- 1Faculty of Science, Athena Institute, Vrije Universiteit Amsterdam, Amsterdam, Netherlands
- 2Procurement and Finance in Supply Chain Management, Fraunhofer Institute for Material Flow and Logistics IML, Dortmund, Germany
- 3Department of Product Design, Oslo Metropolitan University, Oslo, Norway
Introduction: Blockchain technology (BCT) has been proposed as a solution for many challenges facing global food systems, including environmental issues related to carbon, biodiversity, and other ecosystem services. Efforts to evaluate the potential of BCT to contribute to these issues are nascent and, in particular, little research has been conducted with the diversity of food system actors who will be implicated in and affected by the use of BCT.
Methods: This paper draws on a series of eleven co-creative workshops conducted with a wide range of food system actors, and highlights narratives about core issues regarding BCT’s impact on and relation to various challenges facing the food system, including environmental crises such as climate change and biodiversity loss. The workshops were conducted in 2023 and 2024, and involved over 100 participants including farmers, researchers, blockchain developers, policymakers, agribusiness companies, and other actors. These workshops began by developing idealized visions of blockchain-based future food systems, interrogated the barriers between the present and these futures, and co-created strategies to overcome these barriers.
Results: The data that emerged from these workshops, including audio recordings as well as digital whiteboards, were subject to thematic analysis. We present three scenarios of BCT-enabled future food systems, which were produced from this analysis. Subsequently, we highlight four tensions–related to decentralization, inclusion, transparency, and tokenization–underlying these scenarios.
Discussion: How the core tensions we identified can be linked to the broader trend of datafication is discussed. We emphasis that how these tensions are negotiated between different, disparate actors will have significant implications on the impacts that BCT may have on food system transformation.
Introduction
Blockchain technology (BCT) is a contentious, flexible technology surrounded by much hype. As such, it remains unclear precisely whether, in what context, and to what ends BCT may be useful. However, the growing interest in BCT amongst powerful actors (e.gs., WEF, 2020; WEF, 2023), its status as a “disruptive” technology (Ronaghi, 2021), and its connection to topics of transparency (Bernards et al., 2024) and ownership (Ito and O’Dair, 2019; Wang et al., 2019), among many others, means that much is at stake regarding how and to what ends the technology is implemented. BCT’s original uses were in the financial sector, but since its emergence with Bitcoin in 2009, the technology has been applied to a wide range of sectors and uses beyond finance (Zhao et al., 2016). In the context of agriculture, BCT is one of a suite of “Agriculture 4.0” technologies, along with others such as Internet of Things (IoT) and artificial intelligence (AI), which are ostensibly poised to usher in a new agricultural revolution (Klerkx and Rose, 2020). This paper will focus on the use of BCT as it is applied in efforts towards sustainable food system transformation.
BCT is a flexible technology that has many uses beyond those related to Bitcoin and other cryptocurrencies. There are many ways in which BCT can be applied to the food system, and numerous sustainability challenges that it is used in an attempt to address. For example, BCT is used in the context of tracking and tracing food products as they travel through increasingly complex supply chains (Xiong et al., 2020; Kechagias et al., 2023); to digitally represent food products and various features associated with them, such as certifications (Kononets and Treiblmaier, 2021), carbon sequestration (Wesbuer et al., 2023), and other ecosystem services (Hartley and Rennie, 2023); among many other uses (Motta et al., 2020). Proponents assert that BCT can act as a disruptive solution to such diverse food system challenges as managing food waste, improving traceability systems, and contributing to shifts towards more sustainable production systems (Pakseresht et al., 2023). However, the true potential remains uncertain. For example, van Hilten et al., 2020, find evidence for the efficacy of BCT for traceability but also note that “whole-chain traceability … does not necessarily require blockchain” (2020).
The EU has recently defined web4 as “the expected fourth generation of the World Wide Web. Using advanced artificial and ambient intelligence, the internet of things, trusted blockchain transactions, virtual worlds and XR capabilities, digital worlds and real objects and environments are fully integrated and communicate with each other” (European Commission, 2023). The technological building blocks will fundamentally change the architecture of the internet and include trust technologies such as blockchain, decentralized identity, privacy-enhancing technologies, cybersecurity and quantum technologies but also AI, IoT, extended reality and future communication networks (including 5G/6G). Collectively, they will allow to blend the physical and digital world in real time (Barcevičius et al., 2025). This blend is exemplified by the various uses of BCT in food system contexts that serve to create integrations between BCT, a key component of web3, with the realities of the food system. The focus on digitally representing real-world objects and their value on blockchains and the effort to leverage BCT to contribute to sustainable transformation of food systems makes this area a prime example of the development of “web4”.
BCT is poised to have a significant impact on various aspects of the food system. However, after almost a decade of applying BCT to food systems the precise impacts and for whom remain uncertain as numerous actors within the food system attempt to understand and negotiate how to best make use of BCT. A key research gap within this area is a dearth of “real world” perspectives on and experiences with BCT and food systems, and especially those of non-experts, such as primary producers (Frizzo-Barker et al., 2020; Wünsche and Fernqvist, 2022). Including such perspectives in research on BCT and food systems can illuminate the diverse values, goals, visions, and objectives that different actors have regarding BCT. How these differences are negotiated will influence how BCT will continue to develop, what forms of BCT are adopted and for what purposes, and the extent and pattern of adoption, therefore influencing BCT’s impact on various aspects of food system sustainability.
A wide range of food system actors were gathered to discuss their perspectives on the various uses of BCT in food system contexts within the EU-Horizon TRUSTyFOOD project. Ultimately, the objectives of the TRUSTyFOOD project include cutting through the hype surrounding BCT and developing actionable strategies for the effective use of BCT in the context of sustainable food system transformation. A core component of the TRUSTyFOOD project involves engagement with a wide range of food system stakeholders (TRUSTyFOOD, 2023). The motivation is’ to gather the perspectives of a diversity of actors who will be implicated in and affected by the use of BCT in food systems, rather than exclusively highlighting expert perspectives. Thus, we pose the following main research question guiding this paper: what are the perspectives and visions of food system actors regarding BCT’s potential to contribute to sustainable food system transformation? As our question specifically concerns the impact of BCT on sustainability of the food system, we will primarily emphasize connections between BCT and issues of environmental sustainability.
Below, we will provide an overview of BCT, its use in food system contexts, and the growing body of research concerning this intersection. Following this, we provide context regarding the TRUSTyFOOD research activities upon which the present research draws, as well as the analytical approach employed. Then, we present our findings in the form of three scenarios of BCT-enabled future food systems derived from workshops held within TRUSTyFOOD, and use this to launch into an exploration of four core tensions underlying different perspectives on BCT. We conclude by connecting these underlying tensions to the broader trend of datafication and discussing areas for future research at the intersection of BCT and sustainable food system transformation.
Blockchain
BCT is, simply put, a distributed digital ledger. It is characterized by a continuously growing list of entries that are combined into blocks, which are cryptographically linked and maintained by a decentralized computer network (Nakamoto, 2008). BCT is therefore also referred to as trustless technology, as trust is not realized via an intermediary, but via the reliability of the technology itself (Filippi and Reijers, 2020). Since 2009, it has served as the technological backbone behind the cryptocurrency Bitcoin (Zhao et al., 2016). Over time, other types of blockchain–including those that do not make use of cryptocurrencies–have emerged to expand the application area of the new technology to other domains such as agriculture. This development was driven by the introduction of new blockchains such as Ethereum that extended the original distributed ledger model of Bitcoin by introducing programmability through so-called smart contracts. Smart contracts are computer programs that are executed on a distributed computer network to simulate “a virtual and global world computer with a shared state” (Dameron, 2018). The programmability of smart contracts can be used to digitally represent tangible and intangible assets on a blockchain and enable the digital verification of ownership of these digital assets (analogous to the representation of electronic coins and whom they belong to in Bitcoin). This process is referred to as tokenization (Samaties et al., 2023). Currently, blockchain is seen as a key technology behind web3, which is founded on principles such as open-source, decentralization, verifiable consensus, and cooperative governance and ownership structures (Beck, 2022; McKinsey, 2023).
As a result of technological development since BCT’s emergence, the precise design of specific blockchains and blockchain systems is subject to a high degree of variation (Tripathi et al., 2023). Consensus mechanisms, smart-contract functionality, permissions, among other features vary from blockchain to blockchain. Regardless, the typical configuration of BCT systems results in shared databases that exist on multiple servers simultaneously, typically without one centralized point of control. In theory, public blockchains such as Bitcoin and Ethereum allow anyone with internet access to read or write data to the shared ledger that is maintained by the decentralized computer network (WEF, 2020). On the other hand, a private blockchain is maintained by an organization or group of people who can impose restrictions on who can join the network, or who can read and write data to the shared ledger (Ledger, 2023).
Due to these general technical configurations, blockchain is typically associated with the functionalities of decentralization (due to the absence of a centralized authority or server), transparency (due to the open accessibility to view data on blockchains like bitcoin), and immutability (as consensus mechanisms like proof-of-work, used by bitcoin, generally preclude retroactive data manipulation by individual actors) (Curry, 2025; Wünsche and Fernqvist, 2022). Within academic literature, these features are often associated with the potential benefits of efficiency, cost savings, accountability, and coordination (Wünsche and Fernqvist, 2022).
Blockchain applications related to food system sustainability
Due to the flexible nature of BCT, it can be applied to the food system in many different ways; Wassenaer et al. raise the importance of properly assessing the variability of BCT systems in practice with their three-tiered analytical framework, focusing on the ledger, governance, and the broader ecosystem (2021b). Some of the earliest significant examples of applying BCT in food system contexts include data sharing and traceability along complex supply chains or for carbon accounting and management (e.gs., Kharif, 2018; Condon and Thackray, 2021, for early examples respectively). A recent WEF report, for example, explores the potential for BCT to contribute to facilitating climate action and draws on interviews from projects such as Regen Network and Reseed.farm, each of which have close involvement in agriculture (WEF, 2023). Similarly, a UNDP report explored the potential for BCT to contribute to improving traceability and transparency within food systems (UNDP, 2021). Accompanying this institutional interest in BCT are numerous projects, such as Open Food Chain or TRACT for traceability, Regen Network and EthicHub attempting to facilitate climate action, and others such as Etherisc using BCT for crop insurance.
Hsu and Schletz, 2023, drawing on a number of case studies, investigate how policy can be aligned to most effectively take advantage of the potential for digital technologies such as BCT to contribute to addressing climate change (2023). The potential of applying BCT for climate change initiatives specifically for agriculture has gained interest (van Wassenaer et al., 2021a; van Wassenaer et al., 2021b). For example, traceability is seen as one main enabler of the Circular Economy. In that regard, several authors discussed how the traceability of BCT supports the transition of food systems towards the Circular Economy to prevent food loss, encourage organic farming practices or ensure biodiversity (Pakseresht et al., 2023; Sharma et al., 2021). Recently, a new trend within Web3, known as Regenerative Finance (ReFi), has emerged. ReFi aims to create a financial system based on regenerative economic principles and BCT to address the global coordination challenges on how to govern global common pool resources (such as the atmosphere) (Schletz et al., 2023) and how to efficiently allocate resources to relevant climate initiatives (Hartley and Rennie, 2023).
Hull et al., 2021 critically examine the dominant narratives on the potential of blockchain in climate governance to enhance reliability, transparency, accountability and democratic quality in climate governance (2021). They conclude that the narratives mainly privilege a tech-driven and market-oriented (focusing particularly on carbon markets) approach to climate governance (ibid). However, Schletz et al. point out, that relying on technology and carbon markets alone are not sufficient to solve climate change relevant challenges of social coordination and economic exchange. On the contrary, BCT may risk perpetuating the status quo of speculation and extractive logic that regards nature as a commodity (2023). In this sense, it is important to note that most, if not all, of the problems (traceability, smart farming, crop insurance, etc.) to which BCT is applied in food systems do not necessarily require the involvement of BCT. As noted by van Hilten et al. note, there are other options for traceability systems. As such, it is useful to consider possible elements of “solutionism” (Morozov, 2013; Guthman, 2024) in the drive to apply blockchain to food systems. Solutionism typically involves “begin[ning] with an already conceived product or device and then … searching for a problem for which it can be put to use” (Guthman, 2024, pp. 10).
In light of the growing interest in applying BCT to food systems, research on this intersection is emerging, but still nascent. For example, Feng et al., 2020, based on a literature review, argue that blockchain has “great potential for improving traceability performance by providing security and full transparency” but caution that current understanding of its context-specific characteristics and variation is lacking (2020). Similarly, Xiong et al. focus on traceability and potential for BCT, as a system for securing and sharing data, for enabling the development of data-driven innovations related to, for example, “smart farming and smart index-based agricultural insurance” (2020). They go on to identify potential challenges of costs, interoperability, and the motivation of involved actors (ibid). Further, the use of BCT for such purposes is subject to the “garbage-in, garbage-out” (GIGO) problem. This is also referred to as the “blockchain oracle problem”, reflecting the fact that BCT systems do not have direct access to data outside of the blockchain itself, resulting in unique challenges related to data veracity (e.g., Caldarelli, 2020).Many other authors offer similar contributions that contain conceptual optimism about blockchain’s potential without introducing new empirical insights (e.gs., Rejeb et al., 2020; Sendros et al., 2022; Mohammed et al., 2023). An excess of conceptual work unbalanced by empirical insights from the numerous real-world attempts to apply BCT to food systems poses a major challenge for this growing body of literature (Frizzo-Barker et al., 2020).
Barring few exceptions, most of the research on BCT in the context of its use in food systems focuses on its technical features, and often at a highly conceptual level (Frizzo-Barker et al., 2020). What empirical work has been conducted primarily centers on blockchain developers, entrepreneurs, and other experts. Kohler and Pizzol, 2020 conducted a technology assessment of blockchain in food supply chains, using six case studies with blockchain service providers to develop their assessment (2020). Similarly, Van Hilten et al. conducted case studies of blockchain applications to organic food supply chains, focusing again on collecting qualitative data from technology providers (2020). Wünsche and Fernqvist, in their general study on the potential of blockchain technology in sustainable food system transformation, similarly conducted interviews exclusively with technology providers (2022). Ordoñez et al., 2024 came to a similar conclusion in their systematic review for using BCT to improve agricultural sustainability in South America. The majority of articles were narrative reviews or purely technical investigations without relevant information on the implementation in sustainable agriculture (2024).
While these offer valuable contributions, they focus on actors in positions of power. Wünsche and Fernqvist highlight succinctly the need to bring a broader range of stakeholders into research on this topic: “future research should include qualitative assessment of firms throughout the chain, including upstream supply chain players (e.g., farmers and smaller agri-food firms) as their use of the technology must function in their daily operations” (2022). Parry and Collomosse explore what ‘good’ means in the context of ‘blockchain for good’ and assert that we need to consider impacts for different actors as well as different conceptualizations of goodness, fairness, worth, and value (Parry and Collomosse, 2021). In this effort, it is crucial to consider the perspectives of diverse stakeholders that may be impacted by BCT’s use in food systems. Research of this kind is valuable, as collaboration and partnerships between stakeholders may be crucial for the ongoing development of BCT (Motta et al., 2020).
Little research involving actors within the food system, specifically “upstream” actors such as producers, who will be involved in and affected by BCT systems has been conducted, representing a substantial research gap. Some notable exceptions are Singh et al. (2022), Thompson and Rust (2023), Dudder et al. (2021), and Heitlinger et al. (2021), each of which have offered valuable insights.
Thompson and Rust, in their investigation of challenges surrounding BCT adoption in Australian seafood supply chains, provide valuable insights into how tensions between different stakeholder positions may influence the adoption of BCT and thus its potential impact. Some actors within these supply chains were resistant to sharing certain information in a transparent, openly accessible blockchain environment because their business models are to some extent dependent on keeping information private. This impact of BCT could compromise the market position of these actors. As a result, Thompson and Rust assert “that blockchain will only spur transformative change if the most influential supply chain actors can see value in using it” (2023). Part of our research objectives are to explore potential differences and contradictions in perspectives amongst different actors (i.e., where and under what circumstances they “see value” in BCT) and how this might influence BCT and its potential. Similarly, Singh et al.’s research on Colombian coffee farmers addresses this research gap by illustrating differential expectations and abilities to influence BCT systems amongst different actors within complex supply chains (2022). Dudder et al describe the need to provide thorough education on BCT systems to a wide range of actors, and they report positive impacts from a pilot project that aided participants in gaining valuable knowledge and experience related to the technology (2021). Heitlinger et al. explore the alignment between BCT, notions of food commons, and creating more convivial relationships with nature and nonhumans (2021).
The aim of this paper is to contribute to addressing the lack of diverse perspectives in research on BCT and food system by exploring the perspectives of wide range of food system actors on BCT and its potential to effect change. Through this, we are able to focus on the levels of governance and ecosystem–referring to the management of a specific BCT project and the broader sets of interrelations, respectively–within Wassenaer et al.’s framework (2021b). Adding to the contributions above, our research offers insights drawn from throughout and centering on the EU food system.
Methodology
Context of the project
TRUSTyFOOD is a 3-year research initiative funded by the European Commission that started in July 2022. It explores the role of blockchain technology in transforming the agri-food sectors over the coming years. TRUSTyFOOD involves the collaboration of 13 consortium partners, based throughout Europe and in Northern Africa. The project also aims to provide guidance to policymakers on supporting investments that will help farmers and food producers adopt this technology. The TRUSTyFOOD project seeks to elucidate the current incomplete and fragmented understanding of blockchain technology applications within the agri-food sector; one aim of TRUSTyFOOD is thus to cut through the hype surrounding BCT (TRUSTyFOOD, 2023).
Stakeholder engagement process
The methodology of this study is centered on the TRUSTyFOOD stakeholder engagement process, designed to collaboratively explore and shape sustainable future food systems. This process was structured around a series of 11 online, co-creative focus-group discussions, which incorporated several participatory techniques to achieve its objectives. The process facilitated active participation from diverse stakeholders, providing a platform for expansive discussions and the integration of multi-sectoral perspectives.
The stakeholder engagement process was designed primarily by drawing on the methodology of backcasting (Vergagt and Quist, 2011) and the three horizons framework (Sharpe et al., 2016). Backcasting is a future-oriented methodology that begins by developing “normative” (Vergagt and Quist, 2011; Jiren et al., 2023) or “ideal-type” (Brown, Harris, and Russell, 2010) scenarios of the future, working backwards to identify gaps between these ideal futures and the present, and finally developing strategies to overcome these gaps. Processes such as this “can lead to recommendations and even transformations of for instance food system (R&I) policies, priorities, strategies, investments, socioeconomic research and innovation systems, behaviours and attitudes, education, products and services” (Athena InstituteVU University, 2018, p. 2).
Each of the three steps in the backcasting process–visioning, exploring gaps in the present, and developing strategies to realize ideal futures–aligns with one of the three phases in the stakeholder engagement process (Table 1). The aim of the first phase was to co-create shared visions of sustainable food systems and identify actionable pathways to address critical environmental issues through the workshops. In the second phase, participants explored barriers and drivers related to using BCT to achieve the visions derived from phase I. Finally, in phase III, workshop participants worked to co-create strategies and pathways that could aid with overcoming the barriers from phase II and realize the visions from phase I.
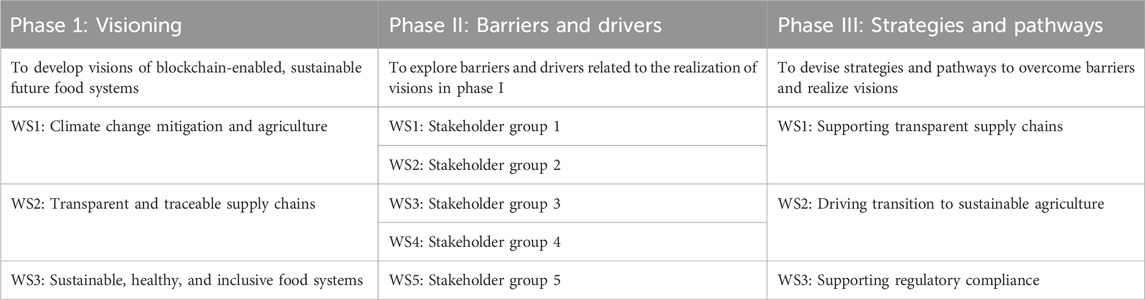
Table 1. Overview of workshop (WS) themes and foci per phase of TRUSTyFOOD sectoral working groups. Phase I and III involved three workshops with heterogenous groups of stakeholders, while phase II involved five workshops with homogenous groups.
These workshops allowed for in-depth discussions and collaborative problem-solving. The sectoral working groups were composed of stakeholders from various sectors, including agriculture, food production, policy-making, and environmental advocacy; a breakdown of the stakeholder categories used throughout the TRUSTyFOOD’s stakeholder engagement is given in Table 2. Overall, participation in the workshops involved actors with traditional expertise–such as BCT developers and technically oriented researchers–as well as ample quantities of “upstream” supply chain players (Wünsche and Fernqvist, 2022) such as farmers. Further, efforts were taken to ensure that a reasonable balance of actors who were skeptical or critical of BCT were involved in the workshops. The majority of sampling took place through the networks of the various consortium partners1, and thus leverages the rich, existing networks in which each partner is embedded. As a result, these workshops involved participation from a diverse range of actors, primarily from the EU, involved to some extent with BCT’s application to food system contexts. This created a unique opportunity for collaboration and discussion amongst these actors and adds to the value of the present research.
A total of 124 stakeholders participated within the 11 total workshops conducted throughout the sectoral working groups. Some participants, such as BCT developers, held high degrees of technical expertise concerning BCT, while others held experiential knowledge about BCT through involvement with pilot projects or exploring implementing it in their own operations. Similarly, participants held a range of levels and forms of expertise regarding the food and agriculture system. This diversity within the workshops ensured that a wide range of views and expertise were considered, fostering a holistic approach. Workshops lasted 2 h each and employed participatory techniques to facilitate stakeholder engagement and allow for in-depth discussions and collaborative problem-solving.
Data collection methods
Visioning sessions (phase I) formed the core component of the analysis. These sessions were designed to collaboratively develop shared visions of sustainable future food systems. Stakeholders engaged in deliberations to articulate aspirations, goals, and priorities for the future, ensuring a co-created and inclusive approach to envisioning sustainable food systems. During these sessions, stakeholders engaged in various activities to articulate visions of sustainable future food systems and BCT’s role in these imagined futures. This approach helped in creating detailed and actionable visions that were grounded in current realities.
In each visioning workshop, participants were invited to imagine a future food system that would exist around 25–30 years in the future. They were first asked to fill in this vision, discussing different aspects that they imagined (Davies, 2014). Following this more general exercise, participants would discuss the role of BCT in their general visions of future food systems. Each of the three visioning workshops (phase I) was structured around a specific theme, illustrated in Table 1 above. Participants would work on a digital whiteboard (e.g., Google Jamboard, Miro) as well as discussing verbally through the video calling application.
In addition to visioning, barriers and drivers sessions (phase II) focused on identifying key factors that hinder or promote the transition to sustainable food systems. These sessions also included discussions on pathways to overcome barriers and leverage drivers, with particular emphasis on relevant environmental issues. Strategies to address these barriers and leverage drivers were co-created (phase III), ensuring that the proposed solutions were practical and supported by the stakeholders. Workshops in phases I and III involved heterogeneous mixtures of stakeholder groups. In contrast, phase II workshops involved one workshop per stakeholder group (Table 2) in order minimize power imbalances and provide an open space for candid discussions.
Analytical approach
Scenario development was a key analytical framework used to synthesize findings and generate project deliverables. For example, preliminary scenarios were presented to participants as part of the introduction to phase II workshops, as well as being shared internally within the TRUSTyFOOD consortium to garner input from all consortium members. Scenarios were developed iteratively to explore potential trajectories for achieving sustainable food systems. These scenarios served as tools to organize and communicate complex interrelations among environmental, social, and economic factors. The scenario development process involved creating three scenarios that represented different possible futures. A systematic abductive and iterative coding process was employed in parallel with scenario development. The analysis began with an inductive approach, drawing out key themes directly from the data; this stage involved only data from phase I workshops. This initial exploration informed the development of a codebook that identified key environmental issues as primary analytical categories. Subsequently, a deductive coding process was applied to further analyze the data using the established codebook; at this point, our analysis expanded to selectively include data from workshops in phases II and III. This iterative approach ensured that the analysis remained grounded in the data while also allowing for refinement and validation of the thematic structure. The abductive approach allowed for the integration of new insights and theories as they emerged from the data, while the iterative nature of the coding process ensured that the analysis was thorough and comprehensive. This combination of inductive and deductive methods provided a robust framework for understanding the complex dynamics of sustainable food systems. A description of how data from each phase entered into the analytical process is given in Figure 1.
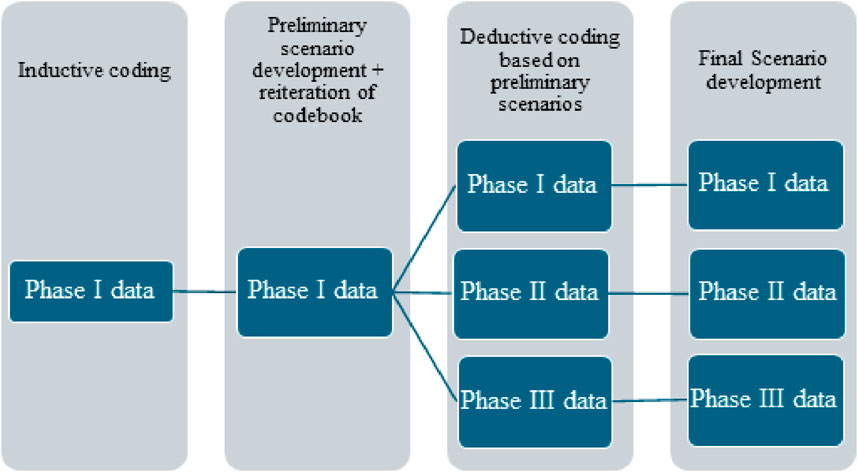
Figure 1. Depiction of abductive coding procedure. Initial codes and scenarios were developed exclusively based on Phase I data. Phase II and III data were integrated selectively in the deductive phase of coding as well as in the final scenario development.
The analysis extended beyond thematic identification to explore interrelations among the identified elements. Specifically, the relationships between environmental issues and other contextual factors were examined to uncover underlying tensions. These interrelations provided insights into the systemic dynamics of food systems and informed the development of robust, integrative scenarios.
Results and discussion
Data analysis began immediately following the completion of phase I workshops. Our initial analysis was primarily intended to provide information and framing for participants in phase II workshops, and therefore focused on generating a broad, comprehensive picture of the phase I workshops. In this stage, we were able to identify core driving values–such as transparency, decentralization, or bottom-up participation–that would ultimately play a role in differentiating the scenarios. Additionally, our initial analysis highlighted areas in the discussions connected to environmental issues, such as regarding energy use or financing sustainable transformations. A secondary component of this stage of analysis was identifying contradictory points and ideas within phase I discussions; this served an immediate function of preparing the research team for phase II workshops, given the focus of that phase (barriers and drivers). This first step in analysis provided the building blocks for the first version of the scenarios.
Following phase II workshops, our analysis deepened. We built on and refined preliminary contradictions identified in the first round of workshops and, through this, produced the first iteration of scenarios. These preliminary scenarios were presented to and discussed with the TRUSTyFOOD consortium and further refined following this input. Concurrently, we began analysis of workshops from phase II and, later, from phase III, although our aim here is not to provide a comprehensive overview of the results of those workshops. The data from these phases was not used to fundamentally alter the scenarios, but rather to build on and deepen them as they currently existed. Following the completion of phase III workshops and their analysis, as well as further discussions within the consortium concerning updated versions of the scenarios, we produced the final scenarios that will be presented below.
This section will begin by presenting these three scenarios of blockchain-enabled, sustainable future food systems and, second, underlying tensions and contradictions. The three scenarios are: efficiency, transparency, and resiliency; compliance and accountability; and ecologically-integrated and place based, and each reflects different visions of BCT’s role in sustainable future food systems. Through a discussion of these scenarios, we will highlight environmental issues raised within the visioning sessions and their implications for how BCT may impact the environmental sustainability of food systems. Presenting the scenarios will then lead to a deeper exploration of tensions underlying these immediate environmental issues and the perspectives that were expressed in the workshops. We will then explicate four key underlying tensions that were identified in our analysis: decentralization and centralization; inclusion and exclusion; privacy and transparency; and tokenization as a pathway towards further commodification or commoning of nature and natural resources.
Scenarios of blockchain-enabled, sustainable future food systems
Three scenarios of the future were developed, beginning with our initial analysis of the visioning workshops (phase I) and subsequently following an iterative process involving discussions within the TRUSTyFOOD consortium and the incorporation of insights from phase II and III workshops. Following the design of the visioning sessions, these scenarios are loosely based around 2050 and focus primarily on EU food systems with some attention paid to global linkages. Many of the primary uses of BCT–such as for carbon trading or product traceability–feature in each of the scenarios, though in different contexts. Paying fealty to the data upon which these scenarios are based, the scenarios are not purely discrete; ambiguities and contradictions will be discussed further below. The three scenarios are summarized in Table 3.
Two substantial distinguishing features of the scenarios are the leading actors–that is, those actors at the forefront of transformational efforts–and the driving values that underlie the impetus towards transformation. In Scenario 1 – efficiency, transparency, and resiliency–the leading actors are primarily made up of large scale, often incumbent, agribusiness companies (e.gs., Syngenta, Bayer, John Deere), as well as (blockchain) technology companies. The driving values are centered around technological innovation driven by a general sense of techno-optimism, market-based efficiency, and transparency.
Given that large scale corporate actors drive food system transformation in this scenario, it involves substantial investments into BCT and other associated technologies, high levels of innovation, and a strong emphasis on market-based sustainability initiatives such as voluntary standards and the expansion of the voluntary carbon market (VCM) or other payments for ecosystem services (PES) markets. Much of the sustainability-oriented action is intended to satisfy market demand for sustainable and ethical products, such as providing data about sustainability to consumers.
Some substantial moves towards more sustainable food systems have been made in this scenario, but the concentration of power amongst a handful of leading actors also creates significant challenges. In practice, the decentralized nature of BCT systems is limited by, for example, leading actors prioritizing the privacy of their proprietary data over the ideal of transparency. Many small actors are excluded from new technological systems, further exacerbating existing trends of corporate consolidation within the agrifood system. While there is a shift towards more sustainable and ethical production, these are proximal goals in, ultimately, the pursuit of profit. As such, this scenario involves significant challenges related to greenwashing, tensions around the control and ownership of data, and unfettered financial speculation on environmental and other tokenized assets.
Scenario 2 – compliance and accountability–like scenario 1, involves a transformation driven by a small number of powerful actors. In this case, the leading actors national and supra-/international governments, supported to some extent by regional and municipal governments. This state-led transformation of the food system is underpinned by the values of regulatory compliance, transparency, and social and environmental sustainability.
Using BCT to ensure traceability and transparency of the food system is a similarity between scenarios 1 and 2. Scenario 2, in contrast to the above, involves fewer issues related to greenwashing and data manipulation due to strict guidelines imposed and enforced by governments. BCT is used, for example, to reduce bureaucratization and streamline enforcement of policies such as EUDR and CSDDD. As a result, general levels of trust in the veracity of data shared via BCT is higher in this scenario than scenario 1. In contrast, however, the strong version of transparency in this scenario has the potential to destabilize the current market structures, resulting in negative consequences for some actors. Beyond this, governments play a key role in terms of facilitating technology transfer, investing in education, training, and otherwise supporting small, resource poor, and/or digitally immature actors to adopt BCT.
In spite of investment in technology transfer, many actors are still excluded from these systems. In general, the centralized control of BCT systems in the hands of governments is the source of potential challenges in this scenario, such as by increasing the capacity for surveillance or creating possibilities for bureaucratic overreach.
Finally, Scenario 3 – ecologically integrated and place based–is driven by an array of medium- and small-scale farmers, SMEs, small-scale and place-based tech companies, NGOs, and regional/municipal governments. This scenario is closest, in some ways, to the original philosophies associated with BCT through, for example, its emphasis on the values of decentralization and local autonomy. Beyond this, social and environmental sustainability are driving values in this scenario.
This scenario’s basis in locally driven transformation comes with some advantages and disadvantages relative to the previous two scenarios. Many small actors and communities are empowered, for example through increased access to financing. Similarly, a wide range of niche innovations based on local need emerge, with high levels of flexibility and adaptability in the range of technologies that are employed. In contrast to the above scenarios, this translates into greater flexibility for and thus emphasis on ecologically integrated production systems such as agroecology.
However, the local orientation of this scenario also results in fragmentation, leading to unequal access to technology and technological knowledge across diverse communities. This scenario involves substantial coordination and governance issues which are exacerbated by a lack of interoperability amongst the numerous BCT systems used by different communities. Finally, while some local areas see a dramatic transformation in terms of sustainability and resilience, others do not: the fragmented nature of BCT’s use in this scenario results in limits to scalability and thus to impact on the food system overall.
Underlying tensions
The remainder of this section will explore in further detail core themes that emerged within the data and the deeper tensions that underlies them. A fully comprehensive discussion of the workshops and each issue that arose is beyond the scope of this paper. Therefore, in order to add coherence and focus to our discussion we will attend specifically to the issues related to BCT’s impact on the environmental sustainability of the food system that arose within the workshops. Exploring how BCT could be used to improve the environmental sustainability of the food system was an overarching goal of TRUSTyFOOD’s stakeholder engagement process in its entirety and connects to many of the other issues that were raised.
Centralization and decentralization
In popular discourses, the link between BCT and environmental issues is usually based on BCT’s energy use. The issue of BCT’s energy and resource intensity did emerge within the various workshops. In general, the critique expressed concerned the logic behind using an intensive technology like BCT in efforts towards sustainability. Participants expressing this general argument raised both usage of electricity and other raw materials.
Some participants noted that the energy intensity of BCT is in fact variable based on, among other factors, the consensus mechanism used to validate data. The image of BCT as an energy intensive technology is based on the fact that Bitcoin uses a proof-of-work (PoW) consensus mechanism, which is highly energy intensive. Other consensus mechanisms, however, such as proof-of-stake (PoS) are much less energy intensive than PoW. The initial insight offered here is that the negative environmental impacts of PoW-based blockchains should not be considered a given but rather can be subject to change towards less energy intensive systems. As one participant stated, “we have to make a separation on the kind of blockchain” based on differences in consensus mechanism and other characteristics (SH4, Phase I, workshop 3); beyond PoW and PoS, BCT applications in food systems use a wide range of other consensus mechanisms among other characteristics This participant clarifies that some blockchain systems are “public and permissionless meaning everyone has access.” On the other hand, there is a “spectrum of the other choices, which is all the way to private and permissioned blockchains”, which, they argue, are “especially useful for” situations in which “business or farmers or various stakeholders coming together and forming a consortium” (SH4, Phase I, workshop 3). Workshop participants indicated the complexity of this trade-off as they connected the issue of energy use to that of decentralization and, through it, to the issues of privacy and inclusion that we will be discussed below.
Beyond energy intensity, there are differences between species of blockchain in terms of their degree of centralization. Decentralization is often considered a main characteristic of blockchain technology. Workshop discussions pointed to the fact that decentralization is not a characteristic of BCT as such, but rather is something that exists to varying degrees in different contexts. For example, most PoS blockchains allocate power (e.g., for voting) based on the number of tokens (i.e., amount of money) different actors have. Thus, more money means more power, and that power can become centralized in situations with uneven distributions of resources. One implication of this is that public and permissionless blockchains should not be understood as decentralized by default but may also be vulnerable to forms of manipulation and the concentration of power.
The trade-off between degree of centralization and energy use in this example is, though simplified, a clear illustration of a core underlying tension within the workshop discussions and reflects broader trends in the development of BCT and its application to food system contexts. Some participants note that the majority of their experience is with private, permissioned blockchains, which are increasingly being used in food system contexts. This aligns with the trends for many actors within the food system to prioritize private and permissioned blockchains, which raises major concerns for some participants. One, for example, argues that when certain actors.
“want to go to … private blockchains, you’re back at the centralization issue because private [blockchain] options usually have some centralized part, and then again the question: why, if you have centralized parts, use a blockchain at all and … not just the central database?” (SH4, Phase I, workshop 1).
The tension between centralization and decentralization reflects, among other factors, differences between the leading actors in the three scenarios presented above. Both scenario 1 and 2 prioritize centralization in different forms. Scenario 1 reflects the trend towards permissioned and private blockchains noted above and thus prioritizes the introduction of centralized elements into BCT systems themselves. Scenario 2, in contrast, prioritizes centralization in terms of the (external) governance, management, and oversight of BCT systems. Scenario 3, finally, emphasizes decentralization both of BCT itself and the management thereof.
Inclusion and ensuring high quality data
While energy and resource intensity did emerge in the workshop discussions, it was not the most significant point related to environmental sustainability. More significant in these discussions was the notion that BCT could, in various ways, be a vehicle for financing the sustainable transformation of the food system. In most cases, these discussions referred to providing financial support for farmers as they attempt to adopt more environmentally sustainable production methods.
The connection between BCT, access to financial capital and services, and environmental sustainability was established in various ways. In the first instance, BCT was seen as a tool that could create access to financial services in general. The notion of “banking the unbanked” is often presented as one of the benefits of BCT, although it is as yet unclear the extent to which BCT results in tangible benefits via the promise of financial inclusion. However, from the perspective of some participants, adopting BCT would enable some actors to access loans, insurance, or other financial services that are currently inaccessible and could be used in efforts towards sustainable transformation. BCT was also seen as being a vehicle for financial capital as a side-effect of its use for transparency and traceability. Some participants argued that this would add legitimacy to claims about, for example, organic or fair-trade certifications, thus increasing access to price premia for these quality markers. Similarly, some participants anticipated BCT being used for direct selling, resulting in market disintermediation and more value being captured by primary producers. Some participants envisioned the use of BCT for crowdfunding or mutual aid as additional avenues to provide financial support.
Another major use of BCT discussed throughout the workshops that is related to financial access/support concerns the expansion of carbon and other ecosystem service markets. BCT was seen as a tool to vastly increase accessibility of these markets, eliminating barriers to entry and enabling the participation of many small actors. Each of these uses of BCT features to some extent and in some form in each of the three scenarios. The differences between how BCT is used to facilitate financial access in each scenario is based on an underlying tension between inclusion and exclusion and how this is expected to influence data quality.
Most, if not all, of the uses of BCT described above require the direct involvement of relatively small actors in BCT systems themselves. Some participants envisioned, for example, farmers being responsible for inputting information (regarding certifications, product origin, production methods, carbon sequestration, etc.) directly onto a blockchain themselves. In many cases, participants asserted that farmers should also own these data. This creates a tension when introducing a common BCT-related issue–GIGO, or the blockchain oracle problem (Caldarelli, 2020). Inputting data was not the only way in which participants imagined farmers could participate in BCT systems, also emphasizing their potential role in, for example, envisioning and designing BCT systems. In spite of this, a core concern amongst some participants is how to ensure that actors with little education and few resources correctly input data onto a blockchain, and this concern was closely connected by participants to the inclusion or exclusion of actors such as farmers.
For some participants, the answer to this question centered around education, training, and auditing systems. Cases where the necessity of providing such training was emphasized–often to the end of empowering farmers and other small-scale actors–were closely linked with scenarios 2 and 3. In other cases, participants explored strategies to eliminate human error through the elimination of (some) humans from these systems. In particular, one participant argued that much data will be “input from IoTs [internet of things sensors] and machines and not from individuals, because that will just verify that data is not tampered [with]” (SH4, Phase 1, workshop 2); this approach is more closely aligned with scenario 1.
In their extreme forms, the ideal of empowering farmers and ensuring that only high-quality data is input onto blockchains were considered as potentially at odds. As a result, other than being viewed as stakeholders with the right to participate in and contribute to the management of blockchain systems, less educated actors with fewer resources were seen as potential liabilities that may be excluded from these systems. This prospect raises the broader question of whether and to what extent implementing BCT might afford benefits to small actors such as smallholder farmers. As one participant argues,
“too often these kind of technical solutions are designed by people sitting in a city in an office that have never spent time on a farm, have never worked with farmers, have no idea of farmer realities, and then try to come up with a solution. And that just doesn’t work” (SH3, Phase I, workshop 1).
This misalignment–reflecting Guthman’s concept of solutionism in the agrifood system (2024) - is exacerbated by the impulse to exclude some actors for the sake of ensuring data quality.
Scenario 1 prioritizes technological innovation and, thus, the exclusion of smaller actors where necessary to ensure high quality data or otherwise streamline innovation processes. In contrast, governments in scenario 2 attempt to overcome the tension through substantial investments in education and technology transfer, although some actors are still excluded from this. Scenario 3, finally, emphasizes participation and inclusion, but at the cost of reduced trust in the veracity of claims made using BCT as many opportunities for fraud, manipulation, or negligence persist in the highly fragmented system.
Privacy and transparency
The discussions from these workshops and the tensions underlying them largely reflect Thompson and Rust’s aforementioned insight: “that blockchain will only spur transformative change if the most influential supply chain actors can see value in using it” (2023). The discussions of decentralization and participation above point to some ways in which such powerful actors can alter BCT and BCT systems so that it will be more valuable for them to use. These tensions culminate in a trade-off between transparency and privacy.
Transparency–and through it, traceability of food products and other things as they move along food supply chains–was considered a key benefit afforded by BCT throughout the workshops. However, it was recognized that transparency comes with trade-offs, namely that “transparency also means … less privacy” (SH4, Phase I, workshop 2) and that these trade-offs must be balanced to some extent. One way that some actors attempt to balance the apparent trade-off between the ideals of transparency and privacy is by employing blockchains with some degree of privacy or permissions, as noted with respect to decentralization above.
Many participants discussed the desirability of such closed blockchains because they would ensure that proprietary information is kept private. This would ensure that, for example, corporate actors are able to maintain competitive advantages based on information asymmetries. However, drifting too far towards the centralized, permissioned, and private side of the spectrum may compromise whatever transparency BCT may be able to afford: “without public blockchain, we have the same problem [as with standard, centralized databases]. I mean, if we have a closed blockchain, there is no transparency” (SH4, Phase I, workshop 2). This claim, however, is only relevant in cases when transparency for all, rather than transparency amongst a specified set of actors, is the desired outcome.
Expanding on this dilemma, two participants in phase III workshops provided additional insight into the challenges and implications of public vs private blockchains. One participant, a BCT expert, argues that public blockchains will provide the most benefits typically associated with BCT, such as immutability, accessibility, and decentralization. In contrast, they argue that private chains are “similar to … a private cloud; you can still eliminate and destroy or delete the transactions if you want, so as long as you have an entity that’s above that system” (Phase III, workshop 1, room 2). From this perspective, using a completely private blockchain precludes many of the benefits BCT may afford.
In another workshop from phase III, a different BCT expert similarly argues that public blockchains afford the most benefits, and that for this reason they will be the most common type of blockchain used in the future. However, echoing Thompson and Rust’s (2023) insight above, this participant notes that “[a] lot of these decisions are being made by foundations or by governance systems where you have large holders of … tokens who end up making all the decisions” (phase III, workshop 2, plenary). As noted above, these issues may exist in public as well as private BCT systems, albeit in different forms. This reality is in tension with the purported benefits of open, decentralized BCT in which all affected actors may participate equally. A critical challenge moving forward, from this perspective, is managing the “balancing act of how to create proper stakeholder engagement in a way that makes the inclusion and makes everyone feel his participation and voices their concerns and at the same time being able to move forward on implementing solutions” (Phase III, workshop 2, plenary).
How this “balancing act” is negotiated will have implications for the effects BCT may have and on whom. In scenario 1, the emphasis is placed on implementing solutions over stakeholder engagement and inclusion which results in more challenges related to greenwashing, data manipulation, and exclusion than the other scenarios. In contrast, scenario 2 places emphasis on inclusion and transparency driven by a centralized power. This creates fewer opportunities for greenwashing or data manipulation, but also more rigidity that may destabilize current market structures and results in significant costs for some actors. Finally, scenario 3 emphasizes the ideals of decentralization and inclusion but at the cost of a highly fragmented system, resulting in substantial challenges stemming from a lack of interoperability and broader coordination, among other issues.
Commodification or commoning: tokenization of natural resources
The tensions discussed so far – (de)centralization, in/exclusion, and the balance of transparency and privacy–primarily concern the impacts of different models of designing, organizing, and managing BCT systems and the data stored and shared on them. One specific aspect of sustainable food system transformation that each of these tensions relate to is the quality and veracity of environmental data, a major component of transparency and carbon related uses of BCT. The final tension concerns what can and should be done with these data once they are input into a BCT system.
One of the main kinds of data that was discussed throughout the workshops concerns environmental and social sustainability. Many kinds of environmental data were discussed–including data related to biodiversity, fertilizer and pesticide use, among others–but the main aspect that will serve as the basis for this discussion concerns carbon emissions and sequestration.
As noted above, participants discussed the possibility of using BCT to expand existing carbon markets, opening up access to them to a wider range of actors, and thus providing a source of financial support for the adoption of more sustainable agricultural practices. In general, such uses of BCT are predicated on the tokenization of data related to carbon, such as a certified carbon credit. One participant wonders “how can we properly tokeniz[e] agrifood assets?”, and explain that “the assets could be farmland, could be fertilization, [or] could be enabling ethical ways of farming and ensuring that the farmers will have fair pay” (SH5, Phase I, workshop 1). Many participants perceived tokenization of agrifood assets, such as environmental data, as a tool to “to allow people to invest in [agrifood assets], because once we invest in it, we can create additional liquidity to fight climate change” (SH5, Phase I, workshop 1) by, for example, enabling farmers to adopt sustainable practices that otherwise may be too costly. In general, tokenization in this form can be considered as an attempt to use BCT to streamline market-based efforts to internalize environmental externalities.
However, participants expressed different views on the implications of internalization via tokenization. Two participants (SH3 and SH4) in the third visioning workshop grappled with the potential negative side effects of further “commodifying” nature through this process of tokenization. Ultimately, one perceived such commodification as categorically negative, expressing the concern that BCT will “reinforce this … capitalist relationship with land that we’re trying to free ourselves from” (SH3, Phase I, workshop 3). In contrast, the other participant in this exchange saw the issues with focusing on monetary value but saw no other way to “compensate [farmers] in a fair way” (SH4, phase I, workshop 1) for the environmental benefits they provide.
Beyond this disagreement, other participants rejected the idea that tokenization would inevitably lead to the commodification of these environmental data, instead arguing that this could be a route towards instantiating some pieces of nature (such as carbon) as commons. In these visions, carbon (and other environmental data) is still tokenized but in a context in which BCT is used to fairly distribute shared ownership of the token and its underlying data. This reflects some niche trends within Web3 and ReFi, such as commonsstack, that attempt to use BCT to instantiate commons-based principles. However, it is unclear the extent to which this is effective, and further research should scrutinize the potential for BCT and tokenization to be used for commoning.
In all cases, participants imagined that environmental data would but input on to and therefore digitally represented on blockchains; contradictions emerged in discussions of what would happen after to these tokens after they come into being. The concerns about the commodification of nature manifest most clearly in scenario 1, while scenario 3 is closest to the vision of tokens representing common resources. Scenario 2 straddles these extremes, treating commodification qua tokenization as a lever towards the provision of fairer remuneration and the creation of incentives for sustainability.
Datafication
Storing and sharing data underpins all uses of BCT discussed within the workshops. One participant, from an agrifood sector company (SH2), shared their experience of being involved in information-technology based projects in the agricultural sector. They make note of, first, the volume of money invested to finance such projects, and second, the amount of data that it produces. The participants acknowledge that “we need data on, for instance, [the] state of a forest, forest threats like loss of biodiversity, for fires, and so on”, but wonder what can actually be done with these fine-grain data concerning wide ranging issues. For them, the critical question is “how to commercialize that data?” (SH2, Phase I, workshop 3). Different answers to this question and others like it–how to make use of that data? - are the crux of the differing perspectives expressed by workshop participants.
The question raised by this participant about how to commercialize data is not unique to the case of BCT but applies to “ICT and data-based business” (SH2, Phase I, workshop 3) as it applies to the food system in general. From this perspective, the success of BCT is dependent on the ability to “extract” (Calvão and Archer, 2021) value from data. Following this logic, BCT was seen by many participants as, in an ideal situation, an “enabling tool” (SH2, Phase I, workshop 3) that optimizes rather than disrupts business as usual. The centrality of storing, sharing, and commercializing data within the workshops discussions indicates that efforts to apply BCT to the food system are connected to broader trends towards datafication in the food system and beyond.
Dourish and Gómez Cruz, 2018 for example, build on Mayer-Schoenberger and Cukier’s (2013) definition of datafication as “the transformation of social action into online quantified data, thus allowing for real-time tracking and predictive analysis” by also emphasizing the “symbolic and imaginative work that underlie coming to think of something as ‘data’ in the first place” (2018). This concept has been applied to food systems by, among others, Bronson with her concept of “the immaculate conception of data”, a sociotechnical imaginary she argues underpins digital transformation of agriculture (2022). Bronson, 2022 argues that, in this imaginary, data are conceived as objective and direct representations of a reality that is already out there; they purely represent a reality that exists a priori to their collection or generation (ibid). Montenegro de Wit and Canfield, 2023 have taken this idea further, arguing that we are entering a new food system regime of “data productivism,” in which the traditional productivist impulses–industrial scale production aiming to maximize yields and profits - persist, augmented by the use of data (2023).
The issue of datafication is an overarching theme within the workshops, especially regarding data as the primary driver of environmental decision making and transformations towards environmental sustainability. In the context of increasing transparency, for example, the function of BCT was considered to be providing in-demand information to consumers and, building on the association with BCT and immutability, increasing the veracity of that information. In this light, BCT is closely linked to other technologies, such as IoT sensors and AI, that are part of the fourth agricultural revolution (Klerkx and Rose, 2020). Blockchain serves the function of being a space in which data is stored and shared and is therefore dependent on the function of other technologies for collecting and processing data. Increasingly, data are considered the primary tool for understanding and managing the food system, and thus the key lever for guiding sustainable transformation (e.g., Fanzo et al., 2021). The tensions discussed above are all connected to the implications of the datafication of sustainable food system transformation, and they point to critical questions such as whose data, for what purpose, and for whose benefit?
While datafication is a broad trend within each of the scenarios presented above, they can be distinguished in this context along the lines of “data valences”, which reflect “people’s expectations of and values for data that emerge from their discourses and practices across different contexts” (Fiore-Gartland and Neff, 2015). In our case, the different data valences in play within the workshops express different expectations and values regarding, for example, how BCT will alter the ownership of certain kinds of data, regarding what data should or should not be publicly accessible, or regarding what data should or should not have a monetary value assigned to it.
Some of the specific data valences identified by Fiore-Gartland and Neff (2015) are identifiable within the tensions that we have presented. The data valence of transparency plays a major role in the tensions we have presented. Fiore-Gartland and Neff describe how “people evoke the data valence of transparency when they talk about the benefits of making data accessible, open, shareable, or comparable across cases or contexts […] the transparency valence privileges the power of transparent data for individual and social - change” (ibid).
Ideas about the benefits of BCT-based transparency abound within the workshops we have analyzed. However, a number of counterpoints to this data valence were also presented. Multiple challenges stemming from the tension between transparency and privacy–and the related tensions of centralization and decentralization, and inclusion and exclusion–were raised within the workshop discussions. As such, the data valence of transparency that was explored by workshop participants was inclusive of the “benefits of making data accessible” (Fiore-Gartland and Neff, 2015; emphasis added) as well as the potential costs, side-effects, and trade-offs.
Annany and Crawford (2018) critically interrogate the notion of transparency, conceptualizing it primarily as an ideal and then exploring ten limitations to this ideal. Combining the insights from Fiore-Gartland and Neff (2015) on the positive aspects of the transparency ideal with those from Annany and Crawford (2018) on its limitations may be a useful approach to better understanding the complexities of efforts to foster BCT-based transparency and how the data valence of transparency manifests in discussions surrounding BCT. The tensions around this issue that emerged in our data indicate, at least, that the data valence of transparency should be understood in light of its internal contradiction. Our data indicate numerous challenges with realizing the ideal of transparency in practice. For example, discussions in our workshops about how different actors are influencing the design of BCT systems based on how they balance the values of transparency and privacy illustrates how the (dynamic) materiality of BCT influences the practical potential BCT-based transparency, and thus discourses about it. Therefore, discursive tensions surrounding the data valence of transparency will result in real-world trade-offs (i.e., between transparency and privacy) in attempts to realize the ideal of transparency in practice.
Another data valence that was identifiable within our data was that of actionability, and how this emerged supports the idea of data valences containing internal contradictions and ambiguities. Fiore-Gartland and Neff describe the data valence of actionability as “[referring] to the expectation that data drive or do something within a social setting or that data can be leveraged for action” (2015). This data valence is particularly relevant in the context of BCT-based representations of (the value of) nature and the potential for BCT to contribute to improving environmental sustainability.
Discussions in the workshops clearly pointed to the fact that, through tokenization, various natural assets will have digital representations in BCT-based environments. The tension emerges when considering what will be done with these digital representations and the implications this will have for the nature that they represent. Some perspectives, as noted, view tokenization as a pathway towards more convivial relationships with nature, in which natural assets are formalized as commons, involving shared ownership and management. Alternatively, other perspectives within our workshops expressed concerns that tokenization will lead to further commodification of nature and thus expanded opportunities for its exploitation. In either case, the burgeoning volumes of environmental data expected to be involved in BCT-systems will “[lead] to knowledge, which leads to change” (Fiore-Gartland and Neff, 2015) with the caveat that this change may be for better and/or worse.
Blockchain’s role in sustainable food system transformation
Many of the tensions highlighted above are rooted in competing, often contradictory data valences, that is, competing narratives about how data can and should be used and how it may impact different actors and issues within food systems. The workshops we have analyzed point to critical questions and tensions regarding who will have access to data input in BCT systems, who will have the ability to input these data, and what the implications of this will be. Further, the (original) nature of BCT and the social dynamics surrounding its development point to questions about the valuation and thus ownership of data.
These insights suggest that BCT and its potential impacts must be considered in light of their social embeddedness. A wide range of actors have a stake in the future of the food system and will therefore be involved in and affected by efforts to make use of BCT. Our results have indicated that the contestation around the development of BCT will influence how the technology functions and thus how it impacts different actors. For example, participants raised the distinction between different kinds of blockchains (public vs private chains, or chains with different consensus mechanisms) as a critical difference that is influenced by contestation amongst actors. The “politics” (Winner and Wunsche, 1980) of blockchain are in flux.
Beyond this, our analysis indicates that the trend to implement BCT based solutions in food system contexts is rooted in broader trends about datafication and contestation over how this process will unfold. BCT plays a function, above all, of a backend layer supporting the storage and sharing of data in the larger process of datafication. In this context, data becomes the driving force for understanding/generating knowledge about the food system–including, critically, the potential to provide consumers more detailed information about the social and environmental sustainability of food products they consume–as well as a driving force of sustainable transformation.
These larger trends–datafication and sustainable transformation–each extend beyond BCT’s specific role. Taking BCT as a case of a specific technology provides, however, insights into the challenges of understanding and transforming increasingly complex and interconnected food systems.
Workshop participants did offer some optimism regarding BCT’s potential, and outlined a number of ways in which it could contribute positively to sustainable transformation of the food system. These include, but are not limited to: increasing the transparency of the food system and traceability of specific products, with benefits for both consumers interested in making more sustainable and ethical choices as well as policymakers interested in understanding and regulating the food system; providing access to financial services for a range of actors; acting as a platform for the secure storage and sharing of high resolution data about a range of aspects of the food system; creating opportunities, through the tokenization of carbon and other ecosystem services, for novel mechanisms of financing the adoption of sustainable agricultural practices; among various others. From the perspective of many workshop participants, BCT has the potential to address a range of real challenges within the food system.
However, discussions within the workshops also raised criticism and concerns regarding these potential benefits. While data on BCT is generally considered to beimmutable, this should not be considered an absolute truth and, further, does not eliminate external problems such as GIGO. The real transparency, accessibility, and decentralization of BCT–associated with many potential benefits–may be limited in practice through technological change. Taken as a whole, the workshop discussions contain a pervasive ambivalence regarding BCT’s actual potential, expressing both optimism and concern. Making arguments about the potential of BCT in each of these specific cases is beyond the scope of this paper, as this does not reflect the nature of the data we collected. Rather, our data provides a snapshot of competing perspectives and expectations surrounding BCT. This, as we have illustrated, raises critical issues that deserve scrutiny and that cannot be derived from expert opinions or conceptual research alone (Frizzo-Barker et al., 2020).
How contestation over the tensions we have described unfolds will influence the impacts BCT has on the food system in general as well as on various actors in particular. As BCT is applied in real-world settings, these underlying tensions will take manifest as trade-offs, and the negotiation of these tensions will determine the balance of trade-offs. Differences in the design of BCT systems can have complex implications for environmental issues and for different actors. Following this, it is critical to scrutinize which kinds of blockchains are used in which contexts, and which will become dominant within the food system overall. Similarly, differences in the external organization of BCT systems–who designs, manages, educates about, and implements these systems–can have similarly complex implications. It is therefore crucial to explore how BCT systems will be regulated and overseen, and by what entities or institutions.
Considering the connection between BCT as a specific technology (or rather, a specific set of technologies, as our results clearly indicate that there is no blockchain as such but rather a diverse assortment of related blockchains) in light the broader trend of datafication provides insights into both BCT and the process of datafication. There are unique features of BCT and the discourses surrounding it that distinguish it from more general discussions of datafication. One such aspect is the significance of the transparency ideal, deriving from the original “blockchain philosophy” and that emphasizes immutability, decentralization, democratization, and open accessibility (Phase II, workshop 4). Similarly, the financial embedding of much BCT has created the possibility to use it to digitally represent (the value of) material and immaterial objects through tokenization. This has important implications for how natural resources (such as carbon tokens) are represented, valued, and made exchangeable. Each of these issues, among others, requires further research and scrutiny.
Conclusion
We have drawn on the discussions in 11 workshops to explore the perspectives of food system actors on BCT’s potential to contribute to sustainable food system transformation, paying particular attention to aspects related to environmental sustainability. In terms of environmental sustainability, issues related to energy and resource did emerge in these discussions, but were secondary compared to other connections to environmental issues, such as the potential for BCT to facilitate the financing of sustainable transition and provide a digital space for storage and sharing of environmental data.
Overall, our results indicate a diverse, often contradictory array of perspectives regarding BCT’s potential. While explicit disagreements or arguments were rare within the workshops, subsequent analysis illuminated underlying contradictions and tensions that pervaded these discussions. Understanding these perspectives and the tensions that underlie them is a critical component of research on BCT (e.g., following Frizzo-Barker et al., 2020) as a wide range of actors with differing interests will be involved in and affected by using BCT.
We have presented three scenarios of blockchain enabled future food systems, developed by the research team from insights derived from the first phase of workshops. Following this, we explored four core tensions that we identified as underlying differences in these scenarios and workshop participants’ perspectives: decentralization vs centralization, inclusion vs exclusion, transparency vs privacy, and the representation of environmental assets as a pathway towards commoning vs commodification. These identified tensions were then connected to the broader process of datafication and the concept of data valences.
In terms of environmental sustainability, the issue of energy use emerged within the workshops but other issues–such as the balance between including actors in BCT systems or excluding them on grounds of ensuring high quality data; or the potential for BCT to facilitate financing a transition to sustainable production systems–are more significant from our participants’ perspectives. Following this, we consider BCT as embedded in a larger process of datafication, in which data takes an increasingly significant role as a means for understanding and affecting change within the food system. As such, the critical environmental sustainability issues regard how BCT is leveraged in the datafication of environmental management and sustainable transformation, for example in the context of environmental monitoring or the tokenization of natural assets.
Further research on some of the underlying tensions we have identified would be valuable for understanding ongoing attempts to apply BCT to food system contexts. The tensions that we have identified in discourse about BCT will manifest as real-world trade-offs as BCT is applied in practice. In particular, how the balance between transparency and privacy in BCT-based traceability systems unfolds, as well as the implications of tokenization natural assets such as carbon or other ecosystem services, warrant further scrutiny. Additionally, while our research has answered Frizzo-Barker et al.’s (2020) concerns about the lack of real-world perspectives in research on BCT and food systems, we have been able to provide only a snapshot. For example, investigations of alternatives to BCT (e.g., for traceability, PES based financing) would be valuable for avoiding solutionist approaches to using BCT. Further, deper investigations of diverse stakeholder perspectives involved in specific BCT projects, use-cases, or across geographic regions would provide greater insight into how BCT can best be leveraged to contribute to sustainable food system transformation.
Data availability statement
The raw data supporting the conclusions of this article will be made available by the authors, without undue reservation.
Ethics statement
Ethical review and approval was not required for the study on human participants in accordance with the local legislation and institutional requirements. The studies were conducted in accordance with the local legislation and institutional requirements. Written informed consent from the workshop participants was not mandatory in accordance with the national legislation and the institutional requirements; verbal informed consent was given at the beginning of each workshop and participants were informed about the study aim.
Author contributions
LK: Conceptualization, Formal Analysis, Writing – original draft, Writing – review and editing. TN: Conceptualization, Formal Analysis, Writing – original draft, Writing – review and editing. SL: Formal Analysis, Writing – review and editing. TS: Conceptualization, Methodology, Writing – original draft, Writing – review and editing. Lv: Conceptualization, Writing – review and editing. AL: Conceptualization, Writing – review and editing.
Funding
The author(s) declare that financial support was received for the research and/or publication of this article. This work has received funding from the European Union’s Horizon 2020 research and innovation programme under the Grant agreement No. 101060534.
Acknowledgments
We extend gratitude to members of the TRUSTyFOOD consortium for their support with recruitment, organization, and facilitation of the workshops analyzed above.
Conflict of interest
The authors declare that the research was conducted in the absence of any commercial or financial relationships that could be construed as a potential conflict of interest.
Generative AI statement
The author(s) declare that no Generative AI was used in the creation of this manuscript.
Publisher’s note
All claims expressed in this article are solely those of the authors and do not necessarily represent those of their affiliated organizations, or those of the publisher, the editors and the reviewers. Any product that may be evaluated in this article, or claim that may be made by its manufacturer, is not guaranteed or endorsed by the publisher.
Footnotes
1Further details about the TRUSTyFOOD consortium can be found at https://cordis.europa.eu/project/id/101060534/results
References
Ananny, M., and Crawford, K. (2018). Seeing without knowing: limitations of the transparency ideal and its application to algorithmic accountability. New Media and Soc. 20 (3), 973–989. doi:10.1177/1461444816676645
Athena Institute, VU University (2018). Visioning. Available online at: https://knowledgehub.fit4food2030.eu/wpcontent/uploads/2020/06/FIT4FOOD2030_Tool_training_module_visioning-1.pdf.
Barcevičius, E., Bobrovnikova, E., Chen, M., Danielle, L., van Deventer, O., Gunkel, S., et al. (2025). Background document: input for the global multistakeholder high level conference on governance for web 4.0 and virtual worlds. Available online at: https://ec.europa.eu/newsroom/dae/redirection/document/113701.
Beck, J. (2022). What is Web3? Here are some ways to explain it to A friend. Available online at: https://consensys.io/blog/what-is-web3-here-are-some-ways-to-explain-it-to-a-friend.
Bernards, N., Campbell-Verduyn, M., and Rodima-Taylor, D. (2024). The veil of transparency: blockchain and sustainability governance in global supply chains. Environ. Plan. C Polit. Space 42 (5), 742–760. doi:10.1177/23996544221142763
Bronson, K. (2022). The immaculate conception of data: agribusiness, activists, and their shared politics of the future. Montreal: McGill-Queen's University Press.
Brown, V. A., Harris, J. A., and Russell, J. Y. (2010). Tackling wicked problems through the transdisciplinary imagination. London: Earthscan.
Caldarelli, G. (2020). Understanding the blockchain oracle problem: a call for action. Information 11 (11), 509. doi:10.3390/info11110509
Calvão, F., and Archer, M. (2021). Digital extraction: blockchain traceability in mineral supply chains. Polit. Geogr. 87, 102381. doi:10.1016/j.polgeo.2021.102381
Condon, M., and Thackray, L. (2021). Rupert murdoch’s NSW wool property cavan station signs $500,000 carbon deal with microsoft. Australia: ABC News. Available online at: https://www.abc.net.au/news/2021-04-28/rupert-murdoch-microsoft-carbon-credit-farm-deal/100099058 (Accessed April 28, 2021).
Curry, D. (2025). Limitations of trust and legitimacy in blockchain: exploring the effectiveness of decentralisation, immutability and consensus mechanisms in blockchain governance. Int. J. Public Sect. Manag. 38 (1), 98–117. doi:10.1108/IJPSM-12-2023-0368
Dameron, M. (2018). Beigepaper: an Ethereum technical specification. Available online at: https://cryptopapers.info/assets/pdf/eth_beige.pdf.
Davies, A. R. (2014). Co-creating sustainable eating futures: technology, ICT and citizen–consumer ambivalence. Futures 62 (Part B), 181–193. doi:10.1016/j.futures.2014.04.006
Dourish, P., and Gómez Cruz, E. (2018). Datafication and data fiction: narrating data and narrating with data. Big Data and Soc. 5 (2). doi:10.1177/2053951718784083
Düdder, B., Fomin, V., Gürpinar, T., Henke, M., Iqbal, M., Janavičienė, V., et al. (2021). Interdisciplinary blockchain education: utilizing blockchain technology from various perspectives. Front. Blockchain, 3. doi:10.3389/fbloc.2020.578022
European Commission (2023). Communication from the commission to the European parliament, the council, the European economic and social committee and the committee of the regions: an eu initiative on web 4.0 and virtual worlds: a head start in the next technological transition. Strasbourg. Available online at: https://eur-lex.europa.eu/legal-content/EN/ALL/?uri=COM:2023:442:FIN.
Fanzo, J., Haddad, L., Schneider, K. R., Béné, C., Covic, N. M., Guarin, A., et al. (2021). Viewpoint: rigorous monitoring is necessary to guide food system transformation in the countdown to the 2030 global goals. Food Policy 104, 102163. doi:10.1016/j.foodpol.2021.102163
Feng, H., Wang, X., Duan, Y., Zhang, J., and Zhang, X. (2020). Applying blockchain technology to improve agri-food traceability: a review of development methods, benefits and challenges. J. Clean. Prod. 260, 121031. doi:10.1016/j.jclepro.2020.121031
Filippi, P. de M. M., and Reijers, W. (2020). Blockchain as a confidence machine: the problem of trust & challenges of governance. Technol. Soc. 62, 101284. doi:10.1016/j.techsoc.2020.101284
Fiore-Gartland, B., and Neff, G. (2015). Communication, mediation, and the expectations of data: data valences across health and wellness communities. Int. J. Commun. 9, 1466–1484.
Frizzo-Barker, J., Chow-White, P. A., Adams, P. R., Mentanko, J., Green, D., and Green, S. (2020). Blockchain as a disruptive technology for business: a systematic review. Int. J. Inf. Manag. 51, 102029. doi:10.1016/j.ijinfomgt.2019.10.014
Guthman, J. (2024). The problem with solutions: why Silicon Valley can’t hack the future of food. Oakland: University of California Press.
Hartley, S., and Rennie, E. (2023). Regenerative finance (ReFi): blockchain for climate action. APO. Available online at: https://apo.org.au/node/321467?__cf_chl_tk=.PiZfWTDiiRYWN5n3TQplQJ00YSh3ketYbALBb6TrEA-1737115980-1.0.1.1-2vb755_RnKXYZU1lOjgS82VcD4UAVnEs0sl.e_P9vGk.
Heitlinger, S., Houston, L., Taylor, A., and Catlow, R. (2021). “Algorithmic food justice: Co-designing more-than-human blockchain futures for the food commons,” in Proceedings of the 2021 CHI conference on human factors in computing systems (Yokohama, Japan: Association for Computing Machinery), 1–14. Available online at: https://chi2021.acm.org/.
Hsu, A., and Schletz, M. (2023). Digital technologies – the missing link between climate action transparency and accountability? Clim. Policy 24 (2), 193–210. doi:10.1080/14693062.2023.2237937
Hull, J., Gupta, A., and Kloppenburg, S. (2021). Interrogating the promises and perils of climate cryptogovernance: blockchain discourses in international climate politics. Earth Syst. Gov. 9, 100117. doi:10.1016/j.esg.2021.100117
Ito, K., and O’Dair, M. (2019). “A critical examination of the application of blockchain technology to intellectual property management,” in Business Transformation through blockchain, Editor H. Treiblmaier, and R. Beck Cham: Palgrave Macmillan. doi:10.1007/978-3-319-99058-3_12
Jiren, T. S., Abson, D. J., Schultner, J., Riechers, M., and Fischer, J. (2023). Bridging scenario planning and backcasting: a Q-analysis of divergent stakeholder priorities for future landscapes. People Nat. 5, 572–590. doi:10.1002/pan3.10441
Kechagias, E. P., Gayialis, S. P., Papadopoulos, G. A., and Papoutsis, G. (2023). An ethereum-based distributed application for enhancing food supply chain traceability. Foods 12 (6), 1220. doi:10.3390/foods12061220
Kharif, O. (2018). Walmart tackles food security with trial of blockchain. New York City, United States: Bloomberg. Available online at: https://www.bloomberg.com/news/articles/2016-11-18/wal-mart-tackles-food-safety-with-test-of-blockchain-technology?in_source=embedded-checkout-banner.
Klerkx, L., and Rose, D. (2020). Dealing with the game-changing technologies of agriculture 4.0: how do we manage diversity and responsibility in food system transition pathways? Glob. Food Secur. 24, 100347. doi:10.1016/j.gfs.2019.100347
Kohler, S., and Pizzol, M. (2020). Technology assessment of blockchain-based technologies in the food supply chain. J. Clean. Prod. 269, 122193. doi:10.1016/j.jclepro.2020.122193
Kononets, Y., and Treiblmaier, H. (2021). The potential of bio certification to strengthen the market position of food producers. Mod. Supply Chain Res. Appl. 3 (1), 41–55. doi:10.1108/MSCRA-05-2020-0013
Ledger (2023). Public blockchain meaning. Available online at: https://www.ledger.com/academy/glossary/public-blockchain.
Mayer-Schoenberger, V., and Cukier, K. (2013). Big data. A revolution that will transform how we live, work, and think. London: John Murray.
McKinsey (2023). What is Web3? Available online at: https://www.mckinsey.com/featured-insights/mckinsey-explainers/what-is-web3.
Mohammed, A., Potdar, V., Quaddus, M., and Hui, W. (2023). Blockchain adoption in food supply chains: a systematic literature review on enablers, benefits, and barriers. IEEE Access 11, 14236–14255. doi:10.1109/ACCESS.2023.3236666
Montenegro de Wit, M., and Canfield, M. (2023). Feeding the world, byte by byte’: emergent imaginaries of data productivism. J. Peasant Stud. 51 (2), 381–420. doi:10.1080/03066150.2023.2232997
Morozov, E. (2013). To save everything, click here: the folly of technological solutionism. New York: PublicAffairs. Available online at: http://archive.org/details/tosaveeverything0000moro.
Motta, G. A., Tekinerdogan, B., and Athanasiadis, I. N. (2020). Blockchain applications in the agri-food domain: the first wave. Front. Blockchain 3, 00006. doi:10.3389/fbloc.2020.00006
Nakamoto, S. (2008). Bitcoin: a peer-to-peer electronic cash system. SSRN Electron. J. doi:10.2139/ssrn.3440802
Ordoñez, C. C., Gonzales, G. R., and Corrales, J. C. (2024). Blockchain and agricultural sustainability in SouthSouth America: a systematic review. Front. Sustain. Food Syst. 8, 1347116. doi:10.3389/fsufs.2024.1347116
Pakseresht, A., Yavari, A., Kaliji, S. A., and Hakelius, K. (2023). The intersection of blockchain technology and circular Economy in the agri-food sector. Sustain. Prod. Consum. 35, 260–274. doi:10.1016/j.spc.2022.11.002
Parry, G., and Collomosse, J. (2021). Perspectives on “good” in blockchain for good. Front. Blockchain 3. doi:10.3389/fbloc.2020.609136
Rejeb, A., Keogh, J. G., Zailani, S., Treiblmaier, H., and Rejeb, K. (2020). Blockchain technology in the food industry: a review of potentials, challenges and future research directions. Logistics 4 (4), 27. doi:10.3390/logistics4040027
Ronaghi, M. H. (2021). A blockchain maturity model in agricultural supply chain. Inf. Process. Agric. 8 (3), 398–408. doi:10.1016/j.inpa.2020.10.004
Samaties, P., Steger, S., Maus, S., and Biesaga, A. (2023). Tokenization of real-world assets: unlocking a new era of ownership, trading, and investment. Available online at: https://www.rolandberger.com/en/Insights/Publications/Tokenization-of-real-world-assets-unlocking-a-new-era-of-ownership-trading.html.
Schletz, M., Constant, A., Hsu, A., Schillebeeckx, S., Beck, R., and Wainstein, M. (2023). Blockchain and regenerative finance: charting a path toward regeneration. Front. Blockchain 6, 1165133. doi:10.3389/fbloc.2023.1165133
Sendros, A., Drosatos, G., Efraimidis, P. S., and Tsirliganis, N. C. (2022). Blockchain applications in agriculture: a scoping review. Appl. Sci. 12 (16), 8061. doi:10.3390/app12168061
Sharma, R., Samad, T. A., Chiappetta Jabbour, C. J., and de Queiroz, M. J. (2021). Leveraging blockchain technology for circularity in agricultural supply chains: evidence from a fast-growing Economy. J. Enterp. Inf. Manag. 38,32–67. doi:10.1108/JEIM-02-2021-0094
Sharpe, B., Hodgson, A., Leicester, G., Lyon, A., and Fazey, I. (2016). Three horizons: a pathways practice for transformation. Ecol. Soc. 21 (2), art47. doi:10.5751/es-08388-210247Available online at: http://www.jstor.org/stable/26270405.
Singh, C., Wojewska, A., Martin Persson, U., and Bager, S. (2022). Coffee producers’ perspectives of blockchain technology in the context of sustainable global value chains. Front. Blockchain 5. doi:10.3389/fbloc.2022.955463
Thompson, B. S., and Rust, S. (2023). Blocking blockchain: examining the social, cultural, and institutional factors causing innovation resistance to digital technology in seafood supply chains. Technol. Soc. 73, 102235. doi:10.1016/j.techsoc.2023.102235
Tripathi, G., Abdul Ahad, M., and Casalino, G. (2023). A comprehensive review of blockchain technology: underlying principles and historical background with future challenges. Decis. Anal. J. 9, 100344. doi:10.1016/j.dajour.2023.100344
TRUSTyFOOD (2023). Homepage. Available online at: https://www.trustyfood.eu/.
UNDP (2021). Blockchain for agri-food traceability: exploring the technology’s potential for sustainable development. Available online at: https://www.undp.org/publications/blockchain-agri-food-traceability.
van Hilten, M., Ongena, G., and Ravesteijn, P. (2020). Blockchain for organic food traceability: case studies on drivers and challenges. Front. Blockchain 3 Article 3, 567175. doi:10.3389/fbloc.2020.567175
van Wassenaer, L., van Hilten, M., van Asseldonk, M., and van Ingen, E. (2021a). Applying blockchain for climate action in agriculture: state of play and outlook - world. Available online at: https://reliefweb.int/report/world/applying-blockchain-climate-action-agriculture-state-play-and-outlook.
van Wassenaer, L., Verdouw, C., and Wolfert, S. (2021b). What blockchain are we talking about? An analytical framework for understanding blockchain applications in agriculture and food. Front. Blockchain 4 Article 4, 653128. doi:10.3389/fbloc.2021.653128
Vergragt, P., and Quist, J. (2011). Backcasting for sustainability: introduction to the special issue. Technol. Forecast. & Soc. Change 78, 747–755. doi:10.1016/j.techfore.2011.03.010
Wang, J., Wang, S., Guo, J., Du, Y., Cheng, S., and Li, X. (2019). A summary of research on blockchain in the field of intellectual property. Procedia Comput. Sci. 147, 191–197. doi:10.1016/j.procs.2019.01.220
WEF (2020). WEF blockchain toolkit: structure: public/private. Available online at: https://widgets.weforum.org/blockchain-toolkit/structure-public-private/index.html.
WEF (2023). Blockchain for scaling climate action. Geneva, Switzerland: White Paper. Available online at: https://www3.weforum.org/docs/WEF_Blockchain_for_Scaling_Climate_Action_2023.pdf.
Wesbuer, A., Ceglar, K., Palkova, Z., Casey, O., Kelly, K., Šimek, P., et al. (2023). Blockchain teaching in higher education in the agri-food sector. Available online at: https://www.hb.fh-muenster.de/opus4/frontdoor/deliver/index/docId/18168/file/Baseline_Research_Report_en.pdf.
Winner, L. (1980). Do artifacts have politics? Daedalus 109 (1), 121–136. Available online at: https://www.jstor.org/stable/20024652.
Wünsche, J. F., and Fernqvist, F. (2022). The potential of blockchain technology in the transition towards sustainable food systems. Sustainability 14 (13), 7739. doi:10.3390/su14137739
Xiong, H., Dalhaus, T., Wang, P., and Huang, J. (2020). Blockchain technology for agriculture: applications and rationale. Front. Blockchain 3. 00007. doi:10.3389/fbloc.2020.00007
Keywords: blockchain, web4, web3, sustainable food systems, agriculture 4.0, agri-food, sustainability, datafication
Citation: Kingfisher L, Nguyen Thi TT, Lehtonen S, Saidi T, van de Burgwal L and Loeber A (2025) Tensions in stakeholder perspectives on blockchain technology in sustainable food system transformation. Front. Blockchain 8:1569106. doi: 10.3389/fbloc.2025.1569106
Received: 31 January 2025; Accepted: 28 April 2025;
Published: 12 May 2025.
Edited by:
Tan Gürpinar, Quinnipiac University, United StatesReviewed by:
Larry C. Bates, Independent Researcher, Detroit, United StatesAlex Butean, Lucian Blaga University of Sibiu, Romania
Copyright © 2025 Kingfisher, Nguyen Thi, Lehtonen, Saidi, van de Burgwal and Loeber. This is an open-access article distributed under the terms of the Creative Commons Attribution License (CC BY). The use, distribution or reproduction in other forums is permitted, provided the original author(s) and the copyright owner(s) are credited and that the original publication in this journal is cited, in accordance with accepted academic practice. No use, distribution or reproduction is permitted which does not comply with these terms.
*Correspondence: Levi Kingfisher, bC5tLnMua2luZ2Zpc2hlckB2dS5ubA==