- 1 Department of Civil Engineering, University of Kentucky, Lexington, KY, United States
- 2 ProMission FZ LLC, Dubai, United Arab Emirates
- 3 Department of Civil Engineering, TU Wien, Vienna, Austria
As the notion of data-driven analytics and turning data into action is becoming more salient in the construction industry, researchers and practitioners have recently devoted considerable effort to investigate the digital transformation of the industry. Along this journey, Digital Twin has been introduced to the industry as a concept that holds the promise to challenge the status-quo and address long standing problems of productivity, efficiency, and value. While this concept is becoming more familiar among practitioners, there is a lack of universal definitions of what the Digital Twin of a construction project is. Additionally, while identifying the purpose of Digital Twin is recognized as the first step in implementing Digital Twins, there is little discussion on the perception of construction practitioners of the extent to which Digital Twin can deliver value. To address these research gaps and building on the existing work on Digital Twins in the context of the construction industry, this paper first proposes a definition of the Digital Twin of a construction project. Next, a series of semi-structured interviews are conducted with nine construction practitioners to understand their perceptions on the use and challenges of Digital Twins. Thematic analysis is then used to analyze interview data and summarize Digital Twins applications, capabilities, and challenges. Forty direct applications were identified and grouped into seven capabilities. Digital Twins capabilities of Increase Transparency of Information and Real-Time Monitoring, Analysis, and Feedback were the most discussed with a total of eight applications each, followed by Better Stakeholder Collaboration which had seven applications. The discussion on challenges led to the identification of 34 challenges to implementing Digital Twin, grouped into six categories coded through thematic analysis. The category on Data Understanding, Preparation, and Usage Challenges was found to be the most critical for the interviewees. Additionally, the paper presents a case study on how building authority can be integrated into Digital Twins and leverage its use throughout the lifecycle of a building. Future work can further investigate the challenges and develop prototypes that can help in quantifying the benefits of implementing Digital Twins on a Construction Project.
Introduction and Background
If we are to write the next chapter of the journey of shaping the future of the construction industry, an appropriate title would be Transforming Data in Action: Unearthing the Value of Data. A construction project can be analogized as a nexus from which various stakeholders connect from planning to decommissioning and from information exchange networks to realize the project. A construction project is built on reams of data generated from various sources throughout the project lifecycle (Dave and Koskela, 2009; Hatoum et al., 2020). Thus, it would not be an overstatement to say that a critical element of the future of construction companies and industry is data. With the advancement of information technologies and the emergence of a new generation of information technologies such as the Internet of things (IoT), cloud computing, big data analytics, and artificial intelligence (AI), the notion of data and a data-driven decision is becoming a commonly used phrase (Qi et al., 2021).
The quality of the collected data is critical for an organization to make informed decisions and gain insights into the progress and performance of the construction project (Thomas and Bowman, 2021). While data is an essential ingredient in the industry, it was estimated that the cost of bad data in the global construction industry amounted to approximately $1.84 trillion in 2020 (Thomas and Bowman, 2021). In the United States, poor project data alone pertains to 48% of the rework that occurs in the construction industry (Thomas et al., 2018). With the increase of challenges facing the industry and the inherited poor productivity and labor shortage, the industry is under tremendous pressure to deliver projects on schedule, on budget, while adding value and meeting the expectations of the owner. Such challenges force organizations and leaders to make critical decisions and emphasize the necessity of having access to comprehensive and reliable data (Ammar and Dadi, 2021). The increased focus on data and the opportunities created by digitization promoted the potential of the emerging phenomenon of Digital Twins in the manufacturing industry with the wave of Industry 4.0 to fulfill the requirements of Smart Factory (Negri et al., 2017; Kritzinger et al., 2018; Tao et al., 2019). The concept of Digital Twins then found its way to the Oil and Gas industry to optimize offshore operations, reduce risks of health, safety, and environment, and facilitate complex integrated processes (Wanasinghe et al., 2020).
Similarly, the construction industry began considering Digital Twins a key enabler for its digital transformation that could improve the industry’s poor record in digitization (Brilakis et al., 2019). However, the technology is the least investigated within the Architectural, Engineering and Construction (AEC) industry (Ammar and Nassereddine, 2022). Researchers believe that the integration between the IoT and Building Information Modeling (BIM) paved the way for the emergence of the concept of Digital Twin in the built environment (Deng et al., 2021). Others consider that the adoption of the Cyber-Physical Systems (CPS) in the construction industry, i.e., the adoption of multidimensional and complex systems that integrate the cyber world and the dynamic physical world (Tao et al., 2019), has facilitated the use of Digital Twin (Kan and Anumba, 2019).
In the academic literature of the construction industry, the term Digital Twin was mainly used as an extension of the static BIM models generated during the design and construction phases to more dynamic ones used in the operation and maintenance of complex building assets. This extension was achieved by embracing the integration with semantic web technologies, IoT, sensors, and implementing higher degrees of algorithms and AI (Alonso et al., 2019; Brilakis et al., 2019; Boje et al., 2020; Deng et al., 2021). Sacks et al. (2020) suggested replacing the BIM concept and developed the concept of Digital Twin Construction (DTC), a “data-centric construction management” workflow used to monitor and control the design and construction of projects using Digital Twin information systems. Conversely, Digital Twins and CPS share the same concept of bridging the cyber model (i.e., virtual) to the physical model and the physical model to the cyber model. However, the nature of the cyber-to-physical bridge is different. For Digital Twins the control is achieved by predicting the future state of the physical model which allows for making decisions in a passive way and in a timely manner. For CPS, on the other hand, the control of linking the cyber model to the physical model can be either passive or active where devices such as actuators can control the state of the physical system (Akanmu et al., 2021).
Several researchers conducted a comprehensive review of the Digital Twin concept in the built environment and compared it to BIM. Delgado and Oyedele (2021) discussed that the concepts of BIM and Digital Twin are different, yet both are necessary for different approaches depending on the industry’s requirements. The relationship between BIM and Digital Twin in the construction industry was thus further investigated. Deng et al. (2021) conducted a systematic review of the evolution of BIM to Digital Twins and developed a five-level ladder to reflect this evolution where level 1 represents BIM solely and is used for construction conceptual design and scheduling, level 2 is BIM with supported simulations implemented for facility management operation and estimation, level 3 illustrates the integration of BIM with IoT technologies supporting real-time tracking and real-time visualization, level 4 highlights a smarter integration of BIM with AI for data-based predictions and decision-making, and level 5 is the ideal Digital Twin which allows for control feedback, optimization, and integration. Furthermore, Atkins, an engineering and design company, proposed a Digital Twin maturity spectrum by defining six elements on a logarithmic scale of increasing complexity and connectedness (Evans et al., 2020). According to their report, a Digital Twin of an asset can be developed at any stage of the lifecycle of the asset and the process begins with data collection and reality capture (element 0) followed with the use of the collected data to create 2D maps/systems or 3D models (element 1) which is usually employed to create the as-built model. Then, information obtained from element 1 such as design information, material specifications, inspection reports, and asset management information enriched with data from BIM is extracted and connected to a static data set (element 2). Element 2 is later used for project planning, maintenance and operation, and decommissioning. Through the use of sensors and IoT technologies, data can be then updated in real-time enabling a continuous flow of data from the physical asset to the digital asset (element 3). Element 3 is used to predict asset performance and support decision making. The remaining two elements, element 4 and element 5, represent a higher level of complexity represented by the bi-directional integration and communication between the physical asset and the digital asset, and autonomous operation and maintenance supported by advanced algorithms and artificial intelligence.
With the emergence of the concept of Digital Twins in the construction industry, several researches identified the applications of Digital Twins in the built environment and discussed the potential of the technology in improving workforce productivity, health and safety, allow for efficient facility management, enhance the proficiency of the workforce, and reduce the cost of construction and operation (Bolton et al., 2018; Evans et al., 2020; Akanmu et al., 2021). Researchers further explained that Digital Twins uses multi-sourced data collected from sensors, historical data, or obtained by simulations to optimize the performance of the assets by monitoring and diagnosing the asset’s condition and enabling preventive predictions (Yitmen et al., 2021). Other researchers investigated the differences in the application emphasis of BIM and Digital Twin. Khajavi et al. (2019) conducted a comprehensive literature review on the visions and benefits of the Digital Twin for the built environment. The authors explained that the use of BIM for buildings is focused on applications related to design visualization and consistency, clash detection, lean construction, time and cost estimation, and stakeholder interoperability. Conversely, Digital Twin applications for buildings support predictive maintenance, enhance user’s comfort, allow for efficient consumption of resources, what-if-analysis, and closed loop designs. However, the authors further discussed that the essential components to create a Digital Twin of a building is to extract data from BIM and use various sensors to create a real-time view of the building. More recently, Opoku et al. (2021) conducted a literature review on the applications of Digital Twin in the construction industry and they classified the applications based on the project phase. It was found that the applications of Digital Twin are mainly focused on the construction and operation phase and limited applications are implemented in the design and planning stage where BIM is still mainly used. BIM capabilities of providing geometric modeling, coordination, Level of Detail (LOD), data schemes, and ontologies should be integrated with Digital Twin capabilities in the built environment without duplicating efforts (Delgado and Oyedele, 2021).
Additionally, Zhang et al. (2022) proposed a framework for utilizing Digital Twins for managing and monitoring construction sites by extending the adopted level of details for BIM models, by utilizing the digital presentation of the construction site provided by BIM and integrating the Digital Twin enabling technologies such as IoT, databases used for data storage and data analytics, and interaction with the existing construction site. Deng et al. (2021) discussed that implementing Digital Twins in the construction industry and collecting data from the building indoor environment or from construction sites by incorporating IoT enabling technologies such as WiFi, wireless sensor networks (WSN), 5G, LP-WAN, and monitoring cameras allows for real-time visualization of the building/construction site status and supports more efficient management. The authors further highlighted that Digital Twins can be implemented to conduct preventive maintenance and predict the building status by utilizing simulations including 4D construction simulations, energy and thermal consumption simulations, emergencies, and user comfort prediction or adopt machine learning techniques to target specific parameters that requires ground data such as the comfort of occupants. Moreover, the authors emphasized that Digital Twins support data-based decision making that relies on data collected in a real-time and used to inform decisions related to the construction progress and/or current status of the building and to predict future outcomes related to the building performance such as energy consumption and occupant’s thermal comfort. Other researchers proposed a Digital Twin platform “SPHERE” (Service Platform to Host and sharE REseidential data) which allows for seamless data integration between citizens, construction stakeholders, city administrators, and urban developers to better assess the design, construction, and performance of residential buildings. The main purpose of the developed platform is to improve and optimize the energy performance and design of residential buildings by reducing their emission of carbon dioxide and improving their environmental impact (Alonso et al., 2019).
In addition to exploring Digital Twins frameworks and platforms, few studies have investigated direct applications of Digital Twins in the construction industry. Examples of such applications include the use of Digital Twins system to monitor building assets and detect anomalies in a real-time manner by attaching sensors to assets with critical functionalities such as monitoring the vibration frequency of pumps or by monitoring the Quality of Service (QoS) of a combined system such as the HVAC system (Lu et al., 2020; Xie et al., 2020). The proposed model allowed the integration of multi-sourced data and facilitated data sharing across different stakeholders. Another application of Digital Twins considered the assessment of the structural integrity of certain structural members at the Cathedral of Milan by developing an exact 3D model of the building and study the response of the system under different conditions (Angjeliu et al., 2020). The developed Digital Twin model allowed the researchers to understand previous failures of structural elements of the building and conduct future preventive maintenance by taking necessary intervention. Additionally, Digital Twin frameworks formed of wearable sensors, VIVE Trackers, machine learning, and virtual reality were investigated to train construction workers on how to avoid musculoskeletal injuries by creating a postural training environment (Akanmu et al., 2020). Also, another Digital Twin framework was developed and used to support the digital supply chain by creating a Digital Twin of the construction material silos and having access to the silo’s current fill level by integrating sensors and IoT technologies (Greif et al., 2020). The proposed model supported the real-time tracking and repositioning of the silo transportation to construction sites and allowed planners to reduce time and cost required for construction material transportation and replenishment.
Along with the growing interest in Digital Twins among academicians, construction practitioners have been also exploring the practicability of the concept. For instance, the Center for Digital Built Britain (CDBB) has been working on setting definitions and principles across the built environment towards a vision for a National Digital Twin (NDT) an ecosystem of connected Digital Twins of physical assets to leverage the use of asset data for the benefit of the public (Bolton et al., 2018). buildingSMART International, the worldwide industry body driving the digital transformation of the built asset industry, formed the Digital Twins Working Group (DTWG) in 2020 to define what Digital Twins are and unlock potential social, environmental, and societal value for the entire built asset industry (buildingSMART International, 2021). Additionally, the European Commission funded a 4-year project in 2020 entitled “Cloud-based Building Information Modelling” or CBIM project to educate researchers in the development of innovative solutions that will automate the generation and automation of Digital Twins and boost the implementation of BIM to digitize the built infrastructure (European Commission, 2021). Recently, GlobalData published a report in 2021 on “Digital Twins in Oil and Gas—Thematic Research” highlighting the increased use of Digital Twins in the Oil and Gas industry (GlobalData, 2021). Moreover, McCarthy launched the “Digital Twin Challenge” in 2021, a competition that invites companies, startups, and other innovators in the technology community to submit innovative solutions that will enhance the Digital Twin approach in the construction phase and operation and maintenance phase of the construction project (McCarthy, 2021).
Research Gaps and Questions
As the research on Digital Twins continue to grow in the construction body of knowledge the term “Digital Twin” is used for a wide variety of purposes where Digital Twins was discussed either by comparing it to BIM or by presenting the benefits of using the technology across the project lifecycle. However, this vagueness and lack of understanding of the concept, where the distinction between a model and a digital twin is not yet clear, might prevent users from adopting the technology and therefore diminish the overall adoption of the technology (Wright and Davidson, 2020). Moreover, resources argued that understanding the value of Digital Twins in the construction industry stem from having a good understanding of the concept (Shahzad et al., 2022). Additionally, and as noted by several researchers, the cornerstone of creating a Digital Twin is the identification of its purpose and challenges from the perspective of its users, i.e., construction stakeholders (Khajavi et al., 2019; Abusohyon et al., 2021). Even though, the Digital Twins applications identified in the current body of knowledge explore how Digital Twins can be implemented in the construction project, there is no research project that has yet investigated Digital Twins applications and challenges from the industry perspective. As noted by Khajavi et al. (2019) and Abusohyon et al. (2021), involving practitioners in the Digital Twins dialogue is critical to gain a better understanding of the concept and the purpose of Digital Twins and the areas practitioners need to focus on to ensure a proper implementation of Digital Twins. Consequently, more research is needed to collect the input of construction practitioners on their perception of the use of Digital Twins throughout the different phases of the construction project and the challenges hindering construction practitioners from implementing Digital Twins. Additionally, Delgado and Oyedele (2021) stated that grounding Digital Twins applications in real-world contexts plays a critical role in communicating the potential of Digital Twins, subsequently, more case studies that showcase the use of Digital Twins are needed.
Therefore, this research presents a holistic definition of the Digital Twin of a construction project and aims to understand the perception of construction practitioners of the extent to which Digital Twin can deliver value throughout the project lifecycle by investigating Digital Twin applications, capabilities, and challenges. To ground this research in real-world context, a case study is also presented to showcase an example of Digital Twins in action. Specifically, this research addresses four research gaps by answering the following research questions:
1) What defines a Digital Twin of a construction project?
2) What are potential applications of Digital Twins in construction and what capabilities does this concept offer?
3) What challenges are perceived to hinder the implementation of Digital Twins on construction projects and need to be addressed?
4) How is Digital Twin implemented in action?
To answer the above questions, the paper is structured as follows: the research methodology is presented next to outline the approach undertaken to answer each research question. The remaining sections aim to fulfill the identified research gaps and answer the corresponding research questions. The proposed definition of the Digital Twin of a construction project is first provided (answering the first research question). Digital Twins capabilities and applications are then presented (answering the second research question), followed by the Digital Twins implementation challenges (answering the third research question). Next, a case study demonstrating the use of Digital Twins is discussed. Finally, conclusions and limitations are presented.
Research Methodology
A review of the existing work on Digital Twins in the construction industry led to the identification of four research gaps that this research investigates. The methodology employed to address each research gap and its corresponding research question is illustrated in Figure 1.
The first research question on the need to formulate a holistic definition for Digital Twins in the construction industry was addressed by reviewing and building on existing definitions. The second and third research questions targeting the need to solicit industry input on the applications, capabilities, and challenges of Digital Twins in the construction industry were sought by conducting interviews and analyzing the collected information using thematic analysis. Lastly, a demonstration of how Digital Twins can be implemented throughout the lifecycle of the construction project, identified as the fourth research question, was presented using a case study.
The approach undertaken to address every research question is discussed in the following sections.
Research Question 1 (RQ1)
The culminating effort of the literature review and elaborating on the various definitions of Digital twins from various industries assisted the authors to build on the existing knowledge and proposed their definition of the Digital Twin of a construction project, thus filling the first identified research question (RQ1 in Figure 1).
Research Questions 2 and 3 (RQ2 and RQ3)
Interviews
To answer the second and third research questions (RQ2 and RQ3 in Figure 1) and understand the potential of Digital Twins in the construction industry from the perspective of practitioners, qualitative research was used. Creswell and Poth (2016) explained that qualitative research is an inquiry process of understanding that allows the researcher to build a complex, holistic picture, analyze words, report detailed views of informants, and conduct the study in a natural setting. Among the different data collection methods used in qualitative research, structured interviews were selected to gather data on Digital Twins from the point of view of practitioners. Interviews enable the researcher to gain depths of information on the subject matter, especially when the subject matter is complex and unclear (Sheperis et al., 2010). Additionally, the primary uniqueness of interviews is the highest degree of interaction between the researcher and the participants (Sheperis et al., 2010).
The interview protocol, which is the first step in answering RQ2 and RQ3, started by stating the objective of the research and providing the definition of the Digital Twin of a construction project proposed by the authors. The visual was also presented to aid in the explanation of Digital Twins (Figure 2).
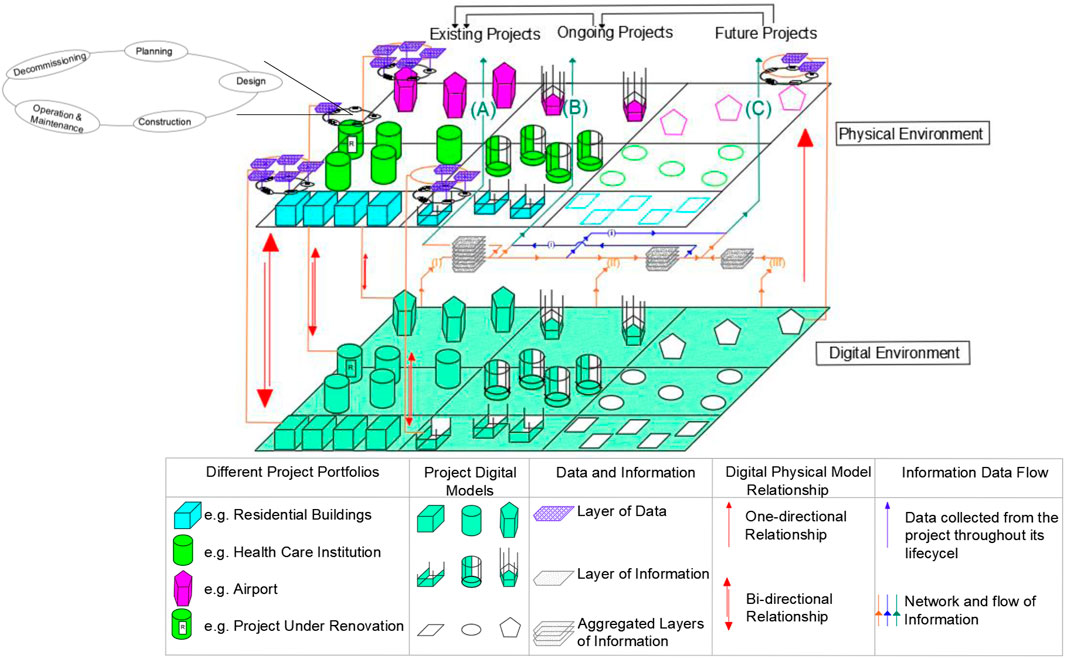
FIGURE 2. Visual representation of the proposed definition of the Digital Twin of a construction project.
Interviewees were then asked about their experience in the construction industry and if they are familiar with Digital Twins. To ensure the interviewees have a good understanding of Digital Twins, the interviewer answered any questions the interviewees had on the concept. Next, interviewees were asked to share their perspectives on the following:
- Challenges encountered in the construction industry that Digital Twins can assist in addressing
- Challenges that must be addressed to implement Digital Twins
Interviews were conducted virtually with nine construction practitioners with construction experience ranging from 5 years to 35 years. The profile of the interviewees is presented in Table 1. Virtual and in-person interviews were worth 29 h, where 23 h were transcribed verbatim from the online recordings and 6 h were captured in written notes from in-person interviews. Interviewers obtained the consent of the interviewees to record the virtual meeting.
Thematic Analysis
Once the data needed to answer RQ2 and RQ3 was collected through the first step (i.e., interviews), the second step was to analyze this data. Interview data was analyzed using thematic analysis, a technique used to thematically analyze and make sense of qualitative data in a systematic way that results in credible answers to the research objectives (Guest et al., 2011). The purpose of the thematic analysis employed in this research is exploratory (Guest et al., 2011) and aims to identify themes within qualitative data to address the second and third research questions (Maguire and Delahunt, 2017).
Thematic analysis of interview data proceeded in six phases as described by Braun and Clarke (2006):
- Phase 1: become familiar with the data by reading and re-reading the transcripts.
- Phase 2: generate initial codes that identify features of the data (semantic content or latent) that are deemed interesting to the research.
- Phase 3: search for themes, i.e., patterns that capture something significant about the data and its relation to the research objective. The codes generated in Phase 2 are examined in this phase and organized into broader themes.
- Phase 4: review the themes to refine those identified in the previous phase.
- Phase 5: define themes to identify the essence of what each theme is about.
- Phase 6: report on the results.
For the interview question on Digital Twins applications, the authors read the transcript of each interview and began coding the discussion that tacked the challenges faced by construction stakeholders and insights on how Digital Twins can address these challenges. A code is a type of raw data extracted from interviews and is a summary attribute that symbolically represents data or information generated by the researcher (Labra et al., 2020). Example of codes used to describe the interview data include concrete temperatures, materials, approvals, monitoring, project performance, project information, HVAC, project cost, procurement, flow of information, information exchange, sustainability, collaboration, analyzing, among others. The generation of these codes from the raw data led to the identification of 40 different Digital Twins applications shared by the interviewees.
Once the applications were extracted, the authors noted that some applications targeted specific areas or disciplines of the construction process, such as concrete, mechanical, fire systems, procurement, earthwork, among others. Upon reviewing all applications, the authors noticed that the divisions of the MasterFormat of the Construction Specifications Institute (CSI) can be used to define the scope of each application. The MasterFormat is the standard often used for formatting construction specifications in various countries including the US, Canada, and the Middle East and is a format that construction practitioners are familiar with. Additionally, the use of the CSI MasterFormat provides a means to organize the Digital Twins applications into work divisions that enables a structured and standardized way to investigate the potential use of Digital twins on a construction project. The most recent CSI MasterFormat published in 2016 and including 50 divisions was used to arrange the identified applications.
Next, Digital Twins applications were reviewed for the identification of themes. A theme is a sequence of words that serve as a synoptic and accurate representation of the signification that interviewees attribute to the subject matter (Labra et al., 2020). This inductive approach resulted in the identification of seven themes representing capabilities of Digital Twins in the construction industry as perceived by industry practitioners. Each theme, i.e., capability, is discussed in detail along with its corresponding applications to provide insights into the value of Digital Twins in the construction industry from the perspective of construction practitioners.
For the interview question on Digital Twins Challenges, similar analysis was performed to identify challenges associated with Digital Twins. Interviewees were asked to share their perception on what obstacles and challenges they expect their organization and the industry need to overcome to implement Digital Twins. The interview transcripts were reviewed and analyzed, and challenges were extracted. The essence of each challenge was then described using a code such as data, stakeholders, investments, and technology, to name a few. The codes were then reviewed and condensed further into themes that represent categories of challenges that need to be addressed to facilitate the implementation of Digital Twins on construction projects.
Research Question 4 (RQ4)
To illustrate the use of Digital Twins for different stakeholders and answer the fourth research question (RQ4 in Figure 1), a case study is discussed. A case study is form of qualitative research and offers an in-depth examination of the topic (Marczyk et al., 2010). The case study presented in this paper is developed in a research project with the City of Vienna titled Building Regulations Information for Submission Envolvement or BRISE-Vienna (Urban Innovative Actions, 2019; Krischmann et al., 2020). In this case study, the authors work together with stakeholders of the administration of the City of Vienna and experts in the construction industry to explore how Digital Twins can be used throughout the lifecycle of a construction project while integrating the building authority into the digital process. A step-by-step approach is discussed highlighting the benefits gained by the building authority of Vienna from using Digital Twins.
Digital Twins Definition
Existing Definitions
Digital Twins originated in aerospace engineering (Niederer et al., 2021) and are defined by the American Institute of Aeronautics and Astronautics (AIAA) in the context of engineering systems as “a set of virtual information constructs that mimic the structure, context, and behavior of an individual unique physical asset, or a group of physical assets, is dynamically updated with data from its physical twin throughout its lifecycle and informs decisions that realize value” (American Institute of Aeronautics and Astronautics, 2020).
The elements of Digital Twin as outlined in the AIAA definition, i.e., physical space, virtual space, and the data linking the two spaces, first appeared as elements of the “Conceptual Ideal for Product Lifecycle Management” in a presentation to the industry given by Dr. Michael Grieves in 2002 at the University of Michigan. The Product Lifecycle Management (PLM) signifies a dynamic representation between the physical product model and the virtual system throughout the entire lifecycle of the model, i.e., through the phases of creation, production, operation, and disposal (Grieves and Vickers, 2017). The concept of PLM was then replaced by “Mirrored Space Model” in 2005 in a journal article by Grieves (2005) and later was referred to as “Information Mirror Modeling” in books by Grieves (2006) and Grieves (2011). However, the explicit term “Digital Twin” first appeared in journal papers published by researchers at the National Aeronautics and Space Administration (NASA) in 2011 (Tuegel et al., 2011) and 2012 (Glaessgen and Stargel, 2012), where they adopted the concept in their technology roadmaps as a proposition for their next-generation fighter aircraft and NASA vehicles.
However, with the advancement in technology, the concept of Digital Twin is not limited to complex systems and can be implemented to provide a digital representation of any system. Research on Digital Twins has been conducted in a broad range of industries, reflecting a growing interest in and appreciation of the capabilities of this concept (Niederer et al., 2021). Table 2 provides an overview of some existing definitions of Digital Twins in different industries.
Examining the definitions provided for Digital Twins in the Construction Industry (Table 2), it can be noted that the context of the definitions varies. Some definitions focused only on the bidirectional relationship between the physical and digital environment. Other definitions were limited to including the components of Digital Twins and either ignored the capability of Digital Twins in sharing data between the physical and digital environment throughout the project life cycle and/or neglected the need to integrate with other digital technologies to make use of the shared data. These inconsistencies in the Digital Twins definitions were further investigated by Shahzad et al. (2022) where the authors concluded that a consensus about the definition of Digital Twins in the building environment is still lacking. The authors noted, however, that there is agreement that any definition of Digital Twins should be data-centric to leverage the development of Digital Twins for the built-environment. Consequently, a more holistic definition of Digital Twins for construction project is required to enable a more informed dialogue in the construction industry around Digital Twins.
Proposed Definition
Given the recognized need for a holistic definition of Digital Twin in the construction industry, this section builds on the existing definitions as well as the gaps identified and proposes a definition for the Digital Twin of a Construction project. The subsequent paragraphs present the rationale behind the proposed definition.
While the development of the Digital Twin of an asset requires the physical asset to be available to be twinned as noted in the definitions mentioned above, the creation and use of the Digital Twin of an asset and the insights it generated is not limited to the availability of the physical asset (Madni et al., 2019; Evans et al., 2020). Even though the concept of Digital Twin emerged initially in the manufacturing industry to monitor a product throughout its lifecycle, it is now being used to design and predict the behavior of the product in a virtual environment, thus allowing to differentiate between two types of Digital Twin: Digital Twin Prototype (DTP) and Digital Twin Instance (DTI) both operated in a Digital Twin Environment (DTE) (Grieves and Vickers, 2017). DTP includes all the information necessary to design and produce a physical asset. DTI refers to the digital twin of the physical asset in a specific instance and can be interrogated for their current state or past histories (Grieves and Vickers, 2017). The accumulation of data from multiple instances of the asset can be used to predict the product’s future state (Grieves and Vickers, 2017). This was further explained by Madni et al. (2019) where the authors described four levels of Digital Twin virtual representation. Level 1 is said to not require the presence of a physical twin and it represents a conceptual model to support decision making related to the design of an envisioned system to be built. After the establishment of a physical twin, a level 2 Digital Twin can be generated and is able to incorporate data from the physical model such as historical, performance, and maintenance data. However, an advanced level is an adaptive Digital Twin, i.e., level 3, which takes into consideration the user interface by learning the preferences and priorities of the user in different concepts achieved by pulling data from the physical model in real-time and continuously update the digital model. Finally, a level 4 Digital Twin is an intelligent model generated by integrating machine learning capabilities associated with a higher degree of autonomy by optimizing self-learning capabilities without human involvement.
In the context of the construction industry, this means that while the development of the digital twin can happen during construction and post-construction when the physical asset comes to life, the use of the digital twin spans the entire lifecycle of the construction project. Sacks et al. (2020) considered all the information used in a project workflow in their work on Digital Twin and put fore two types: 1) information about the future state of a project in both design and construction plans and is defined as Project Intent Information (PII) and refers to the as-designed and as-built state of the project, and 2) information about the past state of a project with records about the different versions of the condition of the project with details about the progress of construction and time and date at which it was measured and is defined as Project Status Information (PSI) and refers to the as-built product and as-performed process information. Both the PII and PSI of a project are considered part of the authors’ developed concept of Digital Twin Construction.
Building on the existing discussions on Digital Twins in the construction industry and to demystify the meaning of Digital Twins and account for the entire lifecycle of a construction project and the direction of data and flow of information exchange, the following definition is proposed by the authors: the Digital Twin of a construction project is the concept where a digital model related to either an existing, on-going, or future construction project is created and is linked throughout its lifecycle. When the project is existing or on-going, the digital model is a replica of the physical project and the two aspects (i.e., virtual and physical) have a bi-directional relationship enabled through advanced technologies including IoT, CPS, AI, and sensors. In the case of a future project, the digital models built from historical projects feed into the realization of the future project creating a one-directional relationship, which becomes bi-directional when the future project is in construction mode. Through this bi-directional relationship, the digital model is enriched in real-time with layers of up-to-date semantic data such as geometric, specifications, drawings, schedules, and financial reports that builds up through every phase of the construction project lifecycle and is an accurate representation of the project at a time t. This digital model is translated into information that construction stakeholders can use at time t and in the future.
The proposed definition of the Digital Twin of a construction project is visually represented in Figure 2.
Figure 2 begins with the physical environment which contains different project portfolios, each encompassing a number of projects designated with a certain shape and color. The projects in the physical environment can reflect existing projects that have already been constructed (existing projects), projects that are under construction (ongoing projects), or projects that are being considered in the future (future projects). Throughout the lifecycle of a project, and depending on its status (i.e., existing, on-going, or future), layers of data are being generated and collected.
The second environment illustrated in Figure 2 is the digital environment which hosts the digital models of the different projects. These digital models are enriched with semantic data obtained and collected from the physical environment. The data maturity of these digital models depends on the availability of data that feeds into them. For existing projects, two scenarios are considered. The first scenario is where a digital model is created for the project during its early phases allowing layers of data to be collected from all phases of the construction project lifecycle (i.e., planning, design, construction, and operation and maintenance). The generated data is used to create and continuously update the digital model of the project, which is then translated into layers of information [represented in orange, dashed arrow labeled (I)] that are analyzed and used to act and influence decisions made to the physical project itself in the operation and maintenance phase [flow represented in green, dashed arrow labeled (A)] or other on-going projects that share similar characteristics of the physical project under consideration [flow represented with green, dashed arrow labeled (B)] and future projects [low represented in blue, dashed arrow labeled (ii) and green, dashed arrow labeled (C)]. The second scenario considered a project that was planned, designed, and constructed with no Digital Twin established and needs to be renovated (example project illustrated in the green cylinder labeled R). Such projects can still benefit from Digital Twins where a digital model can be created with available data and enriched with data collected from the operation and maintenance phase of the project. Similar to the first scenario, the digital model is translated into layers of information that can be linked and used on the physical project being renovated or other on-going and future projects. For both scenarios, the relationship between the physical and digital assets is said to be bi-directional where data from the physical asset is being collected to enrich the digital model, which is then used to analyze the data and generate information to act upon in the physical asset.
Similarly, for ongoing projects, the physical and digital assets have a bi-directional relationship where data collected from the physical asset is linked to the digital model which represents the project at time t and is translated into layers of aggregated information [represented in orange, dashed arrow labeled (II)] that is integrated into the ongoing project itself [flow represented in blue, dashed arrow labeled (i) and green, dashed arrow labeled (B)] or into future projects [flow represented with green, dashed arrow labeled (C)].
Future projects benefit from the digital models and the layer of information aggregated from prior existing and on-going projects, creating a one-directional relationship between the digital and physical environment. Future projects when they get into construction mode become ongoing and a physical model is then available. Ongoing projects, in turn, become existing projects once completed (cycle illustrated in the upper part of Figure 2).
Digital Twins Capabilities and Applications
Interviewees were asked to elaborate on challenges the construction industry face and their perception on how Digital Twin can assist in addressing these challenges. The feedback collected from the interviewees was then analyzed using thematic analysis. The interview data was first coded and organized into the corresponding divisions of the 2016 MasterFormat of the Construction Specifications Institute (CSI). For instance, interviewees mentioned that their projects faced major challenges when pouring concrete, especially in hot weather, when project teams could not predict how the temperature of the cast-in-place concrete will vary, thus, failing to take preventive actions to ensure the quality of the concrete structure. Interviewees then elaborated that with Digital Twins, they can be better prepared for such situations and take preventive measures when high concrete temperatures are forecast. This data was coded as “concrete temperature,” “predict,” and “prevent” and lead to the identification of the Digital Twins application of “Predict peak concrete temperature to take preventive measures when limit is expected to be exceeded.” This application was then matched with the CSI division 03 which covers concrete works. The analysis of all interview data resulted in a total of 40 Digital Twins applications. The next step in the thematic analysis was to review the 40 identified Digital Twins applications to detect any patterns or themes that present an interpretation of how Digital Twins is perceived to be used (i.e., what Digital Twin can do). This step resulted in the identification of seven themes which are referred to as Digital Twins capabilities. The 40 Digital Twins application were aggregated into seven themes that illustrate capabilities of Digital Twins (i.e., what Digital Twin can do), namely: Increased Transparency of Information (with eight applications); Real-Time Monitoring, Analysis, and Feedback (with eight applications); Better Stakeholder Collaboration (with seven applications); Advanced Preventive Measures (with six applications); Advanced What-If Scenario Analysis and Simulations (with six applications); Real-Time Tracking (with three applications); and Higher Accuracy (with two applications). Considering the concrete example provided above, the “predict peak concrete temperature” coded Digital Twin application was found to illustrate an example of the “advanced predictive measures” capability of Digital Twins.
To visually illustrate the potential of Digital Twins, a 3D model of a house was obtained from the 3D Warehouse of SketchUp, and its components were broken down into the corresponding CSI divisions, showcasing examples of the Digital Twin applications shared by the interviews as outlined in Figure 3.
By examining the 40 identified Digital Twins applications outlined in Tables 3–9, it can be observed that most applications shared by the interviewees belong to the CSI division 01 entitled General Requirements where prices and payment procedures, administrative requirements, quality requirements, product requirements, execution and closeout requirements, performance requirements, and lifecycle activities are discussed. This observation highlights the idea of integrating Digital Twins in early phases of the construction project lifecycle, reinforcing the observation made by Evans et al. (2020) in the Atkins study.
The seven themes identified through the interviews are discussed in the following sections where each section includes 1) a table that presents the Digital Twins applications corresponding to a particular Digital Twins capability, and 2) a discussion that elaborates on some of the identified applications as perceived by the interviewees.
Increased Transparency of Information
Project success relies heavily on the management of the flow of information among players. The multidisciplinary and fragmented nature of construction creates a challenging environment for successful project implementation. Thus, increasing process transparency and bridging information gaps between construction stakeholders are key ingredients to the success of the project and to building strong relationships between the involved parties. According to Merriam-Webster (2021), the definition of transparent include “free from pretense or deceit”; “easily detected or seen through”; “readily understood”; and “characterized by visibility or accessibility of information especially concerning business practices.” While current practices and tools in construction, for instance relying on BIM as a static 3D visualization tool of the built environment do not promote full transparency of information, Digital Twin has the potential to build a culture of transparency by integrating real-time building environment data collected using smart IoT sensors which will contribute to better transparency of information flows within processes and among stakeholders. The applications identified by the interviewees for the Increased Transparency of Information are outlined in Table 3, and selected examples on how Digital Twins can create transparency in the construction industry are displayed below.
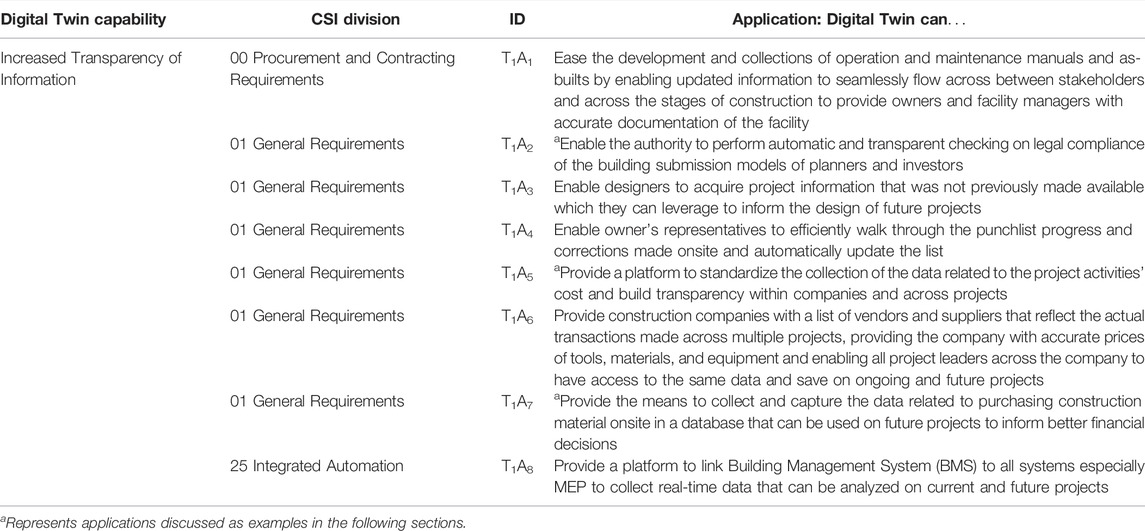
TABLE 3. Digital Twin capability of Increased Transparency of Information and the identified applications.
Example 1 on Submission Process (CSI Division 00 Procurement and Contracting): Existing processes established by the building authority to approve permits are based on 2D plans and result in a lengthy approval process for planners and investors (i.e., applicants). Having a Digital Twin of the planned facility with regulatory requirements associated with it enables a model-based submission process where geometric and semantic information obtained from the applicants’ model is checked by the building authority for legal compliance through Digital Twin. The compliance report is then seamlessly shared with the planner and the building authority. Automating the submission process with Digital Twins provides the city with an efficient strategy to ensure that all buildings have met certain minimum requirements, thus, increasing the quality of the model not only in the planning phase, but also for further use by downstream companies (e.g., construction companies) and the building owner and facility managers in the operation phase.
Example 2 on Payroll (CSI Division 01): Cost codes are central to resource allocation and management and activity-based costing of future projects and provide contractors and subcontractors with a strategy to determine where money is being spent, leading to identifying areas in need of productivity improvement. Keeping track of “who did what and how much was spent” is a challenge for contractors and subcontractors. Digital Twin provides a platform to standardize the collection of this data, building transparency within companies and across projects.
Example 3 on Purchased Materials (CSI Division 01): Contractors and subcontractors often purchase construction materials onsite, the information for which is not documented properly for later use or even shared with other sites. Digital Twin provides the means to collect and capture this data in a database that can be used on future projects to inform better financial decisions.
Real-Time Monitoring, Analysis, and Feedback
The physical-digital connection ensured by the concept of Digital Twin and powered through the advent of CPS, IoT, sensors, and AI enables real-time data to be captured, streamed to a digital platform, and shared with project stakeholders, which, in term, can monitor the live status of the project, perform analysis, and engage in closed feedback cycles across the construction project lifecycle. The applications identified by the interviewees for Real-Time Monitoring, Analysis, and Feedback are outlined in Table 4, and selected examples shared by the interviewees on potential Digital Twins applications to enable real-time monitoring, analysis, and feedback are illustrated below.
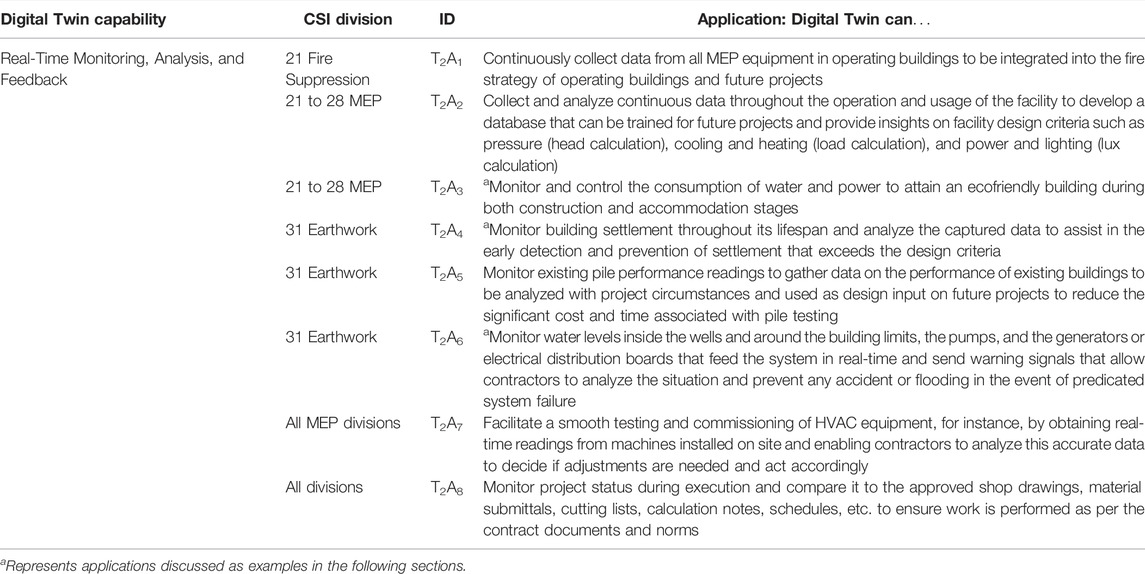
TABLE 4. Digital Twin capability of Real-Time Monitoring, Analysis, and Feedback and the identified applications.
Example 1 on Going Green with (Mechanical/Electrical/Plumbing) MEP Systems (All CSI Divisions related to MEP): Construction companies are engaging in addressing the threat of climate change and environmental degradation and more emphasis is being placed on sustainability and sustainable systems. For instance, buildings need to be energy-efficient and water-efficient, and thus, water and power consumption must be kept at its lowest rate to attain an ecofriendly building during both construction and operation phases. Users’ consumption of water and energy can be tracked through IoT sensors installed throughout the building. This information can be transferred from the physical asset to the digital asset where systems can be monitored, and data can be gathered for analysis and feedback to study how the systems are operated and what activities need to be further analyzed to achieve a more sustainable environment. Digital Twins of buildings in their operating phase can serve as input for the planning and design phases of future projects to add more value to the customer and build sustainability into design.
Example 2 on Earth Movement (CSI Division 31): Soil settlement in building especially differential settlement is one of the biggest challenges in construction as it threatens the building integrity, leading to concrete cracks and major structural damages to the building itself. Having a Digital Twin during and after construction can enable the real-time monitoring of the building settlement throughout its lifespan. Such application provides owners, engineers, contractors, and operators with the right data to closely monitor the performance of the building and analyze the captured data which can assist in the early detection and prevention of settlement that exceeds design criteria.
Example 3 on Dewatering (CSI Division 31): Dewatering is one of the most dangerous activities on site that carriers a lot of uncertainty when the ground water table levels are high, and the excavation level is deep. While contractors account for this uncertainty by applying appropriate safety factors and increasing the number of wells when designing the dewatering systems and by taking various precautions during execution and using standby pumps and generators, accidents still happen, and floods invade the jobsite. Digital Twin can be a powerful strategy that enables contractors to monitor water levels inside the wells and around the building limits, the pumps, and the generators or electrical distribution boards that feed the system in real-time and send warning signals that allow contractors to analyze the situation and prevent any accidents or flooding in the event of predicated system failure.
Better Stakeholder Collaboration
The making and operation of a construction project is a collective effort that requires the involvement of a wide range of organizations throughout its lifecycle. Construction project teams, thus, differ from teams in other industries as they comprise individuals who are employed by organizations that conduct different businesses. Building strong relationships between the different players is important for the success of the project. Communication, coordination, cooperation, and collaboration, collectively referred to as the Four Cs Model, are critical for forming a high-performing team. Among these four Cs, collaboration is perceived to have the most power because it represents the mutual engagement of participants in a coordinated effort to solve problems together. Digital Twins provides the means to promote better collaboration among all construction stakeholders and throughout the lifecycle of the construction project. The integration and synchronization of the physical and virtual assets enable a collaborative environment for stakeholders to create a shared meaning about a problem or a situation and formulate effective solutions or ideas. The applications identified by the interviewees for Better Stakeholder Collaboration are outlined in Table 5, and selected examples of how Digital Twin can achieve better collaboration for construction stakeholders are discussed below.
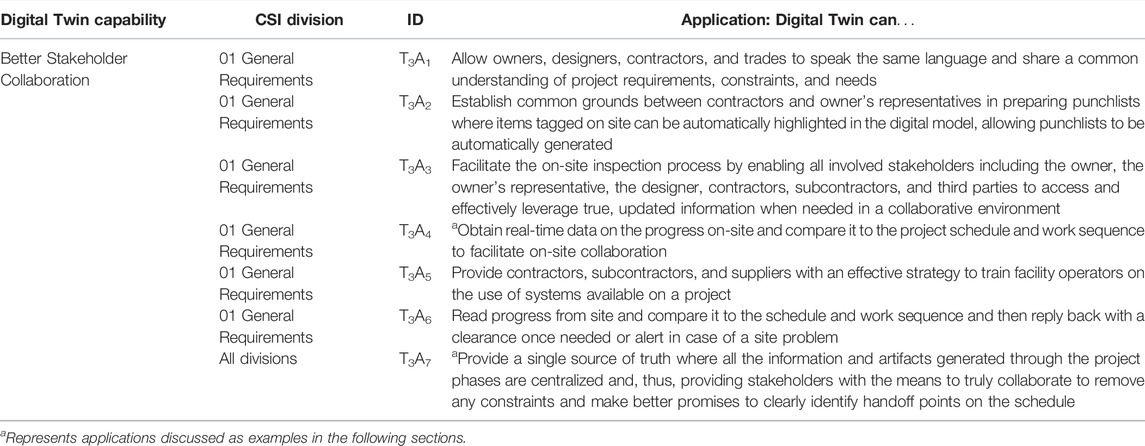
TABLE 5. Digital Twin capability of Better Stakeholder Collaboration and the identified applications.
Example 1 on On-Site Collaboration Between Trades (CSI Division 01): It is no surprise that trade contractors often work in silos and are focused on their specific scope of work, which leads to non-collaborative and adversarial relationships that negatively impact the project. MEP and finishing works conflicts, for instance, can cost the contractor and its subcontractors major losses if the trades don’t collaborate to fix the coordination conflicts and avoid rework. Having a Digital Twin of the MEP and finishing works installed in the field (as well as other construction works) provides the contractors and subcontractors with a platform to obtain real-time data on the progress on-site, which can be compared to the project schedule and work sequence. This comparison can improve collaboration on-site by facilitating clearance and handoffs between trades and bringing potential conflicts and problems to the fore.
Example 2 on Handoffs between trades (CSI Division 01): Identifying handoffs between trades and crews requires commitment from the project team and is critical to the schedule and flow of work. Removing constraints on handoffs is important for a seamless interface between trades. Digital Twin can provide a single source of truth where all the information and artifacts generated through the project phases are centralized and, thus, providing stakeholders with the means to truly collaborate to remove any constraints and make better promises to clearly identify handoff points on the schedule.
Advanced Preventive Measures
By providing an accessible platform to connect information from different sources and stakeholders, Digital Twins can enable construction project players to foresee scenarios by incorporating algorithms and data collected from smart IoT sensors to generate predictions and take necessary preventive measures in the construction project lifecycle. Interviewees noted that more often than not, project designers, constructors, and operators are responsive and not proactive in addressing issues that arise throughout the lifecycle of the construction project. They indicated that the unavailability and inaccessibility of the right data when needed and the ineffective use of available data in a timely manner are the major reasons that prevent construction stakeholders from being proactive and taking preventive measures on the construction project. The applications identified by the interviewees for Advanced Preventive Measures are outlined in Table 6, and selected examples shared by the interviewees to highlight the capabilities of Digital Twins in enabling preventive measures are discussed next.
Example 1 on Health, Safety, and Emergency Response Procedures (CSI Division 01): As safety continues to be a challenge in the construction industry, Digital Twin is perceived to have the potential in enabling a zero-accident site, a practice that every construction stakeholder strive to achieve. Wearable sensing technologies, coupled with visualization technologies, IoT, and AI can create opportunities for a proactive safety management to detect and resolve safety issues before incidents or injuries occur. Safety managers and officers can keep track of the location of danger zones, workers, and equipment and effectively prevent injuries that may be caused by unsafe location-based behaviors. Digital Twins can offer a two-way communication stream that enables workers to send supervisors data that the latter can use to protect the former from potential safety hazards. Additionally, safety knowledge captured from different projects can be fed into the Digital Twin of a future or on-going project to run safety simulations and identify potential hazards before they occur. Digital Twin can also be used for complex buildings with large flow of people to improve indoor safety management by inserting sensors inside the buildings and running different danger simulation for example illegal intrusion, overcrowding, and fire. Digital Twin can be implemented to improve construction work safety by documenting the occurrences of all possible risk behaviors, help in customizing training programs based on the documented risk behaviors, and trigger real-time warnings related to a risk behavior.
Example 2 on Product Storage and Handling Requirements (CSI Division 01): The storage of hazardous material at a job site is dangerous and must be carefully handled as lives are at stake. With Digital Twin, hazardous materials can be tracked to ensure they are properly stored and can be monitored to send early warning signs to prevent any disasters on the jobsite.
Example 3 on Model-Based Representation of Maintenance Space (CSI Division 01): Failing to consider minimum sizes of maintenance space for trades to install their equipment and for facility managers to perform their work in the planning phase leads to high costs and unfavorable special solutions in the construction and operation phase. A Digital Twin of the construction project allows the representation of these abstract aspects which do not exist in reality. These model-based maintenance spaces are verified and validated in the digital environment by means of collision checks (e.g., checking maintenance space in mechanical rooms). This enables early detection of problems that would otherwise only occur during execution and maintenance activities. Early detection of space constraints prevents clashes and increases efficiency in the operational phase of the building.
Example 4 on Concrete Temperature (CSI Division 03): Monitoring the temperature of cast-in-place concrete after pouring according to the American Society for Testing and Materials (ASTM) guidelines is a major step in the construction of concrete structures. Being proactive in predicting how the concrete temperature will vary, especially during extreme weather conditions, is critical to ensuring the quality, strength, and durability of the poured concrete structure. According to the interviewees, Digital Twins can empower contractors to forecast concrete performance and take preventive measures to ensure optimal curing conditions for the concrete structure. Interviewees explained that sensors can be installed in the to-be-poured concrete structure. Real-time data on the temperature of concrete can be continuously collected and linked to a BIM model of the concrete structure to visualize the progress and status of concrete performance. Artificial Intelligence can be then used to make data-driven predictions of concrete temperatures over time, indicating when the temperature is most likely to peak and what the peak value could be. The predicted peak temperature can be then compared to the ASTM guidelines to know if the value is within the acceptable limits or not, thus, allowing the contractor to take preventive measures when the temperature is expected to be beyond the allowed limit. Interviewees noted that readings of concrete temperature are already being collected on construction projects, providing a historical dataset that can be trained to inform predictions.
Example 5 on Concrete Mix Design (CSI Division 03): Rejecting a ready-mix concrete truck upon arrival on site because the concrete mix design used at the batch plant is different from the approved concrete mix design is unfortunately a common challenge for contractors which results in schedule delays, cost overruns, and increased conflicts on the jobsites. Digital Twins can be the link between the batch plant and the jobsite. Contractors can have access to real-time data of 1) the making of their concrete at the batch-plant which enables contractors to verify the mix ratios and concrete constituent proportions and 2) the delivery of the truck to make sure the right concrete batch will arrive to the jobsite.
Advanced What-If Scenario Analysis and Simulations
A construction project entails a myriad of decisions stakeholders need to constantly make across the lifecycle of the project. With the rapid advances in computing, data science, and AI technology, Digital Twin allows for these technologies to be leveraged and creates new opportunities to run what-if scenario analysis and nD simulations to learn from data and assist decision-making. What-if scenario analysis and nD simulations are key management techniques to assess risks and predict outcomes, performance, and challenges, and act proactively. The applications identified by the interviewees for Advanced What-If Scenario Analysis and Simulations are outlined in Table 7, and selected examples of how Digital Twins enable what-if scenario analysis and nD simulations are highlighted below.
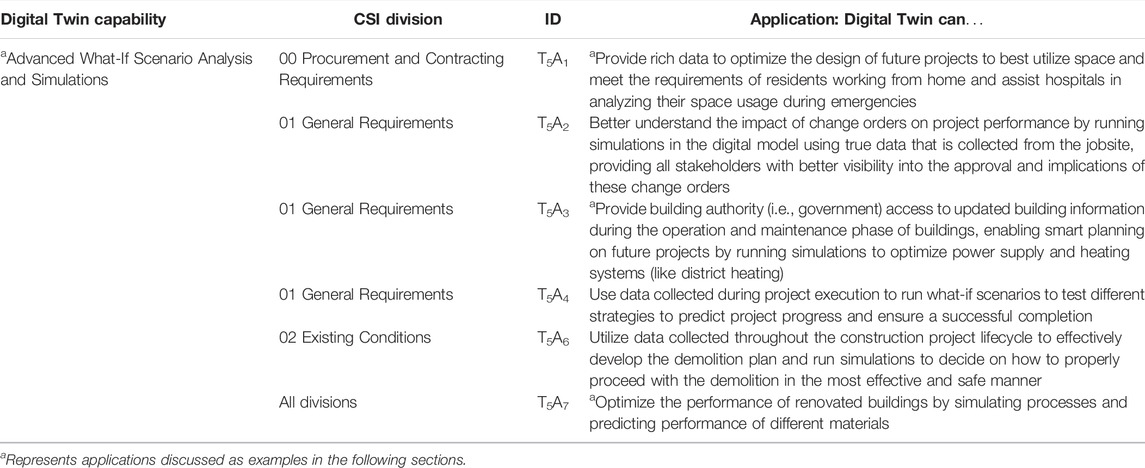
TABLE 7. Digital Twin capability of Advanced What-If Scenario Analysis and Simulations and the identified applications.
Example 1 on Occupants’ Use of Space (CSI Division 00): The COVID-19 pandemic involuntarily forced employees to transition to work remotely from home and to maintain social distancing when sharing space with others. This change in the working environment can influence the design of future projects where more focus is placed on the optimal use of space to satisfy the needs of end-users and meet new preferences. Using data collected on how occupants make use of their space, whether in their units or to maintain social distance in offices, and through Digital Twin, future project layouts and designs can be simulated in a digital environment to predict specific parameters such as occupant’s comfort, occupant’s use of oxygen, lighting access, and acoustic insulations. In the case of future pandemics, Digital Twins can be a powerful strategy for hospitals and healthcare facilities to run through what-if scenarios and simulations to analyze how their spaces can be best used to keep patients, healthcare professionals, and society safe.
Example 2 on Smart City Planning (CSI Division 01): To ensure compliance of all buildings throughout their lifecycle (i.e., from building submission to building operation), the building authority (i.e., the government) needs to check building characteristics (such as building height for the building submission process and building consumption of power during operation) and collect this data for future use as well. Digital Twins provide a platform for the building authority to access and collect this data automatically for all buildings. These individual Digital Twins can then enable the building authority to run simulations (e.g., energy supply, fire protection, land use simulations, and urban mining) to optimize the performance of future buildings and adjust requirements and specifications to fit strategies developed to address climate change and enforce sustainable processes.
Example 3 on Energy Performance (All CSI Divisions): Sustainable construction requires the prediction and optimization of a building’s energy consumption and performance. With the pressing need to address climate change and the drive toward a low carbon emission economy, the construction industry is challenged to adopt new construction approaches and use sustainable materials. Existing buildings can benefit from Digital Twin to explore different renovation processes that allow for cost reduction and optimized energy performance. Creating a Digital Twin for a building including its design, construction, operation, and maintenance phase will allow for conducting energy performance simulations and making decisions from an economical and environmental perception.
Real-Time Tracking
Traceability is an important concept for construction projects, especially complex projects. Being able to trace and track materials, products, equipment, people, and information across the project lifecycle can be challenging. Tracking produces real-time, up-to-date information that construction stakeholders in different phases of the project can leverage to inform and perform their tasks. Digital Twin can facilitate real-time tracking by its ability to integrate heterogenous data sources and, thus, achieve traceability of the construction project and its multi-varied components. The applications identified by the interviewees for Real-Time Tracking are outlined in Table 8, and selected examples of real-time tracking enabled through Digital Twin are presented next.
Example 1 on Material Storage (CSI Division 01): Materials are a key component of any construction project and ensuring the availability of materials on site is essential for continuous operations. Digital Twin can serve as a decision support system that enables construction players to make sure materials are available on-site to use when needed based on real-time data that shows where materials are being used and how much more is needed and when to eliminate delays and reduce operational costs.
Example 2 Construction Equipment Tracking (CSI Division 01): Construction sites can be haphazard environments with multiple heavy construction equipment operating at high speeds under different site conditions. Improving the productivity and safety of construction equipment requires awareness about the equipment’s surroundings, tasks to be executed, and site conditions. A Digital Twin of the construction equipment and the construction site can allow for direct interaction with operators to inform them of any change that requires instant intervention to optimize operations and enhance the safety of the workers and the equipment.
Higher Accuracy
Accuracy and access to accurate information are fundamental for construction stakeholders. Digital Twin, through visualization technologies, sensing technologies, IoT, and laser scanning, enables the creation of a useable, dynamic, and accurate digital model of a construction project (during construction and operation and maintenance). The accurate representation of the construction project digitally empowers construction stakeholders to validate their work, inform better decisions, and build more reliable databases for future use. The applications identified by the interviewees for Higher Accuracy are outlined in Table 9, and the following examples are shared by the interviewees to illustrate their thoughts on how Digital Twins can result in higher accuracy.
Example 1 on Site Surveying (CSI Division 02): Shoring is usually the footprint of the building and if done wrong, the entire design of the building will be affected. Digital Twin can enable the collection of accurate readings on the shoring coordinates on the job site which can be compared to the approved layout and design. The accuracy of the data collected from site provides contractors with a higher confidence level to detect discrepancies and prevent any misalignment, verticality, or layout problems.
Example 2 on Layout Work (All CSI Divisions): Start right. It is important when embarking on a new construction project to start right and set up the project properly. Wireless and sensing technologies can now enable contractors to accurately overly the digital model over the actual, physical asset. Interviewees added that checking the first fix of MEP installations can be challenging and is critical to avoid rework. When the physical and digital model are connected and synched, the data they both provide is thus accurate, allowing the contractors and subcontractors to inspect installation and check for misalignments virtually.
Digital Twins Challenges
Once interviewees discussed Digital Twin applications that they suspect to address current challenges and improve project performance, they were asked to elaborate on challenges the industry and construction companies must address and overcome. The authors collected 34 challenges from the interviews and grouped them into six themes or categories using Thematic Analysis as outlined (alphabetically) in Table 10. The identified categories are: Contractual Awareness and Knowledge; Data Understanding, Preparation, and Usage; Financial Uncertainties; Human Capital Development; Organizational Structure and Processes; and Technology Deployment. Interviewees were not asked to rank the challenges, and thus, the results provided in this section are exploratory and provide an overview of what practitioners are most concerned about. According to the analysis of the interviews, it can be observed that Data and the lack of understanding of its aspects, features, and guidelines is a major challenge for construction organizations when considering Digital twins.
Digital Twin in Action: A Smart City Planning Case Study
This case study is part of the ongoing research project titled BRISE-Vienna (Urban Innovative Actions, 2019), in which a research team is developing an openBIM based building submission process and is analyzing the surplus value of the submitted BIM model for the City of Vienna using Construction 4.0 technologies, including Augmented Reality (e.g., Schranz et al., 2021) and Digital Twins. The case study presented in this paper focuses on the use of Digital Twins by the building authority on future construction projects.
Recently, Vienna has been experiencing continued growth and demand for new buildings, pressuring the City of Vienna, i.e., the building authority, to issue thousands of new building permits every year. The established building verification and permission procedures are sophisticated and often based on 2D plans and documents submitted by the planners and investors, resulting in a lengthy process that is prone to information loss between the building authority and the planners and investors.
As digitization is becoming a must, planners and investors have increased their use of BIM when planning their projects and are able of producing a BIM model of their future facility by the time they submit the required project documents to the building authority. The transition of planners and investors to be more digital, coupled with the increased awareness of Digital Twins, has planted the seed for the digital transformation of the building authority as well to enable a fast and efficient building verification and permission process. The case study discussed below is a joint effort between the City of Vienna, TU Wien, experts from the engineering firm ODE (based in Vienna), and the Austrian Chamber of Architects and Chartered Engineering Consultants and aims to develop the process for integrating the building authority into the Digital Twin of a construction project throughout its lifecycle—i.e., from cradle-to-cradle. This end-to-end digital process is illustrated in Figure 4 which describes the relationship between the building authority and the actual building site.
As displayed in Figure 4, a building, throughout its lifecycle, has four major contact points with the building authority: building submission, completion notification, conversion, and demolition. At each interface, the BIM model is enriched with geometric information (e.g., model) and alphanumeric information (e.g., superstructure) and is handed over to the building authority by the owner.
The first interaction planners and investors have with the building authority is the building submission, where project required documents and a BIM model that contains both geometric and alphanumeric information are submitted. At this point of time, the building site is the only physical asset that is available. In the digital environment, however, the building authority will produce a 3D digital model of the building before it is erected using the documents submitted by the planners and investors. This 3D digital model is developed by the building authority and is enriched with the regulatory requirements that are specific to the project under consideration to ensure alignment with the existing regulations and specifications of the site. This model-based representation of the future construction project is considered to be the starting point for the Digital Twin of the building. Having a Digital Twin of the future building enables the building authority to perform a semi-automated check of the building code (e.g., building height and escape route length) and speed up the approval process for planners and investors (Krischmann et al., 2020). This application showcases the preventive measures capability of Digital Twin where potential non-compliances and deviations from regulations can be detected early on (e.g., safety requirements). Moreover, producing a Digital Twin at this phase of the building lifecycle (i.e., planning) allows citizens to be better involved in the planning process and be active decision-makers, as opposed to what they experience when they are provided with 2D plans (Schranz et al., 2021). The application of Digital Twins to improve the efficiency of the submission process is explained in detail in Urban et al. (2021).
In addition to enhancing the submission process, the potential of Digital Twins to improve the documentation of all building submissions was also explored to enable the City of Vienna to create robust databases that can be used on ongoing or future projects. Data fed into the Digital Twin and information generated from the Digital Twin can be integrated into databases that the building authority has control over and can leverage to ensure consistent data management across all buildings. Digitizing the submission and documentation processes through Digital Twins reduces redundant data input and potential sources of error and paves the way for additional applications. For instance, in addition to going through the submission process, each apartment in Vienna is required to be listed in a digital apartment register, where information on the usable living space, the heating system, the type of use, and address is provided. Usually, this information is entered manually into this separate register. Having this information transferred from the BIM model to the Digital Twin created at the onset of the submission process can enable the transparent flow of information where needed information can be accessed directly from the Digital Twin, resulting in time and cost savings and reduced data errors and inconsistencies. This application illustrates the capability of increased transparency of information enabled through the use of Digital Twins.
Moving across the building lifecycle, and during the construction phase of the building, requirements for site equipment and site safety (Zhang et al., 2013) can be collected and documented in the Digital Twin providing the building authority with good visibility into this data. This enables the building authority to perform semi-automated checks to compare the condition of the physical building to the requirements set in the Digital Twin. This allows building authority to give feedback to the construction company or the owner. Hence, this includes the capability of monitoring, analysis, and feedback.
Upon construction completion, the Digital Twin is updated for the building authority to access the needed information to carry out a change detection to the Digital Twin created after the submission. Digital Twins, thus, provide a platform to detect, check, and, if necessary, approve deviations more effectively, promoting a better collaboration between all stakeholders (i.e., building authority, owner, planners, contractors, and subcontractors). In the operation phase, Austria requires the submitting and regularly updating of a construction protocol. In this protocol, maintenance intervals, construction status, and an inspection report are listed. This documentation is ongoing and takes place throughout the project lifespan and is linked to the Digital Twin, which continues to reflect the actual status of the building. The building authority, thus, will continue to have access to the most recent project data through the Digital Twin.
In the case of the conversion of the building (i.e., changing the purpose of the building or performing retrofits), the Digital Twin can be used by the building authority in the same way as in the submission process. Additionally, the Digital Twin allows to use the data for urban mining (i.e., automatically generated an updated list of all materials used in the building along with properties associated with the materials, and appropriate means and methods to recycling and disposing materials). Also, the digital apartment register can be updated automatically. The use of Digital Twin for conversion is also applicable to the use of Digital Twins for demolition.
Looking into the long-term future, the development and maintenance of a Digital Twin for all buildings provides the building authority access to up-to-date Digital Twins throughout the phases of the building lifecycle. The sum of the Digital Twins of all buildings results in a Digital Twin of the city, named an Urban Digital Twin (UDT) which is similar to the vision of CDBB for a National Digital Twin (NDT) and is expressed as:
UDT creates new opportunities for strategic considerations (urban mining, area analysis, and fire protection analysis) and for further research activities (data basis for AI training and thermal simulation). Hence, UDT allows the city to perform advanced what-if scenario analysis and simulations to simulate the change of power supply or heating systems in a whole area for instance (i.e., change to district heating).
Conclusion and Limitations
As the construction industry goes through its digital transformation, interest in Digital Twins continues to grow among researchers and practitioners. While the research conducted to date has offered insights on Digital Twins in the construction industry, there is still no single definition of what this concept is. Additionally, while identifying the purpose of Digital Twin is recognized as the first step in implementing Digital Twins, there is little discussion in the existing body of knowledge on the perception of construction practitioners of the extent to which Digital Twin can deliver value. To answer the research questions, existing definitions on Digital Twins were reviewed and a holistic definition of the Digital Twin of a construction project that addresses the gaps in the existing definitions was proposed. A visual was created to illustrate the proposed definition and facilitate the discourse around Digital Twins in the construction industry by providing a common understanding of the concept, enabling an informed dialogue between the various construction stakeholders.
Next, to understand Digital Twins from the practitioner’s perspective, the authors conducted a series of semi-structured interviews with nine construction practitioners. The interviews discussed two items: 1) interviewee’s perception on the use and value of Digital Twins and 2) interviewee’s perception on the challenges associated with the implementation of Digital Twins. Thematic analysis was used to analyze the data collected from both questions. The analysis of the use of Digital Twins resulted in seven Digital Twin capabilities explained through 40 applications as outlined by the interviewees. The identified applications illustrated specific use-cases shared by the interviewees on the potential use of Digital Twins, thus addressing the gap in the existing work where little discussion was presented to date on direct examples of how Digital Twins can be used. Among the seven identified Digital Twin capabilities, Increased Transparency of Information and Real-Time Monitoring, Analysis, and Feedback were the most discussed, with eight applications articulated by the interviewees for each capability. Additionally, the majority of the applications shared are applied to the entire project, thus reinforcing the idea of the need to integrate Digital Twins in the early phases of the construction project. The findings also highlight the importance of other technologies to support these applications such as sensors and IoT. Construction stakeholders thinking about Digital Twins, need to invest in the right infrastructure to support the technology and must plan, design, construct, and operate their facilities with Digital Twins in mind from the early phases for a true implementation of Digital Twins.
The analysis of the data collected on the interviewee’s perception of Digital Twins challenges led to the identification of 34 challenges clustered into six themes or categories, namely, Contractual Awareness and Knowledge, Data Understanding, Preparation, and Usage, Financial Uncertainties, Human Capital Development, Organizational Structure and Processes, and Technology Deployment. Among the six identified themes, Data Understanding, Preparation, and Usage emerged as the theme with the most concerns for the interviewees, highlighting the need to better understand data requirements and specifications in the construction industry. The notion of data becomes critical in construction given the information-intensive, fragmented nature of the industry. Data is the fundamental underpinning for Digital Twins as it constitutes the channels of communication between the physical and digital environments. Data is the most valuable asset for every construction element, system, and process. Data standardization and governance play a crucial role in addressing data challenges in the construction industry and providing a better foundation for implementing Digital Twins.
This paper also showcases the feasibility of implementing Digital Twins by offering a case study that showcases the usage of Digital Twins of a construction project throughout its lifecycle from the perspective of the building authority. The case study builds on the proposed definition and the findings of the interviews, and illustrate how Digital Twins can be implemented in early phases of the construction project lifecycle. Also, it highlights the important and critical role data plays throughout the implementation process of Digital Twins.
This work contributes to the existing body of knowledge by introducing the practitioner’s point of view into the extent to which Digital Twin can deliver value, however, it has limitations that future work can address. The focus of the interviews was on the vertical section and Digital Twin for infrastructure was not discussed. A broader sample size of experts with more diverse experience in construction projects can generate additional insights on the value of Digital Twins. While Digital Twins challenges were identified from the interviews, the discussion of how these challenges can be addressed is outside the scope of this research and can be pursued in future work. Moreover, further research is needed to investigate the benefits of Digital Twins. Furthermore, the findings of this paper are based on qualitative research, which can be further validated as more Digital Twins applications are developed and tested, allowing the generation and analysis of quantitative data and the development of cost-benefit analysis to investigate full-scale implementation of Digital Twins.
Data Availability Statement
The raw data supporting the conclusion of this article will be made available by the authors, without undue reservation.
Author Contributions
All authors listed have made a substantial, direct, and intellectual contribution to the work and approved it for publication.
Funding
The project including the case study is funded by the Urban Innovative Actions initiative of the EU (Grant No. UIA04-081).
Conflict of Interest
Authors NA, AA, and JT were employed by ProMission FZ LLC.
The remaining authors declare that the research was conducted in the absence of any commercial or financial relationships that could be construed as a potential conflict of interest.
Publisher’s Note
All claims expressed in this article are solely those of the authors and do not necessarily represent those of their affiliated organizations, or those of the publisher, the editors and the reviewers. Any product that may be evaluated in this article, or claim that may be made by its manufacturer, is not guaranteed or endorsed by the publisher.
Acknowledgments
The authors would like to thank Stefanie Reichman Fassbender, P.E., for providing the 3D model used in Figure 3.
References
Abusohyon, I. A. S., Crupi, A., Bagheri, F., and Tonelli, F. (2021). How to Set up the Pillars of Digital Twins Technology in Our Business: Entities, Challenges and Solutions. Processes 9 (8), 1307. doi:10.3390/pr9081307
Akanmu, A. A., Anumba, C. J., and Ogunseiju, O. O. (2021). Towards Next Generation Cyber-Physical Systems and Digital Twins for Construction. ITcon 26, 505–525. doi:10.36680/j.itcon.2021.027
Akanmu, A. A., Olayiwola, J., Ogunseiju, O., and McFeeters, D. (2020). Cyber-physical Postural Training System for Construction Workers. Automation Constr. 117, 103272. doi:10.1016/j.autcon.2020.103272
Al-Sehrawy, R., and Kumar, B. (2021). “Digital Twins in Architecture, Engineering, Construction and Operations. A Brief Review and Analysis,” in Proceedings of the 18th International Conference on Computing in Civil and Building Engineering (Cham: Springer), 924–939. doi:10.1007/978-3-030-51295-8_64
Alizadehsalehi, S., and Yitmen, I. (2021). Digital Twin-Based Progress Monitoring Management Model through Reality Capture to Extended Reality Technologies (DRX). Bingley, United Kingdom: Smart and Sustainable Built Environment.
Alonso, R., Borras, M., Koppelaar, R. H. E. M., Lodigiani, A., Loscos, E., and Yöntem, E. (2019). SPHERE: BIM Digital Twin Platform. Multidiscip. Digit. Publ. Inst. Proc. 20 (1), 9. doi:10.3390/proceedings2019020009
American Institute of Aeronautics and Astronautics (AIAA) (2020). Digital Twin: Definition and Value – an AIAA and AIA Position Paper. Reston, VA, USA: AIAA.
Ammar, A., and Dadi, G. B. (2021). “Towards an Integrated Building Information Modeling (BIM) and Geographic Information System (GIS) Platform for Infrastructure,” in ISARC. Proceedings of the 38th International Symposium on Automation and Robotics in Construction (Dubai, UAE: IAARC Publications), 129–136. doi:10.22260/isarc2021/0020
Ammar, A., and Nassereddine, H. (2022). Blueprint for Construction 4.0 Technologies: A Bibliometric Analysis. IOP Conf. Ser. Mat. Sci. Eng. 1218 (1), 012011. doi:10.1088/1757-899x/1218/1/012011
Angjeliu, G., Coronelli, D., and Cardani, G. (2020). Development of the Simulation Model for Digital Twin Applications in Historical Masonry Buildings: The Integration between Numerical and Experimental Reality. Comput. Struct. 238, 106282. doi:10.1016/j.compstruc.2020.106282
Boje, C., Guerriero, A., Kubicki, S., and Rezgui, Y. (2020). Towards a Semantic Construction Digital Twin: Directions for Future Research. Automation Constr. 114, 103179. doi:10.1016/j.autcon.2020.103179
Bolton, A., Butler, L., Dabson, I., Enzer, M., Evans, M., Fenemore, T., et al. (2018). Gemini Principles. Cambridge, UK: CDBB_REP_006. doi:10.17863/CAM.32260
Braun, V., and Clarke, V. (2006). Using Thematic Analysis in Psychology. Qual. Res. Psychol. 3 (2), 77–101. doi:10.1191/1478088706qp063oa
Brilakis, I., Pan, Y., Borrmann, A., Mayer, H.-G., Rhein, F., Vos, C., et al. (2019). Built Environment Digital Twinning. International Workshop on Built Environment Digital Twinning Presented by TUM Institute for Advanced Study and Siemens AG. Available at: https://publications.cms.bgu.tum.de/reports/2020_Brilakis_BuiltEnvDT.pdf (Accessed November 5, 2021).
buildingSMART International (2021). Creating an Ecosystem of Digital Twins. The buildingSMART Digital Twins Working Group (DTWG). Available at: https://www.buildingsmart.org/digital-twins/(Accessed November 24, 2021).
buildingSMART International (2020). Enabling an Ecosystem of Digital Twins. Available at: https://buildingsmart-1xbd3ajdayi.netdna-ssl.com/wp-content/uploads/2020/06/Enabling-Digital-Twins-Positioning-Paper-Final.pdf (Accessed December 9, 2021).
Creswell, J. W., and Poth, C. N. (2016). Qualitative Inquiry and Research Design: Choosing Among Five Approaches. Thousand Oaks, CA: Sage publications.
Dave, B., and Koskela, L. (2009). Collaborative Knowledge Management-A Construction Case Study. Automation Constr. 18 (7), 894–902. doi:10.1016/j.autcon.2009.03.015
Davila Delgado, J. M., and Oyedele, L. (2021). Digital Twins for the Built Environment: Learning from Conceptual and Process Models in Manufacturing. Adv. Eng. Inf. 49, 101332. doi:10.1016/j.aei.2021.101332
Deng, M., Menassa, C. C., and Kamat, V. R. (2021). From BIM to Digital Twins: a Systematic Review of the Evolution of Intelligent Building Representations in the AEC-FM Industry. ITcon 26, 58–83. doi:10.36680/j.itcon.2021.005
European Commission (2021). Cloud-based Building Information Modelling | CBIM Project | Fact Sheet | H2020 | CORDIS | European Commission. Available at: https://cordis.europa.eu/project/id/860555 (Accessed October 5, 2021).
European Construction Sector Observatory (ECSO) (2021). Digitalisation in the Construction Sector. Available at: https://ec.europa.eu/docsroom/documents/45547/attachments/1/translations/en/renditions/pdf (Accessed December 13, 2021).
Evans, S., Savian, C., Burns, A., and Cooper, C. (2020). Digital Twins for the Built Environment: An Introduction to the Opportunities, Benefits, Challenges and Risks. London, UK: The Institution of Engineering and Technology. Available at: https://www.theiet.org/media/8762/digital-twins-for-the-built-environment.pdf (Accessed December 10, 2021).
Glaessgen, E., and Stargel, D. (2012). “The Digital Twin Paradigm for Future NASA and U.S. Air Force Vehicles,” in 53rd AIAA/ASME/ASCE/AHS/ASC Structures, Structural Dynamics and Materials Conference 20th AIAA/ASME/AHS Adaptive Structures Conference 14th AIAA (Honolulu, Hawaii: American Institute of Aeronautics and Astronautics). doi:10.2514/6.2012-1818
GlobalData (2021). Digital Twins in Oil and Gas - Thematic Research. London, UK: GlobalData Report Store. Available at: https://store.globaldata.com/report/gdog-tr-s048–digital-twins-in-oil-and-gas-thematic-research-2/(Accessed October 5, 2021).
Greif, T., Stein, N., and Flath, C. M. (2020). Peeking into the Void: Digital Twins for Construction Site Logistics. Comput. Industry 121, 103264. doi:10.1016/j.compind.2020.103264
Grieves, M. (2006). Product Lifecycle Management: Driving the Next Generation of Lean Thinking. New York: McGraw-Hill.
Grieves, M. (2011). Virtually Perfect: Driving Innovative and Lean Products through Product Lifecycle Management. Cocoa Beach, FL: Space Coat Press.
Grieves, M., and Vickers, J. (2017). “Digital Twin: Mitigating Unpredictable, Undesirable Emergent Behavior in Complex Systems,” in Transdisciplinary Perspectives on Complex Systems. Editors F. J. Kahlen, S. Flumerfelt, and A. Alves (Cham: Springer), 85–113. doi:10.1007/978-3-319-38756-7_4
Grieves, M. W. (2005). Product Lifecycle Management: the New Paradigm for Enterprises. Ijpd 2 (1/2), 71–84. doi:10.1504/ijpd.2005.006669
Guest, G., MacQueen, K. M., and Namey, E. E. (2011). Applied Thematic Analysis. Thousand Oaks, CA: Sage publications.
Hatoum, M. B., Piskernik, M., and Nassereddine, H. (2020). “A Holistic Framework for the Implementation of Big Data throughout a Construction Project Lifecycle,” in ISARC Proceedings of the International Symposium on Automation and Robotics in Construction (Kitakyushu, Japan: IAARC Publications), 1299–1306. doi:10.22260/ISARC2020/0178
Hochhalter, J. D., Leser, W. P., Newman, J. A., Glaessgen, E. H., Gupta, V. K., and Yamakov, V. (2014). Coupling Damage-Sensing Particles to the Digital Twin Concept. Hampton, VA: Technical Memorandum, NASA Langley Research Center. Available at: https://nari.arc.nasa.gov/sites/default/files/Hochhalter_NASA-TM-2014-218257_0.pdf (Accessed November 9, 2021).
International Organization for Standardization (ISO) (2021). ISO 23247–3: Automation Systems and Integration — Digital Twin Framework for Manufacturing — Part 3: Digital Representation of Manufacturing Elements. Geneva, Switzerland: ISO. Available at: https://www.iso.org/obp/ui/iso (Accessed November 9, 2021).
Kan, C., and Anumba, C. J. (2019). “Digital Twins as the Next Phase of Cyber-Physical Systems in Construction,” in Computing in Civil Engineering 2019: Data, Sensing, and Analytics (Atlanta, Georgia: American Society of Civil Engineers), 256–264. doi:10.1061/9780784482438.033
Khajavi, S. H., Motlagh, N. H., Jaribion, A., Werner, L. C., and Holmstrom, J. (2019). Digital Twin: Vision, Benefits, Boundaries, and Creation for Buildings. IEEE Access 7, 147406–147419. doi:10.1109/access.2019.2946515
Krischmann, T., Urban, H., and Schranz, C. (2020). Entwicklung eines openBIM-Bewilligungsverfahrens/Development of an openBIM submission process. Bauingenieur 95 (9), 335–344. doi:10.37544/0005-6650-2020-09-61
Kritzinger, W., Karner, M., Traar, G., Henjes, J., and Sihn, W. (2018). Digital Twin in Manufacturing: A Categorical Literature Review and Classification. IFAC-PapersOnLine 51 (11), 1016–1022. doi:10.1016/j.ifacol.2018.08.474
Labra, O., Castro, C., Wright, R., and Chamblas, I. (2020). Thematic Analysis in Social Work: A Case Study. Glob. Soc. Work-Cutting Edge Issues Crit. Reflections 2020, 1–20. doi:10.5772/intechopen.89464
Lu, Q., Xie, X., Parlikad, A. K., and Schooling, J. M. (2020). Digital Twin-Enabled Anomaly Detection for Built Asset Monitoring in Operation and Maintenance. Automation Constr. 118, 103277. doi:10.1016/j.autcon.2020.103277
Madni, A., Madni, C., and Lucero, S. (2019). Leveraging Digital Twin Technology in Model-Based Systems Engineering. Systems 7 (1), 7. doi:10.3390/systems7010007
Maguire, M., and Delahunt, B. (2017). Doing a Thematic Analysis: A Practical, Step-by-step Guide for Learning and Teaching Scholars. All Irel. J. High. Educ. 9, 1.
Marczyk, G. R., DeMatteo, D., and Festinger, D. (2010). Essentials of Research Design and Methodology (Vol. 2). Hoboken, NJ: John Wiley & Sons.
McCarthy (2021). NEXT Coalition Opens Call for Innovations to Tackle Digital Twins and Intelligent Facility Systems Challenge | McCarthy Building Companies. Available at: https://www.mccarthy.com/insights/next-coalition-opens-call-innovations-tackle-digital-twins-and-intelligent-facility-systems (Accessed October 5, 2021).
Merriam-Webster (2021). Definition of Transparent. Transparent. Springfield, MA. Available at: https://www.merriam-webster.com/dictionary/transparent (Accessed December 5, 2021).
Miskinis, C. (2018). Improving Healthcare Using Medical Digital Twin Technology. London, UK: Challenge Advisory. Available at: https://www.challenge.org/insights/digital-twin-in-healthcare/(Accessed December 8, 2021).
Negri, E., Fumagalli, L., and Macchi, M. (2017). A Review of the Roles of Digital Twin in CPS-Based Production Systems. Procedia Manuf. 11, 939–948. doi:10.1016/j.promfg.2017.07.198
Niederer, S. A., Sacks, M. S., Girolami, M., and Willcox, K. (2021). Scaling Digital Twins from the Artisanal to the Industrial. Nat. Comput. Sci. 1 (5), 313–320. doi:10.1038/s43588-021-00072-5
Opoku, D.-G. J., Perera, S., Osei-Kyei, R., and Rashidi, M. (2021). Digital Twin Application in the Construction Industry: A Literature Review. J. Build. Eng. 40, 102726. doi:10.1016/j.jobe.2021.102726
Qi, Q., Tao, F., Hu, T., Anwer, N., Liu, A., Wei, Y., et al. (2021). Enabling Technologies and Tools for Digital Twin. J. Manuf. Syst. 58, 3–21. doi:10.1016/j.jmsy.2019.10.001
Sacks, R., Brilakis, I., Pikas, E., Xie, H. S., and Girolami, M. (2020). Construction with Digital Twin Information Systems. Data-Centric Eng. 1, E14. doi:10.1017/dce.2020.16
Schranz, C., Urban, H., and Gerger, A. (2021). Potentials of Augmented Reality in a BIM Based Building Submission Process. ITcon 26, 441–457. doi:10.36680/j.itcon.2021.024
Shahzad, M., Shafiq, M. T., Douglas, D., and Kassem, M. (2022). Digital Twins in Built Environments: An Investigation of the Characteristics, Applications, and Challenges. Buildings 12 (2), 120. doi:10.3390/buildings12020120
Sheperis, C., Young, J. S., and Daniels, M. H. (2010). Counseling Research: Quantitative, Qualitative, and Mixed Methods. Boston, MA: Pearson.
Tao, F., Qi, Q., Wang, L., and Nee, A. Y. C. (2019). Digital Twins and Cyber-Physical Systems toward Smart Manufacturing and Industry 4.0: Correlation and Comparison. Engineering 5 (4), 653–661. doi:10.1016/j.eng.2019.01.014
Thomas, E., Bowman, J., Schott, P., Snyder, J., and Spare, N. (2018). Construction Disconnected. Mill Valley, CA: PlanGrid and FMI, 41.
Thomas, E., and Bowman, J. (2021). Harnessing the Data Advantage in Construction. San Rafael, CA: AUTODESK and FMI, 110.
Tuegel, E. J., Ingraffea, A. R., Eason, T. G., and Spottswood, S. M. (2011). Reengineering Aircraft Structural Life Prediction Using a Digital Twin. Int. J. Aerosp. Eng. 2011, 1–14. doi:10.1155/2011/154798
Urban, H., Schranz, C., Krischmann, T., Asmera, H., and Pinter, B. (2021). Einsatz von openBIM und KI im Bewilligungsverfahren der Stadt Wien/Use of openBIM and AI in the Approval Process of the City of Vienna. ÖIAZ – Österreichische Ingenieur- und Architektenzeitschrift. Vienna, Austria, 166.
Urban Innovative Actions (UIA) (2019). BRISE-Vienna – Building Regulations Information for Submission Envolvement. Available at: https://www.uia-initiative.eu/en/uia-cities/vienna-call4 (Accessed December 12, 2021).
Wanasinghe, T. R., Wroblewski, L., Petersen, B. K., Gosine, R. G., James, L. A., De Silva, O., et al. (2020). Digital Twin for the Oil and Gas Industry: Overview, Research Trends, Opportunities, and Challenges. IEEE Access 8, 104175–104197. doi:10.1109/access.2020.2998723
Wright, L., and Davidson, S. (2020). How to Tell the Difference between a Model and a Digital Twin. Adv. Model. Simul. Eng. Sci. 7 (1), 13. doi:10.1186/s40323-020-00147-4
Xie, X., Lu, Q., Parlikad, A. K., and Schooling, J. M. (2020). Digital Twin Enabled Asset Anomaly Detection for Building Facility Management. IFAC-PapersOnLine 53 (3), 380–385. doi:10.1016/j.ifacol.2020.11.061
Yitmen, I., Alizadehsalehi, S., Akıner, İ., and Akıner, M. E. (2021). An Adapted Model of Cognitive Digital Twins for Building Lifecycle Management. Appl. Sci. 11 (9), 4276. doi:10.3390/app11094276
Zhang, J., Cheng, J. C. P., Chen, W., and Chen, K. (2022). Digital Twins for Construction Sites: Concepts, LoD Definition, and Applications. J. Manag. Eng. 38 (2), 04021094. doi:10.1061/(asce)me.1943-5479.0000948
Keywords: Digital Twins, capabilities, applications, challenges, case study
Citation: Ammar A, Nassereddine H, AbdulBaky N, AbouKansour A, Tannoury J, Urban H and Schranz C (2022) Digital Twins in the Construction Industry: A Perspective of Practitioners and Building Authority. Front. Built Environ. 8:834671. doi: 10.3389/fbuil.2022.834671
Received: 13 December 2021; Accepted: 17 May 2022;
Published: 13 June 2022.
Edited by:
Zhen Chen, University of Strathclyde, United KingdomReviewed by:
Ibrahim Yitmen, Jönköping University, SwedenDimosthenis Kifokeris, Chalmers University of Technology, Sweden
Saleh Abu Dabous, University of Sharjah, United Arab Emirates
Copyright © 2022 Ammar, Nassereddine, AbdulBaky, AbouKansour, Tannoury, Urban and Schranz. This is an open-access article distributed under the terms of the Creative Commons Attribution License (CC BY). The use, distribution or reproduction in other forums is permitted, provided the original author(s) and the copyright owner(s) are credited and that the original publication in this journal is cited, in accordance with accepted academic practice. No use, distribution or reproduction is permitted which does not comply with these terms.
*Correspondence: Ashtarout Ammar, YXNodGFyb3V0LmFtbWFyQHVreS5lZHU=; Hala Nassereddine, aGFsYS5uYXNzZXJlZGRpbmVAdWt5LmVkdQ==; Nadine AbdulBaky, bmFkaW5lLmJha3lAcHJvbWlzc2lvbm1jc3MuY29t; Anwar AbouKansour, YW53YXJhazE0MUBsaXZlLmNvbQ==