- 1Faculty of Civil Engineering, Technical University of Cluj-Napoca, Cluj-Napoca, Romania
- 2Faculty of Geography, Babes-Bolyai University, Cluj-Napoca, Romania
- 3Cluj-Napoca Subsidiary Geography Section, Romanian Academy, Cluj-Napoca, Romania
- 4Image Processing and Pattern Recognition Research Center, Computer Science Department, Technical University of Cluj-Napoca, Cluj-Napoca, Romania
The uncertainty that comes with planning, constructing, and maintaining buildings is a constant issue for architects and civil engineers. As topography is the framework that unites architecture and landscape, the design and planning projects heavily rely on a range of monitoring, surveying methods and comprehensive field data. Along with the traditional topo-geodetic instrumentation used in land and construction surveying, unmanned aerial vehicles equipped with digital cameras and structure from motion software have been increasingly used recently in a variety of fields to create high-resolution digital elevation models. Despite this widespread use, in the majority of surveying projects it is considered that the topographic representations produced through this technology is inferior to that obtained with surveys conducted using conventional methods, along with other constraints imposed by legislation, environment and weather conditions. While certain limitations of unmanned aerial vehicle (UAV) systems are challenging, their advantage for gathering data from a different perspective and the generated outputs have the potential to significantly advance the construction industry. The present article provides an overview of the usefulness of budget UAV systems in developing a methodology that accompanies the conventional survey process for civil engineering applications. Thus, along with the established survey for cadastral and technical documentations necessary for the architectural process, a complementary UAV survey was developed, with subsequent spatial analysis in a geographic information system (GIS), in order to expand the array of deliverables. These include useful orthophoto map, larger-scale and denser representations of the topography, digital surface and terrain models, slope, aspect and solar radiation maps which will offer helpful information and instructions at the start of the construction planning process. The methodology contains two case studies with different degrees of terrain and vegetation challenges, and also presents an accuracy assessment and overall benefits discussion regarding the UAV implementation.
1 Introduction
The construction industry accounts for over 9% of the overall Gross Domestic Product (GDP) in the majority of nations, making the construction industry one of the most important economic sectors (Asadi et al., 2020). Despite its importance to the economy, this sector is afflicted by slow productivity and inefficiency at certain stages. The productivity rate has been continuously rising over the past few decades in many industries, but it has scarcely increased or stagnated in the construction sector (Pheng and Meng, 2018). By identifying productivity issues and implementing modern techniques of enhancing the design process or construction process, automation in construction systems is regarded as technologies or tools that have the potential to change the construction sector (Bock, 2015). Implementations with unmanned aerial vehicles (UAVs) have drawn a lot of attention recently for their use in the construction industry, with the four main applications in the construction sector today being: photography or videography, surveying, inspections, and safety or security monitoring (Tatum and Liu, 2017; Koukouvelas et al., 2020). UAVs give civil engineers and land surveyors new options and advantages by providing aerial perspectives that are challenging to obtain using conventional terrain instruments (Tkáč and Mésároš, 2019). Planning, constructing, and maintaining structures always presents a challenge for civil engineers and architects, with many different surveying and investigations necessary in these works. Thus, UAVs are becoming an indispensable tool for land surveying and civil engineering.
UAVs are aircrafts that are operated remotely from the ground. UAVs can be used for autonomous or remote-control observation or detection missions (Ham and Kamari, 2019). They are mostly utilized for mapping purposes and monitoring environmental change in applications related to Earth sciences. UAVs have two main benefits over other flying objects and satellite remote sensing technologies for taking aerial pictures: low cost and great mobility. With the right sensors and camera systems, UAVs offer a practical platform for collecting topographical data. GPS-enabled UAVs automatically follow a pre-planned GPS-controlled flight path (Barrile et al., 2019). In order to create high resolution 3D surface models, overlapping photos taken by the UAV are combined into a mosaic using photogrammetric systems that can produce high resolution images. These models can be used for topographic mapping, volumetric calculations, or three-dimensional representations of the terrain or construction sites (Liu et al., 2014; Kyriou et al., 2022).
A UAV offers a versatile and affordable transportable platform for gathering geographical data about items of interest. UAV-acquired datasets provide superior resolution in both temporal and spatial aspects than those collected by conventional aerial or satellite platforms (Turner et al., 2013). A UAV is also possible to collect multi-view spatial information because of its low flying height and agile posture, which makes it less vulnerable to cloud shadows, thus UAVs are seen as a reliable platform for mapping and monitoring applications (Carrera-Hernández et al., 2020). Nevertheless, UAVs also have some limitations, such as numerous environmental, meteorological or legal constraints, as well as lower flight stability (Mohamed et al., 2020). Consequently, a crucial question affecting the deployment of UAVs is how to employ them in various contexts to reliably process and generate geospatial outputs for qualitative and quantitative analysis (Jumani et al., 2022).
The use of aerial and satellite surveys that use photogrammetry as well as more recently airborne laser scanning, also known as Light Detection and Ranging (LiDAR), have all improved how the elevation of land surface can be sampled (Nouwakpo et al., 2015). Topographic data is often collected from ground surveys utilizing total station, differential Global Positioning System (GPS) or Global Navigation Satellite System (GNSS) readings, which can be time-consuming and laborious for high-resolution topography (Ouédraogo et al., 2014; Kyriou et al., 2022). In the previous two decades, LiDAR technology progressed significantly and now offers a high-precision topography mapping method (Oskin et al., 2012; Lin et al., 2013). The LiDAR technology can offer precise data and can quickly cover large areas, with the sensor advantage that can record data day or night. Yet, the high expense of this approach may have an impact on the project’s overall budget. High-resolution Digital Elevation Models (DEMs) can now be created using LiDAR, which has both high sample density and vertical precision. Active remote-sensing technology uses the travel time of the reflected laser pulse to calculate target distance. LiDAR can penetrate vegetation canopies to measure ground elevation more accurately than traditional survey methods (Lin et al., 2013; Li et al., 2017). Unfortunately, widespread LiDAR technology and surveys are still emerging and only recently are becoming affordable, but still possess logistical difficulties, and specific user expertise for data processing, which has limited their use in certain applications (Johnson and Ouimet., 2018). However, the combination of Structure from Motion (SfM) software and consumer-grade cameras mounted on lightweight unmanned aerial vehicles has made it possible to create both high-resolution topography and orthophotos (Bi et al., 2017). With the use of a number of tracked 2D characteristics on overlapping images, the computer vision technique known as SfM can simultaneously reconstruct 3D camera motion and 3D scene structure (Carrera-Hernández et al., 2020). Figure 1 highlights the mapping potential of UAV platforms, in regards to the greater array of instrumentations used for surveying.
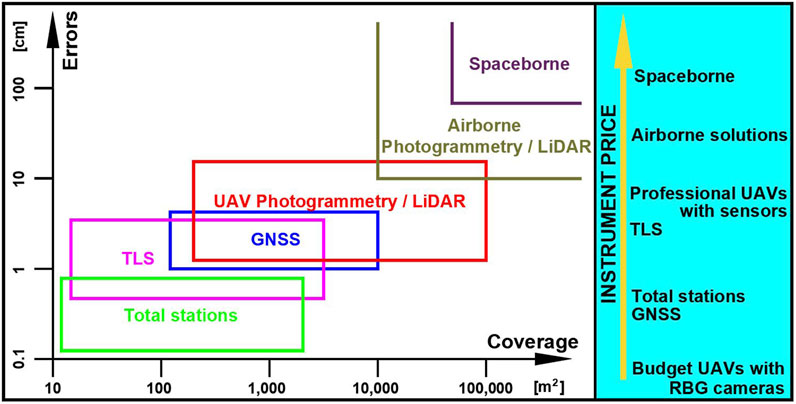
FIGURE 1. The UAV mapping potential vs. other instrumentation; modified and updated after Siebert and Teizer (2014).
UAV’s nimble mobility makes it feasible to capture spatial data from multiple viewpoints. A UAV can also get closer to a target item than any other remote sensing platform. As a result, it is simple to acquire thorough and high-resolution observations (Varbla et al., 2020; Nikolakopoulos et al., 2022). By closing the gap between the present aerial and ground platforms, this offers a fresh perspective on how to create high-fidelity 3D models. A UAV can gather spatial observations in a hostile environment that is too risky or inaccessible for other conventional mapping platforms due to its tiny size and remote moving capability. For example, Siebert and Teizer (2014) established the viability of UAV surveys for earth-works-related civil engineering applications by contrasting them to the conventional point-wise approaches. In the case of a 33-ha built-up terrain, Carrera-Hernández et al. (2020) established the superiority of the UAV survey over the traditional TS method. Alternately, Julge et al. (2019) demonstrated the advantages of UAV surveying for tracking road construction earthworks.
As topography is the framework that unites architecture and landscape, the purpose of this research was to offer a spatial methodology for the integration of budget drone systems with and GIS processing implementation of the derived UAV deliverables, in order to establish a spatial analysis and complementary terrain data that would be beneficial in the construction design process. Along with traditional approach applications of data gathering with topo-geodetic instrumentation and the conventional CAD outputs, this approach offers additional perspectives, both in term of coverage size, as well as the GIS generated maps, which will enhance the decisional process and aid the architectural and civil engineering team. With the primary goal of showing the interactions between terrain and built environment, the focus was on designing and implementing a GIS-enabled methodology of generating digital terrain model map, slope and aspect maps, solar radiation map. The present application further cements the usefulness of UAV surveys as additional data from the field, complementary to the established instrumental use of total stations (TS) and GNSS systems. The current photogrammetry and GIS methodology strengthens UAV implementation for supporting decision-making, assessing user perception, archiving personal knowledge maps that can be shared and reused, and validating the potential economic resource of terrains and built environments.
2 Materials and methods
2.1 Study area and proposed methodology
The focus areas of the research study are two locations inside Cluj County, which is situated in the middle of Romania’s ancient province of Transylvania, close to the wider metropolitan area of Cluj-Napoca City (Figure 2; Figure 3). Geographically, Cluj County is located in the central-western part of Romania, covering an area of 6,674 km2 (2.8% of the total land area). It has elements that could be categorized as a plateau, hilly relief, and mountains.
Romania’s rapidly developing built-up areas require high-quality, safe, timely, and affordable survey engineering services. As the world urbanizes, land becomes scarcer, the construction market is in high demand and the building industry is rising (Dolean et al., 2021; Sestras, 2021). Cluj-Napoca’s limits expanded and adjacent villages became suburbs due to urban development. After the 2020 pandemic limitations and lockdown, scenic mountainous places near urban centers have massively expanded in touristic potential and demand. Surveying engineers are in high demand because they can provide investors with legal and technical advice on property law, preliminary field measurements for construction design, and on-site technical assistance until the project is complete.
The current technical survey project involves two terrains (Figure 3) situated in mountainous regions situated a few dozens of kilometers of Cluj-Napoca. Due to the different configuration of the terrain, as well as to the different degree of vegetation coverage, the current research will include both studies with the distinction of Case Study A and Case Study B. Case Study A consists of a terrain with approximately 4,000 square meters, where the natural configuration consists of a significant slope and a high degree of vegetation, from low herbaceous vegetation, to medium and even high vegetation represented by trees. Case Study B consists of a terrain with approximately 1,400 square meters, where severe anthropic interventions have occurred in the last years, consisting of systematic earth works and fillings in order to generate a flat surface in an otherwise steep coniferous slope. The systematic configuration of the current terrain has no vegetation and presents a flat surface from the access road. Both properties were subjected to field measurements for cadastral and planning documentations, with the additional methodology of including UAV and GIS assessment in order to complement and expand the final product (Figure 4).
This study is divided into four phases: 1) a preliminary investigation and data collecting stage, which include the land survey and deliverables for cadastral and technical documentations; 2) complementary UAV survey with a budget system in order to generate photogrammetric outputs such as Digital Surface Model (DSM), Digital Terrain Models (DTM), orthophoto etc.; 3) the subsequent processing in GIS software in order to obtain spatial analysis maps and large-scale topographic representations useful in the planning and design process; 4) analysis of the obtained elevation accuracies from the established DSM versus the GNSS control points measured, as well as an overall evaluation of the added benefits of the established methodology.
2.2 Instruments and instrumentation used
Land surveying required a considerable amount of time, especially for large areas that need to be mapped (Herban et al., 2017). Consequently, the total cost of the project and the quantity of labor needed to complete the project increased when this strategy was implemented. In order to conduct construction surveying, the land survey method relies solely on human resources and available equipment. Due to this, design and planning can be susceptible to the repetitive errors that humans make. The undulating terrain poses challenges for land surveying methods, as humans must ascent and descend slopes in order to access the challenging site on their own. In addition to all the hazards and risks present in the field or on construction sites, one must also consider the environment and weather conditions (Sestras, 2021). Total stations (TS) automatically display, maintain, and measure angles and distances (Ghilani, 2017). Total stations ensure geometrical correctness in most surveying tasks. Instrument, operator, and peripheral equipment affect TS accuracy (Drewes et al., 2017). Topographical surveys also use GNSS systems in ideal field conditions (adequate satellite availability, network RTK services, broad field). GNSS has given accurate measurements, monitoring, and construction stakeouts for decades.
Due to the optimal field conditions present in both case study terrain (good satellite availability, internet connection, open field with no signal obstruction etc.), as well as the single person field mission, the topographical measurements were obtained with GNSS instrumentation. The National Cadastral and Land Registration Agency (ANCPI Romania) created the ROManian POSition Determination System (ROMPOS system), a nationwide network of permanent GNSS reference stations that ensures accurate location in the Stereographic 1970 coordinate system (the national projection system of Romania). Using a Stonex S9+ GNSS system in real-time kinematics (RTK) mode, the planimetric and altimetric positioning of the surveyed points was obtained (Figure 5).
The fundamental issue is that labor-intensive conventional point-wise surveying techniques, such as total station (TS) and GNSS (global navigation satellite systems) measurements, are not feasible for extensive 3D mapping. Thus, the surveying community can utilize recent technological advancements for data collection. One fast evolving surveying technique for reassembling the surface geometry of 3D objects is the use of UAVs and SfM photogrammetry (Westoby et al., 2012). Moreover, a conventional TS or GNSS survey’s spatial resolution is only as good as the points it covers, in contrast to the UAV SfM-derived topography, which is a byproduct of the resulting three-dimensional point cloud (Shahbazi et al., 2015). Thus, besides the GNSS instrumentation used, the drone utilized was a medium-priced, semi-professional DJI Mavic Pro with a 4K camera, made by Da-Jiang Innovations Science and Technology Co. Sharp photos are guaranteed by the 4K camera, which has an active stabilizing camera cradle head to counteract UAV vibrations and wind-induced tilt. In terms of processing power, a workstation with the following software was used: Autodesk products with TopoLT add-on for the CAD topographical plans, 3D models, contour lines and longitudinal profiles or cross sections; Drone Deploy was used for programming the flight mission, and Agisoft Metashape was the SfM photogrammetry application; ArcGIS was used for the subsequent spatial analysis and generated maps or figures.
2.3 UAV survey with DSM/DTM outputs
The DJI Mavic Pro UAV was employed for the acquisition phase of the photographic dataset for the photogrammetric 3D reconstruction of the terrains. Photogrammetry is used to create three-dimensional models from two-dimensional photos by triangulating images taken using high-quality cameras. The creation of 3D building models, contour maps, volumetric surveys, and other goods is possible using images taken by UASs or the LiDAR technology (Forlani et al., 2018). The image acquisition plan was divided into three steps: specification of image acquisition plan type, determination of Ground Sampling Distance (GSD), and definition of picture overlap (Bilasco et al., 2022) in order to obtain a model with centimeter accuracy and reconstruct a high-quality model. In order for the UAV to fly automatically, the image acquisition type was set to automatic waypoint flight mission. The following parameters were defined by the program to automatically determine the picture acquisition plan and mission settings: flight height (and subsequently GSD), overlap (%), and region to be covered. UAV imagery can be processed using a variety of conventional photogrammetric software programs. However, new software programs (e.g., Agisoft Metashape, Pix4D, 3Dsurvey, etc.) have been created that are more focused on analyzing UAV picture data and combine expertise from computer vision and conventional photogrammetry (Ćatić et al., 2020). These programs employ a strategy known as “structure from motion”. The photogrammetry program Agisoft Metashape was used to carry out the 3D reconstruction. Figure 6 depict the general photogrammetric workflow with the finalized orthophoto and 3D model.
UAV mapping sensors often use digital cameras. It records target object geometry and spectral information for mapping (Ismael and Henari, 2019), with some limitations. First, the elevations represent the digital surface model (DSM), which includes all vegetation, anthropic features, and structures from the field. Second, ambient light affects everyday photography. Shadows degrade photo calibration (Anders et al., 2020). Laser scanning or multi-spectral image sensors could address these concerns. Due to the UAV platform’s payload and economic constraints, carrying all of these mapping sensors is impracticable. This study compares traditional monitoring of known methodologies and instrumentation with UAV, which ensures accuracy and viability as an economical and effective tool that enhances traditional surveys for more detailed data. Table 1 displays the flying characteristics, metrics, as well as the obtained RMSE values. The equations used to assess the achieved accuracies of the generated 3D model are presented below Table 1, respectively Eq. 1, 2, 3 and 4.
UAVs' photogrammetric mapping capabilities are most beneficial to survey engineering. These benefits include: relatively low equipment costs; high levels of automation in photographic survey; extremely low operating costs; small size that is especially well suited for flying in confined spaces; high repeatability of the survey at low costs; ability to prepare the terrain in advance with ground control points (GCPs); and possibility of immediate results. The georeferencing process relies on the onboard GPS unit’s predicted camera positions (Stott et al., 2020). It speeds conjugate point detection and approximates georeferencing for the sparse point cloud with GPS receiver precision of 5–10 m. The sparse point cloud and camera positions are shown in the processing software, and the GNSS field recorded GCPs are used to precisely locate this 3D model in respect to the geodetic system (Sestras et al., 2022). Since their placements are predicted in all photographs, GCP recognition is manual but quick (Bilasco et al., 2021). A free block adjustment in any reference system can yield a 3D model, which can then be converted to a cartographic reference system using a 3D conformal transformation. A larger number of GCPs is preferable since it adds redundancy, which helps to improve the least squares adjustment and prevent drifting in long blocks of images connected only by conjugate points (Oniga et al., 2020). In the case of indirect georeferencing, it is important to correctly plan the quantity and spatial distribution of GCPs. When GCPs are distributed equally and put around the study area’s margins, greater accuracy can be achieved; more GCPs also generally produce better findings (Shahbazi et al., 2015; Martinez-Carricondo et al., 2018). However, Tonkin and Midgley (2016) and Agüera-Vega et al. (2017) research findings show that there is a maximum number of GCPs beyond which there is no additional improvement in accuracy. Based on expert knowledge and previous studies, at least one GCP is required for a few hundreds of square meters, and they should ideally be distributed uniformly and at different elevations depending on the configuration of the terrain (Oniga et al., 2020). A Stonex S9 GNSS receiver, a dual frequency receiver that can operate in RTK mode (Real Time Kinematics) via a data connection to a permanent GNSS network, was used to take the precise coordinate measurements for the GCPs. This technology produces high precision, particularly in situations without obstructions, which is the case for open field terrains with lower vegetation as in the two studied areas. Each point was often determined using more than 15 satellites. In planimetry and height, the instrumental indicated accuracy was approximately 1.5 cm on XY and 2.5 cm on Z. Repeatability tests were used to establish these accuracy values. Several independent check points (CPs) were surveyed in order to evaluate the final DSM vertical accuracy. These sites were selected since they were solely meant to be used to confirm the height accuracy. They were often chosen between and along the chosen GCPs, and are used in the photogrammetric processing in order to evaluate the obtained accuracy based on the RMSE values. A root mean square error (RMSE) between the DEM and a group of independent control points is used to measure the accuracy of a SfM-derived DEM. While the accuracy of the model is assessed using independent control points not previously utilized in the georeferenced model, it is commonly acknowledged that the more ground control points employed, the greater the resulting accuracy is. Since the geometry of the model changes depending on the referred points, evaluating the accuracy of a georeferenced model using GCPs is not entirely objective. Because of this, utilizing independent control points (CPs) offers a far more objective way to measure the actual accuracy of georeferencing techniques (Sanz-Ablanedo et al., 2018). The georeferencing (GCPs) and accuracy assessment (CPs) points were placed on various terrain features, as shown on Figure 7, in order to capture the change in elevation and provide optimal distribution.
Situational distinctiveness must be considered since SfM image matching algorithms depend on uniqueness, salience, and visibility of interest spots in the surroundings. Nouwakpo et al. (2015) demonstrated how monotonous vegetation produces unequal picture matching and model voids. Geometry determination may improve with a higher overlap ratio and many imaging directions. Multi-view stereo creates a dense point cloud for a triangle DSM. Figure 6 depicts the intermediate outputs of the photogrammetric process, including the sparse point cloud or tie points, dense point cloud, digital surface model (DSM), and orthophoto. For GIS analysis, both datasets can be exported in Geotiff format from the DSM orthomosaic. Figure 8 displays the initial inconclusive DSM-based elevation and slope map due to the tallest vegetation’s high altitudes and the overall concept of DSM versus DTM to grasp terrain representations needed for surveying and GIS analysis.
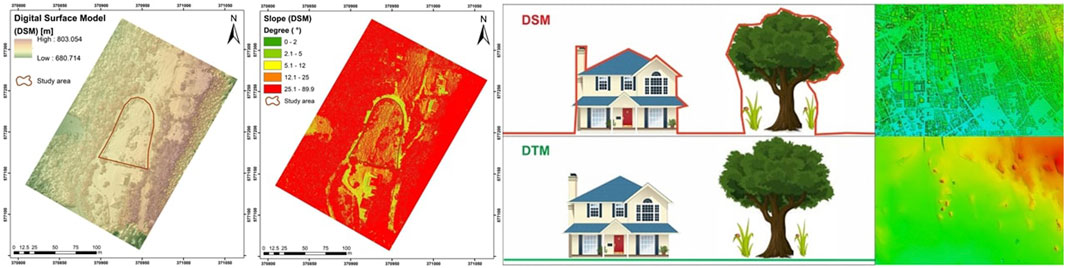
FIGURE 8. Initial inconclusive DSM based elevation and slope map, and the general concept DSM vs. DTM.
The Digital Terrain Model (DTM) is the desired output, whereas the Digital Surface Model (DSM) depicts the tallest neighboring surfaces. LiDAR technology can map bare terrain by penetrating vegetation (Fernández et al., 2021). Due to its higher operational cost, this technique is still being considered for small and medium surveying projects. Photogrammetric processing of the dense point cloud can produce accurate terrain renderings. After accurate categorization of the dense point cloud, clean ground points can be identified and separated from the dataset (Solazzo et al., 2018; Bandini et al., 2020). A mesh is generated from categorized ground points using one of several interpolation algorithms, creating a jointed fabric that matches the real-world ground model. Semi-automatic processing of the dense point cloud classified all features, partially removes noise, eliminated high and medium vegetation and anthropic structures, and interpolated terrain gaps to generate the DTM.
3 Results
3.1 Topo-geodetic instrumentation data with CAD generated results
Demand for medium and large-scale topographic mapping is expanding significantly. These maps display all natural and man-made features on the ground and give topographic information from contour lines and a 3D model. Traditional land surveying with total stations and GNSS instruments measures three-dimensional coordinates from the terrain for topographical mapping. Price, survey coverage, time efficiency, precision, and learning curve affect every tool and method. GNSS and total stations enable precise surveying, positioning, and observations. Despite updated technology, this equipment is still essential for land, cadastre, and construction surveys. Topographic maps accurately portray cultural and natural features. Newer topographic plans are essential for prefeasibility or feasibility evaluations for any investment projects (Figure 9).
Surveys, layouts, and monitoring projects require time and attention from a surveyor. Surveyor engineers are thought to be the last people to leave a construction site after they have arrived. Any investment construction project starts with site legal documents and feasibility studies that include topographic surveys to supply architects and civil engineers with geospatial data. This involves thorough fieldwork and data processing. These software and tasks combine field geodetic equipment for observations and data collection with advanced CAD platform graphical capabilities (Sestras, 2021).
The present research and professional endeavor began at the office with a Land Registry analysis to determine the technical and legal documentation needed. This topographic survey can include cadastral measures, technical needs for building planning, or both. Cadastral measurements focus on property lines and terrain accessibility. Technical measurement is more complicated for topographic drawings for building permits and other outputs for architects, civil engineers, and design engineers. Survey engineers must accurately depict the terrain surface for investment projects. The most common deliverables depend on the project: cadastre boundaries, mapping adjacent construction with their height or height regimen, a 3D model of the topography and contour lines created using CAD software, cross-sections or longitudinal profiles (especially important for infrastructure), utilities (sewage, water, electricity, gas, and telecommunication infrastructure), etc. After a complete survey of the area, accurate Land Registry data, and several architect and civil engineer documents, local authorities can issue a Building Permit. The project’s complexity and local zoning requirements may extend this process to 6 months. Besides the straightforward cadastral or technical documentations, the case study A property needed an additional boundary repositioning. This was due to severe inaccuracies between the measured boundary of the terrain and adjacent road, in regards to the boundary from the digital archive of the Land Registry. The subsequent cadastral documentation was very efficiently resolved with the complimentary attachment of an annex consisting of the orthophoto of the study area, with the two cadastral boundaries displayed, in order for the agency inspector to have a clear confirmation of the past error. All the mentioned stages and outputs are displayed as a graphical workflow in Figure 9.
3.2 GIS spatial analysis for further expanding field data
Geographic information systems (GIS) can be used to analyse visual qualities, the overall landscape scene, and spatial planning restrictions and opportunities. With the proposed methodology that can identify and formulate suitable criteria and spatial models for the right spatial planning integration, the focus was on integrating a GIS-based application alongside land surveying for building planning to highlight the interrelationships between landscape and building potential. This study’s application may be an innovative way to help decision-making, analyse user perception, generate personal knowledge maps that can be shared and reused, and validate possible economic resources associated to construction. These implementations and proposed methodology allow contemporary techniques to expand and acquire comprehensive digital maps, georeferenced orthophotos that provide field metrics and better visualization, pivotal digital surface models (DSM), and digital terrain models (DTM) used in GIS spatial analysis (Figure 10).
The technical stage of GIS spatial analysis based on UAV data aimed to arrange terrains within relief limitations. The point cloud from processed UAV pictures was analysed to create raster databases and themed maps for DTM-based elevation, aspect, slope, and solar radiation. These databases show how geomatic implementation supports planning and design. The high-resolution digital terrain model acquired using UAV technology and the aforementioned phases ensured that the medium to high vegetations and anthropic features have no effect on the geographical analysis and that the developed figures, including the DTM-based thematic maps, are more reliable and contain information that civil engineers, architects, land surveyors, and contractors can communicate to help the construction process.
The key element influencing where residential infrastructures are located is the relief and their features. The slope of the terrain and the orientation of the inclined surfaces are the primary factors that cause restrictiveness. The relief forms give benefits from the standpoint of enhancing any residential infrastructure, in contrast to the restrictions. The GIS generated DTM based elevation and slope maps for the two case studies highlights the fact that the relief of the analysed locations provide certain advantages and disadvantages, and anthropogenic changes are required. In case study A, it is high-lighted the natural configuration of the terrain, which presents an accentuated slope, with the most prominent category being in the interval 12.1–25°. In case study B, it is highlighted the anthropic configuration of the terrain, where severe man-made interventions have occurred in the last years, consisting of systematic earth works and fillings in order to generate a flat surface in an otherwise steep coniferous slope. Thus, the current terrain presents a relative flat surface, with the most prominent category being in the interval 2.1–5°. In order to lower construction costs, reduce the risk of natural disasters like flooding and landslides, and lessen the negative effects of the proposed development on natural resources like soils, plants, and water systems, it is crucial to take the slope of the ground into consideration. The graphical, colour gradient and larger scale maps generated in GIS offer complementary and additional information or perspective which is useful in the design process.
An essential factor in analyzing the terrain’s use during the colder months is the aspect of the sloping surfaces, and consequently its orientation. The direction of the area for both case studies is shown by the examination of the raster database, which was built using the digital terrain model. Thus, it is highlighted that both case study A and B have a dominant preponderance in the west and north-west oriented surfaces. Taking into account that the ideal aspect for the most optimal solar heat and light is the south and south-east orientation (Takebayashi et al., 2017), the design team can take the GIS generated maps into consideration, and plan the structures and roofs accordingly in order to increase the reception of higher quantities of light and implicitly of heat (Figure 11).
The ArcGIS Spatial Analyst extension modeled solar radiation (insolation) using solar radiation analysis. It depends on the location’s elevation, slope and aspect, topographic features, microclimate, air and soil temperature regimes, evapotranspiration, snowmelt patterns, soil moisture, and photosynthetic light (Yeom et al., 2019). Solar radiation affects building energy efficiency. This radiation lowers energy costs, increasing building value and competitiveness. Architects construct sunny structures with bigger window systems to maximize heat gain. Case study A has the most potential because to its high Sun exposure. However, example study B shows a decreased solar radiation potential, thus the design team must adapt the insulation accordingly.
Local PV potential must be considered when developing and funding photovoltaic (PV) systems in communities. The present GIS-based methodology allows spatio-temporal analysis of solar energy potential, starting from terrain potential data and extrapolating to roof and facade solar radiation models. The 3D database visualizes green energy plans and solutions. This approach is ideal for determining which portions of a site (roofs, facades, or bare land) are best for solar array construction and the space available for installation to maximize Sun collection. The Sun radiation database can solve many solar energy concerns, such as how to plan solar thermal or photovoltaic system installations or how to evaluate solar-related architectural design in building renovations.
Integrating UAV data with structure from motion software made field metrics attainable. Importing data into GIS systems allows for many spatial studies. Topographic maps, land-related services, construction surveying, volumetric calculations, surface areas, and other metrics, including hazard and risk assessment maps, are produced faster and in greater quantity with these analyses. The spatial analysis can also be linked into a BIM platform to improve and speed up analysis. While using the realistic 3D model that contains key spatial data and metrics used in architecture and construction design, exporting UAV data and integrating it into BIM tools, CAD platforms, or other architectural software is still arduous and limited. By isolating important aspects from photogrammetry or LiDAR data, new software is emerging that can vectorize anything on the point cloud. However, most small to medium land surveying organizations cannot afford these programs yet. Thus, the present methodology offers an alternative (Figure 12) to the convoluted or rather inaccessible process of creating spot elevations and vectorizations from point clouds, by taking full advantage of the GIS spatial analysis and already created database and thematic terrain maps.
The collage of Figure 12 illustrates the methodologic steps in creating a CAD ready survey, derived from the UAV data and GIS implementation. UAV-derived digital terrain model performs better in term of coverage and recorded features than a conventional GNSS or TS survey, which are prone to under sampling. Thus, the DTM was used and represented as a raster in GIS, with the subsequent steps: 1) a fishnet was created with the cell size of 2 × 2 m, with the possibility to lower the size and thus increase the generated elevation points; 2) a centroid was generated for each 2 × 2 m cell, thus obtaining more than 9,400 points on the study area DTM (on case study A); 3) the raster elevation value was extracted for each of the generated points, which can then be displayed in the GIS attribute table for further usage; 4) the GIS attribute table data, which included the planimetric position of each centroid point, as well as the DTM extracted elevation, was transferred to a spreadsheet software, where the data was easily arranged as traditional XYZ coordinates; 5) the XYZ coordinates were then imported into a traditional architectural and engineering CAD platform and generating required deliverables for the architectural design process, which include 3D model and contour lines, cross section or longitudinal profiles, etc. The right section of Figure 12 highlights the size and comprehensibility difference between the traditional GNSS or TS survey, versus the UAV and GIS derived survey. Steep parts are not accurately reflected by the GNSS or TS survey, as did not have enough surveying points, thus creating ununiformed topography representations. The UAV and GIS derived survey offer a better perspective of the studied area, with numerous additional useful metrics, in the detriment of a lower elevation accuracy, which will further be presented in the next section.
4 Discussion
The accuracy of the UAV-SfM approach has been examined in a number of studies; some of these studies have focused on which of the existing Structure from Motion software (Turner et al., 2013; Sona et al., 2014) generate better results, while others have evaluated the accuracy of the Digital Elevation Models generated with SfM (Ouédraogo et al., 2014) versus the Digital Terrain Model created from laser scanning. On a challenging rocky surface Westoby et al. (2012) compared a Structure from Motion derived DSM with one obtained by using a terrestrial laser scanning (TLS). All the previous research discovered that consistent decimetre-scale vertical accuracy can be attained with SfM, with even centimetre level accuracy when optimal conditions are available (Kršák et al., 2016; Devoto et al., 2020). Despite the advantages of using a TLS or airborne LiDAR for surveying, its drawbacks include its high cost, and the likelihood that multiple scan positions will be required to avoid blind spots and gain spatial coverage (Carrera-Hernández et al., 2020). A three-dimensional point cloud that has clarity and resolution on par with a LiDAR-generated point cloud can be created using UAV photogrammetry when the conditions are optimum (Leberl et al., 2010; Fonstad et al., 2013). It has been widely used for a variety of purposes because this technology makes it possible to generate high-resolution topography that was previously only possible through more expensive and labour-intensive methods.
The achieved accuracies are adequate for case study A and very good for case study B, when compared to the specifications for a preliminary or complementary topographic survey, which is the primary emphasis of this work. The results are corresponding to the Agisoft Methashape software’s robustness, to a properly designed network of GCPs, as well as the flight metrics selected in the mission planner. The layout of the targets used as GCPs, whose centres were precisely and clearly identified in the software as well as in the aerial photographs, appears to be another important factor in the accuracy attained. The precision of the GNSS system in RTK mode used to gather the GCP positions also directly influences the obtained results, as these kinds of measurements are known to have a vertical accuracy of 2–3 cm. This inaccuracy contributes to the estimation of the error because it is not significantly smaller than the obtained photogrammetric accuracy. The main factors remain the terrain’s features, which are primarily made up of different degrees of vegetation in case study A, while case study B was an ideal scenario with flat and bare ground terrain. In order to evaluate the accuracy of the obtained UAV derived DTM, it was calculated the vertical disparities between the DTM and the traditional GNSS land survey using the elevation values and the planimetric position of each point (ΔH = HDTM - HGNSS). To assess the deviation of the distribution of those differences, Figure 13 presents the obtained values.
While aerial surveys have tremendous coverage capabilities, less human intervention, and a large array of generated output and functionalities, the precision attained generally does not satisfy the geodetic standards as indicated in standardization normative, such as those produced by governmental organizations. Case study A represents a challenging environment, with a significant degree of vegetation and a sloping terrain. Figure 13 (left part) highlights the vertical differences between the obtained DTM versus the surveyed points, both on the infrastructure and in the vegetated field. Thus, while the values from the unpaved road are fairly adequate, averaging 4 cm in elevation difference, the values acquired in the field represent the topmost surface of the vegetation, resulting in considerable discrepancies between the measurements. In certain parts of the terrain, where the vegetation was lower or there were beaten paths, the elevation difference started from 7 cm, whereas in the higher grass the differences reached expected values of 60 cm. While the obtained results do not satisfy the required accuracies for building design, the data is still very useful for the presented spatial analysis, as well as for preliminary earthworks planning or feasibility studies. Case study B represents an optimal and rarely encountered scenario, where there was no vegetation present in the terrain, and the bare ground was compacted, offered a good structure and the lighting and meteorological conditions were on par. Thus, the obtained values are very good and quite serviceable in almost all engineering projects. Figure 13 (right part) highlights the vertical differences between the obtained DTM versus the surveyed points, both on the infrastructure and on the bare ground. In the case of the asphalted road, the vertical difference was very low, averaging 2–3 cm, with few exceptions that reached 4 cm on the extremities. For the bare ground recordings, the obtained differences averaged 4 cm, with some points reaching 8 cm near the northern extremity, due to poorer GCPs coverage. Nevertheless, the overall results for case study B confirms that the accuracy gained was within the bounds of criteria, UAV photogrammetry for medium and large-scale topographic mapping may effectively be used alongside technologies such as GNSS and TS, and be considered as alternative mapping solutions in certain ideal scenarios. Even if the obtained accuracies in case study B were appropriate for a large number of engineering projects, certain application still require the finesse only geodetic instrumentation possesses. Based on expert knowledge and the double-edged advantage of the flat surface present in case study B, the precision measurements required for lateral drainage system, where even less than 1% slope difference must be determined, can only be made with precise trigonometric levelling, or geometric levelling.
UAV-SfM topographic mapping is accurate enough for early engineering projects in optimum site circumstances and low vegetation. Thus, professional surveyors can use UAV-SfM technology since it creates a digital elevation model rather than points, is faster, and reduces human error. UAV flights can appease certain surveyed objectives straight into BIM or CAD, finer structural and terrain details need additional manual expertise. As a result, exclusively UAV surveys have not yet been able to completely replace the traditional surveying techniques. Nonetheless, an ideal solution, as presented in the current methodology, can be achieved by combining UAV with traditional point-wise measurements made with a GNSS or TS instrumentation. Since both instrumental surveys complement one another, good precision and efficient surface data acquisition are made possible.
5 Conclusion
UAVs have been under extensive development in the last decades, and their advancement in technology and applicability represents a quantum leap for many activity domains. Large-scale surveys are typically used in civil engineering to address uncertainties that may arise prior to, during, and after construction. UAVs give land surveyors, architects and civil engineers additional ways to comprehend their projects or the issues they encounter, as well as supplement the acquired data from the field. It is concluded that the use of UAVs and GIS spatial analysis can be a significant advancement in the research and professional applications of building design. These devices' very simple operation and the potential for obtaining high-resolution DSM, DTM and georeferenced orthophoto, make it possible to expand the databases and mapping techniques currently used in the construction industry. Operational difficulties still exist when using UAV photogrammetry for surveying. The greatest challenge is the environment, particularly the presence of medium and high vegetation. Systems with superior direct georeferencing, including dual frequency GPS on the UAV, as well as more precise and advantageous measurement sensors such as LiDAR solutions for better DTM determination will soon be available on a larger scale and affordability plan. The multidisciplinary methodology used in the study was practical, dependable, and successful. Data, interpretation, and discussion provide scientific and useful information pertinent to the study area and other research areas around the world. Based on the findings, further investigations and instruments will be expanded. As a result, LiDAR-equipped UAVs are the next desideratum for more thorough measurements which can penetrate the vegetation layer and provide more accurate representations of the bare ground, for further advances in architectural and civil engineering projects.
Data availability statement
The original contributions presented in the study are included in the article/supplementary material, further inquiries can be directed to the corresponding author.
Author contributions
All authors listed have made a substantial, direct, and intellectual contribution to the work and approved it for publication.
Funding
This work was supported by a grant of the Ministry of Research, Innovation and Digitization, CNCS—UEFISCDI, project number PN-III-P1-1.1-PD-2021-0145, within PNCDI III. The APC was funded by the Technical University of Cluj-Napoca.
Acknowledgments
The authors would like to thank the editor and reviewers for their helpful, valuable comments and suggestions that helped improve this paper.
Conflict of interest
The authors declare that the research was conducted in the absence of any commercial or financial relationships that could be construed as a potential conflict of interest.
Publisher’s note
All claims expressed in this article are solely those of the authors and do not necessarily represent those of their affiliated organizations, or those of the publisher, the editors and the reviewers. Any product that may be evaluated in this article, or claim that may be made by its manufacturer, is not guaranteed or endorsed by the publisher.
References
Agüera-Vega, F., Carvajal-Ramírez, F., and Martínez-Carricondo, P. (2017). Assessment of photogrammetric mapping accuracy based on variation ground control points number using unmanned aerial vehicle. Measurement 98, 221–227. doi:10.1016/j.measurement.2016.12.002
Anders, N., Smith, M., Suomalainen, J., Cammeraat, E., Valente, J., and Keesstra, S. (2020). Impact of flight altitude and cover orientation on Digital Surface Model (DSM) accuracy for flood damage assessment in Murcia (Spain) using a fixed-wing UAV. Earth Sci. Inf. 13, 391–404. doi:10.1007/s12145-019-00427-7
Asadi, K., Suresh, A. K., Ender, A., Gotad, S., Maniyar, S., Anand, S., et al. (2020). An integrated UGV-UAV system for construction site data collection. Automation Constr. 112, 103068. doi:10.1016/j.autcon.2019.103068
Bandini, F., Sunding, T. P., Linde, J., Smith, O., Jensen, I. K., Köppl, C. J., et al. (2020). Unmanned Aerial System (UAS) observations of water surface elevation in a small stream: comparison of radar altimetry, LIDAR and photogrammetry techniques. Remote Sens. Environ. 237, 111487. doi:10.1016/j.rse.2019.111487
Barrile, V., Fotia, A., Candela, G., and Bernardo, E. (2019). Integration of 3D model from UAV survey in BIM environment. Int. Archives Photogrammetry, Remote Sens. Spatial Inf. Sci. 42, 195–199. doi:10.5194/isprs-archives-xlii-2-w11-195-2019
Bi, H., Zheng, W., Ren, Z., Zeng, J., and Yu, J. (2017). Using an unmanned aerial vehicle for topography mapping of the fault zone based on structure from motion photogrammetry. Int. J. Remote Sens. 38 (8-10), 2495–2510. doi:10.1080/01431161.2016.1249308
Bilașco, Ș., Hognogi, G. G., Roșca, S., Pop, A. M., Iuliu, V., Fodorean, I., et al. (2022). Flash flood risk assessment and mitigation in digital-era governance using unmanned aerial vehicle and GIS spatial analyses case study: small river basins. Remote Sens. 14 (10), 2481. doi:10.3390/rs14102481
Bilașco, Ș., Roșca, S., Vescan, I., Fodorean, I., Dohotar, V., and Sestras, P. (2021). A GIS-based spatial analysis model approach for identification of optimal hydrotechnical solutions for gully erosion stabilization. Case Study. Appl. Sci. 11 (11), 4847. doi:10.3390/app11114847
Bock, T. (2015). The future of construction automation: technological disruption and the upcoming ubiquity of robotics. Automation Constr. 59, 113–121. doi:10.1016/j.autcon.2015.07.022
Carrera-Hernández, J. J., Levresse, G., and Lacan, P. (2020). Is UAV-SfM surveying ready to replace traditional surveying techniques? Int. J. remote Sens. 41 (12), 4820–4837. doi:10.1080/01431161.2020.1727049
Ćatić, J., Mulahusić, A., Tuno, N., and Topoljak, J. (2020). “Using the semi-professional UAV system in surveying the medium size area of complex urban surface,” in New technologies, development and application III 6 (Berlin, Germany: Springer International Publishing), 853–860.
Devoto, S., Macovaz, V., Mantovani, M., Soldati, M., and Furlani, S. (2020). Advantages of using UAV digital photogrammetry in the study of slow-moving coastal landslides. Remote Sens. 12 (21), 3566. doi:10.3390/rs12213566
Dolean, B. E., Bilașco, Ș., Petrea, D., Moldovan, C., Vescan, I., Roșca, S., et al. (2020). Evaluation of the built-up area dynamics in the first ring of Cluj-Napoca Metropolitan Area, Romania by semi-automatic GIS analysis of Landsat satellite images. Appl. Sci. 10 (21), 7722. doi:10.3390/app10217722
Drewes, H., Kuglitsch, F. G., Adám, J., and Rózsa, S. (2016). The geodesist’s handbook 2016. J. geodesy 90 (10), 907–1205. doi:10.1007/s00190-016-0948-z
Fernández, T., Pérez-García, J. L., Gómez-López, J. M., Cardenal, J., Moya, F., and Delgado, J. (2021). Multitemporal landslide inventory and activity analysis by means of aerial photogrammetry and LiDAR techniques in an area of Southern Spain. Remote Sens. 13 (11), 2110. doi:10.3390/rs13112110
Fonstad, M. A., Dietrich, J. T., Courville, B. C., Jensen, J. L., and Carbonneau, P. E. (2013). Topographic structure from motion: A new development in photogrammetric measurement. Earth Surf. Process. Landforms 38 (4), 421–430. doi:10.1002/esp.3366
Forlani, G., Dall’Asta, E., Diotri, F., Morra di Cella, U., Roncella, R., and Santise, M. (2018). Quality assessment of DSMs produced from UAV flights georeferenced with on-board RTK positioning. Remote Sens. 10 (2), 311. doi:10.3390/rs10020311
Ghilani, C. D. (2017). Adjustment computations: Spatial data analysis. Hoboken, New Jersey, United States: John Wiley and Sons.
Ham, Y., and Kamari, M. (2019). Automated content-based filtering for enhanced vision-based documentation in construction toward exploiting big visual data from drones. Automation Constr. 105, 102831. doi:10.1016/j.autcon.2019.102831
Herban, S. I., Vîlceanu, C. B., and Grecea, C. (2017). Road-Structure monitoring with Modern geodetic technologies. J. Surv. Eng. 143 (4), 05017004. doi:10.1061/(ASCE)SU.1943-5428.0000218
Ismael, R. Q., and Henari, Q. Z. “Accuracy assessment of UAV photogrammetry for large scale topographic mapping,” in Proceedings of the 2019 International Engineering Conference (IEC), Erbil, Iraq, 2019, June (IEEE), 1–5.
Johnson, K. M., and Ouimet, W. B. (2018). An observational and theoretical framework for interpreting the landscape palimpsest through airborne LiDAR. Appl. Geogr. 91, 32–44. doi:10.1016/j.apgeog.2017.12.018
Julge, K., Ellmann, A., and Köök, R. (2019). Unmanned aerial vehicle surveying for monitoring road construction earthworks. baltic J. road bridge Eng. 14 (1), 1–17. doi:10.7250/bjrbe.2019-14.430
Jumani, A. K., Laghari, R. A., and Nawaz, H. (2022). Unmanned aerial vehicles: A review. Cogn. Robot. 3, 8–22. doi:10.1016/j.cogr.2022.12.004
Koukouvelas, I. Κ., Nikolakopoulos, K. G., Zygouri, V., and Kyriou, A. (2020). Post-seismic monitoring of cliff mass wasting using an unmanned aerial vehicle and field data at Egremni, Lefkada Island, Greece. Geomorphology 367, 107306. doi:10.1016/j.geomorph.2020.107306
Kršák, B., Blišťan, P., Pauliková, A., Puškárová, P., Kovanič, Ľ. M., Palková, J., et al. (2016). Use of low-cost UAV photogrammetry to analyze the accuracy of a digital elevation model in a case study. Measurement 91, 276–287. doi:10.1016/j.measurement.2016.05.028
Kyriou, A., Nikolakopoulos, K. G., and Koukouvelas, I. K. (2022). Timely and low-cost remote sensing practices for the assessment of landslide activity in the service of hazard management. Remote Sens. 14 (19), 4745. doi:10.3390/rs14194745
Leberl, F., Irschara, A., Pock, T., Meixner, P., Gruber, M., Scholz, S., et al. (2010). Point clouds. Photogrammetric Eng. Remote Sens. 76 (10), 1123–1134. doi:10.14358/pers.76.10.1123
Li, Y., Yong, B., Van Oosterom, P., Lemmens, M., Wu, H., Ren, L., et al. (2017). Airborne LiDAR data filtering based on geodesic transformations of mathematical morphology. Remote Sens. 9 (11), 1104. doi:10.3390/rs9111104
Lin, Z., Kaneda, H., Mukoyama, S., Asada, N., and Chiba, T. (2013). Detection of subtle tectonic–geomorphic features in densely forested mountains by very high-resolution airborne LiDAR survey. Geomorphology 182, 104–115. doi:10.1016/j.geomorph.2012.11.001
Liu, P., Chen, A. Y., Huang, Y. N., Han, J. Y., Lai, J. S., Kang, S. C., et al. (2014). A review of rotorcraft unmanned aerial vehicle (UAV) developments and applications in civil engineering. Smart Struct. Syst. 13 (6), 1065–1094. doi:10.12989/sss.2014.13.6.1065
Martínez-Carricondo, P., Agüera-Vega, F., Carvajal-Ramírez, F., Mesas-Carrascosa, F. J., García-Ferrer, A., and Pérez-Porras, F. J. (2018). Assessment of UAV-photogrammetric mapping accuracy based on variation of ground control points. Int. J. Appl. earth observation geoinformation 72, 1–10. doi:10.1016/j.jag.2018.05.015
Mohamed, N., Al-Jaroodi, J., Jawhar, I., Idries, A., and Mohammed, F. (2020). Unmanned aerial vehicles applications in future smart cities. Technol. Forecast. Soc. change 153, 119293. doi:10.1016/j.techfore.2018.05.004
Nikolakopoulos, K. G., Kyriou, A., and Koukouvelas, I. K. (2022). Developing a guideline of unmanned aerial vehicle’s acquisition geometry for landslide mapping and monitoring. Appl. Sci. 12 (9), 4598. doi:10.3390/app12094598
Nouwakpo, S. K., Weltz, M. A., and McGwire, K. (2016). Assessing the performance of structure-from-motion photogrammetry and terrestrial LiDAR for reconstructing soil surface microtopography of naturally vegetated plots. Earth Surf. Process. Landforms 41 (3), 308–322. doi:10.1002/esp.3787
Oniga, V. E., Breaban, A. I., Pfeifer, N., and Chirila, C. (2020). Determining the suitable number of ground control points for UAS images georeferencing by varying number and spatial distribution. Remote Sens. 12 (5), 876. doi:10.3390/rs12050876
Oskin, M. E., Arrowsmith, J. R., Corona, A. H., Elliott, A. J., Fletcher, J. M., Fielding, E. J., et al. (2012). Near-field deformation from the El Mayor–Cucapah earthquake revealed by differential LIDAR. Science 335 (6069), 702–705. doi:10.1126/science.1213778
Ouédraogo, M. M., Degré, A., Debouche, C., and Lisein, J. (2014). The evaluation of unmanned aerial system-based photogrammetry and terrestrial laser scanning to generate DEMs of agricultural watersheds. Geomorphology 214, 339–355. doi:10.1016/j.geomorph.2014.02.016
Pheng, L. S., and Meng, C. Y. (2018). Managing productivity in construction: JIT operations and measurements. England, UK: Routledge.
Sanz-Ablanedo, E., Chandler, J. H., Rodríguez-Pérez, J. R., and Ordóñez, C. (2018). Accuracy of unmanned aerial vehicle (UAV) and SfM photogrammetry survey as a function of the number and location of ground control points used. Remote Sens. 10 (10), 1606. doi:10.3390/rs10101606
Sestras, P., Bilașco, Ș., Roșca, S., Veres, I., Ilies, N., Hysa, A., et al. (2022). Multi-instrumental approach to slope failure monitoring in a landslide susceptible newly built-up area: topo-geodetic survey, UAV 3D modelling and ground-penetrating radar. Remote Sens. 14 (22), 5822. doi:10.3390/rs14225822
Sestras, P. (2021). Methodological and on-site applied construction layout plan with batter boards stake-out methods comparison: A case study of Romania. Appl. Sci. 11 (10), 4331. doi:10.3390/app11104331
Shahbazi, M., Sohn, G., Théau, J., and Menard, P. (2015). Development and evaluation of a UAV-photogrammetry system for precise 3D environmental modeling. Sensors 15 (11), 27493–27524. doi:10.3390/s151127493
Siebert, S., and Teizer, J. (2014). Mobile 3D mapping for surveying earthwork projects using an Unmanned Aerial Vehicle (UAV) system. Automation Constr. 41, 1–14. doi:10.1016/j.autcon.2014.01.004
Solazzo, D., Sankey, J. B., Sankey, T. T., and Munson, S. M. (2018). Mapping and measuring aeolian sand dunes with photogrammetry and LiDAR from unmanned aerial vehicles (UAV) and multispectral satellite imagery on the Paria Plateau, AZ, USA. Geomorphology 319, 174–185. doi:10.1016/j.geomorph.2018.07.023
Sona, G., Pinto, L., Pagliari, D., Passoni, D., and Gini, R. (2014). Experimental analysis of different software packages for orientation and digital surface modelling from UAV images. Earth Sci. Inf. 7, 97–107. doi:10.1007/s12145-013-0142-2
Stott, E., Williams, R. D., and Hoey, T. B. (2020). Ground control point distribution for accurate kilometre-scale topographic mapping using an RTK-GNSS unmanned aerial vehicle and SfM photogrammetry. Drones 4 (3), 55. doi:10.3390/drones4030055
Takebayashi, H., Kasahara, M., Tanabe, S., and Kouyama, M. (2017). Analysis of solar radiation shading effects by trees in the open space around buildings. Sustainability 9 (8), 1398. doi:10.3390/su9081398
Tatum, M. C., and Liu, J. (2017). Unmanned aircraft system applications in construction. Procedia Eng. 196, 167–175. doi:10.1016/j.proeng.2017.07.187
Tkáč, M., and Mésároš, P. (2019). Utilizing drone technology in the civil engineering. Sel. Sci. Papers-Journal Civ. Eng. 14 (1), 27–37. doi:10.1515/sspjce-2019-0003
Tonkin, T. N., and Midgley, N. G. (2016). Ground-control networks for image based surface reconstruction: an investigation of optimum survey designs using UAV derived imagery and structure-from-motion photogrammetry. Remote Sens. 8 (9), 786. doi:10.3390/rs8090786
Turner, D., Lucieer, A., and Wallace, L. (2013). Direct georeferencing of ultrahigh-resolution UAV imagery. IEEE Trans. Geoscience Remote Sens. 52 (5), 2738–2745. doi:10.1109/tgrs.2013.2265295
Varbla, S., Puust, R., and Ellmann, A. (2021). Accuracy assessment of RTK-GNSS equipped UAV conducted as-built surveys for construction site modelling. Surv. Rev. 53 (381), 477–492. doi:10.1080/00396265.2020.1830544
Westoby, M. J., Brasington, J., Glasser, N. F., Hambrey, M. J., and Reynolds, J. M. (2012). ‘Structure-from-Motion’ photogrammetry: A low-cost, effective tool for geoscience applications. Geomorphology 179, 300–314. doi:10.1016/j.geomorph.2012.08.021
Keywords: land survey, mapping, UAV, photogrammetry, GIS, digital terrain model, construction planning
Citation: Sestras P, Roșca S, Bilașco Ș, Șoimoșan TM and Nedevschi S (2023) The use of budget UAV systems and GIS spatial analysis in cadastral and construction surveying for building planning. Front. Built Environ. 9:1206947. doi: 10.3389/fbuil.2023.1206947
Received: 16 April 2023; Accepted: 01 August 2023;
Published: 11 August 2023.
Edited by:
Zhen Chen, University of Strathclyde, United KingdomReviewed by:
Ioannis K. Koukouvelas, University of Patras, GreeceNazirul Mubin Zahari, Universiti Tenaga Nasional, Malaysia
Copyright © 2023 Sestras, Roșca, Bilașco, Șoimoșan and Nedevschi. This is an open-access article distributed under the terms of the Creative Commons Attribution License (CC BY). The use, distribution or reproduction in other forums is permitted, provided the original author(s) and the copyright owner(s) are credited and that the original publication in this journal is cited, in accordance with accepted academic practice. No use, distribution or reproduction is permitted which does not comply with these terms.
*Correspondence: Sanda Roșca, c2FuZGEucm9zY2FAdWJiY2x1ai5ybw==