- Department of Built Environment and Energy Technology, Faculty of Technology, Linnaeus University, Växjö, Sweden
The construction sector is a major consumer of natural resources and a significant contributor to environmental degradation through resource depletion, waste generation, and greenhouse gas emissions. As the industry shifts toward sustainable and circular practices, improving material traceability and resource efficiency becomes crucial. Digital Tracking Technologies (DTT) offer a promising solution, yet their adoption in the precast concrete sector remains limited, necessitating an in-depth examination of influencing factors. This study explores DTT adoption in Sweden’s precast concrete industry, addressing a critical gap in understanding how digital technologies support Circular Economy (CE) objectives. By identifying key barriers and drivers, the research provides a holistic view of the challenges and opportunities shaping this transition. A mixed-methods approach was employed, integrating bibliometric analysis, social media sentiment analysis, and stakeholder interviews. Data analysis was conducted using VOS Viewer, Orange Data Miner, and NVivo, while findings were interpreted through a sociotechnical lens. The study utilizes PESTLE and SWOT analyses within the Multi-Level Perspective (MLP) framework to examine dynamics at the niche, regime, and landscape levels. Findings highlight systemic barriers such as high investment costs, fragmented infrastructure, limited knowledge, and cultural resistance. However, opportunities exist, including enhanced operational efficiency, improved information dissemination, CE alignment, supportive policies, and targeted incentives. Innovations like RFID tags, QR codes, and Digital Product Passports can enhance material traceability and circularity. Given regime inertia and landscape pressures, cohesive policy interventions and industry collaboration are essential. This study provides actionable insights for industry stakeholders, policymakers, and sustainability advocates to advance digital innovation and sustainable construction practices.
1 Introduction
The construction sector is one of the largest global consumers of natural resources, significantly contributing to environmental degradation through resource depletion, waste generation, and greenhouse gas emissions (Bonoli et al., 2021; Fei et al., 2021). This is rooted in the linear economy (LE) model of the sector, which operates on a “take-make-dispose” approach (Ellen MacArthur Foundation, 2015; Oberle et al., 2019). Thus, transitioning to a Circular Economy (CE) framework, which emphasizes material reuse, repair, and recycling, has become imperative in mitigating these adverse impacts (Ellen MacArthur Foundation, n. d). This shift not only promotes sustainable resource use but also minimizes environmental impact, improves waste management practices, and advances several United Nations Sustainable Development Goals (SDGs) (Ellen MacArthur Foundation, 2015; Eurostat, 2023; European Environmental Agency, 2024).
Sweden, a global leader in climate neutrality, has made considerable strides in reducing greenhouse gas emissions and fostering sustainable urban development (RE:Source, 2022). However, the construction sector continues to pose major environmental challenges. Accounting for 125 million tonnes or ca 47% of the country’s material consumption and generating approximately 14 million tonnes of waste annually, this sector is the second largest waste producer after the mining industry (Zakrisson, 2018; RE:Source, 2022). This highlights the urgent need for the Swedish construction sector to shift from a linear to a circular model to help close the nation’s Circularity Gap and support its ambitious sustainability targets (RE:Source, 2022).
Concrete is a dominant construction material, which is valued for its durability and strength (Babor et al., 2009). However, its highly resource-intensive production process contributes significantly to CO2 emissions. Cement alone is responsible for nearly 8% of global CO2 emissions (Andrew, 2018; Habert et al., 2020; Statista, 2023). Consequently, these environmental impacts pressure the industry to explore lower-carbon, traceability, and circularity-focused alternatives (Habert et al., 2020; Nilimaa, 2023). One such alternative is the pre-casting of concrete in controlled environments, which aims to mitigate some of the environmental challenges (Wong and Loo, 2022). However, it also presents unique operational, logistical, and storage challenges as it heavily relies on modular components that require precise tracking from production to installation (Wong and Loo, 2022; Liu and Zainul Abidin, 2024).
The shift toward circularity in construction is emphasized in the European Parliament’s harmonized rules for marketing construction products (European Union, 2023). This regulation stresses the importance of sustainable practices, aiming to reduce environmental impact by promoting resource efficiency and lifecycle management of construction materials. Given this, digital innovation has emerged as a crucial enabler of transformation within the construction sector (UNEP, 2023). Initial tools like Computer-Aided Design (CAD) laid the groundwork for this digital shift (Javaid et al., 2022), which has evolved to include advanced systems such as Building Information Modelling (BIM) (Biswas et al., 2024), the Internet of Things (IoT) (Giovanardi et al., 2022; Shishehgarkhan et al., 2024), and real-time tracking technologies that improve material flow and asset management (Zhao et al., 2017; Daniotti et al., 2022). A key advancement within the BIM framework is the integration of digital twins, which streamline construction management and maintenance processes, ultimately enhancing operational efficiency (Nguyen and Adhikari, 2023; Jiang et al., 2024). This evolution aligns with the Green Deal and the Circular Economy Action Plan (CEAP) of the European Union. These initiatives not only advocate for resource efficiency and emissions reduction, but also introduce the Digital Product Passport (DPP) as a transformative mechanism to ensure traceability, transparency, and material reuse across industries (European Commission, 2020; 2021).
Recent studies have highlighted the emergence of Digital Tracking Technologies (DTT) to facilitate circular construction practices by enhancing traceability, transparency, and compliance, while enabling data-driven decision-making (Camodeca and Almici, 2021; Del Rio et al., 2021; Davari et al., 2023). Despite the potential benefit of these technologies, their broader implementation globally has been slow (ECSO, 2021; Wuni, 2022; Dervishaj et al., 2023; Davari et al., 2023; Sepasgozar et al., 2023; Giovanardi, 2024) due to technical constraints, financial limitations, cultural resistance, and a shortage of skilled expertise (Gerhardsson et al., 2020; Lobo et al., 2021; Kaufman et al., 2022; Thirumal et al., 2024). Sweden has an advanced digital infrastructure to achieve various sustainable development goals (Business Sweden, 2022; RE:Source, 2022; UNEP, 2023), but still the adoption of DTT in the construction sector is slow.
This study examines the adoption of DTT in Sweden’s precast concrete sector using a mixed-method approach to examine both macro-environmental factors and stakeholder perspectives. By combining bibliometric analysis, social media sentiment analysis, and expert interviews to capture the trends, barriers, and opportunities related to DTT adoption, the study offers a holistic understanding of the dynamics driving DTT adoption in the context of CE practices. To further contextualize the findings, the study applies the socio-technical transition (STT) framework. A socio-technical system involving diverse stakeholders, institutions, and technologies faces unique challenges in adapting to change due to the long lifespan of buildings and decentralized decision-making (Gerhardsson et al., 2020; Vogel, 2020; Kaufman et al., 2022). Digital transformation in this context requires more than mere technological adoption; it demands a culture of continuous change, exploration, experimentation, and the strategic use of emerging tools to drive sustainability (Herbert, 2017). Social acceptance of digital tools and shifting attitudes toward technology, influence user interactions and propel the evolution of STT (Graf-Vlachy et al., 2018).
The Multi-Level Perspective (MLP) is a widely used framework in sociotechnical transition studies, which illustrates the dynamic relationships between niche innovations (e.g., DTT), stable societal practices and regulatory structures (regimes), and the contextual factors (landscapes) (Geels, 2002; 2011). These transitions involve changes in technology, user practices, infrastructure, regulatory frameworks, and symbolic meanings (Geels, 2002; Schot and Geels, 2008). Previous research has applied MLP to sustainability transitions in energy, low-carbon initiatives, and governance (Li, 2020; Kanger, 2021; Jayaraj et al., 2024). However, studies focusing on transitions in the construction industry, particularly in the precast concrete sector, remain limited. While several frameworks and strategies exist to guide digitalization and CE transitions within the EU, including the Digital Product Passport (DPP) and various BIM-based solutions, a comprehensive analytical framework integrating both PESTLE and SWOT analyses in the Swedish precast concrete sector has yet to be established. Most existing studies on digitalization of the construction sector focus on the technical aspects of individual technologies, such as BIM, IoT, RFID, and blockchain, while overlooking the broader socio-technical dynamics that consider macro-environmental influences and stakeholder interactions (Teisserenc and Sepasgozar, 2021; Giovanardi et al., 2022; Dervishaj et al., 2023; Dervishaj and Gudmundsson, 2024; Giovanardi, 2024). This narrow focus creates a significant knowledge gap in the role of socio-technical interactions in the transition to digitalization of the construction sector, especially the precast concrete sector. To address these research gaps, this study applies the PESTLE-SWOT approach in conjunction with the MLP framework as a foundation to provide a holistic understanding of the trends, opinions, and dynamics influencing the adoption of DTT in the Swedish precast concrete sector.
2 Materials and methods
The study adopted a deductive approach, which emphasizes the progression from theoretical foundations to empirical validation (Bryman and Bell, 2015). The MLP framework served as the theoretical framework, providing a structured approach to examining socio-technical interactions across niche, regime, and landscape levels. The dynamics of the sociotechnical interactions were analyzed through PESTLE (Political, Economic, Social, Technological, Legal, and Environmental) and SWOT (Strengths, Weaknesses, Opportunities, and Threats) frameworks. These methods have been previously applied in studies, such as assessing sustainability in adaptive reuse projects (Vardopoulos et al., 2021) and analyzing strategies for the deep renovation of detached housing markets (Mainali et al., 2021). PESTLE identified macro-environmental factors, such as regulatory, technological, and societal pressures, while SWOT examined internal and external dynamics (Gurel and Tat, 2017; Rastogi and Trivedi, 2022). This integrated, multi-layered methodology enabled a robust examination of systemic drivers and barriers within the Swedish precast concrete sector, while also capturing broader trends and challenges across the construction industry.
A mixed-method approach, integrating both quantitative and qualitative data, facilitates a comprehensive analysis of a research problem (Creswell, 2014). This method was organized into three steps: bibliometric analysis identified research trends and clusters, social media analysis provided insights into industry attitudes and public discourse, while expert interviews offered in-depth perspectives from key stakeholders.
2.1 Bibliometric analysis
The bibliometric analysis aimed to uncover existing barriers and opportunities linked to the adoption of Digital Technologies (DT) by examining academic abstracts sourced from Scopus and Web of Science databases. The following search string was utilized to capture relevant publications: “(“circular economy” AND (digit* OR “industry 4.0 OR passports) AND (barrier OR challenge OR problems OR limit* OR opportunities OR benefits OR possibilities) AND (construction* OR concrete* OR “built environment”)).” From the initial search, 168 articles were identified in Scopus, and 83 in Web of Science. These results were refined based on publication year (2010–2024) and relevance to identifying barriers and opportunities in adopting DT for circularity in the construction sector. After applying this criteria, 31 articles from Scopus and 10 from Web of Science were selected. The dataset was further refined by merging both sources and removing 15 duplicates, resulting in a core set of 26 unique articles. To ensure a focus on peer-reviewed academic and industry-relevant research, only journal papers and conference proceedings were included in the final selection. A snowballing technique by reviewing references from the selected articles was applied to expand the dataset, which led to the inclusion of 31 additional sources, resulting in a final dataset of 57 articles. These were then exported in RIS format and analyzed using a VOS viewer to generate a keyword co-occurrence map. This map provided visual insights into key themes and clusters, facilitating a nuanced understanding of the interaction between DT and CE in construction. Each identified cluster was interpreted and mapped to the corresponding MLP level in the STT, offering a foundational insight into trends, drivers, and barriers surrounding DT adoption in the broader construction industry.
2.2 Social media analysis
Social media posts from Facebook, X (formerly Twitter), and LinkedIn were scraped to assess sentiments toward DT adoption for CE in the construction industry. The majority of the authors of these posts were professionals and industry practitioners, all within the construction industry, with only a minimal number of contributions from academic researchers. Similar to Tsironis et al. (2024), who scraped LinkedIn posts to identify common CE practices, this study collected posts using targeted search phrases, such as “Digital technologies + Circular Economy + Construction,” “Digitalisering + Byggbranschen + Spårbarhet,” “Digitalisering Byggbranschen för Cirkulär ekonomi,” and “#Digitalisering #Byggbranschen.” Orange Data Miner, an open-source software created for interactive data visualization, machine learning, and data analysis (Demšar and Zupan, 2013; Ljubljana, no date) was used for the analysis. While its application for data analysis in engineering studies remains scarce, Orange has been widely utilized in computing, geoinformatics, and social sciences (Tiwari et al., 2023; Dobesova, 2024). Following a manual data cleaning, a structured text analysis workflow (illustrated in Figure 1) was applied to analyze a total of 241 social media posts. The process began with importing the text dataset into the corpus module, where the raw text was preprocessed to remove inconsistencies and improve readability. Next, the preprocessed text was passed into the Sentiment Analysis module, which evaluated the emotional tone of the posts and categorized them as positive, neutral, or negative (Dang and Ahmad, 2015; Tang and Feng, 2019; NLTK Sentiment Analysis Tutorial: Text Mining & Analysis in Python, no date). The model assessed the text polarity using a range from −1.0 (negative) to 1.0 (positive). To visualize key patterns, the data was further analyzed using multiple modules in the Data Miner workflow:
• A Word Cloud (Figure 3) was generated to highlight the most frequently occurring terms.
• A Sentiment heat map (Figure 4) provided a structured representation of sentiment distribution across different categories.
• A Data Table enabled further inspection of textual data, allowing for group-based and categorical analysis. While the Corpus Viewer facilitated manual exploration of the processed text data.
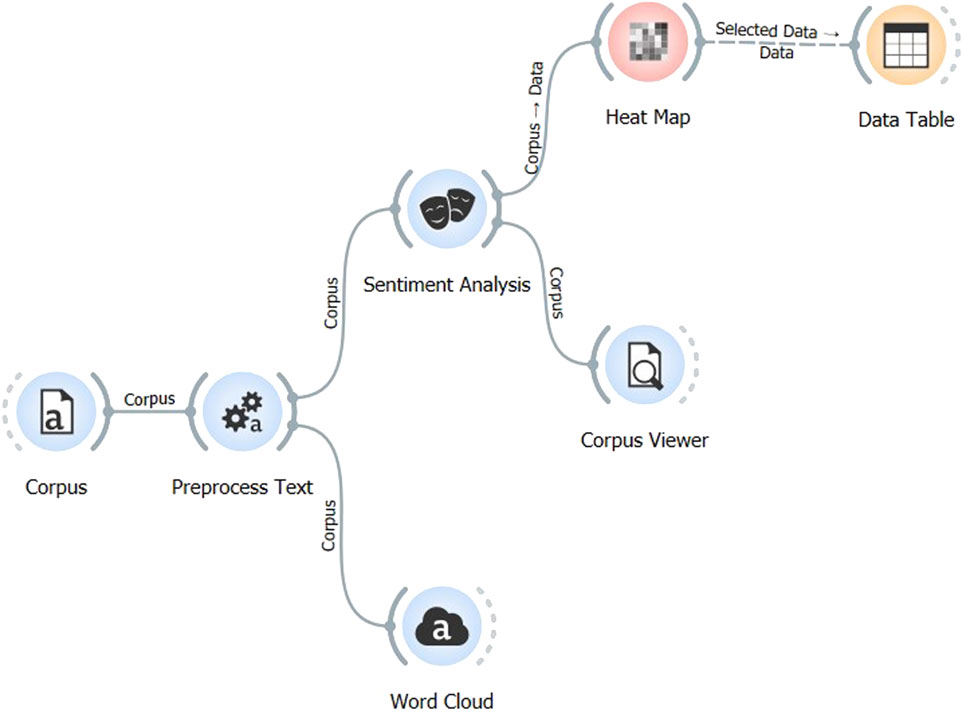
Figure 1. Workflow diagram illustrating the text analytics process used for social media analysis with the Orange Data Mining software.
These visualizations helped capture sentiment trends, identify discussion themes, and highlight key topics relevant to DT adoption for circularity in construction. This analysis also supported the MLP framework, linking positive sentiments to potential opportunities or drivers and negative sentiments to perceived barriers in the adoption of DTs.
2.3 Stakeholder interviews
Semi-structured interview has flexibility to explore a specific topic in detail (Adams, 2015), while purposive sampling is appropriate to obtain in-depth insights from participants with relevant expertise, ensuring a comprehensive understanding of the research problem (Bryman and Bell, 2015). Therefore, a purposive sample of 10 participants was chosen, following the guidance of Galvin (2015), who recommends a sample size of six to nine participants for key reliable results. Participants were chosen for their expertise in DT, DTT adoption, and CE practices in Sweden’s precast concrete sector. This included heads of three precast concrete manufacturing companies, three technology providers, one architect, one BIM expert, and two CE and digitalization experts. All participants were briefed on research objectives, data handling, and confidentiality measures. This approach ensured the collection of rich, relevant data that complements broader analyses. A thematic analysis of the interview data was conducted using NVivo software. This step involved systematically coding the responses, identifying key themes related to the barriers and drivers of DTT adoption, and interpreting these themes in the context of CE practices. These coded themes were exported to the Python programming IDE to generate a heatmap. The analysis followed the six-phase process as outlined by Braun and Clarke (2012), ensuring a rigorous approach. The thematic analysis specifically focused on the PESTLE factors influencing DTT adoption in the precast concrete sector. This process was done to critically understand the external and internal sociotechnical dynamics influencing the adoption.
2.4 Data triangulation and merging strategy
To ensure the validity and reliability of the findings, data and methodological triangulation as established by Jick, (1979) were applied. This process integrated insights from all three steps to identify key themes (i.e., the barriers and drivers), and effectively mapped them across PESTLE, SWOT, and MLP frameworks. This triangulated approach provided a comprehensive, multi-layered understanding of the research problem, supporting well-grounded conclusions and actionable recommendations for advancing DTT adoption and CE practices.
3 Results
This section presents and discusses the findings from the multi-method analysis from bibliometric analysis (keyword co-occurrence map), social media analysis, and interview insights.
3.1 Key themes from bibliometric analysis
The bibliometric analysis with the aid of VOS viewer generated a keyword co-occurrence map (Figure 2), which provides a structured overview of interconnected themes related to the barriers and opportunities in adopting DT for CE in the construction sector. Given the limited literature specifically addressing DTT in this area, the analysis was expanded to include broader discussions on DT within the construction sector. The analysis identified six interconnected clusters; each assigned a title reflecting their central theme (as illustrated by circles in the figure). These themes are critically analyzed below in relation to the research objective and interpreted through the different MLP levels. The interconnectedness of these clusters reflects similarities in the work of the corresponding authors and the systemic nature of challenges and solutions, emphasizing the need for integrated approaches to address CE objectives.
3.1.1 Digitalization as an enabler of circular economy (CE)
This cluster highlights keywords such as “digitalisation,” “waste management,” “building industry,” and “blockchain technology.” A study by Rodrigo et al. (2023) discusses how these DTs streamline resource optimization by addressing inefficiencies, while Çetin et al. (2022) identify DTs like digital twins and scanning technologies as key drivers for sustainable construction practices. Furthermore, Teisserenc and Sepasgozar (2021) emphasizes the importance of digitalization in material tracking, waste management, precise data management, and lifecycle analysis. Few studies by Trevisan et al. (2023) and Munaro and Tavares (2023) illustrate how DTs overcome systemic barriers such as inefficiencies in construction workflows, while Honic et al. (2019) argue that integrated digital solutions foster stakeholder collaboration and advance broader CE objectives. Collectively, these tools contribute to landscape, regime, and niche-level transformations. Digitalization is both a transformative force and an integral part of an evolving industry, reshaping norms, opening new avenues for CE, and supporting sustainable goals within the prefab concrete and construction sector. However, systemic incentives remain necessary to bridge the gap between potential and actual implementation (Munaro and Tavares, 2021).
3.1.2 Data and information management
Effective data management is necessary for successfully adopting DT in construction (Honic et al., 2019; Bellini and Bang, 2022). Keywords like “data,” “information,” “BIM,” and “material passport” emphasize the criticality of structured data systems for enabling CE (Kedir et al., 2021; Q; Liu et al., 2022). Accurate tracking of materials from production to end-of-life and material passports enhances traceability, reusability, and recyclability of construction materials (Kedir et al., 2021; Munaro and Tavares, 2021; Mêda et al., 2023). However, the fragmented integration of such tools (Honic et al., 2019) as well as data interoperability issues and inconsistent data-sharing practices (Bellini and Bang, 2022) limit cross-stakeholder collaboration, which hinders the broader adoption of DT and seamless CE practices. Reflecting the regime and niche level of the MLP, this cluster underscores the need for industry-wide standards and consistent practices to unlock the full potential of structured data systems for CE.
3.1.3 Transition to circular economy (CE) and barriers to digital technology integration
The transition from linear to circular business models represents a paradigm shift, requiring the adoption of innovative business models, restructured workflows, and enhanced digital infrastructures. However, significant challenges including data management, limited access to reliable data (Bellini and Bang, 2022), and integrating DT into construction practices persist (Munaro and Tavares, 2021). Keywords such as “transition,” “circular economy,” “digital technologies,” and “role” in the transition cluster (dark blue) along with “infrastructure,” “understanding,” “production,” and “sector” in the challenges’ cluster (red) highlight the reconfiguration of norms and processes alongside barriers such as infrastructure deficits, high costs, and interoperability issues. Overcoming the challenges of a shift from the entrenched linear models to circular models requires institutional flexibility and governance frameworks to incentivize collaboration (Munaro and Tavares, 2021). The lack of cohesive policy alignment across regions often leads to piecemeal efforts, and therefore there is a need for an integrated policy environment to overcome such regulatory barriers and drive systemic change (Adriana Hofmann Trevisan et al., 2023). While Liu et al. (2022) proposed a framework identifying critical digital functions for circularity, achieving scalability remains challenging. This cluster highlights that resistance to DT adoption stems from entrenched practices and structures at the regime, requiring significant investments in infrastructure, training, and policy integration at the landscape level to overcome these barriers.
3.1.4 Digital technologies for tracking and waste management
Focusing on material lifecycle tracking and waste management, the yellow and orange clusters align closely with the core principles of CE, with keywords like “QR codes,” “IoT,” and “RFID.” Dervishaj et al. (2023) explored the integration of tracking technologies with BIM to support reuse strategies for prefabricated concrete components. Additionally, Jiang et al. (2023) emphasize blockchain’s potential for creating secure and traceable material flows, though technological complexity and cost barriers hinder widespread adoption. Although Uçar et al. (2020) identified IoT-enabled systems that facilitate efficient waste segregation and recycling, yet these innovations are not widely adopted across the industry for CE practices and face regulatory and industry uptake challenges. Coordinated efforts are essential to scale these technologies and integrate them into mainstream waste management practices, as they hold significant potential for supporting circular practices by improving material traceability and accountability.
3.1.5 Traceability and transparency for circular economy
With circular economy as the central focus, terms such as “traceability,” “transparency,” and “sustainability” indicate the importance of robust frameworks to verify material flows and ensure accountability for achieving CE across the entire supply chain. Studies such as Rahel Kebede et al. (2023) present a framework for implementing Digital Product Passports (DPP) for improved and detailed data sharing and tracking of material flows, ultimately for transparency purposes. However, Katenbayeva et al. (2016) highlight that achieving transparency requires addressing significant data accessibility and privacy concerns. This cluster can be positioned between niche innovations and regime levels as they are emerging innovations in the digital space. This reveals a paradox: while traceability tools are critical for CE, their effectiveness depends on achieving industry-wide data-sharing agreements, often constrained by competitive industry and regulatory barriers.
3.1.6 Strategy and future research in the circular economy
This cluster highlights the importance of strategic initiatives and future research to address gaps in CE implementation. Keywords such as “circular economy strategy,” “ future research,” and “digital function” reflect the ongoing quest for clear pathways and actionable solutions. Benachio et al. (2020) call for localized material banks and digital inventories to support CE practices. Munaro and Tavares (2021) emphasize aligning policy and research agendas to facilitate the transition to circular models. Furthermore, Teisserenc and Sepasgozar (2021) note that cross-disciplinary collaboration is often lacking, limiting the scalability of strategic efforts. This cluster stresses the need for bridging research and practice to ensure that strategic initiatives result in tangible outcomes, aligning with the transition to circularity.
3.2 Social media analysis
3.2.1 Key themes identified from social media discussions
The word cloud visualization, as shown in Figure 3, highlights key topics frequently discussed concerning DT within the construction industry, with prominent words like “circular economy,” “sustainability,” “digital technologies,” and “construction” indicating a clear focus on aligning DT with circular economy principles. These terms reflect broader societal pressures and shifts toward sustainability and resource conservation, which drive DT adoption. Meanwhile, words like “materials,” “management,” “efficiency,” and “energy” point to specific operational areas where DT could enhance resource efficiency and traceability, aligning with established industry practices. Additionally, terms such as “innovation,” “environmental,” “industry,” and “future” suggest that stakeholders see DT as a forward-looking approach to modernizing the construction sector. The presence of these terms highlights both niche innovations and the integration of DT within existing industry norms. However, the appearance of words like “challenges” and “critical” indicates that, while there is substantial interest in digital transformation, significant perceived obstacles remain, reflecting the barriers that need to be overcome for DT adoption to gain traction across the broader construction industry.
3.2.2 Sentiment distribution on social media: Insights from heatmap and bar chart
The heatmap in Figure 4 visualizes the intensity and distribution of sentiments across social media posts on DT adoption in the construction industry. The first calibrated line aggregates all posts, while the first three labeled rows break down the presence of positive (row 1), neutral (row 2), and negative (row 3) sentiments within individual posts. The final row, labeled “compound,” represents the overall sentiment of each post, ranging from strongly negative (−0.8053, blue) to neutral (0, white) and highly positive (1, red). This structure allows for a nuanced understanding of how sentiment varies across discussions, with the compound score providing a conclusive measure of each post’s prevailing tone. These insights are further supported by specific excerpts from social media posts, which illustrate the sentiment categories in more detail:
3.2.2.1 Positive sentiment (151 occurrences)
Most posts reflect a positive sentiment, indicating optimism about the potential of DT generally to drive circularity. For instance, one post states:
“The potential of Building Information Modelling (BIM) and digital twin technologies in the construction industry highlights the benefits of using BIM and digital twins, such as improved collaboration, increased efficiency, enhanced stakeholder engagement, and sustainability through the application of circular economy principles.”
This post is categorized as positive due to its emphasis on the benefits of DT, such as improved collaboration, increased efficiency, and sustainability. Keywords like “improved,” “increased,” “enhanced,” and “sustainability” contribute to the positive sentiment score, illustrating that social media users see DT as an enabler of better practices and sustainable outcomes in the industry.
3.2.2.2 Neutral sentiment (77 occurrences)
A considerable portion of posts falls into the neutral category, suggesting that while there is interest in DT, many discussions are still exploratory or cautious. A representative excerpt includes:
“I have been guilty of some sweeping reasoning about how the construction industry is far behind the manufacturing industry in digitalization. But Y has a more nuanced view, and I learned a lot during our conversation. Go in and listen.”
This post reflects a neutral sentiment because it does not explicitly endorse or criticize DT. Instead, it suggests an evolving understanding and a balanced view. The absence of strong positive or negative words aligns with the sentiment score, indicating an objective or observational tone. This neutrality could suggest that many stakeholders are still in the information-gathering phase regarding DTT.
3.2.2.3 Negative sentiment (13 occurrences)
A smaller proportion of posts express negative sentiment, highlighting specific challenges or criticisms related to DT adoption. An example of such a post reads:
“We identify challenges in retrofitting digital technologies to existing structures and emphasize the need for a unified digital transformation strategy and increased cross-industry collaboration.”
This post is categorized as negative due to phrases like “identify challenges” and “need for a unified strategy,” which underscore the difficulties and strategic gaps in adopting DTT. Words like “challenges” and “need” contribute to negative sentiment, reflecting concerns over integration difficulties and the lack of cohesive digital strategies.
3.3 Stakeholder interview
The content analysis of the stakeholder interview is presented in the form of a heatmap in Figure 5. It visualizes the frequency of mentions (from 10 stakeholders) for both barriers and drivers of DTT within the context of circularity in Sweden’s prefabricated concrete sector. A deeper colour represents a higher frequency of responses. Each barrier and driver are categorized according to the PESTLE framework, allowing for a structured analysis of the factors influencing DTT adoption. These PESTLE categories will be further examined to assess the implications of each factor, with insights triangulated against findings from previous analyses to create a comprehensive understanding of the sector’s challenges and enablers for achieving a CE. Several key observations emerge from the heatmap analysis:
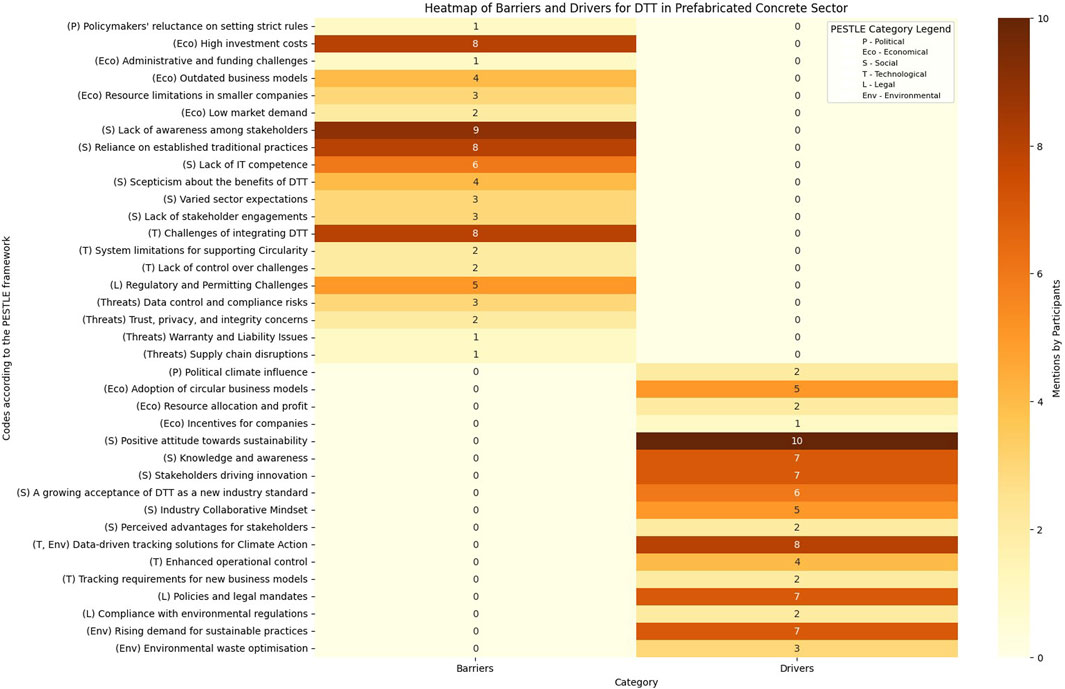
Figure 5. Heatmap of barriers and drivers identified through thematic analysis of stakeholder interviews.
3.3.1 High-Frequency Barriers
Among the most frequently mentioned barriers are limited stakeholder awareness (9 mentions), reliance on established traditional practices (8 mentions), difficulties in integrating DTT (8 mentions), high investment costs (9 mentions), and lack of IT competence (6 mentions). These factors suggest that, despite recognition of DTT’s potential, resistance from conventional structures and limited awareness serve as substantial impediments to progress.
3.3.2 High-Frequency Drivers
Conversely, a positive attitude towards sustainability (10 mentions), the data-driven nature of DTT, knowledge/awareness, and rising demand for sustainable practices stand out as major drivers. These opinions reflect a growing shift toward sustainable practices, highlighting a crucial motivational factor for adopting digital solutions within the sector.
4 Discussion
4.1 PESTLE analysis
4.1.1 Political and legal factors
The regulatory environment and policy frameworks play a critical role in driving the adoption of DTT. Interviews revealed that policymakers significantly shape this landscape, with initiatives like the EU Green Deal promoting innovation and enforcing environmental compliance standards. However, regulatory and permitting challenges remain formidable barriers to DTT adoption. Given DTT’s intersection with compliance-heavy sectors, such as the precast concrete industry, companies face stringent regulatory requirements that complicate implementation. One participant noted, “Meeting regulatory requirements often complicates the DTT process; however, we recognize that compliance is also a motivator in aligning with sustainable practices.” This dual role of regulations indicates their complexity: while they pose hurdles, they also create structured frameworks encouraging sustainable practices. Legal compliance, therefore, serves as both a barrier and a driver, pushing companies toward responsible adoption of DTT. Additional challenges and threats include trust and privacy concerns, data control issues, warranty and liability uncertainties, and policymakers’ hesitance to enforce stricter regulations. These factors, compounded by unclear guidance on material tracking, hinder progress. Studies, such as Thirumal et al. (2024) and Trevisan et al. (2023) identified from the bibliometric analysis, have highlighted a lack of cohesive policy alignment and inadequate management support as significant obstacles to adopting innovations for CE, such as DTT. These insights highlight the need for clearer regulations and streamlined permitting processes, as well as the need for institutional and governmental incentives to drive systemic change (Munaro and Tavares, 2021). Social media discussions also reflect the political dynamics at play. Terms such as “strategy,” “Sweden,” and “European,” which appeared prominently in word cloud analyses, emphasize the influence of national and regional strategies on CE initiatives. While regulations and building codes like the EU Energy Performance Directive (EPBD), Swedish Building Code (BBR), and the Construction Products Regulation (CPR) encourage resource management, they fall short of mandating digital material tracking. This regulatory limitation constrains the full integration of DTT in the sector.
4.1.2 Economic factors
Economic and market-related challenges are key barriers to implementing DTT, especially for smaller companies. Interviews highlighted the significant time and financial investments required to integrate DTT into existing systems and circular practices. All the respondents (9/10) except one sustainability and digitalization expert emphasized the high costs, and time demands of tracking building materials, with one participant noting: “Mostly money and time, because just tracking and tracing the material is costly.” Smaller companies face additional obstacles, including limited resources, insufficient infrastructure, and capital, which hinder their ability to adopt advanced technologies and comply with regulations. Additionally, the terms “economic” and “infrastructure” on social media word cloud and bibliometric keyword map, further emphasize the infrastructural challenges in adopting DT generally. These challenges may arise from the high costs of upgrading existing systems, limited technological readiness, and fragmented digital infrastructure across companies in the construction sector. Despite acknowledging the investment challenge, the Software/Technology Providers expressed optimism about the economic potential of DTT. One provider remarked, “Investment in digital tools enhances operational efficiency and can yield significant Return on Investment (ROI) when applied correctly.” The respondents also view digital transformation as essential for maintaining competitiveness, as clients increasingly expect digitally enabled services. These findings align with previous studies that identify financial constraints as critical barriers to adopting advanced digital solutions (Lobo et al., 2021; Dervishaj et al., 2023). Similarly, insights from the bibliometric analysis (Munaro and Tavares, 2021) highlight the importance of adequate infrastructural investment to facilitate the adoption of DT. Interestingly, despite widespread concerns about costs, only one respondent mentioned incentives as a driver for long-term adoption. This raises important questions about whether there are implicit regulatory restrictions limiting access to public funding such as grants, regional funding programs and tax rebates for green innovations or if the industry is generally unaware of the available financial support mechanisms. These types of financial incentives might alleviate the burden on individual companies and encourage consensus and standardization in how such work is undertaken.
4.1.3 Social and cultural factors
Industry attitudes toward sustainability and technology adoption vary widely, shaped by regime-level cultural and organizational norms. A positive attitude toward sustainability emerged as a key driver for DTT implementation. Social media analyses further reinforced this strength, revealing widespread enthusiasm and approval for CE initiatives. However, the Swedish construction industry’s complexity, marked by diverse projects, processes, and actors creates unique challenges. As (Bygballe and Ingemansson, 2014; Gerhardsson et al., 2020; Vogel, 2020) note, that the heterogeneity of the sector necessitates multi-level interactions among developers, contractors, building owners, and consultants, each with varying levels of digital maturity. Traditionally, the industry is known to be resistant to change and sceptical toward innovation (Akintoye et al., 2012; Gerhardsson et al., 2020). A notable knowledge gap within the industry further exacerbates these challenges, as stakeholders often lack a clear understanding of DTT’s functionality, benefits, and implementation pathways. This gap is particularly pronounced among traditional actors such as precast concrete manufacturers, who perceive DTT as disruptive. One concrete manufacturer observed, “There’s a strong reliance on traditional methods, and many stakeholders are not convinced of the value digital systems bring.” Such views highlight not only entrenched practices but also the absence of comprehensive awareness and education about DTT’s potential to transform operations and improve sustainability outcomes. On the other hand, Sustainability and Digitalization experts expressed optimism, noting growing acceptance of digital standards and a collaborative mindset among stakeholders. One expert stated, “Stakeholders are beginning to see these tools as essential for achieving sustainability goals.” This cultural divide shows the need for education, case studies, and collaborative approaches to bridge gaps and foster industry-wide acceptance of DTT. Themes of knowledge sharing and collaborative learning, as seen in word cloud analysis and bibliometric analysis (Bellini and Bang, 2022), and the interviews appeared as both drivers and barriers of adoption. This dynamic interplay of actors and attitudes illustrates the social complexities inherent in the transition toward digital and sustainable practices in the construction industry. These barriers further align with studies showing the construction sector as one of the least digitized industries, hampered by attitudinal and cultural resistance (ECSO, 2021; Munaro and Tavares, 2021; 2023).
4.1.4 Technological factors
The data-driven nature of DTT emerged as a key technological strength, enabling enhanced operational efficiency and circular practices. However, barriers identified in the bibliometric analysis (Honic et al., 2019; Teisserenc and Sepasgozar, 2021; Bellini and Bang, 2022) were also evident in the interview findings, particularly among concrete manufacturers. These challenges include difficulties with data integration and adapting to new technologies. One respondent remarked, “Integrating digital systems into our traditional processes is challenging and disrupts our current operations.” This reflects a hesitance to move away from established practices, highlighting the need for technologies that seamlessly integrate into existing workflows to minimize disruptions. In contrast, Software/Technology Providers highlighted the transformative potential of DTT. They emphasized its ability to enhance operational control, track materials, and enable innovative design solutions. As one provider stated, “Digital systems allow us to monitor and optimize operations in real-time, which is a game-changer for our clients.” The architect and AEC expert also echoed this, noting that new technologies enable innovative design solutions that can flexibly respond to evolving client needs. These suggest that while some stakeholders see DTT as disruptive, others perceive it as transformative, enhancing operational efficiency and flexibility. Existing studies further support these findings, emphasizing the importance of digital solutions like asset tracking and resource management in promoting CE practices (Zhao et al., 2017; Uçar et al., 2020; Daniotti et al., 2022; Thirumal et al., 2024). Additionally, the word cloud and bibliometric keyword co-occurrence map prominently featured terms such as ‘digital,’ ‘technology,’ and ‘innovation,’ alongside emerging technologies like ‘RFID,’ ‘blockchain,’ ‘material passports,’ ‘QR codes,’ and ‘IoT.’ These tools are recognized for their potential to transform conventional practices, enabling real-time monitoring, improved resource management, and the reuse of materials through DTT (Zhao et al., 2017; Camodeca and Almici, 2021; Del Rio et al., 2021; Kedir et al., 2021; Munaro and Tavares, 2021; Daniotti et al., 2022; Davari et al., 2023; Mêda et al., 2023).
4.1.5 Environmental factors
Environmental drivers, including the rising demand for sustainable practices and waste reduction, strongly support DTT adoption. Organizations increasingly view DTT as a critical tool for achieving sustainability goals amid societal and market pressures for greener practices. One participant emphasized, “Sustainability is becoming a non-negotiable standard, and digital solutions are seen as a viable means to achieve it.” Findings of Camodeca and Almici (2021) and Del Rio et al. (2021) further highlight the environmental benefits of DTT, such as improved resource management, waste reduction, and material recycling, aligning with the 2030 Sustainable Development Goals (SDGs). The word cloud and keyword map reinforced this environmental focus, with terms like “circular economy,” “recycling,” and “sustainability” dominating the industry, academic, and social media discourse. Digital solutions enable precise material tracking, quality control, and smarter production practices, fostering the transition to circular and sustainable approaches.
4.2 SWOT analysis
The PESTLE factors identified are grouped into Strengths (S), Weaknesses (W), Opportunities (O), and Threats (T) within the SWOT framework (Table 1), providing a comprehensive evaluation of the dynamics of the change process driving digitalization in the prefab concrete sector. A key insight from this analysis is the interaction between internal barriers (Weaknesses-W), external opportunities (O), and external barriers (Threats-T), which provides a clear pathway for stakeholders to address barriers. For example, high investment costs and resource limitations in smaller companies (W2 & W3) often hinder their ability to adopt innovative technologies. However, these challenges present opportunities for targeted incentives or government support (O3) to alleviate financial burdens. Similarly, regulatory and permitting challenges (T1) can be addressed by leveraging environmental policies like the EU Green Deal (S1), which may drive innovation.
To overcome these barriers, different industry stakeholders can take distinct approaches. Small concrete manufacturers, for instance, could benefit from government incentives (O3) and strategic collaborations (O8) to reduce high adoption costs and expand access to digital technologies. Larger manufacturers, with more resources, could lead industry-wide initiatives to integrate digital technologies and share knowledge across the value chain, supporting smaller companies in the process. Technology providers can capitalize on opportunities to improve stakeholder collaboration (O8), ensuring that digital solutions are adaptable to the diverse needs of the market and can be integrated more easily into existing processes. Meanwhile, policymakers can promote a supportive environment by enforcing regulations that incentivize digital adoption and circular practices while providing guidance to ease regulatory challenges (T1) and support the adoption of new business models. Addressing the complex network of actors (W11) and the need for significant mindset shifts (W12), overcoming resistance to change (T2) will require stronger stakeholder engagement and education initiatives (O6 & O8), focusing on building trust and creating a unified vision for the digital transformation of the sector. This multi-faceted approach will enable the industry to capitalize on opportunities while mitigating the challenges that hinder the adoption of digital technologies for circular economy practices.
4.3 MLP dynamics
The integrated PESTLE and SWOT analysis identified that the Swedish prefab concrete sector, like the broader construction industry, operates within a complex socio-technical system in which dynamics at the landscape, regime, and niche levels interact and shape the digital transformation trajectory for circularity (Vogel, 2020). This interaction illustrated in Figure 6 represents subcategories of the observed barriers and drivers from the study.
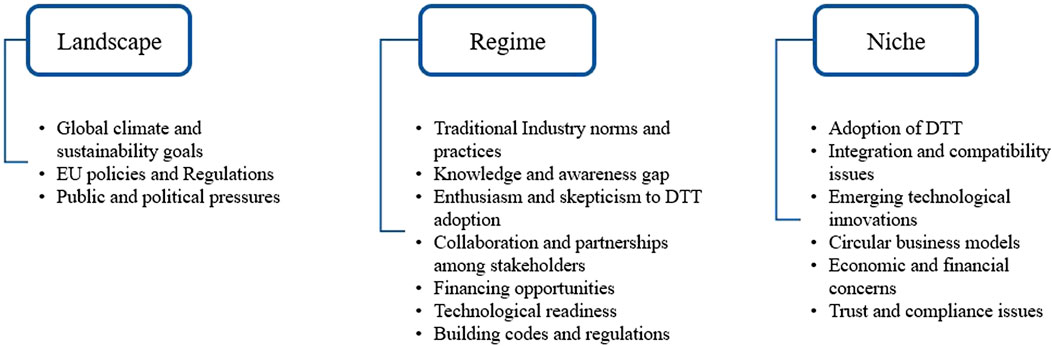
Figure 6. A diagram illustrating the subcategories of observed barriers and drivers according to the three MLP levels.
At the landscape level, overarching drivers such as global climate and sustainability goals, EU policies and regulations, and public and political pressures create a compelling need for change. Frameworks like the UN SDGs, the EU Green Deal, and Sweden’s commitment to carbon neutrality are accelerating the shift towards circularity in the construction sector. These forces place significant pressure on the industry (regime) to adopt DT to meet sustainability targets, enhance resource efficiency, traceability, and transparency, and reduce emissions. However, external pressures (economic and environmental) revealed through themes in academic literature, social media discourse, and interviews, highlight disparities in preparedness among actors within the prefab concrete sector. While larger firms are often better positioned to respond proactively to regulatory incentives, smaller companies face considerable challenges in meeting these expectations, primarily due to limited resources and capacity.
At the regime level, various PESTLE factors interact simultaneously, shaping industry dynamics. Interviews revealed that a significant number of barriers remain, particularly the influence of established industry practices and the tension between entrenched norms and innovation. The precast concrete sector, in particular, exhibits notable stability due to its deeply rooted norms and the complex network of influential actors, as described by Geels (2002) and Vogel (2020). This stability, however, often translates into resistance to change, driven by a conservative mindset and ingrained practices. The study identified several critical weaknesses within the industry. Smaller companies face significant resource limitations, which hinder their ability to adopt new technologies. A major challenge lies in the lack of IT knowledge required for the implementation of DTT, compounded by scepticism regarding the tangible benefits DTT offers to companies. This scepticism is further worsened by the lack of technological readiness due to fragmented digital infrastructure and a heavy reliance on traditional norms. Additionally, low market demand for DTT-enabled products from customers further impedes the industry’s digital transformation efforts. Tensions between traditional industry practitioners and technology developers highlight divergent perspectives on digitalization. While some stakeholders view digitalization as disruptive to established practices, others perceive it as a transformative force, paving the way for a more innovative and sustainable construction sector.
Although building codes and regulations such as the Construction Products Regulation (CPR) and Swedish Building Code (BBR) have yet to mandate digital tracking of construction materials, implementing Digital Products Passports (DPP) provides a pathway for future adoption. As these frameworks evolve to prioritize sustainability, they are fostering an environment that is both stable and adaptable, encouraging the gradual integration of DTT. This structured progression not only aligns with sustainability objectives but also enables the industry to incrementally reshape its standards, creating a foundation for a more circular and technologically advanced construction sector.
At the niche level, innovative technologies such as QR codes, BIM, material passports, and the IoT have emerged as powerful tools identified in the analysis for improving resource management and advancing the principles of the CE in the Swedish prefab concrete sector. For example, the implementation of material passports provides detailed information about concrete components, including their composition, origin, and recycling potential (Dervishaj et al., 2023; Ali et al., 2024). This traceability reduces waste by ensuring that materials can be reused or recycled efficiently at the end of their lifecycle (Giovanardi et al., 2022). Findings from this study indicated that QR codes were primarily utilized for project management, logistics, and the storage of concrete components, rather than directly contributing to CE objectives. However, when viewed collectively, DT like BIM, RFID, and IoT are driving incremental advancements in data management and material traceability (Mallawaarachchi and Jayakodi, 2023; Shishehgarkhaneh et al., 2024). These innovations are gradually reshaping industry norms and laying the groundwork for a broader digital transformation. Despite their potential, these technologies remain in the early stages of development and face significant challenges. High upfront costs, integration complexities, and uncertain returns on investment present substantial barriers to widespread adoption. Furthermore, concerns persist regarding how data can be effectively retrieved and verified throughout the supply chain, as well as the security and privacy risks associated with tracking every construction component. These challenges pinpoint the experimental nature of these technologies and highlight the need for further refinement to unlock their full potential. Nonetheless, these innovations play a critical role in driving the initial stages of the Socio Technical Transition toward a CE in the prefab concrete sector. They provide a foundation for broader adoption and systemic change, enabling disruptive innovations to challenge entrenched norms at the regime level. As more actors within the industry adopt circular business models and leverage DT for sustainability, the sector moves closer to achieving systemic transformation.
5 Conclusion
This study aimed to examine the key barriers and drivers influencing the adoption of DTT for transitioning to a CE in Sweden’s precast concrete sector. A triangulation approach was employed, cross-validating findings from bibliometric analysis, social media data, and interviews to ensure robustness and depth. The analysis of the drivers and barriers in the form of an integrated PESTLE and SWOT framework uncovered the systemic socio-technical dynamics shaping DTT adoption for circularity in Sweden’s precast concrete sector. Key dynamics observed highlighted the interplay between stakeholders, innovation, and the challenges and opportunities inherent in systemic transformation. While landscape-level drivers, such as sustainability regulations, create pressure for change, regime-level inertia underlines the need for targeted interventions to overcome resistance. Meanwhile, niche innovations when supported by effective policies and collaborative initiatives could emerge as potential disruptors capable of reshaping entrenched practices and advancing the sector toward CE objectives. Despite its complexity, this transition represents a critical step in aligning the construction industry with broader sustainability goals.
To accelerate the adoption of DTT, a multifaceted approach involving policy initiatives, industry collaboration, and technological investments is essential. From a policy perspective, introducing targeted financial subsidies and tax incentives can significantly reduce the upfront costs of DTT implementation, particularly for small and medium-sized companies that may struggle with limited resources. Additionally, developing standardized data-sharing protocols is crucial to addressing issues of fragmentation and fostering greater interoperability among stakeholders, enabling smoother integration of DTT solutions across the supply chain. In terms of industry collaboration and social media discourse, organizing multi-stakeholder workshops and forums can help build trust and foster collaboration, ensuring that industry actors are aligned in their expectations and efforts. Such platforms also serve as opportunities to address resistance to change by highlighting the shared benefits of DTT adoption. Moreover, enhancing stakeholder engagement and awareness of the environmental and operational advantages of DTT is vital to bridge existing knowledge gaps. For example, initiatives like the Ellen MacArthur Foundation demonstrate how collaborative networks can connect industries, researchers, and policymakers to scale circular innovations. Such efforts illustrate the tangible impact of aligning strategic objectives with actionable outcomes in CE transitions. Technologically, investing in scalable and user-friendly digital tracking solutions is imperative to ensure that these technologies can be seamlessly integrated into existing workflows without disrupting daily operations. Furthermore, promoting the use of advanced tools like Digital Product Passports (DPP) in the construction sector can boost material traceability efforts and support compliance with regulatory standards, reinforcing the role of DTT in transitioning to a CE. Together, these strategic efforts can address existing barriers, leverage opportunities, and create an enabling environment for the widespread adoption of DTT in the precast concrete sector.
This study makes a theoretical contribution to the socio-technical systems and CE transition literature by adopting a triangulated approach that integrates the PESTLE, SWOT, and MLP frameworks. By applying these frameworks to bibliometric analysis, social media data, and interview findings, the study offers a replicable methodology for future research exploring similar themes. However, the research is subject to certain limitations. The scope of the study was constrained by the relatively small sample size of interview participants and its focus on the Swedish precast concrete sector. While the initial intention was to investigate this sector specifically, the responses often reflected the dynamics of the broader construction industry. As a result, some nuances unique to the precast sector may not have been fully captured.
To address these gaps, future research could expand the sample size to include a more diverse range of stakeholders relevant to DTT adoption. Similarly, engaging local policymakers and municipal regulators could reveal additional barriers, such as inconsistencies in regional compliance standards. Additionally, assessing digital readiness and benchmarking maturity levels across different sizes of precast concrete firms in Sweden and the EU could offer valuable insights. Comparative market gap analyses across regions would further help benchmark CE transition rates and identify region-specific barriers and drivers influencing DTT adoption.
Data availability statement
The raw data supporting the conclusions of this article will be made available by the authors, without undue reservation.
Ethics statement
Ethical approval was not required for this study involving human participants, as per local and institutional guidelines. Verbal informed consent was obtained from all participants in accordance with GDPR. Prior to participation, each individual was thoroughly briefed on the research objectives, data handling procedures, and confidentiality measures. Written consent was not required under national legislation and institutional policy, provided that verbal consent was obtained and no sensitive personal data was collected.
Author contributions
MO: Conceptualization, Data curation, Formal Analysis, Investigation, Methodology, Software, Validation, Visualization, Writing – original draft, Writing – review and editing. RA: Supervision, Validation, Writing – review and editing, Conceptualization, Project administration. KM: Funding acquisition, Supervision, Validation, Writing – review and editing, Resources, Project administration.
Funding
The author(s) declare that financial support was received for the research and/or publication of this article. This study is partially financed by the Interreg North Sea region and Region Kronoberg under the project “Circular Trust Building”.
Acknowledgments
The authors acknowledge the interviewees for their time and insightful opinions. This research builds on a master’s thesis (Shehu, 2024) originally published at Linnaeus University and an earlier, shorter version presented in the IEEE Conference Proceedings (Mahapatra et al., 2024).
Conflict of interest
The authors declare that the research was conducted in the absence of any commercial or financial relationships that could be construed as a potential conflict of interest.
Generative AI statement
The author(s) declare that no Generative AI was used in the creation of this manuscript.
Publisher’s note
All claims expressed in this article are solely those of the authors and do not necessarily represent those of their affiliated organizations, or those of the publisher, the editors and the reviewers. Any product that may be evaluated in this article, or claim that may be made by its manufacturer, is not guaranteed or endorsed by the publisher.
References
Adams, W. C. (2015). “Conducting semi-structured interviews,” in Handbook of practical program evaluation (John Wiley & Sons, Ltd), 492–505. doi:10.1002/9781119171386.ch19
Adriana Hofmann Trevisan Lobo, A., Guzzo, D., Gomes, L. A. d. V., and Mascarenhas, J. (2023). Barriers to employing digital technologies for a circular economy: a multi-level perspective. J. Environ. Manag. 332, 117437. doi:10.1016/j.jenvman.2023.117437
Akintoye, A., Goulding, J., and Zawdie, G. (2012). “Construction innovation and process improvement,” in Construction innovation and Process improvement. Editors A. Akintoye, J. Goulding, and G. Zawdie (Wiley-Blackwell), 3–17. doi:10.1002/9781118280294.ch1
Ali, V., Gebremariam, A. T., Di Maio, F., Meister, K., Koulaeian, T., and Rem, P. (2024). RFID-based material passport system in a recycled concrete circular chain. J. Clean. Prod. 442, 140973. doi:10.1016/j.jclepro.2024.140973
Andrew, R. M. (2018). Global CO2 emissions from cement production. Earth Syst. Sci. Data 10 (1), 195–217. doi:10.5194/essd-10-195-2018
Babor, D., Plian, D., and Judele, L. (2009). Environmental impact of concrete. Bull. Polytech. Inst. Jassy, Constr. Archit. Sect. Tomme LV (LIX), Fascicle 4, 27–36.
Bellini, A., and Bang, S. (2022). Barriers for data management as an enabler of circular economy: an exploratory study of the Norwegian AEC-industry. IOP Conf. Ser. Earth Environ. Sci. 1122, 012047. doi:10.1088/1755-1315/1122/1/012047
Benachio, G. L. F., Freitas, M. d. C. D., and Tavares, S. F. (2020). Circular economy in the construction industry: a systematic literature review. J. Clean. Prod. 260, 121046. doi:10.1016/j.jclepro.2020.121046
Biswas, H. K., Sim, T. Y., and Lau, S. L. (2024). Impact of building information modelling and advanced technologies in the AEC industry: a Contemporary review and future Directions. J. Build. Eng. 82, 108165. doi:10.1016/j.jobe.2023.108165
Bonoli, A., Zanni, S., and Serrano-Bernardo, F. (2021). Sustainability in building and construction within the framework of circular Cities and European new green deal. The contribution of concrete recycling. Sustainability 13 (4), 2139. doi:10.3390/su13042139
Business Sweden (2022). Sweden’s digital technologies Ecosystem, business Sweden. Available online at: https://www.business-sweden.com/insights/articles/swedens-digital-technologies-ecosystem/(Accessed September 15, 2024).
Bygballe, L. E., and Ingemansson, M. (2014). The logic of innovation in construction. Ind. Mark. Manag. 43 (3), 512–524. doi:10.1016/j.indmarman.2013.12.019
Camodeca, R., and Almici, A. (2021). Digital transformation and convergence toward the 2030 Agenda’s sustainability development goals: Evidence from Italian listed firms. Sustainability 13 (21), 11831. doi:10.3390/su132111831
Çetin, S., Gruis, V. H., and Straub, A. (2022). Digitalization for a circular economy in the building industry: multiple-case study of Dutch social housing organizations. Resour. conservation & Recycl. Adv. doi:10.1016/j.rcradv.2022.200110
Creswell, J. W. (2014). A Concise introduction to mixed methods research. Thousand Oaks, CA: SAGE Publications.
Dang, D. S., and Ahmad, P. H. (2015). A review of text mining techniques associated with various application areas. Available online at: https://www.semanticscholar.org/paper/A-Review-of-Text-Mining-Techniques-Associated-with-Dang-Ahmad/58be9b174785c74444beea35c944b4aa57ce23f7 (Accessed April 16, 2024).
Daniotti, B., Pavan, A., Bolognesi, C., Mirarchi, C., and Signorini, M. (2022). ‘Digital transformation in the construction sector: from BIM to digital twin. doi:10.5772/intechopen.103726
Davari, S., Jaberi, M., Yousfi, A., and Poirier, E. (2023). A traceability framework to enable circularity in the built environment. Sustainability 15 (10), 8278. doi:10.3390/su15108278
Del Rio, G., González, C., and Colsa, Á. (2021). Unleashing the convergence amid digitalization and sustainability towards pursuing the Sustainable Development Goals (SDGs): a holistic review. J. Clean. Prod. 280, 122204. doi:10.1016/j.jclepro.2020.122204
Demšar, J., and Zupan, B. (2013). Orange: data mining fruitful and fun - a historical perspective. Inform. Slov. 37, 55–60.
Dervishaj, A., and Gudmundsson, K. (2024). From LCA to circular design: a comparative study of digital tools for the built environment. Resour. Conservation Recycl. 200, 107291. doi:10.1016/j.resconrec.2023.107291
Dervishaj, A., Vargas, J. H., and Gudmundsson, K. (2023). ‘Enabling reuse of prefabricated concrete components through multiple tracking technologies and digital twins’. doi:10.35490/ec3.2023.220
Dobesova, Z. (2024). Evaluation of Orange data mining software and examples for lecturing machine learning tasks in geoinformatics. Comput. Appl. Eng. Educ. 32 (4), e22735. doi:10.1002/cae.22735
ECSO (2021). Digitalisation in the construction sector - ECSO report. Available online at: https://bimaplus.org/uncategorized/digitalisation-in-the-construction-sector-ecso-report/(Accessed April 18, 2024).
Ellen MacArthur Foundation (2015). Towards a circular economy: business rationale for an accelerated transition. Available online at: https://www.ellenmacarthurfoundation.org/towards-a-circular-economy-business-rationale-for-an-accelerated-transition (Accessed April 17, 2024).
Ellen MacArthur Foundation (no date). The circular economy in detail. Available online at: https://www.ellenmacarthurfoundation.org/the-circular-economy-in-detail-deep-dive (Accessed April 18, 2024).
European Commission (2020). New circular economy action plan. Eur. Comm. - Eur. Comm. Available online at: https://ec.europa.eu/commission/presscorner/detail/en/ip_20_420 (Accessed April 19, 2024).
European Commission (2021). Delivering the European green deal - European Commission. Available online at: https://commission.europa.eu/strategy-and-policy/priorities-2019-2024/european-green-deal/delivering-european-green-deal_en (Accessed March 17, 2024).
European Environmental Agency (2024). Capturing the climate change mitigation benefits of circular economy and waste sector policies and measures. European Environment Agency. Available online at: https://www.eea.europa.eu/publications/capturing-the-climate-change-mitigation/capturing-the-climate-change-mitigation (Accessed June 22, 2024).
European Union (2023). New regulation on construction products. Available online at: http://data.europa.eu/eli/C/2024/4018/oj/eng (Accessed November 11, 2024).
Eurostat (2023). Waste statistics, Waste statistics. Available online at: https://ec.europa.eu/eurostat/statistics-explained/index.php?title=Waste_statistics (Accessed June 4, 2024).
Fei, W., Opoku, A., Agyekum, K., Oppon, J. A., Ahmed, V., Chen, C., et al. (2021). The critical role of the construction industry in achieving the sustainable development goals (SDGs): Delivering projects for the common Good. Sustainability 13 (16), 9112. doi:10.3390/su13169112
Galvin, R. (2015). How many interviews are enough? Do qualitative interviews in building energy consumption research produce reliable knowledge? J. Build. Eng. 1, 2–12. doi:10.1016/j.jobe.2014.12.001
Geels, F. W. (2002). Technological transitions as evolutionary reconfiguration processes: a multi-level perspective and a case-study. Res. Policy 31 (8), 1257–1274. doi:10.1016/S0048-7333(02)00062-8
Geels, F. W. (2011). The multi-level perspective on sustainability transitions: responses to seven criticisms. Environ. Innovation Soc. Transitions 1 (1), 24–40. doi:10.1016/j.eist.2011.02.002
Gerhardsson, H., Lindholm, C. L., Andersson, J., Kronberg, A., Wennesjö, M., and Shadram, F. (2020). Transitioning the Swedish building sector toward reuse and circularity. IOP Conf. Ser. Earth Environ. Sci. 588 (4), 042036. doi:10.1088/1755-1315/588/4/042036
Giovanardi, M. (2024). “RFId for construction sector. Technological innovation in circular economy perspective,” in Lecture notes in Mechanical Engineering, 315–329. doi:10.1007/978-3-031-36922-3_18
Giovanardi, M., Konstantinou, T., Pollo, R., and Klein, T. (2022). Internet of Things for building façade traceability: a theoretical framework to enable circular economy through life-cycle information flows. J. Clean. Prod. 382, 135261. doi:10.1016/j.jclepro.2022.135261
Graf-Vlachy, L., Buhtz, K., and König, A. (2018). Social influence in technology adoption: taking stock and moving forward. Manag. Rev. Q. 68, 37–76. doi:10.1007/s11301-017-0133-3
Gurel, E., and Tat, M. (2017). Swot Analysis: A Theoretical Review. J. Int. Soc. Res. 10 (51), 994–1006. doi:10.17719/jisr.2017.1832
Habert, G., Miller, S. A., John, V. M., Provis, J. L., Favier, A., Horvath, A., et al. (2020). Environmental impacts and decarbonization strategies in the cement and concrete industries. Nat. Rev. Earth & Environ. 1, 559–573. doi:10.1038/s43017-020-0093-3
Herbert, L. (2017). Digital transformation: B.U.I.L.D Your Organization’s future. London, UK: Bloomsbury Business.
Honic, M., Kovacic, I., Sibenik, G., and Rechberger, H. (2019). Data- and stakeholder management framework for the implementation of BIM-based Material Passports. J. Build. Eng. 23, 341–350. doi:10.1016/j.jobe.2019.01.017
Javaid, M., Haleem, A., Singh, R. P., Suman, R., and Gonzalez, E. S. (2022). Understanding the adoption of Industry 4.0 technologies in improving environmental sustainability. Sustain. Operations Comput. 3, 203–217. doi:10.1016/j.susoc.2022.01.008
Jayaraj, N., Klarin, A., and Ananthram, S. (2024). The transition towards solar energy storage: a multi-level perspective. Energy Policy 192, 114209. doi:10.1016/j.enpol.2024.114209
Jiang, P., Zhang, L., You, S., Fan, Y. V., Tan, R. R., Klemeš, J. J., et al. (2023). Blockchain technology applications in waste management: overview, challenges and opportunities. J. Clean. Prod. 421, 138466. doi:10.1016/j.jclepro.2023.138466
Jiang, Y., Su, S., Zhao, S., Zhong, R. Y., Qiu, W., Skibniewski, M. J., et al. (2024). Digital twin-enabled synchronized construction management: a roadmap from construction 4.0 towards future prospect. Dev. Built Environ. 19, 100512. doi:10.1016/j.dibe.2024.100512
Jick, T. D. (1979). Mixing qualitative and quantitative methods: triangulation in action. Adm. Sci. Q. 24 (4), 602. doi:10.2307/2392366
Kanger, L. (2021). Rethinking the Multi-level Perspective for energy transitions: from regime life-cycle to explanatory typology of transition pathways. Energy Res. & Soc. Sci. 71, 101829. doi:10.1016/j.erss.2020.101829
Katenbayeva, A., Glass, J., Anvuur, A., and Ghumra, S. (2016). Developing a theoretical framework of traceability for sustainability in the construction sector. Available online at: https://repository.lboro.ac.uk/articles/conference_contribution/Developing_a_theoretical_framework_of_traceability_for_sustainability_in_the_construction_sector/9436811/1 (Accessed April 18, 2024).
Kaufman, S., Saeri, A. K., Raven, R., Malekpour, S., and Smith, L. D. G. (2022). Behaviour in sustainability transitions: a mixed methods literature review. OSF. doi:10.31219/osf.io/krmcv
Kebede, R., Moscati, A., Tan, H., and Johansson, P. (2023). Circular economy in the built environment: a framework for implementing digital product passports with knowledge graphs. Comput. Constr. doi:10.35490/ec3.2023.245
Kedir, F., Bucher, D. F., and Hall, D. M. (2021). A proposed material passport Ontology to enable circularity for Industrialized construction. null. doi:10.35490/ec3.2021.159
Li, L. (2020). The governance of low-carbon transitions in a Multilevel perspective framework: how does the Concept of ‘system transformation’ work? Energy Res. J. 11, 45–53. doi:10.3844/erjsp.2020.45.53
Liu, H., and Zainul Abidin, N. (2024). A review on research of prefabricated building costs: exploring collaborations, Intellectual Basis, and research trends. Sustainability 16, 9823. doi:10.3390/su16229823
Liu, Q., Trevisan, A. H., Yang, M., and Mascarenhas, J. (2022). A framework of digital technologies for the circular economy: digital functions and mechanisms. Bus. Strategy Environ. 31, 2171–2192. doi:10.1002/bse.3015
Liu, Q., Trevisan, A. H., Yang, M., and Mascarenhas, J. (2022). A framework of digital technologies for the circular economy: digital functions and mechanisms. Bus. Strategy Environ. 31, 2171–2192. doi:10.1002/bse.3015
Ljubljana, B. L. University of (no date) orange data mining, orange data mining. Available online at: https://orangedatamining.com (Accessed December 10, 2024).
Lobo, A., Trevisan, A. H., Liu, Q., Yang, M., and Mascarenhas, J. (2021). “Barriers to transitioning towards Smart circular economy: a systematic literature review,” in Sustainable design and manufacturing. Editors S. G. Scholz, R. J. Howlett, and R. Setchi (Singapore: Springer), 245–256. doi:10.1007/978-981-16-6128-0_24
Mahapatra, K., Shehu, M. O., and Almeida, R. (2024). “Exploring the prospect of digital tracking technology in enhancing the circularity of the Swedish precast concrete sector,” in 2024 International conference on sustainable energy: energy transition and Net-Zero climate future (ICUE). 2024 International conference on sustainable energy: energy transition and Net-Zero climate future (ICUE), 1–7. doi:10.1109/ICUE63019.2024.10795516
Mainali, B., Mahapatra, K., and Pardalis, G. (2021). Strategies for deep renovation market of detached houses. Renew. Sustain. Energy Rev. 138, 110659. doi:10.1016/j.rser.2020.110659
Mallawaarachchi, B. H., and Jayakodi, S. (2023). Internet of things (iot)-enabled industrial symbiosis model for construction material sharing: bibliometric analysis, 595, 605. doi:10.31705/wcs.2023.49
Mêda, P., Munir, M., Calvetti, D., and Sousa, H. (2023). ‘Information needs in digital products passport – discussing data framework and reasonability’. doi:10.35490/ec3.2023.244
Munaro, M., and Tavares, S. (2021). Materials passport’s review: challenges and opportunities toward a circular economy building sector. Built Environ. Proj. Asset Manag. 11, 767–782. doi:10.1108/BEPAM-02-2020-0027
Nguyen, T. D., and Adhikari, S. (2023). The role of BIM in integrating digital twin in building construction: a literature review. Sustainability 15 (13), 10462. doi:10.3390/su151310462
Nilimaa, J. (2023). Smart materials and technologies for sustainable concrete construction. Dev. Built Environ. 15, 100177. doi:10.1016/j.dibe.2023.100177
NLTK Sentiment Analysis Tutorial: Text Mining & Analysis in Python (no date). Available online at: https://www.datacamp.com/tutorial/text-analytics-beginners-nltk (Accessed December 10, 2024).
Oberle, B., Bringezu, S., Hatfield-Dodds, S., Hellweg, S., Schandl, H., Clement, J., et al. (2019). UN global resources Outlook 2019: natural resources for the future We want.
Rastogi, N., and Trivedi, M. (2022). ‘Pestle technique -a tool to identify external risks in construction projects’.
Regina Munaro, M., and Tavares, S. F. (2023). A review on barriers, drivers, and stakeholders towards the circular economy: the construction sector perspective. Clean. Responsible Consum. 8, 100107. doi:10.1016/j.clrc.2023.100107
RE:Source (2022). Circularity gap report Sweden, RE:source. Available online at: https://resource-sip.se/en/circularity-gap-report-sweden-en/(Accessed September 15, 2024).
Rodrigo, N., Omrany, H., Chang, R., and Zuo, J. (2023). Leveraging digital technologies for circular economy in construction industry: a way forward. SMART Sustain. BUILT Environ. 13, 85–116. doi:10.1108/SASBE-05-2023-0111
Schot, J., and Geels, F. W. (2008). Strategic niche management and sustainable innovation journeys: theory, findings, research agenda, and policy. Technol. Analysis & Strategic Manag. 20 (5), 537–554. doi:10.1080/09537320802292651
Sepasgozar, S. M. E., Khan, A., Smith, K., Romero, J., Shen, X., Shirowzhan, S., et al. (2023). BIM-And digital twin for developing convergence technologies as future of digital construction. Buildings 13 (2), 441. doi:10.3390/buildings13020441
Shehu, M. O. (2024). A multi-level analysis of the barriers 850 and opportunities in adopting digital tracking technologies for concrete circularity in Sweden. Available online at: https://urn.kb.se/resolve?urn=urn:nbn:se:lnu:diva-130716 (Accessed January 27, 2025).
Shishehgarkhaneh, M. B., Keivani, A., Moehler, R. C., Jelodari, N., and Laleh, S. R. (2024). Internet of things (IoT), building information modeling (BIM), and digital twin (DT) in construction industry: a review, bibliometric, and network analysis. Available online at: https://www.mdpi.com/2075-5309/12/10/1503 (Accessed November 10, 2024).
Statista (2023). Global cement CO2emissions 1960-2022, Statista. Available online at: https://www.statista.com/statistics/1299532/carbon-dioxide-emissions-worldwide-cement-manufacturing/(Accessed: November 9, 2024).
Tang, L., and Feng, Y. (2019). ‘Sentiment analytics of social media data in the construction industry: preliminary study on Weibo’, Constructing Smart Cities: proceedings of the 22nd CIB World building Congress (CIB2019), 17-21 June 2019, The Hong Kong Polytechnic University, Hong Kong, China. Available online at: https://researchdirect.westernsydney.edu.au/islandora/object/uws%3A55408/(Accessed: 23 December 2024).
Teisserenc, B., and Sepasgozar, S. (2021). Adoption of blockchain technology through digital twins in the construction industry 4.0: a PESTELS approach. BUILDINGS 11 (12), 670. doi:10.3390/buildings11120670
Thirumal, S., Udawatta, N., Karunasena, G., and Al-Ameri, R. (2024). Barriers to adopting digital technologies to implement circular economy practices in the construction industry: a systematic literature review. Sustainability 16 (8), 3185. doi:10.3390/su16083185
Tiwari, R., Kumar, G., and Gunjan, V. (2023). ‘Effect of environment on Students performance through orange tool of data mining’, in, pp. 283–292. doi:10.1007/978-981-99-2058-7_26
Tsironis, G., Daglis, T., and Tsagarakis, K. P. (2024). The 21 most practiced RE-s of circular economy from LinkedIn company profiles on a global scale. Resour. Conservation & Recycl. Adv. 21, 200202. doi:10.1016/j.rcradv.2024.200202
Uçar, E., Dain, M. A. L., and Joly, I. (2020). Digital technologies in circular economy transition: Evidence from case studies. Procedia CIRP 90, 133–136. doi:10.1016/j.procir.2020.01.058
UNEP (2023). Digital transformations | UNEP - UN environment Programme. Available online at: https://www.unep.org/topics/digital-transformations (Accessed November 10, 2024).
Vardopoulos, I., Tsilika, E., Sarantakou, E., Zorpas, A., Salvati, L., and Tsartas, P. (2021). An integrated SWOT-PESTLE-AHP model assessing sustainability in adaptive reuse projects. Appl. Sci. 11 (15), 7134. doi:10.3390/app11157134
Vogel, J. (2020). Incentivising innovation in the Swedish construction industry. doi:10.13140/RG.2.2.25481.47203
Wong, R. W. M., and Loo, B. P. Y. (2022). Sustainability implications of using precast concrete in construction: an in-depth project-level analysis spanning two decades. J. Clean. Prod. 378, 134486. doi:10.1016/j.jclepro.2022.134486
Wuni, I. Y. (2022). Mapping the barriers to circular economy adoption in the construction industry: a systematic review, Pareto analysis, and mitigation strategy map. Build. Environ. 223, 109453. doi:10.1016/j.buildenv.2022.109453
Zakrisson, A. (2018). Swedish step-by-step guide to construction waste. Available online at: https://iimpcoll.com/content/swedish-guide-construction-waste (Accessed September 16, 2024).
Zhao, J., Olivieri, H., Seppänen, O., Peltokorpi, A., Badihi, B., and Lundström, P. (2017). “Data analysis on applying real time tracking in production control of construction,” in 2017 IEEE International conference on industrial engineering and engineering management (IEEM). 2017 IEEE International conference on industrial engineering and engineering management (IEEM), 573–577. doi:10.1109/IEEM.2017.8289956
Keywords: barriers, circular economy, concrete sector, digital tracking technology, drivers, multilevel perspective
Citation: Shehu MO, Almeida R and Mahapatra K (2025) Opportunities for digital tracking technologies in the precast concrete sector in Sweden. Front. Built Environ. 11:1566784. doi: 10.3389/fbuil.2025.1566784
Received: 25 January 2025; Accepted: 31 March 2025;
Published: 24 April 2025.
Edited by:
Vagelis Plevris, Qatar University, QatarReviewed by:
Vanni Nicoletti, Marche Polytechnic University, ItalyAlberto Cerezo Narváez, University of Cádiz, Spain
Copyright © 2025 Shehu, Almeida and Mahapatra. This is an open-access article distributed under the terms of the Creative Commons Attribution License (CC BY). The use, distribution or reproduction in other forums is permitted, provided the original author(s) and the copyright owner(s) are credited and that the original publication in this journal is cited, in accordance with accepted academic practice. No use, distribution or reproduction is permitted which does not comply with these terms.
*Correspondence: Roma Almeida, cm9tYS5hbG1laWRhQGxudS5zZQ==
†ORCID: Krushna Mahapatra, orcid.org/0000-0003-4405-1056