Future scenarios for the infrastructure digitalization: The road ahead
- 1Network Operations, Telecom Italia, Rome, Italy
- 2Technology Innovation Institute, Autonomous Robotics Research Center, Abu Dhabi, United Arab Emirates
Nowadays, every industry across the globe is facing a disruptive transformation enabled by digitalization processes. Also, the industry of infrastructures, such as telecom, energy, transportation, and water systems, will be reshaped by the digitalization of physical assets mainly enabled by IoT technologies. An outstanding role in this scenario will be played by digital platforms, which will mediate the provisioning of services based on infrastructure. This process can revolutionize the industry-changing business models, unlocking great opportunities and posing complex challenges. In this paper, we investigate digitalization and the platformization processes and how they affect the infrastructures. We describe the models related to these processes in a systematic and interdisciplinary way and provide a comprehensive survey on technological, economic, and social issues. As a result of the research, we discuss the opportunities and risks of the processes, and we propose several approaches to address them.
1 Introduction
The last few years have seen an explosion of the Internet of Things (IoT) paradigm in both Academia and Industry, thanks to the several advantages in different application scenarios Tedeschi et al. (2020). Most of the time, the deployment of an IoT network requires a platform to manage and log the data acquired from the sensors. Many proposals for platforms such as smart housing, smart agriculture, smart city, or even smart driving have been proposed Fahmideh and Zowghi (2020).
Therefore the diffusion of IoT technologies, such as 5G, is expected to transform homes, buildings, and cities into “smart homes”, “smart buildings”, and “smart cities”. Also, critical infrastructures, which are the base for essential service provisioning, are being transformed into “smart infrastructures” thanks to their digitalization through the IoT paradigm. IoT devices are going to extract data from the physical infrastructure, and these data are going to be used by platforms to manage the infrastructures themselves Muciaccia (2020).
As a consequence, infrastructures are undergoing a process of “platformization” by opening their architectural and governance control points through digitization. At the same time, digital platforms are undergoing a process of “infrastructuring” by making them more physical, while expanding their reach and scope Constantinides et al. (2018).
These processes are worthy of study since the economic stability and competitiveness of a nation are based on properly functioning infrastructure systems. Indeed, the entire life cycle of infrastructures generally has a significant effect on economic growth Oyedele (2014a).
According to the Organisation for Economic Co-operation and Development (OECD) definition, the term “infrastructure” is defined as “the system of public works in a country, state or region, including roads, utility lines, and public buildings”. In general, definitions of “infrastructure” deal with basic physical structures and assets providing essential services to society Inderst (2020).
Infrastructures have commonly been divided into two broad categories: economic and social. Economic infrastructures consist of assets that support commerce and for which a fee is typically charged; it includes transportation (e.g., ports, airports, roads, bridges, tunnels, parking), utilities (e.g., energy distribution networks, storage, power generation, water, sewage, waste) and communications (e.g., transmission medium, cable networks, towers, satellites). On the contrary, the social infrastructure comprises schools and education facilities, healthcare facilities, affordable and social housing, defense and judicial buildings, prisons, government buildings, cultural and recreational buildings (libraries, museums, stadiums, public parks) Oyedele (2014b); Inderst (2020). Economic infrastructure is also defined as “engineering infrastructure”that comprises all transportation systems, electrical generation, transmission and distribution, pipelines, recreation, and telecommunications. Infrastructure can be further classified into utility and non-utility infrastructures: utility infrastructure, such as electricity, water, and gas, provide essential services to communities but are subject to strict regulation by government-related entities; non-utility infrastructure assets include social infrastructure and economic infrastructures, such as transportation and communication assets which can generate surplus returns through increased volume growth even if there are controls on price increases Oyedele (2014b).
The services generated from infrastructure assets are usually consumed by both households and enterprises. Infrastructure is both a final consumption item and an intermediate consumption. Even if the infrastructure is not an end in itself, its quality and provision guarantee the delivery of essential services that enhance economic growth and contribute to the quality of life Oyedele (2014a,b).
The digitalization of infrastructures is leading to the fact the delivery of services based on them is going to be mediated by platforms: the following sections will show how this is happening and what consequences it could have.
Contribution. In this paper, we focus on the aforementioned theme by providing a comprehensive survey on technological, economic, and social issues faced when infrastructures are undergoing a process of digitalization and platformization. As a novel contribution, we describe the models, opportunities, and risks related to this process in a systematic and interdisciplinary way. Finally, we also outline several approaches to exploit the opportunities and mitigate the risks.
Methodolgy and State of the Art. The methodology applied for the work presented in this paper is both an inductive and deductive process. Mainly in the first part of the work, the general feature of platformization and digitalization have been inducted by the study of the working principles of about a dozen existing digital platforms in other industries: Airbnb, Uber, Alibaba, Facebook, Amazon, YouTube, Craiglist, eBay, Wikipedia, Twitter, Instagram, and Pinterest. Mainly in the second part of the work, once defined a general model, the main research question to be answered is how this model could be applied to the infrastructure industry. In order to answer this question and to predict the evolution of this industry in a deducted way, the peculiarity of infrastructures has been analyzed, taking into account recent trends and several examples, e.g., the telecommunication industry. Some decades of papers and reports have been analyzed in the study, as reported in the reference section; each of them focuses on a particular scope or discipline: the literature gap intended to be covered in a multidisciplinary and comprehensive study of this phenomenon in order to enable a complete understanding and awareness and a better policy and decision making. To the best of our knowledge, there is still a literature gap in this field which is really important to be addressed to manage future economic, technological, and social challenges.
Roadmap. The rest of this paper is organized as follows. Section 2 introduces the IoT technologies, the definition of platform, and the platform enabling the infrastructure platformization; Section 3 introduces the proposed framework, focusing on platform features and modeling and describing the process of infrastructure platformization; Section 4 analyzes the opportunities and the economical and social risks of the platformization; Section 5 outlines several proposals to achieve financial and social sustainability; and, finally, in Section 7 we tighten some conclusions.
2 Background and technological enabling factors
2.1 Overview of IoT enabling technologies
Nowadays, wireless technologies enable several IoT applications, such as remote tracking, smart parking, waste management, environmental monitoring, real-time health, fitness monitoring, and public safety, to name a few. A network of IoT devices can be represented as a set of wireless nodes and distributed sensors that communicate with each other to detect, share and process the acquired data in the surrounding environment. Typically, a node is provided with different types of sensors, and usually, it is equipped with a battery. The primary goal of a deployed IoT network consists of collecting the data and forwarding them by leveraging a communication technology.
Figure 1 depicts a generic IoT architecture typically defined by three main components: sensors or actuators, a gateway, and a cloud-based platform.
• Sensors/Actuators: These IoT devices can sense or measure different parameters such as temperature, humidity, light, sound, motion, etc. They are equipped with microcontrollers and wireless transceiver modules to enable data communication over a wireless network. Further, the crucial aspects are memory, battery lifetime, and processing constraints.
• Gateway: It is an intermediary node between the IoT devices and the cloud. The goal of a gateway is to collect data from the IoT devices, perform basic processing on the data, and forward the data to the cloud. Further, it can be adopted for filtering, aggregation, and security operations.
• Cloud: This component analyses the data received from the Gateway. It stores and processes the data for further analysis. It can be configured to run machine/deep learning techniques to detect potential patterns and outliers Li et al. (2015).
The main wireless technologies to enable the IoT paradigm and allow the infrastructure platformization relies on well-defined communication standards such as IEEE 802.11ah, IEEE 802.15.1, IEEE 802.15.4, LoRa and LoRaWAN, SigFox, RFID, and 5G Samie et al. (2016).
For example, the IEEE 802.15.4 Baronti et al. (2007) standard is designed for control and sensor networks. It defines the PHY and the MAC layers to operate at frequencies of 868 MHz, 902–928 MHz, and 2.4 GHz with a data rate of 250 kbps. These features allow for achieving an efficient battery lifetime and moderate energy consumption. The communication range
On the same 2.4 GHz band frequency, Bluetooth Low Energy (BLE) Cäsar et al. (2022) is an ultra-low-energy communication technology that enables several applications in the IoT domain. It was introduced by the Bluetooth Special Interest Group in version 4.0 of Bluetooth based on IEEE 802.15.1. The data rate and the power consumption are 2 Mbit/s and 0.01 ∼ 0.50 W, respectively, as well as the nominal maximum communication range, which is
The technologies such as LoRa and LoRaWAN Ertürk et al. (2019) define the PHY layer and the MAC layers to operate at the frequency 863–870 MHz and 433 MHz, respectively. According to the LoRa standard, the communication range is up to 5 km in urban areas and 15 km in rural areas in Line of Sight (LoS) conditions, while the data rate varies from 300 bps up to 37.5 kbps.
Further, Sigfox Zuniga and Ponsard (2016) is a narrow-band Low-Power Wide Area Network (LPWAN) proprietary protocol that uses the unlicensed Industrial, Scientific and Medical (ISM) frequencies bands, i.e., 868 ∼ 869 MHz and 902 ∼ 928 MHz with a data rate of 100 bps in uplink to 600 bps in downlink. The Sigfox protocol stack consists of PHY, MAC, Frame, and Application layers. It is worth mentioning that Sigfox frames are not encrypted by design.
WLAN technologies will also play a fundamental role in the IoT and M2M. To decrease the energy consumption, the IEEE 802.11ah Adame et al. (2014) groups proposed specifications for PHY and MAC layers for the Sub-GHZ ISM band. Compared to the IEEE 802.11ac standard, several features have been added, such as the extended coverage of 1 km and a data rate of 10 kbps.
Narrow-Band IoT (NB-IoT) Ratasuk et al. (2016) is a LPWAN standard radio protocol designed for wide-range communications with a keen eye on low-power consumption and bandwidth management. This technology has a channel bandwidth of 200 kHz but occupies only 180 kHz. NB-IoT defines 26 frequency bands in total, where most of them are in the sub-GHz frequency range and above the 1.8 GHz. NB-IoT standard allows transfers up to 250 kbit with latency from 1.6 to 10 s. NB-IoT can work in three different ways such as i) the GSM band (standalone), ii) the LTE band (in-band), or iii) the spacing between the LTE band, thus maximizing the communication spectrum (guard band).
Finally, the fifth Generation or 5G Li et al. (2018) defines the next step toward mobile communication technology after 1G, 2G, 3G, and 4G networks. It is approved by the third Generation Partnership Project (3GPP), and it promises low latency of 1 m and data rates up to 20 Gbps. The band spectrum is defined between 30 GHz and 300 GHz for mmWave. Further, 5G investigated the possibility of adopting a lower-frequency spectrum for 5G networks such as 600 ∼ 700 MHz, or 2.5 ∼ 3.5 GHz. The 5G market is expected to grow up to 720 billion USD dollars by 2030.
2.2 Platforms
The concept of “platform” has been adopted for a long time to characterize products, services, firms, or institutions that mediate transactions between two or more groups of agents Rochet and Tirole (2003). Technically speaking, platform architectures are defined as complex modular systems in which certain components (the platform itself) remain stable while others (the complements) vary in cross-section or over time. The most stable elements in a platform architecture are the modular interfaces mediating between the platform and its complements since the control over the interfaces allows the control over the platform and its evolution Baldwin and Woodard (2009). Extending the concept of “platform” to the economic field, according to Parker et al. in Parker et al. (2016), it can be defined as a “business based on enabling value-creating interactions between external producers and consumers“. A platform sets the rules for these interactions; in fact, its purpose is to build a relationship among users and facilitate the exchange of goods and services, enabling value creation for all participants.
2.3 IoT platforms
The exponential growth of the IoT and Industrial IoT devices requires a platform that collects, processes, archives, manages, and analyzes all the acquired data. Sensors, autonomous vehicles, robots, CCTV cameras, and any “smart“ object that needs to interact with the surrounding environment and exchange data in real time should be interconnected with a smart platform. An IoT platform guarantees cooperation with heterogeneous devices, it provides the requirements to protect and secure the collected data, and it can support trusted resource sharing among different IoT platforms.
At the same time, companies and international institutions are developing communication standards and protocols for Machine To Machine (M2M), industrial IoT, body area networks, and so on. Nowadays, the fragmentation of the IoT ecosystem, the emergent heterogeneous application-specific platforms, and the poor cooperation between the IoT platforms need a solution that eases communication with each other.
In an IoT architecture, data i) are generated by sensors and actuators, such as proximity, inductive, temperature, capacitive, accelerometers, and infrared (PIR) sensors; ii) they are sent and aggregated by the gateway (or an edge node) that pre-processes and organizes them, and finally iii) they are stored for analysis in a proprietary data center or, in a cloud environment. Once uploaded, machine learning techniques and artificial intelligence algorithms are also adopted to predict and deduce correlations for business objectives as well as failures before they occur.
Security is also an important cornerstone to guarantee the success of IoT platforms. Each proposed solution needs to protect also the privacy of data and users against attacks and unauthorized access. Indeed, it is crucial to verify where the data are stored and if the platform provides data encryption as well as is compliant with the security standards.
Some examples of the most popular IoT platforms are Amazon AWS IoT Core, Cisco Kinetic IoT platform, Google Cloud IoT, Microsoft Azure IoT Suite, Arduino Cloud IoT, OpenRemote, and ThingsBoard, adopted for i) real-time monitoring of the production processes, ii) the analysis of the product life-cycle and iii) the maintenance of supply chains Muhammed and Ucuz (2020).
3 Framework description
IoT platforms are just an example of digital platforms. The platform-based business model is progressively invading every industry and has led several start-up companies to conquer the role of the market leader in a very short time. Platforms such as Airbnb, Uber, Alibaba, Facebook, Amazon, YouTube, eBay, Wikipedia, Twitter, Instagram, and Pinterest are just a few examples of “platform power” derived from the exploitation of technology to put in touch with people, resources, and organizations in an interactive ecosystem van der Aalst et al. (2019).
Platforms are already introducing radical changes in the economy and society, but this transformation is supposed to be still deeper when the platform business model is increasingly extended to the infrastructure industry by exploiting the widespread deployment of IoT. In this section, we provide a schematic framework to conceptualize the working principles of the current digital platforms and how these can be eventually extended to future platform-based infrastructures.
3.1 Platform features
Three main features are common to all the platforms: network effects, scalability, and information-intensity. Network effects refer to the impact that the number of users’ platform has on the value created for each user: “positive” network effects refer to the ability of a large and well-managed platform community to produce significant value for each user of the platform. On the contrary, “negative” network effects refer to the risk that the value for users may also decrease if the growth in the number of users is not managed properly. To reduce this risk, the platform managers take care of the quality of the relationships that are established among users to increase the chances of creating a satisfactory relationship between producers and consumers on the platform. In this way, the platform increases its value at the same speed as the exponentially growing communities. An analogy could be established between large enterprises in the Industrial Age and platforms in the Digital Age: the former exploit large economies of scale on the supply front, and the latter exploit economies of scale on the demand front enabled by network effects Parker et al. (2016).
High scalability is possible for platforms because they are based on disintermediation in the relationship between users or rather on the elimination of so-called gatekeepers. Before the advent of platforms, the most common business models were based exclusively on the pipeline scheme, which is a logical sequence of steps that led the products to be designed and manufactured at one end of the “pipe” and delivered to the customer at the opposite term. This type of process still exists in several industries, but when an operator introduces a platform architecture, the latter is destined to defeat any competitor quickly. The competitive advantage of platforms is based on their faster and more efficient capability to scale; whilst traditional pipelines need inefficient gatekeepers controlling the flow of value from producer to consumer, platforms unlock the value creation in the ecosystem by disintermediating the relationship between any producer and any consumer: only one new mediator is introduced, i.e., the platform itself. Therefore some of the industries most exposed to the platform revolution are the ones with expensive and not easily scalable gatekeepers: this is valid, for example, in the retail or in advertising industry where platforms such as eBay and Amazon allow millions of manufacturers to sell and advertise their products without the need for mediation, except to the one actuated by eBay and Amazon themselves Muciaccia (2020).
Finally, another important feature of platforms is high information intensity; in fact, platforms play a disruptive role in reducing fragmentation and information asymmetries. The first function is provided through market aggregation, entailing efficiencies and reduction of research costs among many small suppliers/producers. The second function is provided through the distribution of information to all users, generating a climate of widespread trust that facilitates commercial exchanges. Therefore the industries where information is the main source of value are the best candidate to be affected by “platformization” Parker et al. (2016). For this reason, the media and telecommunications industries were among the first ones who experienced the disruptive entry of the platforms.
3.2 Platform modeling
In synthesis, platforms introduce disruptive innovation in traditional industries by providing a sort of common base and ideally leveled playing field (in fact, that is the etymology of the word “plat-form”, a raised level surface), where providers/suppliers and consumers can share information and exchange goods and services. At a first approximation, it is possible to consider the platform as an intermediate layer between the producer layer and the consumer layer in a 3-layer stack model. To provide an example, we can consider the evolution of the traditional media industry. This industry is traditionally characterized by all the features described in the paragraph 3.1: i) it is information-intensive since the news are one of its main products; ii) it does not scale well because of an inefficient pipeline (printing, shipping, retailing, delivery) affected by high distribution costs and because of inefficient targeted advertising; iii) it is highly fragmented, considering the numerous players involved in such a complex pipeline; iv) it is high informative asymmetry since the producer can filter and elaborate news before being published and become known to readers. In the last 2 decades, this industry has been disrupted by digital platforms that operated a disaggregation (unbundling) and a successive re-aggregation (bundling) of journalistic content, public, and advertising Van Dijk et al. (2018).
For example, a newspaper is a product aggregating news and advertising in one single bundle; therefore, its customers are readers interested in news, and advertisers want to catch the attention of the readers. At the end of the 1990s, websites with classified advertising, such as Craiglist and eBay, started to appear, and in 2006 their ads sales surpassed the ones coming from newspapers. This fact started the unbundling process, which was reinforced by web search engines which rapidly gained a dominant market position in online advertising, reducing revenues for newspapers; they created a further disaggregation in the relationship between readers and content: the readers didn’t flip anymore through a mix of stories and ads, but they went directly to the story that interests them, in many cases bypassing the newspaper’s front page. Moreover, thanks to feed readers or headline aggregators, such as Google News, Digg, and Daylife, the readers could access the news even not aware of which newspaper’s site they arrived at: “each story becomes a separate product standing naked in” Carr (2008). The disaggregation process has been followed by the re-aggregation one: at that stage, platforms appeared on the scene. Whilst news aggregators use employees or algorithms to find and filter news edited from professional publishers, social media platforms allow any user to share content with every other user from any kind of source (consumers become prosumers). Therefore news is re-aggregated and arranged in a way established by platforms themselves Van Dijk et al. (2018). A simplified stack model for platformization in the media industry is illustrated in Figure 2: on the left is presented the old scenario with publishers directly interfacing with readers and advertisers; on the right, this relationship is mediated through an intermediate layer, i.e., the platform. The platformization process in the media industry can be generalized to many other industries. A similar stack model can be adopted in all the industries affected by platformization: on top of the bottom layer representing traditional players, digital platforms introduce an additional “unbundling” layer that mediates the relationship with customers. The platformizazion is a direct effect of the spreading of technologies for data generation, storage, communication, and analytics. These technologies enable the creation of a data layer capable of mirroring the physical value chain. Online platforms are establishing themselves as managers of this new data layer. Emerging power relationships and financial flows among these layers depend on the way platformization could happen: by substitution, i.e., platforms simply substitute the traditional player (e.g., emails against printed letters); by displacement, i.e., platforms create new services in competition with traditional players (e.g., Uber against taxis); or by commoditization, i.e., platforms build larger and more powerful network than the underlying players, considered as subdued suppliers along the value chain (e.g., Amazon marketplace against its merchants) Finger and Montero (2018).
The process described above is also valid for the infrastructure industry, such as energy, telecommunication, mobility, water management, and logistics. Sensors installed in the physical assets can capture data recreating the status (location, damage, etc.) and the usage (capacity, flows, etc.) of the infrastructure in the data layer built on top of the layer representing the traditional physical infrastructure itself. The data layer creates a “digital twin“ of the asset, not only mirroring the physical world but also identifying the opportunities to improve the organization of the system Montero and Finger (2021b). In the infrastructure industry, platformization happens by commoditization: the underlying infrastructures continue to exist but are duplicated in the data layer. In this industry, the peculiarity of the data layer is the fact that it works as an infrastructure itself: in fact, the data layer provides the foundation to offer and develop traditional and new services thanks to the algorithms implemented in the platform to elaborate the data collected Montero and Finger (2021a).
A simplified stack model for platformization in the infrastructure industry is depicted in Figure 3: on the left, the current scenario is shown, where service is provided directly based on the infrastructure; on the right, the future scenario is shown, where the data layer managed by the platform is interposed between the infrastructure and the services. This Figure has been inserted in order to enable a better and gradual understanding of the platform modeling; in particular, Figure 3 could be better understood by analogy with Figure 2, which shows a similar platform modeling in the media industry. Moreover, Figure 3 could be useful for the reader for a better comprehension of the evolution expected in the infrastructure industry as depicted in Figure 4 and described in the next section.
3.3 Infrastructure platformization
The introduction and the spread of platforms in the infrastructure industry are transforming the market deeply, with opportunities and risks presented in Section 4.
Today the market is characterized by oligopolistic features or by limited competition. Infrastructure providers are very few, typically operating at the national or regional level, and very often, they are directly or indirectly owned or controlled by the government. Sometimes, in fact, the infrastructure itself is considered a “natural monopoly“ since the building of a parallel or alternative infrastructure would be considered highly expensive, and therefore, the introduction of competition is considered inefficient because it would lead to higher costs.
Service providers are few and typically operate at the national or continental level in respect of a regulatory framework which can be particularly strict in the case of vertically integrated companies, i.e., whether one player plays the role of both infrastructure and service provider. In fact, in this case, especially if the infrastructure is considered a natural monopoly, price regulation is crucial to avoid the vertically integrated player exercising excessive market power on its retail and wholesale customers. Sometimes obligations and rules are introduced to compel a service provider to provide a kind of “universal service“, i.e., a baseline level of services to every country resident at reasonable and affordable prices. Both infrastructure and service providers are typically sharply sectorized. For example, a telco normally does not operate in the energy sector Finger and Montero (2018).
Infrastructure are long-term assets with long economic life, and public services based on them typically have a strongly non-elastic demand; therefore, the infrastructure market today is characterized by low technological risk, high entry barriers, fixed prices for end-users, and quite stable revenues and predictable cash flows. Moreover, the infrastructure value has a low correlation with traditional asset classes and overall economic performance and frequently represents a natural edge against inflation. Finally, it is worth noting that infrastructures have been typically managed by state-owned companies and have benefited from public investments, although in the latest decades, these have been reduced Inderst (2020).
In the future, after the digital transformation induced by platforms, it will be possible to envision that platform managers will configure themselves as new “digital“ service providers operating in a weak regulatory framework and in an almost monopolistic global market. New services and new business models could be created by these companies with a vertical integration between the data and service layers. It is also possible that, in some cases, end-users could be transformed into prosumers operating in competition with traditional service providers, as it is already happening in industries such as mobility (Uber), media (YouTube), and hospitality (Airbnb). Moreover, the new business models introduced by platforms will enable different ecosystems, i.e., a plethora of subjects establishing reciprocal interactions with each other and with the platform itself in a dynamic equilibrium that generates value for all. For instance, in the ecosystem of Airbnb, core and extension developers collaborate: the former depends directly on the platform and is entitled to the design, creation, and maintenance of the basic mechanisms for the interaction among hosts and guests; the latter belongs to third parties and develop a lot of other minor functionalities, such as applications facilitating guest check-in or adding cleaning services, travel, and dinner reservations or babysitting services. Similar ecosystems could be created around platforms managing infrastructures (e.g., smart roads, smart grids, smart cities) Parker et al. (2016).
Interaction among the players in the ecosystem will depend on interfaces. If the platform is open (with many standardized interfaces), third parties could cooperate for the continuous improvement of the platform itself, even if service quality would be challenging. Although new service providers could influence the evolution of the market, the key role will be played by platform managers since they will control the data layer; unlike infrastructure providers, with a high probability, they will be a generalist and private companies.
The simplified stack model for a possible future scenario about the platformization in the infrastructure industry is shown in Figure 4: on the bottom of the stack, infrastructure providers provide access to the infrastructures they own or manage; on top of them, several platforms are instantiated which could interface with one or more infrastructure providers; on the highest layer of the stack, services providers are interfaced with customers and citizens. Service providers can be the traditional ones or innovative ones; sometimes, they can be vertically integrated with platforms, and, in some cases, they can be even prosumers (for clarity’s sake, represented here, even if they can be considered small infrastructure providers).
4 Risk/opportunities analysis
Since technological enabling factors and the consequent platformization of the infrastructure market are evolving rapidly, it is urgent to analyze opportunities and risks emerging from this process. This analysis, enhanced by a democratic public debate, would allow policymakers (cities, government, institutions, and international organizations) to develop and actuate the best strategies to guide the digitalization of infrastructures in order to exploit all the benefits and mitigate the risks.
4.1 Opportunities
One of the greatest benefits introduced by platformization is efficiency. It is obtained by “sweating assets”, i.e., by optimizing the usage of physical resources and reducing waste. For instance, in the mobility industry, Uber uses big data and complex algorithms to develop a logistics program that allows Uber cars to maximize efficiency, picking up passengers wherever they go and minimizing the time spent waiting for new users by realizing a “perpetual ride”. Similarly, in the hotel industry, Airbnb aims to obtain the “perpetual lease” of hosts’ houses thanks to algorithms of pricing and the reputation of users and third parties Edelman and Geradin (2016).
The extension of platform efficiency could open interesting scenarios for infrastructures and public services: some cities in Florida and New Jersey are already subcontracting part of the public transportation to Uber, while Washington D.C. already uses it for transport services reserved for disabled people, preferring it to costly investment programs in new trains, bus lines or other forms of public transport Bria and Morozov (2018). Thanks to platforms, infrastructures can be leveraged with greater efficiency and reduced operational costs. Internet of Things is considered the first general technology platform in history that can potentially foster an economy with marginal costs close to zero. The virtuous intertwining of the Internet of communications, the Internet of energy, and the Internet of Logistics is pushing efficiency to the point where the marginal cost of numerous goods and services, or the cost of producing an additional unit of a good or service, depreciated the fixed costs, would have been almost zero, making them of done practically free, abundant and no longer subject to market forces Rifkin (2014).
Moreover, the information managed in the data layer can be used by the infrastructure provider for predictive maintenance resulting in increased resilience and extended life of the assets; this allows the infrastructure owner to avoid or delay expensive investments to renew the assets. Another important benefit triggered by platformization is innovation. Indeed, innovation is the result of the cooperation built in the ecosystem generated and fostered by the platform, enhanced by the information exchange between providers and consumers through the data layer. Innovation allows value creation potential for all the players in the ecosystem and for the platform owner. Creating new business models enables new monetization ways and stimulates the design and development of new services that can address new needs or problems or propose new solutions to pre-existing needs and problems.
Thanks to platformization, the physical asset can be used for uses other than those intended by the owner, and its usage can be exchanged on a secondary market, generating efficiency and creating value. In this way, a product or service that is sold in a Business to Consumer environment can be converted into a platform that operates in a Business to Business area. One of the techniques used by platforms to obtain this effect is de-linking assets from value, i.e., decoupling the ownership of the physical assets from the value they create.
An example of this de-linking technique in the energy industry is the proposal submitted to the New York State Energy Research and Development Authority in 2016 for the development of a platform to integrate different distributed energy sources, such as photovoltaic panels, domestic generators, battery accumulators, and “virtual” accumulators into the national electricity grid. The purpose of the platform is to enable smart management of the fluctuations in the demand and supply of electricity over the course of a day or the cycle of seasons. To this aim, all resources should be managed in a coordinated manner by creating a dynamic tariff system and a platform that allows all the subjects involved (energy producers and consumers) to respond on the basis of information relating to local variations in the supply and demand of energy. The platform has to separate the ownership of the physical asset, i.e., the distribution network and the various energy sources, from the value they create, i.e., the energy produced. Such a system could lead to the creation of small energy sellers who sell to large buyers who, in turn, interface with end-users; the benefits that would be obtained would be linked to delaying or canceling substantial investments in a new transmission, distribution and generation plants, to stimulate the production of electricity from renewable sources and, ultimately, to reducing energy waste Tabors (2016).
Both efficiency and innovation introduced by platforms enable the generation of positive externalities. It is to understand how platforms facilitate the meeting between supply and demand. This aspect determines benefits for suppliers (infrastructure, platform, and service providers), which realize revenues, and for the end customers, who draw various benefits from the purchased services. Moreover, the introduction of platforms brings advantages to the environment in which suppliers and buyers act, for example, to the entire production system or to the community. Positive externalities are typical of “general-purpose technology”, such as telecommunications, electricity, and combustion engines, which create drastic discontinuities due to their nature of “enabling technologies“. Further, they are distinguished by three features: they have i) a pervasive use that affects many industries; ii) a remarkable technological dynamism which is the consequence of their ability to stimulate innovation; iii) they bring complementary innovations to the sectors in which they are applied and then change the same technologies. Digital platforms can be considered “general-purpose technology” because they fulfill all these features; this is even more valid for the platforms aiming to manage infrastructures since infrastructures themselves can be considered as “general-purpose technologies” Bresnahan and Trajtenberg (1995).
One of the most relevant positive externalities which can be induced by infrastructure platformization consists of reducing the human impact on the environment. In fact, the efficiency introduced by platforms in conventional processes can also be referred to as more efficient usage of natural resources. From the platform, efficiency descends other kinds of positive externalities in terms of social advantages. Whether operational costs are reduced for infrastructure providers, end-users also benefit from it since they can benefit from lower rates and fees thanks to the effect of the economy of scale. Besides the increased service affordability, another social advantage could be the enhanced productivity enabled by platforms allowing faster and less expensive transactions.
4.2 Risks
As opportunities offered by infrastructure platformization can be described in terms of “positive externalities”, risks can be symmetrically described in terms of “negative externalities”. Basically, they descend from the high probability that this kind of platform will be owned and managed by private companies operating in a sort of private global monopolistic/oligopolistic regime. Private ownership and management could imply a lack of long-term vision and insufficient coordination with public policies, whilst a monopolistic or oligopolistic regime could lead to excessive market power in the hands of such companies. This is a “per se” market failure situation since it destroys competition and could have negative consequences on prices in the whole ecosystem. In particular, the decoupling between the ownership of the asset and the value generated can undermine the possibility for the infrastructure provider to support significant investments to modernize its assets. Today infrastructure owners can rely on stable cash flows from the users of the infrastructures themselves; if platforms revolutionize the current business model, the value of the infrastructure could be drained by platforms, and thus the infrastructure owner’s revenues could be reduced to the advantage of the platform manager. If the financial sustainability of infrastructure providers is undermined, they will no more able to invest in the modernization of their assets, and the whole value chain could be affected.
This is essentially what is happening these years in the telecommunications industry. Well-known platform operators, such as Google and Facebook, appeared alongside telcos, the traditional players of the market, performing the roles of both infrastructure and service providers; in fact, platform operators have drained value from the network infrastructure. These new players, namely, Over The Tops (OTTs), have created a huge business by “platforming” the assets in which telcos have invested and are still investing but leaving the latter the ownership of the infrastructure with all the operational costs in terms of management and maintenance. Thus while the turnover of the OTTs rose, the operating margins of the telcos compressed. For instance, the overall Earnings Before Interest, Taxes, Depreciation and Amortization (EBITDA) of the telco sector in Italy fell from 15.8 billion euros in 2008 to 10.0 billion euros in 2020. In the meantime, the investments that became necessary to develop the new generation networks remained almost constant: annually in the last 10 years, the Italian telcos have invested a total of around 6 ∼ 7 billion euros per year. The difference between EBITDA and investments in relation to total revenues can be considered a good parameter to measure the sustainability of the business. This indicator dropped from 33% in 2008% to 9% in 2020 AssTel (2021). If this trend were to perpetuate, the possibility of making the necessary investments to build ultra-broadband 5G and fiber optics networks is put at risk. Moreover, these investments are indispensable for all parties in the supply chain, including OTTs, which benefit from the availability of ultra-broadband networks built by the telcos themselves. This issue has even more general implications since telecommunication infrastructures are one of the most relevant enabling factors for the whole digitalization process involving all other strategic industries. In other words, the data layer management risk undermining not only the financial stability of the underlying infrastructure layer but of the whole ecosystem, including the platform themselves, like the scorpion which stings the frog carrying itself across the rivers and kills them both in the ancient Aesop’s story. In order not to collapse under the pressure of platform providers and to avoid a market crunch, infrastructure providers need public investments or innovative ways to raise capital from private investors.
Economic risks are not the only ones to be taken into account; from the significant market power of the platform, managers also descend social risks, which are particularly relevant when platformization involves strategic infrastructure. Social risks are related to the impact that platforms could have on society through the values installed in them by their developers and managers. In the medium and long term, these values can have a deep influence not only on the ecosystem but on the whole of society since strategic infrastructures are the basis on which human society is based. Equity, security, privacy, and even democracy could be put at risk by platforms if their values are not aligned with those of an equitable, secure, privacy-compliant, and democratic society. This cannot be taken for granted if platforms are managed by global private monopolies.
Since platforms are going to operate in a global context, another risk implicated by platformization is the impact on the geopolitical equilibrium among countries or blocs of countries. The control of infrastructures has always been a way to gain or maintain political power; similarly, platforms managing infrastructures in a global scenario could be a way to affirm the domain of one country or bloc on the other ones. It could be envisioned that in the future, wars could no longer be the only way to acquire control of strategic infrastructures, but it would be sufficient to access data from them and build an efficient and effective data layer on them. The most powerful States are already beginning to compete and cooperate with global platforms to establish nothing less than a new world order where State-nations and democratic national institutions risk becoming less powerful than private hi-tech data-driven companies Van Dijk et al. (2018). Challenges will become harder with the rise of general artificial intelligence: the competition among States and platforms will be sharpened by the awareness that if the transition from a human artificial intelligence to superhuman intelligence is fast, the first project to reach this result will get a decisive strategic advantage unreachable for other projects Bostrom (2014). Nowadays, if a company (such as Google, Facebook, or Amazon) enjoys a strong position in an area where it is possible to collect data, this company has more information about individuals than any other company, even if it does not share them (or it incentivized to do that). In other words, artificial intelligence can increase the power market of platforms significantly Stiglitz (2020).
5 Approaches to sustainable solutions
In the previous section, we described opportunities and threats related to the platformization of infrastructures. It is clear that this process is built on a delicate unstable equilibrium among juxtaposed forces and different needs. In order to save and enforce the undeniable benefits introduced by platforms and contrast dangerous trends, a new and more stable equilibrium is highly desirable. In other words, innovative approaches to sustainable solutions are needed to mitigate both the economic risks (financial sustainability) and the social ones (social sustainability).
5.1 Financial sustainability
The research for innovative means of financing infrastructure has become crucial in the latest decades for different reasons, not only as a consequence of the process of platformization. In fact, an increasing infrastructure financing gap has emerged as a consequence of aging infrastructure, environmental factors such as climate change, and rising quality standards. Moreover, other infrastructure financing lacuna includes government fiscal budgetary constraints and historical underinvestment in crucial infrastructure projects Oyedele (2014b). Since the 1970s, developing countries have faced an increasing divergence in the supply and demand for infrastructure Yehoue et al. (2006). In particular, the investment in social infrastructure has received low attention from both politicians and investors despite growing concerns by the public. Social infrastructure has endured a long period of neglect in most developed and emerging countries, with chronic underinvestment exposed by the coronavirus crisis in 2020 Inderst (2020). The platformization of infrastructures is enlarging the financial gap because, on the one hand, it demands infrastructure providers to renew their assets in order to enable digitalization, and, on the other hand, it drains revenues through the interposition of a data layer between the infrastructure and the service layer.
The last global financial crisis in 2007/2008 and the consequent austerity measures have led to reducing government spending on capital investments (including funding new infrastructure projects and maintaining existing ones). Fortunately, in the latest years, a slow revival of economic infrastructure policies and the growing involvement of institutional investors have been registered. The public sector is still remaining the dominant funding and financing source, but much more private capital is flowing towards this sector Inderst (2020). According to the European Investment Bank, from 2018, the share of infrastructure investment in EU GDP started increasing again after hitting a low of 1.5% of Gross Domestic Product (GDP) in 2017, as is shown in Figure 5. In the wake of the COVID-19 pandemic, infrastructure investment declined slightly less than EU GDP, so, despite contracting in nominal terms in 2020, infrastructure investment’s share of EU GDP marginally increased again. In the breakdown by asset, depicted in Figure 6, transport and utilities, including energy, jointly make up some 60% of infrastructure investment; by far the largest increase since 2013 has been in communications, however, rising from 9% to 13% in the same period EY (2015). In most developed countries, the public sector was central to the ownership, financing, and delivery of infrastructure services post-World War II. Since the 1980s, private sector participation has risen in several countries as a result of privatization. Private capital can be provided in two main forms: corporate finance (financed “on balance sheet” from the own resources of companies) and project finance (financed by debt and equity used paid back from the cash flow generated by the project, typically, a special purpose entity or vehicle). Within project finance, from the 1990s, Public Private Partnership (PPP) schemes have become a common policy option as an alternative to public procurement of infrastructure. PPPs are contractual agreements between public bodies, local authorities or central Government, and private companies to deliver a public infrastructure project. The United Kingdom, Australia, and Canada were early adopters of PPPs; today, Europe still has the largest share of projects (over one-third), but North America, Asia, and Latin America are becoming more active. Project financing is also being used for social infrastructure, but volumes are rather small (well below 0.1% of global GDP) Inderst (2020). Within the various categories of investment vehicles, there is considerable differentiation in terms of geography, industry sectors, and development stages.
• Greenfield: the asset needs to be designed and constructed so investors fund the building of the infrastructure asset as well as the maintenance when it is operational.
• Brownfield: the asset is existing and usually partially operational, but it requires improvements, repairs, or expansion.
• Secondary: the asset is already fully operational.
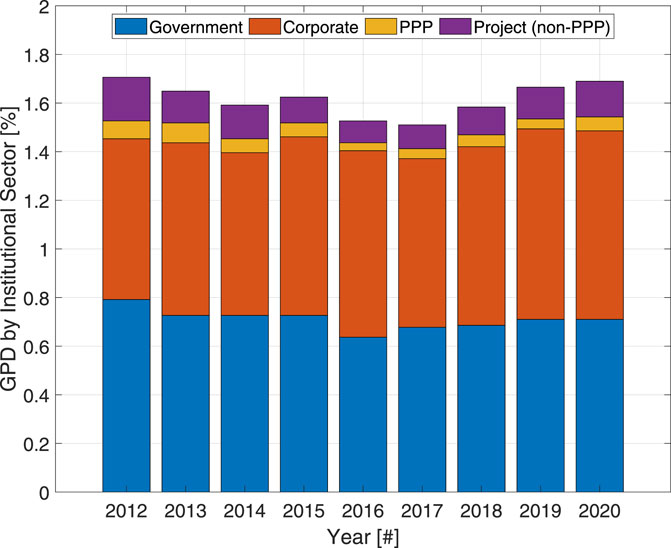
FIGURE 5. EU infrastructure investment (% GDP), by institutional sector (Source: GIH, 2022).
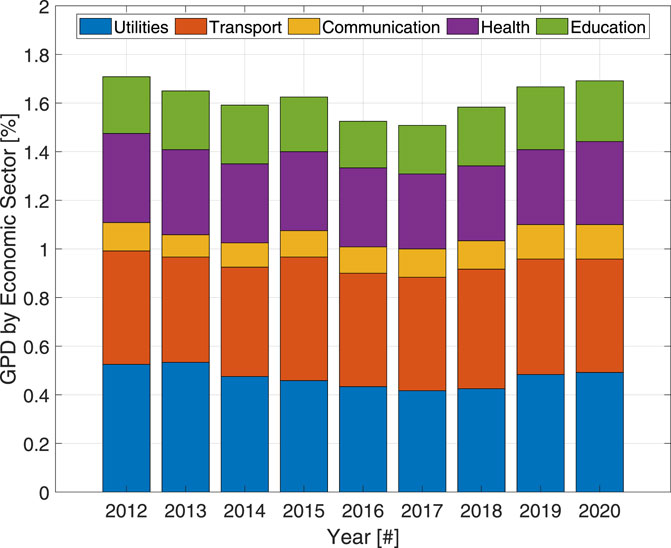
FIGURE 6. EU infrastructure investment (% GDP), by economic sector (Source: GIH, 2022).
Another difference is the one between i) availability-base projects, where the sponsor (e.g., the Government) procures facilities or services in return for payments linked to the availability rather than the usage level, and ii) demand-based projects, where the investor bears the revenue risk (often these ones have inflation-linked returns with greater exposure so economic risk and tend to be long term) EY (2015); Inderst (2010).
The recourse to financial vehicles and to private investments could be a possible solution to finance the digitalization of infrastructures, maintaining an equilibrium with the new platform model. The financial market seems to be quite mature: according to Prequin, in 2020, there were already 4,000 institutions making allocations to infrastructure and more than 700 active infrastructure fund managers around the world; about 100 USD billions are raised every year from investors. Figure 7 shows the various typologies of infrastructure investors in 2015 and in 2019. The main investors are pension funds, foundations, and insurance companies (median net Internal Return Ratios (IRRs) for infrastructure funds have hovered around 10 ∼ 11% in recent vintage years) Preqin (2020). One of the main reasons why infrastructure investments are an interesting option for an insurer’s portfolio is the fact that they provide a long-term risk exposure, which may provide a good match for long-term liabilities; this is particularly important under risk-based capital regulatory regimes, such as Solvency II. Furthermore, infrastructure investments may provide illiquidity and sector diversity (which could increase portfolio diversification) and an opportunity to lend money to sectors in need of funding (leading to social and potentially reputational benefits) EY (2015). Different opinions have been debated in literature to argue if infrastructures have to be considered as a separate asset class or not. Asset classes are important to investors, as each different asset class has a different risk profile and effect on portfolio performance and allocation: in fact, investors have a number of options in terms of financial objectives, expectations, and selections with respect to risk tolerance and return Oyedele (2014a). Several economists argue that infrastructure has very peculiar features with precise consequences for an investor, e.g., it requires a large initial capital, involves long duration and complex contracts, it provides yield stable, predictable, long-term cash flows which may be inflation-linked, and it is often influenced by a regulatory regime EY (2015). These facts lead us to consider infrastructure a separate asset class. On the other hand, other economist underlines the strong heterogeneity of infrastructure assets and suggests treating infrastructure simply as a sub-asset class or a sector —- further broken down into sub-sectors such as energy, utilities, and transport–within the conventional financing vehicle on which it comes (e.g., listed stocks, private equity, and bonds) Inderst (2010). As it is illustrated in Figure 8, risk-return profiles of infrastructure investments vary widely in relation to traditional asset classes: brownfield infrastructure investments are naturally characterized by lower expected risks and returns than greenfield ones. These theoretical speculations do not undermine the fact that infrastructure investments are an interesting option for a private portfolio and that various investment strategies can realistically be improved and expanded. This scenario could realistically be a sustainable answer to the financial challenges posed by the platformization of infrastructures.
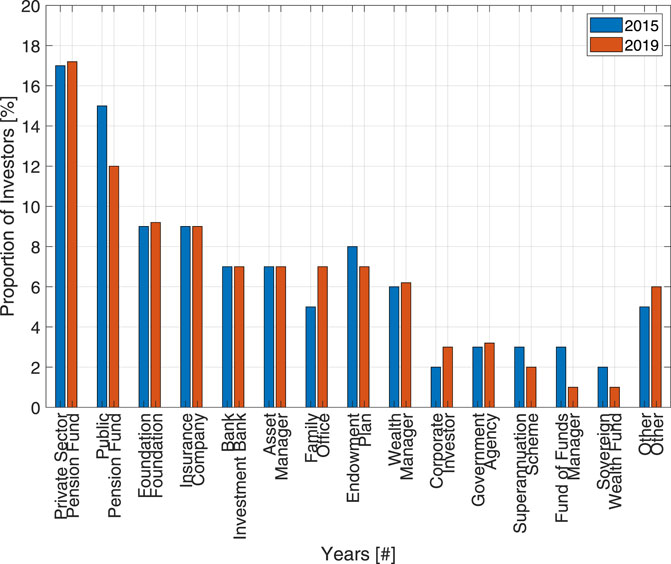
FIGURE 7. Investors in infrastructures by type, 2015 vs. 2019 (Source: Prequin, 2020).
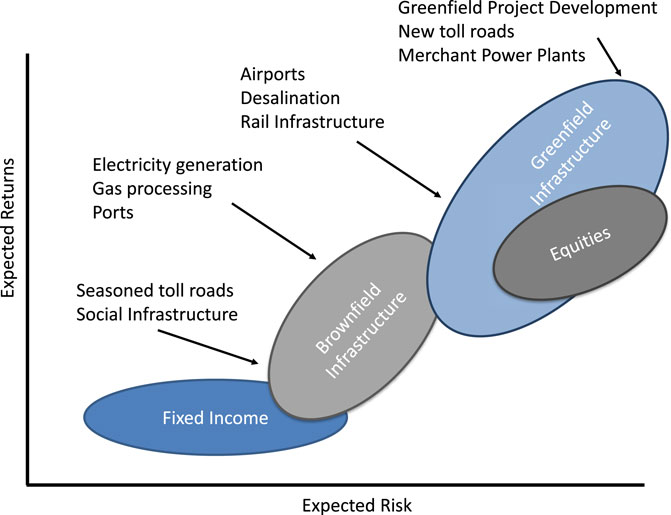
FIGURE 8. Risk-return profiles infrastructure investments (Source: Credit Suisse Asset Management, 2010).
5.2 Social sustainability
The social challenges posed by the platoformization process of infrastructures are even more difficult to be addressed than financial ones. In fact, market forces appear insufficient to establish an acceptable equilibrium. Therefore the intervention of public decision-makers seems to be necessary. In the latest years, the direct intervention of the State in the economic field has been invoked more and more often. For instance, Mazzucato has theorized that markets are not the result of an individual decision-making process but of rules every value-creating player, including the State itself, must obey; indeed, the State cannot be relegated to the role of “market fixer” addressing situations of market failure but has to assume the role of “market shaper“ cooperating with other market forces in the building of new markets and new ecosystems in order to accomplish the results needed by the society Mazzucato (2021). Moreover, the governance of platforms cannot be delegated to markets also because they are already penetrating every industry. Since a large area of the world is getting used to an infrastructural ecosystem that is designed, owned, and managed mainly by private companies operating in a global space, a higher vigilance is needed with reference to public values and the common good. The ecosystems built around platforms (their algorithms and business models) are not neutral, but the ideological principles inscribed in their architectures deeply influence the process of attributing public value and interests to be privileged: technology shapes society and vice versa. For example, i) data security can influence the trust in the usability of the infrastructure; ii) privacy is basic to maintain control of personal life; iii) transparency can be useful for the community; iv) accuracy can be key in various sectors, mainly for health infrastructure; v) responsibility and vi) accountability are also important and to be considered Van Dijk et al. (2018).
One of the possible approaches for the State to shape the platformization process is acting as a regulator. This approach poses many challenges. While it is widely shared that Government must maintain a level of supervision over platforms and infrastructure, the reality is that the pace of technological and social change is moving faster than the pace of regulatory change. Sometimes the national authorities even lack the right vocabulary to grasp the socio-technical nuances of evolving platform ecosystems. A right balance between control and innovation is needed by creating regulatory frameworks that are flexible and durable in the face of technological change KMPG (2019). Moreover, the regulation responsibility is traditionally highly fragmented: each governance level manages a limited policy framework. On the one hand, this fact makes it difficult and expensive for platform managers to relate with plenty of different authorities; on the other hand, it limits the power of regulation of the State Van Dijk et al. (2018). Furthermore, since geopolitical considerations will significantly influence the development of some infrastructure and platforms, international relations will become increasingly important, and the distinction between “international” and “domestic“ policy will become more blurred. Therefore the need for managing common resources more effectively across jurisdictions will call for a greater role for transnational governance OECD (2006). Another challenge to be faced by regulators is the difficulty of clarifying the right accountability since platform ecosystems are often based on complicated mechanisms Van Dijk et al. (2018). Finally, it is difficult for policymakers to classify platforms as well-defined industries subjected to specific authorities since platforms and technology are creating a high inter-dependency among infrastructures. Indeed, the introduction of smart vehicles will impact road, transit, power, and distribution networks; navigation apps are encouraging integration between public and private transit options; novel wireless technologies such as 5G/6G networks will improve the daily life of users and the user experience, e.g., in the healthcare, education, transportation, automation, and more KMPG (2019).
Another possible approach for the State is to assume control of platforms and act as a developer. According to the theory described by John M. Keynes in “The End of Laissed Faire” in 1926, “the important thing for Government is not to do things that individuals are doing already, and to do them a little better or a little worse, but to do those things which at present are not done at all“. In this perspective, an active role of the State as owner or manager of platforms appears improbable. However, in a more comprehensive approach to the platform society, governments could go beyond their traditional roles and act as a partner in multi-stakeholder cooperation. As public developers, governments can lead the platformization process in a proactive way with the aim of reaching a balance among the market, State, and civil society. In fact, in the past, the States have always had leadership in the creation of common infrastructures to generate democratic value while producing economic value. For instance, States could develop centralized plugins to be implemented in private platforms, such as a calibrated digital taximeter that is accessible to public auditors, and at the same time, it should be integrated into the platforms for digital transport service. Another example could be the influence that public administration could have on the platform as users and buyers of digital services: whilst today it has no choice but to accept the platform and its embedded values as they could become more demanding customers choosing platform services only if their values are aligned with social needs. Obviously, this means that firstly a public debate should be developed to define democratically what public values should be granted by platforms Van Dijk et al. (2018).
6 Additional approaches to sustainability
Beyond social and financial aspects, considered the main aspects of sustainable infrastructure digitalization, we present additional approaches that can be taken into account.
• Environmental sustainability: To reduce the environmental impact of digital infrastructure, we can consider the adoption of energy-efficient technologies to reduce carbon emissions by leveraging renewable energies—for example, smart grids, RF energy harvesting sensors, thermoelectric, and wind sources.
• Resilience: The reliability of the systems is an essential factor to consider during the deployment of infrastructure resilient to disruptions, cyber-attacks, and natural disasters. Indeed, adopting digital technologies, such as remote monitoring and predictive analysis, can help identify potential threats before they become major problems.
• Safety and security: Ensuring the safety and security of the systems is another crucial aspect of responding to and detecting potential cyber and physical attacks. For example, smart video surveillance systems, fine-grained access control, and the adoption of the best-practice for communication and system security can help to prevent unauthorized access and detect potential threats.
• Accessibility: In a society, this aspect should be taken into account regardless of background, age, or physical ability. Indeed, approaches such as accessible interfaces, voice and face recognition, digital assistants, and artificial intelligence can help individuals with disabilities and constraints to access and use digital infrastructures without effort.
• Circular Economy: Mitigating waste and promoting the reuse of recycling materials can help design and operate digital infrastructure. For example, adopting energy-efficient servers and energy-harvesting technologies can be leveraged to reduce energy consumption and waste.
It is worth noticing that these are just a few examples of promoting sustainable infrastructure digitalization beyond social and financial aspects. Indeed, it is crucial to have a holistic approach to ensure that digital infrastructure is sustainable, resilient, and inclusive.6.1 Environmental sustainability
The digitalization and the platformization of infrastructures are key processes for enabling global environmental sustainability and green transition: for instance, the adoption of digital technologies in the infrastructure industry can reduce the energy consumption of consumers and companies, reduce traffic congestion, and improve rail and air transport Secchi and Gili (2022). In fact, infrastructure is responsible for 79% of total greenhouse gas emissions worldwide, with most associated with energy, buildings, and transport UNOPS (2021). Digitalization can help the infrastructure industry to become cleaner, more energy efficient, and more resilient to the rapidly intensifying effects of climate change, with four typologies of transformative outcomes: circularity (i.e., enabling circular economy activity); environmental regeneration (i.e., supporting rehabilitation or restoration of environmental assets or biodiversity); low-carbon transition (i.e., supporting the transition to net zero emissions of carbon dioxide); pollution reduction (i.e., supporting the reduction of air, water, noise, and land pollution) (GIH).
Since environmental sustainability is a global goal, the race for digital infrastructure is also a geopolitical one; moreover, it is not easy reconciling the concepts of national sovereignty with the open and global nature of the digital space in which data flow: the United States and China are the main players in the global competition for digital leadership and Europe stands in between (recently the European Union has received pressures from the US to ban Chinese digital hardware, particularly that destined for critical infrastructure) Secchi and Gili (2022). Even if a level playing field appears far from being agreed upon, the United Nations Sustainable Development Goals (SDGs) strategically address global challenges to ensure an equitable, environmentally sustainable, and healthy society, and the digitalization of infrastructure plays a key role in supporting their achievement since digitalization provides access to an integrated network of big unexploited data with potential benefits for society and the environment Mondejar et al. (2021). It has been demonstrated that infrastructure systems influence the achievement of all SDGs, including up to 92% of targets: this influence may be direct through improving water accessibility (SDG 6), promoting renewable energy (SDG 7) or, more generally, enhancing infrastructure and innovation (SDG 9). It may also be indirect, for example, providing schools and facilities that enable the equitable attainment of quality learning and skills (SDG 4) Forum (2019).
In order to achieve these goals, high coordination is required across all stages of the infrastructure lifecycle, from planning and implementing to delivery, management, and decommissioning; it involves hundreds of stakeholders and spans decades UNOPS (2021). Fortunately, this challenge is addressed by the platformization of infrastructure since the data layer transforms infrastructure assets into data assets Forum (2019). In this context, the term “InfraTech” is becoming common to describe “any technology that significantly impacts the development, delivery, and ongoing operation of infrastructure.” World Bank (2020)
Today an array of technologically innovative solutions are available to lead to reductions in emissions, delay times, energy consumption, and other key indicators while maintaining or even improving safety levels overall Secchi and Gili (2022).
In the transportation sector, for instance, which is responsible for approximately 16% of global greenhouse gas emissions (given that fossil fuels remain the dominant source of energy consumption) UNOPS (2021), digitalization means more information on traffic and tracking, easier passenger access to services, more efficient use of infrastructure capacity and a higher degree of predictability on timing for railway transport. It also means improving the efficiency of air traffic and more flexibility and scalability to cope with crisis situations. At the same time, smart port strategies can include container automation, and the digitalization of maritime freight transport and port organization Secchi and Gili (2022).
In the water sector, which is expected to require the most investment to adapt to the impacts of climate change (54% of all adaptation costs) mainly to protect coastal cities from sea-level rise UNOPS (2021), digitalization means the exploitation of big data and artificial intelligence to improve the operations and support decision-making in water treatment systems (WTSs), such as wastewater treatment plants (WWTPs), drinking water treatment plants (DWTPs), and desalination plants, which are complex systems that integrate different processes to remove pollutants, impurities, and salts from target water bodies. Smart water refers to the holistic approach to managing this resource, related infrastructure, treatment, and delivery; during the treatment processes, vast volumes of data can be generated and collected simultaneously via various sensing technologies; real-time water quality audits at critical control points can define when decentralized water treatment may be required to operate to ensure public health; for instance, artificial intelligence systems can identify a water quality issue (e.g., high nitrate levels, bacteria presence, etc.) and activate immediate action for its remediation in order to ensure sustainable and healthy environments in cities Mondejar et al. (2021).
In the building sector, moreover, digitalization means increasing the efficiency and resilience of buildings through clean lighting and efficient heating and cooling systems Mondejar et al. (2021).
6.2 Digitalization impact on the energy sector
The digitalization impact on the energy sector deserves wider insights since it includes direct and indirect effects: the former includes energy consumption of the ICT sector; it is affected by the growth of the share of ICT in overall GDP and mitigated by energy efficiency improvements in the delivery of ICT services; the latter includes energy efficiency and rebound effects (i.e., efficiency improvements to the rest of the economy and resulting rebound effects of increasing service demand), economic growth (via increased efficiency and labor productivity) and sectoral change Mondejar et al. (2021). According to the International Energy Agency, digital technologies are expected to make energy systems more connected, intelligent, efficient, reliable, and sustainable; the pace of digitalization in energy is increasing, as it is demonstrated by the sharp rise of investment in digital technologies by energy companies over the last few years Agency (2017) Borowski (2021). Moreover, in October 2022, the European Commission adopted the ‘Digitalisating the energy system - EU action plan’, a system-wide digitalization energy action plan that aims to support the development of a sustainable (cyber)secure, transparent, and competitive market for digital energy services, ensuring data privacy and sovereignty, and supporting investment in digital energy infrastructure European Commission (2022).
All over the world, energy systems are facing a wide transformation: centralized and decentralized ever-fluctuating renewable energy resources (such as wind and solar) continue to be added to the grid, the electrification of energy consumption is increasing, and “prosumers” (people who both consume and produce energy) are emerging. Demand-side flexibility is increasingly important to ensure efficiency and to supply energy when it is needed and consume when it is available Agency (2017) Mondejar et al. (2021). In particular, the renewable energy attributes significantly reduce their energy efficiency, which is a strategic goal to secure energy supply and reduce energy consumption and greenhouse gas emissions globally, but also an essential source of competitive advantage that can reduce operational costs Borowski (2022). In this context, digitalization opens a broad range of possibilities along the entire energy value chain, ranging from generation to transport, distribution, supply, and consumption. Data gathering technologies such as sensors and smart meters can collect data on energy use and other conditions affecting energy use (like climate); data processing technologies, such as artificial intelligence algorithms, can provide useful information that can be finally sent to devices that can affect physical changes to optimize energy use Agency (2017). In the case of distributed energy systems, intermittent in nature, digitalization can help also model the contribution of individual electricity supplies into the grid and their dynamics due, for instance, to changing weather conditions Mondejar et al. (2021). Despite the high level of complexity, the concept of a “smart grid” offers a solid platform for a safe and sustainable transition. It consists of the coordination of the energy flow between generation and consumption, the coordination of diverse energy resources, and the integration of distributed and diverse energy storage systems Mondejar et al. (2021). This may allow policies to support and encourage the users to avoid the use of electricity during peak times, to choose whether it is more valuable to use excess electricity to charge the home battery (e.g., heating hot water) or export to the grid, to act peer-to-peer electricity trading with neighboring consumers rather than selling it to the electricity provider Mondejar et al. (2021).
On the other hand, the explosion of the digital industry has also brought some controversies: some scholars have suggested that the spread of ICT tools and the growth of the digital economy may affect energy consumption and has rapidly increased the demand for electricity in developing countries Borowski (2022). In other words, there is potential for big rebound effects throughout the economy Mondejar et al. (2021). Anyway, it has been demonstrated that overall digitalization reduces energy consumption, decreases energy intensity, and optimizes energy structure Xu et al. (2022); in fact, it can reduce energy demand in various sectors, such as the industrial and transportation sectors, which represent more than half of the total energy consumption in the world. Energetic processes in the transportation sector have efficiencies between 45% (i.e., Diesel engines) to about 85% (i.e., gas boilers), meaning that much energy is wasted in the form of residual heat Mondejar et al. (2021). Smart mobility systems promise to increase efficiency: CAVs (Connected Autonomous Vehicles) equipped with V2V (vehicle-to-vehicle) or V2I (vehicle-to-infrastructure) communication systems can reduce greenhouse gas emissions and time spent in traffic; it has been demonstrated that a 70% CAV penetration rate in a city like Milan in Italy can achieve to a reduction in emissions of approximately 400 t/year CO2 eq with a V2V solution, and around 2,700 t/year in a V2I scenario Secchi and Gili (2022). In the manufacturing sector, computational modeling can be used as a powerful tool for energy analysis as an effective decision-making technique in order to optimize throughput, effectively plan and manage operations, reduce bottlenecks and test various scenarios Borowski (2022). Moreover, this sector could benefit from the introduction of a paradigm such as Industry 5.0.
6.3 Industry 5.0 challenges and opportunities
The new Industry 5.0 paradigm, built on the fundamentals of the Industry 4.0 paradigm, focuses on integrating artificial intelligence and human intelligence to create a sustainable and digitalized autonomous system. However, some challenges and key considerations must be taken into account. For example, the complexity and the security aspects are crucial to ensure system integration, optimization, and protection against cyber threats. As future trends, Industry 5.0 emphasizes the integration of human-machine cooperation, the creation of sustainable and reliable infrastructure, the consolidation with a data-driven decision-making approach (from the data collection to the data analysis), and the enhancement of the systems by adopting augmented and virtual reality Maddikunta et al. (2022).
7 Conclusion
The process of digitalization and platformization involving economic and social infrastructures has been outlined through the description of technological enabling factors and the modeling of a probable scenario where platforms manage a data layer between infrastructure and service providers. Implications have been investigated both from an economic and a social point of view, highlighting the opportunities in terms of efficiency, value creation, and positive externalities but also warning against risks in terms of market failures and negative externalities threatening equity, security, privacy, and even democracy. Finally, possible solutions have been explored to achieve a state of greater financial and social balance: the role of institutional investors and of the State is expected to be crucial to reaching this important goal.
Data availability statement
The original contributions presented in the study are included in the article/Supplementary Material, further inquiries can be directed to the corresponding author.
Author contributions
TM and PT worked in parallel on all the contribution.
Funding
This publication was partially supported by Technology Innovation Institute. The findings reported herein are solely responsibility of the authors.
Acknowledgments
The authors would like to thank the reviewers for their comments and insights, that have helped improving the quality of the paper.
Conflict of interest
The authors declare that the research was conducted in the absence of any commercial or financial relationships that could be construed as a potential conflict of interest.
Publisher’s note
All claims expressed in this article are solely those of the authors and do not necessarily represent those of their affiliated organizations, or those of the publisher, the editors and the reviewers. Any product that may be evaluated in this article, or claim that may be made by its manufacturer, is not guaranteed or endorsed by the publisher.
References
Adame, T., Bel, A., Bellalta, B., Barcelo, J., and Oliver, M. (2014). Ieee 802.11 ah: The WiFi approach for M2M communications. IEEE Wirel. Commun. 21, 144–152. doi:10.1109/mwc.2014.7000982
AssTel (2021). Rapporto sulla filiera delle telecomunicazioni in italia – edizione 2021. Berlin, Germany: Springer.
Baldwin, C. Y., and Woodard, C. J. (2009). The architecture of platforms: A unified view. SSRN Electron. J. 32, 19–44. doi:10.2139/ssrn.1265155
Baronti, P., Pillai, P., Chook, V. W., Chessa, S., Gotta, A., and Hu, Y. F. (2007). Wireless sensor networks: A survey on the state of the art and the 802.15.4 and ZigBee standards. Comput. Commun. 30, 1655–1695. doi:10.1016/j.comcom.2006.12.020
Borowski, P. F. (2021). Digitization, digital twins, blockchain, and industry 4.0 as elements of management process in enterprises in the energy sector. Energies 14, 1885. doi:10.3390/en14071885
Borowski, P. F., Lin, X., Luo, H., Zhang, J., and Lian, Y. (2022). Effects of digitalization on energy efficiency: Evidence from zhejiang province in China. Front. Energy Res. 10. 847339. doi:10.3389/fenrg.2022.847339
Bostrom, N. (2014). Superintelligence: Paths, dangers, strategies. United Kingdom: Oxford University Press.
Bresnahan, T. F., and Trajtenberg, M. (1995). General purpose technologies ‘Engines of growth. J. Econ. 65, 83–108. doi:10.1016/0304-4076(94)01598-t
Carr, N. (2008). The big switch: Rewiring the world, from edison to Google. New York, United States: W. W. Norton and Company.
Cäsar, M., Pawelke, T., Steffan, J., and Terhorst, G. (2022). A survey on Bluetooth Low Energy security and privacy. Comput. Netw. 205, 108712. doi:10.1016/j.comnet.2021.108712
Constantinides, P., Henfridsson, O., and Parker, G. (2018). Introduction—platforms and infrastructures in the digital age. Inf. Syst. Res. 29, 381–400. doi:10.1287/isre.2018.0794
Edelman, B. G., and Geradin, D. (2016). Efficiencies and regulatory shortcuts: How should we regulate companies like Airbnb and uber? Stanf. Technol. Law Rev. 19, 293–328. doi:10.2139/ssrn.2658603
Ertürk, M. A., Aydın, M. A., Büyükakkaşlar, M. T., and Evirgen, H. (2019). A survey on LoRaWAN architecture, protocol and technologies. Future Internet 11, 216. doi:10.3390/fi11100216
EY (2015). Infrastructure investments: An attractive option to help deliver a prosperous and sustainable economy. Berlin, Germany: Springer.
Fahmideh, M., and Zowghi, D. (2020). An exploration of IoT platform development. Inf. Syst. 87, 101409. doi:10.1016/j.is.2019.06.005
Finger, M., and Montero, J. (2018). A programme for the new network industries. Italy: Robert Schuman Centre for Advanced Studies. Research Paper 2018.
Forum, W. E. (2019). Transforming infrastructure: Frameworks for bringing the fourth industrial revolution to infrastructure. Netherlands: European Union.
Inderst, G. (2020). A global perspective social infrastructure finance and institutional investors. Berlin, Germany: Springer.
Li, S., Da Xu, L., and Zhao, S. (2018). 5G internet of things: A survey. J. Industrial Inf. Integration 10, 1–9. doi:10.1016/j.jii.2018.01.005
Li, S., Xu, L. D., and Zhao, S. (2015). The internet of things: A survey. Inf. Syst. Front. 17, 243–259. doi:10.1007/s10796-014-9492-7
Maddikunta, P. K. R., Pham, Q.-V., Prabadevi, B., Deepa, N., Dev, K., Gadekallu, T. R., et al. (2022). Industry 5.0: A survey on enabling technologies and potential applications. J. Industrial Inf. Integration 26, 100257. doi:10.1016/j.jii.2021.100257
Mazzucato, M. (2021). Mission economy. A moonshot guide to changing capitalism. London, United Kingdom: Penguin Books.
Mondejar, M. E., Avtar, R., Lellani Baños Diaz, H., Kant Dubey, R., Esteban, J., Gómez-Morales, A., et al. (2021). Digitalization to achieve sustainable development goals: Steps towards a smart green planet. Sci. Total Environ. 794, 148539. doi:10.1016/j.scitotenv.2021.148539
Montero, J., and Finger, M. (2021a). A modern guide to the digitalization of infrastructure. Cheltenham, United Kingdom: Edward Elgar Publishing.
Montero, J., and Finger, M. (2021b). The rise of the new network industries: Regulating digital platforms (routledge). Netherlands: European Union.
Muciaccia, T. (2020). Dal bel paese alla smart nation. Infrastrutture per la Digital transformation. Rome, Italy: Aracne Editrice.
Muhammed, A. S., and Ucuz, D. (2020). Comparison of the IoT platform vendors, Microsoft azure, Amazon web services, and Google cloud, from users’ perspectives, Proceedings of the 2020 8th International Symposium on Digital Forensics and Security, 12 June 2020, IEEE, 1–4. doi:10.1109/ISDFS49300.2020.9116254
OECD (2006). Infrastructure to 2030 (volume 2): Mapping policy for electricity, water and transport. Washington, D.C., United States: World Bank.
Oyedele, J. (2014a). A conceptualisation of the emergence of infrastructure investment as a separate asset class. Acad. J. Interdiscip. Stud. 3, 213–222. doi:10.5901/ajis.2014.v3n1p213
Oyedele, J. (2014b). Infrastructure investment and the emerging role of institutional investors: The case of pension funds and sovereign wealth funds. Acad. J. Interdiscip. Stud. 3. 43. doi:10.5901/ajis.2014.v3n1p43
Parker, G., Van Alstyne, M., and Choudary, S. (2016). Platform Revolution. How networked markets are transforming the economy and how to make them work for you. New York, United States: W. W. Norton and Company.
Ratasuk, R., Vejlgaard, B., Mangalvedhe, N., and Ghosh, A. (2016). NB-IoT system for M2M communication. In 2016 Proceedings of the IEEE wireless communications and networking conference. Doha, Qatar, 03-06 April 2016, IEEE, 1–5.
Rifkin, J. (2014). The zero marginal cost society: The internet of things, the collaborative commons, and the eclipse of capitalism. London, United Kingdom: Palgrave Macmillan.
Rochet, J.-C., and Tirole, J. (2003). Platform competition in two-sided markets. J. Eur. Econ. Assoc. 1, 990–1029. doi:10.1162/154247603322493212
Samie, F., Bauer, L., and Henkel, J. (2016). IoT technologies for embedded computing: A survey. In 2016 International Conference on Hardware/Software Codesign and System Synthesis (CODES+ISSS). Pittsburgh, PA, USA, 16 October 2016, 1–10.
Secchi, C., and Gili, A. (2022). Digitalisation for sustainable infrastructure: The road ahead. Milan, Italy: Ledizioni LediPublishing.
Stiglitz, J. (2020). People, power, and profits: Progressive capitalism for an age of discontent. London, United Kingdom: Penguin Books.
Tabors, R. e. a. (2016). White paper on developing competitive electricity markets and pricing structures. New York State Energy Research and Development Authority (NYSERDA) and New York State Department of Public Service: Cambridge, MA, United States.
Tedeschi, P., Sciancalepore, S., Eliyan, A., and Di Pietro, R. (2020). LiKe: Lightweight certificateless key agreement for secure IoT communications. IEEE Internet Things J. 7, 621–638. doi:10.1109/jiot.2019.2953549
van der Aalst, W., Hinz, O., and Weinhardt, C. (2019). Big digital platforms. Berlin, Germany: Springer.
Van Dijk, J., Poell, T., and De Waal, M. (2018). Public values in a connective world. United Kingdom: Oxford University Press.
Xu, Q., Zhong, M., and Li, X. (2022). How does digitalization affect energy? International evidence. Energy Econ. 107, 105879. doi:10.1016/j.eneco.2022.105879
Yehoue, E., Hammami, M., and Ruhashyankiko, J. (2006). Determinants of public-private partnerships in infrastructure. IMF Working Paper WP/06/99.
Keywords: digitalization, infrastructures, platforms, IoT, sustainability
Citation: Muciaccia T and Tedeschi P (2023) Future scenarios for the infrastructure digitalization: The road ahead. Front. Internet. Things 2:1140799. doi: 10.3389/friot.2023.1140799
Received: 09 January 2023; Accepted: 28 February 2023;
Published: 14 March 2023.
Edited by:
Luis Antonio Garcia, University of Southern California, United StatesReviewed by:
Teodoro Montanaro, University of Salento, ItalyMohamed Rahouti, Fordham University, United States
Copyright © 2023 Muciaccia and Tedeschi. This is an open-access article distributed under the terms of the Creative Commons Attribution License (CC BY). The use, distribution or reproduction in other forums is permitted, provided the original author(s) and the copyright owner(s) are credited and that the original publication in this journal is cited, in accordance with accepted academic practice. No use, distribution or reproduction is permitted which does not comply with these terms.
*Correspondence: Pietro Tedeschi, pietro.tedeschi@tii.ae