- 1Wood Industry Division, National Institute of Forest Science, Seoul, Republic of Korea
- 2Department of Forest Science, Seoul National University, Seoul, Republic of Korea
Harvested wood products are a carbon pool that can be included in the national greenhouse gas inventory and are attracting attention as an important tool for reducing greenhouse gas emissions. This study evaluates the carbon reduction potential of harvested wood products by performing policy scenario analysis. It reveals that maximizing wood resource efficiency under existing policies to boost timber and sawn wood production offers the greatest reduction potential. This study found that the greenhouse gas reduction potential of the scenario with increased harvesting, in line with current government policies, is comparable to the scenario of cascading wood resource utilization without increasing harvest levels. This study provides valuable policy insights that promote national greenhouse gas reduction, while leading to a societal transformation that guarantees ecological soundness through the efficient use of domestic timber resources.
1 Introduction
Global forests play a crucial role in either significantly increasing or decreasing atmospheric greenhouse gas (GHG) concentrations (Geng et al., 2017). Harvested wood products (HWPs) are a vital component of the forest-atmosphere carbon cycle, contributing significantly to the mitigation of carbon dioxide emissions. HWPs have remarkable potential to lessen the impacts of climate change, with the ability to reduce carbon dioxide emissions by as much as 441 Mt annually by 2030, an increase from 335 Mt in 2015 (Johnston and Radeloff, 2019). Recognized as a carbon pools that can be included in the National Inventory Report (NIR), HWPs are becoming increasingly valued as essential tools for decreasing greenhouse gas emissions.
However, a critical gap exists in the life cycle of wood products, particularly in the transfer of raw timber from forests to the wood-processing industry, and subsequently to primary wood products, which then leads to significant variations in the potential for GHG depending on how these products are utilized across different industries (Profft et al., 2009). The carbon storage capacity of HWPs varies depending on their usage (Geng et al., 2017).
This may lead to either the carbon stock of HWP becoming a net source or turning into an emission source, depending on the end-use utilization pattern. In this context, only some countries with favorable conditions may contribute to mitigating the impacts of climate change.
While HWP was previously known to have a trade-off relationship with other ecosystem services, there is increasing interest in achieving synergy based on sustainable forest management and the efficient utilization of wood products from the perspective of climate change mitigation. According to a previous study (Sikkema et al., 2016), the cascading use of resources based on the order of priority is emphasized while utilizing wood resources at the national level (European Commission, 2013). Wood resources are efficiently utilized by using hardwoods in products with high durability and high added value, such as sawn wood, recycling as boards, and recovering as energy at the last stage (Wakelin et al., 2020).
The need for the cascading use of wood resources lies in their limited supply. Wood is a renewable resource used to achieve greenhouse gas emission-reduction targets, and its energy demand has increased significantly (Heinimo and Junginger, 2009; Bais et al., 2015). To achieve the emission reduction goals of the Paris Agreement, the energy demands of wood as a renewable energy source are expected to increase continuously. Meanwhile, several countries have introduced the use of biomass as an important instrument for the transition to a low-carbon green economy (Keegan et al., 2013). While there is increasing awareness of the eco-friendly and low-carbon properties of wood (Petersen and Solberg, 2005), demand for wood that surpasses the marginal line of sustainable wood supply may result in unexpected negative environmental impacts. Increased competition for wood resources (Hagemann et al., 2016) may cause a trade-off between wood production and other ecosystem services (Bradford and D’Amato, 2011). Moreover, increasing demand for wood resources may lead to increased logging in forested areas, thereby increasing the pressure for retrogressive succession in forest ecosystems (Haberl and Geissler, 2000). Consequently, the importance of accounting monitoring also holds emphasis. An increase in the cascading utilization of wood resources can be a solution to relieve pressure on forest ecosystems.
The supply of domestic wood resources in South Korea is limited. Policy instruments for increasing the carbon stock of harvested wood products can be divided in terms of quantitative and qualitative increases. The means of a quantitative increase include increased logging and increased production of wood products, such as sawn wood and wooden boards. The means of qualitative increases include increased technical efficiency in sawn wood mills, as well as increased recycling of waste wood resources. This qualitative increase enhances the efficiency of the resources used to produce wood products and increases the amount of HWPs relative to the timber supply. The re-use of wood resources also extends the lifetime of HWPs. Optimizing the production process and extending life of HWPs reduce carbon emissions and enhance the carbon stocks stored in the HWP (Lun et al., 2012). Studies in the field of construction emphasize optimizing structural design and resource use to enhance environmental sustainability (; Cucuzza et al., 2024a, 2024b; Domaneschi and Cucuzza, 2023; Kalemi et al., 2024).
Although the government has established plans to cope with climate change through HWP carbon pools, the cascading utilization of wood resources must be guaranteed to achieve these goals. In this context, this study evaluates the emission-reduction potential of HWPs by applying a policy scenario with quantitative and qualitative measures for domestic wood resources in South Korea. Based on the evaluation results, this study offers policy implications for efficiently increasing the amount of carbon stored in HWPs and contributing to efforts to reduce greenhouse gas emissions in South Korea.
2 Scenario analysis
Scenario analysis is a representative strategic prediction method that includes the process of suggesting visions and deriving strategies based on various prospects (KISTEP, 2009). As it is difficult to cope with uncertainty in such situations, research that envisions the future, returns to the present, and suggests preliminary measures for preparing for the future with new insights is necessary.
Scenario analysis is a useful tool for predicting an uncertain future and analyzing its impact. As the effects of introducing environmental policies appear over a long period of time, setting up various scenarios for the effects of various alternative policy measures and estimating these effects using an appropriate method for each scenario is one way to prevent policy failure due to information inconsistency. This could be a solution.
Climate change and sustainable development have normative legitimacy as a global regime, but the means to achieve their goals sometimes conflict. In particular, quantitatively increasing the carbon storage of HWPs inevitably leads to forest degeneration, a decrease in forest carbon, and deterioration of the ecological environment because logging in the forest is inevitable. Under conditions that can bring about synergy, it can be the appropriate means to achieve sustainable development and mitigate climate change. In this context, it is necessary to estimate policy effects through normative scenario design.
Although there are numerous uncertainties in the utilization of domestic wood resources in South Korea, scenario analysis takes a step beyond conclusive future predictions. Consequently, scenario analysis predicts future carbon stocks in HWPs and provides policy information for coping with climate change.
This study assumed a number of wood-utilization scenarios for the entire process of utilizing domestic woods in South Korea and calculated the carbon emission reduction potentials with the goal of efficiently increasing carbon stocks in the HWPs. Figure 1 illustrates the variables used in the wood-utilization scenario, including 1) roundwood production, 2) the percentage of roundwood processed into sawn wood and wooden boards, 3) the percentage of sawn wood and wooden boards utilized as building materials, and 4) the percentage of sawn wood recycled into wooden board materials. Each scenario is defined with distinct setting as depicted in Figure 1.
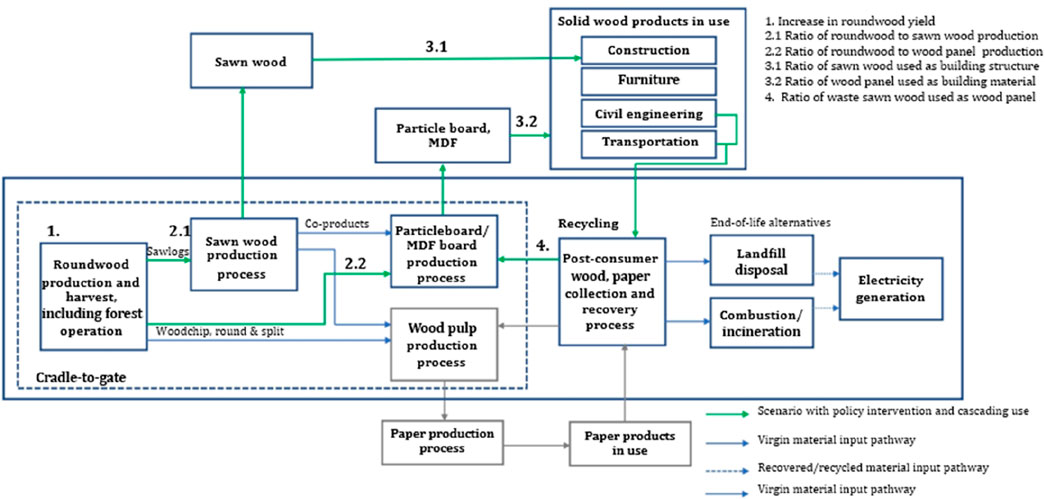
Figure 1. Scope of wood utilization scenarios (Source: revised from Bais-Moleman et al., 2018).
2.1 Scenario 1. change in log production
In accordance with the policy on the self-supply of wood resources from domestic timber, a policy to increase domestic timber production from 4,913,000 m3 in 2015 to 8,618,000 m3 by 2030 in South Korea (Joint Ministry Committee, 2016) applied a scenario for gradually increasing roundwood production by twice as much as the current level. Under this scenario, the roundwood supply would increase by approximately 400,000 m3 annually until 2030. In the first Climate Change Action Plan, the Korea Forest Service established a plan for a phased increase in roundwood production to twice the current production by 2030 (Joint Ministry Committee, 2016).
2.2 Scenario 2. change in percentage of roundwood converted for sawn wood wooden board production
2.2.1 Percentage of roundwood converted for sawn wood production
This scenario reflected the 1st Climate Change Action Plan on increasing the sawn wood production percentage by 10% from 2015 to 2030 (Joint Ministry Committee, 2016).
2.2.2 Percentage of roundwood converted for wooden board production
As of 2020, 13% of roundwoods are utilized for energy use, and there is political support for projects aimed at biofuel production. In terms of cascading the utilization of wood resources in a sustainable way, using it as fuel wood after first using it as a wood product can further contribute to greenhouse gas reduction. To extend the lifetime of wood products by cascading the use of wood resources, the quality of timber must be sufficient to produce high-quality sawn wood. The timber used as fuel is of low grade, and it is difficult to produce sawn wood, which can be used as a long-lived building material with the greatest potential for carbon storage. Generally, wood resources for energy use must have at least one quality that can be used as a raw material for wooden boards. Using low-quality wood as a wooden board material and then using it as a fuel material is one way to increase the amount of carbon storage by extending the lifetime of wood and increasing the carbon efficiency of wood utilization. Therefore, utilizing domestic wood roundwoods as wood products first and then reutilizing them as fuels would contribute to greenhouse gas emissions.
Under this context, this study examined the reduction in greenhouse gas emissions by utilizing 10% of the wood used as an energy source as board material.
2.3 Scenario 3. change in percentage of sawn wood and board used for building materials
Strategies to extend the lifetime include distributing policies that encourage wood products used as long-lived industries, as well as changing the primary types of wood products so that they can be produced for a longer period.
2.3.1 Percentage of sawn wood used for building materials
This scenario increases the percentage of sawn wood used for building materials from 11 to 31 percent. The scenario also assumes that imported wood-based building structural materials can be replaced by domestic sawn wood, based on a preferential domestic sawn wood procurement policy. As the half-life of wood products differ depending on the industry, the half-life of sawn wood varies significantly from 1 year (for civil engineering use) to 100 years (for building structure use) (Eggers, 2002). As sawn wood has the highest durability among wood products, carbon can be stored for a long time when using sawn wood as a building material. To use domestic sawn wood in South Korea as a building material, high-quality wood roundwoods must be supplied. This study considers a proper policy to promote the harvest of timber produced from first-class 30–50 year old forest (KFS, 2018), which can produce sawn wood with sufficient quality for buildings. This is not exactly used for building structural materials, but rather for interior materials.
2.3.2 Percentage of board used for building materials
This scenario sets on increasing the percentage of sawn wood used for building materials from 42 to 62 percent and changing usage patterns from transportation and civil engineering, which have lifetimes of 4 years and 1 year, respectively.
Approximately 99.9% of particleboards produced in Korea use recycled waste wood (KFS, 2018), which is important for the reuse of wood resources in a cascading manner. In South Korea, there were four particleboard manufacturers as of 2015, and one company closed down because of a lack of raw material supply. As of 2020, Korea had three manufacturers: Dongwha, Daesung, and Sungchang. According to interviews with the particleboard manufacturer and the Korea Wood Panel Association, domestic particleboards can replace imported particleboards if the lack of raw material supply is solved and an additional factory is created. Waste wood is divided into pre and post-consumer waste wood. An efficient way to use wood resources is to reuse the pre-consumer waste and use the post-consumer waste wood as fuel. Grade 1 and 2 waste wood in South Korea can be recycled as pre-consumer waste wood and reused in the wood industry to produce particleboards. The recycling of pre-consumer wood waste has a trade-off relationship with the energy industry. In addition, the carbon storage efficiency of HWPs can be enhanced by reusing the domestic sawn wood used in the civil engineering and transportation industries, which has a short lifespan and low economic value. The procurement policy, which prioritizes the purchase of board materials produced from recycled Korean sawn wood, serves as a market-driven incentive, encouraging board manufacturers to utilize sawn wood as a raw material for recycling. Therefore, the scenario suggests the cascading use of wood resources to promote their utilization in building materials based on preferential procurement for wooden board materials produced by recycling sawn wood for additional incentives.
2.4 Scenario 4. change in percentage of sawn wood recycled into board materials
In South Korea, 58% of sawn wood is used for civil engineering and transportation, where it has a short lifespan, as temporary construction materials (ex. scaffolding), sheet piles, molds, transportation boxes, and wood pallets (Jang and Youn, 2021). According to an interview with a board manufacturer, approximately 45%–50% of the waste wood produced by the civil engineering and transportation sectors is used as a fuel material for particleboards. By recycling the sawn wood used in civil engineering and transportation as board materials and extending their lives, the emission of carbon stored in wood can be reduced. While board materials can be technically recycled as wooden board materials up to six times (Korea Plywood Board Association, 2011), this scenario assumes one instance of recycling in consideration of processing costs and other practical matters.
2.5 Scenarios
Scenario A (Increasing the quantitative production of HWPs) was implemented with governmental policies to increase roundwood production and the percentage of timber used for the production of sawn wood to increase the amount of carbon stored in the HWPs. In Scenario A-1, timber production increased by 400,000 m3 annually for 10 years from 2020 to 2030, and the percentage of domestic roundwood used for the production of sawn wood increased from 10% to 20%, with an additional 10% increase (Korea Forest Service, 2018). Under Scenario A-2, the percentage of domestic roundwood used for sawn wood production increased to 30%, which was 10% higher than that under Scenario A-1 (Table 1).
Scenario B (Changing the usage pattern of HWPs to industries with longer service lives) included proper policies to extend lifetime that encouraged wood products to be used in long-lived industries, as well as changing the primary types of wood products so that they can be produced for a longer period. Currently, 58% of sawn wood is utilized in short-lived industries and sawn wood has low carbon efficiency. Scenario B-1 utilized sawn wood as a building material with improved carbon efficiency. Under Scenario B-2, 10% of the domestic roundwood used for energy in Scenario B-1 was additionally used as a raw material to produce wooden boards. Scenario B-2 also set a situation where the imported wooden boards for building materials were replaced with domestic wooden boards in South Korea. Accordingly, 20% of the domestic wooden boards used in non-building industries (ex. civil engineering, or transportation) would be used in the building industry.
Scenario C (Utilizing HWPs through cascading ways) had the optimum cascading use of wood resources. Under this scenario, the distribution of domestic wood resources was controlled by cascading in a more efficient manner in terms of carbon storage effects. Under Scenario C, the domestic sawn wood used for short-lived industries under Scenario B-2 was recycled as wooden board materials. Under Scenario C-1, waste wood from the building industry was recycled as materials of wooden boards, and a 45% recycling ratio was applied to the maximum technically possible recycling percentage from the building sector (Hoglmeier et al., 2013; Bais-Moleman et al., 2018). Scenario C-2 was the same as Scenario C-1, but set the input of domestic roundwood to the same level as the current level. While Scenario C-1 set both qualitative and quantitative improvements, Scenario C-2 set only qualitative improvements without a quantitative increase (Table 1). Scenarios B and C intend to improve the efficiency of the use of domestic wood resources.
To account for the carbon stock of HWPs, this study adopted the formula of Jang and Youn (2021) and used the two approaches of Tier 2 and Tier 31; This study also compared the carbon-reduction potential of HWPs according to the policy scenarios of domestic wood utilization in the early (2030), middle (2050), and late (2080) stages. This study used the Tier 3 method, which is applicable to changes in industrial use and compared the results of Tier 2 and 3.
3 Results
Among the scenarios, Scenario C-1 showed the highest carbon emission reduction potential, with a quantitative increase and optimum cascading use of domestic wood resources. This was twice as much as that under current policy scenario A-1, which quantitatively increased the production of domestic roundwood and sawn wood. Under scenario C-2, the carbon emission reduction potential was 30.3 million tCO2, which was higher than the baseline scenario’s 2030 carbon emission reduction potential of 19.2 million tCO2. This shows that the cascading use of wood resulted in an additional reduction in carbon emissions of 11 million tCO2. Scenario C-2 showed a carbon emission reduction potential of 11 million tCO2 compared with the baseline, which is equivalent to 29% of the target for forest carbon absorption and internationally transferred mitigation in the Korean greenhouse gas reduction goals (38.3 million tCO2). This difference in emissions reduction will increase by approximately 2.3 times in 2080. In addition, Scenario C-2’s carbon emission reduction potentials were similar to that of the current policy scenario A-1 in 2030. In the middle and late stages, Scenario C-2 exhibited a higher carbon emission reduction potential than Scenario A-1. These results emphasize the importance of the cascading use of wood, as well as a quantitative increase in domestic HWPs (Table 2).
Scenario A-1, with a governmental policy to increase domestic timber and sawn wood production, ranked fifth among the six scenarios in the early and middle stages. In the late stage, however, Scenario A-1’s carbon emission reduction potentials were lower than those of Scenario C-2, which used a cascading method of domestic wood resources without an additional quantitative increase in roundwood harvested from forests (Table 2, 3).
Under the scenario that adopted the policy for the preferential procurement of domestic wood products to increase the use of domestic sawn wood and boards as building materials, the carbon emission reduction potentials were higher than the carbon emission reduction potentials of domestic roundwood, and sawn wood production increased by 2.2–8.2 million tCO2. This figure is equivalent to 6%–22% of the target for forest carbon absorption and internationally transferred mitigation (38.3 million tCO2) in the Korean greenhouse gas reduction goals (Tables 2, 3).
The carbon emission reduction potential using the HWP carbon pools showed a greater increase in the later stages (Figure 2). The HWP’s carbon emission-reduction potentials for each scenario were 49.3–90.4 million tCO2 and 58.2 to 118.7 million tCO2 for the middle stage (2050) and late stage (2080), respectively. This figure surpasses the target value of 38.3 million tCO2 for forest carbon absorption and internationally transferred mitigation for the Korean greenhouse gas reduction goals. This shows that HWP carbon pools have huge potential for contributing to greenhouse gas reduction in Korea.
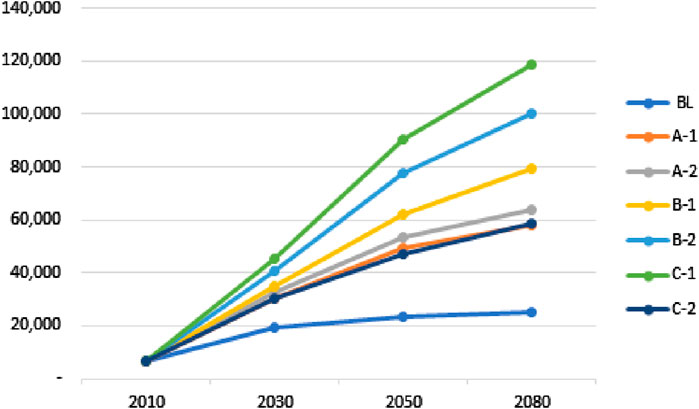
Figure 2. HWP carbon reduction potential graph by policy scenario (Unit: 1,000 tCO2) (source: Jang, 2021).
The existing government policy was established based on the results of the Tier 2 method to contribute to greenhouse gas reduction by increasing the carbon stocks of the HWP. However, as the Tier 2 and Tier 3 methods showed different carbon stock reduction potentials (Table 4), it was necessary to examine the accuracy of Tier 2 method-based policy information.
The Tier 2 method failed to reflect the actual use of wood products in each industry. In particular, Tier 2 did not show qualitative changes that occur when introducing wood products to certain industries and converting short-lived uses to medium and long-lived uses. In contrast, the Tier 3 method measured changes in qualitative wood use efficiency with increased carbon emission reduction potential by cascading the use of wood resources. The difference in the carbon emission reduction potentials for the Tier 2 and Tier 3 methods increased in the later stages. In particular, scenarios A-2 and C-1, applied with the cascading use of wood in long-lived industries, showed a difference in the carbon emission reduction potentials.
4 Discussion
According to the results, the expansion of HWP carbon stocks has great potential to contribute to the achievement of greenhouse emission reduction goals in South Korea. As the HWP is spotlighted as an important instrument for achieving national greenhouse gas reduction goals, policies on the use of domestic wood in South Korea will significantly affect the soundness of the forest ecosystem and the sustainable use of wood resources. Tree growth may take more than 100 years and inconsistent information from such a long growth period may result in incorrect policy directions. Thus, the policy on the expansion of HWP carbon pools should be consistent and continuous. This can be achieved by establishing scientific grounds based on integrated research.
In the wood-use scenario, Scenario B-2, with a 20% increase in the use of wooden boards as building materials (half-life: 16 years), showed carbon emission reduction potentials higher than those under Scenario B-1, with a 30% increase in the use of sawn wood as building structural materials (half-life: 50 years), reaching 6 million tCO2 as of 2030. This is equivalent to 16% of the target value of 38.3 million tCO2 for forest carbon absorption and internationally transferred mitigation in the Korean greenhouse gas reduction goals. This is because the carbon stored in wooden boards accounts for the largest amount of wooden boards used in South Korea; therefore, the absolute input, compared with the percentage, has increased.
Scenario C-2 set the optimum cascading use of domestic wood at the current wood supply level and showed significantly high carbon emission reductions. Meanwhile, the carbon emissions reduction potential of Scenario C-1 was twice that of the scenario with governmental policy on the quantitative increase in domestic timber and sawn wood. These results show that the cascading use of wood resources has great potential to increase carbon stocks in the HWP in South Korea. This is associated with the low carbon storage efficiency of domestic wood in South Korea. Scenario C-1, applied with both a quantitative increase in domestic roundwood and sawn wood and a qualitative increase in the use of wood, showed the highest carbon emission-reduction potential, which corresponds to the results of other studies.
In a scenario analysis of the Irish case, an increase in yield and extended half-life resulted in increased carbon stocks in the HWP (Donlan et al., 2012). A scenario that increases forest yields for a higher supply of wood roundwoods leads to a reduction in carbon in forests. Therefore, a qualitative increase enables sustainable increase in the carbon content of HWPs (Neilson et al., 2008). Under Scenario C-2, which sets the cascading use of wood without a quantitative increase, the carbon emission reduction potentials reached 30.3 million tCO2 by 2030, which is capable of additionally reducing 11 million tCO2 compared with the baseline scenario’s carbon emission reduction potentials of 19.2 million tCO2. This is equivalent to 29% of the target value of 38.3 million tCO2 for forest carbon absorption and internationally transferred mitigation in the Korean greenhouse gas reduction goals. It also shows the high significance of the cascading use of wood, and it shares the same context as research by Lun et al. (2012), who pointed out the extension of lifetime to increase carbon stocks in the HWP without harvesting more wood roundwoods. According to Sharma et al. (2011), carbon stocks can be increased by as much as 4% by extending the lifespan of wood products. Considering these results, the cascading use of wood has a high carbon emission reduction potential owing to inefficient carbon storage in terms of the cascading use of domestic wood resources in South Korea.
The cascading use of wood requires a supply of higher quality wood for forest management and a higher level of wood recycling by industries and consumers. Such innovations can be seen as efforts to enhance the turnover rate of domestic wood resources in South Korea and change the quality and structure of industrial metabolism for ecological modernization. People involved in related fields play an active role (Olsthoorn and Wieczorek, 2006). Industries and wood consumers play important roles in the cascading use of wood.
Large and high-quality roundwoods are planted in forests and supplied as raw materials for sawn wood, which utilizes domestic sawn wood produced from quality roundwoods as building materials and sequentially recycled as raw materials for industrial materials (ex. wooden board materials) and provides market incentives for the consumption sector to push the supply side. The consumption sector motivates the supply sector to encourage cascading use of wood. To support this cascading use of wood, it is necessary to introduce a policy that considers wood resource consumption (EIO, 2012). In addition, it is necessary to develop long-term strategies, such as extended harvest cutting age, rather than existing forestry practices (Pingoud et al., 2010) and to provide sufficient incentives to encourage the participation of mountain owners (Nepal et al., 2012). Furthermore, providing incentives through market attraction policies, such as procurement policies, will create an institutional framework for the continuous cascading use of wood (Little, 2005). In particular, a system that provides incentives for people who utilize carbon market mechanisms, such as forest carbon offset schemes, to supply and use wood products with carbon stocks higher than the baseline will provide a framework for supporting the sustainable use of domestic wood in South Korea.
According to the concept of waste hierarchy, waste wood is applied to different hierarchical levels in terms of recycling (European Commission, 2008). In consideration of this hierarchy, the International Organization for Standardization (ISO) categorized waste into pre and post-consumer materials in the ISO 14021 Environmental Label and Declarations–Self-Declared Environmental Claims (Type II environmental labeling). Based on waste hierarchy, sawn wood free from chemical substances is not regarded as waste. Instead, it is regarded as a resource that can be reused as a raw material without the need for retreatment. On the other hand, waste furniture or wood products treated with antiseptic go through a separate treatment for reuse as wooden boards or fuel woods. Such waste wood is incinerated and buried and is classified as post-consumer waste that requires additional effort before reuse. While such an approach is defined only as a concept, the Act on ‘Promotion of Transition to Circular Economy’ states that “waste resources with high recycling value should be designated as waste resources and excluded from waste regulations.” The arrangement of detailed guidelines for an enforcement decree shall review the application of such matters.
5 Conclusion
At this point of requiring a future policy for forest ecosystems and the use of domestic wood, this study holds significance in providing the necessary information for establishing a forest policy that increases carbon stocks in HWP to contribute to enhancing forest ecological efficiency in terms of climate change mitigation. This would lead to sustainable development with a synergistic effect between the use of wood resources and other ecological services as well as the co-evolution of humans and nature.
To maximize the carbon sequestration potential of harvested wood products in South Korea, we recommend the implementation of targeted policies that promote the cascading use of wood and enhance the lifespan and recycling rates of wood products. These policies should be supported by market incentives and a legislative framework that encourages sustainable forestry practices, supports ecological modernization, and transitions towards a circular economy, ensuring the effective and sustainable use of wood resources in alignment with national greenhouse gas reduction goals.
It is necessary to provide economic incentives for the industry to adopt the concept of staged utilization based on the irreversible characteristics of wood, along with the establishment of a wood utilization tracking system.
This would lead to sustainable development with a synergistic effect between the use of wood resources and other ecological services as well as the co-evolution of humans and nature.
Data availability statement
The raw data supporting the conclusions of this article will be made available by the authors, without undue reservation.
Author contributions
E-KJ: Writing – original draft, Writing – review and editing, Data curation, Methodology, Conceptualization, Project administration, Funding acquisition, Visualization, Software. M-JK: Writing – review and editing, Data curation, Methodology, Project administration, Software. S-MY: Writing – review and editing, Data curation, Methodology, Project administration, Software. C-DE: Writing – review and editing, Conceptualization, Formal Analysis, Project administration, Validation. Y-CY: Writing – original draft, Supervision, Conceptualization, Validation, Funding acquisition.
Funding
The author(s) declare that financial support was received for the research and/or publication of this article. This research was funded by the National Institute of Forest Science, grant project No. FM0200-2023-01-2025. This research was supported by R&D Program for Forest Science Technology, project No. 2020233A00-2021-0001 provided by the Korea Forest Service (Korea Forestry Promotion Institute).
Conflict of interest
The author declares that the research was conducted in the absence of any commercial or financial relationships that could be construed as a potential conflict of interest.
Generative AI statement
The author(s) declare that no Generative AI was used in the creation of this manuscript.
Publisher’s note
All claims expressed in this article are solely those of the authors and do not necessarily represent those of their affiliated organizations, or those of the publisher, the editors and the reviewers. Any product that may be evaluated in this article, or claim that may be made by its manufacturer, is not guaranteed or endorsed by the publisher.
Footnotes
1https://www.mdpi.com/2071-1050/13/120/6737
References
Bais, A. L. S., Lauk, C., Kastner, T., and Erb, K. H. (2015). Global patterns and trends of wood harvest and use between 1990 and 2010. Ecol. Econ. 119, 326–337. doi:10.1016/j.ecolecon.2015.09.011
Bais-Moleman, A. L., Sikkema, R., Vis, M., Reumerman, P., Theurl, M. C., and Erb, K. (2018). Assessing wood use efficiency and greenhouse gas emissions of wood product cascading in the European Union. J. Clean. Prod. 172, 3942–3954. doi:10.1016/j.jclepro.2017.04.153
Bradford, J. B., and D’Amato, A. W. (2011). Recognizing trade-offs in multi-objective land management. Front. Ecol. Environ. 10 (4), 210–216. doi:10.1890/110031
Cucuzza, R., Movahedi Rad, M., di Bari, R., Domaneschi, M., and Marano, G. C. (2024a). A sustainable approach for reversing the structural design process of steel structures: from the traditional minimum-weight approach to the cutting losses minimization. Adv. Transdiscipl. Eng. doi:10.3233/ATDE240578
Cucuzza, R., Rad, M. M., Domaneschi, M., and Marano, G. C. (2024b). Sustainable and cost-effective optimal design of steel structures by minimizing cutting trim losses. Automation Constr. 167, 105724. doi:10.1016/j.autcon.2024.105724
Domaneschi, M., and Cucuzza, R. (2023). Structural resilience through structural health monitoring: a critical review. Data Driven Methods Civ. Struct. Health Monit. Resil. Latest Dev. Appl., 1–13. doi:10.1201/9781003306924-1
Donlan, J., Skog, K., and Byrne, K. A. (2012). Carbon storage in harvested wood products for Ireland 1961-2009. Biomass Bioenergy 46, 731–738. doi:10.1016/j.biombioe.2012.06.018
Eggers, T. (2002). The impacts of manufacturing and utilisation of wood products on the European carbon budget. Eur. For. Insititue Intern. Rep. 2002, 90.
EIO (2012). Methodological Report. Eco-Innovation Observatory. Available online at: http://www.eco-innovation.eu.
European Commission (2008). Directive 2008/98/EC on waste and repealing certain Directives (Waste framework Directive). Off. J. Eur. Union L312 (EN), 3–30.
European Commission (2013). “A new EU Forest Strategy: for forests and the forest based sector,” in In: in the communication from the commission to the European parliament, the council, the European economic and social committee and the committee of the regions (Brussels: COM, 659 final).
Geng, A. X., Yang, H. Q., Chen, J. X., and Hong, Y. X. (2017). Review of carbon storage function of harvested wood products and the potential of wood substitution in greenhouse gas mitigation. For. Policy Econ. 85 (Part 1), 192–200. doi:10.1016/j.forpol.2017.08.007
Haberl, H., and Geissler, S. (2000). Cascade utilization of biomass: strategies for a more efficient use of a scarce resource. Ecol. Eng. 16 (Suppl. 1), 111–121. doi:10.1016/s0925-8574(00)00059-8
Hagemann, N., Gawel, E., Purkus, A., Pannicke, N., and Hauck, J. (2016). Possible futures towards a wood-based bioeconomy: a scenario analysis for Germany. Sustainability 8, 98. doi:10.3390/su8010098
Heinimo, J., and Junginger, M. (2009). Production and trading of biomass for energy – an overview of the global status. Biomass Bioenerg. 33, 1310–1320. doi:10.1016/j.biombioe.2009.05.017
Hoglmeier, K., Weber-Blaschke, G., and Richter, K. (2013). Potentials for cascading of recovered wood from building deconstruction—a case study for south-east Germany. Resour. Conserv. Recycl. 78, 81–91. doi:10.1016/j.resconrec.2013.07.004
Jang, E. (2021). The ecological efficiency of wood resources utilization: focusing on carbon storage effects for climate change mitigation in Korea. Korea: Seoul National University. Ph.D. Thesis.
Jang, E., and Youn, Y. (2021). Effects of wood product utilization on climate change mitigation in South Korea. Sustainability 13 (12), 6737. doi:10.3390/su13126737
Johnston, C. M. T., and Radeloff, V. C. (2019). Global mitigation potential of carbon stored in harvested wood products. PNAS 116 (29), 14526–14531. doi:10.1073/pnas.1904231116
Kalemi, B., Caputo, A. C., Corritore, D., and Paolacci, F. (2024). A probabilistic framework for the estimation of resilience of process plants under Na-Tech seismic events. Bull. Earthq. Eng. 22 (1), 75–106. doi:10.1007/s10518-023-01685-z
Keegan, D., Kretschmer, B., Elbersen, B., and Panoutsou, C. (2013). Cascading use: a systematic approach to biomass beyond the energy sector. Biofuels Bioprod. Bioref. 7, 193–206. doi:10.1002/bbb.1351
KISTEP (2009). Scenario analysis and system construction plan for future forecasting. Eumseong, Republic of Korea: Korea Institute of S&T Evaluation and Planning. Available online at: https://www.kistep.re.kr/board.es?mid=a10306070000&bid=0031&b_list=10&act=view&list_no=35058&nPage=88&keyField=&orderby= (Accessed March 11, 2025).
Korea Plywood Board Association (2011). LCA and cost-benefit analysis for each recycling method of recycled timber resources. Seoul, Korea.
Little, A. D. (2005). “Study for the conception of a programme to increase material efficiency,” in Small and medium sized enterprises (Wuppertal, Germany: Wuppertal Institute).
Lun, F., Li, W., and Liu, Y. (2012). Complete forest carbon cycle and budget in China 1999-2008. For. Ecol. Manag. 264, 81–89. doi:10.1016/j.foreco.2011.10.004
Min, K. T., Byun, S. Y., Jang, E. K., and Ryu, K. S. (2019). Study on the domestic wood market and the impact analysis of prioritizing domestic wood purchases. Naju, Republic of Korea: Korea Institute of S&T Evaluation and Planning. Available online at: https://repository.krei.re.kr/handle/2018.oak/24682 (Accessed September 19, 2024).
Neilson, E. T., MacLean, D. A., Meng, F. R., Hennigar, C. R., and Arp, P. A. (2008). Optimal on- and off-site forest carbon sequestration under existing timber supply constraints in northern New Brunswick. Can. J. For. Res. 38 (11), 2784–2796. doi:10.1139/X08-120
Nepal, P., Grala, R. K., and Grebner, D. L. (2012). Financial feasibility of increasing carbon sequestration in harvested wood products in Mississippi. For. Policy Econ. 14 (1), 99–106. doi:10.1016/j.forpol.2011.08.005
Olsthoorn, X., and Wieczorek, A. (2006). Understanding industrial transformation. Views from different disciplines. Dordrecht, Netherlands: Springer.
Petersen, A. K., and Solberg, B. (2005). Environmental and economic impacts of substitution between wood products and alternative materials: a review of micro-level analyses from Norway and Sweden. Policy Econ. 7 (3), 249–259. doi:10.1016/s1389-9341(03)00063-7
Pingoud, K., Pohjola, J., and Valsta, L. (2010). Assessing the integrated climatic impacts of forestry and wood products. SILVA Fenn. 44 (1), 155–175. doi:10.14214/sf.166
Profft, I., Mund, M., Weber, G. E., Weller, E., and Schulze, E. D. (2009). Forest management and carbon sequestration in wood products. Eur. J. For. Res. 128, pages399–413. doi:10.1007/s10342-009-0283-5
Sharma, B. D., Wang, J., and Liu, S. (2011). Modeling of sustainable biomass utilization and carbon emission reduction. Sens. Lett. 9 (3), 1175–1179. doi:10.1166/sl.2011.1366
Sikkema, R., Dallemand, J. F., Matos, C. T., van der Velde, M., and San-Miguel-Ayanz, J. (2016). How can sustainable and efficient wood-sourcing practices support the ambitious goals for the EU’s future bioeconomy? Scand. J. For. Res. 32, 551–558. doi:10.1080/02827581.2016.1240228
Keywords: harvested wood products (HWPs), carbon reduction potential, domestic wood utilization, cascading use, national greenhouse gas reduction
Citation: Jang E-K, Kim M-J, Yoon S-M, Eom C-D and Youn Y-C (2025) Climate change mitigation potential of cascading utilization of wood resources. Front. Built Environ. 11:1490984. doi: 10.3389/fbuil.2025.1490984
Received: 13 November 2024; Accepted: 18 February 2025;
Published: 16 April 2025.
Edited by:
Krushna Mahapatra, Linnaeus University, SwedenReviewed by:
Josef Křeček, Czech Technical University in Prague, CzechiaRaffaele Cucuzza, Polytechnic University of Turin, Italy
Copyright © 2025 Jang, Kim, Yoon, Eom and Youn. This is an open-access article distributed under the terms of the Creative Commons Attribution License (CC BY). The use, distribution or reproduction in other forums is permitted, provided the original author(s) and the copyright owner(s) are credited and that the original publication in this journal is cited, in accordance with accepted academic practice. No use, distribution or reproduction is permitted which does not comply with these terms.
*Correspondence: Yeo-Chang Youn, youn@snu.ac.kr