Male infertility and the human microbiome
- 1McGovern Medical School, University of Texas Health Science Center at Houston, Houston, TX, United States
- 2Department of Urology, The Pennsylvania State University College of Medicine, Hershey, PA, United States
The historical belief in urology was that the genitourinary system should be sterile in a normal, healthy, asymptomatic adult. This idea was perpetuated for decades until research revealed a diverse microbiota existing in human anatomical niches that contributed to both human health and disease processes. In recent years, the search for an etiology and modifiable risk factors in infertility has turned to the human microbiome as well. Changes in the human gut microbiome have been associated with changes in systemic sex hormones and spermatogenesis. Certain microbial species are associated with higher levels of oxidative stress, which may contribute to an environment higher in oxidative reactive potential. Studies have demonstrated a link between increased oxidative reactive potential and abnormal semen parameters in infertile men. It has also been hypothesized that antioxidant probiotics may be able to correct an imbalance in the oxidative environment and improve male fertility, with promising results in small studies. Further, the sexual partner's microbiome may play a role as well; studies have demonstrated an overlap in the genitourinary microbiomes in sexually active couples that become more similar after intercourse. While the potential applications of the microbiome to male fertility is exciting, there is a need for larger studies with uniform microbial sequencing procedures to further expand this topic.
Introduction
The Human Genome Project (HGP), launched in 1990, aimed to map the entire human genome, which led to significant advancements in our understanding of human biology and genetics (1). As part of this project, researchers also started to investigate the microbial communities that inhabit the human body and their role in human health (2). The microorganisms inside humans, known as the microbiota, outnumber human cells ten to one (3). The microbiota's genomes, collectively referred to as the microbiome, can provide traits and functions that humans did not evolve on their own, and produce a composition of small molecules and downstream products, referred to as the metabolome (3). If we are thought of as a composite of microbial and our own cells, then human health and disease develops from both genomes, the microbiome and our own (3). With advances in molecular biology techniques such as Next Generation Sequencing and 16S rRNA subunit analysis, scientists were able to characterize an entire microbial community in a sample, rather than just an individual species (4). These advances revealed that microbiomes exist in nearly every anatomical niche in the body, and seem to play a role beyond infection (3). The individuality of the microbiome and dynamic changes it undergoes in adulthood may allow an opportunity to treat disease on a more personalized level, and to understand variances in disease susceptibility and treatment response.
Gut-testes axis
As demonstrated in other systems, there is a hypothesized link between the gut microbiome and the male urogenital tract. The gut-testes axis refers to an interaction between the microbiome of the gut and its impact on regulating testicular function, and is thought to play a role in male reproductive health and infertility. There is significant evidence that an alteration in the GM can lead to systemic changes and inflammation, thus may affect the testicular environment and form an interdependent connection (5). Trimethylamine N-oxide (TMAO) is thought to be a key signaling molecule. TMAO is a biologically active molecule generated by the GM and associated with an increased risk of atherosclerosis and endothelial dysfunction (6). High levels of TMAO are associated with fewer and less healthful endothelial progenitor cells and more reactive oxygen species, potentially contributing to vasculogenic erectile dysfunction (7).
A paper written by Tremellen in 2016 highlighted a potential mechanism that links gut dysfunction in obese men and late onset hypogonadism, formally referred to as “The Gut Endotoxin Leading to a Decline in Gonadal Function” (GELDING) theory (8). The theory states that a high fat, high calorie diet can trigger a breakdown in intestinal mucosal barrier, leading to a leakage of bacterial endotoxin and a chronic state of low grade inflammation (8). This state of low grade inflammation can then lead to testicular and hormonal dysfunction (8). There is evidence that a high fat diet can alter disordered tight junction proteins that separate the metabolome of gut microbes from leaking into systemic circulation (9). One study in male mice demonstrated the consumption of a high-fat diet altered the seminal fluid and gut microbiomes (10). This theory is also supported by data in animal studies that show certain endotoxins can impair luteinizing hormone (LH) pulses and interfere with intra-testicular production of testosterone (11, 12).
Several studies have demonstrated that the gut microbiome influences testosterone levels and sperm production. Bacterial fermentation produces certain short-chain fatty acids, which trigger the release of gut hormones such as GLP-1 and peptide YY (PYY), two anorexigenic peptides that regulate satiety (13, 14). GLP-1 agonists have also been shown to increase serum testosterone in diabetic and/or obese patients, improve sperm metabolism, motility, and insulin secretion in the testes (15). PYY administration inhibits GnRH secretion in male rats and delays the LH surge in female ewes (16, 17). The GM also directly generates androgens, thus changes in the GM may modulate intestinal and androgen metabolism (18). Additionally, androgen deprivation can alter fecal microbiota and exacerbate risks for other systemic diseases such as obesity and cardiovascular disease (19).
Animal studies have also shown that a FMT can treat infertility and improve sperm quality, demonstrating another link between gut dysbiosis and fertility. A study in mice by Zhang et al. looked at the effects of a FMT in mice with damaged spermatogenesis and sperm quality. The study found that a FMT improved spermatogenesis, increased sperm concentration, improved motility, increased levels of testicular antioxidants and key reproductive proteins, and increased gene expression related to spermatogenesis (20). In mice with induced Type 1 diabetes and infertility, a FMT significantly decreased blood glucose and increased semen quality, improved spleen and liver functions to strengthen the systemic environment for sperm development, and increased blood and testicular key metabolomes, such as docosahexaenoic acid (DHA) and testosterone (21). Other studies have demonstrated decreased DHA in the testes of animals with high fat diets, and supplemental DHA has been associated with restored fertility in infertile male mice (22, 23).
In addition to evidence from animal experiments, a few clinical trials have shown an association between the gut microbiome, serum testosterone levels, and sperm production (24–27). In an observational study of men with type 2 diabetes, significant differences in microbiota species were noted between men with and without low testosterone (26). Men with low serum testosterone also had an increased abundance of opportunistic pathogens (26). Another study demonstrated a significantly positive association between gut levels of Firmicutes and serum testosterone in adult men, while controlling for age, BMI and lipoprotein levels (28). There is further evidence through endocrine research that certain hormones involved in steroidogenesis are actively synthesized in the colon and under the influence of the GM (29–31). One study found that a specific microbial species found in the gut and genitourinary tract is capable of metabolizing both endogenous glucocorticoids and pharmaceutical derivatives, potentially influencing bioavailability of certain metabolites that may influence hypertension and prostate growth (29). Overall, the combination of these studies demonstrates a link between hormones and microbes, but more research especially in humans is needed in regards to male fertility specifically.
Male infertility
While the urogenital microbiome shows similarity with the gastrointestinal microbiome in individuals, there are significant differences that suggest a distinct upper genital tract contribution (32). Studies have shown that the list of bacteria was shorter and the total count of bacteria was lower in first catch-urine (representing urethra microbiome) than in first-catch seminal fluid, suggesting a unique contribution of the upper genital tract (33). The diversity of seminal microbiome has been demonstrated with several studies, with one citing >104 bacteria per ml in both healthy and infertile men, without any differences detected in semen parameters or semen specimens with and without bacteria (34). Analysis of 97 semen samples showed that 83% contained bacteria, and the presence of multiple bacterial species in semen was not associated with abnormal sperm function (35). The seminal vesicles, prostate, and testes have their own unique microbiomes, which differ between individuals and at different points in time, and can be altered based on individual hygiene, circumcision, sexual practices, sexual partners, and diet (36). For example, lower bacterial concentration and diversity were observed in men without sexual experience compared to those with sexual experience (37). In a healthy man, the most abundant species varies throughout the urogenital tract, which makes it difficult to fully understand what constitutes a healthy seminal microbiome (36).
There have been several studies that have looked at the role of seminal dysbiosis and its relation to prostatitis. While a number of microorganisms have been reported to cause chronic prostatitis, there is a growing hypothesis that prostatitis could be the result of seminal dysbiosis (38). Other studies have linked chronic prostatitis to inflammatory bowel syndrome, with simultaneous presence of syndromes occurring in about 30% of patients (39). The pathophysiology of these two simultaneous conditions is thought to be secondary to intestinal and or seminal dysbiosis and persistent mucosal inflammation (40). A study by Mandar et al. in 2017 revealed men with prostatitis had overall higher species richness and lower counts of Lactobacilli (41). Lactobacilli are associated with protective functions in oral cavity, vagina, and gastrointestinal tract, and may possibly play a similar role in the male reproductive tract (42). In studies of semen quality in infertility, >80% of normal semen samples clustered into a Lactobacillus predominant group (43).
The microbiome may be a tool to help improve male factor fertility. Estimates of idiopathic male infertility range from 30%–50%, citing underlying factors such as oxidative stress, lifestyle, and unidentifiable hormonal imbalances (44, 45). Oxidative stress (OS) from both exogenous (e.g., alcohol) and endogenous metabolic processes is a contributor to idiopathic male infertility in an estimated 80% of cases (44). Dysfunctional cellular processes contribute to the generation of reactive oxygen species (ROS) in semen, which in turn negatively impacts sperm function and membrane fluidity (46). An increase in oxidation-reduction potential, a measure of ROS, is associated with abnormal semen parameters in infertile men (47).
There has also been an established link between dysbiosis and increased oxidative stress; certain bacterial species may generate ROS through bacteriospermia, adhesion, toxin production, and inflammation (48). Studies of sperm quality and oxidative markers revealed that bacteriospermia is associated with inflammation, oxidative stress and sperm structural deterioration (49). Another study revealed that the intake of ultra-processed foods (UPFs) and a disruption of the normal GM was positively associated with the odds of asthenozoospermia (50). There is also an established link between gut dysbiosis and an increase in systemic oxidative stress potentially associated with other diseases such as Alzheimer's dementia, depression, and type 2 diabetes mellitus (51).
Since the establishment of a microbiome in the testis and semen, research has focused on trying to identify specific taxonomic correlations with infertility (36, 52). While studies have tried to find an association between the presence of seminal dysbiosis and infertility, the results are still conflicting (52, 53). Several studies conclude Lactobacillus is associated with a protective impact on semen parameters, yet Ureaplasma urealyticum, Mycoplasma hominis, and Aerococcus have been shown to have a negative impact on semen parameters. Bacterioidetes and Firmicutes are associated with azoospermatic semen (32, 43, 52–58). A recent study from Garcia-Segura in 2022 identified several bacteria strains in the seminal microbiome in infertile men that were negatively correlated with sperm global DNA fragmentation, specifically Moraxella, Brevundimos, and Flavobacterium (59). Other species correlated with reduced chromatin protamination status and increased in DNA fragmentation, negatively effective sperm reproductive potential (59). There is still a scarcity of data on this subject, with meta-analyses incorporating data from non-human subjects to supplement human trials, however, the data overall supports the hypothesis that certain bacterial strains in semen can alter the quality of sperm (53).
Although the link between male infertility and the taxonomy of the microbiome is unclear, the function of the microbiome may be more important (60). Despite differences in microbiota composition between individuals, their overall microbiome may perform the same functions, producing the same metabolites and causing the same changes in human cells (61). A study by Lundy et al. found that infertile men had significant alterations in the S-adenosyl-L-methionine (SAM) cycle overrepresented in their urine and semen (32). The SAM cycle is involved in DNA methylation, oxidative stress, and polyamine synthesis, however, its specific role in fertility is currently unknown (32). This study suggests that the specific metabolic processes that the microbiome performs, rather than its composition, may be affecting fertility. Further research is needed to better understand the functional processes of the microbiome and their association with infertility.
Another area for further exploration is the role of combined seminovaginal microbiome and its potential influence on the couples' fertility. Sexual intercourse can alter both the urinary and vaginal microbiomes in women, with studies showing an increased G. vaginalis in young women after sexual activity (62). There is additional data to suggest sexual intercourse can change the male urethral microbiome, with certain species such as Ureaplasma, Mycoplasma and Sneathia only found in sexually experiences males (63). While data around the microbiome and male infertility is rare, parallel studies examining the microbiota of sexually active couples are even rarer (64). A study done by Mandar et al. found major shifts in the vaginal microbiome after intercourse; a predominance of G. vaginalis in women was significantly associated with leukocytospermia in their male partners (64). Leukocytes in sperm are also the number one contributor to seminal ROS, potentially contributing to male infertility (44). In addition, Wittemer et al. demonstrated that positive bacterial cultures from both vagina and semen in couples undergoing in vitro fertilization decreased the clinical pregnancy rate and increased the spontaneous miscarriage rate significantly more than vaginal infection alone (65). The concept of olfactory receptors and volatile short-chain fatty acids (SCFAs) could provide the underlying mechanism for a seminovaginal microbial role in fertility. Olfactory receptors are key receptors involved in spermatozoa chemotaxis, and can be activated by SCFAs, metabolic by-products of anaerobic bacterial fermentation (66). A study by Teveroni et al. in 2022 demonstrated spermatozoa activation after olfactory receptors bound to SCFAs produced by microbiota metabolism in cervical mucus (66). This mechanism relied on a bacterial process in the vagina, thus providing a possible mechanism for a microbiome's role in a couple's fertility.
Probiotics for male infertility
In the case of a disordered microbiome and excess ROS contributing to male infertility, probiotics and prebiotics with antioxidant properties may be a way to create an environment that is more favorable for spermatozoa. Probiotics contain live microorganisms, while prebiotics are food for human microbiota. There are seven core genera of organisms that are most often used in probiotics, those being Lactobacillus, Bifidobacterium, Saccharomyces, Streptococcus, Enterococcus, Escherichia, and Bacillus (67). Probiotics may be able to reset reproductive tract dysbiosis (68). Antioxidants have been shown to enhance the semen quality in smokers, prevent release of immature sperm, and have a positive impact on assisted reproductive technology (44). Oral probiotics have shown to be successful in treating female reproductive concerns, and thus have the potential to optimize the male microbiome as well (69, 70). A meta-analysis on probiotics for female reproductive tract concerns highlighted that oral probiotics can effectively restore vaginal microbiomes to optimal Lactobacillus spp dominance (71). An imbalance in this species has been associated with adverse pregnancy outcomes, a significant decrease in endometrial implantation, and altered IVF outcomes (71). The species also produces bacteriocins with antimicrobial activity against relevant urogenital pathogens (71). Some probiotic species can also improve immune responses, with one study demonstrating a reduction in endometrial lesions through endometrial epithelial cell barrier function with probiotic administration (72).
Certain studies have examined the use of probiotics couples with antibiotics in treating infertility associated with infections, which are outlined in Table 1. A study by Grande et al. treated 104 infertile patients with semen and/or prostatic secretions positive for gram-negative bacteria with fluoroquinolones, with 84 receiving probiotic Enterococcus faecium and Saccharomyces boulardii, followed with Lactobacilli, in addition to antibiotics (73). A negative semen culture was observed in 76.2% of patients in the combination treatment group, compared to only 50% in the antibiotics alone group (73). Another study looked at the progression of chronic bacterial prostatitis (CBP) in patients with infertility and concomitant inflammatory bowel disease after treatment with antibiotics (40). Patients were treated with a combination of rifaximin and a probiotic strain of eight gram-positive bacteria species (40). The men in the therapeutic treatment group had a lower risk of progression of prostatitis into prostate-vesiculitis than men given no treatment (40). These studies demonstrate a benefit to adding probiotics to antibiotic treatment when treating for potential infections that contribute to infertility.
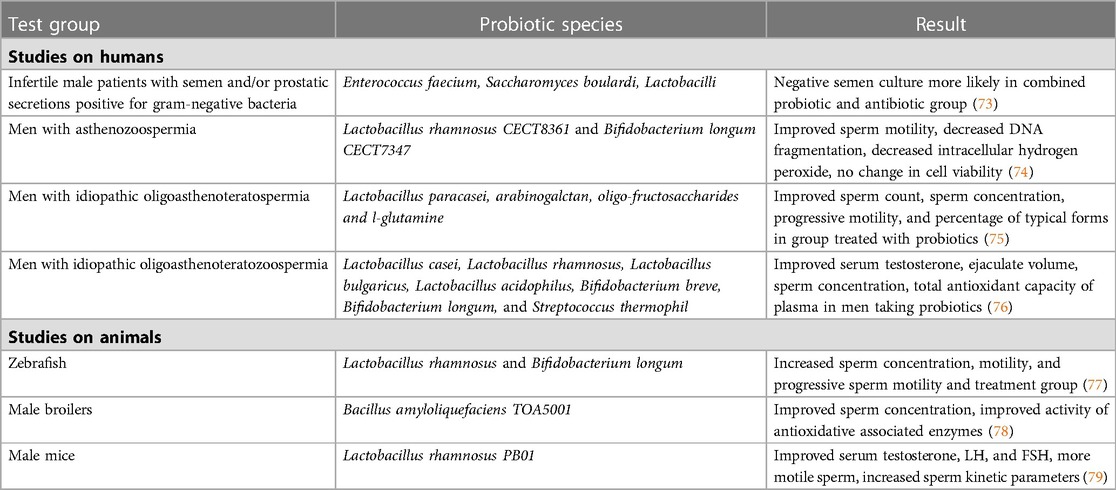
Table 1. Examples of studies on the effects of probiotics and male reproductive health and fertility.
There are very few studies that have looked at the effects of probiotic administration on male fertility not attributable to a possible genitourinary infection. In a study with zebrafish, Valcarce et al. demonstrated that supplementation with probiotic species Lactobacillus rhamnosus and Bifidobacterium longum was associated with increased sperm concentration, total motility, and progressive sperm motility in the treatment group (77). The two probiotic species also had known antioxidant properties, potentially highlighting their mechanism of action in male infertility (68). In a small pilot study of nine men with asthenozoospermia treated with the same oral antioxidant probiotic strains, sperm motility was drastically improved after 6 weeks of ingestion (74). DNA fragmentation and intracellular hydrogen peroxide were also decreased in sperm (74). Cell viability was not affected by the treatment (74). In a randomized controlled trial conducted by Maretti and Cavallini, the administration of a probiotic and prebiotic combination for six months was significantly associated with improved sperm count, ejaculate volume, sperm concentration, progressive motility and the progression of typical forms (75). The study also found the treatment to be significantly associated with an increased FSH, LH, and T levels in patients, with five men fathering a child compared to none in the control group. Another randomized control trial by Helli et al. looked at probiotic supplementation in men with idiopathic oligoasthenoteratozoospermia over a course of 10 weeks (76). In the treatment group, the ejaculate volume, number, concentration and percentage of motile sperm, and total antioxidant capacity of plasma significantly increased compared to the control, and the concentration of inflammatory markers significantly decreased (76). Probiotic supplementation led to a significant increase in sperm concentration and motility with a significant reduction in oxidative stress and inflammatory markers (76). The data from these studies is promising, but larger studies will be needed to fully understand the impact of probiotic supplementation.
Conclusion
The Human Microbiome Project (HMP) has revealed that microbiota exist in nearly every anatomical niche in the body and play a role beyond infection. An individual's microbiome is unique and changes throughout a lifetime and is influenced by factors such as genetics, diet, drugs, body size, surrounding environment, and hygiene. The gut and male reproductive system are interdependent through the microbiome, and this axis influences male reproductive health and infertility. Several studies have shown that the GM can influence testosterone levels and sperm production, modulate intestinal and androgen metabolism, and affect testicular function. Dysbiosis in the gut and reproductive system may lead to an increase in ROS and may contribute to male infertility. Treatment with probiotics offers an exciting opportunity to potentially correct microbiome dysregulation, but larger human studies are needed to fully examine this question.
Author contributions
RM and SM contributed to conception and design of the article. RM conducted literature review and wrote manuscript. SM edited manuscript. All authors contributed to the article and approved the submitted version.
Conflict of interest
The authors declare that the research was conducted in the absence of any commercial or financial relationships that could be construed as a potential conflict of interest.
Publisher's note
All claims expressed in this article are solely those of the authors and do not necessarily represent those of their affiliated organizations, or those of the publisher, the editors and the reviewers. Any product that may be evaluated in this article, or claim that may be made by its manufacturer, is not guaranteed or endorsed by the publisher.
References
1. Hood L, Rowen L. The human genome project: big science transforms biology and medicine. Genome Med. (2013) 5(9):79. doi: 10.1186/gm483
2. Relman D. The origins of human microbiota research. Nat Res. (2019) :1–5. doi: 10.1038/d42859-019-00073-5. https://www.nature.com/articles/d42859-019-00073-5#MO0
3. Turnbaugh PJ, Ley RE, Hamady M, Fraser-Liggett CM, Knight R, Gordon JI. The human microbiome project. Nature. (2007) 449(7164):804–10. doi: 10.1038/nature06244
4. Perez-Carrasco V, Soriano-Lerma A, Soriano M, Gutiérrez-Fernández J, Garcia-Salcedo JA. Urinary microbiome: yin and yang of the urinary tract. Front Cell Infect Microbiol. (2021) 11:617002. doi: 10.3389/fcimb.2021.617002
5. de Oliveira GLV, Cardoso CdB, Taneja V, Fasano A. Editorial: intestinal dysbiosis in inflammatory diseases. Front Immunol. (2021) 12:727485. doi: 10.3389/fimmu.2021.727485
6. Liu Y, Dai M. Trimethylamine N-oxide generated by the gut microbiota is associated with vascular inflammation: new insights into atherosclerosis. Mediators Inflamm. (2020) 2020:4634172. doi: 10.1155/2020/4634172
7. Ostfeld RJ, Allen KE, Aspry K, Brandt EJ, Spitz A, Liberman J, et al. Vasculogenic erectile dysfunction: the impact of diet and lifestyle. Am J Med. (2021) 134(3):310–6. doi: 10.1016/j.amjmed.2020.09.033
8. Tremellen K. Gut endotoxin leading to a decline IN gonadal function (GELDING)—a novel theory for the development of late onset hypogonadism in obese men. Basic Clin Androl. (2016) 26:7. doi: 10.1186/s12610-016-0034-7
9. Cani PD, Possemiers S, Van de Wiele T, Guiot Y, Everard A, Rottier O, et al. Changes in gut microbiota control inflammation in obese mice through a mechanism involving GLP-2-driven improvement of gut permeability. Gut. (2009) 58(8):1091–103. doi: 10.1136/gut.2008.165886
10. Javurek AB, Spollen WG, Johnson SA, Bivens NJ, Bromert KH, Givan SA, et al. Consumption of a high-fat diet alters the seminal fluid and gut microbiomes in male mice. Reprod Fertil Dev. (2017) 29(8):1602–12. doi: 10.1071/RD16119
11. Shang T, Zhang X, Wang T, Sun B, Deng T, Han D. Toll-like receptor-initiated testicular innate immune responses in mouse leydig cells. Endocrinology. (2011) 152(7):2827–36. doi: 10.1210/en.2011-0031
12. Daniel JA, Abrams MS, deSouza L, Wagner CG, Whitlock BK, Sartin JL. Endotoxin inhibition of luteinizing hormone in sheep. Domest Anim Endocrinol. (2003) 25(1):13–9. doi: 10.1016/s0739-7240(03)00042-0
13. Tolhurst G, Heffron H, Lam YS, Parker HE, Habib AM, Diakogiannaki E, et al. Short-chain fatty acids stimulate glucagon-like peptide-1 secretion via the G-protein-coupled receptor FFAR2. Diabetes. (2012) 61(2):364–71. doi: 10.2337/db11-1019
14. Topping DL, Clifton PM. Short-chain fatty acids and human colonic function: roles of resistant starch and nonstarch polysaccharides. Physiol Rev. (2001) 81(3):1031–64. doi: 10.1152/physrev.2001.81.3.1031
15. Cannarella R, Calogero AE, Condorelli RA, Greco EA, Aversa A, La Vignera S. Is there a role for glucagon-like peptide-1 receptor agonists in the treatment of male infertility? Andrology. (2021) 9(5):1499–503. doi: 10.1111/andr.13015
16. Liu R, Zhang C, Shi Y, Zhang F, Li L, Wang X, et al. Dysbiosis of gut Microbiota associated with clinical parameters in polycystic ovary syndrome. Front Microbiol. (2017) 8:324. doi: 10.3389/fmicb.2017.00324
17. Khan D, Moffett C, Moffett RC. Commentary: Emerging role of GIP and related gut hormones in fertility and PCSOS. Available at: https://www.endocrinoljournal.com/articles/commentary-emerging-role-of-gip-and-related-gut-hormones-in-fertility-and-pcos.pdf (Accessed February 6, 2023).
18. Colldén H, Landin A, Wallenius V, Elebring E, Fändriks L, Nilsson ME, et al. The gut microbiota is a major regulator of androgen metabolism in intestinal contents. Am J Physiol Endocrinol Metab. (2019) 317(6):E1182–92. doi: 10.1152/ajpendo.00338.2019
19. Harada N, Hanaoka R, Hanada K, Izawa T, Inui H, Yamaji R. Hypogonadism alters cecal and fecal microbiota in male mice. Gut Microbes. (2016) 7(6):533–9. doi: 10.1080/19490976.2016.1239680
20. Zhang P, Feng Y, Li L, Ge W, Yu S, Hao Y, et al. Improvement in sperm quality and spermatogenesis following faecal microbiota transplantation from alginate oligosaccharide dosed mice. Gut. (2021) 70(1):222–5. doi: 10.1136/gutjnl-2020-320992
21. Hao Y, Feng Y, Yan X, Chen L, Zhong R, Tang X, et al. Gut microbiota-testis axis: FMT improves systemic and testicular micro-environment to increase semen quality in type 1 diabetes. Mol Med. (2022) 28(1):45. doi: 10.1186/s10020-022-00473-w
22. Bunay J, Gallardo LM, Torres-Fuentes JL, Aguirre-Arias MV, Orellana R, Sepúlveda N, et al. A decrease of docosahexaenoic acid in testes of mice fed a high-fat diet is associated with impaired sperm acrosome reaction and fertility. Asian J Androl. (2021) 23(3):306–13. doi: 10.4103/aja.aja_76_20
23. Roqueta-Rivera M, Stroud CK, Haschek WM, Akare SJ, Segre M, Brush RS, et al. Docosahexaenoic acid supplementation fully restores fertility and spermatogenesis in male delta-6 desaturase-null mice. J Lipid Res. (2010) 51(2):360–7. doi: 10.1194/jlr.M001180
24. Cai H, Cao X, Qin D, Liu Y, Liu Y, Hua J, et al. Gut microbiota supports male reproduction via nutrition, immunity, and signaling. Front Microbiol. (2022) 13:977574. doi: 10.3389/fmicb.2022.977574
25. He S, Li H, Yu Z, Zhang F, Liang S, Liu H, et al. The gut microbiome and sex hormone-related diseases. Front Microbiol. (2021) 12:711137. doi: 10.3389/fmicb.2021.711137
26. Liu S, Cao R, Liu L, Lv Y, Qi X, Yuan Z, et al. Correlation between gut microbiota and testosterone in male patients with type 2 diabetes mellitus. Front Endocrinol. (2022) 13:836485. doi: 10.3389/fendo.2022.836485
27. Li X, Cheng W, Shang H, Wei H, Deng C. The interplay between androgen and gut microbiota: is there a microbiota-gut-testis axis. Reprod Sci. (2022) 29(6):1674–84. doi: 10.1007/s43032-021-00624-0
28. Matsushita M, Fujita K, Motooka D, Hatano K, Hata J, Nishimoto M, et al. Firmicutes in gut microbiota correlate with blood testosterone levels in elderly men. World J Mens Health. (2022) 40(3):517–25. doi: 10.5534/wjmh.210190
29. Ly LK, Rowles JL 3rd, Paul HM, Alves JMP, Yemm C, Wolf PM, et al. Bacterial steroid-17,20-desmolase is a taxonomically rare enzymatic pathway that converts prednisone to 1,4-androstanediene-3,11,17-trione, a metabolite that causes proliferation of prostate cancer cells. J Steroid Biochem Mol Biol. (2020) 199:105567. doi: 10.1016/j.jsbmb.2019.105567
30. Diviccaro S, Giatti S, Borgo F, Falvo E, Caruso D, Garcia-Segura LM, et al. Steroidogenic machinery in the adult rat colon. J Steroid Biochem Mol Biol. (2020) 203:105732. doi: 10.1016/j.jsbmb.2020.105732
31. Diviccaro S, Giatti S, Borgo F, Barcella M, Borghi E, Trejo JL, et al. Treatment of male rats with finasteride, an inhibitor of 5alpha-reductase enzyme, induces long-lasting effects on depressive-like behavior, hippocampal neurogenesis, neuroinflammation and gut microbiota composition. Psychoneuroendocrinology. (2019) 99:206–15. doi: 10.1016/j.psyneuen.2018.09.021
32. Lundy SD, Sangwan N, Parekh NV, Selvam MKP, Gupta S, McCaffrey P, et al. Functional and taxonomic dysbiosis of the gut, urine, and semen microbiomes in male infertility. Eur Urol. (2021) 79(6):826–36. doi: 10.1016/j.eururo.2021.01.014
33. Kermes K, Punab M, Lõivukene K, Mändar R. Anaerobic seminal fluid micro-flora in chronic prostatitis/chronic pelvic pain syndrome patients. Anaerobe. (2003) 9(3):117–23. doi: 10.1016/S1075-9964(03)00085-4
34. Jarvi K, Lacroix JM, Jain A, Dumitru I, Heritz D, Mittelman MW. Polymerase chain reaction-based detection of bacteria in semen. Fertil Steril. (1996) 66(3):463–7. doi: 10.1016/S0015-0282(16)58520-3
35. Willén M, Holst E, Myhre EB, Olsson AM. The bacterial flora of the genitourinary tract in healthy fertile men. Scand J Urol Nephrol. (1996) 30(5):387–93. doi: 10.3109/00365599609181315
36. Altmäe S, Franasiak JM, Mändar R. The seminal microbiome in health and disease. Nat Rev Urol. (2019) 16(12):703–21. doi: 10.1038/s41585-019-0250-y
37. Mändar R, Türk S, Korrovits P, Ausmees K, Punab M. Impact of sexual debut on culturable human seminal microbiota. Andrology. (2018) 6(3):510–2. doi: 10.1111/andr.12482
38. Liu L, Yang J, Lu F. Urethral dysbacteriosis as an underlying, primary cause of chronic prostatitis: potential implications for probiotic therapy. Med Hypotheses. (2009) 73(5):741–3. doi: 10.1016/j.mehy.2009.04.035
39. Vicari E, La Vignera S, Arcoria D, Condorelli R, Vicari LO, Castiglione R, et al. High frequency of chronic bacterial and non-inflammatory prostatitis in infertile patients with prostatitis syndrome plus irritable bowel syndrome. PLoS One. (2011) 6(4):e18647. doi: 10.1371/journal.pone.0018647
40. Vicari E, La Vignera S, Castiglione R, Condorelli RA, Vicari LO, Calogero AE. Chronic bacterial prostatitis and irritable bowel syndrome: effectiveness of treatment with rifaximin followed by the probiotic VSL#3. Asian J Androl. (2014) 16(5):735–9. doi: 10.4103/1008-682X.131064
41. Mändar R, Punab M, Korrovits P, Türk S, Ausmees K, Lapp E, et al. Seminal microbiome in men with and without prostatitis. Int J Urol. (2017) 24(3):211–6. doi: 10.1111/iju.13286
42. Wang B, Yao M, Lv L, Ling Z, Li L. The human microbiota in health and disease. Proc Est Acad Sci Eng. (2017) 3(1):71–82. doi: 10.1016/J.ENG.2017.01.008
43. Weng SL, Chiu CM, Lin FM, et al. Bacterial communities in semen from men of infertile couples: metagenomic sequencing reveals relationships of seminal microbiota to semen quality. PLoS One. (2014) 9(10):e110152. doi: 10.1371/journal.pone.0110152
44. Sengupta P, Roychoudhury S, Nath M, Dutta S. Oxidative stress and idiopathic male infertility. In: Kesari KK, Roychoudhury S, editors. Oxidative stress and toxicity in reproductive biology and medicine. Advances in Experimental Medicine and Biology. Cham: Springer (2022). Vol. 1358. doi: 10.1007/978-3-030-89340-8_9
45. Agarwal A, Baskaran S, Parekh N, Cho CL, Henkel R, Vij S, et al. Male infertility. Lancet. (2021) 397(10271):319–33. doi: 10.1016/S0140-6736(20)32667-2
46. Henkel RR. Leukocytes and oxidative stress: dilemma for sperm function and male fertility. Asian J Androl. (2011) 13(1):43–52. doi: 10.1038/aja.2010.76
47. Agarwal A, Parekh N, Panner Selvam MK, Henkel R, Shah R, Homa ST, et al. Male oxidative stress infertility (MOSI): proposed terminology and clinical practice guidelines for management of idiopathic male infertility. World J Mens Health. (2019) 37(3):296–312. doi: 10.5534/wjmh.190055
48. Tvrdá E, Benko F, Ďuračka M. Oxidative stress as an underlying mechanism of bacteria-inflicted damage to male gametes. Oxygen. (2022) 2(4):547–69. doi: 10.3390/oxygen2040036
49. Tvrdá E, Lovíšek D, Gálová E, Schwarzová M, Kováciková E, Kunová S, et al. Possible implications of bacteriospermia on the sperm quality, oxidative characteristics, and seminal cytokine network in normozoospermic men. Int J Mol Sci. (2022) 23(15):8678. doi: 10.3390/ijms23158678
50. Lv JL, Wu QJ, Wang XB, Du Q, Liu FH, Guo RH, et al. Intake of ultra-processed foods and asthenozoospermia odds: a hospital-based case-control study. Front Nutr. (2022) 9:941745. doi: 10.3389/fnut.2022.941745
51. Luca M, Di Mauro M, Di Mauro M, Luca A. Gut microbiota in Alzheimer's disease, depression, and type 2 diabetes mellitus: the role of oxidative stress. Oxid Med Cell Longev. (2019) 2019:4730539. doi: 10.1155/2019/4730539
52. Farahani L, Tharakan T, Yap T, Ramsay JW, Jayasena CN, Minhas S. The semen microbiome and its impact on sperm function and male fertility: a systematic review and meta-analysis. Andrology. (2021) 9(1):115–44. doi: 10.1111/andr.12886
53. Doroftei B, Ilie OD, Dabuleanu AM, Hutanu D, Vaduva CC. A retrospective narrative Mini-review regarding the seminal Microbiota in infertile male. Medicina (Kaunas). (2022) 58(8):1067. doi: 10.3390/medicina58081067
54. Tomaiuolo R, Veneruso I, Cariati F, D’Argenio V. Microbiota and human reproduction: the case of male infertility. High Throughput. (2020) 9(2):10. doi: 10.3390/ht9020010
55. Wang H, Xu A, Gong L, Chen Z, Zhang B, Li X. The microbiome, an important factor that is easily overlooked in male infertility. Front Microbiol. (2022) 13:831272. doi: 10.3389/fmicb.2022.831272
56. Alfano M, Ferrarese R, Locatelli I, Ventimiglia E, Ippolito S, Gallina P, et al. Testicular microbiome in azoospermic men-first evidence of the impact of an altered microenvironment. Hum Reprod. (2018) 33(7):1212–7. doi: 10.1093/humrep/dey116
57. Hou D, Zhou X, Zhong X, Settles ML, Herring J, Wang L, et al. Microbiota of the seminal fluid from healthy and infertile men. Fertil Steril. (2013) 100(5):1261–9. doi: 10.1016/j.fertnstert.2013.07.1991
58. Monteiro C, Marques PI, Cavadas B, Damião I, Almeida V, Barros N, et al. Characterization of microbiota in male infertility cases uncovers differences in seminal hyperviscosity and oligoasthenoteratozoospermia possibly correlated with increased prevalence of infectious bacteria. Am J Reprod Immunol. (2018) 79(6):e12838. doi: 10.1111/aji.12838
59. Garcia-Segura S, Del Rey J, Closa L, Garcia-Martínez I, Hobeich C, Castel AB, et al. Seminal microbiota of idiopathic infertile patients and its relationship with sperm DNA integrity. Front Cell Dev Biol. (2022) 10:937157. doi: 10.3389/fcell.2022.937157
60. Gilbert JA, Blaser MJ, Caporaso JG, Jansson JK, Lynch SV, Knight R. Current understanding of the human microbiome. Nat Med. (2018) 24(4):392–400. doi: 10.1038/nm.4517
61. Whiteside SA, Razvi H, Dave S, Reid G, Burton JP. The microbiome of the urinary tract–a role beyond infection. Nat Rev Urol. (2015) 12(2):81–90. doi: 10.1038/nrurol.2014.361
62. Vodstrcil LA, Twin J, Garland SM, Fairley CK, Hocking JS, Law MG, et al. The influence of sexual activity on the vaginal microbiota and Gardnerella vaginalis clade diversity in young women. PLoS One. (2017) 12(2):e0171856. doi: 10.1371/journal.pone.0171856
63. Nelson DE, Dong Q, Van der Pol B, Toh E, Fan B, Katz BP, et al. Bacterial communities of the coronal sulcus and distal urethra of adolescent males. PLoS One. (2012) 7(5):e36298. doi: 10.1371/journal.pone.0036298
64. Mändar R, Punab M, Borovkova N, Lapp E, Kiiker R, Korrovits P, et al. Complementary seminovaginal microbiome in couples. Res Microbiol. (2015) 166(5):440–7. doi: 10.1016/j.resmic.2015.03.009
65. Wittemer C, Bettahar-Lebugle K, Ohl J, Rongières C, Viville S, Nisand I. Colonisation bactérienne vaginale anormale et implantation en assistance médicale à la procréation. Gynecol Obstet Fertil. (2004) 32(2):135–9. doi: 10.1016/j.gyobfe.2003.11.017
66. Teveroni E, Di Nicuolo F, Vergani E, Bruno C, Maulucci G, Bianchetti G, et al. Short-chain fatty acids modulate sperm migration through olfactory receptor 51E2 activity. Int J Mol Sci. (2022) 23(21):12726. doi: 10.3390/ijms232112726
67. Guarner F, Khan AG, Garisch J, Eliakim R, Gangl A, Thomson A, et al. World gastroenterology organisation global guidelines: probiotics and prebiotics October 2011. J Clin Gastroenterol. (2012) 46(6):468–81. doi: 10.1097/MCG.0b013e3182549092
68. Hashem NM, Gonzalez-Bulnes A. The use of probiotics for management and improvement of reproductive eubiosis and function. Nutrients. (2022) 14(4):902. doi: 10.3390/nu14040902
69. Chenoll E, Moreno I, Sánchez M, Garcia-Grau I, Silva á, González-Monfort M, et al. Selection of new probiotics for endometrial health. Front Cell Infect Microbiol. (2019) 9:114. doi: 10.3389/fcimb.2019.00114
70. Russo R, Edu A, De Seta F. Study on the effects of an oral lactobacilli and lactoferrin complex in women with intermediate vaginal microbiota. Arch Gynecol Obstet. (2018) 298(1):139–45. doi: 10.1007/s00404-018-4771-z
71. López-Moreno A, Aguilera M. Vaginal probiotics for reproductive health and related dysbiosis: systematic review and meta-analysis. J Clin Med Res. (2021) 10(7):1461. doi: 10.3390/jcm10071461
72. Molina NM, Sola-Leyva A, Saez-Lara MJ, Plaza-Diaz J, Tubic-Pavlovic A, Romero B, et al. New opportunities for endometrial health by modifying uterine microbial composition: present or future? Biomolecules. (2020) 10(4):593. doi: 10.3390/biom10040593
73. Grande G, Pompa G, Astorri AL, Pontecorvi A, Milardi D. Association of probiotic treatment with antibiotics in male accessory gland infections. Am J Mens Health. (2022) 16(5):15579883221119064. doi: 10.1177/15579883221119064
74. Valcarce DG, Riesco MF, Martínez-Vázquez JM, Robles V, et al. Probiotic administration improves sperm quality in asthenozoospermic human donors. Benef Microbes. (2017) 8(2):193–206. doi: 10.3920/BM2016.0122
75. Maretti C, Cavallini G. The association of a probiotic with a prebiotic (Flortec, Bracco) to improve the quality/quantity of spermatozoa in infertile patients with idiopathic oligoasthenoteratospermia: a pilot study. Andrology. (2017) 5(3):439–44. doi: 10.1111/andr.12336
76. Helli B, Kavianpour M, Ghaedi E, Dadfar M, Haghighian HK. Probiotic effects on sperm parameters, oxidative stress index, inflammatory factors and sex hormones in infertile men. Hum Fertil. (2022) 25(3):499–507. doi: 10.1080/14647273.2020.1824080
77. Valcarce DG, Riesco MF, Martínez-Vázquez JM, Robles V. Diet supplemented with antioxidant and anti-inflammatory probiotics improves sperm quality after only one spermatogenic cycle in zebrafish model. Nutrients. (2019) 11(4):843. doi: 10.3390/nu11040843
78. Inatomi T, Otomaru K. Effect of dietary probiotics on the semen traits and antioxidative activity of male broiler breeders. Sci Rep. (2018) 8(1):5874. doi: 10.1038/s41598-018-24345-8
Keywords: male infertility, microbiome, probiotics, andrology, testicular microbiota, oxidative stress, azoospermia
Citation: Magill RG and MacDonald SM (2023) Male infertility and the human microbiome. Front. Reprod. Health 5:1166201. doi: 10.3389/frph.2023.1166201
Received: 14 February 2023; Accepted: 23 May 2023;
Published: 9 June 2023.
Edited by:
Alberto Ferlin, University of Padua, ItalyReviewed by:
Giuseppe Grande, University of Padua, ItalyAndrea Delbarba, Asst degli Spedali Civili di Brescia, Italy
© 2023 Magill and MacDonald. This is an open-access article distributed under the terms of the Creative Commons Attribution License (CC BY). The use, distribution or reproduction in other forums is permitted, provided the original author(s) and the copyright owner(s) are credited and that the original publication in this journal is cited, in accordance with accepted academic practice. No use, distribution or reproduction is permitted which does not comply with these terms.
*Correspondence: Resa G. Magill resa.magill@gmail.com