- 1Zhonglian Runshi Xinjiang Coal Industry Company Limited, Changji, Xinjiang, China
- 2Zhengzhou Bole Information Technology Company Limited, Zhengzhou, Henan, China
Introduction: Road hazards and some traffic accidents are often caused by damage of the performance of the pavement due to cracking of asphalt concrete.
Methods: A simulation test was designed to investigate asphalt pavement cracking under different temperature conditions (15°C, 0°C, −5°C, −15°C) with a fixed traffic load strip. Using Gaussian filtering to eliminate texture noise (5 × 5 convolution kernels), extracting continuous crack contours through Canny operator (dual threshold 0.7/0.3), and combining with improved Otsu algorithm (32 × 32 block dynamic threshold) to achieve accurate segmentation under complex lighting conditions; Innovatively introducing a dual parameter system of fractal dimension (box dimension method) and crack rate (crack area ratio) to quantitatively characterize the complexity of crack morphology and degree of damage.
Results: And proposed using two crack characteristic parameters, fractal dimension and crack rate, to describe the extension characteristics of cracks. The results indicate that the road surface is more prone to cracking in negative zero temperature environments, with the number and rate of cracks generated at −15°C being much higher than the other three low-temperature environments. Compared to the 15°C environment, the crack rate increased by 18.26%.
Discussion: It has been confirmed that the occurrence and propagation of cracks will be exacerbated in low-temperature environments. The research results provide a theoretical basis and reference significance for the study of pavement crack resistance in cold regions.
1 Introduction
Asphalt concrete pavement highways have the advantages of convenient construction and high smoothness, and which are widely used in modern highway construction. However, due to vehicle loads and changes in external temperature, road structures are prone to crack hazards (Alsheyab et al., 2024; Sun et al., 2025; Wang et al., 2017). The materials that make up asphalt roads are mostly elastic and viscoelastic materials. Due to their inherent thermal expansion characteristics, they undergo volume expansion and contraction during heating and cooling. As shown in Figure 1, it can cause stress concentration within the road structure, leading to the occurrence of disasters such as longitudinal cracks, potholes, and mesh cracks (Baradaran et al., 2024). Although it is believed that the effect of climate on pavement structure in cold regions is one of the main causes of pavement structure damage, only a small number of models have studied frost heave and thaw settlement. Asphalt pavement disease is the result of vehicle load and environmental factors (Ameri et al., 2012; Li et al., 2021; Yang Binbin et al., 2024), among which the repeated action of vehicle loads is an important reason for inducing fatigue damage in asphalt pavement. The working temperature range of asphalt pavement is very wide, and long-term loading causes the deformation of asphalt pavement to gradually increase, stress to gradually relax, and asphalt mixture exhibits typical viscoelastic properties (Guo et al., 2023). If the damage level of road surface cracks is not identified and maintained in a timely manner, hazards such as road surface damage, road collapse, and increased traffic accidents will be caused, directly threatening the lives and health of the people (Liang et al., 2021; Yang B. et al., 2024; Sarsam, 2015). So, it is necessary to identify road cracks and damages in a timely manner.
In the destruction of asphalt highways, pavement cracking is the main form of damage. Asphalt pavement cracks are an important manifestation of road diseases, directly affecting the integrity and service life of the pavement structure. Among them, transverse cracks will penetrate the entire road surface, and the crack walls will scatter, mainly caused by temperature stress (Krysiński and Sudyka, 2013). In areas with significant seasonal temperature differences, regular cracking with a spacing of 20–50 m is caused by the low-temperature shrinkage of asphalt mixtures. The initial width of the cracks is about 1–3 mm, which may be expanded to over 10 mm over time, and peripheral network cracks may be induced (Marasteanu et al., 2007; Marasteanu et al., 2012; Shan et al., 2022). Longitudinal cracks with wide crack width and scattered or slightly scattered crack walls often occur in the wheel track area. Mainly due to uneven settlement of the roadbed, improper treatment of construction joints, or repeated overloading of vehicles, the crack width is generally between 2 and 15 mm, but the length exceeds 3 m (Ameri et al., 2011; Yang Binbin et al., 2024; Yuan B. et al., 2024). In high-traffic sections, such cracks may develop as a precursor to structural damage. In the past, most highways used cement concrete pavement, but this type of pavement has relatively low friction, especially on rainy days, which can easily cause traffic accidents. In the later stage, an additional asphalt layer was used, but this structure is prone to forming reflective cracks, which expand upwards from the cracks in the lower layer structure. The crack shape corresponds to the cracks in the base layer, forming a straight or broken line with a width of 1–5 mm (Canestrari and Ingrassia, 2020; Zhao et al., 2018). This type of crack has obvious mechanical transmission characteristics, and its expansion speed can reach 3 mm/year under the coupling effect of temperature difference and load. The bonding failure area between asphalt layers may sometimes form crescent shaped or arc-shaped cracks, and even interlayer peeling due to interlayer pollution, waterproof bonding layer failure, and other reasons (Ai et al., 2022; Zhang et al., 2022). Affected by the environment, the aging and brittleness of asphalt mixture can result in the road surface being fragmented into rectangular or square pieces, with a diameter generally greater than 50cm, and crack widths and crack areas being scattered, which are often observed on roads with a long service life. Some cracks form in the early stages, with no deformation or scattering in the crack area, fine cracks, obvious cracking state, and mild scattering or deformation in the crack area.
Asphalt pavement cracks are recognized as the initial form of road diseases, and their harm is characterized by progressive and systematic features, directly impacting road service performance and service life. Cracks cause damage to the integrity of the pavement structure, and water infiltrates the base layer through cracks, leading to secondary diseases such as softening of the base material (Gong et al., 2024; Yuan Bingxiang et al., 2024; Zhang et al., 2023). Experimental data shows that the larger the crack width, the higher the water infiltration rate. Continuous water infiltration causes a greater decrease in the rebound modulus of the base layer, accelerating the fatigue damage of the structural layer (Sun et al., 2019; Si et al., 2019; Dan et al., 2017). The deflection value of the reflection crack area increases compared to the normal road section, significantly reducing the overall bearing capacity of the road surface. The uneven road surface formed by crack development significantly affects driving comfort and safety. When the crack width exceeds a certain value, the vehicle impact load will increase exponentially, leading to an increased risk of vehicle jumping (Wang et al., 2021; Zeng et al., 2024; Hou et al., 2021; Xi et al., 2025). The friction coefficient in the mesh cracking area will be rapidly decreased, which can easily lead to vehicle side slip accidents being caused on rainy days. Highway safety research shows that the accident rate is higher in sections with dense cracks than in normal sections. Crack propagation is nonlinear, and the maintenance cost of untreated cracks in the early stage increases exponentially in the later stage (Yu et al., 2020; Yuan et al., 2022; Guo et al., 2021; Ahammed and Tighe, 2012). Research has shown that doubling the width of cracks increases repair costs by more than four times. Crack treatment accounts for a high proportion of the annual maintenance budget, with the highest cost of milling and repaving in cracked areas. The surface of road damage caused by cracks leads to an increase in vehicle fuel consumption and carbon emissions. The dust pollution caused by the peeling of aggregate in the crack area increases the concentration of PM2.5 in the surrounding area. The expansion of cracks in heavy-duty traffic sections can shorten the lifespan of the road surface (Karlaftis and Badr, 2015). Preventive maintenance construction caused by cracks can also occupy lane time and result in a loss of traffic efficiency. In the context of expressway infrastructure, the risk of accidents in crack repair sections has significantly increased, exacerbating traffic congestion and economic chain losses. The hazard of cracks has a significant time cumulative effect, and the natural expansion of small cracks in the short term may trigger larger network crack areas (Mazumder et al., 2019). Using an infrared thermal imager to monitor and display abnormal temperature gradients in the hidden crack area indicates a potential risk of structural damage. Cracks can be identified in a timely manner, reducing the probability of secondary disasters, extending the service life of road surfaces, and highlighting the key role of crack prevention and control in road lifecycle management.
The traditional method of detecting road surface diseases is mainly reliant on manual inspection, which is associated with disadvantages such as high labor intensity, long time consumption, subjectivity, large errors, and impacts on traffic. With the development of computer technology, image-based automated detection techniques or laser radar detection methods have emerged (Zakeri et al., 2017; Kheradmandi and Mehranfar, 2022). The identification of asphalt pavement cracks is an important research direction in the field of intelligent transportation infrastructure detection. Digital image processing technology is one of the core methods for identifying cracks in asphalt pavement. Through algorithm optimization and process design, the full chain processing from image acquisition to feature extraction has been achieved, providing basic support for automated detection. In the preprocessing stage, histogram equalization is used to enhance image contrast, and Gaussian filtering is used to eliminate asphalt surface texture noise (Ji et al., 2020; Hu et al., 2021). After a rainstorm, the road reflection reduces the image saturation by 40%, the oil stain pollution leads to the confusion of texture features, and the tree shadow causes the artifact interference. The crack recognition method in machine vision provides great impetus for road crack detection. From traditional image processing, machine learning, and the recent rise of deep learning-based road crack detection technology, road detection technology has been widely studied. The future detection accuracy is expected to exceed 98%, providing accurate data support for road maintenance decisions (Darshan and Kataware, 2024). Technological development requires continuous attention to core issues such as adaptability to complex environments, interpretability of models, and standardization of detection standards.
The cracking of concrete pavement under different low-temperature conditions is simulated in this article through experiments conducted using high and low-temperature test chambers under the same traffic load. Using digital image processing methods, cracks in asphalt pavement were processed, and two crack characteristic parameters, fractal dimension and crack rate, were proposed to describe the propagation characteristics of cracks.
2 Materials and methods
2.1 Materials
Firstly, impurities in additives such as asphalt, coarse aggregate (crushed stone), and fine aggregate (natural sand) are removed through screening and manual inspection. Then, the mixture comprised asphalt (4.2%), coarse aggregate (41.2%), fine aggregate (54.0%), and additives (0.6%) by mass. “The aggregates were heated and dried in a heater at 180°C, then mixed with mineral powder and additives.” Finally, the asphalt is melted at 160°C and added to the above mixture, stirred for 1 min to fully mix with the aggregate, forming an asphalt pavement mixture. The thickness of asphalt mixture is similar to a ratio of 1:5, with an actual thickness of 10 cm and a simulated thickness of 2 cm in the experiment. Use rectangular modules with a length and width of 25 cm. The load is calculated based on the average traffic flow rate, with 3 vehicles per minute and a total weight of 50 tons per vehicle. The vehicle typically has 4 wheels on the front axle and 8 wheels on the rear axle, with a tire ground pressure of 0.66 Mpa. The vehicle runs every 20 s and moves back and forth. The camera above takes a picture every 0.5 days. The experimental process is shown in Figure 2.
2.2 Digital image processing
To address the issues of uneven lighting and noise interference in road surface images, histogram equalization is used to enhance contrast and expand the grayscale value difference in crack areas by 30%–50%. Gaussian filtering effectively eliminates asphalt texture noise and improves the signal-to-noise ratio (SNR) to over 25 dB through 5 × 5 convolution kernels for smoothing. The image processing flowchart is shown in Figure 3.
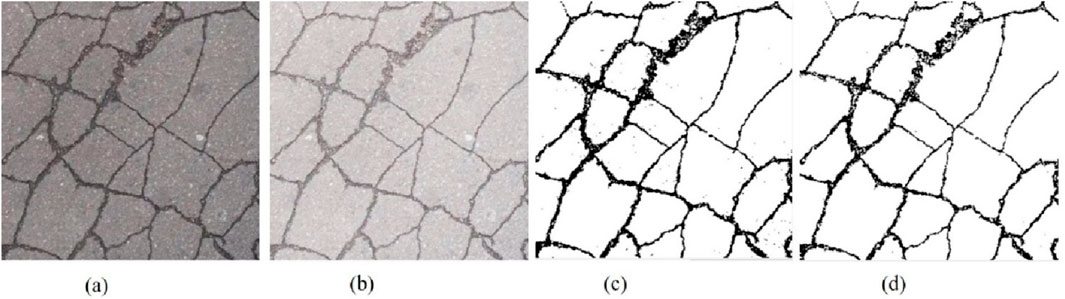
Figure 3. Image processing. (a) RGB→Grayscale; (b) Filter enhancement; (c) Threshold segmentation; (d) Denoising→Binarization.
Grayscale transformation. Grayscale images can reduce data volume, better highlight image brightness information, reduce color interference in color images, and better analyze image shape and edge information. Using the average method, the RGB values of each pixel are obtained, and then added and averaged to obtain a new pixel value. The new pixel value is used as the grayscale value of that pixel value.
Accurate extraction of edge features. In classic edge detection algorithms, the Canny operator accurately extracts continuous crack contours through non maximum suppression and a dual threshold (high threshold 0.7, low threshold 0.3) strategy, achieving a localization accuracy of ±2 pixels for linear cracks (Xu et al., 2017). The Sobel operator utilizes a 3 × 3 gradient template for fast computation, with a real-time performance of up to 50 F PS, but is susceptible to false edges caused by oil stains. The Prewitt operator enhances oblique crack recognition through directional filtering, resulting in a 15% improvement in the repair effect of fracture connections for network cracks.
The optimal segmentation threshold is automatically calculated by the maximum inter-class variance method (Otsu), enabling the crack area to be separated from the background under uniform illumination conditions with an accuracy of 78% (Xu et al., 2011; Liu and Yu, 2009). The improved local thresholding method divides the image into blocks (32 × 32 pixels) and dynamically adjusts the threshold by combining it with the neighborhood grayscale mean, thereby improving the segmentation accuracy to 85% in complex lighting scenes. The region growing algorithm takes seed points as the core and iteratively expands the crack area through grayscale similarity, which has a better effect on maintaining the continuity of curved cracks than traditional segmentation methods.
The global threshold Otsu method (OTSU) automatically adapts the most suitable boundary value by calculating the relative difference between the foreground and background in the image. Then, using this as a reference, the grayscale value of a pixel in the image is compared with the boundary value. If the grayscale value is greater than the boundary value, the pixel is defined as white, otherwise it is defined as black. Processed images can highlight specific features and details, reduce processing difficulty, and facilitate subsequent analysis of the image.
Calculation of fractal dimension. We use the box counting method to completely cover the analyzed images with grids of different sizes r (1 × 1; 3 × 3; 9 × 9…), obtain the number of r values, calculate
The crack rate is defined as the proportion of cracks in a certain area that occupy the entire area. It can intuitively reflect the severity of sample cracking. The crack rate reflects the overall damage of the specimen, and there is a positive correlation between them, and the worse the overall stability. Firstly, the black or white pixels in the binarized image are counted, and then multiplied by the actual area of a single pixel to obtain
3 Results
3.1 The effect of different temperatures on asphalt crack rate
From Figure 5, it can be seen that under the four temperature states, the overall crack rate shows an upward trend with the passage of simulation time. Among them, the crack rate in the simulation experiment at −15°C increased the fastest, eventually reaching 13.25%. Mainly because at this ambient temperature, the viscoelasticity of asphalt pavement mixture is lost and eventually becomes brittle at low temperatures. A decrease in temperature can also cause a rapid increase in the stiffness modulus of asphalt. Under the same external load, the brittle pavement and the increase in stiffness modulus will reduce the ability of the pavement to resist deformation, making it unable to effectively buffer the concentrated stress generated by vehicles with its own elasticity, resulting in the pavement reaching the load limit and eventually cracking more quickly. Compared to the low-temperature state, a slower increase in the crack rate of asphalt pavement at 15°C was observed in the simulation experiment, ultimately reaching 10.83%. This is attributed to the asphalt material being in a viscoelastic state and exhibiting a certain degree of flexibility. When the vehicle’s own load acts on the road surface, asphalt can disperse the load stress generated by the vehicle through plastic deformation, and can withstand stress for a longer period of time. Cracks appear the latest and have the lowest crack rate. Compared to environments at 15°C and −15°C, asphalt gradually transitions from viscoelasticity to brittleness at intermediate temperatures of 0°C and −5°C. At this point, asphalt still retains some plasticity, but its ability to disperse loads is slightly weaker than at 15°C, but higher than at −15°C. Therefore, the crack rates at 0°C and −5°C are 11.23% and 12.35%, respectively. Overall, cracks are more likely to occur in low-temperature environments, and under the same external load, materials in low-temperature conditions are more likely to reach their tensile strain limit, resulting in a higher ultimate crack rate.
3.2 The influence of temperature on the fractal dimension changes of asphalt cracks
Figure 6 shows the curves of the fractal dimension of the experimental samples over time at different temperature states. With the passage of time, the fractal dimension of the experimental samples at temperatures of 0°C, −5°C, and −15°C showed an upward trend, while at a temperature of 15°C, the fractal dimension of the experimental samples first increased and then showed a certain downward trend. As the temperature rises, the shrinkage strain of asphalt pavement tends to decrease. Under external loads, it can absorb and resist more unbalanced stresses, making it difficult for internal cracks to crack. Compared to low-temperature environments, asphalt materials at 15°C have higher plasticity and can be compressed by vehicles under the same vehicle load when cracks appear, causing some cracks to re heal. However, in low-temperature environments, the material has lower plasticity and fewer cracks to heal, ultimately showing an upward trend. The energy consumption rate of asphalt pavement in low-temperature environments is lower than that in environments above 0°C, and its impact absorption ability is weaker. This makes it easier for asphalt pavement to reach the material limit, and the energy threshold required for cracks is lower, resulting in earlier crack occurrence. The appearance of cracks can damage the overall stability of asphalt pavement, resulting in uneven stress distribution and low plasticity of the material itself, which cannot be effectively restored. This weakens the stress around the crack when it is subjected to vehicle loads, accelerating the appearance of cracks on the surrounding side. The cycle repeats, and the number of cracks will increase, ultimately leading to an increase in fractal dimension. The final fractal dimensions of the experimental samples in the 15°C, 0°C, −5°C, and −15 °C environments were 1.482, 1.603, 1.673, and 1.757, respectively. Compared with 0°C, −5°C, and −15°C, the fractal dimensions of the experimental samples in the 15°C environment decreased by 7.54%, 14.64%, and 15.65%, respectively. The control group decreased by 6.05%, 9.98%, and 14.28%, respectively. Therefore, asphalt pavement materials have lower elastic-plastic properties and are more prone to cracking in low-temperature environments.
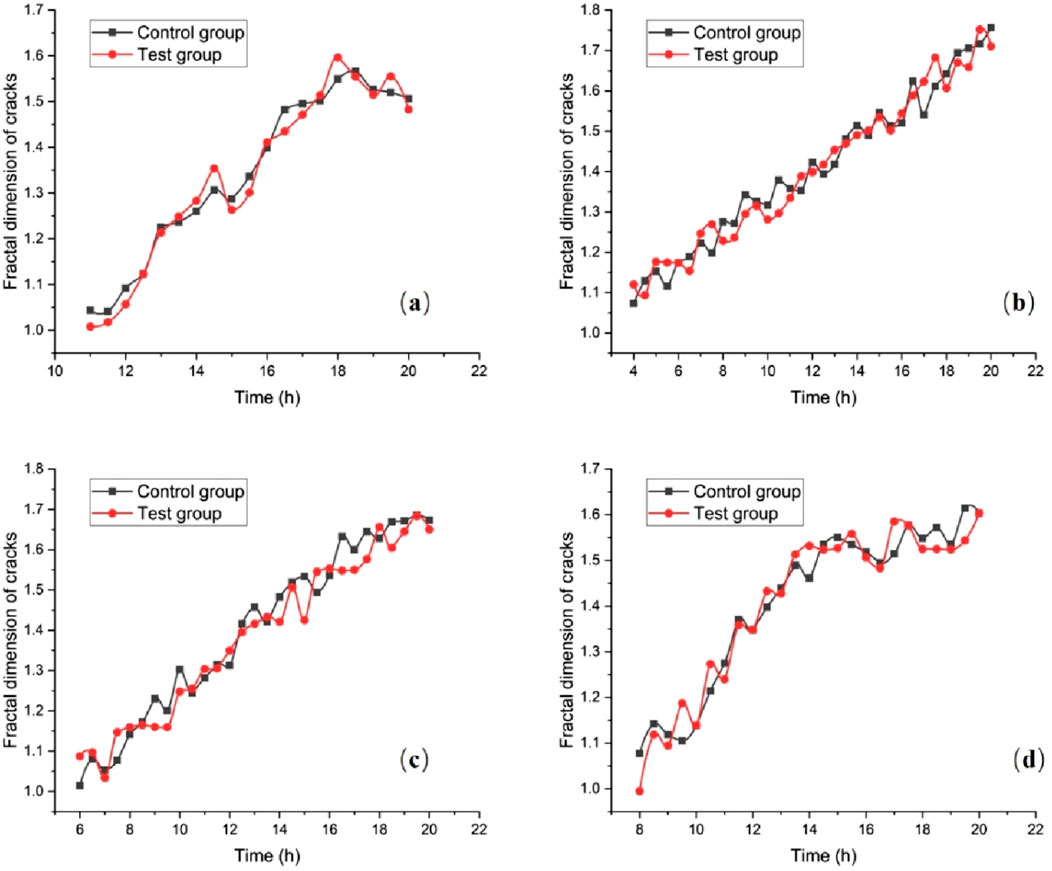
Figure 6. Fractal dimension versus time curve under different temperature environments (a) the experimental temperature is 15°C; (b) The ambient temperature is 150°C; (c) The ambient temperature is −5°C; (d) Ambient temperature is −15°C.
3.3 The effect of temperature on the total number of asphalt cracks
As shown in Figure 7- The total number of cracks at 15°C (364) is 63% higher than that at 15°C (223), consistent with the fractal dimension trend in Figure 6. This correlation indicates that lower temperatures not only increase crack density, but also increase crack complexity. The experimental results showed that the final total number of cracks at 15°C, 0°C, −5°C, and −15°C were 223, 245, 254, and 364, respectively. During temperature rise,the final total number of cracks showed a decreasing trend, and over time, with the change of experimental temperature, the number of cracks increases, especially at −15°C, where the rate of increase in crack number was much higher than that of the other three temperature samples. The surface of asphalt is mainly covered by asphalt, which is a viscoelastic material. Changes in temperature directly affect the performance of asphalt. When simulating ambient temperatures between 15°C and 0°C, asphalt will gradually increase in viscoelasticity as the temperature decreases, resulting in increased brittleness and decreased ductility of the overall road surface. Meanwhile, when the external load vehicle generates tensile stress, cracks will appear over time, leading to an accelerated crack propagation rate. In the simulated environment of −5°C to −15°C, the plasticity of asphalt materials rapidly decreases, brittleness gradually increases, and ductility gradually loses. The stress generated by external loads cannot be offset by the material’s ductility, exacerbating the stress concentration of microcracks. Cracks will be observed earlier and their number will be gradually increased over time. On the other hand, at a low temperature of −15°C, the fatigue resistance of asphalt mixtures decreases rapidly, resulting in a faster crack propagation rate compared to environments above 0°C. At the same time, the stiffness of the contact between the tire and the road surface increases under low temperature conditions (i.e., the contact stress increases), and under the same load, the road surface is more prone to damage and the appearance of a large number of cracks. But the number of such cracks will not increase infinitely. The overall number of cracks can be divided into three stages: slow increase, rapid growth, and eventually flattening. The first stage is attributed to the fact that a certain degree of plasticity is still retained by the material when the road surface is first damaged. It can buffer external stress in a timely manner, resulting in a slow increase in the number of cracks. However, as the number of cracks continues to increase, the overall damage of the material becomes severe, and external loads can no longer be buffered by its own elastic-plastic properties, leading to a rapid increase in the number of cracks. In the third stage, the surface is covered by cracks, and stress can no longer be absorbed by the road surface, being mainly transmitted to the roadbed. The number of cracks increases gradually and finally tends to be stable.
4 Discussion
The external conditions affecting the performance of asphalt materials mainly include temperature, light, humidity, load, soil conditions, etc. Among them, in the application of asphalt pavement, there are significant temperature differences in different regions, attention must be paid to the effect of temperature on asphalt mixture when paving asphalt pavement (Lee et al., 2007; Gong et al., 2017). Under negative temperature conditions, the brittleness of asphalt mixture increases, and insufficient tensile strength can easily lead to shrinkage cracks under external loads. The accumulation of stress caused by external loads accelerates the occurrence of pavement cracks (Santos et al., 2018; Isacsson and Zeng, 1998; Zhang et al., 2014; Xue et al., 2013). As shown in the Figure 8, with the decrease of experimental temperature, the maximum crack rate, maximum total number of cracks, and fractal dimension of the sample all show an increasing trend, where 15°C < 0°C < 5 °C < −15°C. Therefore, under other constant factors, the number of cracks is negatively correlated with temperature, and the lower the temperature, the more cracks there will be. This only applies to asphalt materials, which are highly sensitive to temperature. When the temperature is high, the load is dispersed and transmitted through plastic deformation, while in low temperature environments, it is dispersed through a rigid skeleton. However, under repeated load cycles, the fatigue performance of the material intensifies, and as time goes on, the number of surface cracks increases.
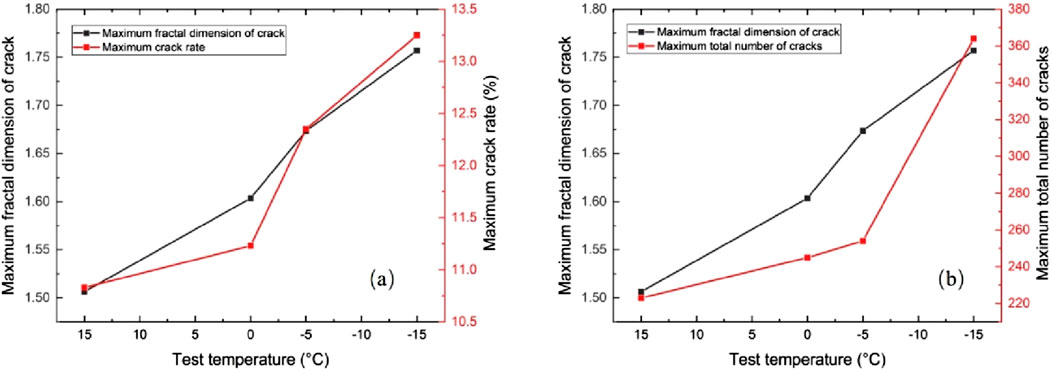
Figure 8. Crack correlation curves under different temperature environments (a) Relationship curve between maximum fractal dimension and maximum crack rate; (b) Relationship curve between large fractal dimension and maximum number of cracks.
The current research only focuses on quantitative analysis of the number of surface cracks on asphalt pavement under different temperature environments. However, asphalt is not only affected by environmental temperature, but also related to asphalt materials and the presence or absence of additives. Although this experiment has well verified that pavement is more prone to cracking and has a shorter failure time under low temperature environments, there is a lack of relevant research on actual day night temperature differences. At 15°C, the fractal dimension first increases and then decreases, attributed to the viscoelastic properties of asphalt: the initial load leads to the generation of microcracks (fractal dimension increases); As the temperature increases, the plasticity of the material increases, and some cracks reopen due to stress relaxation (fractal dimension decreases). However, the experiment did not consider the impact of dynamic load fluctuations (such as changes in vehicle speed) on crack propagation. In the future, it is necessary to deepen research by combining real-time load monitoring and multi-scale models. However, the experiment can provide scientific guidance for subsequent research on temperature effects, as well as ideas and theoretical references for asphalt improvement.
5 Conclusion
This article simulates the specific cracking of asphalt pavement under the same vehicle load in environments of 15°C, 0°C, −5°C, and −15°C. The cracking behavior of asphalt pavement under vehicle load cycles was quantitatively described using digital image processing, and the relationship between fractal dimension and crack rate was analyzed. The influencing factors of pavement performance under different environmental temperatures were analyzed, and the cracking mechanism of asphalt pavement under different temperature environments was analyzed. The influence of temperature on fractal dimension and crack rate was discussed. Based on the above discussion, we have drawn the following conclusions.
As the temperature decreases, the number of cracks appearing on asphalt pavement shows an increasing trend. The crack rates at 15°C, 0°C, −5°C, and −15°C environments are 10.83%, 11.23%, 12.35%, and 13.25%, respectively. Among them, the crack rate at −15°C is the highest, increasing by 18.26% compared to the crack rate at 15°C environment. The final fractal dimensions of the samples in the experimental group at 15°C, 0°C, −5°C, and −15°C environments are 1.482, 1.603, 1.673, and 1.757, respectively. The fractal dimensions of the experimental samples at 15°C environment decreased by 7.54%, 14.64%, and 15.65% compared to 0°C, −5°C, and −15°C environments, respectively. The total number of cracks at 15°C, 0°C, −5°C, and −15°C corresponds to 223.245, 254, 364.
In low-temperature environments, the generation and propagation of surface cracks in asphalt pavement are mainly affected by material plasticity and impact stress absorption at different temperatures. When the temperature drops to −15°C, the viscoelasticity of asphalt mixture significantly decreases, the material rigidity increases, and the deformation ability sharply decreases, resulting in its inability to release shrinkage stress through stress relaxation mechanism. At this point, the temperature gradient between the asphalt layer and the semi-rigid base layer intensifies, and the displacement difference caused by the shrinkage of the base layer forms a stronger stress concentration at the bottom of the asphalt surface layer, promoting the rapid initiation of cracks in weak areas. At the same time, low temperature increases the brittleness of asphalt mixture, and the stress on cracks will gradually increase, resulting in a chaotic distribution and expansion of crack propagation (increasing fractal dimension). Compared to temperature conditions above −5°C, the low-temperature tensile strength and ultimate strain threshold of asphalt at −15°C are broken earlier, and microcracks quickly penetrate to form a macroscopic crack network, resulting in a significant increase in the total number of cracks and an earlier occurrence time.
Data availability statement
The original contributions presented in the study are included in the article/supplementary material, further inquiries can be directed to the corresponding author.
Author contributions
JJ: Funding acquisition, Investigation, Project administration, Supervision, Writing – review and editing. KX: Data curation, Visualization, Writing – original draft. YS: Formal Analysis, Investigation, Writing – original draft. LG: Investigation, Resources, Software, Writing – original draft. JZ: Formal Analysis, Methodology, Writing – review and editing.
Funding
The author(s) declare that financial support was received for the research and/or publication of this article. This work is financial support from the Zhonglian Runshi Xinjiang Coal Industry Company Limited under Grant No. ZR-CX2023010.
Conflict of interest
Authors JJ, KX, YS, LG, and JZ were employed by Zhonglian Runshi Xinjiang Coal Industry Company Limited. Author JJ was employed by Zhengzhou Bole Information Technology Company Limited.
Generative AI statement
The author(s) declare that no Generative AI was used in the creation of this manuscript.
Publisher’s note
All claims expressed in this article are solely those of the authors and do not necessarily represent those of their affiliated organizations, or those of the publisher, the editors and the reviewers. Any product that may be evaluated in this article, or claim that may be made by its manufacturer, is not guaranteed or endorsed by the publisher.
References
Ahammed, M. A., and Tighe, S. L. (2012). Asphalt pavements surface texture and skid resistance—exploring the reality. Can. J. Civ. Eng. 39 (1), 1–9. doi:10.1139/l11-109
Ai, C., He, H., Zhang, X., Meng, H., and Ren, D. (2022). Investigation of inter-layer bonding property in asphalt pavement based on 3D morphology of reconstructed interface. Constr. Build. Mater. 317, 125983. doi:10.1016/j.conbuildmat.2021.125983
Alsheyab, M. A., Khasawneh, M. A., Abualia, A., and Sawalha, A. (2024). A critical review of fatigue cracking in asphalt concrete pavement: a challenge to pavement durability. Innov. Infrastruct. Solutions 9 (10), 386. doi:10.1007/s41062-024-01704-1
Ameri, M., Mansourian, A., Khavas, M. H., Aliha, M., and Ayatollahi, M. R. (2011). Cracked asphalt pavement under traffic loading–A 3D finite element analysis. Eng. Fract. Mech. 78 (8), 1817–1826. doi:10.1016/j.engfracmech.2010.12.013
Ameri, M., Mansourian, A., Pirmohammad, S., Aliha, M. R. M., and Ayatollahi, M. R. (2012). Mixed mode fracture resistance of asphalt concrete mixtures. Eng. Fract. Mech. 93, 153–167. doi:10.1016/j.engfracmech.2012.06.015
Baradaran, S., Aliha, M. R. M., Maleki, A., and Underwood, B. S. (2024). Fracture properties of asphalt mixtures containing high content of reclaimed asphalt pavement (RAP) and eco-friendly PET additive at low temperature. Constr. Build. Mater. 449, 138426. doi:10.1016/j.conbuildmat.2024.138426
Canestrari, F., and Ingrassia, L. P. (2020). A review of top-down cracking in asphalt pavements: causes, models, experimental tools and future challenges. J. Traffic Transp. Eng. Engl. Ed. 7 (5), 541–572. doi:10.1016/j.jtte.2020.08.002
Dan, H. C., Tan, J. W., Zhang, Z., and He, L. H. (2017). Modelling and estimation of water infiltration into cracked asphalt pavement. Road Mater. Pavement Des. 18 (3), 590–611. doi:10.1080/14680629.2016.1182057
Darshan, N., and Kataware, A. V. (2024). Review on porous asphalt pavements: a comprehensive resolution for stormwater management and applications in current built environment. Int. J. Pavement Res. Technol., 1–25. doi:10.1007/s42947-024-00444-w
Gong, M., Zhang, H., and Zhu, J. (2024). Study on the interlayer critical response of asphalt pavement with different paving methods based on cohesive zone model. Aust. J. Civ. Eng., 1–15. doi:10.1080/14488353.2024.2313831
Gong, X., Romero, P., Dong, Z., and Li, Y. (2017). Investigation on the low temperature property of asphalt fine aggregate matrix and asphalt mixture including the environmental factors. Constr. Build. Mater. 156, 56–62. doi:10.1016/j.conbuildmat.2017.08.142
Guo, F., Pei, J., Zhang, J., Li, R., Zhou, B., and Chen, Z. (2021). Study on the skid resistance of asphalt pavement: a state-of-the-art review and future prospective. Constr. Build. Mater. 303, 124411. doi:10.1016/j.conbuildmat.2021.124411
Guo, M., Yao, X., and Du, X. (2023). Low temperature cracking behavior of asphalt binders and mixtures: a review. J. Road Eng. 3, 350–369. doi:10.1016/j.jreng.2023.11.001
Hou, F., Li, T., Li, X., Li, Y., and Guo, M. (2021). Research on the anti-reflective cracking performance of a full-depth asphalt pavement. Sustainability 13 (17), 9499. doi:10.3390/su13179499
Hu, G. X., Hu, B. L., Yang, Z., Huang, L., and Li, P. (2021). Pavement crack detection method based on deep learning models. Wirel. Commun. Mob. Comput. 2021 (1), 5573590. doi:10.1155/2021/5573590
Isacsson, U., and Zeng, H. (1998). Cracking of asphalt at low temperature as related to bitumen rheology. J. Mater. Sci. 33, 2165–2170. doi:10.1023/a:1004383506240
Ji, A., Xue, X., Wang, Y., Luo, X., and Xue, W. (2020). An integrated approach to automatic pixel-level crack detection and quantification of asphalt pavement. Automation Constr. 114, 103176. doi:10.1016/j.autcon.2020.103176
Karlaftis, A. G., and Badr, A. (2015). Predicting asphalt pavement crack initiation following rehabilitation treatments. Transp. Res. Part C Emerg. Technol. 55, 510–517. doi:10.1016/j.trc.2015.03.031
Kheradmandi, N., and Mehranfar, V. (2022). A critical review and comparative study on image segmentation-based techniques for pavement crack detection. Constr. Build. Mater. 321, 126162. doi:10.1016/j.conbuildmat.2021.126162
Krysiński, L., and Sudyka, J. (2013). GPR abilities in investigation of the pavement transversal cracks. J. Appl. Geophys. 97, 27–36. doi:10.1016/j.jappgeo.2013.03.010
Lee, H. J., Lee, J. H., and Park, H. M. (2007). Performance evaluation of high modulus asphalt mixtures for long life asphalt pavements. Constr. Build. Mater. 21 (5), 1079–1087. doi:10.1016/j.conbuildmat.2006.01.003
Li, L., Wu, C., Cheng, Y., Tan, G., and Li, H. (2021). “Study on numerical simulation of asphalt mixtures and asphalt pavement based on finite element software: a Review,” in 2021 IEEE 11th annual international conference on CYBER technology in automation, control, and intelligent systems (CYBER) (IEEE), 560–565.
Liang, B., Lan, F., Shi, K., Qian, G., Liu, Z., and Zheng, J. (2021). Review on the self-healing of asphalt materials: mechanism, affecting factors, assessments and improvements. Constr. Build. Mater. 266, 120453. doi:10.1016/j.conbuildmat.2020.120453
Liu, D., and Yu, J. (2009). “Otsu method and K-means,” in 2009 Ninth International conference on hybrid intelligent systems (IEEE), 1, 344–349. doi:10.1109/his.2009.74
Marasteanu, M., Buttlar, W., Bahia, H., Williams, C., Moon, K. H., Teshale, E. Z., et al. (2012). Investigation of low temperature cracking in asphalt pavements national pooled fund study–phase II.
Marasteanu, M., Zofka, A., Turos, M., Li, X., Velasquez, R., Li, X., et al. (2007). Investigation of low temperature cracking in asphalt pavements national pooled fund study 776.
Mazumder, M., Kim, H. H., and Lee, S. J. (2019). Comparison of field performance of crack treatment methods in asphalt pavement of Texas. J. Transp. Eng. Part B Pavements 145 (1), 04018057. doi:10.1061/jpeodx.0000087
Santos, J., Bressi, S., Cerezo, V., Presti, D. L., and Dauvergne, M. (2018). Life cycle assessment of low temperature asphalt mixtures for road pavement surfaces: a comparative analysis. Resour. Conservation Recycl. 138, 283–297. doi:10.1016/j.resconrec.2018.07.012
Sarsam, S. I. (2015). Crack healing potential of asphalt concrete pavement. Int. J. Sci. Res. Knowl. 3 (1), 001–012. doi:10.12983/ijsrk-2015-p0001-0012
Shan, J., Zhang, Y., Wu, S., Wu, Q., Jiao, Y., and Du, Y. (2022). Cracking behavior of asphalt pavement with a graded gravel layer based on computational granular mechanics. Constr. Build. Mater. 345, 128199. doi:10.1016/j.conbuildmat.2022.128199
Si, C., Chen, E., You, Z., Zhang, R., Qiao, P., and Feng, Y. (2019). Dynamic response of temperature-seepage-stress coupling in asphalt pavement. Constr. Build. Mater. 211, 824–836. doi:10.1016/j.conbuildmat.2019.03.183
Sun, X., Qin, X., Liu, Z., and Yin, Y. (2025). Damaging effect of fine grinding treatment on the microstructure of polyurea elastomer modifier used in asphalt binder. Measurement 242, 115984. doi:10.1016/j.measurement.2024.115984
Sun, Y., Guo, R., Wang, X., and Ning, X. (2019). Dynamic response characteristics of permeable asphalt pavement based on unsaturated seepage. Int. J. Transp. Sci. Technol. 8 (4), 403–417. doi:10.1016/j.ijtst.2019.09.005
Wang, H., Wu, Y., Yang, J., and Wang, H. (2021). Numerical simulation on reflective cracking behavior of asphalt pavement. Appl. Sci. 11 (17), 7990. doi:10.3390/app11177990
Wang, T., Xiao, F., Amirkhanian, S., Huang, W., and Zheng, M. (2017). A review on low temperature performances of rubberized asphalt materials. Constr. Build. Mater. 145, 483–505. doi:10.1016/j.conbuildmat.2017.04.031
Xin, L., Li, H., Niu, M., Yang, M., Xu, W., Wang, X., et al. (2025). Experimental and numerical investigation of thermal cracking of overlying rock in underground coal gasification. Eng. Fract. Mech. 315, 110808. doi:10.1016/j.engfracmech.2025.110808
Xu, X., Xu, S., Jin, L., and Song, E. (2011). Characteristic analysis of Otsu threshold and its applications. Pattern Recognit. Lett. 32 (7), 956–961. doi:10.1016/j.patrec.2011.01.021
Xu, Z., Baojie, X., and Guoxin, W. (2017). “Canny edge detection based on Open CV,” in 2017 13th IEEE international conference on electronic measurement and instruments (ICEMI) (IEEE), 53–56.
Xue, Q., Liu, L., Zhao, Y., Chen, Y. J., and Li, J. S. (2013). Dynamic behavior of asphalt pavement structure under temperature-stress coupled loading. Appl. Therm. Eng. 53 (1), 1–7. doi:10.1016/j.applthermaleng.2012.10.055
Yang, B., Chen, Y., Zhao, C., and Li, Z. (2024a). Effect of geotextiles with different masses per unit area on water loss and cracking under bottom water loss soil conditions. Geotext. Geomembranes 52 (2), 233–240. doi:10.1016/j.geotexmem.2023.10.006
Yang, B., Yang, C., and Yuan, S. (2024b). Investigation on structure, evaporation, and desiccation cracking of soil with straw biochar. Land Degrad. and Dev. 35 (9), 3126–3135. doi:10.1002/ldr.5122
Yu, M., You, Z., Wu, G., Kong, L., Liu, C., and Gao, J. (2020). Measurement and modeling of skid resistance of asphalt pavement: a review. Constr. Build. Mater. 260, 119878. doi:10.1016/j.conbuildmat.2020.119878
Yuan, B., Chen, W., Li, Z., Zhao, J., Luo, Q., Chen, W., et al. (2022). Sustainability of the polymer SH reinforced recycled Granite Residual Soil: properties, physicochemical mechanism and applications. J. Soils Sediments 23, 246–262. doi:10.1007/s11368-022-03294-w
Yuan, B., Liang, J., Huang, X., Huang, Q., Zhang, B., Yang, G., et al. (2024a). Eco-efficient recycling of engineering muck for manufacturing low-carbon geopolymers assessed through LCA: exploring the impact of synthesis conditions on performance. Acta Geotech., doi:10.1007/s11440-024-02395-9
Yuan, B., Liang, J., Lin, H., Wang, W., and Xiao, Y. (2024b). Experimental study on influencing factors associated with a new tunnel waterproofing for improved impermeability. J. Test. Eval. 52 (1), 344–363. doi:10.1520/jte20230417
Zakeri, H., Nejad, F. M., and Fahimifar, A. (2017). Image based techniques for crack detection, classification and quantification in asphalt pavement: a review. Archives Comput. Methods Eng. 24, 935–977. doi:10.1007/s11831-016-9194-z
Zeng, Z., Underwood, B. S., and Kim, Y. R. (2024). A state-of-the-art review of asphalt mixture fracture models to address pavement reflective cracking. Constr. Build. Mater. 443, 137674. doi:10.1016/j.conbuildmat.2024.137674
Zhang, H., Sun, B., Li, Y., and Li, Y. (2022). Adhesive property and road performance evaluation of asphalt overlay pavement with geotextile interlayer. Adv. Mater. Sci. Eng. 2022 (1), 1–11. doi:10.1155/2022/3084668
Zhang, Y., Luo, X., Luo, R., and Lytton, R. L. (2014). Crack initiation in asphalt mixtures under external compressive loads. Constr. Build. Mater. 72, 94–103. doi:10.1016/j.conbuildmat.2014.09.009
Keywords: asphalt concrete, highway pavement, cracking, image processing, fractal
Citation: Jiang J, Xu K, Song Y, Gao L and Zhang J (2025) Investigation of cracking behavior in asphalt pavement using digital image processing technology. Front. Built Environ. 11:1580379. doi: 10.3389/fbuil.2025.1580379
Received: 20 February 2025; Accepted: 27 March 2025;
Published: 08 April 2025.
Edited by:
Yang Chen, Sun Yat-sen University, ChinaReviewed by:
Yong Liu, China University of Mining and Technology, ChinaLichuang Jin, Henan Polytech Univ, China
Copyright © 2025 Jiang, Xu, Song, Gao and Zhang. This is an open-access article distributed under the terms of the Creative Commons Attribution License (CC BY). The use, distribution or reproduction in other forums is permitted, provided the original author(s) and the copyright owner(s) are credited and that the original publication in this journal is cited, in accordance with accepted academic practice. No use, distribution or reproduction is permitted which does not comply with these terms.
*Correspondence: Jie Jiang, amlhbmdqaWUyMDI0NTE4OEAxNjMuY29t