- Department of Cardiovascular Surgery, West China Hospital, Sichuan University, Chengdu, China
Having a bicuspid aortic valve (BAV) is the most common congenital heart disease, affecting 0.5%–2% of the population, with significant heterogeneity in clinical presentation, complications, and outcomes. Hemodynamic disturbances, including wall shear stress (WSS), eccentric flow, helical flow and energy turbulence, are critical in the development and progression of BAV-associated aortopathy, which is characterized by ascending aortic dilation, aortic aneurysm, and dissection. The interplay between genetic factors and hemodynamic abnormalities further complicates disease mechanisms, influencing clinical management and prognosis. To investigate the hemodynamic characteristics of BAV-associated aortic disease before and after surgery, this study reviewed recent advances in the understanding of the hemodynamic and genetic mechanisms underlying BAV-associated aortic disease, as well as clinical treatment strategies and recommendations for managing cases with additional genetic factors. This paper systematically summarizes the changes in hemodynamic parameters related to aortopathy in patients with BAV before and after surgery and their correlation with aortic dilation. This paper also explores the influence of different aortic valve morphotypes and functional phenotypes on hemodynamic parameters. Notably, this review focuses on the unique hemodynamic features of paediatric and young patients with BAVs and reviews clinical management recommendations for this group. The relationship between postoperative hemodynamic changes and clinical outcomes, such as redilation and long-term survival rates, warrants further exploration in BAV patients.
1 Introduction
Having a bicuspid aortic valve (BAV) is the most common congenital heart disease, with a prevalence of 0.5%–2%, and it occurs approximately three times more frequently in males (Michelena et al., 2008; Siu and Silversides, 2010; Kong et al., 2017b). The clinical manifestations, therapeutic approaches, complications, and prognosis of BAV are highly heterogeneous. The Sievers classification (Figure 1) is widely used to characterize BAV valve fusion morphophenotypes by counting raphes and assessing cusp and raphe fusion orientations (Sievers and Schmidtke, 2007). The phenotype-based nomenclature predicts complications and outcomes and guides follow-up strategies. For example, patients with right and noncoronary cusp fusion (RN) exhibit more severe aortic valve dysfunction and require earlier interventions. Patients with RN display greater ascending aortic (AAo) dilation than do those with right and left cusp fusion (RL), while RL is more commonly associated with aortic root dilation (Grattan et al., 2020). BAV manifests variably in children and adolescents, depending on onset age, prompting studies to classify it by clinical phenotype (Niaz et al., 2020). To standardize the terminology, an international consensus in 2021 proposed a new clinical classification system dividing patients with BAVs into three subgroups: (i) Complex valvulo-aortopathy, characterized by more severe clinical and pathological phenotypes, often associated with syndromic conditions. (ii) Typical valvulo-aortopathy, the most common subgroup, which includes progressive BAV dysfunction and/or aortic dilation without significant associated syndromic conditions. (iii) Uncomplicated or undiagnosed BAV, representing a lifelong asymptomatic condition associated with mild or nonprogressive valvular disease, with no distinctive clinical features (Michelena et al., 2021).
Patients with BAVs may present in various clinical scenarios, ranging from asymptomatic young children identified during routine examinations to elderly patients with end-stage disease and severe complications (Michelena et al., 2014; Michelena et al., 2018). Compared with patients with tricuspid aortic valves (TAVs) have a significantly greater cardiovascular risk (Mennander et al., 2020). The most common complications of BAVs are valvular dysfunctions such as aortic stenosis (AS) (14%–50%) and aortic regurgitation (AR) (23%–70%) which affect 50%–86% of cases and represent the primary indications for aortic valve surgery (Roberts and Ko, 2005; Michelena et al., 2011; Detaint et al., 2014; Kong et al., 2017a; Evangelista et al., 2018; Cheng et al., 2021; Yang et al., 2023). Aortic dilation occurs in more than 50% of BAV patients, but its prevalence varies widely across studies due to inconsistent definitions, particularly in paediatric and adolescent populations (Detaint et al., 2014; Evangelista et al., 2018; Yang et al., 2020). Progressive thoracic aortic dilation increases the risk of cardiovascular events such as aortic dissection or thoracic aortic aneurysm rupture, but the absolute incidence is relatively low (<1%) (Fedak et al., 2002; Yang et al., 2020). Additionally, infective endocarditis (∼2%), mitral valve prolapse (1.6%–2.7%), and heart failure (7% ± 2%) are notable complications (Michelena et al., 2008; Padang et al., 2019). In paediatric BAV patients, the primary complications are similar to those in adults (Michelena et al., 2008; Padang et al., 2019). Overall, aortic diameter and moderate-to-severe AS were significantly associated with all-cause mortality (Ye et al., 2023). Therefore, monitoring the diameter and progression of aortopathy is crucial for predicting clinical outcomes and assessing the risks of complications.
Transthoracic echocardiography (TTE) and transoesophageal echocardiography are commonly used to diagnose and classify BAV (Sievers and Schmidtke, 2007; Galian-Gay et al., 2020; Cramer and Prakash, 2019). However, relying solely on diameter or volume may not fully represent the progression of aortopathy, necessitating the incorporation of hemodynamic assessments. With advances in technology, new techniques such as ECG-gated CT and MRI angiography for observing valve morphology, 3D or 4D flow parameter reconstruction and artificial intelligence machine learning have been employed to analyse changes in aortic hemodynamics (Franco et al., 2022; Peper et al., 2022). These innovations provide novel predictive parameters for the clinical management and prognosis of aortic complications. Among the extensively studied parameters are the following: Jet angle: The angle of the jet relative to the central line. Normalized displacement: The distance between the lumen centre and velocity centre, typically standardized by the lumen diameter. Wall shear stress (WSS): The tangential force per unit area exerted on the aortic wall. Rotational/Helical flow: The integral of vorticity across the cross-sectional area. Retrograde flow: Reverse flow occurring along the longitudinal axis of the lumen during systole. Turbulent kinetic energy (TKE): The intensity of velocity fluctuations due to turbulence (Figure 2) (Bissell et al., 2023).
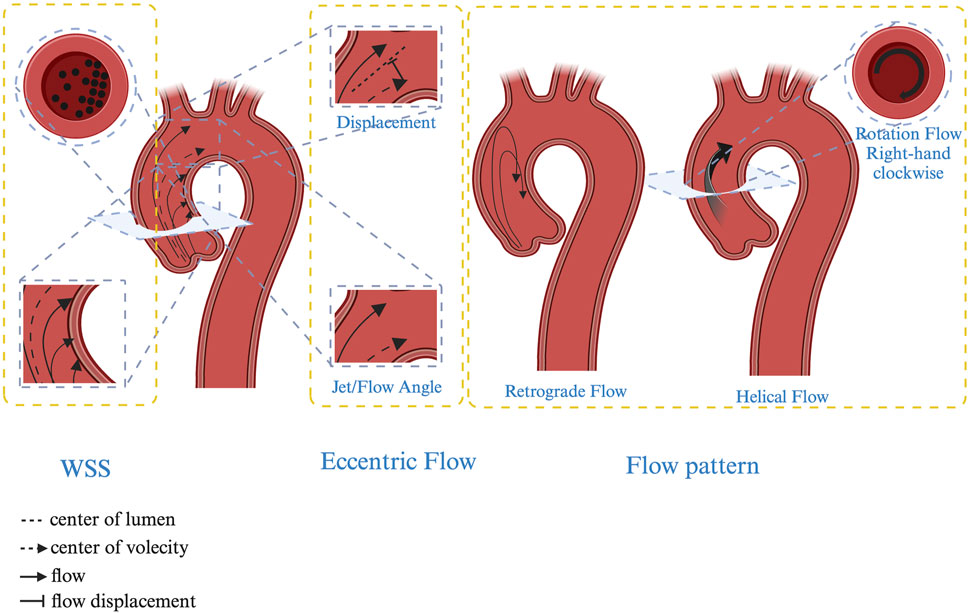
Figure 2. Hemodynamic characteristics in BAV patients. Jet/Flow angle: The angle of the jet relative to the central line; Displacement: The distance between the lumen centre and velocity centre, typically standardized by the lumen diameter. Wall shear stress (WSS): The tangential force per unit area exerted on the aortic wall. Rotational/Helical flow: The integral of vorticity across the cross-sectional area. Retrograde flow: Reverse flow occurring along the longitudinal axis of the lumen during systole.
Aortic dilation typically begins in childhood and progresses gradually, with the incidence of ascending aortic dilation increasing with age. It is currently believed that aortopathy in BAV patients may be caused by genetic defects in the vascular wall, hemodynamic changes, or a combination of both factors, which remains a subject of significant discussion and debate (Figure 3). (Mahadevia et al., 2014; Guala et al., 2022; Minderhoud et al., 2022; Soulat et al., 2022).
2 Aortopathy
Aortopathy, including aortic dilation, aortic aneurysm, and aortic dissection, is an ongoing process in BAV patients. The definition of aortic aneurysm in the aortic root and ascending aorta differs from that of aneurysms in other parts of the aorta, where an aneurysm is typically defined as having a diameter greater than 1.5 times the average size. However, aneurysms that occur in these regions are much smaller than this threshold (Johnston et al., 1991; Isselbacher et al., 2022). Many studies suggest that, in comparison with the general population, the risk of aortic aneurysm or dissection begins to rise when the ascending aorta diameter exceeds 40 mm, with a significant increase in risk at 45 mm, particularly for BAV patients (Borger et al., 2004; Paruchuri et al., 2015). Therefore, defining aortic dilation as a diameter greater than 40 mm is reasonable, whereas 45 mm is defined as the threshold for an aortic aneurysm (Isselbacher et al., 2022). The diameter of the aorta is influenced by various factors, including age, sex, body size, genetic predisposition, comorbidities, measurement site, and accuracy (Hiratzka et al., 2010; Devereux et al., 2012; Donadei et al., 2019; Modica et al., 2022; Keuning et al., 2024).
Defects in vascular wall integrity have been implicated as a pathophysiological mechanism in BAV-associated aortopathy. Complex inflammatory reactions and tissue changes, such as fragmentation of elastic fibres, release of matrix metalloproteinases, and structural alterations in vascular smooth muscle cells within the tunica media, may lead to progressive cystic medial necrosis and dysfunction of the aortic media (Nataatmadja et al., 2003; Pisano et al., 2012).
2.1 Patterns of aortopathy and relationships with BAV morphotypes
Aortopathy affects all segments of the aorta, from the root to the mid-segment of the proximal aortic arch. In the 2022 guidelines, dilation phenotypes were redefined as the ascending phenotype, extending phenotype, and root phenotype (Figure 1). Ascending phenotype: Features of tubular dilation of the AAo, especially along its curvature, are often accompanied by varying degrees of root dilation, which is associated with increased age at diagnosis (>50 years) and AS. Extending phenotype: This phenotype involves dilation of the root plus the ascending aorta and the AAo plus the arch. Root phenotype: This refers to isolated dilation of the aortic root, a rare phenotype associated with younger age at diagnosis (<40 years), male sex, and AR (Michelena et al., 2021; Isselbacher et al., 2022). Approximately 10%–30% of BAV patients exhibit root dilation, whereas AAo dilation is observed in 55%–80% of BAV patients (Galian-Gay et al., 2019; Rodríguez-Palomares et al., 2023). Overall, the root phenotype is an independent predictor of AAo diameter growth, whereas the ascending phenotype is a stable entity with slower progression (Della Corte et al., 2013). Compared with other phenotypes, the pattern of aortopathy strongly correlates with the BAV morphotype BAV-RN (right and noncoronary cusp fusion), which is more strongly associated with and significantly higher rates of the ascending and extending phenotype. BAV-LN (left and noncoronary cusp fusion): This fusion is more frequently linked to an extended phenotype. BAV-RL (right and left cusp fusion): This fusion is more frequently linked to the root phenotype. For aortic diameter, no significant differences were observed between BAV morphotypes in AAo. However, in other regions, such as the aortic root, the diameter of BAV-RL was significantly greater than that of BAV-RN, whereas it was comparable to that of BAV-RL at the aortic root. Aortic arch: BAV-RN had a significantly larger arch diameter than did BAV-RL, with BAV-RN and BAV-LN showing similar arch diameters (Kang et al., 2013; Ruzmetov et al., 2015; Evangelista et al., 2018).
2.2 Genetic mechanisms of aortopathy in BAV
BAV primarily follows autosomal dominant or X-linked inheritance with familial clustering, resulting in incomplete penetrance and variable expressivity. On the one hand, BAV is often associated with complex congenital diseases such as Turner syndrome, aortic coarctation, and connective tissue disorders. On the other hand, genetic studies of BAV have revealed the interplay of several genes. For example, defects in the Jagged 1/Notch1 pathway (e.g., unbalanced translocation) can disrupt epithelial‒mesenchymal transition (EMT), cardiac neural crest cell (CNC) migration, and mesenchymal filling. Similarly, defects in GATA4/5/6, which regulate CNCs and directly contribute to aortic valve formation, play a role in BAV development (Bravo-Jaimes and Prakash, 2020; Ma et al., 2021). During cardiac development, the formation of a BAV likely occurs in three key stages: endocardial cushion formation, outflow tract (OFT) septation, and valve cushion invagination. Typically, four endocardial cushions are formed; however, during BAV development, only three cushions are created, combined with typical OFT septation, resulting in the formation of RN and LN. Abnormalities in EMT and CNC migration are thought to be the primary drivers of irregular endocardial cushion formation leading to RN or LN. Conversely, BAV-RL is hypothesized to result from abnormal or excessive fusion during embryonic OFT septation (Anderson et al., 2003; Laforest and Nemer, 2012; Soto-Navarrete et al., 2020).
The genetic correlation between BAV and thoracic aortic dilatation can be observed in the following syndromes. BAV occurs in more than 30% of Turner syndrome patients, with a significantly greater prevalence of aortic aneurysm and aortic dissection than nonsyndromic BAV patients, especially in females (Lin et al., 2021; Galian-Gay and Rodriguez-Palomares, 2022; Thunström et al., 2023). Patients with Loeys–Dietz syndrome, which involves pathogenic mutations in TGFBR1/2, often experience proximal aortic dilation and dissection at a younger age and have smaller diameters compared to those with non-complex BAVs (Loeys et al., 2005; Andelfinger et al., 2016). Marfan syndrome, characterized by connective tissue abnormalities, has an earlier onset and larger aortic aneurysms than in nonsyndromic BAV patients, particularly those involving root dilation (Sherrah et al., 2016; Yassine et al., 2017).
In a meta-analysis, the prevalence of aortic dilation among relatives of patients with BAV was found to be 29.2%, which is far higher than the 9.6% reported in relatives of patients with TAV in multicentre cohort studies (Galian-Gay et al., 2019; Bray et al., 2023). Even among relatives of patients with BAV with TAV, the overall incidence of aortic dilation was greater at approximately 10%, with a root phenotype of 3% and an ascending phenotype of 7% (Bray et al., 2023). A large-scale population-based study demonstrated that the risk of diagnosed aortic dilation in first-degree relatives of patients with BAV was 6.88 times greater and that the risk of aortic dissection was 3.63 times greater than that in the general population, which further supports a genetic association of aortopathy with BAV (Glotzbach et al., 2023). Asymptomatic thoracic aortic dilation observed in children or young adults can validate the genetic susceptibility of BAV-associated aortic dilation (Yang et al., 2020).
In addition, the genetic contributors to aortopathy associated with BAV likely involve the combined effects of abnormalities in aortic dilation-related genes, which are categorized as follows: 1. Extracellular matrix-related genes including proteoglycan-related genes (BGN, ACAN), collagen-related genes (COL1A1, COL1A2, and COL3A1), elastin-related genes (EFEMP2, ELN, FBN1, and FBN2), etc. 2. Vascular smooth muscle-related genes such as contractile protein-related genes (ACTA2), myosin-related genes (MYH11), smooth muscle-related genes (FOXE3, MAT2A, MYLK, and PRKG1), etc. 3. TGF-B signalling pathway genes such as FBN1, NOTCH1, SKI, SLC2A10, SMAD2, SMAD3, SMAD4, TGFB2, TGFB3, TGFBR1, TGFBR2, etc. (Milewicz et al., 2008; Yassine et al., 2017; Du et al., 2021).
2.3 Hemodynamic factors
Hemodynamic analyses using 3D or 4D MRI have revealed that long-term valvular abnormity leads to hemodynamic disturbances and altered aortic flow patterns. These include increased eccentric flow, flow velocity, eccentric jets, altered flow patterns, helical flow, systolic retrograde flow, viscous energy loss, and abnormal wall shear stress. As patients with BAV age, complication rates increase, with the onset of valvular dysfunction, particularly AS or moderate-to-severe AR, identified as independent predictors of aortic disease (Evangelista et al., 2018; Avila-Vanzzini et al., 2022). Over time, these disturbances cause thinning of the elastic fibres in the aortic wall, extracellular matrix (ECM) dysregulation, vascular wall remodelling, and the induction of atherosclerotic plaque formation. These processes promote aortic dilation and aneurysm generation, ultimately resulting in aortopathy (Bollache et al., 2018b).
There is no doubt that the biomechanical effects generated by hemodynamics play a crucial role in the pathological progression of aortic disease. As endothelial cells capable of sensing mechanical forces, they detect different characteristics of flow profiles and accordingly regulate vascular physiology and vascular remodeling. Although endothelial dysfunction and genetic susceptibility are significantly correlated, numerous studies indicate that pathological blood flow effects should not be overlooked. Endothelial cells have a variety of mechanical receptors, which can perceive alterations in blood flow hemodynamics and produce different biological responses. It has been demonstrated the inducible tyrosine phosphorylation of PECAM-1 and indicated that PECAM-1 and Src family kinases are involved in sensing/signal transduction of mechanical stimuli in endothelial cells (Osawa et al., 1997; Conway et al., 2013). Blood flow increases tension of PECAM-1, triggering the association of this protein with vimentin cytoskeleton (Conway et al., 2013). Tension on PECAM-1 triggers activation of a Src family kinase, resulting in ligand-independent transactivation of VEGF receptors and subsequent activation of PI3K, endothelial NOS, and production of nitric oxide (NO) to induce vasodilation (Fleming et al., 2005; Baeyens et al., 2016). Especially, the WSS-induced endothelial dysfunction leads to a dose-dependent secretion of NO and prostacyclin, which relaxes smooth muscle and reduces vascular tone, disrupting the homeostasis of hemodynamic mechanisms. This also induces the abnormal dose-dependent expression of the transcription factor Krüppel-like factor 2/4, increasing the expression of growth factors, oxidative elements, vasoconstrictors, acute inflammatory mediators, and proteolytic enzymes, exacerbating acute inflammation. The persistence of this process ultimately leads to arterial remodeling (Nigro et al., 2011; Baeyens et al., 2016; He et al., 2019). Similarly, in atherosclerosis, endothelial-to-mesenchymal transition in response to hemodynamic-induced vascular remodeling leads to a reduction in the expression of endothelial-specific molecules, including VE-cadherin and PECAM-1, in order to balance the effects of pro-inflammatory factors (Chen et al., 2015). By modulating the extracellular matrix, it facilitates the progression of inflammation, such as the matricellular protein CCN1, which persistently promotes atherogenesis (Hsu et al., 2019; Chen et al., 2024). Furthermore, endothelial dysfunction can lead to dysregulate NO expression, aberrant NOTCH signaling, and increased calcium deposition, which collectively contribute to the development of calcific aortic valve disease. This calcification impairs valve function, resulting in AS or AR, which further exacerbates hemodynamic disturbances (Antequera-González et al., 2020). Recent studies also highlight the role of hemodynamic forces in inducing epigenetic modifications, with endothelial cells producing Mechano-microRNAs in response to WSS exposure, thereby intensifying arterial remodeling and promoting inflammatory responses (Rashad et al., 2020).
In response to altered hemodynamics, such as high or low WSS, cells can activate various signaling pathways (e.g., NOTCH and TGF-β), leading to changes in gene expression that influence vascular function (Milewicz et al., 2017; Baek et al., 2022). A basic research has found that changes in hemodynamics and increased WSS mediate the Notch-ephrinb2 pathway, promoting the formation of new arterial rings and restoring microcirculation (Baek et al., 2022). Numerous studies have shown that elevated WSS is closely associated with downstream molecules of TGF-β, such as MMPs, SMAD2/3, and SM22 alpha protein, indicating that hemodynamic signals activate the TGF-β signaling pathway, leading to changes in vascular elastin molecules and resulting in vascular remodeling (Guzzardi et al., 2015; Kunnen et al., 2017; Sophocleous et al., 2018).
3 Clinical management of BAV
The surgical treatment of aortopathy associated with BAV focuses on addressing the underlying causes of aortic valve dysfunction. Procedures include surgical aortic valve replacement (SAVR), transcatheter aortic valve replacement (TAVR) and BAV repair (Fraser et al., 1994; Ehrlich et al., 2020; Halim et al., 2020; Kalogeropoulos et al., 2022; Bavaria et al., 2024). For patients with aortic aneurysms, aortic dissection, aortic rupture, or those at high risk for cardiovascular events such as those mentioned previously, surgical or prophylactic interventions are recommended. These include ascending aorta replacement (AAR), aortic root replacement (ARR), valve-sparing aortic root replacement (VSARR), and Bentall root replacement (Sievers et al., 2014; Beckmann et al., 2020; Mokashi et al., 2022; Levine et al., 2023).
Recommendations from the 2022 Guidelines: Class I Recommendation: Surgery is advised for BAVs with an aortic root or ascending aortic diameter ≥55 mm, or both. Class IIa Recommendation: It is reasonable for experienced surgeons within a multidisciplinary aortic team to perform surgery for patients with aortic root or ascending aortic cross-sectional area-to-height ratios ≥10 cm2/m. For BAVs and aortic root or AAo diameters of 50–54 mm, along with additional risk factors for dissection, surgery is also considered reasonable. Concurrent AAR or ARR is advised in patients who undergo surgical aortic valve repair or replacement when the diameter reaches ≥45 mm. Class IIb Recommendation: For BAVs and aortic root or ascending aortic diameters of 50–54 mm without additional risk factors, surgery may be considered reasonable (Masri et al., 2017; Borger et al., 2018; Otto et al., 2021; Isselbacher et al., 2022).
Partial arch replacement is the most common surgical technique, with a lower incidence of concomitant cardiac procedures. The VSARR is increasingly used for BAVs with root dilation (Kayatta et al., 2019; Nguyen et al., 2021; Levine et al., 2023). Most patients do not reach the elective repair threshold of 55 mm, even in the presence of aortic dissection. Therefore, prophylactic aortic surgery should be considered on the basis of a comprehensive assessment. Given that familial thoracic aortic aneurysm patients often present dissection at younger ages with poorer outcomes and a greater likelihood of reintervention, prophylactic surgery is necessary when the maximum diameter of the root or ascending aorta reaches ≥50 mm (Brownstein et al., 2018; Saeyeldin et al., 2019). In a retrospective study, more than two-thirds of patients with BAV underwent prophylactic aortic root replacement during the perioperative period of aortic dissection, whereas this was the case for only 19% of patients with TAV (Kreibich et al., 2020).
The choice of aortic surgical intervention varies across different valve types. BAV type 2 valves are significantly more likely to require AAR at threshold diameters. BAV-RL cusp fusion and valvular dysfunction are associated with increased frequencies of AAR. Root interventions (Bentall, David, Yacoub, or other remodelling procedures) are more common in BAV-RL patients with AR than in those with associated AS (Sievers et al., 2014).
Age is an independent predictor of aortic pathology in patients with BAV and plays a crucial role in surgical decision-making, especially in paediatric patients. For paediatric patients with BAV, the treatment of aortic dilation is individualized. In children, the 2024 scientific statement suggests standardizing the measurement of body surface area-adjusted z scores to define aortic root and ascending aortic dilation, where dilation is classified as a z score >2 SD (Devereux et al., 2012; Grattan et al., 2020; Morris et al., 2024). Isolated dilation of the aortic root or ascending aorta is rarely an indication for surgery in children and adolescents (Niaz et al., 2020). The statement recommends tailoring surgical thresholds for the root or ascending aorta on the basis of genetic mutations and high-risk profiles (Hiratzka et al., 2010; Morris et al., 2024). For patients with heritable thoracic aortic disease (HTAD), elective surgery for root aneurysms should involve replacing the entire ascending aorta along with the root, and selective proximal arch replacement (partial arch replacement) may offer protection when performed by experienced centres (Malaisrie et al., 2015; Preventza et al., 2017). Total arch replacement is not recommended for isolated root aneurysms undergoing elective repair (Regalado et al., 2018). For paediatric patients with BAV, medical conservative treatment aims to slow the progression of aortic dilation. A retrospective study demonstrated that, compared with no treatment, treatment with losartan or atenolol resulted in slower aortic growth. Additionally, guidelines emphasize the management of activity and exercise in paediatric patients (Baleilevuka-Hart et al., 2020; Niaz et al., 2020; Flyer et al., 2021).
4 Hemodynamic characteristics in BAV patients
4.1 Wall shear stress
WSS is a widely studied hemodynamic parameter in BAV patients. The congenital anomaly of the BAV is thought to increase the WSS, exerting a vicious force on the aortic wall and increasing regional abnormalities. We summarized the distribution of abnormal WSS in different aortic regions on the basis of recent studies (Table 1). However, the BAV morphology, aortic diameter, and valvular dysfunction are all factors influencing hemodynamics. In patients with BAV, the presence of aortic dilation influences the WSS distribution, with greater wall stress at the aortic root and significant increases on the inner and right sides of the AAo and the outer and left sides of the arch, whereas those without dilation present significantly elevated WSS throughout most of the inner AAo and the entire arch (Dux-Santoy et al., 2020; Gomez et al., 2020). We hypothesize that long-term WSS abnormalities lead to compensatory aortic dilation to mitigate the effects of WSS.
Recent research involving 72 BAV patients by Soulat et al. further demonstrated that elevated WSS regions are predictive of an increased aortic expansion rates (Soulat et al., 2022). A prospective study demonstrated the critical role of the WSS and its circumferential component in predicting progressive aortic dilation (Guala et al., 2022). In addition to the WSS, derived parameters such as axial, circumferential, and magnitude WSS, and the oscillatory shear index (OSI), have shown wide value in hemodynamic analyses. Aortic diameter growth in patients with BAV is strongly correlated with the circumferential WSS and WSS angle, whereas the axial WSS and OSI behave in opposite directions, resulting in a decrease in areas of aortic expansion (Table 2) (Guala et al., 2022; Minderhoud et al., 2022; Soulat et al., 2022). Table 1 highlights that despite varying regional segmentation methods, WSS abnormalities and derived parameters are predominantly concentrated in the aortic root and proximal ascending aorta. JosΩ Fernando and Patrick Geeraert et al. reported that the circumferential WSS and WSS angle are strongly correlated with the root and ascending phenotypes, whereas the magnitude of the WSS and axial WSS are more strongly associated with the root phenotype (Rodríguez-Palomares et al., 2018; Geeraert et al., 2021b). Notably, WSS-derived parameters at the aortic arch level are less significant than those in the AAo are, particularly in the distal arch, where the WSS tends to decrease without notable differences compared with that of controls (Dux-Santoy et al., 2019).
Age is an independent factor for BAV-associated aortic events (Tzemos et al., 2008; Thompson et al., 2023). Following the initial diagnosis, temporal changes in the WSS are factors to be considered. The systolic peak WSS and the relative area of elevated WSS showed no significant changes over several scans, and patients with BAV with a low aortic growth rate presented favourable long-term stability across multiple imaging sessions (Maroun et al., 2024). In contrast, a 3-year follow-up study of young patients with BAV demonstrated increasing magnitude, axial, and circumferential WSS over time, regardless of aortic growth. Compared with that of controls, the circumferential WSS was greater across all ascending aorta regions, with the most significant WSS abnormalities occurring in the outer proximal AAo (Minderhoud et al., 2024).
In vascular biology studies, elevated WSS levels have been linked to increased nitric oxide production by endothelial cells, leading to vascular dilation and subsequent structural changes in the vessel wall. Hemodynamic changes due to valvular abnormalities result in WSS inversely correlated with elastin quantity, widening interstitial spaces, thinning fibrillin fibres, and increased matrix metalloproteinases, driving extracellular matrix (ECM) remodelling and ascending aortic wall structural changes (Guzzardi et al., 2015; Bollache et al., 2018b).
4.2 Eccentric blood flow
Previous studies have demonstrated that eccentric blood flow (quantified by normalized displacement and flow angle) is associated with accelerated aortic growth in patients with BAV, particularly in patients with the ascending aorta dilation phenotype (Bissell et al., 2013; Hope et al., 2014; Raghav et al., 2018; Rodríguez-Palomares et al., 2018). Eccentric flow can be characterized using the parameters of normalized displacement and flow angle (Edlin et al., 2019). The velocity angle proved to be an excellent biomarker for differentiating between volunteers and patients with BAV, BAV morphotypes, and BAV phenotypes (Sotelo et al., 2022). Compared with patients with TAV with matched aortic diameters and valve function, patients with BAV exhibit significantly greater normalized displacement, which varies by valve morphology (Mahadevia et al., 2014). These variables are greater in the proximal aorta and progressively decrease in the distal AAo, indicating that the flow tends to become more symmetric in the distal AAo (Rodríguez-Palomares et al., 2018). In addition, patients with BAV with significantly eccentric flow demonstrated faster growth (Hope et al., 2014).
4.3 Flow patterns
Altered flow patterns in aortopathy can accelerate its progression. Helical flow, defined as localized fluid circulation along the longitudinal axis of a vessel, is a key parameter reflecting the relationship between flow velocity and vorticity. In healthy individuals, aortic blood flow follows a right-handed (clockwise) helical pattern through the ascending aorta and aortic arch (Bogren and Buonocore, 1999; von Knobelsdorff-Brenkenhoff et al., 2014). This requires the WSS and helicity index to measure the degree of helicity.
Patients with BAV exhibit increased right-handed helical flow (pathological right-handed helicity) characterized by spiral flow lines extending from the aortic root to the beginning of the aortic arch (Shan et al., 2017). In a study conducted by Patrick Geeraert and colleagues of 73 BAV patients, small helical flow regions were observed in the aortic arch and proximal descending aorta, whereas larger helical flow zones with significant reverse flow directions were detected in the AAo (Geeraert et al., 2021a). In patients with BAV with pathological right-handed helical flow, the aortic diameter increased in proportion to the severity of flow turbulence. Conversely, in patients with normal flow patterns, the aortic dimensions were similar to those in the control group (Bissell et al., 2013). The WSS angle, an indicator of helicity near the aortic wall, typically decreases in dilated aortic regions. It peaks in the medial proximal ascending aorta, indicating maximal helical flow at the aortic wall in this region, which is consistent with the previously described zones of flow disturbance (Minderhoud et al., 2024).
4.4 Viscous energy loss and kinetic energy
Viscous energy loss (EL) represents the energy dissipated within blood flow due to frictional forces and, in the ascending aorta, can serve as an indicator of left ventricular afterload and cardiac events (Geeraert et al., 2021a). In patients with BAV, both the systolic EL and the EL index in the aorta are significantly elevated compared with those in healthy volunteers, which correlates with increased aortic diameters at the mid AAo and proximal AAo to the first aortic branch planes (Geeraert et al., 2021b). Kinetic energy (KE) quantifies flow velocity and energy dynamics within the aorta. Some studies have reported minimal differences in KE at regions of the aortic root and AAo, whereas other investigations have failed to observe statistically significant changes (Elbaz et al., 2019; Geeraert et al., 2021a). KE emphasizes the overall energy of blood flow, whereas turbulent kinetic energy (TKE) focuses on the energy fluctuations caused by turbulent blood flow. Therefore, the TKE might better represent the hemodynamics observed in BAV patients (Ferrari and Obrist, 2024). Both EL and TKE show substantial increases in BAV patients, particularly within the AAo (Dux-Santoy et al., 2019; Elbaz et al., 2019).
4.5 BAV morphotypes association with aortopathy
Valve morphology is a key determinant of the severity of aortic dilation, with distinct hemodynamic patterns observed among different BAV phenotypes (Bissell et al., 2013; Rodríguez-Palomares et al., 2018). Different cusp fusion patterns (BAV-RL and BAV-RN) create distinct regional variations in WSS patterns. In BAV-RL patients, the WSS along the outer curvature of the aortic root and ascending aorta is significantly elevated by 9%–34% compared with that in controls, whereas in BAV-RN patients, the WSS increases by 30% in the distal aorta (van Ooij et al., 2017; Rodríguez-Palomares et al., 2018; Dux-Santoy et al., 2020). Compared with BAV-RL, BAV-RN results in more severe flow abnormalities characterized by increased AAo dilation, exacerbated eccentric flow, extensive regions of significantly elevated normalized displacement localized at the proximal AAo, and intensified rotational flow in the proximal aortic arch (Mahadevia et al., 2014; Shan et al., 2017). Regarding flow patterns, both the RN and RL types are associated with a predominance of right-handed helical flow; however, the RL type results in a greater proportion of normal flow and an absence of left-handed helical flow (Bissell et al., 2013). This may explain the significant differences in hemodynamic parameters between BAV-RN and BAV-RL observed in the aforementioned studies (Table 2).
Many studies now focus on the morphological characteristics of the valve itself, particularly the commissural angle. BAVs with highly asymmetric commissures (120° commissural angle) exhibit a reduced aortic orifice area during systolic peak flow, with jet streams impacting the right posterior wall of the proximal ascending aorta, leading to elevated WSS. In contrast, more symmetric commissures (180° commissural angle) diminish jet impacts on the posterior wall, resulting in linearly reduced stress and strain levels on the unfused noncoronary cusp (Murphy et al., 2017; Yeats et al., 2024).
4.6 BAV dysfunction association with aortic pathology
Numerous studies have shown that BAV functional phenotypes (e.g., aortic stenosis and aortic regurgitation) are significantly associated with the severity and progression of aortopathy (Girdauskas et al., 2017). In AS patients, the WSS, viscous EL, TKE, and retrograde flow in the AAo are all elevated and correlate with the severity of stenosis, which is primarily localized to the outflow tract and proximal AAo (Dyverfeldt et al., 2013; Binter et al., 2017; Christian et al., 2017; Elbaz et al., 2019; Geeraert et al., 2021b). Hemodynamic studies on aortic valve dysfunction have revealed that all degrees of AS lead to localized WSS increases in both BAV and TAV patients. Compared with healthy individuals, mild AS significantly increases the WSS in the lateral region of the proximal AAo, which contrasts with TAV-associated aortic dilation accompanied by mild AS. Moderate to severe AS results in a significant increase in WSS across nearly the entire AAo and aortic root, irrespective of the valve phenotype (van Ooij et al., 2017). Yan Shan et al. further confirmed that, regardless of the presence of valvular dysfunction, the location of the peak WSS in the BAV-RL aligns with the valve type and tends to converge in the middle and distal regions of the AAo (Shan et al., 2017; 2019). Thus, we believe that an elevated WSS appears to be a characteristic mechanism underlying aortic dilation in patients with AS, overriding the influence of aortic valve morphology.
In contrast, severe AR is characterized by localized, noneccentric WSS increases that are positively correlated with stroke volume (Shan et al., 2017). A recent cross-sectional study by Elizabeth K. Weiss indicated that in BAV patients, with increasing severity of AR, there is a time-dependent increase in retrograde flow in the AAo, particularly during diastole. Moreover, they demonstrated that AAo dilation is independently associated with AR in BAV patients (Weiss et al., 2023; Fujiwara et al., 2024). The oscillatory shear index (OSI) appears to be the sole hemodynamic parameter capable of identifying abnormalities in BAV patients with AR. OSI elevation predominantly occurs in the outer mid-to-distal AAo and aortic root, but it does not appear to be associated with the region of maximal dilation in patients with BAV. An elevated OSI may result from the combined effects of inherent regurgitant flow and increased stroke volume associated with AR. Moreover, the greater the severity of AR is, the higher the OSI value. Therefore, OSI elevation may represent a characteristic mechanism of aortic valve regurgitation but is weakly associated with the region of aortic dilation (Dux-Santoy et al., 2020; Trenti et al., 2024).
4.7 Hemodynamic characteristics of paediatric BAV patients
Compared with adults, paediatric BAV patients exhibit greater variability in age, weight, and aortic dimensions, along with distinct hemodynamic parameters. Similar to adults, the severity of valvular dysfunction and the BAV-RN phenotype can serve as independent factors for AAo dilation in paediatric or young patients (Grattan et al., 2020). Significant correlations exist between age and peak systolic velocity and WSS, whereas retrospective studies on paediatric BAV patients have shown that the WSS direction and velocity direction are correlated with AAo z scores rather than with the WSS magnitude as in adults (Allen et al., 2015; van Ooij et al., 2016; Fujiwara et al., 2024). A longitudinal study with a follow-up of 3.4 years confirmed that peak velocity in the AAo serves as a predictor of aortic dilation in young and paediatric BAV patients (Rose et al., 2019). In particular, in children with valvular dysfunction, the peak velocity is significantly increased across all aortic segments (Stefek et al., 2019). Similar to adults, the correlation between hemodynamic parameters and aortic diameter varies across anatomical planes in paediatric BAV patients. For example, the diameters of the aortic root and the sino tubular junction (STJ) are significantly associated with vorticity and KE but not with peak velocity (Henry et al., 2023). Peak velocity and WSS are greater in the AAo region than in the other regions (Allen et al., 2015; Stefek et al., 2019; Fujiwara et al., 2024). Overall, compared with that in adults, research on paediatric BAV patients remains limited, with fewer hemodynamic parameters studied and substantial variability in aortic dimensions hindering the establishment of reliable reference data.
5 Hemodynamic characteristics of postsurgical BAV patients
Currently, significant hemodynamic changes are observed in patients prior to surgery. We also reviewed their hemodynamic characteristics after surgery.
5.1 Aortic valve replacement (AVR) and aortic root replacement (ARR)
Multiple studies have shown that post-AVR peak flow and WSS remain elevated in patients compared with healthy individuals, and there are also significant differences compared with native valves (von Knobelsdorff-Brenkenhoff et al., 2014; Collins et al., 2015; Farag et al., 2019). A study of the postoperative outcomes of various valve types in AVR revealed that all valve types demonstrated eccentric flow, which was primarily concentrated on the right-anterior aortic wall (von Knobelsdorff-Brenkenhoff et al., 2014). Hemodynamic studies comparing TAVR and SAVR have suggested that post-TAVR, the eccentricity and displacement of blood flow are altered in the middle and distal segments of the ascending aorta, whereas SAVR primarily affects the distal AAo (Farag et al., 2019). However, this study did not clarify the extent of hemodynamic improvement postoperatively compared with the preoperative state. In a 4D flow study of AS patients, comparisons of hemodynamic changes before and after AVR revealed a 30% increase in flow rate and a reduction in the AAo flow angle from 39° to 25° (Kamada et al., 2020). Yuki Yoshioka and colleagues suggested that surgeries such as AVR and AAR reduce eccentric jets, thereby lowering WSS (Bollache et al., 2018a; Yoshioka et al., 2023). Recently, an in-silico study demonstrated that post-AVR hemodynamics exhibit weaker, less eccentric jets and increased OSI. Significant reductions are observed in flow helicity, time-averaged WSS and WSS divergence in localized regions of the proximal AAo (Bailoor et al., 2024).
Eric J. Keller et al. reported that in 10 patients who underwent the standard Bentall procedure, the abnormal hemodynamics observed before aortic root replacement (ARR) were significantly reduced. The helicity and vorticity levels significantly decreased, restoring flow patterns similar to those in healthy individuals (Keller et al., 2016). ARR reduces the local WSS, whereas the proximal WSS decreases after ARR, the graft distal WSS increases, and no significant temporal changes are observed (Bollache et al., 2018a). As WSS is a primary flow parameter for aortic dilation in BAVs, we hypothesize that changes in postoperative WSS could predict alterations in aortic diameter. However, large-scale studies on this correlation are still lacking. Altered flow patterns may reflect improved aortopathy and normalization of the aortic flow pattern postsurgery.
5.2 Valve-sparing aortic root replacement (VSARR)
In a cohort primarily comprising TAV patients with approximately 3 years of follow-up, VSARR was associated with significantly greater EL in the proximal aorta than was the healthy group. The maximum flow velocity in the distal AAo was significantly elevated relative to that in healthy controls. However, parameters such as pulse wave velocity, WSS, and flow patterns (eccentricity, vorticity, and helicity) did not significantly differ (Cvitkovic et al., 2022). In a mixed TAV and BAV population study, VSARR patients retained valves and exhibited lower flow eccentricity with no AAo wall jetting. However, helical flow remained significantly greater than that in healthy individuals, and the systolic peak velocity was also elevated, which was different from previous findings. In studies involving only TAV patients, the flow velocity and WSS in the ascending and descending aorta were significantly greater, particularly in distal graft regions where the axial WSS was notably greater (Collins et al., 2015; Gaudino et al., 2019; Oechtering et al., 2020). TAV patients cannot fully represent BAV patients who undergo AVR (Kaiser et al., 2022). Stephens et al. reported that among 19 BAV patients who underwent VSARR, most exhibited prominent right-handed or left-right helical flow in the AAo, although the study did not specify whether these were pathological patterns (Stephens et al., 2015). After VSARR, BAV patients continue to exhibit greater asymmetric WSS throughout the entire AAo than healthy individuals do (Stephens et al., 2015). Post-VSARR, BAV patients indeed show significant hemodynamic improvement and clinical outcomes, indicating long-term survival rates and reoperation rates comparable to those of the Bentall procedure (Kalra et al., 2021; Bethancourt et al., 2022; Zuo et al., 2023). However, whether retained abnormal leaflets and age-related valve dysfunction could further impact the postoperative aorta, as well as unoperated native arteries, requires further follow-up studies.
Overall, there is significant improvement in postoperative hemodynamics following AVR/ARR, but eccentric blood flow and localized WSS remain present postoperatively. In the VSARR data reviewed, the TAV cohort was able to return to normal hemodynamic characteristics. Meanwhile, the mixed cohort or solely BAV cohort exhibited less eccentric blood flow, with only the flow patterns remaining abnormal. Of course, this result is limited by factors such as surgical selection bias in the population and the insufficient postoperative hemodynamic data for VSARR. However, these postoperative hemodynamic data will also provide valuable references for surgical approach selection and postoperative prognostic evaluation.
6 Limitations and future directions
The relationship between aortic dilation and hemodynamics in BAV patients is complex. However, in current studies, most patients do not have documented genetic characteristics, making it difficult to distinguish the overlapping contributions of hemodynamic and genetic factors. Aortic dilation may result from hemodynamic alterations, or alternatively, it could stem from genetic factors that lead to dilation, subsequently causing hemodynamic abnormalities. Recently, via fluid‒structure interaction simulations, it has become possible to isolate the impact of valve morphology on hemodynamics in patients with aortic disease. This study may isolate the contribution of valve-related genetic abnormalities to the aortic dilation (Kaiser et al., 2022). Current international guidelines and criteria for surgical intervention now incorporate genetic considerations, which aid in the early identification of complications and prognosis in BAV patients. However, the genetic factors influencing complications and surgical outcomes at this stage remain unclear (Michelena et al., 2021; Isselbacher et al., 2022).
Although there has been extensive preoperative 4D flow hemodynamic research on BAV patients, the focus has largely been limited to parameters such as the WSS and peak velocity. The data types are inconsistent, and even when similar data types are used, their representation varies, lacking standardized formats (Cave et al., 2021). Comprehensive large-scale, multicentre cohort studies are still lacking, as are outcome prediction models. The potential value of hemodynamic parameters in aortopathy has yet to be fully explored. The assessment of pediatric BAV patients still faces significant challenges in the future. On one hand, there are difficulties in standardizing evaluations due to age, size and genetic variability. On the other hand, there is also a lack of hemodynamic data regarding long-term outcomes after different surgical procedures for pediatric BAV patients. There is also a lack of clinical research data comparing the hemodynamics of children and adults.
Postoperative 4D flow hemodynamic studies are limited in quantity and diversity, with most failing to differentiate between bicuspid and tricuspid valve patients. There is a lack of clarity regarding whether hemodynamic parameters improve postoperatively in BAV patients, what constitutes improvement criteria, and what the definitive prognostic outcomes are (Collins et al., 2015; Gaudino et al., 2019; Oechtering et al., 2020; Cvitkovic et al., 2022). Hemodynamic assessments for evaluating prognostic correlations in postoperative patients also remain underexplored. There is a lack of postoperative hemodynamic data research focused on specific surgical techniques particularly AAR.
The integration of machine learning and AI has advanced the automated evaluation of hemodynamic patterns. However, there remains a need for further development in the qualitative and quantitative analysis of relevant parameters, as well as in the breadth of data types. Prognostic assessments in this domain also remain underdeveloped (Haji-Valizadeh et al., 2021; Peper et al., 2022; Kim et al., 2023). Developing predictive models based on future advancements in hemodynamics holds significant importance not only for predicting outcomes in BAV patients but also for those with other aortic-related diseases.
Author contributions
MY: Conceptualization, Data curation, Formal Analysis, Investigation, Resources, Visualization, Writing – original draft, Writing – review and editing. ZN: Writing – original draft, Writing – review editing. HY: Investigation, Resources, Supervision, Visualization, Writing – review and editing. WL: Funding acquisition, Methodology, Project administration, Supervision, Writing – review and editing. ZW: Funding acquisition, Methodology, Project administration, Supervision, Writing – review and editing.
Funding
The author(s) declare that no financial support was received for the research and/or publication of this article.
Acknowledgments
The authors are grateful to AJE website for English revision. The authors are grateful to BioRender for creating the figures.
Conflict of interest
The authors declare that the research was conducted in the absence of any commercial or financial relationships that could be construed as a potential conflict of interest.
Generative AI statement
The authors declare that no Generative AI was used in the creation of this manuscript.
Publisher’s note
All claims expressed in this article are solely those of the authors and do not necessarily represent those of their affiliated organizations, or those of the publisher, the editors and the reviewers. Any product that may be evaluated in this article, or claim that may be made by its manufacturer, is not guaranteed or endorsed by the publisher.
References
Allen B. D., van Ooij P., Barker A. J., Carr M., Gabbour M., Schnell S., et al. (2015). Thoracic aorta 3D hemodynamics in pediatric and young adult patients with bicuspid aortic valve. J. Magn. Reson Imaging 42 (4), 954–963. doi:10.1002/jmri.24847
Andelfinger G., Loeys B., Dietz H. (2016). A decade of discovery in the genetic understanding of thoracic aortic disease. Can. J. Cardiol. 32 (1), 13–25. doi:10.1016/j.cjca.2015.10.017
Anderson R. H., Webb S., Brown N. A., Lamers W., Moorman A. (2003). Development of the heart: (3) formation of the ventricular outflow tracts, arterial valves, and intrapericardial arterial trunks. Heart 89 (9), 1110–1118. doi:10.1136/heart.89.9.1110
Antequera-González B., Martínez-Micaelo N., Alegret J. M. (2020). Bicuspid aortic valve and endothelial dysfunction: current evidence and potential therapeutic targets. Front. Physiol. 11, 1015. doi:10.3389/fphys.2020.01015
Avila-Vanzzini N., Medina-Paz L., Berrios-Bárcenas E., Arias-Godínez A., Díaz-Marín J., Herrera-Bello H., et al. (2022). Anatomical and clinical factors associated with valvulopathy and aortopathy in Mexican patients with bicuspid aortic valves. Am. J. Cardiol. 184, 96–103. doi:10.1016/j.amjcard.2022.08.034
Baek K. I., Chang S. S., Chang C. C., Roustaei M., Ding Y., Wang Y., et al. (2022). Vascular injury in the zebrafish tail modulates blood flow and peak wall shear stress to restore embryonic circular network. Front. Cardiovasc Med. 9, 841101. doi:10.3389/fcvm.2022.841101
Baeyens N., Bandyopadhyay C., Coon B. G., Yun S., Schwartz M. A. (2016). Endothelial fluid shear stress sensing in vascular health and disease. J. Clin. Invest. 126 (3), 821–828. doi:10.1172/jci83083
Bailoor S., Seo J. H., Schena S., Mittal R. (2024). Changes in aorta hemodynamics in Left-Right Type 1 bicuspid aortic valve patients after replacement with bioprosthetic valves: an in-silico study. PLoS One 19 (4), e0301350. doi:10.1371/journal.pone.0301350
Baleilevuka-Hart M., Teng B. J., Carson K. A., Ravekes W. J., Holmes K. W. (2020). Sports participation and exercise restriction in children with isolated bicuspid aortic valve. Am. J. Cardiol. 125 (11), 1673–1677. doi:10.1016/j.amjcard.2020.02.039
Bavaria J. E., Mumtaz M. A., Griffith B., Svensson L. G., Pibarot P., Borger M. A., et al. (2024). Five-year outcomes after bicuspid aortic valve replacement with a novel tissue bioprosthesis. Ann. Thorac. Surg. 118 (1), 173–179. doi:10.1016/j.athoracsur.2023.11.036
Beckmann E., Martens A., Krüger H., Korte W., Kaufeld T., Stettinger A., et al. (2020). Aortic valve-sparing root replacement in patients with bicuspid aortic valve: long-term outcome with the David I procedure over 20 years. Eur. J. Cardiothorac. Surg. 58 (1), 86–93. doi:10.1093/ejcts/ezaa083
Bethancourt C. N., Blitzer D., Yamabe T., Zhao Y., Nguyen S., Nitta S., et al. (2022). Valve-sparing root replacement versus bio-bentall: inverse propensity weighting of 796 patients. Ann. Thorac. Surg. 113 (5), 1529–1535. doi:10.1016/j.athoracsur.2021.05.044
Binter C., Gotschy A., Sündermann S. H., Frank M., Tanner F. C., Lüscher T. F., et al. (2017). Turbulent kinetic energy assessed by multipoint 4-dimensional flow magnetic resonance imaging provides additional information relative to echocardiography for the determination of aortic stenosis severity. Circ. Cardiovasc Imaging 10 (6), e005486. doi:10.1161/circimaging.116.005486
Bissell M. M., Hess A. T., Biasiolli L., Glaze S. J., Loudon M., Pitcher A., et al. (2013). Aortic dilation in bicuspid aortic valve disease: flow pattern is a major contributor and differs with valve fusion type. Circ. Cardiovasc Imaging 6 (4), 499–507. doi:10.1161/circimaging.113.000528
Bissell M. M., Raimondi F., Ait Ali L., Allen B. D., Barker A. J., Bolger A., et al. (2023). 4D Flow cardiovascular magnetic resonance consensus statement: 2023 update. J. Cardiovasc Magn. Reson 25 (1), 40. doi:10.1186/s12968-023-00942-z
Bogren H. G., Buonocore M. H. (1999). 4D magnetic resonance velocity mapping of blood flow patterns in the aorta in young vs. elderly normal subjects. J. Magn. Reson Imaging 10 (5), 861–869. doi:10.1002/(sici)1522-2586(199911)10:5<861::aid-jmri35>3.0.co;2-e
Bollache E., Fedak P. W. M., van Ooij P., Rahman O., Malaisrie S. C., McCarthy P. M., et al. (2018a). Perioperative evaluation of regional aortic wall shear stress patterns in patients undergoing aortic valve and/or proximal thoracic aortic replacement. J. Thorac. Cardiovasc Surg. 155 (6), 2277–2286. doi:10.1016/j.jtcvs.2017.11.007
Bollache E., Guzzardi D. G., Sattari S., Olsen K. E., Di Martino E. S., Malaisrie S. C., et al. (2018b). Aortic valve-mediated wall shear stress is heterogeneous and predicts regional aortic elastic fiber thinning in bicuspid aortic valve-associated aortopathy. J. Thorac. Cardiovasc Surg. 156 (6), 2112–2120. doi:10.1016/j.jtcvs.2018.05.095
Borger M. A., Fedak P. W. M., Stephens E. H., Gleason T. G., Girdauskas E., Ikonomidis J. S., et al. (2018). The American Association for Thoracic Surgery consensus guidelines on bicuspid aortic valve-related aortopathy: full online-only version. J. Thorac. Cardiovasc Surg. 156 (2), e41–e74. doi:10.1016/j.jtcvs.2018.02.115
Borger M. A., Preston M., Ivanov J., Fedak P. W., Davierwala P., Armstrong S., et al. (2004). Should the ascending aorta be replaced more frequently in patients with bicuspid aortic valve disease? J. Thorac. Cardiovasc Surg. 128 (5), 677–683. doi:10.1016/j.jtcvs.2004.07.009
Bravo-Jaimes K., Prakash S. K. (2020). Genetics in bicuspid aortic valve disease: where are we? Prog. Cardiovasc Dis. 63 (4), 398–406. doi:10.1016/j.pcad.2020.06.005
Bray J. J. H., Freer R., Pitcher A., Kharbanda R. (2023). Family screening for bicuspid aortic valve and aortic dilatation: a meta-analysis. Eur. Heart J. 44 (33), 3152–3164. doi:10.1093/eurheartj/ehad320
Brownstein A. J., Kostiuk V., Ziganshin B. A., Zafar M. A., Kuivaniemi H., Body S. C., et al. (2018). Genes associated with thoracic aortic aneurysm and dissection: 2018 update and clinical implications. Aorta (Stamford) 6 (1), 13–20. doi:10.1055/s-0038-1639612
Cave D. G. W., Panayiotou H., Bissell M. M. (2021). Hemodynamic profiles before and after surgery in bicuspid aortic valve disease-A systematic review of the literature. Front. Cardiovasc Med. 8, 629227. doi:10.3389/fcvm.2021.629227
Chen L. J., Li J. Y., Nguyen P., He M., Chen Z. B., Subramaniam S., et al. (2024). Single-cell RNA sequencing unveils unique transcriptomic signatures of endothelial cells and role of ENO1 in response to disturbed flow. Proc. Natl. Acad. Sci. U. S. A. 121 (5), e2318904121. doi:10.1073/pnas.2318904121
Chen P. Y., Qin L., Baeyens N., Li G., Afolabi T., Budatha M., et al. (2015). Endothelial-to-mesenchymal transition drives atherosclerosis progression. J. Clin. Invest. 125 (12), 4514–4528. doi:10.1172/jci82719
Cheng C., Bohbot Y., Michelena H. I., Rusinaru D., Fay F., Elmkies F., et al. (2021). Clinical outcomes of adults with bicuspid aortic valve: a European perspective. Mayo Clin. Proc. 96 (3), 648–657. doi:10.1016/j.mayocp.2020.04.047
Christian B., Alexander G., Simon H. S., Michelle F., Felix C. T., Thomas F. L., et al. (2017). Turbulent kinetic energy assessed by multipoint 4-dimensional flow magnetic resonance imaging provides additional information relative to echocardiography for the determination of aortic stenosis severity. Circ. Cardiovasc. imaging 10 (6), e005486. doi:10.1161/circimaging.116.005486
Collins J. D., Semaan E., Barker A., McCarthy P. M., Carr J. C., Markl M., et al. (2015). Comparison of hemodynamics after aortic root replacement using valve-sparing or bioprosthetic valved conduit. Ann. Thorac. Surg. 100 (5), 1556–1562. doi:10.1016/j.athoracsur.2015.04.109
Conway D. E., Breckenridge M. T., Hinde E., Gratton E., Chen C. S., Schwartz M. A. (2013). Fluid shear stress on endothelial cells modulates mechanical tension across VE-cadherin and PECAM-1. Curr. Biol. 23 (11), 1024–1030. doi:10.1016/j.cub.2013.04.049
Cramer P. M., Prakash S. K. (2019). Misclassification of bicuspid aortic valves is common and varies by imaging modality and patient characteristics. Echocardiography 36 (4), 761–765. doi:10.1111/echo.14295
Cvitkovic T., Bobylev D., Horke A., Avsar M., Beerbaum P., Martens A., et al. (2022). 4D-flow cardiac magnetic resonance imaging after aortic root replacement with long-valved decellularized aortic homografts: comparison to valve-sparing aortic root replacement and healthy controls. Eur. J. Cardiothorac. Surg. 61 (6), 1307–1315. doi:10.1093/ejcts/ezac016
Della Corte A., Bancone C., Buonocore M., Dialetto G., Covino F. E., Manduca S., et al. (2013). Pattern of ascending aortic dimensions predicts the growth rate of the aorta in patients with bicuspid aortic valve. JACC Cardiovasc Imaging 6 (12), 1301–1310. doi:10.1016/j.jcmg.2013.07.009
Detaint D., Michelena H. I., Nkomo V. T., Vahanian A., Jondeau G., Sarano M. E. (2014). Aortic dilatation patterns and rates in adults with bicuspid aortic valves: a comparative study with Marfan syndrome and degenerative aortopathy. Heart 100 (2), 126–134. doi:10.1136/heartjnl-2013-304920
Devereux R. B., de Simone G., Arnett D. K., Best L. G., Boerwinkle E., Howard B. V., et al. (2012). Normal limits in relation to age, body size and gender of two-dimensional echocardiographic aortic root dimensions in persons ≥15 years of age. Am. J. Cardiol. 110 (8), 1189–1194. doi:10.1016/j.amjcard.2012.05.063
Donadei M., Casatori L., Bini V., Galanti G., Stefani L. (2019). Diagnostic pathway and clinical significance of premature ventricular beats (PVBs) in trained bicuspid aortic valve (BAV) athletes. J. Funct. Morphol. Kinesiol 4 (4), 69. doi:10.3390/jfmk4040069
Du Q., Zhang D., Zhuang Y., Xia Q., Wen T., Jia H. (2021). The molecular genetics of marfan syndrome. Int. J. Med. Sci. 18 (13), 2752–2766. doi:10.7150/ijms.60685
Dux-Santoy L., Guala A., Sotelo J., Uribe S., Teixidó-Turà G., Ruiz-Muñoz A., et al. (2020). Low and oscillatory wall shear stress is not related to aortic dilation in patients with bicuspid aortic valve: a time-resolved 3-dimensional phase-contrast magnetic resonance imaging study. Arterioscler. Thromb. Vasc. Biol. 40 (1), e10–e20. doi:10.1161/atvbaha.119.313636
Dux-Santoy L., Guala A., Teixidó-Turà G., Ruiz-Muñoz A., Maldonado G., Villalva N., et al. (2019). Increased rotational flow in the proximal aortic arch is associated with its dilation in bicuspid aortic valve disease. Eur. Heart J. Cardiovasc Imaging 20 (12), 1407–1417. doi:10.1093/ehjci/jez046
Dyverfeldt P., Hope M. D., Tseng E. E., Saloner D. (2013). Magnetic resonance measurement of turbulent kinetic energy for the estimation of irreversible pressure loss in aortic stenosis. JACC Cardiovasc Imaging 6 (1), 64–71. doi:10.1016/j.jcmg.2012.07.017
Edlin J., Youssefi P., Bilkhu R., Figueroa C. A., Morgan R., Nowell J., et al. (2019). Haemodynamic assessment of bicuspid aortic valve aortopathy: a systematic review of the current literature. Eur. J. Cardiothorac. Surg. 55 (4), 610–617. doi:10.1093/ejcts/ezy312
Ehrlich T., de Kerchove L., Vojacek J., Boodhwani M., El-Hamamsy I., De Paulis R., et al. (2020). State-of-the art bicuspid aortic valve repair in 2020. Prog. Cardiovasc Dis. 63 (4), 457–464. doi:10.1016/j.pcad.2020.04.010
Elbaz M. S. M., Scott M. B., Barker A. J., McCarthy P., Malaisrie C., Collins J. D., et al. (2019). Four-dimensional virtual catheter: noninvasive assessment of intra-aortic hemodynamics in bicuspid aortic valve disease. Radiology 293 (3), 541–550. doi:10.1148/radiol.2019190411
Evangelista A., Gallego P., Calvo-Iglesias F., Bermejo J., Robledo-Carmona J., Sánchez V., et al. (2018). Anatomical and clinical predictors of valve dysfunction and aortic dilation in bicuspid aortic valve disease. Heart 104 (7), 566–573. doi:10.1136/heartjnl-2017-311560
Farag E. S., Vendrik J., van Ooij P., Poortvliet Q. L., van Kesteren F., Wollersheim L. W., et al. (2019). Transcatheter aortic valve replacement alters ascending aortic blood flow and wall shear stress patterns: a 4D flow MRI comparison with age-matched, elderly controls. Eur. Radiol. 29 (3), 1444–1451. doi:10.1007/s00330-018-5672-z
Fedak P. W., Verma S., David T. E., Leask R. L., Weisel R. D., Butany J. (2002). Clinical and pathophysiological implications of a bicuspid aortic valve. Circulation 106 (8), 900–904. doi:10.1161/01.cir.0000027905.26586.e8
Ferrari L., Obrist D. (2024). Comparison of hemodynamic performance, three-dimensional flow fields, and turbulence levels for three different heart valves at three different hemodynamic conditions. Ann. Biomed. Eng. 52 (12), 3196–3207. doi:10.1007/s10439-024-03584-z
Fleming I., Fisslthaler B., Dixit M., Busse R. (2005). Role of PECAM-1 in the shear-stress-induced activation of Akt and the endothelial nitric oxide synthase (eNOS) in endothelial cells. J. Cell Sci. 118 (Pt 18), 4103–4111. doi:10.1242/jcs.02541
Flyer J. N., Sleeper L. A., Colan S. D., Singh M. N., Lacro R. V. (2021). Effect of losartan or atenolol on children and young adults with bicuspid aortic valve and dilated aorta. Am. J. Cardiol. 144, 111–117. doi:10.1016/j.amjcard.2020.12.050
Franco P., Sotelo J., Guala A., Dux-Santoy L., Evangelista A., Rodríguez-Palomares J., et al. (2022). Identification of hemodynamic biomarkers for bicuspid aortic valve induced aortic dilation using machine learning. Comput. Biol. Med. 141, 105147. doi:10.1016/j.compbiomed.2021.105147
Fraser C. D., Wang N., Mee R. B., Lytle B. W., McCarthy P. M., Sapp S. K., et al. (1994). Repair of insufficient bicuspid aortic valves. Ann. Thorac. Surg. 58 (2), 386–390. doi:10.1016/0003-4975(94)92212-8
Fujiwara T., Malone L. J., Chatfield K. C., Berthusen A., Fonseca B., Browne L. P., et al. (2024). Assessment of abnormal transvalvular flow and wall shear stress direction for pediatric/young adults with bicuspid aortic valve: a cross-sectional four-dimensional flow study. J. Cardiovasc Magn. Reson 26 (2), 101102. doi:10.1016/j.jocmr.2024.101102
Galian-Gay L., Carro Hevia A., Teixido-Turà G., Rodríguez Palomares J., Gutiérrez-Moreno L., Maldonado G., et al. (2019). Familial clustering of bicuspid aortic valve and its relationship with aortic dilation in first-degree relatives. Heart 105 (8), 603–608. doi:10.1136/heartjnl-2018-313802
Galian-Gay L., Rodríguez-Palomares J., Guala A., Michelena H. I., Evangelista A. (2020). Multimodality imaging in bicuspid aortic valve. Prog. Cardiovasc Dis. 63 (4), 442–451. doi:10.1016/j.pcad.2020.06.003
Galian-Gay L., Rodriguez-Palomares J. F. (2022). Turner syndrome and aortic complications: more benign than previously thought. Heart 109 (2), 82–83. doi:10.1136/heartjnl-2022-321330
Gaudino M., Piatti F., Lau C., Sturla F., Weinsaft J. W., Weltert L., et al. (2019). Aortic flow after valve sparing root replacement with or without neosinuses reconstruction. J. Thorac. Cardiovasc Surg. 157 (2), 455–465. doi:10.1016/j.jtcvs.2018.06.094
Geeraert P., Jamalidinan F., Burns F., Jarvis K., Bristow M. S., Lydell C., et al. (2021a). Hemodynamic assessment in bicuspid aortic valve disease and aortic dilation: new insights from voxel-by-voxel analysis of reverse flow, stasis, and energetics. Front. Bioeng. Biotechnol. 9, 725113. doi:10.3389/fbioe.2021.725113
Geeraert P., Jamalidinan F., Fatehi Hassanabad A., Sojoudi A., Bristow M., Lydell C., et al. (2021b). Bicuspid aortic valve disease is associated with abnormal wall shear stress, viscous energy loss, and pressure drop within the ascending thoracic aorta: a cross-sectional study. Med. Baltim. 100 (26), e26518. doi:10.1097/md.0000000000026518
Gilles S., Michael B. S., Bradley D. A., Ryan A., Robert O. B., Malaisrie S. C., et al. (2022). Association of regional wall shear stress and progressive ascending aorta dilation in bicuspid aortic valve. JACC. Cardiovasc. imaging 15 (1), 33–42. doi:10.1016/j.jcmg.2021.06.020
Girdauskas E., Rouman M., Disha K., Fey B., Dubslaff G., von Kodolitsch Y., et al. (2017). Morphologic and functional markers of aortopathy in patients with bicuspid aortic valve insufficiency versus stenosis. Ann. Thorac. Surg. 103 (1), 49–57. doi:10.1016/j.athoracsur.2016.05.085
Glotzbach J. P., Hanson H. A., Tonna J. E., Horns J. J., McCarty Allen C., Presson A. P., et al. (2023). Familial associations of prevalence and cause-specific mortality for thoracic aortic disease and bicuspid aortic valve in a large-population database. Circulation 148 (8), 637–647. doi:10.1161/circulationaha.122.060439
Gomez A., Wang Z., Xuan Y., Wisneski A. D., Hope M. D., Saloner D. A., et al. (2020). Wall stress distribution in bicuspid aortic valve-associated ascending thoracic aortic aneurysms. Ann. Thorac. Surg. 110 (3), 807–814. doi:10.1016/j.athoracsur.2019.12.035
Grattan M., Prince A., Rumman R. K., Morgan C., Petrovic M., Hauck A., et al. (2020). Predictors of bicuspid aortic valve-associated aortopathy in childhood: a report from the mibava consortium. Circ. Cardiovasc Imaging 13 (3), e009717. doi:10.1161/circimaging.119.009717
Guala A., Dux-Santoy L., Teixido-Tura G., Ruiz-Muñoz A., Galian-Gay L., Servato M. L., et al. (2022). Wall shear stress predicts aortic dilation in patients with bicuspid aortic valve. JACC Cardiovasc Imaging 15 (1), 46–56. doi:10.1016/j.jcmg.2021.09.023
Guzzardi D. G., Barker A. J., van Ooij P., Malaisrie S. C., Puthumana J. J., Belke D. D., et al. (2015). Valve-related hemodynamics mediate human bicuspid aortopathy: insights from wall shear stress mapping. J. Am. Coll. Cardiol. 66 (8), 892–900. doi:10.1016/j.jacc.2015.06.1310
Haji-Valizadeh H., Guo R., Kucukseymen S., Paskavitz A., Cai X., Rodriguez J., et al. (2021). Highly accelerated free-breathing real-time phase contrast cardiovascular MRI via complex-difference deep learning. Magn. Reson Med. 86 (2), 804–819. doi:10.1002/mrm.28750
Halim S. A., Edwards F. H., Dai D., Li Z., Mack M. J., Holmes D. R., et al. (2020). Outcomes of transcatheter aortic valve replacement in patients with bicuspid aortic valve disease: a report from the society of thoracic surgeons/American college of cardiology transcatheter valve therapy registry. Circulation 141 (13), 1071–1079. doi:10.1161/circulationaha.119.040333
He M., Huang T. S., Li S., Hong H. C., Chen Z., Martin M., et al. (2019). Atheroprotective flow upregulates ITPR3 (inositol 1,4,5-trisphosphate receptor 3) in vascular endothelium via KLF4 (Krüppel-Like factor 4)-mediated histone modifications. Arterioscler. Thromb. Vasc. Biol. 39 (5), 902–914. doi:10.1161/atvbaha.118.312301
Henry M., Fadnes S., Lovstakken L., Mawad W., Mertens L., Nyrnes S. A. (2023). Flow dynamics in children with bicuspid aortic valve: a blood speckle tracking study. Ultrasound Med. Biol. 49 (11), 2354–2360. doi:10.1016/j.ultrasmedbio.2023.07.012
Hiratzka L. F., Bakris G. L., Beckman J. A., Bersin R. M., Carr V. F., Casey D. E., et al. (2010). 2010 ACCF/AHA/AATS/ACR/ASA/SCA/SCAI/SIR/STS/SVM guidelines for the diagnosis and management of patients with thoracic aortic disease: executive summary: a report of the American college of cardiology foundation/American heart association task force on practice guidelines, American association for thoracic surgery, American college of radiology, American stroke association, society of cardiovascular anesthesiologists, society for cardiovascular angiography and interventions, society of interventional radiology, society of thoracic surgeons, and society for vascular medicine. Anesth. Analg. 111 (2), 279–315. doi:10.1213/ANE.0b013e3181dd869b
Hope M. D., Sigovan M., Wrenn S. J., Saloner D., Dyverfeldt P. (2014). MRI hemodynamic markers of progressive bicuspid aortic valve-related aortic disease. J. Magn. Reson Imaging 40 (1), 140–145. doi:10.1002/jmri.24362
Hsu P. L., Chen J. S., Wang C. Y., Wu H. L., Mo F. E. (2019). Shear-induced CCN1 promotes atheroprone endothelial phenotypes and atherosclerosis. Circulation 139 (25), 2877–2891. doi:10.1161/circulationaha.118.033895
Isselbacher E. M., Preventza O., Hamilton Black J., Augoustides J. G., Beck A. W., Bolen M. A., et al. (2022). 2022 ACC/AHA guideline for the diagnosis and management of aortic disease: a report of the American heart association/American college of cardiology joint committee on clinical practice guidelines. Circulation 146 (24), e334–e482. doi:10.1161/cir.0000000000001106
Johnston K. W., Rutherford R. B., Tilson M. D., Shah D. M., Hollier L., Stanley J. C. (1991). Suggested standards for reporting on arterial aneurysms. Subcommittee on reporting standards for arterial aneurysms, ad hoc committee on reporting standards, society for vascular surgery and north American chapter, international society for cardiovascular surgery. J. Vasc. Surg. 13 (3), 452–458. doi:10.1067/mva.1991.26737
Kaiser A. D., Shad R., Schiavone N., Hiesinger W., Marsden A. L. (2022). Controlled comparison of simulated hemodynamics across tricuspid and bicuspid aortic valves. Ann. Biomed. Eng. 50 (9), 1053–1072. doi:10.1007/s10439-022-02983-4
Kalogeropoulos A. S., Redwood S. R., Allen C. J., Hurrell H., Chehab O., Rajani R., et al. (2022). A 20-year journey in transcatheter aortic valve implantation: evolution to current eminence. Front. Cardiovasc Med. 9, 971762. doi:10.3389/fcvm.2022.971762
Kalra K., Wagh K., Wei J. W., McPherson L., Binongo J. N., Leshnower B. G., et al. (2021). Regurgitant bicuspid aortopathy: is valve-sparing root replacement equivalent to Bentall procedure? Ann. Thorac. Surg. 112 (3), 737–745. doi:10.1016/j.athoracsur.2020.08.074
Kamada H., Ota H., Nakamura M., Imai Y., Ishida S., Sun W., et al. (2020). Perioperative hemodynamic changes in the thoracic aorta in patients with aortic valve stenosis: a prospective serial 4D-flow MRI study. Semin. Thorac. Cardiovasc Surg. 32 (1), 25–34. doi:10.1053/j.semtcvs.2019.07.006
Kang J. W., Song H. G., Yang D. H., Baek S., Kim D. H., Song J. M., et al. (2013). Association between bicuspid aortic valve phenotype and patterns of valvular dysfunction and bicuspid aortopathy: comprehensive evaluation using MDCT and echocardiography. JACC Cardiovasc Imaging 6 (2), 150–161. doi:10.1016/j.jcmg.2012.11.007
Kayatta M. O., Leshnower B. G., McPherson L., Zhang C., Lasanajak Y., Chen E. P. (2019). Valve sparing root replacement provides similar midterm outcomes in bicuspid and trileaflet valves. Ann. Thorac. Surg. 107 (1), 54–60. doi:10.1016/j.athoracsur.2018.07.045
Keller E. J., Malaisrie S. C., Kruse J., McCarthy P. M., Carr J. C., Markl M., et al. (2016). Reduction of aberrant aortic haemodynamics following aortic root replacement with a mechanical valved conduit. Interact. Cardiovasc Thorac. Surg. 23 (3), 416–423. doi:10.1093/icvts/ivw173
Keuning Z. A., Hendriks P. M., Duijnhouwer A. L., Meccanici F., Siebelink H. J., van den Hoven A. T., et al. (2024). Blood and imaging biomarkers in the long-term follow-up of bicuspid aortic valve patients. CJC Open 6 (1), 1–10. doi:10.1016/j.cjco.2023.09.012
Kim D., Jen M. L., Eisenmenger L. B., Johnson K. M. (2023). Accelerated 4D-flow MRI with 3-point encoding enabled by machine learning. Magn. Reson Med. 89 (2), 800–811. doi:10.1002/mrm.29469
Kong W. K., Delgado V., Poh K. K., Regeer M. V., Ng A. C., McCormack L., et al. (2017a). Prognostic implications of raphe in bicuspid aortic valve anatomy. JAMA Cardiol. 2 (3), 285–292. doi:10.1001/jamacardio.2016.5228
Kong W. K., Regeer M. V., Ng A. C., McCormack L., Poh K. K., Yeo T. C., et al. (2017b). Sex differences in phenotypes of bicuspid aortic valve and aortopathy: insights from a large multicenter, international registry. Circ. Cardiovasc Imaging 10 (3), e005155. doi:10.1161/circimaging.116.005155
Kreibich M., Rylski B., Czerny M., Pingpoh C., Siepe M., Beyersdorf F., et al. (2020). Type A aortic dissection in patients with bicuspid aortic valve aortopathy. Ann. Thorac. Surg. 109 (1), 94–100. doi:10.1016/j.athoracsur.2019.05.022
Kunnen S. J., Leonhard W. N., Semeins C., Hawinkels L., Poelma C., Ten Dijke P., et al. (2017). Fluid shear stress-induced TGF-β/ALK5 signaling in renal epithelial cells is modulated by MEK1/2. Cell Mol. Life Sci. 74 (12), 2283–2298. doi:10.1007/s00018-017-2460-x
Laforest B., Nemer M. (2012). Genetic insights into bicuspid aortic valve formation. Cardiol. Res. Pract. 2012, 180297. doi:10.1155/2012/180297
Levine D., Patel P., Zhao Y., Childress P., Chung M., Leshnower B. G., et al. (2023). Bicuspid aortic valve durability with valve-sparing aortic root replacement: comparison to tricuspid valve. Eur. J. Cardiothorac. Surg. 63 (4), ezad030. doi:10.1093/ejcts/ezad030
Lin A., Rajagopalan A., Nguyen H. H., White A. J., Vincent A. J., Mottram P. M. (2021). Dilatation of the ascending aorta in turner syndrome: influence of bicuspid aortic valve morphology and body composition. Heart Lung Circ. 30 (1), e29–e36. doi:10.1016/j.hlc.2020.10.005
Loeys B. L., Chen J., Neptune E. R., Judge D. P., Podowski M., Holm T., et al. (2005). A syndrome of altered cardiovascular, craniofacial, neurocognitive and skeletal development caused by mutations in TGFBR1 or TGFBR2. Nat. Genet. 37 (3), 275–281. doi:10.1038/ng1511
Ma M., Li Z., Mohamed M. A., Liu L., Wei X. (2021). Aortic root aortopathy in bicuspid aortic valve associated with high genetic risk. BMC Cardiovasc Disord. 21 (1), 413. doi:10.1186/s12872-021-02215-y
Mahadevia R., Barker A. J., Schnell S., Entezari P., Kansal P., Fedak P. W., et al. (2014). Bicuspid aortic cusp fusion morphology alters aortic three-dimensional outflow patterns, wall shear stress, and expression of aortopathy. Circulation 129 (6), 673–682. doi:10.1161/circulationaha.113.003026
Malaisrie S. C., Duncan B. F., Mehta C. K., Badiwala M. V., Rinewalt D., Kruse J., et al. (2015). The addition of hemiarch replacement to aortic root surgery does not affect safety. J. Thorac. Cardiovasc Surg. 150 (1), 118–124. doi:10.1016/j.jtcvs.2015.03.020
Maroun A., Scott M. B., Catania R., Berhane H., Jarvis K., Allen B. D., et al. (2024). Multiyear interval changes in aortic wall shear stress in patients with bicuspid aortic valve assessed by 4D flow MRI. J. Magn. Reson Imaging 60 (6), 2580–2589. doi:10.1002/jmri.29305
Masri A., Kalahasti V., Svensson L. G., Alashi A., Schoenhagen P., Roselli E. E., et al. (2017). Aortic cross-sectional area/height ratio and outcomes in patients with bicuspid aortic valve and a dilated ascending aorta. Circ. Cardiovasc Imaging 10 (6), e006249. doi:10.1161/circimaging.116.006249
Mennander A., Olsson C., Jeppsson A., Geirsson A., Hjortdal V., Hansson E. C., et al. (2020). The significance of bicuspid aortic valve after surgery for acute type A aortic dissection. J. Thorac. Cardiovasc Surg. 159 (3), 760–767. doi:10.1016/j.jtcvs.2019.03.012
Michelena H. I., Chandrasekaran K., Topilsky Y., Messika-Zeitoun D., Della Corte A., Evangelista A., et al. (2018). The bicuspid aortic valve condition: the critical role of echocardiography and the case for a standard nomenclature consensus. Prog. Cardiovasc Dis. 61 (5-6), 404–415. doi:10.1016/j.pcad.2018.11.003
Michelena H. I., Della Corte A., Evangelista A., Maleszewski J. J., Edwards W. D., Roman M. J., et al. (2021). International consensus statement on nomenclature and classification of the congenital bicuspid aortic valve and its aortopathy, for clinical, surgical, interventional and research purposes. J. Thorac. Cardiovasc Surg. 162 (3), e383–e414. doi:10.1016/j.jtcvs.2021.06.019
Michelena H. I., Desjardins V. A., Avierinos J. F., Russo A., Nkomo V. T., Sundt T. M., et al. (2008). Natural history of asymptomatic patients with normally functioning or minimally dysfunctional bicuspid aortic valve in the community. Circulation 117 (21), 2776–2784. doi:10.1161/circulationaha.107.740878
Michelena H. I., Khanna A. D., Mahoney D., Margaryan E., Topilsky Y., Suri R. M., et al. (2011). Incidence of aortic complications in patients with bicuspid aortic valves. Jama 306 (10), 1104–1112. doi:10.1001/jama.2011.1286
Michelena H. I., Prakash S. K., Della Corte A., Bissell M. M., Anavekar N., Mathieu P., et al. (2014). Bicuspid aortic valve: identifying knowledge gaps and rising to the challenge from the International Bicuspid Aortic Valve Consortium (BAVCon). Circulation 129 (25), 2691–2704. doi:10.1161/circulationaha.113.007851
Milewicz D. M., Guo D. C., Tran-Fadulu V., Lafont A. L., Papke C. L., Inamoto S., et al. (2008). Genetic basis of thoracic aortic aneurysms and dissections: focus on smooth muscle cell contractile dysfunction. Annu. Rev. Genomics Hum. Genet. 9, 283–302. doi:10.1146/annurev.genom.8.080706.092303
Milewicz D. M., Prakash S. K., Ramirez F. (2017). Therapeutics targeting drivers of thoracic aortic aneurysms and acute aortic dissections: insights from predisposing genes and mouse models. Annu. Rev. Med. 68, 51–67. doi:10.1146/annurev-med-100415-022956
Minderhoud S. C. S., Arrouby A., van den Hoven A. T., Bons L. R., Chelu R. G., Kardys I., et al. (2024). Regional aortic wall shear stress increases over time in patients with a bicuspid aortic valve. J. Cardiovasc Magn. Reson 26 (2), 101070. doi:10.1016/j.jocmr.2024.101070
Minderhoud S. C. S., Roos-Hesselink J. W., Chelu R. G., Bons L. R., van den Hoven A. T., Korteland S. A., et al. (2022). Wall shear stress angle is associated with aortic growth in bicuspid aortic valve patients. Eur. Heart J. Cardiovasc Imaging 23 (12), 1680–1689. doi:10.1093/ehjci/jeab290
Modica G., Sollazzo F., Bianco M., Cammarano M., Pella R., Monti R., et al. (2022). Bicuspid aortic valve and premature ventricular beats in athletes. Int. J. Environ. Res. Public Health 19 (19), 12188. doi:10.3390/ijerph191912188
Mokashi S. A., Rosinski B. F., Desai M. Y., Griffin B. P., Hammer D. F., Kalahasti V., et al. (2022). Aortic root replacement with bicuspid valve reimplantation: are outcomes and valve durability comparable to those of tricuspid valve reimplantation? J. Thorac. Cardiovasc Surg. 163 (1), 51–63.e5. doi:10.1016/j.jtcvs.2020.02.147
Morris S. A., Flyer J. N., Yetman A. T., Quezada E., Cappella E. S., Dietz H. C., et al. (2024). Cardiovascular management of aortopathy in children: a scientific statement from the American heart association. Circulation 150 (11), e228–e254. doi:10.1161/cir.0000000000001265
Murphy I. G., Collins J., Powell A., Markl M., McCarthy P., Malaisrie S. C., et al. (2017). Comprehensive 4-stage categorization of bicuspid aortic valve leaflet morphology by cardiac MRI in 386 patients. Int. J. Cardiovasc Imaging 33 (8), 1213–1221. doi:10.1007/s10554-017-1107-1
Nataatmadja M., West M., West J., Summers K., Walker P., Nagata M., et al. (2003). Abnormal extracellular matrix protein transport associated with increased apoptosis of vascular smooth muscle cells in marfan syndrome and bicuspid aortic valve thoracic aortic aneurysm. Circulation 108 (Suppl. 1), Ii329–334. doi:10.1161/01.cir.0000087660.82721.15
Nguyen S. N., Yamabe T., Zhao Y., Kurlansky P. A., George I., Smith C. R., et al. (2021). Bicuspid-associated aortic root aneurysm: mid to long-term outcomes of David V versus the bio-bentall procedure. Semin. Thorac. Cardiovasc Surg. 33 (4), 933–943. doi:10.1053/j.semtcvs.2021.02.004
Niaz T., Fernandes S. M., Sanders S. P., Michelena H., Hagler D. J. (2020). Clinical history and management of bicuspid aortic valve in children and adolescents. Prog. Cardiovasc Dis. 63 (4), 425–433. doi:10.1016/j.pcad.2020.05.012
Nigro P., Abe J., Berk B. C. (2011). Flow shear stress and atherosclerosis: a matter of site specificity. Antioxid. Redox Signal 15 (5), 1405–1414. doi:10.1089/ars.2010.3679
Oechtering T. H., Sieren M. M., Hunold P., Hennemuth A., Huellebrand M., Scharfschwerdt M., et al. (2020). Time-resolved 3-dimensional magnetic resonance phase contrast imaging (4D Flow MRI) reveals altered blood flow patterns in the ascending aorta of patients with valve-sparing aortic root replacement. J. Thorac. Cardiovasc Surg. 159 (3), 798–810. doi:10.1016/j.jtcvs.2019.02.127
Osawa M., Masuda M., Harada N., Lopes R. B., Fujiwara K. (1997). Tyrosine phosphorylation of platelet endothelial cell adhesion molecule-1 (PECAM-1, CD31) in mechanically stimulated vascular endothelial cells. Eur. J. Cell Biol. 72 (3), 229–237.
Otto C. M., Nishimura R. A., Bonow R. O., Carabello B. A., Erwin J. P., Gentile F., et al. (2021). 2020 ACC/AHA guideline for the management of patients with valvular heart disease: executive summary: a report of the American college of cardiology/American heart association joint committee on clinical practice guidelines. Circulation 143 (5), e35–e71. doi:10.1161/cir.0000000000000932
Padang R., Enriquez-Sarano M., Pislaru S. V., Maalouf J. F., Nkomo V. T., Mankad S. V., et al. (2019). Coexistent bicuspid aortic valve and mitral valve prolapse: epidemiology, phenotypic spectrum, and clinical implications. Eur. Heart J. Cardiovasc Imaging 20 (6), 677–686. doi:10.1093/ehjci/jey166
Paruchuri V., Salhab K. F., Kuzmik G., Gubernikoff G., Fang H., Rizzo J. A., et al. (2015). Aortic size distribution in the general population: explaining the size paradox in aortic dissection. Cardiology 131 (4), 265–272. doi:10.1159/000381281
Peper E. S., van Ooij P., Jung B., Huber A., Gräni C., Bastiaansen J. A. M. (2022). Advances in machine learning applications for cardiovascular 4D flow MRI. Front. Cardiovasc Med. 9, 1052068. doi:10.3389/fcvm.2022.1052068
Pisano C., Maresi E., Balistreri C. R., Candore G., Merlo D., Fattouch K., et al. (2012). Histological and genetic studies in patients with bicuspid aortic valve and ascending aorta complications. Interact. Cardiovasc Thorac. Surg. 14 (3), 300–306. doi:10.1093/icvts/ivr114
Preventza O., Coselli J. S., Price M. D., Simpson K. H., Yafei O., de la Cruz K. I., et al. (2017). Elective primary aortic root replacement with and without hemiarch repair in patients with no previous cardiac surgery. J. Thorac. Cardiovasc Surg. 153 (6), 1402–1408. doi:10.1016/j.jtcvs.2016.10.076
Raghav V., Barker A. J., Mangiameli D., Mirabella L., Markl M., Yoganathan A. P. (2018). Valve mediated hemodynamics and their association with distal ascending aortic diameter in bicuspid aortic valve subjects. J. Magn. Reson Imaging 47 (1), 246–254. doi:10.1002/jmri.25719
Rashad S., Han X., Saqr K., Tupin S., Ohta M., Niizuma K., et al. (2020). Epigenetic response of endothelial cells to different wall shear stress magnitudes: a report of new mechano-miRNAs. J. Cell Physiol. 235 (11), 7827–7839. doi:10.1002/jcp.29436
Regalado E. S., Mellor-Crummey L., De Backer J., Braverman A. C., Ades L., Benedict S., et al. (2018). Clinical history and management recommendations of the smooth muscle dysfunction syndrome due to ACTA2 arginine 179 alterations. Genet. Med. 20 (10), 1206–1215. doi:10.1038/gim.2017.245
Roberts W. C., Ko J. M. (2005). Frequency by decades of unicuspid, bicuspid, and tricuspid aortic valves in adults having isolated aortic valve replacement for aortic stenosis, with or without associated aortic regurgitation. Circulation 111 (7), 920–925. doi:10.1161/01.Cir.0000155623.48408.C5
Rodríguez-Palomares J. F., Dux-Santoy L., Guala A., Galian-Gay L., Evangelista A. (2023). Mechanisms of aortic dilation in patients with bicuspid aortic valve: JACC state-of-the-art review. J. Am. Coll. Cardiol. 82 (5), 448–464. doi:10.1016/j.jacc.2022.10.042
Rodríguez-Palomares J. F., Dux-Santoy L., Guala A., Kale R., Maldonado G., Teixidó-Turà G., et al. (2018). Aortic flow patterns and wall shear stress maps by 4D-flow cardiovascular magnetic resonance in the assessment of aortic dilatation in bicuspid aortic valve disease. J. Cardiovasc Magn. Reson 20 (1), 28. doi:10.1186/s12968-018-0451-1
Rose M. J., Rigsby C. K., Berhane H., Bollache E., Jarvis K., Barker A. J., et al. (2019). 4-D flow MRI aortic 3-D hemodynamics and wall shear stress remain stable over short-term follow-up in pediatric and young adult patients with bicuspid aortic valve. Pediatr. Radiol. 49 (1), 57–67. doi:10.1007/s00247-018-4257-y
Ruzmetov M., Shah J. J., Fortuna R. S., Welke K. F. (2015). The association between aortic valve leaflet morphology and patterns of aortic dilation in patients with bicuspid aortic valves. Ann. Thorac. Surg. 99 (6), 2101–2107. doi:10.1016/j.athoracsur.2015.02.036
Saeyeldin A., Zafar M. A., Li Y., Tanweer M., Abdelbaky M., Gryaznov A., et al. (2019). Decision-making algorithm for ascending aortic aneurysm: effectiveness in clinical application? J. Thorac. Cardiovasc Surg. 157 (5), 1733–1745. doi:10.1016/j.jtcvs.2018.09.124
Shan Y., Li J., Wang Y., Wu B., Barker A. J., Markl M., et al. (2017). Aortic shear stress in patients with bicuspid aortic valve with stenosis and insufficiency. J. Thorac. Cardiovasc Surg. 153 (6), 1263–1272. doi:10.1016/j.jtcvs.2016.12.059
Shan Y., Li J., Wang Y., Wu B., Barker A. J., Markl M., et al. (2019). Aortic stenosis exacerbates flow aberrations related to the bicuspid aortic valve fusion pattern and the aortopathy phenotype. Eur. J. Cardiothorac. Surg. 55 (3), 534–542. doi:10.1093/ejcts/ezy308
Sherrah A. G., Andvik S., van der Linde D., Davies L., Bannon P. G., Padang R., et al. (2016). Nonsyndromic thoracic aortic aneurysm and dissection: outcomes with marfan syndrome versus bicuspid aortic valve aneurysm. J. Am. Coll. Cardiol. 67 (6), 618–626. doi:10.1016/j.jacc.2015.11.039
Sievers H. H., Schmidtke C. (2007). A classification system for the bicuspid aortic valve from 304 surgical specimens. J. Thorac. Cardiovasc Surg. 133 (5), 1226–1233. doi:10.1016/j.jtcvs.2007.01.039
Sievers H. H., Stierle U., Mohamed S. A., Hanke T., Richardt D., Schmidtke C., et al. (2014). Toward individualized management of the ascending aorta in bicuspid aortic valve surgery: the role of valve phenotype in 1362 patients. J. Thorac. Cardiovasc Surg. 148 (5), 2072–2080. doi:10.1016/j.jtcvs.2014.04.007
Siu S. C., Silversides C. K. (2010). Bicuspid aortic valve disease. J. Am. Coll. Cardiol. 55 (25), 2789–2800. doi:10.1016/j.jacc.2009.12.068
Sophocleous F., Milano E. G., Pontecorboli G., Chivasso P., Caputo M., Rajakaruna C., et al. (2018). Enlightening the association between bicuspid aortic valve and aortopathy. J. Cardiovasc Dev. Dis. 5 (2), 21. doi:10.3390/jcdd5020021
Sotelo J., Franco P., Guala A., Dux-Santoy L., Ruiz-Muñoz A., Evangelista A., et al. (2022). Fully three-dimensional hemodynamic characterization of altered blood flow in bicuspid aortic valve patients with respect to aortic dilatation: a finite element approach. Front. Cardiovasc Med. 9, 885338. doi:10.3389/fcvm.2022.885338
Soto-Navarrete M. T., López-Unzu M., Durán A. C., Fernández B. (2020). Embryonic development of bicuspid aortic valves. Prog. Cardiovasc Dis. 63 (4), 407–418. doi:10.1016/j.pcad.2020.06.008
Soulat G., Scott M. B., Allen B. D., Avery R., Bonow R. O., Malaisrie S. C., et al. (2022). Association of regional wall shear stress and progressive ascending aorta dilation in bicuspid aortic valve. JACC Cardiovasc Imaging 15 (1), 33–42. doi:10.1016/j.jcmg.2021.06.020
Stefek H. A., Berhane H., Robinson J. D., Reilly B., Ruh A., Markl M., et al. (2019). Comprehensive MR analysis of cardiac function, aortic hemodynamics and left ventricular strain in pediatric cohort with isolated bicuspid aortic valve. Pediatr. Cardiol. 40 (7), 1450–1459. doi:10.1007/s00246-019-02157-5
Stephens E. H., Hope T. A., Kari F. A., Kvitting J. P., Liang D. H., Herfkens R. J., et al. (2015). Greater asymmetric wall shear stress in Sievers' type 1/LR compared with 0/LAT bicuspid aortic valves after valve-sparing aortic root replacement. J. Thorac. Cardiovasc Surg. 150 (1), 59–68. doi:10.1016/j.jtcvs.2015.04.020
Thompson M. A., Kramer B., Tarraf S. A., Vianna E., Gillespie C., Germano E., et al. (2023). Age is superior to aortopathy phenotype as a predictor of aortic mechanics in patients with bicuspid valve. J. Thorac. Cardiovasc Surg. 169, 531–541.e4. doi:10.1016/j.jtcvs.2023.12.018
Thunström S., Thunström E., Naessén S., Berntorp K., Laczna Kitlinski M., Ekman B., et al. (2023). Aortic size predicts aortic dissection in Turner syndrome - a 25-year prospective cohort study. Int. J. Cardiol. 373, 47–54. doi:10.1016/j.ijcard.2022.11.023
Trenti C., Fedak P. W. M., White J. A., Garcia J., Dyverfeldt P. (2024). Oscillatory shear stress is elevated in patients with bicuspid aortic valve and aortic regurgitation: a 4D flow cardiovascular magnetic resonance cross-sectional study. Eur. Heart J. Cardiovasc Imaging 25 (3), 404–412. doi:10.1093/ehjci/jead283
Tzemos N., Therrien J., Yip J., Thanassoulis G., Tremblay S., Jamorski M. T., et al. (2008). Outcomes in adults with bicuspid aortic valves. Jama 300 (11), 1317–1325. doi:10.1001/jama.300.11.1317
van Ooij P., Garcia J., Potters W. V., Malaisrie S. C., Collins J. D., Carr J. C., et al. (2016). Age-related changes in aortic 3D blood flow velocities and wall shear stress: implications for the identification of altered hemodynamics in patients with aortic valve disease. J. Magn. Reson Imaging 43 (5), 1239–1249. doi:10.1002/jmri.25081
van Ooij P., Markl M., Collins J. D., Carr J. C., Rigsby C., Bonow R. O., et al. (2017). Aortic valve stenosis alters expression of regional aortic wall shear stress: new insights from a 4-dimensional flow magnetic resonance imaging study of 571 subjects. J. Am. Heart Assoc. 6 (9), e005959. doi:10.1161/jaha.117.005959
von Knobelsdorff-Brenkenhoff F., Trauzeddel R. F., Barker A. J., Gruettner H., Markl M., Schulz-Menger J. (2014). Blood flow characteristics in the ascending aorta after aortic valve replacement--a pilot study using 4D-flow MRI. Int. J. Cardiol. 170 (3), 426–433. doi:10.1016/j.ijcard.2013.11.034
Weiss E. K., Jarvis K., Maroun A., Malaisrie S. C., Mehta C. K., McCarthy P. M., et al. (2023). Systolic reverse flow derived from 4D flow cardiovascular magnetic resonance in bicuspid aortic valve is associated with aortic dilation and aortic valve stenosis: a cross sectional study in 655 subjects. J. Cardiovasc Magn. Reson 25 (1), 3. doi:10.1186/s12968-022-00906-9
Yang L. T., Tribouilloy C., Masri A., Bax J. J., Delgado V., Girdauskas E., et al. (2020). Clinical presentation and outcomes of adults with bicuspid aortic valves: 2020 update. Prog. Cardiovasc Dis. 63 (4), 434–441. doi:10.1016/j.pcad.2020.05.010
Yang L. T., Ye Z., Wajih Ullah M., Maleszewski J. J., Scott C. G., Padang R., et al. (2023). Bicuspid aortic valve: long-term morbidity and mortality. Eur. Heart J. 44 (43), 4549–4562. doi:10.1093/eurheartj/ehad477
Yassine N. M., Shahram J. T., Body S. C. (2017). Pathogenic mechanisms of bicuspid aortic valve aortopathy. Front. Physiol. 8, 687. doi:10.3389/fphys.2017.00687
Ye Z., Lane C. E., Beachey J. D., Medina-Inojosa J., Galian-Gay L., Dentamaro I., et al. (2023). Clinical outcomes in patients with bicuspid aortic valves and ascending aorta ≥50 mm under surveillance. JACC Adv. 2 (8), 100626. doi:10.1016/j.jacadv.2023.100626
Yeats B. B., Galvez D., Sivakumar S. K., Holst K., Polsani V., Yadav P. K., et al. (2024). 3D characterization of the aortic valve and aortic arch in bicuspid aortic valve patients. Ann. Biomed. Eng. 52 (8), 2258–2268. doi:10.1007/s10439-024-03527-8
Yoshioka Y., Yajima S., Sakaniwa R., Tadokoro N., Kainuma S., Kawamoto N., et al. (2023). Does the residual aorta dilate after replacement of the bicuspid aortic valve and ascending aorta? J. Thorac. Dis. 15 (3), 994–1008. doi:10.21037/jtd-22-1118
Keywords: bicuspid aortic valve, WSS, aortopathy, hemodynamics, aortic dilation, paediatric BAV
Citation: Yang M, Nie Z, Yue H, Liang W and Wu Z (2025) Aortopathy associated with bicuspid aortic valve: advances in clinical and hemodynamics research. Front. Physiol. 16:1576072. doi: 10.3389/fphys.2025.1576072
Received: 13 February 2025; Accepted: 07 April 2025;
Published: 06 May 2025.
Edited by:
Alberto Zullo, University of Sannio, ItalyReviewed by:
Aike Qiao, Beijing University of Technology, ChinaLucia La Mura, Federico II University Hospital, Italy
Copyright © 2025 Yang, Nie, Yue, Liang and Wu. This is an open-access article distributed under the terms of the Creative Commons Attribution License (CC BY). The use, distribution or reproduction in other forums is permitted, provided the original author(s) and the copyright owner(s) are credited and that the original publication in this journal is cited, in accordance with accepted academic practice. No use, distribution or reproduction is permitted which does not comply with these terms.
*Correspondence: Zhong Wu, d3V6aG9uZzcxQHNjdS5lZHUuY24=; Weitao Liang, bGlhbmctd2VpdGFvQHFxLmNvbQ==
†These authors have contributed equally to this work and share first authorship
‡These authors share senior authorship
§These authors share last authorship