Lin et al., 2007; Moioli et al., 2007; Yang et al., 2012). When the BMP/Smad signaling pathways are inhibited, it can negatively affect tooth development (Yamamoto et al., 2004). It has been reported that rhBMP-2 is involved in odontoblast differentiation in vitro (Saito et al., 2004; Casagrande et al., 2010). Therefore, it is clear that the BMP/Smad signaling pathways play a crucial role in the regeneration of the pulp–dentin complex.
2.3 Mitogen-activated protein kinase signaling pathways
The MAPK signaling pathways present in the cytoplasm play an important role in cell proliferation, differentiation, and apoptosis (English and Cobb, 2002). A typical MAPK cascade is mainly composed of three kinases, a MAPK (MPK), a MAPK kinase (MAPKK or MEK), and a MAPK kinase kinase (MAPKKK or MEKK). When a stimulus is detected, MAPKKKs phosphorylate and activate downstream MAPKKs, which then phosphorylate and activate the MAPKs. In turn, the activated MAPKs can phosphorylate numerous downstream substrates and activate cellular responses (Chen et al., 2021; Zhang and Zhang, 2022). The MAPK family is composed of three main subfamilies: extracellular signal-regulated kinase (ERK), P38MAPK, and c-Jun N-terminal kinase (JNKMAPK) (Pearson et al., 2001).
Ngo et al. concluded that leptin can stimulate the differentiation of DPSCs into odontoblasts by activating the MAPK signaling pathways. When DPSCs were treated with leptin, there was an increase in alkaline phosphatase (ALP) expression and mineralization, as well as the phosphorylation levels of ERK, P38MAPK, and JNK. However, leptin-induced DSPP protein expression levels and mineralization in DPSCs were blocked by ERK, JNK, or P38 inhibitors (Ngo et al., 2018). Cui et al. discovered that epiregulin enhanced the odontoblastic differentiation of DPSCs by MAPK signaling pathways. Treatment with recombinant human EREG led to an increase in the expression levels of odontogenic differentiation markers in DPSCs, as well as upregulation of phosphorylated P38MAPK and ERK. However, when EREG was knocked down using lentiviral EREG short hairpin RNAs or when P38MAPK or ERK was inhibited with specific inhibitors, the expression of DSPP, OCN, and Runx2 in DPSCs was reduced (Cui et al., 2019).
According to the aforementioned studies, when certain factors promote the odontogenic differentiation of DPSCs, the related protein of MAPK signaling pathways could be enhanced. MAPK signaling pathways are one of the crucial mechanisms that regulate cell proliferation and odontogenic differentiation (Liu et al., 2014; He et al., 2015; Sun et al., 2015; Lv et al., 2016; Rodriguez-Carballo et al., 2016; Wu et al., 2019; Cui et al., 2019). It has been reported that BMP-2, biodentine, and MTA can promote the odontogenic differentiation of DPSCs through activating MAPK signaling pathways (Qin et al., 2012a; Zhao et al., 2012; Luo et al., 2014; Sanz et al., 2021). The ERK signaling pathway, which is one of the characteristic MAPK signaling pathways, is involved in the regulation of cytodifferentiation. The P38MAPK signaling pathways are responsible for regulating cytokine expression and are activated by inflammatory cytokine signals (Su et al., 2019). JNKMAPK signaling pathways are stress-activated kinases associated with anti-proliferative and apoptotic functions (Johnson and Lapadat, 2002). However, further research is necessary to figure out the detailed mechanisms of the ERK P38 and JNK signaling pathways as well as their relationships.
2.4 Nuclear factor-kappa B signaling pathways
The NF-κB signaling pathways are involved in various biological processes, such as cell proliferation and apoptosis, inflammation, and immune response. The NF-κB proteins usually exist as inactive cytoplasmic complexes associated with inhibitors of the κB (IκB) family. NF-κB signaling pathways are divided into canonical and non-canonical signaling pathways (Figure 4). In canonical pathways, signals from numerous immune receptors can activate the TGFβ-activated kinase 1 (TAK1). TAK1 then phosphorylates IκB kinase-β (IKKβ), which in turn activates a complex called IκB kinase (IKK). The IKK complex is made up of two catalytic subunits (IKKα and IKKβ) and one regulatory subunit (IKKγ). Then, IκB family members, particularly IκBα, are phosphorylated by the IKK complex and eventually undergo ubiquitylation and proteasomal degradation. Consequently, various NF-κB complexes, predominantly the p50/RelA dimers, translocate to the nucleus.
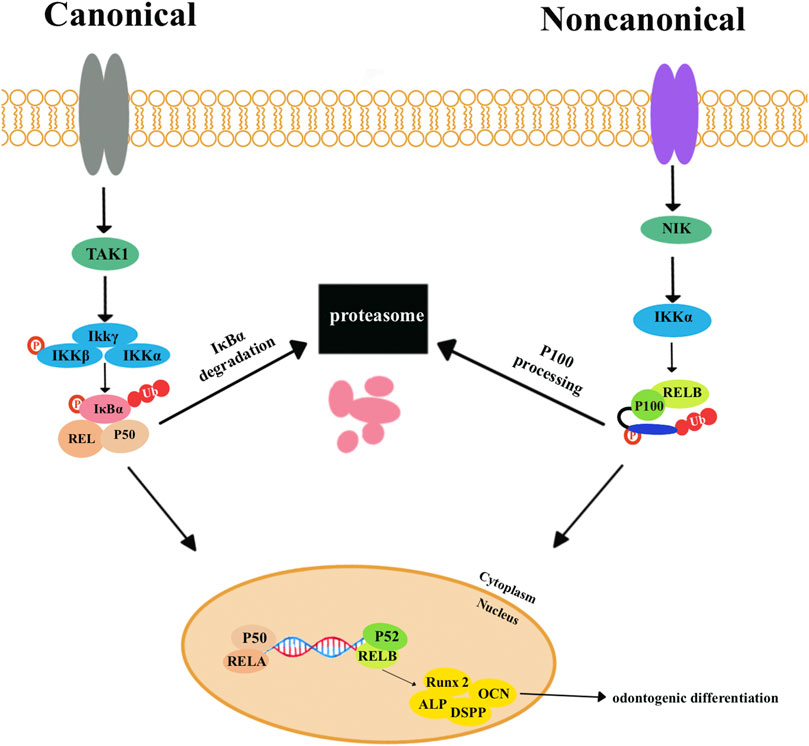
FIGURE 4. The canonical nuclear factor-κB (NF-κB) signaling pathways are activated by signals from various immune receptors. These signals activate the kinase TGFβ-activated kinase 1 (TAK1). TAK1 phosphorylates IκB kinase-β (IKKβ) to activate the trimeric IκB kinase (IKK) complexes, which are composed of two catalytic subunits (IKKα and IKKβ) and one regulatory subunit (IKKγ). IκB family members, particularly IκBα, are phosphorylated by IKK complexes and then undergo ubiquitylation and proteasomal degradation. Eventually, various NF-κB complexes, predominantly the p50/RELA dimers, translocate to the nucleus. Non-canonical NF-κB signaling pathways depend on phosphorylation-induced p100 processing, which is activated by signals from a subset of tumor necrosis factor receptor (TNFR) members. TNFR activates kinase NFκB-inducing kinase (NIK). NIK phosphorylates and triggers IKKα, which can phosphorylate the carboxy-terminal serine residues of p100, resulting in the degradation of the C-terminal IκB-like structure of p100. P52 and RELB translocate to the nucleus to activate target genes and proteins related to odontogenic differentiation, including Runx2, ALP, DSPP, and OCN.
The non-canonical pathway responds primarily to the members of the tumor necrosis factor receptor (TNFR), which can activate the kinase NF-κB-inducing kinase (NIK). IKKα is phosphorylated and activated by NIK, which then phosphorylates and degenerates p100 and generates p52. P52 and RELB translocate to the nucleus to activate target genes and proteins related to odontogenic differentiation, including Runx2, ALP, DSPP, and OCN (Sun, 2011; Sun, 2017).
Wang et al. discovered that estrogen deficiency decreased the odonto/osteogenic capacity of DPSCs via the NF-κB signaling pathways (Wang et al., 2013). They established an estrogen-deficient rat model by bilateral ovariectomy (OVX). The results revealed that compared to DPSCs from the sham-operated group (sham DPSCs), the odonto/osteogenic differentiation capacity of OVX-DPSCs was significantly decreased both in vitro and in vivo. Meanwhile, suppression of NF-κB signaling pathways could enhance the reduced odonto/osteogenic potential in ovariectomized mice. Li et al. (2023) confirmed that baicalin can promote odonto/osteogenic differentiation of dental pulp inflammatory stem cells by inhibiting NF-κB signaling pathways.
According to the aforementioned studies, when a certain factor promotes the odontogenic differentiation of DPSCs, the related protein of NF-κB signaling pathways could be enhanced (Hozhabri et al., 2015; Pei et al., 2016; Wu et al., 2019). It has also been found that activation of NF-κB can inhibit the odontoblast differentiation in an inflammatory microenvironment (Pei et al., 2016). Therefore, NF-κB signaling pathways do play an important role in the odontogenic differentiation of DPSCs.
2.5 Phosphoinositide-3-kinase/protein kinase B/mammalian target of rapamycin signaling pathways
The PI3K/AKT/mTOR signaling pathways (Figure 5) are essential for cellular processes, including cell growth, survival, and metabolism. These pathways involve key components including PI3Ks, AKT, and mTOR. When growth factor stimulus combines with a receptor tyrosine kinase (RTK), activated PI3K can convert phosphatidylinositol 4,5-bisphosphate (PIP2) into phosphatidylinositol 3,4,5-triphosphate (PIP3). This conversion can be reversed by the phosphatase and tensin homolog (PTEN). PIP3 then activates PI3K-dependent kinase 1 (PDK1). Subsequently, PDK1 and mTORC2 will, respectively, phosphorylate amino acid residues T308 and S473, leading to the activation of AKT to phosphorylate target proteins (Keppler-Noreuil et al., 2016; Yu and Cui, 2016).
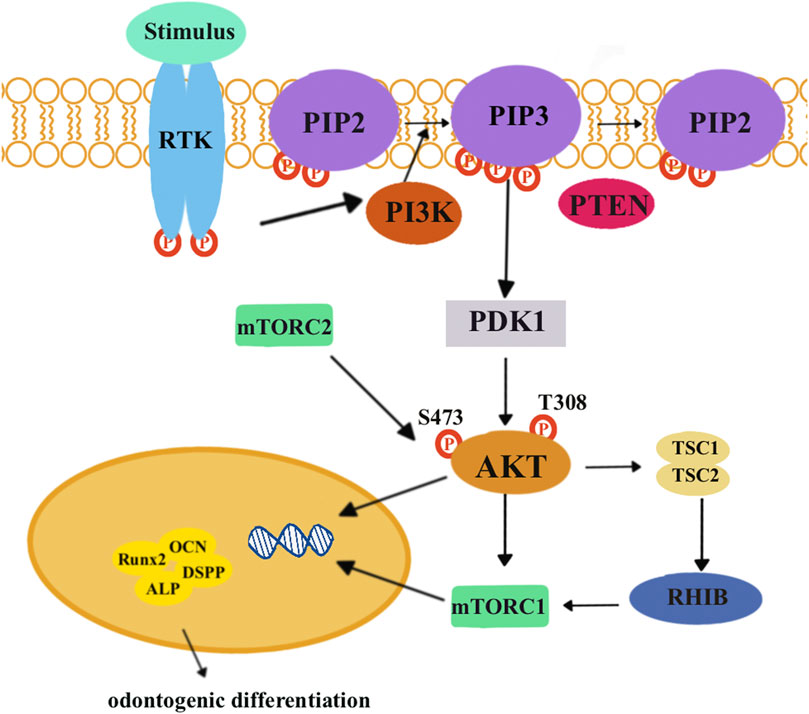
FIGURE 5. When growth factor stimulus combines with receptor tyrosine kinase (RTK), it activates a protein called PI3K. This activated PI3K can then phosphorylate phosphatidylinositol (4,5)-bisphosphate (PIP2) to phosphatidylinositol (3,4,5)-triphosphate (PIP3). PIP3 can be further dephosphorylated by tensin homolog (PTEN) and activate PI3K-dependent kinase 1 (PDK1). PDK1, along with another protein called mTORC2, can phosphorylate specific amino acid residues, T308 and S473, respectively, to activate AKT. Eventually, activated AKT can phosphorylate target proteins to promote cell growth and survival and metabolism. Activated AKT inhibits inactive tuberous sclerosis complexes 1 and 2 (TSCs 1 and 2), which are unable to bind the RAS homolog enriched in the brain (RHEB). Then, mTORC1 is activated and exerts its effects on downstream target genes and proteins related to odontogenic differentiation, including Runx2, ALP, DSPP, and OCN.
Lin et al. discovered that low concentrations of graphene oxide quantum dots (GOQDs) could enhance the odontoblastic differentiation of DPSCs. After 14-day odontoblastic differentiation, the expression level of phosphorylated AMPK increased, while the expression level of phosphorylated mTOR decreased. This indicated that GOQDs would activate the AMPK signaling pathways but inhibit the mTOR signaling pathways in DPSCs (Lin et al., 2023). Xu et al. (2021) found that NaF promoted osteo/odontogenic differentiation of DPSCs by suppressing the PI3K/AKT/mTOR signaling pathways. KEGG analysis and the results of PCR and Western blotting revealed that there was a differential expression in the PI3K/AKT/mTOR signaling pathways between NaF-treated DPSCs and the control group. The p-AKT/AKT ratio was lower in NaF-treated DPSCs. After inhibiting the PI3K/AKT/mTOR signaling pathways, NaF-treated DPSCs upregulated the expression of DSPP and DMP-1 and mineralization.
The aforementioned studies indicated that when a certain factor promotes the odontogenic differentiation of DPSCs, the related proteins of the PI3K/AKT/mTOR signaling pathways decreased. They concluded that the PI3K/AKT/mTOR signaling pathways negatively regulated the odontogenic differentiation of DPSCs. Osteogenic/dentinogenic differentiation of stem cells from apical papilla (SCAP) could be promoted by inhibiting PI3K/AKT/mTOR signaling pathways (Bhaskar and Hay, 2007). Consistently, odontogenic differentiation of DPSCs could be blocked by PI3K/AKT/mTOR signaling pathways (Kajiura et al., 2021; Park et al., 2022). However, PI3K/AKT/mTOR signaling pathways could promote the osteogenic differentiation of periodontal ligament stem cells (Huang et al., 2021). Considering this difference, we need further studies to figure out the mechanisms.
2.6 Notch signaling pathways
Notch signaling pathways (Figure 6) are highly conserved pathways that enable communication between neighboring cells that influence proliferation, differentiation, and apoptotic events during development (Artavanis-Tsakonas et al., 1999). The Notch signaling pathways include four transmembrane Notch receptors (Notch-1, Notch-2, Notch-3, and Notch-4) and two families of ligands (Delta and Jagged). When a receptor binds to a ligand from a neighboring cell, Notch receptors will undergo proteolytic cleavages and release the Notch intracellular domain (NICD). The NICD then moves into the nucleus and combines with members of the CSL (CBF-1, Suppressor of Hairless, and Lag-1) transcription factor family to activate Notch target genes (Lai, 2004; Espinoza et al., 2013; Nandagopal et al., 2018).
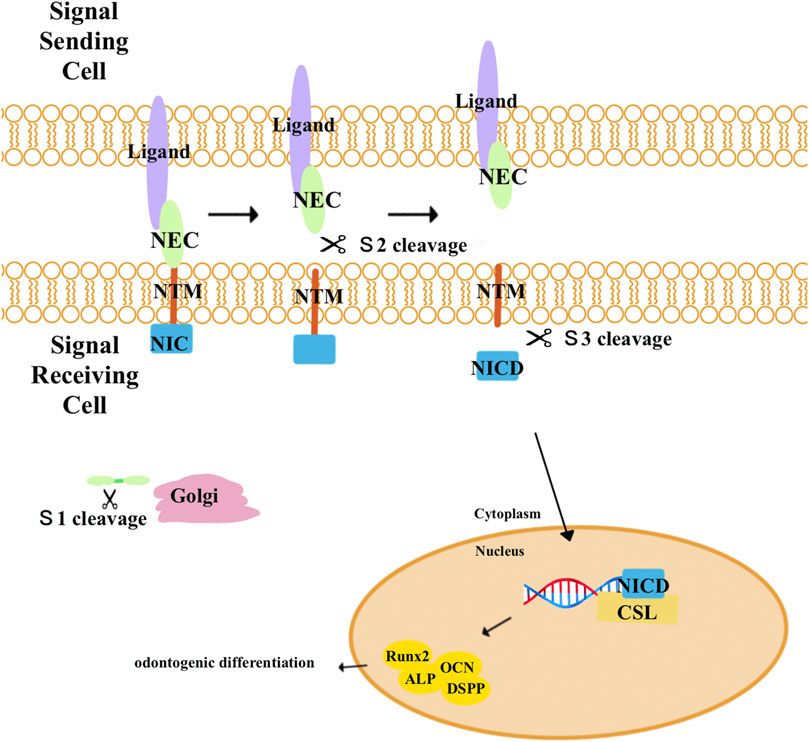
FIGURE 6. Notch receptors are activated by combination with a ligand from a neighboring cell and undergo three proteolytic cleavages. First, Notch precursor proteins are cleaved (S1) by a furin-like convertase in the trans-Golgi apparatus to generate the mature Notch receptor. Composed of Notch extracellular (NEC) and Notch transmembrane (N™) and an intracellular portion of Notch (NIC) subunits, the mature Notch receptor is delivered to the plasma membrane. When the receptors bind to a ligand from a neighboring cell, a second cleavage (S2) by ADAM metalloproteases dissociates the NEC from N™ and NIC. Lastly, N™ and NIC undergo another cleavage by the γ-secretase complex (S3), leading to the release of NIC. NIC then translocates into the nucleus where it combines with the CBF-1-Suppressor of Hairless/Lag1 (CSL) to activate target genes and proteins related to odontogenic differentiation, including Runx2, ALP, DSPP, and OCN.
Notch signaling has been found to promote osteo/odontogenic differentiation in human bone marrow MSCs, human periodontal ligament stem cells, human adipose stem cells, and stem cells isolated from human exfoliated deciduous teeth (Lough et al., 2016; Liao et al., 2017; Tian et al., 2017; Bagheri et al., 2018). However, the impact of Notch signaling on osteogenic differentiation remains unclear. Zhang et al. (2008) demonstrated that the activation of Notch signaling pathways by Jagged-1 inhibited the odontogenic differentiation of DPSCs in vitro and in vivo. Meanwhile, overexpression of the constitutively activated Notch-ICD could also inhibit the differentiation of DPSCs. When DPSCs were engineered to express Jagged-1 (DPSC/Jag) using retroviruses, they showed decreased levels of ALP activity and the formation of calcified nodules and dentin sialoprotein (DSP). Two months after transplanting cells into nude mice, the results revealed that control cells formed mineralized tissues, but DPSC/Jag cells did not generate any mineralized tissues in vivo. According to their results, since Notch signaling inhibited DSPP expression in DPSCs, it increases the possibility that Notch signaling could inhibit Runx2 transcriptional activities. In contrast, Manokawinchoke et al. discovered that after maintaining the DPSCs on indirect immobilized Jagged1 surfaces in an osteogenic medium, the odonto/osteogenic differentiation of DPSCs was enhanced (Manokawinchoke et al., 2017). Furthermore, the effects of Notch signaling on the differentiation of DPSCs may vary depending on the specific Notch ligands. He et al. discovered that the activation of Notch signaling pathways by Delta1 enhanced the proliferation and odontogenic differentiation of DPSCs. The cells transduced with Delta1 formed more calcified nodules in less time and with enhanced DSPP expression (He et al., 2009). Wang et al. revealed that inhibition of Delta1 could promote odontogenic differentiation of DPSCs in vitro. Lentivirus-mediated Delta1-RNAi stably knocked down the expression of Delta1 and Notch signaling. Furthermore, the differentiating capability of DPSCs/Delta1-RNAi into odontoblasts is much higher than that of control groups (Wang et al., 2011).
Notch receptors and ligands were expressed in the dental mesenchyme and kept DPSCs in an undifferentiated state to protect the dental pulp from injury (Mitsiadis et al., 1997; Mitsiadis et al., 1998; Harada et al., 1999). According to the aforementioned studies, different ligands had varying effects on the odontogenic differentiation of DPSCs, which may result from different experimental conditions or stimuli. The exact mechanisms of Notch signaling pathways remain unclear; thus, we need more research to figure out.
2.7 Multipath parallel signaling pathways
The process of odontogenic differentiation of DPSCs is considerably complex, involving the activation of multiple signaling pathways simultaneously. However, most studies failed to explore the underlying mechanisms between activated signaling pathways. It is crucial to figure out synergistic effects between different signaling pathways. In this section, we introduce several interactions between different signaling pathways. According to our search, most signaling pathways interact with MAPK signaling pathways. It appears that MAPK signaling pathways play an important role in promoting the odontogenic differentiation of DPSCs. In addition, we need further studies to figure out the detailed mechanisms.
2.7.1 MAPK signaling pathways and Wnt signaling pathways
It has been reported that MAPK signaling pathways and Wnt signaling pathways can interact with each other (Li et al., 2014). Li revealed that Wnt6 can activate the JNK signaling pathways in DPSCs and promote odontogenic differentiation. Wnt6 could upregulate the phosphorylation of JNK. While treated with the JNK pathway inhibitor (SP600125), the activation of JNK activity and the expression of c-Jun mRNA were decreased. This indicates that Wnt6 activated JNK signaling in DPSCs. Meanwhile, they discovered that Wnt6 enhanced the level of DSPP, Runx2, and DMP-1 mRNA as well as upregulated the activity of ALP and the formation of calcium deposits. However, when DPSCs were treated with SP600125, these effects of Wnt6 on DPSCs were blocked.
2.7.2 MAPK signaling pathways and Smad signaling pathways
MAPK signaling pathways can interact with the Smad signaling pathway through the phosphorylation process in DPSCs. Kong et al. demonstrated that high extracellular Mg2+ could enhance the odontogenic differentiation of DPSCs by activating the ERK/BMP2/Smad signaling pathways. KEGG pathway analysis revealed that after odontogenic differentiation with high extracellular Mg2+, genes related to the MAPK and TGF-β signaling pathways in DPSCs were differentially expressed. Compared to DPSCs treated with 0 mM Mg2+, those treated with high extracellular Mg2+ showed increased levels of Runx2, DMP-1, DSPP, phosphorylated ERK, BMP2, BMPR1, and phosphorylated Smad1/5/9. Meanwhile, these effects could be reduced by 2-APB (inhibiting Mg2+entry) or U0126 (inhibiting ERK signaling)(Kong et al., 2019). Li et al. confirmed that extracellular Ca2+ triggered the BMP2-mediated Smad1/5/8 and Erk1/2 signaling pathways of DPSCs, and these pathways converged on the Runx2 gene to control the odontogenic differentiation of DPSCs (Li et al., 2015). In addition, P38MAPK has been found to mediate the phosphorylation of Smad3 in rat myofibroblasts (Furukawa et al., 2003). Furthermore, Wang et al. (2006); Li et al. (2022) discovered that P38MAPK differentially affected the phosphorylation of Smad2 and Smad3 during TGF-β signaling and affected the odontogenic differentiation of DPSCs, and ERK1/2 may be involved in the process.
2.7.3 MAPK signaling pathways and Wnt signaling pathways and Smad signaling pathways
Yang et al. suggested that P38MAPK signaling pathways can mediate BMP2 to enhance the levels of β-catenin in DPSCs. By treating DPSCs with rhBMP2, they observed an increase in the expression of β-catenin in both the cytoplasm and nucleus, as well as overall protein expression. Stimulation with BMP2 would increase the obtained TOPflash values, indicating that BMP2 could activate canonical Wnt signaling. They found that BMP2 could activate P38MAPK signaling, and when canonical BMP signaling pathways were blocked with LDN193189, the levels of p-P38MAPK were decreased. When they blocked P38MAPK signaling with SB203580, the levels of β-catenin were not changed after BMP2 stimulation, indicating that blockade of the P38MAPK pathway could prevent BMP2-induced activation of Wnt signaling pathways and differentiation of DPSCs (Yang et al., 2015). Based on these findings, we infer that MAPK signaling pathways, Wnt signaling pathways, and Smad signaling pathways might have a relationship in the differentiation of DPSCs.
2.7.4 MAPK signaling pathways and PI3K/AKT/mTOR signaling pathways
Li J. et al. (2022) indicated that the ERK and JNK pathways and the PI3K/AKT/mTOR pathways were involved in the AREG-induced differentiation of DPSCs. Treatment with AREG could increase the protein expression levels of p-ERK, p-JNK, and p-AKT in DPSCs. These effects were inhibited by the PI3K, ERK, JNK, and P38 pathway inhibitors as well as reduced the expression of the mineralization markers and mineralized nodule formation in DPSCs. This research indicated that MAPK signaling pathways and PI3K/AKT/mTOR signaling pathways are interconnected in the odontogenic differentiation of DPSCs.
2.7.5 MAPK signaling pathways and NF-κB signaling pathways
He et al. (2017) demonstrated that low concentrations of the inflammatory cytokine (IFN-γ) inhibited the odonto/osteogenic differentiation of DPSCs through the activation of the NF-κB and MAPK signaling pathways. However, when the NF-κB inhibitor or MAPK inhibitors were applied, it was observed that the odonto/osteogenic differentiation of DPSCs was enhanced, both in vivo and in vitro, in comparison to the group treated with IFN-γ alone. This study indicated that a certain factor could promote the odontogenic differentiation of DPSCs through NF-κB and MAPK signaling pathways.
2.7.6 Notch signaling pathways and Wnt signaling pathways
Previous studies have reported on the interplay between the Notch and Wnt signaling pathways (Ross and Kadesch, 2001; Foltz et al., 2002; Brack et al., 2008). Kornsuthisopon et al. proved that Wnt5A is involved in the Jagged1-induced mineralization in DPSCs (Kornsuthisopon et al., 2022). This indicated that there may be interactions between the Notch and Wnt signaling pathways during the process of odontogenic differentiation in DPSCs. Kornsuthisopon et al. revealed that Jagged1-mediated Notch activation enhanced the expression of Wnt-related genes, including Wnt2B and Wnt5A. Treatment with Wnt5A enhances mineralization, indicating its potential involvement in the Notch-induced osteo/odontogenic differentiation of DPSCs.
3 Conclusion
At present, numerous types of stem cells have shown their clinical therapeutic potential (Kuehnle and Goodell, 2002). DPSCs can be easily isolated with versatile differentiation potential, leading to them being the focus in bone and dental tissue engineering. However, DPSCs rapidly lose their ability to proliferate and multipotent differentiation in in vitro culture, emphasizing the need for improvement (Horibe et al., 2014).
Many researchers have conducted mineralization experiments in vitro using cell culture and have found that certain factors can increase the expression of odontogenic differentiation markers in DPSCs, as well as the phosphorylation or expression of key molecules in related signaling pathways. It indicated that these factors could be used to activate related signaling pathways to mediate the odontogenic differentiation of DPSCs.
In this review, we introduced six signaling pathways with different effects on the odontogenic differentiation of DPSCs. Wnt and Notch signaling pathways have opposite influences on the odontogenic differentiation of DPSCs by distinct stimuli, and the detailed mechanisms need to be figured out. However, due to the complex mechanisms of the signaling pathways, most studies have only assessed the basic mechanisms in vitro. We need to explore the odontoblast differentiation and repair capability of DPSCs through in vivo studies to understand the further mechanisms. Meanwhile, through mineralization experiments and gene expression analysis, most studies have only verified that a certain factor triggered or inhibited a certain signaling pathway to influence the odontogenic differentiation of DPSCs. As is known to all, each signaling pathway does not exist in isolation but rather has certain interactions between them. Nevertheless, a few literature works summarize all the signaling pathways related to the odontogenic differentiation of DPSCs.
In brief, gaining a comprehensive understanding of the signaling pathways in the odontogenic differentiation of DPSCs will help researchers discover the most effective materials in promoting the regeneration of the pulp–dentin complex. We need further studies to figure out the detailed mechanisms of signaling pathways through in vivo and in vitro studies. Similarly, researchers should pay attention to the relationships between parallel multipath signaling pathways, when assessing the influence of different factors on the odontogenic differentiation of DPSCs.
Author contributions
LZ: writing–original draft, conceptualization, and methodology. SZ: writing–review and editing. XX: writing–review and editing.
Funding
The author(s) declare financial support was received for the research, authorship, and/or publication of this article. The work was supported by the Six Talent Peaks Project in Jiangsu Province (2019-wsw-128) and the “3456” Cultivation Program for Junior Talents of Nanjing Stomatological School, Medical School of Nanjing University (0222C114).
Conflict of interest
The authors declare that the research was conducted in the absence of any commercial or financial relationships that could be construed as a potential conflict of interest.
Publisher’s note
All claims expressed in this article are solely those of the authors and do not necessarily represent those of their affiliated organizations, or those of the publisher, the editors, and the reviewers. Any product that may be evaluated in this article, or claim that may be made by its manufacturer, is not guaranteed or endorsed by the publisher.
References
Amri N., Djole S. X., Petit S., Babajko S., Coudert A. E., Castaneda B., et al. (2016). Distorted patterns of dentinogenesis and eruption in Msx2 null mutants: involvement of sost/sclerostin. Am. J. Pathol. 186 (10), 2577–2587. doi:10.1016/j.ajpath.2016.06.013
Arana-Chavez V. E., Massa L. F. (2004). Odontoblasts: the cells forming and maintaining dentine. Int. J. Biochem. Cell Biol. 36 (8), 1367–1373. doi:10.1016/j.biocel.2004.01.006
Artavanis-Tsakonas S., Rand M. D., Lake R. J. (1999). Notch signaling: cell fate control and signal integration in development. Science 284 (5415), 770–776. doi:10.1126/science.284.5415.770
Ba P., Duan X., Fu G., Lv S., Yang P., Sun Q. (2017). Differential effects of p38 and Erk1/2 on the chondrogenic and osteogenic differentiation of dental pulp stem cells. Mol. Med. Rep. 16 (1), 63–68. doi:10.3892/mmr.2017.6563
Bagheri L., Pellati A., Rizzo P., Aquila G., Massari L., De Mattei M., et al. (2018). Notch pathway is active during osteogenic differentiation of human bone marrow mesenchymal stem cells induced by pulsed electromagnetic fields. J. Tissue Eng. Regen. Med. 12 (2), 304–315. doi:10.1002/term.2455
Bhaskar P. T., Hay N. (2007). The two TORCs and Akt. Dev. Cell 12 (4), 487–502. doi:10.1016/j.devcel.2007.03.020
Brack A. S., Conboy I. M., Conboy M. J., Shen J., Rando T. A. (2008). A temporal switch from notch to Wnt signaling in muscle stem cells is necessary for normal adult myogenesis. Cell Stem Cell 2 (1), 50–59. doi:10.1016/j.stem.2007.10.006
Casagrande L., Demarco F. F., Zhang Z., Araujo F. B., Shi S., Nor J. E. (2010). Dentin-derived BMP-2 and odontoblast differentiation. J. Dent. Res. 89 (6), 603–608. doi:10.1177/0022034510364487
Chen J., Wang L., Yuan M. (2021). Update on the roles of rice MAPK cascades. Int. J. Mol. Sci. 22 (4), 1679. doi:10.3390/ijms22041679
Chen L., Song Z., Huang S., Wang R., Qin W., Guo J., et al. (2016). lncRNA DANCR suppresses odontoblast-like differentiation of human dental pulp cells by inhibiting wnt/β-catenin pathway. Cell Tissue Res. 364 (2), 309–318. doi:10.1007/s00441-015-2333-2
Chen S., Gluhak-Heinrich J., Martinez M., Li T., Wu Y., Chuang H. H., et al. (2008). Bone morphogenetic protein 2 mediates dentin sialophosphoprotein expression and odontoblast differentiation via NF-Y signaling. J. Biol. Chem. 283 (28), 19359–19370. doi:10.1074/jbc.M709492200
Chen S., Gluhak-Heinrich J., Wang Y. H., Wu Y. M., Chuang H. H., Chen L., et al. (2009). Runx2, osx, and dspp in tooth development. J. Dent. Res. 88 (10), 904–909. doi:10.1177/0022034509342873
Cho Y. D., Yoon W. J., Woo K. M., Baek J. H., Park J. C., Ryoo H. M. (2010). The canonical BMP signaling pathway plays a crucial part in stimulation of dentin sialophosphoprotein expression by BMP-2. J. Biol. Chem. 285 (47), 36369–36376. doi:10.1074/jbc.M110.103093
Cui D., Xiao J., Zhou Y., Zhou X., Liu Y., Peng Y., et al. (2019). Epiregulin enhances odontoblastic differentiation of dental pulp stem cells via activating MAPK signalling pathway. Cell Prolif. 52 (6), e12680. doi:10.1111/cpr.12680
English J. M., Cobb M. H. (2002). Pharmacological inhibitors of MAPK pathways. Trends Pharmacol. Sci. 23 (1), 40–45. doi:10.1016/s0165-6147(00)01865-4
Espinoza I., Pochampally R., Xing F., Watabe K., Miele L. (2013). Notch signaling: targeting cancer stem cells and epithelial-to-mesenchymal transition. Onco Targets Ther. 6, 1249–1259. doi:10.2147/OTT.S36162
Foltz D. R., Santiago M. C., Berechid B. E., Nye J. S. (2002). Glycogen synthase kinase-3beta modulates notch signaling and stability. Curr. Biol. 12 (12), 1006–1011. doi:10.1016/s0960-9822(02)00888-6
Fraser G. J., Graham A., Smith M. M. (2004). Conserved deployment of genes during odontogenesis across osteichthyans. Proc. Biol. Sci. 271 (1555), 2311–2317. doi:10.1098/rspb.2004.2878
Furukawa F., Matsuzaki K., Mori S., Tahashi Y., Yoshida K., Sugano Y., et al. (2003). p38 MAPK mediates fibrogenic signal through Smad3 phosphorylation in rat myofibroblasts. Hepatology 38 (4), 879–889. doi:10.1053/jhep.2003.50384
Gronthos S., Mankani M., Brahim J., Robey P. G., Shi S. (2000). Postnatal human dental pulp stem cells (DPSCs) in vitro and in vivo. Proc. Natl. Acad. Sci. U. S. A. 97 (25), 13625–13630. doi:10.1073/pnas.240309797
Ha J., Bharti D., Kang Y. H., Lee S. Y., Oh S. J., Kim S. B., et al. (2021). Human dental pulp-derived mesenchymal stem cell potential to differentiate into smooth muscle-like cells in vitro. Biomed. Res. Int. 2021, 8858412. doi:10.1155/2021/8858412
Han N., Zheng Y., Li R., Li X., Zhou M., Niu Y., et al. (2014). β-catenin enhances odontoblastic differentiation of dental pulp cells through activation of Runx2. PLoS One 9 (2), e88890. doi:10.1371/journal.pone.0088890
Harada H., Kettunen P., Jung H. S., Mustonen T., Wang Y. A., Thesleff I. (1999). Localization of putative stem cells in dental epithelium and their association with Notch and FGF signaling. J. Cell Biol. 147 (1), 105–120. doi:10.1083/jcb.147.1.105
He F., Yang Z., Tan Y., Yu N., Wang X., Yao N., et al. (2009). Effects of Notch ligand Delta1 on the proliferation and differentiation of human dental pulp stem cells in vitro. Arch. Oral Biol. 54 (3), 216–222. doi:10.1016/j.archoralbio.2008.10.003
He W., Wang Z., Luo Z., Yu Q., Jiang Y., Zhang Y., et al. (2015). LPS promote the odontoblastic differentiation of human dental pulp stem cells via MAPK signaling pathway. J. Cell Physiol. 230 (3), 554–561. doi:10.1002/jcp.24732
He W., Wang Z., Zhou Z., Zhang Y., Zhu Q., Wei K., et al. (2014). Lipopolysaccharide enhances Wnt5a expression through toll-like receptor 4, myeloid differentiating factor 88, phosphatidylinositol 3-OH kinase/AKT and nuclear factor kappa B pathways in human dental pulp stem cells. J. Endod. 40 (1), 69–75. doi:10.1016/j.joen.2013.09.011
He X., Jiang W., Luo Z., Qu T., Wang Z., Liu N., et al. (2017). IFN-γ regulates human dental pulp stem cells behavior via NF-κB and MAPK signaling. Sci. Rep. 7, 40681. doi:10.1038/srep40681
Ho C. T., Huang Y. W., Chen T. R., Lo C. H., Lo W. C. (2021). Discovering the ultimate limits of protein secondary structure prediction. Biomolecules 11 (11), 1627. doi:10.3390/biom11111627
Horibe H., Murakami M., Iohara K., Hayashi Y., Takeuchi N., Takei Y., et al. (2014). Isolation of a stable subpopulation of mobilized dental pulp stem cells (MDPSCs) with high proliferation, migration, and regeneration potential is independent of age. PLoS One 9 (5), e98553. doi:10.1371/journal.pone.0098553
Hozhabri N. S., Benson M. D., Vu M. D., Patel R. H., Martinez R. M., Nakhaie F. N., et al. (2015). Decreasing NF-κB expression enhances odontoblastic differentiation and collagen expression in dental pulp stem cells exposed to inflammatory cytokines. PLoS One 10 (1), e0113334. doi:10.1371/journal.pone.0113334
Hu X., Zhong Y., Kong Y., Chen Y., Feng J., Zheng J. (2019). Lineage-specific exosomes promote the odontogenic differentiation of human dental pulp stem cells (DPSCs) through TGFβ1/smads signaling pathway via transfer of microRNAs. Stem Cell Res. Ther. 10 (1), 170. doi:10.1186/s13287-019-1278-x
Huang T. Y., Shahrousvand M., Hsu Y. T., Su W. T. (2021). Polycaprolactone/polyethylene glycol blended with dipsacus asper wall extract nanofibers promote osteogenic differentiation of periodontal ligament stem cells. Polym. (Basel) 13 (14), 2245. doi:10.3390/polym13142245
Hull P. S., Worthington H. V., Clerehugh V., Tsirba R., Davies R. M., Clarkson J. E. (1997). The reasons for tooth extractions in adults and their validation. J. Dent. 25 (3-4), 233–237. doi:10.1016/s0300-5712(96)00029-2
Jahanbin A., Rashed R., Alamdari D. H., Koohestanian N., Ezzati A., Kazemian M., et al. (2016). Success of maxillary alveolar defect repair in rats using osteoblast-differentiated human deciduous dental pulp stem cells. J. Oral Maxillofac. Surg. 74 (4), e821–e9. doi:10.1016/j.joms.2015.11.033
Janebodin K., Horst O. V., Ieronimakis N., Balasundaram G., Reesukumal K., Pratumvinit B., et al. (2011). Isolation and characterization of neural crest-derived stem cells from dental pulp of neonatal mice. PLoS One 6 (11), e27526. doi:10.1371/journal.pone.0027526
Jeeruphan T., Jantarat J., Yanpiset K., Suwannapan L., Khewsawai P., Hargreaves K. M. (2012). Mahidol study 1: comparison of radiographic and survival outcomes of immature teeth treated with either regenerative endodontic or apexification methods: a retrospective study. J. Endod. 38 (10), 1330–1336. doi:10.1016/j.joen.2012.06.028
Jiang W., Wang D., Alraies A., Liu Q., Zhu B., Sloan A. J., et al. (2019). Wnt-GSK3β/β-Catenin regulates the differentiation of dental pulp stem cells into bladder smooth muscle cells. Stem Cells Int. 2019, 8907570. doi:10.1155/2019/8907570
Johnson G. L., Lapadat R. (2002). Mitogen-activated protein kinase pathways mediated by ERK, JNK, and p38 protein kinases. Science 298 (5600), 1911–1912. doi:10.1126/science.1072682
Kajiura K., Umemura N., Ohkoshi E., Ohta T., Kondoh N., Kawano S. (2021). Shikonin induces odontoblastic differentiation of dental pulp stem cells via AKT-mTOR signaling in the presence of CD44. Connect. Tissue Res. 62 (6), 689–697. doi:10.1080/03008207.2020.1865937
Keppler-Noreuil K. M., Parker V. E., Darling T. N., Martinez-Agosto J. A. (2016). Somatic overgrowth disorders of the PI3K/AKT/mTOR pathway and therapeutic strategies. Am. J. Med. Genet. C Semin. Med. Genet. 172 (4), 402–421. doi:10.1002/ajmg.c.31531
Kim J. H., Liu X., Wang J., Chen X., Zhang H., Kim S. H., et al. (2013a). Wnt signaling in bone formation and its therapeutic potential for bone diseases. Ther. Adv. Musculoskelet. Dis. 5 (1), 13–31. doi:10.1177/1759720X12466608
Kim T. H., Bae C. H., Lee J. C., Ko S. O., Yang X., Jiang R., et al. (2013b). β-catenin is required in odontoblasts for tooth root formation. J. Dent. Res. 92 (3), 215–221. doi:10.1177/0022034512470137
Kong Y., Hu X., Zhong Y., Xu K., Wu B., Zheng J. (2019). Magnesium-enriched microenvironment promotes odontogenic differentiation in human dental pulp stem cells by activating ERK/BMP2/Smads signaling. Stem Cell Res. Ther. 10 (1), 378. doi:10.1186/s13287-019-1493-5
Kornsuthisopon C., Chansaenroj A., Manokawinchoke J., Tompkins K. A., Pirarat N., Osathanon T. (2022). Non-canonical Wnt signaling participates in Jagged1-induced osteo/odontogenic differentiation in human dental pulp stem cells. Sci. Rep. 12 (1), 7583. doi:10.1038/s41598-022-11596-9
Kuehnle I., Goodell M. A. (2002). The therapeutic potential of stem cells from adults. BMJ 325 (7360), 372–376. doi:10.1136/bmj.325.7360.372
Lai E. C. (2004). Notch signaling: control of cell communication and cell fate. Development 131 (5), 965–973. doi:10.1242/dev.01074
Lei T., Zhang X., Du H. (2021). Characteristics, classification, and application of stem cells derived from human teeth. Stem Cells Int. 2021, 8886854. doi:10.1155/2021/8886854
Li B., Ouchi T., Cao Y., Zhao Z., Men Y. (2021). Dental-Derived mesenchymal stem cells: state of the art. Front. Cell Dev. Biol. 9, 654559. doi:10.3389/fcell.2021.654559
Li J., Wang Z., Wang J., Guo Q., Fu Y., Dai Z., et al. (2022a). Amphiregulin regulates odontogenic differentiation of dental pulp stem cells by activation of mitogen-activated protein kinase and the phosphatidylinositol 3-kinase signaling pathways. Stem Cell Res. Ther. 13 (1), 304. doi:10.1186/s13287-022-02971-4
Li M., Wang Y., Xue J., Xu Q., Zhang Y., Liu J., et al. (2023). Baicalin can enhance odonto/osteogenic differentiation of inflammatory dental pulp stem cells by inhibiting the NF-κB and β-catenin/Wnt signaling pathways. Mol. Biol. Rep. 50 (5), 4435–4446. doi:10.1007/s11033-023-08398-1
Li R., Wang C., Tong J., Su Y., Lin Y., Zhou X., et al. (2014). WNT6 promotes the migration and differentiation of human dental pulp cells partly through c-Jun N-terminal kinase signaling pathway. J. Endod. 40 (7), 943–948. doi:10.1016/j.joen.2013.12.023
Li S., Hu J., Zhang G., Qi W., Zhang P., Li P., et al. (2015). Extracellular Ca2+ promotes odontoblastic differentiation of dental pulp stem cells via BMP2-mediated smad1/5/8 and erk1/2 pathways. J. Cell Physiol. 230 (9), 2164–2173. doi:10.1002/jcp.24945
Li X., Yu Z., Jiang S., Dai X., Wang G., Wang Y., et al. (2022b). An amelogenin-based peptide hydrogel promoted the odontogenic differentiation of human dental pulp cells. Regen. Biomater. 9, rbac039. doi:10.1093/rb/rbac039
Liang C., Liao L., Tian W. (2021). Stem cell-based dental pulp regeneration: insights from signaling pathways. Stem Cell Rev. Rep. 17 (4), 1251–1263. doi:10.1007/s12015-020-10117-3
Liao J., Wei Q., Zou Y., Fan J., Song D., Cui J., et al. (2017). Notch signaling augments BMP9-induced bone formation by promoting the osteogenesis-angiogenesis coupling process in mesenchymal stem cells (MSCs). Cell Physiol. Biochem. 41 (5), 1905–1923. doi:10.1159/000471945
Lim W. H., Liu B., Cheng D., Hunter D. J., Zhong Z., Ramos D. M., et al. (2014). Wnt signaling regulates pulp volume and dentin thickness. J. Bone Min. Res. 29 (4), 892–901. doi:10.1002/jbmr.2088
Lin L., Zheng Y., Wang C., Li P., Xu D., Zhao W. (2023). Concentration-dependent cellular uptake of graphene oxide quantum dots promotes the odontoblastic differentiation of dental pulp cells via the AMPK/mTOR pathway. ACS Omega 8 (6), 5393–5405. doi:10.1021/acsomega.2c06508
Lin Z. M., Qin W., Zhang N. H., Xiao L., Ling J. Q. (2007). Adenovirus-mediated recombinant human bone morphogenetic protein-7 expression promotes differentiation of human dental pulp cells. J. Endod. 33 (8), 930–935. doi:10.1016/j.joen.2007.03.010
Liu C. H., Hung C. J., Huang T. H., Lin C. C., Kao C. T., Shie M. Y. (2014). Odontogenic differentiation of human dental pulp cells by calcium silicate materials stimulating via FGFR/ERK signaling pathway. Mater Sci. Eng. C Mater Biol. Appl. 43, 359–366. doi:10.1016/j.msec.2014.06.025
Liu J., Yu F., Sun Y., Jiang B., Zhang W., Yang J., et al. (2015). Concise reviews: characteristics and potential applications of human dental tissue-derived mesenchymal stem cells. Stem Cells 33 (3), 627–638. doi:10.1002/stem.1909
Lough D. M., Chambers C., Germann G., Bueno R., Reichensperger J., Swanson E., et al. (2016). Regulation of adsc osteoinductive potential using notch pathway inhibition and gene rescue: a potential on/off switch for clinical applications in bone formation and reconstructive efforts. Plast. Reconstr. Surg. 138 (4), 642e–652e. doi:10.1097/PRS.0000000000002551
Lowery J. W., Rosen V. (2018). The BMP pathway and its inhibitors in the skeleton. Physiol. Rev. 98 (4), 2431–2452. doi:10.1152/physrev.00028.2017
Lu X., Chen X., Xing J., Lian M., Huang D., Lu Y., et al. (2019). miR-140-5p regulates the odontoblastic differentiation of dental pulp stem cells via the Wnt1/β-catenin signaling pathway. Stem Cell Res. Ther. 10 (1), 226. doi:10.1186/s13287-019-1344-4
Luo Z., Kohli M. R., Yu Q., Kim S., Qu T., He W. X. (2014). Biodentine induces human dental pulp stem cell differentiation through mitogen-activated protein kinase and calcium-/calmodulin-dependent protein kinase II pathways. J. Endod. 40 (7), 937–942. doi:10.1016/j.joen.2013.11.022
Lv T., Wu Y., Mu C., Liu G., Yan M., Xu X., et al. (2016). Insulin-like growth factor 1 promotes the proliferation and committed differentiation of human dental pulp stem cells through MAPK pathways. Arch. Oral Biol. 72, 116–123. doi:10.1016/j.archoralbio.2016.08.011
Ma D., Gao J., Yue J., Yan W., Fang F., Wu B. (2012). Changes in proliferation and osteogenic differentiation of stem cells from deep caries in vitro. J. Endod. 38 (6), 796–802. doi:10.1016/j.joen.2012.02.014
Manokawinchoke J., Nattasit P., Thongngam T., Pavasant P., Tompkins K. A., Egusa H., et al. (2017). Indirect immobilized Jagged1 suppresses cell cycle progression and induces odonto/osteogenic differentiation in human dental pulp cells. Sci. Rep. 7 (1), 10124. doi:10.1038/s41598-017-10638-x
Marchionni C., Bonsi L., Alviano F., Lanzoni G., Di Tullio A., Costa R., et al. (2009). Angiogenic potential of human dental pulp stromal (stem) cells. Int. J. Immunopathol. Pharmacol. 22 (3), 699–706. doi:10.1177/039463200902200315
Mitsiadis T. A., Henrique D., Thesleff I., Lendahl U. (1997). Mouse Serrate-1 (Jagged-1): expression in the developing tooth is regulated by epithelial-mesenchymal interactions and fibroblast growth factor-4. Development 124 (8), 1473–1483. doi:10.1242/dev.124.8.1473
Mitsiadis T. A., Hirsinger E., Lendahl U., Goridis C. (1998). Delta-notch signaling in odontogenesis: correlation with cytodifferentiation and evidence for feedback regulation. Dev. Biol. 204 (2), 420–431. doi:10.1006/dbio.1998.9092
Moioli E. K., Clark P. A., Xin X., Lal S., Mao J. J. (2007). Matrices and scaffolds for drug delivery in dental, oral and craniofacial tissue engineering. Adv. Drug Deliv. Rev. 59 (4-5), 308–324. doi:10.1016/j.addr.2007.03.019
Monroe D. G., McGee-Lawrence M. E., Oursler M. J., Westendorf J. J. (2012). Update on Wnt signaling in bone cell biology and bone disease. Gene 492 (1), 1–18. doi:10.1016/j.gene.2011.10.044
Nakashima M., Reddi A. H. (2003). The application of bone morphogenetic proteins to dental tissue engineering. Nat. Biotechnol. 21 (9), 1025–1032. doi:10.1038/nbt864
Nandagopal N., Santat L. A., LeBon L., Sprinzak D., Bronner M. E., Elowitz M. B. (2018). Dynamic ligand discrimination in the notch signaling pathway. Cell 172 (4), 869–880. doi:10.1016/j.cell.2018.01.002
Ngo V. A., Jung J. Y., Koh J. T., Oh W. M., Hwang Y. C., Lee B. N. (2018). Leptin induces odontogenic differentiation and angiogenesis in human dental pulp cells via activation of the mitogen-activated protein kinase signaling pathway. J. Endod. 44 (4), 585–591. doi:10.1016/j.joen.2017.11.018
Nuti N., Corallo C., Chan B. M., Ferrari M., Gerami-Naini B. (2016). Multipotent differentiation of human dental pulp stem cells: a literature review. Stem Cell Rev. Rep. 12 (5), 511–523. doi:10.1007/s12015-016-9661-9
Oviir T. (2005). Outcome of the root canal treatment on permanent teeth is related to the preoperative diagnosis and the accuracy of the treatment procedure. J. Evid. Based Dent. Pract. 5 (1), 26–28. doi:10.1016/j.jebdp.2005.01.009
Park S. Y., Cho H. S., Chung K. H., Lee B. N., Kim S. H., Kim W. J., et al. (2022). Inactivation of PI3K/Akt promotes the odontoblastic differentiation and suppresses the stemness with autophagic flux in dental pulp cells. J. Dent. Sci. 17 (1), 145–154. doi:10.1016/j.jds.2021.05.013
Pearson G., Robinson F., Beers Gibson T., Xu B. E., Karandikar M., Berman K., et al. (2001). Mitogen-activated protein (MAP) kinase pathways: regulation and physiological functions. Endocr. Rev. 22 (2), 153–183. doi:10.1210/edrv.22.2.0428
Pei F., Wang H. S., Chen Z., Zhang L. (2016). Autophagy regulates odontoblast differentiation by suppressing NF-κB activation in an inflammatory environment. Cell Death Dis. 7 (3), e2122. doi:10.1038/cddis.2015.397
Qin W., Lin Z. M., Deng R., Li D. D., Song Z., Tian Y. G., et al. (2012a). p38a MAPK is involved in BMP-2-induced odontoblastic differentiation of human dental pulp cells. Int. Endod. J. 45 (3), 224–233. doi:10.1111/j.1365-2591.2011.01965.x
Qin W., Yang F., Deng R., Li D., Song Z., Tian Y., et al. (2012b). Smad 1/5 is involved in bone morphogenetic protein-2-induced odontoblastic differentiation in human dental pulp cells. J. Endod. 38 (1), 66–71. doi:10.1016/j.joen.2011.09.025
Rahman S. U., Oh J. H., Cho Y. D., Chung S. H., Lee G., Baek J. H., et al. (2018). Fibrous topography-potentiated canonical wnt signaling directs the odontoblastic differentiation of dental pulp-derived stem cells. ACS Appl. Mater Interfaces 10 (21), 17526–17541. doi:10.1021/acsami.7b19782
Rao T. P., Kuhl M. (2010). An updated overview on Wnt signaling pathways: a prelude for more. Circ. Res. 106 (12), 1798–1806. doi:10.1161/CIRCRESAHA.110.219840
Rodriguez-Carballo E., Gamez B., Ventura F. (2016). p38 MAPK signaling in osteoblast differentiation. Front. Cell Dev. Biol. 4, 40. doi:10.3389/fcell.2016.00040
Ross D. A., Kadesch T. (2001). The notch intracellular domain can function as a coactivator for LEF-1. Mol. Cell Biol. 21 (22), 7537–7544. doi:10.1128/MCB.21.22.7537-7544.2001
Saito T., Ogawa M., Hata Y., Bessho K. (2004). Acceleration effect of human recombinant bone morphogenetic protein-2 on differentiation of human pulp cells into odontoblasts. J. Endod. 30 (4), 205–208. doi:10.1097/00004770-200404000-00005
Salazar V. S., Gamer L. W., Rosen V. (2016). BMP signalling in skeletal development, disease and repair. Nat. Rev. Endocrinol. 12 (4), 203–221. doi:10.1038/nrendo.2016.12
Sanz J. L., Rodriguez-Lozano F. J., Lopez-Gines C., Monleon D., Llena C., Forner L. (2021). Dental stem cell signaling pathway activation in response to hydraulic calcium silicate-based endodontic cements: a systematic review of in vitro studies. Dent. Mater 37 (4), e256–e268. doi:10.1016/j.dental.2021.01.025
Scheller E. L., Chang J., Wang C. Y. (2008). Wnt/beta-catenin inhibits dental pulp stem cell differentiation. J. Dent. Res. 87 (2), 126–130. doi:10.1177/154405910808700206
Song B., Jiang W., Alraies A., Liu Q., Gudla V., Oni J., et al. (2016). Bladder smooth muscle cells differentiation from dental pulp stem cells: future potential for bladder tissue engineering. Stem Cells Int. 2016, 6979368. doi:10.1155/2016/6979368
Su Y., Zong S., Wei C., Song F., Feng H., Qin A., et al. (2019). Salidroside promotes rat spinal cord injury recovery by inhibiting inflammatory cytokine expression and NF-κB and MAPK signaling pathways. J. Cell Physiol. 234 (8), 14259–14269. doi:10.1002/jcp.28124
Sun S. C. (2011). Non-canonical NF-κB signaling pathway. Cell Res. 21 (1), 71–85. doi:10.1038/cr.2010.177
Sun S. C. (2017). The non-canonical NF-κB pathway in immunity and inflammation. Nat. Rev. Immunol. 17 (9), 545–558. doi:10.1038/nri.2017.52
Sun Y., Liu W. Z., Liu T., Feng X., Yang N., Zhou H. F. (2015). Signaling pathway of MAPK/ERK in cell proliferation, differentiation, migration, senescence and apoptosis. J. Recept Signal Transduct. Res. 35 (6), 600–604. doi:10.3109/10799893.2015.1030412
Teufel S., Hartmann C. (2019). Wnt-signaling in skeletal development. Curr. Top. Dev. Biol. 133, 235–279. doi:10.1016/bs.ctdb.2018.11.010
Tian Y., Xu Y., Xue T., Chen L., Shi B., Shu B., et al. (2017). Notch activation enhances mesenchymal stem cell sheet osteogenic potential by inhibition of cellular senescence. Cell Death Dis. 8 (2), e2595. doi:10.1038/cddis.2017.2
Uribe-Etxebarria V., Agliano A., Unda F., Ibarretxe G. (2019). Wnt signaling reprograms metabolism in dental pulp stem cells. J. Cell Physiol. 234 (8), 13068–13082. doi:10.1002/jcp.27977
Uribe-Etxebarria V., Luzuriaga J., Garcia-Gallastegui P., Agliano A., Unda F., Ibarretxe G. (2017). Notch/Wnt cross-signalling regulates stemness of dental pulp stem cells through expression of neural crest and core pluripotency factors. Eur. Cell Mater 34, 249–270. doi:10.22203/eCM.v034a16
Vijaykumar A., Root S. H., Mina M. (2021). Wnt/β-Catenin signaling promotes the formation of preodontoblasts in vitro. J. Dent. Res. 100 (4), 387–396. doi:10.1177/0022034520967353
Wu Q., Li J., Song P., Chen J., Xu Y., Qi S., et al. (2019b). Knockdown of NRAGE induces odontogenic differentiation by activating NF-κB signaling in mouse odontoblast-like cells. Connect. Tissue Res. 60 (2), 71–84. doi:10.1080/03008207.2018.1439484
Wang F. M., Hu T., Tan H., Zhou X. D. (2006). p38 Mitogen-activated protein kinase affects transforming growth factor-beta/Smad signaling in human dental pulp cells. Mol. Cell Biochem. 291 (1-2), 49–54. doi:10.1007/s11010-006-9193-8
Wang X., He F., Tan Y., Tian W., Qiu S. (2011). Inhibition of Delta1 promotes differentiation of odontoblasts and inhibits proliferation of human dental pulp stem cell in vitro. Arch. Oral Biol. 56 (9), 837–845. doi:10.1016/j.archoralbio.2011.02.006
Wang Y., Yan M., Yu Y., Wu J., Yu J., Fan Z. (2013). Estrogen deficiency inhibits the odonto/osteogenic differentiation of dental pulp stem cells via activation of the NF-κB pathway. Cell Tissue Res. 352 (3), 551–559. doi:10.1007/s00441-013-1604-z
Woo S. M., Kim W. J., Lim H. S., Choi N. K., Kim S. H., Kim S. M., et al. (2016). Combination of mineral trioxide aggregate and platelet-rich fibrin promotes the odontoblastic differentiation and mineralization of human dental pulp cells via BMP/Smad signaling pathway. J. Endod. 42 (1), 82–88. doi:10.1016/j.joen.2015.06.019
Wu J., Li N., Fan Y., Wang Y., Gu Y., Li Z., et al. (2019a). The conditioned medium of calcined tooth powder promotes the osteogenic and odontogenic differentiation of human dental pulp stem cells via MAPK signaling pathways. Stem Cells Int. 2019, 4793518. doi:10.1155/2019/4793518
Wu M., Chen G., Li Y. P. (2016). TGF-beta and BMP signaling in osteoblast, skeletal development, and bone formation, homeostasis and disease. Bone Res. 4, 16009. doi:10.1038/boneres.2016.9
Xin T., Li Q., Bai R., Zhang T., Zhou Y., Zhang Y., et al. (2021). A novel mutation of SATB2 inhibits odontogenesis of human dental pulp stem cells through Wnt/β-catenin signaling pathway. Stem Cell Res. Ther. 12 (1), 595. doi:10.1186/s13287-021-02660-8
Xu S., Xie X., Li C., Liu Z., Zuo D. (2021). Micromolar sodium fluoride promotes osteo/odontogenic differentiation in dental pulp stem cells by inhibiting PI3K/AKT pathway. Arch. Oral Biol. 131, 105265. doi:10.1016/j.archoralbio.2021.105265
Xuan K., Li B., Guo H., Sun W., Kou X., He X., et al. (2018). Deciduous autologous tooth stem cells regenerate dental pulp after implantation into injured teeth. Sci. Transl. Med. 10 (455), eaaf3227. doi:10.1126/scitranslmed.aaf3227
Yamamoto H., Cho S. W., Kim E. J., Kim J. Y., Fujiwara N., Jung H. S. (2004). Developmental properties of the Hertwig's epithelial root sheath in mice. J. Dent. Res. 83 (9), 688–692. doi:10.1177/154405910408300906
Yamashiro T., Zheng L., Shitaku Y., Saito M., Tsubakimoto T., Takada K., et al. (2007). Wnt10a regulates dentin sialophosphoprotein mRNA expression and possibly links odontoblast differentiation and tooth morphogenesis. Differentiation 75 (5), 452–462. doi:10.1111/j.1432-0436.2006.00150.x
Yang J., Ye L., Hui T. Q., Yang D. M., Huang D. M., Zhou X. D., et al. (2015). Bone morphogenetic protein 2-induced human dental pulp cell differentiation involves p38 mitogen-activated protein kinase-activated canonical WNT pathway. Int. J. Oral Sci. 7 (2), 95–102. doi:10.1038/ijos.2015.7
Yang W., Harris M. A., Cui Y., Mishina Y., Harris S. E., Gluhak-Heinrich J. (2012). Bmp2 is required for odontoblast differentiation and pulp vasculogenesis. J. Dent. Res. 91 (1), 58–64. doi:10.1177/0022034511424409
Yokose S., Naka T. (2010). Lymphocyte enhancer-binding factor 1: an essential factor in odontoblastic differentiation of dental pulp cells enzymatically isolated from rat incisors. J. Bone Min. Metab. 28 (6), 650–658. doi:10.1007/s00774-010-0185-0
Yoshida S., Wada N., Hasegawa D., Miyaji H., Mitarai H., Tomokiyo A., et al. (2016). Semaphorin 3A induces odontoblastic phenotype in dental pulp stem cells. J. Dent. Res. 95 (11), 1282–1290. doi:10.1177/0022034516653085
Yu J. S., Cui W. (2016). Proliferation, survival and metabolism: the role of PI3K/AKT/mTOR signalling in pluripotency and cell fate determination. Development 143 (17), 3050–3060. doi:10.1242/dev.137075
Zhang C., Chang J., Sonoyama W., Shi S., Wang C. Y. (2008). Inhibition of human dental pulp stem cell differentiation by Notch signaling. J. Dent. Res. 87 (3), 250–255. doi:10.1177/154405910808700312
Zhang M., Zhang S. (2022). Mitogen-activated protein kinase cascades in plant signaling. J. Integr. Plant Biol. 64 (2), 301–341. doi:10.1111/jipb.13215
Zhang Z., Guo Q., Tian H., Lv P., Zhou C., Gao X. (2014). Effects of WNT10A on proliferation and differentiation of human dental pulp cells. J. Endod. 40 (10), 1593–1599. doi:10.1016/j.joen.2014.07.009
Zhao X., He W., Song Z., Tong Z., Li S., Ni L. (2012). Mineral trioxide aggregate promotes odontoblastic differentiation via mitogen-activated protein kinase pathway in human dental pulp stem cells. Mol. Biol. Rep. 39 (1), 215–220. doi:10.1007/s11033-011-0728-z
Zhu L., Ma J., Mu R., Zhu R., Chen F., Wei X., et al. (2018). Bone morphogenetic protein 7 promotes odontogenic differentiation of dental pulp stem cells in vitro. Life Sci. 202, 175–181. doi:10.1016/j.lfs.2018.03.026
Abbreviations
DPSCs, Dental pulp stem cells MSCs, Mesenchymal stem cells APC, Adenomatous polyposis coli GSK-3β, Glycogen synthase kinase-3β Ck1, Casein kinase 1 LRP5/6, Low-density lipoprotein receptor-related proteins 5 and 6 PCP, Planar cell polarity Runx2, Runt-related transcription factor 2 SATB2, Special AT-rich sequence-binding protein 2 OPN, Osteopontin ALP, Alkaline phosphatase DKK1, Dickkopf-1 DANCR, Differentiation antagonizing non-protein coding RNA DSPP, Dentin sialophosphoprotein DMP-1, Dentin matrix protein 1 OCN, Osteocalcin COL1A1, Collagen-type I-alpha1 TGF-β, Transforming growth factor-β BMP, Bone morphogenetic protein MAPK, Mitogen-activated protein kinase MAPKK or MEK, MAPK kinase MAPKKK or MEKK, MAPK kinase kinase ERK, Extracellular signal-regulated kinase JNKMAPK, c-Jun N-terminal kinase NF-kB, Nuclear factor-kappa B TAK1, TGFβ-activated kinase 1 IKKβ, IκB kinase-β IKK, IκB kinase TNFR, Tumor necrosis factor receptor NIK, NFκB-inducing kinase OVX, Ovariectomy Sham DPSCs, Sham-operated group IL-1β, Interleukin-1β IL-6, Interleukin-6 PI3K/AKT/mTOR, Phosphoinositide-3-kinase/protein kinase B/mammalian target of rapamycin RTK, Receptor tyrosine kinase PDK1, PI3K-dependent kinase 1 GOQDs, Graphene oxide quantum dots SCAP, Stem cells from apical papilla NICD, Notch intracellular domain DSP, Dentin sialoprotein IFN-γ, Inflammatory cytokine.
Keywords: dental pulp stem cell, odontogenic differentiation, signaling pathway, pulp–dentin complex regeneration, tissue engineering, review
Citation: Zhou L, Zhao S and Xing X (2023) Effects of different signaling pathways on odontogenic differentiation of dental pulp stem cells: a review. Front. Physiol. 14:1272764. doi: 10.3389/fphys.2023.1272764
Received: 04 August 2023; Accepted: 02 October 2023;
Published: 19 October 2023.
Edited by:
Waruna Dissanayaka, The University of Hong Kong, Hong Kong SAR, ChinaReviewed by:
Hua Zhang, Texas A&M University, United StatesWeiran Li, Peking University Hospital of Stomatology, China
Copyright © 2023 Zhou, Zhao and Xing. This is an open-access article distributed under the terms of the Creative Commons Attribution License (CC BY). The use, distribution or reproduction in other forums is permitted, provided the original author(s) and the copyright owner(s) are credited and that the original publication in this journal is cited, in accordance with accepted academic practice. No use, distribution or reproduction is permitted which does not comply with these terms.
*Correspondence: Xianghui Xing, dr.xing@qq.com
†These authors have contributed equally to this work