- 1Department of Wildlife, Cal Poly Humboldt, Arcata, CA, United States
- 2Department of Biology, Missouri Western State University, St. Joseph, MO, United States
- 3Department of Ecology and Evolutionary Biology, Santa Cruz, CA, United States
Introduction: Agroecosystem practices that aim to increase biodiversity and ecosystem services have the potential to benefit both wildlife and farmers. Shade-coffee systems are well-studied in the Neotropics, but less is known about the relationships among farm management, biodiversity, and ecosystem services in East Africa, where the most commonly used shade trees are native Cordia africana and non-native Grevillea robusta. Ecological theory and empirical evidence suggests that native shade trees should harbor more insects and insectivorous birds than non-native trees, which could translate to more pronounced pest control services on the coffee crop grown below.
Methods: We used artificial plasticine “caterpillars” in a sentinel pest experiment to test the prediction that predation is higher on coffee shrubs near native Cordia than non-native Grevillea shade trees.
Results: We found that there was no significant effect of tree species on bird predation, while predation by arthropods and total predation (birds + arthropods + unknown) was higher near Grevillea than Cordia.
Discussion: While unexpected, these results demonstrated that predators readily attacked sentinel pests on coffee shrubs under both shade tree species. The two tree species may balance and complement each other in attracting avian and arthropod ecosystem providers, with Grevillea attracting arthropod predators and generalist birds, and Cordia attracting insectivorous specialists.
Introduction
Agricultural expansion is the most pervasive contributor to global biodiversity decline, impacting up to 80% of bird, amphibian, and mammal species (Newbold et al., 2015; Joppa et al., 2016; Tilman et al., 2017; Harfoot et al., 2021). This is especially concerning in tropical biodiversity hotspots including the mountains and foothills of East Africa (Otieno et al., 2011; Mulwa et al., 2021), where widespread agricultural land conversion has led to significant biodiversity loss (Ndang’ang’a et al., 2013).
Amidst ongoing agricultural expansion, agroforestry systems such as shade coffee have emerged as more sustainable alternatives to intensive agriculture (Perfecto et al., 1996; Bhagwat et al., 2008; Gonzalez et al., 2024). Coffee plantations grown beneath shade trees, compared to sun-grown coffee systems, generally support greater diversity and abundance of insects (Perfecto et al., 1996; Philpott et al., 2008), mammals (Caudill and Rice, 2016), and especially birds (De Beenhouwer et al., 2013; Buechley et al., 2015), though some regional differences exist, and benefits may be reduced in landscapes characterized by large pools of open-country species (Smith et al., 2015). Some work also suggests that conservation of forests adjacent to relative intensive coffee cultivation (i.e., land sparing approach) can be good for conservation (Chandler et al., 2013). While shade coffee farms cannot fully replace the specialized habitats needed by many endemic insectivorous birds and understory species (Greenberg et al., 1997; Powell et al., 2015; Ong’ondo et al., 2022), evidence suggests they can harbor some bird and arthropod species that in turn can help control insect pests, including the world’s premier coffee pest, the coffee berry borer (Coleoptera: Curculionidae: Scolytinae Hypothenemus hampei, Ferrari 1876; Abasa, 1975; Kellermann et al., 2008; Karp et al., 2013; Morris et al., 2015; Escobar-Ramírez et al., 2019). In contrast the release of biological control agents such as predator wasps and parasitoids, the management of habitat to favor existing native species of natural enemies of pests (aka conservation biological control), especially birds and ants, has received extensive recent research (e.g., Cowal et al., 2023; Muccio et al., 2024; Moreno-Ramirez et al., 2024) These results are encouraging because coffee is one of the most widely consumed global commodities, with production spanning 10 million ha in tropical regions. Global coffee production exceeded 10 billion kg in 2022/2023, with the majority (about 73%) coming from smallholder farms of less than 5 ha (ICO, 2023).
Though shaded coffee farms have conservation benefits over sun coffee farms, the term shade coffee belies tremendous variation among and within farms that contain shade trees (Moguel and Toledo, 1999; Kammerichs-Berke et al., 2022). On small farms, native (i.e., indigenous) trees are often incorporated as part of a more rustic management approach, either as legacy remnants of the former forest or intentionally planted within a farm (Moguel and Toledo, 1999). In contrast, larger plantations, including those established during the colonial era of many countries in the Global South, frequently adopt a “shaded monoculture” approach, relying on one or a few tree species cultivated at regular intervals among coffee rows (Moguel and Toledo, 1999). Globally, a relatively small number of shade tree species dominate shaded monocultural coffee systems, such as Grevillea robusta in Kenya, Uganda, India, and Brazil (Baggio et al., 1997; Muchiri, 2004; Kiyingi et al., 2016; Sinu et al., 2021), Inga species in Mexico and Jamaica (Johnson, 2000; Romero-Alvarado et al., 2000), and Albizia spp., Erythrina poeppigiana, and Mangifera indica in some areas of Central and South America (Perfecto and Vandermeer, 2015; Narango et al., 2019).
The choice of shade tree species has significant implications for both farmers and wildlife. Farmers tend to select tree species based on the ecological and economic benefits they provide, such as improving microclimates that can enhance crop yields (Beer, 1987; Soto-Pinto et al., 2000; Takaoka, 2008; Pinard et al., 2014). Shade trees can also offer additional products like fruit and timber, which can complement revenue for smaller land holdings and buffer them from the economic instability of coffee production (Davis et al., 2017). Additionally, research suggests that shade trees may assist farmers in adapting to climate change (Schooler et al., 2020).
The ecological characteristics of specific shade tree species also play a role in managing coffee pests by attracting natural predators, including birds and ants (Kellermann et al., 2008; Railsback and Johnson, 2014; Jaramillo et al., 2011). For example, Johnson (2000) found that coffee plantations in Jamaica with a dominance of native Inga species supported higher populations of birds and beneficial arthropods. Similarly, research in central Kenya identified greater densities of non-pest arthropods on native Cordia compared to non-native Grevillea trees (Kammerichs-Berke et al., 2022). Ants can be important agents of biological control in coffee farms (Vandermeer et al., 2002; Philpott et al., 2006; Milligan et al., 2016; Cowal et al., 2023), and De la Mora et al. (2015) documented higher diversity of ant species and functional guilds in coffee shaded by native trees in Latin America. This aligns with ecological theories of insect coevolution with plants (Southwood et al., 1982; Tallamy, 2004; Tallamy and Shropshire, 2009) suggesting that native host plants are a key driver of insect diversification (Becerra and Venable, 1999), and most herbivorous insects specialize on a few native plant species with which they share a long evolutionary history (Bernays and Graham, 1988), especially in tropical regions (Schemske et al., 2009). As a result, ecosystems dominated by non-native evolutionarily novel plants generally support lower insect diversity, abundance, and biomass compared to those dominated by native plants (Burghardt et al., 2010; Litt et al., 2014). This has important implications for selecting shade tree species and their impact on insectivorous birds and arthropods in coffee farms (Narango et al., 2018).
In central Kenya, two commonly used trees in shaded coffee systems are Grevillea robusta and various species of Cordia, particularly Cordia africana (Muchiri, 2004; Mayoli and Gitau, 2012). Grevillea, introduced from eastern Australia in the 19th century, is popular due to its fast growth (up to 3 m per year in some areas) and its tall structure, which provides effective wind protection (Negash, 1995). Cordia, an evergreen native to East Africa, has a broader canopy and larger leaves than Grevillea, providing more shade. Both species are often planted in evenly spaced rows among understory coffee plants and are valued for their nitrogen-fixing capabilities (Negash, 1995; Lott et al., 2000). Recent work by Kammerichs-Berke et al. (2022) showed that abundance of foliage-dwelling arthropods, and the species richness and overall abundance of foraging birds were all higher on native Cordia than on Grevillea, prompting the hypothesis that native Cordia may be better at mitigating habitat loss and attracting natural enemies that could promote pest control services.
Sentinel pest experiments can help quantify ecosystem services by examining predation pressure on agropests by wildlife predators, such as insectivorous birds. By affixing “sentinel pests” on study sites, we can investigate pest removal and pest attack rates by predators. Sentinel pest experiments can be conducted using live (e.g., Milligan et al., 2016) or artificial caterpillars (Nimalrathna et al., 2023), which each have the benefits and drawbacks (Nagy et al., 2020). For example, rearing and keeping pests alive can be challenging, and predation of live pests often leaves no trace, or only fragments of the consumed prey can be found (Howe et al., 2009). A key benefit of using plasticine models is that the clay is malleable and does not harden, which allows predation events to be preserved (Howe et al., 2009). The malleability of the plasticine will also allow observation of marks caused by predator’s mandibles, teeth, or beaks (Nagy et al., 2020). Numerous studies suggest that artificial caterpillars can provide quick, easy, and reliable information on diversity and community of predators and predation rates in managed and natural habitats (Low et al., 2014; Posa et al., 2007; Sam et al., 2015; Seifert et al., 2015; Tvardikova and Novotny, 2012; Sinu et al., 2021). Although caterpillar pests are relatively rare for coffee plants (Perfecto et al., 2004; Milligan et al., 2016), they are still present on surrounding vegetation (X. Gil, pers. obs.) and are an important food sources for birds (Greenberg, 1995). Sentinel pest experiments have been shown to reveal the community of generalist predators of coffee pests, differentiating between ant and bird predation (Milligan et al., 2016; Sinu et al., 2021).
In this study, we assessed the hypothesis that avian and arthropod pest control services on Kenyan shade-coffee farms are more pronounced near native than non-native shade trees based on previous work by Kammerichs-Berke et al. (2022) who examined native Cordia and non-native Grevillea shade trees. Specifically we used an artificial sentinel pest experiment to test the prediction that predation rates would be higher near native Cordia shade trees than near the non-native shade tree Grevillea.
Materials and methods
Study area
The study took place in the highlands and foothills of Kiambu County in central Kenya. Coffee farm sites were in the vicinity of the towns of Limuru and Thika. Surrounding areas consisted of agricultural farms, forest patches, and urbanized and rural towns. Agricultural farms in this region predominately produce coffee and tea. The dry season usually runs from December to March in Kenya (Ayugi et al., 2016; Mumo et al., 2018), with rain typically scarce. Annual rain varies between 750 and 1500 mm in March through May (long rains) and between September and November (short rains; Ayugi et al., 2016; Mumo et al., 2018). However, rainfall during the time of the study was characterized by enhanced, above-average rainfall (KMD, 2020; Wainwright et al., 2020).
Predation sampling was carried out at 6 shade coffee sites located along elevational gradient (1,567–1,874 m); Tinganga, Kamundu 1 and 2 (this large farm was split into two distinct survey sites), Gulmarg, Ruiru, and Theta. Farms had a mean of ~20% shade cover primarily by Cordia and Grevillea, with occasional Albizia gummifera, and intercrops of peas, Pisum sativum, and beans, Phaseolus vulgaris, between coffee plants. We performed the sentinel pest experiment during what is normally the dry season for four and half weeks from December 2019 to January 2020, corresponding to when many coffee berries are growing and susceptible to coffee pests. Three of the six farms had active coffee pickers while experiments were in place, though we timed pest deployment to minimize coincidence with workers, choosing the date of experiment to occur when workers were picking in a different plot. There were no significant differences in predation rates between farms with and without pickers (Gill, unpubl.).
Sentinel pest experiment
We performed a sentinel pest experiment of plasticine “caterpillars” (Figure 1) to gauge predation response to a simulated dramatic increase of a novel pest on coffee bushes surrounding the canopy of Cordia and Grevillea shade trees. Sentinel pests consisted of simulated Lepidopteran caterpillars made of plasticine modeling clay (Sargent Art Plastilina Modeling Clay, Van Aken, North Charleston, SC) in the color green in order to remain comparable to other studies (Posa et al., 2007; Howe et al., 2009; Ferrante et al., 2014; Roslin et al., 2017; Muchula et al., 2019). In addition, green caterpillars seem to be perceived by predators as palatable and undefended prey (Howe et al., 2009). Sentinel pests were constructed using a mini stainless-steel extruder (HEVERP Clay & Sugar Paste Extruder, Guang Dong, China) to create long strips of clay. The strips of clay were then cut to a length of 1.5 cm, and molded to resemble arched, geometrid caterpillars (Geometridae; inchworms).
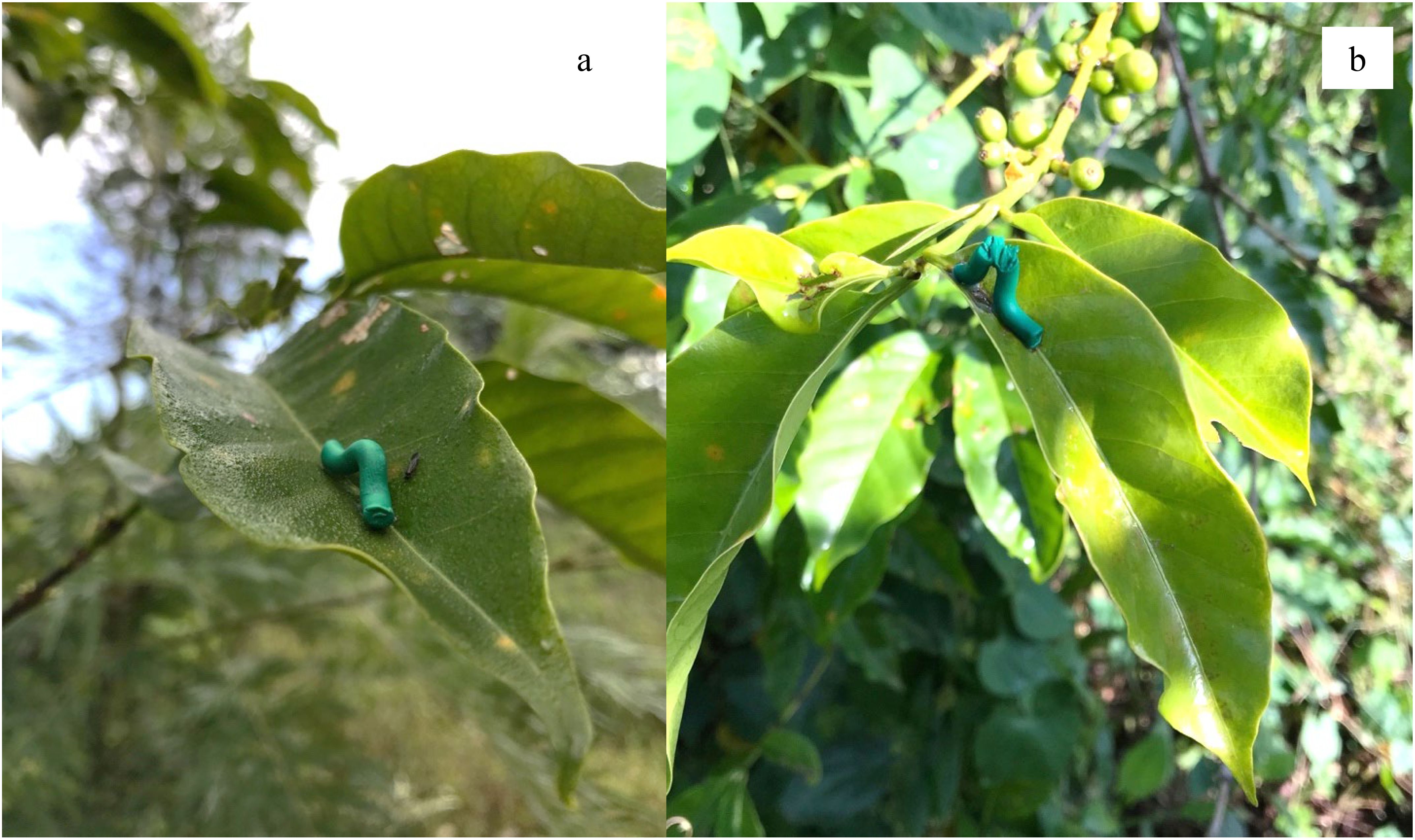
Figure 1. (a) Sentinel pest presentation on coffee shrubs. (b) Artificial plasticine caterpillar showing signs of bird predation, Kiambu County, Kenya, December and January 2019–2020.
On each of the 6 farms, 20 shade trees (10 Cordia and 10 Grevillea) were selected. Selected trees were at least 10 m apart to preserve independence, since the caterpillars simulated (Geometrids) rarely leave their host plants except to pupate (Wagner and Hoyt, 2022). We placed 15 sentinel pests near each tree, with 3 pests deployed on each of 5 coffee shrubs surrounding the canopy on each tree) for a total of 300 sentinel pests per farm site (15/tree × 20 trees). At each tree site, we chose the closest 5 coffee shrubs surrounding the outside canopy of the tree for the sentinel pest presentation stations. Three plasticine caterpillars were affixed to different leaves on the same coffee plant (Figure 2), placed at a minimum distance of approximately 15–30 cm apart, on the outer 2/3 of the branches and at a height of ~1.5 m. All sentinel pests were placed facing the selected study tree and adhered with super glue (Loctite Super Glue) to the dorsal side of a coffee leaf near the midrib. Sentinel pests were deployed in the morning starting at 08:00 a.m., left exposed for 24 hours, and then collected. Flagging tape was used to aid in relocation of sentinel pests. Care was taken to protect the plasticine from accidental marking by handling during setup and takedown. Ziploc bags were used to transport the sentinel pests into the field and labeled 1.5 ml micro-centrifuge tubes (BioChrom, Jiangsu Kangjian Medical Apparatus Co.) were used to transport the plasticine caterpillars back to the lab. In cases where the glue was especially thick and strong and removal of a sentinel pest would result in spoiling the sample, the whole leaf was removed and stored a Ziploc bag.
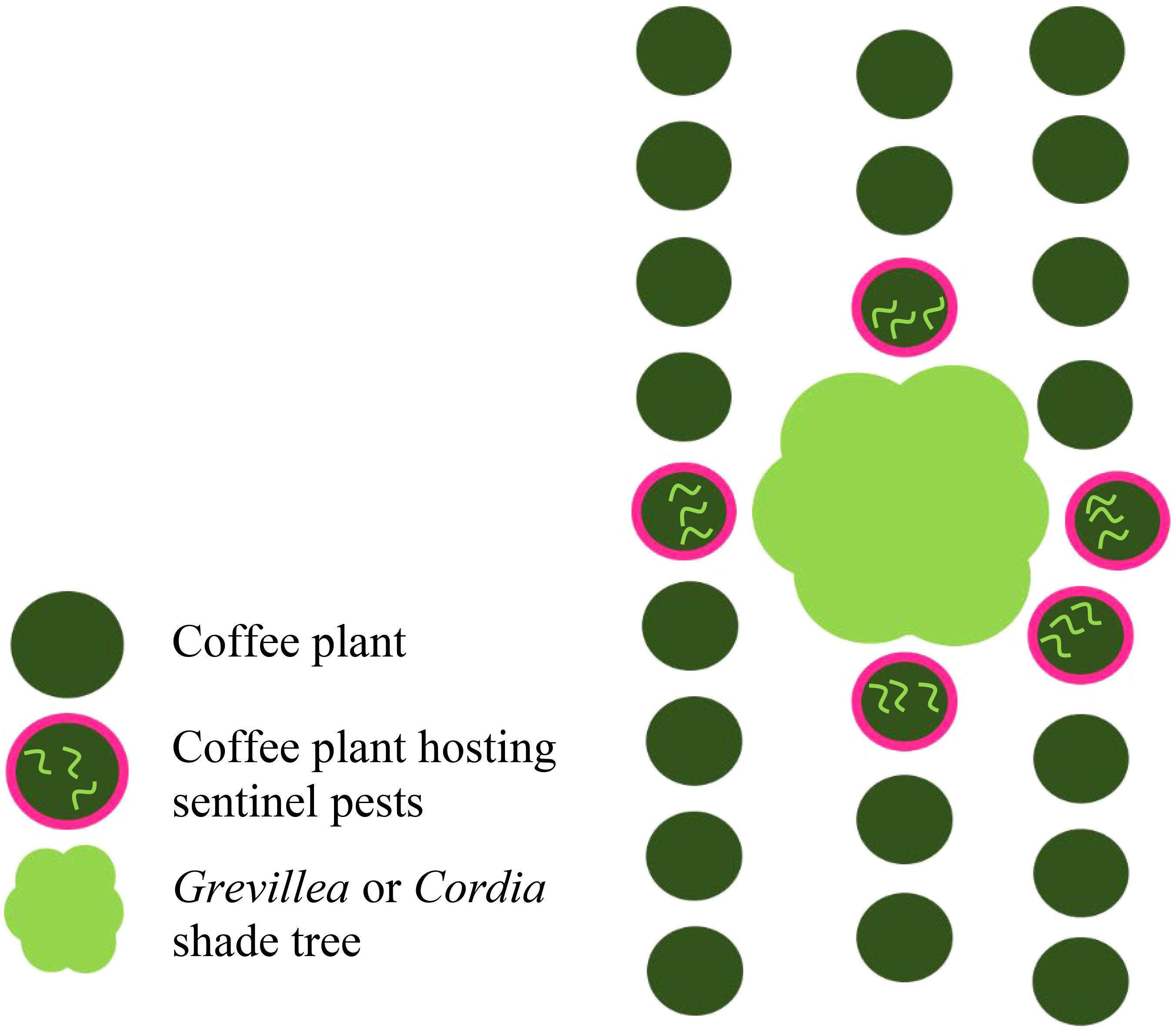
Figure 2. Sentinel pest host shrubs (red) on a shade-coffee farm. At each Cordia and Grevillea tree site (10 on each of 6 farms), the 5 closest coffee plants surrounding the outside canopy of the tree site were chosen to each host 3 sentinel pests per plant for a total of 15 sentinel pests per shade tree site. Study was conducted in Kiambu County, Kenya, December and January 2019–2020.
Once transported back to the lab, marks on sentinel pests were reviewed for predation events with a magnifying lens. A predation event was assigned to samples if they displayed beak or bite marks from birds, arthropods, or other unknown marks. Assignment to predator groups was determined by characteristic marks on plasticine caterpillars. Marks from birds were distinguished by u-shaped or v-shaped beak marks (Howe et al., 2009; Low et al., 2014). Stronger attacks from birds may also result in chunks being removed from the sample. Marks by arthropods were characterized by small slits, scrape marks, stab holes, or paired pincer marks from mandibles (Howe et al., 2009; Low et al., 2014). Ambiguous marks were classified as unknown. Because sentinel pests were left out for 24 hours, multiple predators could attack a single pest or a single predator could attack repeatedly and leave multiple marks, creating multiple predation marks on the same sample. All the field protocols were approved following Humboldt State University Institutional Animal Care and Use Committee (IACUC) permission number 16/17. W306-A.
Statistical analysis
We used two analyses to investigate predation pressure of sentinel pests by insectivorous predators. The first analysis examined the binary response of the presence or absence of predation events, wherein each caterpillar was recorded as marked (1) or unmarked (0) by a predator, using a Generalized Linear Mixed Model (GLMM) with a binomial distribution. The second analysis examined the intensity of predation events, quantified as the number of strikes observed on a caterpillar, by using a GLMM with a negative binomial distribution. For brevity, the first binary response variable is hereafter referred to as frequency of predation, and the second count response referred to as intensity of predation. For both response variables, analysis was performed separately for bird predation, arthropod predation, and total predation which also included predation by unknown sources.
Both frequency and intensity of predation were analyzed to quantify the main effect of shade tree species (factor with two levels: Cordia and Grevillea), on predation with farm (6), tree number (20 per farm), and coffee shrub (5 per tree) modeled as nested random effects. In addition, farm elevation (m), average site temperature (°C), and rain (raining or not raining) were considered as fixed covariates to account for their potential effects, though temperature and rain were collinear so we retained only rain in the final candidate model set (Table 1). Thus, we ran a total of six candidate model sets: three predator groups (arthropods, birds, total) for each of two response variables (frequency and intensity of predation). All model assumptions were verified with the DHARMA package (Hartig, 2022) and all models were run using the function glmer in the lme4 package (Bates et al., 2015) in the software R (R Core Team 2020). The top model(s) were based on the lowest Akaike Information Criterion score corrected for small sample size (AICc). To consider whether the predation by birds could influence the predation rate by arthropods, for example by bird suppressing predatory arthropods (i.e., intraguild predation), we ran added the bird predation rate as a predictor to the top model of arthropod predation rate identified in the candidate model set. Models within two ΔAICc of the top model were considered competitive and were model-averaged using the MuMIn package (Barton, 2023).
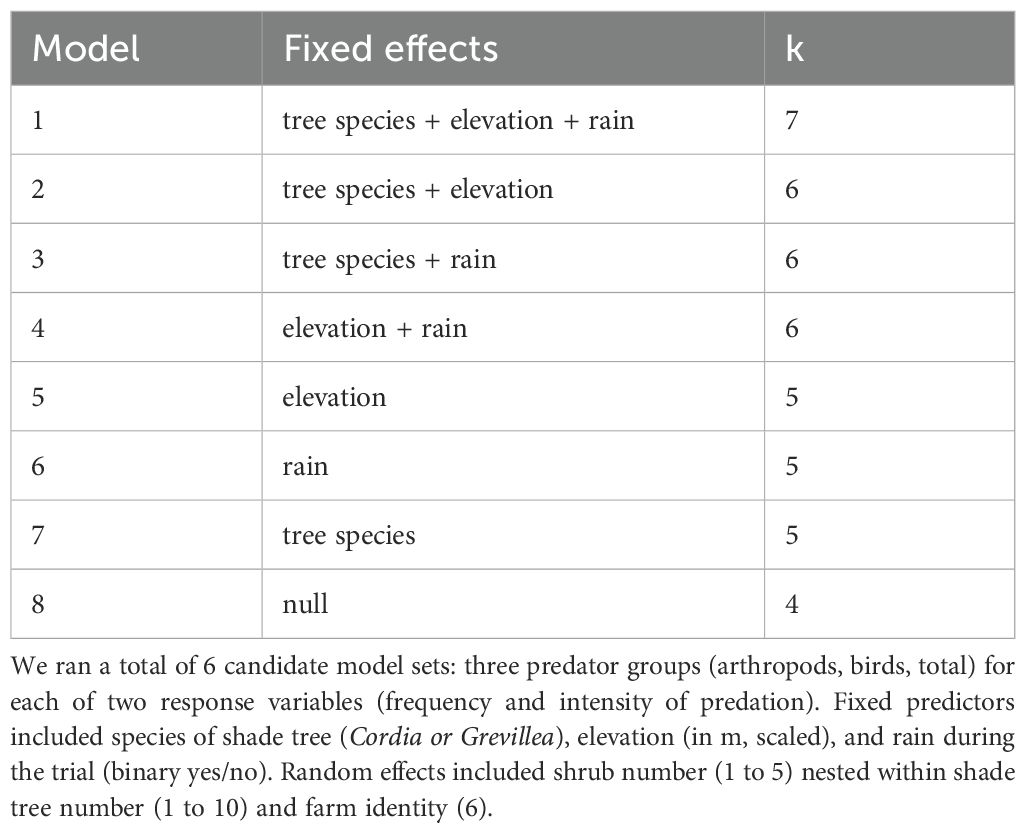
Table 1. Candidate model set and number of parameters (k) for analysis of predation on sentinel pests in 6 coffee farms in Kenya, 2019–2020.
Results
Of the total of 1800 sentinel pests deployed among six farms, 46 (on Cordia) and 80 (on Grevillea) were lost and unable to be recovered. Among recovered samples, 72 (8.4%) of the Cordia pests and 93 (11.3%) of the Grevillea pests showed signs of predation. Among these 165 sentinel pests depredated, there were 172 identifiable individual predation events, with 56 (32.6%) attributed to birds, 81 (47.1%) to arthropods, and 35 (20.3%) unknown predation events (Table 2). The overall frequency of predation confirmed by arthropods and birds was 4.8% and 3.3%, respectively. These 172 individual predation events resulted from a total of 315 individual marks on sentinel pests by all predators (Table 2).
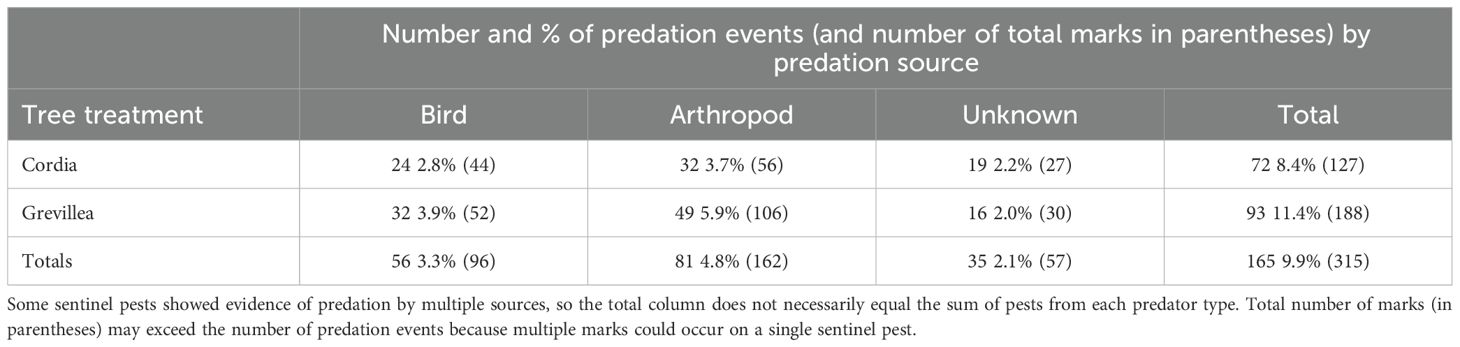
Table 2. Number of sentinel pests with evidence of a predation event, along with the percent of retrieved pests from coffee shrubs under Cordia (n = 854) and Grevillea (n = 819) shade trees (and the total number of predation marks in parentheses) by predator type on shade-coffee farms in Kiambu County, Kenya, December and January 2019–2020.
Frequency of predation
Overall, predation frequency on sentinel pests showed some evidence of being higher on shrubs under Grevillea than under Cordia, though this effect was weaker for birds than for both arthropods and predators overall (Table 3; Figure 3). There was relatively little resolution in the candidate model set for bird predation frequency, with five models included within ΔAICc of the top model, which was the null model (Supplementary Table S1). Model-average coefficients suggested a trend (± 1 SE did not overlap zero) of more frequent bird predation on shrubs under Grevillea than under Cordia and on days with rain, but the 95% confidence intervals for both variables overlapped zero (Table 3). The top four models for arthropod predation frequency all included tree species, with and without the inclusion of elevation and rain as predictors (Supplementary Material S1). The model-averaged coefficients indicated that the frequency of predation was significantly higher on shrubs under Grevillea than those under Cordia, with a 95% confidence interval that did not overlap zero (Table 3). The beta coefficient for tree species (0.52) corresponded to a 68% increase in the probability that a sentinel pest was depredated under Grevillea than under Cordia (Figure 3). Predation by arthropods tended to increase with elevation and the presence of rain, but the 95% confidence intervals for both variables overlapped zero (Table 3). When the bird predation rate was added as a predictor variable to this top model, the fit of the model improved (ΔAICc = 2.22), with arthropod predation rate positively associated with bird predation (β = 1.01 ± 0.45); the effects of tree species and rain were similar to the top model. The top model for the frequency of all predation events, which included unknown predators, included the single variable shade tree species, though models that also included elevation and the presence of rain were competitive (Supplementary Table S1). Model-averaged coefficients indicated that the frequency of predation was significantly higher (by 42%) on shrubs under Grevillea than those under Cordia, with a 95% confidence interval that did not overlap zero (Table 3). Again, predation frequency tended to increase with elevation and the presence of rain, but the 95% confidence intervals for both variables overlapped zero (Table 3).
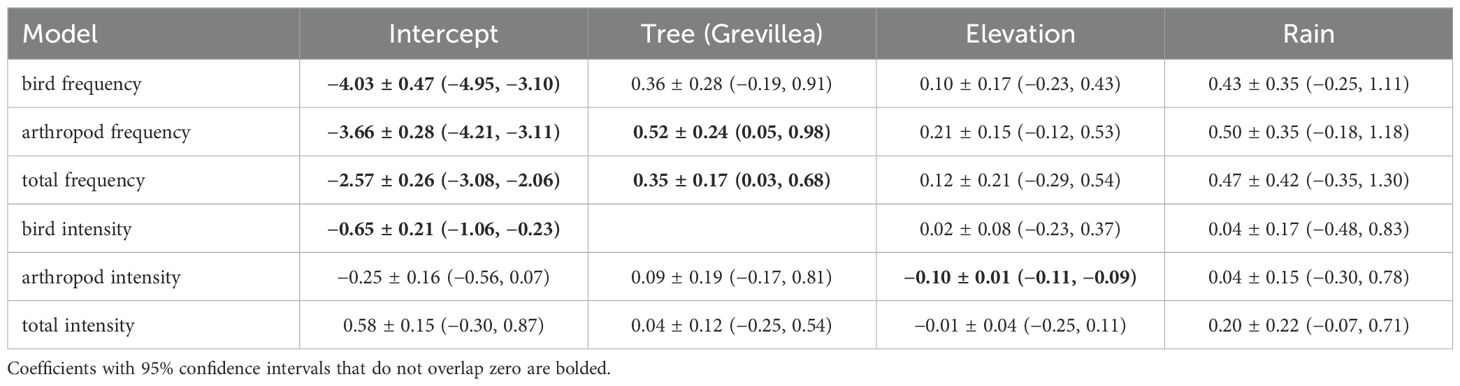
Table 3. Model-averaged coefficients ± 1 SE (upper, lower 95% confidence limit) for the frequency and intensity of predation on sentinel pests by birds, arthropods, and total predators (birds + arthropods + unknown) on shade-coffee farms in Kiambu County, Kenya, December and January 2019–2020.
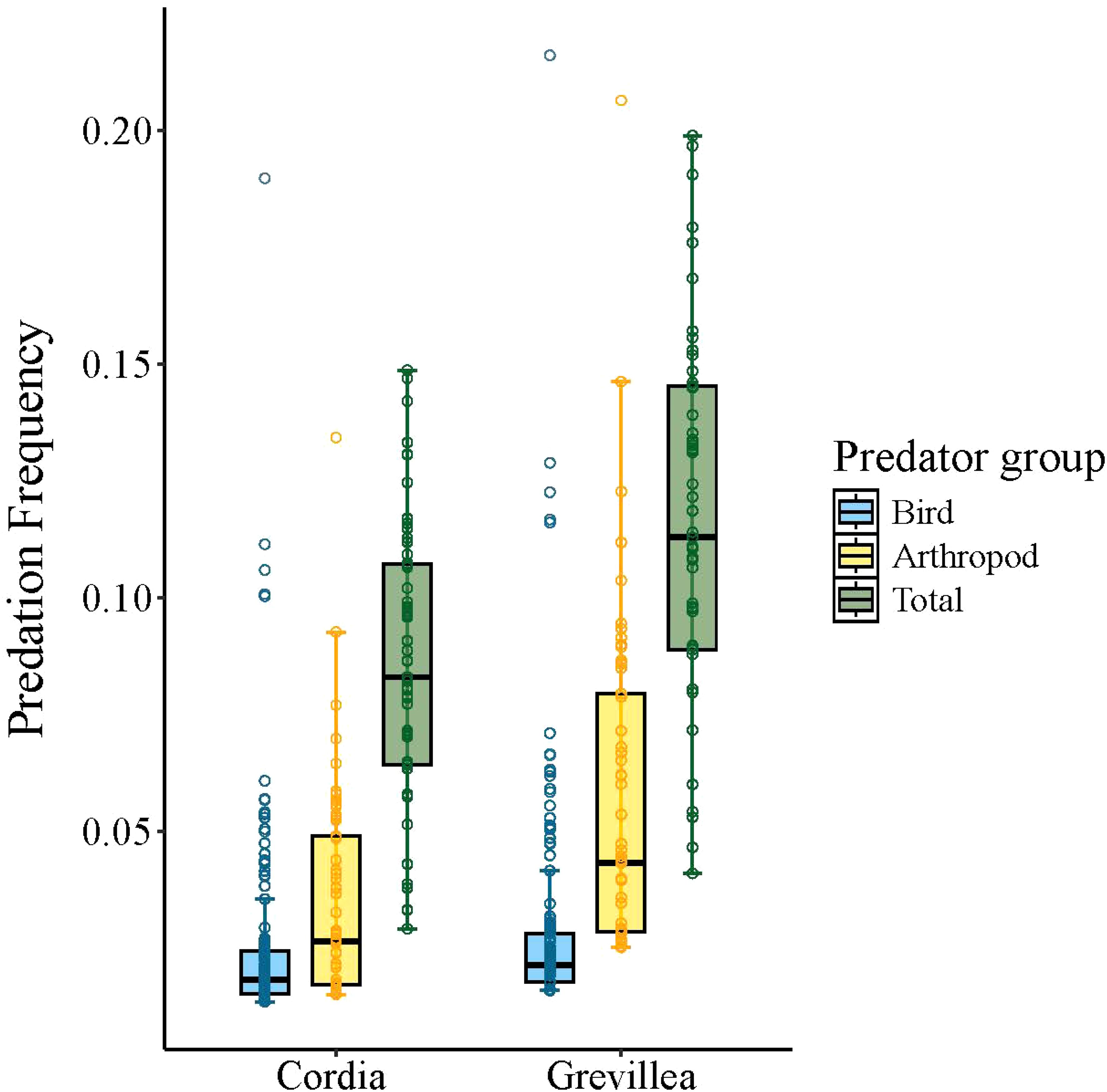
Figure 3. Boxplots of predation probability for birds (blue, 56 predation events), arthropods (gold, 81 predation events), and total predation (green, 172 predation events) between Cordia and Grevillea shade tree treatments at shade-coffee farms in Kiambu County, Kenya, December and January 2019–2020. Predation probabilities were calculated using model-averaged binomial models for each predator type. All other continuous variables in top models were held at their average and rain was considered absent. Boxes represent interquartile range, whiskers represent upper and lower quartile ranges, and circles represent outlier values.
Intensity of predation
Overall, predation intensity showed little consistent variation with shade tree species, elevation, and rain (Table 3). The top three models in the candidate set for the intensity of bird predation included only elevation and/or the presence of rain (Supplementary Table S1), though both of these variables had coefficients that overlapped zero (Table 3). There was very little evidence that predation intensity by birds varied with shade tree species. The top models for predation intensity by arthropods included the null model as well as models with the single variable elevation, rain, or tree species (Supplementary Table S1). The weight of evidence for a significant difference was weak, however, as all these coefficients had standard errors and 95% confidence intervals that overlapped zero. Similarly, the top four models for total predation intensity included the null model, and coefficients for all variables among competitive models had SEs and 95% confidence intervals that overlapped zero (Table 3).
Discussion
Our results suggest that birds and arthropods readily attacked sentinel pests on coffee shrubs near native Cordia and non-native Grevillea shade trees on coffee farms in Kenya. However, we found no evidence to support our hypothesis that predation rates were higher near native Cordia versus non-native Grevillea. Instead, the results suggest that sentinel pests near Grevillea were more likely to depredated by arthropods and all predators combined. The intensity of predation (number of strikes in plasticine caterpillars) did not show consistent variation with tree species. These results were unexpected, especially since foraging “hot spots” are often made up of vegetative components that have evolved with local food webs and ecosystems (Tallamy, 2004; Narango et al., 2017, 2019). Non-native plants and trees that lack this coevolutionary relationship might therefore support a lower abundance of prey items for avian and arthropod predators (Narango et al., 2019). A companion study showed higher foraging activity by insectivorous birds in Cordia compared to Grevillea trees, though bird abundance in the coffee layer was similar regardless of shade tree species (Kammerichs-Berke et al., 2022). Despite theoretical reasons to suspect higher predation under native than non-native shade trees, our results are consistent with a similar experiment recently done by Sinu et al. (2021) in the Western Ghats of India, where they found similar predation rates with plasticine sentinel pests on coffee shrubs grown under a diverse mix of native shade trees versus shrubs under Grevillea, though their predation rates were higher than ours. Like in our study, they found that most predation was by arthropods, though they also had substantial predation by lizards, which were rare in our system. The only lizard species seen at our study sites were < 5 Jackson’s chameleons (Trioceros jacksonii) seen across all farms over a 6-week field season.
We observed frequencies of predation of 3.3% and 4.8% per 24 h period for arthropods and birds, respectively, fell within the range found elsewhere by other studies in other regions on different crops. For example, Howe et al. (2009) reported an overall predation rate of 3.8% on cotton in Uganda, with 88% of that from arthropods. Similarly, Howe et al. (2015) found an overall 2–4% predation rate per day on cotton plants, with arthropods dominating. Lemessa et al. (2015) had mean daily predation rate of 1.5% for birds and 1.6% for arthropods. Tadesse et al. (2023) reported a 31.6% attack rate for arthropods, while the artificial caterpillars attacked by birds were at 22.7%, though their design left the caterpillars out for 30 days. A review paper by Lövei and Ferrante (2017) reported an overall median predation rate of 8.8% per day.
Effects of tree architecture and leaf morphology on the visibility of immobile sentinel prey may have contributed to our observed outcome of similar bird predation on sentinel pests between shade trees despite evidence from Kammerichs-Berke et al. (2022) for more bird activity and more insect prey on Cordia. For instance, Cordia’s canopy is more rounded and dense compared to Grevillea’s, which has a narrower and more open-structure canopy composed of fern-like pinnately compound leaves. The coffee layer surrounding Grevillea might therefore be more visible and more easily navigable for foraging birds and open-flyers, potentially making it easier to locate and depredate sentinel pests. An experimental study on sentinel clay spiders found that bird attack rates were significantly higher in microhabitats with low leaf litter and understory cover (Mason et al., 2018). Birds rely on visual cues for hunting far more than arthropod predators (Sam et al., 2015), so this proposed explanation is unlikely to apply to the higher frequency arthropod-caused predation on sentinel pests we observed.
The frequency of arthropod predation was significantly higher on coffee shrubs near Grevillea trees than near Cordia. On possible explanation for this result could arise from a vegetation structure on and under Grevillea that favors insect predators. Kammerichs-Berke et al. (2022) found that temperatures were higher (and less buffered) under Grevillea than under Cordia, which perhaps favors some insect predators, such as ants. Work in Central America suggests ant abundance and diversity tends to be higher in shaded than in sun-coffee (Philpott et al., 2006), but certain species (e.g., Hymenoptera: Formicidae: Solenopsis geminate, Fabricius 1804) are more associated with sun coffee (Perfecto et al., 1996). In this region of Kenya, Pheidole megacephala (Hymenoptera: Formicidae: Fabricius 1793) tends to be the dominant predatory ant in coffee farms (Milligan et al., 2016), but whether it is more common under Grevillea or Cordia is unknown. Regardless, the net effect of higher temperature under Grevillea could, over time, lead to more pests, as the economically damaging coffee berry borer is expected to increase its range and abundance with climate change (Jaramillo et al., 2011). Thus, farm managers should consider both the biotic and abiotic characteristics of shade trees species that could affect pest abundance, as well as the provisioning of additional services such as carbon sequestration, cooler microclimates, soil health, and mulch production (Nyberg and Högberg, 1995; Yadessa et al., 2008; Alemayehu et al., 2016; Schooler et al., 2020; Sinu et al., 2021; Kammerichs-Berke et al., 2022). Alternative explanation, for example the role of chemical attractants and volatile compounds from Grevillea that may attract insects, remains poorly understood, though its leaf extracts showed termiticidal activities against Heterotermes indicola (Afzal et al., 2019) and bark extracts have antioxidant, antibacterial, antifungal properties, so this seems unlikely. Nonetheless, this topic merits further investigation but was beyond the scope of this study.
Alternatively, intraguild predation could have complicated our results (Müller and Brodeur, 2002). For example, higher bird abundance on native Cordia (Kammerichs-Berke et al., 2022) could contribute to the reduction of populations of predatory arthropods (including ants and spiders) in turn releasing herbivorous prey such as caterpillars, from control. Intraguild predation has been shown to operate in shaded coffee systems, though perhaps more for bats than for bird predators (e.g., Karp et al., 2014). Though our study was not designed to test this idea explicitly, we did see that arthropod predation frequency was positively associated with bird predation, which is not consistent with the hypothesis that birds suppressed predatory arthropods. Understanding the net effect of insectivorous predators requires a better understanding of their diets, work that was traditionally laborious or impossible but increasingly feasible with recent advances in molecular scatology and high throughput sequencing (e.g., PCR as in Karp et al., 2014, or next generation sequencing and metabarcoding as in Jedlicka et al., 2017; Hoenig et al., 2022).
We might also consider the possibility that birds foraging in coffee bushes are those able to adapt to novel environments, and if so, may not be responsive to specific tree species. Some work suggest bolder, more neophilic individuals are best able to exploit novel environments (Holtmann et al., 2017). Insect communities associated with Cordia canopy and understory may be different from the coffee layer surrounding Grevillea due to changes in shade, temperature, and understory density (Withaningsih and Rabbany, 2019). Thus, Grevillea may attract a different bird community than those solely associated with Cordia’s canopy. Perhaps Grevillea’s understory and surrounding areas are better suited for novel environment seekers, with occasional visitations from more specialist insectivores (Greenberg, 1990).
Other contributing variables and factors that may have influenced the data include farm site management variation and human activity. This study was conducted over a 4-week period, limiting the assessment of temporal variation. Sentinel pest experiments were conducted during coffee picking, which could influence predation rates by the presence of coffee workers, though they moved quickly through coffee rows, rarely spending more than 20 min near our experimental shrubs. Moreover, human presence in coffee rows occurs nearly all weeks of the year in Kenya (picking, pruning, planting, understory maintenance, etc.), so human presence in the system is perhaps best considered a regular component part of the environment, rather than as an episodic disturbance. In addition, pickers were present at both Cordia and Grevillea sites, so their presence should not confound our comparison of shade tree species.
In conclusion, the hypothesis that the native shade tree, Cordia, would support higher predation rates on sentinel pests than the non-native shade tree, Grevillea, was not supported. Instead, we observed higher frequency of predation by arthropods and all predators combined on coffee shrubs near Grevillea. However, while we found no support for our hypothesis, we did find that predation attacks on artificial caterpillars occurred on coffee bushes surrounding both trees, providing evidence that avian and arthropod predators were utilizing both native and non-native shade trees and confirming this technique could be useful for future studies in the region. Furthermore, the findings of Kammerichs-Berke et al. (2022) and Milligan et al. (2016) suggest that insectivorous birds prefer Cordia over Grevillea, coupled with evidence that insectivorous birds can meaningfully reduce live pests in East African coffee (Classen et al., 2014; Milligan et al., 2016) suggest value for the native Cordia as a shade tree in coffee farms in Kenya. However, Grevillea may play role in mixed shade-tree systems, where it provides favorable conditions for other birds, such as generalists, and omnivorous residents, and predatory insects. The two tree species may balance and complement each other in attracting avian and arthropod ecosystem providers; with Grevillea attracting arthropod predators and generalist birds, and Cordia attracting insectivorous specialists.
Data availability statement
The raw data supporting the conclusions of this article will be made available by the authors, without undue reservation.
Ethics statement
All the field protocols were approved following Humboldt State University Institutional Animal Care and Use Committee (IACUC) permission number 16/17. W306-A. The study was conducted in accordance with the local legislation and institutional requirements.
Author contributions
XM: Conceptualization, Data curation, Formal Analysis, Investigation, Writing – original draft, Writing – review & editing. JJ: Funding acquisition, Investigation, Supervision, Writing – review & editing. SO: Writing – review & editing. MJ: Conceptualization, Funding acquisition, Investigation, Methodology, Project administration, Supervision, Writing – review & editing.
Funding
The author(s) declare that financial support was received for the research and/or publication of this article. Funding was provided by the National Science Foundation’s International Research Experience for Students.
Acknowledgments
We thank Sasini and Theta Country Farms where the study took place. The National Science Foundation International Research Experience for Undergraduates provided funding (#1657973). The work would not have been possible without the collaboration with the National Museums of Kenya, especially with Frank Ong’ondo, Peter Njoroge, and Edson Mlamba. Deven Kammerichs-Berke provided excellent field training, and Chad Moura provided statistical advice.
Conflict of interest
The authors declare that the research was conducted in the absence of any commercial or financial relationships that could be construed as a potential conflict of interest.
Generative AI statement
The author(s) declare that no Generative AI was used in the creation of this manuscript.
Publisher’s note
All claims expressed in this article are solely those of the authors and do not necessarily represent those of their affiliated organizations, or those of the publisher, the editors and the reviewers. Any product that may be evaluated in this article, or claim that may be made by its manufacturer, is not guaranteed or endorsed by the publisher.
Supplementary material
The Supplementary Material for this article can be found online at: https://www.frontiersin.org/articles/10.3389/fcosc.2025.1529450/full#supplementary-material
References
Abasa R. O. (1975). A review of the biological control of coffee insect pests in Kenya. East Afr. Agric. Forestry J. 40, 292–299. doi: 10.1080/00128325.1975.11662747
Afzal M., Farman M., Rasib K. Z., Qureshi N. A. (2019). Biocidal action of silver oak (Grevillea robusta) leaf extract on the termite Heterotermes indicola Wasmann (Blattodea: Rhinotermitidae). Int. Biodeterioration Biodegradation 139, 1–10. doi: 10.1016/j.ibiod.2019.02.001
Alemayehu G., Asfaw Z., Kelbessa E. (2016). Cordia africana (Boraginaceae) in Ethiopia: A review on its taxonomy, distribution, ethnobotany, and conservation status. Int. J. Bot. Stud. 1, 38–46.
Ayugi B. O., Wen W., Chepkemoi D. (2016). Analysis of spatial and temporal patterns of rainfall variations over Kenya. J. Environ. Earth Sci. 6, 69–83.
Baggio A. J., Caramori P. H., Filho A., Montoya L. (1997). Productivity of southern Brazilian coffee plantations shaded by different stockings of Grevillea robusta. Agroforestry Syst. 37, 111–120. doi: 10.1023/A:1005814907546
Barton K. (2023). MuMIn: Multi-model inference (R package version 1.47.1). Available online at: https://CRAN.R-project.org/package=MuMIn (Accessed February 2, 2025).
Bates D., Maechler M., Bolker B., Walker S., Christensen R. H. B., Singmann H., et al 2015. Package ‘lme4’. Convergence. 12 (1), 2.
Becerra J. X., Venable D. L. (1999). Macroevolution of insect-plant associations: The relevance of host biogeography to host affiliation. Proc. Natl. Acad. Sci. 96, 12626–12631. doi: 10.1073/pnas.96.22.12626
Beer J. (1987). Advantages, disadvantages and desirable characteristics of shade trees for coffee, cocoa and tea. Agroforestry Syst. 5, 3–13. doi: 10.1007/BF00046410
Bernays E. M., Graham M. (1988). On the evolution of host specificity in phytophagous arthropods. Ecology 69, 886–892. doi: 10.2307/1941237
Bhagwat S. A., Willis K. J., Birks H. J. B., Whittaker R. J. (2008). Agroforestry: A refuge for tropical biodiversity? Trends Ecol. Evol. 23, 261–267.
Buechley E. R., Şekercioğlu Ç.H., Atickem A., Gebremichael G., Ndungu J. K., Mahamued B. A., et al. (2015). Importance of Ethiopian shade coffee farms for forest bird conservation. Biol. Conserv. 188, 50–60. doi: 10.1016/j.biocon.2015.01.011
Burghardt K. T., Tallamy D. W., Phillips C., Shropshire K. J. (2010). Non-native plants reduce abundance, richness, and host specialization in lepidopteran communities. Ecosphere 1, 1–22. doi: 10.1890/ES10-00032.1
Caudill S. A., Rice R. A. (2016). Do bird-friendly® coffee criteria benefit mammals? Assessment of mammal diversity in Chiapas, Mexico. PloS One 11, e0165662. doi: 10.1371/journal.pone.0165662
Chandler R. B., King D. I., Raudales R., Trubey R., Chandler C., Arce Chávez V. J. (2013). A small-scale land-sparing approach to conserving biological diversity in tropical agricultural landscapes. Conserv. Biol. 27, 785–795. doi: 10.1111/cobi.2013.27.issue-4
Classen A., Peters M. K., Ferger S. W., Helbig-Bonitz M., Schmack J. M., Maassen G., et al. (2014). Complementary ecosystem services provided by pest predators and pollinators increase quantity and quality of coffee yields. Proc. R. Soc. B: Biol. Sci. 281, 20133148.
Cowal S., Morris J. R., Jiménez-Soto E., Philpott S. M. (2023). Naturally occurring vegetation connectivity facilitates ant-mediated coffee berry borer removal. Insects 14, 869. doi: 10.3390/insects14110869
Davis H., Rice R., Rockwood L., Wood T., Marra P. (2017). The economic potential of fruit trees as shade in blue mountain coffee agroecosystems of the Yallahs River watershed, Jamaica W.I. Agroforestry Syst. 93, 581–589. doi: 10.1007/s10457-017-0152-z
De Beenhouwer M., Aerts R., Honnay O. (2013). A global meta-analysis of the biodiversity and ecosystem service benefits of coffee and cacao agroforestry. Agriculture, ecosystems & environment 175, 1–7.
De la Mora A., García-Ballinas J. A., Philpott S. M. (2015). Local, landscape, and diversity drivers of predation services provided by ants in a coffee landscape in Chiapas, Mexico. Agriculture Ecosyst. Environ. 201, 83–91. doi: 10.1016/j.agee.2014.11.006
Escobar-Ramírez S., Grass I., Armbrecht I., Tscharntke T. (2019). Biological control of the coffee berry borer: Main natural enemies, control success, and landscape influence. Biol. Control 136, 103992. doi: 10.1016/j.biocontrol.2019.05.011
Ferrante M., Lo Cacciato A., Lövei G. L. (2014). Quantifying predation pressure along an urbanization gradient in Denmark using artificial caterpillars. Eur. J. Entomology 111, 649–654. doi: 10.14411/eje.2014.082
Gonzalez C., Rodewald A. D., Arcese P., Bennett R. E., Hernandez-Aguilera J. N., Rueda X., et al. (2024). Effect of local habitat and landscape attributes on bird communities in shade coffee plantations in the Colombian Andes. Global Ecol. Conserv., e03207. doi: 10.1016/j.gecco.2024.e03207
Greenberg R. S. (1990). Ecological plasticity, neophobia, and resource use in birds. Stud. Avian Biol. 13, 431–437.
Greenberg R. (1995). Insectivorous migratory birds in tropical ecosystems: The breeding currency hypothesis. J. Avian Biol. 26, 260–264. doi: 10.2307/3677328
Greenberg R., Bichier P., Cruz-Angon A., Reitsma R. (1997). Bird populations in shade and sun coffee plantations in Central Guatemala. Conserv. Biol. 11, 448–459. doi: 10.1046/j.1523-1739.1997.95464.x
Harfoot M. B., Johnston A., Balmford A., Burgess N. D., Butchart S. H., Dias M. P., et al. (2021). Using the IUCN Red List to map threats to terrestrial vertebrates at global scale. Nat. Ecol. Evol. 5, 1510–1519. doi: 10.1038/s41559-021-01542-9
Hartig F. (2022). DHARMa: Residual diagnostics for hierarchical (multi-level/mixed) regression models (R package version 0.4.6). Available online at: https://CRAN.R-project.org/package=DHARMa (Accessed February 2, 2025).
Hoenig B. D., Snider A. M., Forsman A. M., Hobson K. A., Latta S. C., Miller E. T., et al. (2022). Current methods and future directions in avian diet analysis. Auk 139, ukab077. doi: 10.1093/ornithology/ukab077
Holtmann B., Santos E. S., Lara C. E., Nakagawa S. (2017). Personality-matching habitat choice, rather than behavioural plasticity, is a likely driver of a phenotype–environment covariance. Proc. R. Soc. B: Biol. Sci. 284, 20170943.
Howe A., Lövei G. L., Nachman G. (2009). Dummy caterpillars as a simple method to assess predation rates on invertebrates in a tropical agroecosystem. Entomologia Experimentalis Applicata 131, 325–329. doi: 10.1111/j.1570-7458.2009.00860.x
Howe A. G., Nachman G., Lövei G. L. (2015). Predation pressure in U gandan cotton fields measured by a sentinel prey method. Entomologia Experimentalis Applicata 154, 161–170. doi: 10.1111/eea.2015.154.issue-2
ICO International Coffee Organization (2023).Coffee report and outlook. Available online at: https://ico.org/resources/coffee-market-report-statistics-section/ (Accessed February 2, 2025).
Jaramillo J., Muchugu E., Vega F. E., Davis A., Borgemeister C., Chabi-Olaye A. (2011). Some like it hot: The influence and implications of climate change on coffee berry borer (Hypothenemus hampei) and coffee production in East Africa. PloS One 6, e24528. doi: 10.1371/journal.pone.0024528
Jedlicka J. A., Vo A. T. E., Almeida R. P. (2017). Molecular scatology and high-throughput sequencing reveal predominately herbivorous insects in the diets of adult and nestling Western Bluebirds (Sialia mexicana) in California vineyards. Auk: Ornithological Adv. 134, 116–127. doi: 10.1642/AUK-16-103.1
Johnson M. D. (2000). Effects of shade-tree species and crop structure on the winter arthropod and bird communities in a Jamaican shade coffee plantation. Biotropica 32, 133–145.
Joppa L. N., O’Connor B., Visconti P., Smith C., Geldmann J., Hoffmann M., et al. (2016). Filling in biodiversity threat gaps. Science 352, 416–418. doi: 10.1126/science.aaf3565
Kammerichs-Berke D., Lane F. J., Ong’ondo F. J., Mlamba E. M., Bean W. T., Jedlicka J. A., et al. (2022). The effect of shade tree species on bird communities in central Kenyan coffee farms. Bird Conserv. Int. 32, 655–673. doi: 10.1017/S0959270921000502
Karp D. S., Judson S., Daily G. C., Hadly E. A. (2014). Molecular diagnosis of bird-mediated pest consumption in tropical farmland. SpringerPlus 3, 1–8. doi: 10.1186/2193-1801-3-630
Karp D. S., Mendenhall C. D., Sandí R. F., Chaumont N., Ehrlich P. R., Hadly E. A., et al. (2013). Forest bolsters bird abundance, pest control and coffee yield. Ecol. Lett. 16, 1339–1347. doi: 10.1111/ele.2013.16.issue-11
Kellermann J. L., Johnson M. D., Stercho A. M., Hackett S. C. (2008). Ecological and economic services provided by birds on Jamaican Blue Mountain coffee farms. Conserv. Biol. 22, 1177–1185. doi: 10.1111/j.1523-1739.2008.00968.x
Kenya Meteorological Department [KMD] (2020). KMD homepage. Available online at: https://www.meteo.go.ke/index.php?q=home (Accessed 7 May 2020).
Kiyingi I., Ocama D., Mujuni D., Nyombi K. (2016). A bioeconomic analysis of the carbon sequestration potential of agroforestry systems: A case study Grevillea robusta in Western Uganda. Uganda J. Agric. Sci. 17, 219–229.
Lemessa D., Hambäck P. A., Hylander K. (2015). Arthropod but not bird predation in Ethiopian homegardens is higher in tree-poor than in tree-rich landscapes. PloS One 10, e0126639. doi: 10.1371/journal.pone.0126639
Litt A. R., Cord E. E., Fulbright T. E., Schuster G. L. (2014). Effects of invasive plants on arthropods. Conserv. Biol. 28, 1532–1549. doi: 10.1111/cobi.2014.28.issue-6
Lott J. E., Howard S. B., Ong C., Black C. R. (2000). Long-term productivity of a Grevillea robusta-based overstorey agroforestry system in semi-arid Kenya: II. Crop growth and system performance. For. Ecol. Manage. 139, 187–201. doi: 10.1016/S0378-1127(00)00267-X
Lövei G. L., Ferrante M. (2017). A review of the sentinel prey method as a way of quantifying invertebrate predation under field conditions. Insect Sci. 24, 528–542. doi: 10.1111/ins.2017.24.issue-4
Low P. A., Sam K., McArthur C., Posa M. R. C., Hochuli D. F. (2014). Determining predator identity from attack marks left in model caterpillars: Guidelines for best practice. Entomologia Experimentalis Applicata 152, 120–126. doi: 10.1111/eea.2014.152.issue-2
Mason L. D., Wardell-Johnson G., Luxton S. J., Bateman P. W. (2018). Predators show seasonal predilections for model clay spiders in an urban environment. Sci. Rep. 8, 12444. doi: 10.1038/s41598-018-30778-y
Mayoli R. N., Gitau K. M. (2012). The effects of shade trees on physiology of arabica coffee. Afr. J. Hortic. Science Kenya 6, 35–42.
Milligan M. C., Johnson M. D., Garfinkel M., Smith C. J., Njoroge P. (2016). Quantifying pest control services by birds and ants in Kenyan coffee farms. Biol. Conserv. 194, 58–65. doi: 10.1016/j.biocon.2015.11.028
Moguel P., Toledo V. M. (1999). Biodiversity conservation in traditional coffee systems in Mexico: A review. Conserv. Biol. 12, 1–11. doi: 10.1046/j.1523-1739.1999.97153.x
Moreno-Ramirez N., Bianchi F. J., Manzano M. R., Dicke M. (2024). Ecology and management of the coffee berry borer (Hypothenemus hampei): the potential of biological control. BioControl 69 (2), 199–214. doi: 10.1007/s10526-024-10253-6
Morris J. R., Vandermeer J., Perfecto I. (2015). A keystone ant species provides robust biological control of the coffee berry borer under varying pest densities. PloS One 10, e0142850. doi: 10.1371/journal.pone.0142850
Muccio K. R., Crone E. E., Reed J. M. (2024). A model of coffee berry borer population growth and susceptibility to control by birds. Population Ecol. 66, 263–273. doi: 10.1002/1438-390X.12180
Muchiri M. N. (2004). Grevillea robusta in agroforestry systems in Kenya. J. Trop. For. Sci. 16, 396–401.
Muchula K., Xie G., Gurr G. M. (2019). Ambient temperature affects the utility of plasticine caterpillar models as a tool to measure activity of predators across latitudinal and elevational gradients. Biol. Control 129, 12–17. doi: 10.1016/j.biocontrol.2018.11.006
Müller C. B., Brodeur J. (2002). Intraguild predation in biological control and conservation biology. Biol. Control 25, 216–223. doi: 10.1016/S1049-9644(02)00102-0
Mulwa M., Teucher M., Ulrich W., Habel J. C. (2021). Bird communities in a degraded forest biodiversity hotspot of East Africa. Biodiversity Conserv. 30, 2305–2318. doi: 10.1007/s10531-021-02190-y
Mumo L., Yu J., Fang K. (2018). Assessing impacts of seasonal climate variability on maize yield in Kenya. Int. J. Plant Production 12, 297–307. doi: 10.1007/s42106-018-0027-x
Nagy R. K., Schellhorn N. A., Zalucki M. P. (2020). Fresh, frozen or fake: A comparison of predation rates measured by various types of sentinel prey. J. Appl. Entomology 144, 407–416. doi: 10.1111/jen.v144.5
Narango D. L., Tallamy D. W., Marra P. P. (2017). Native plants improve breeding and foraging habitat for an insectivorous bird. Biol. Conserv. 213, 42–50. doi: 10.1016/j.biocon.2017.06.029
Narango D. L., Tallamy D. W., Marra P. P. (2018). Nonnative plants reduce population growth of an insectivorous bird. Proc. Natl. Acad. Sci. United States America 115, 11549–11554.
Narango D. L., Tallamy D. W., Snyder K. J., Rice R. A. (2019). Canopy tree preference by insectivorous birds in shade-coffee farms: Implications for migratory bird conservation. Biotropica 51, 1–12. doi: 10.1111/btp.2019.51.issue-3
Ndang’ang’a P. K., Njoroge J. B., Ngamau K., Kariuki W., Atkinson P. W., Vickery J. (2013). Effects of crop diversity on bird species richness and abundance in a highland East African agricultural landscape. Ostrich 84, 33–39. doi: 10.2989/00306525.2013.775189
Negash L. (1995). Indigenous trees of Ethiopia: biology, uses and propagation techniques. (Department of Biology, Addis Ababa University).
Newbold T., Hudson L. N., Hill S. L., Contu S., Lysenko I., Senior R. A., et al. (2015). Global effects of land use on local terrestrial biodiversity. Nature 520, 45–50. doi: 10.1038/nature14324
Nimalrathna T. S., Solina I. D., Mon A. M., Pomoim N., Bhadra S., Zvereva E. L., et al. (2023). Estimating predation pressure in ecological studies: Controlling bias imposed by using sentinel plasticine prey. Entomologia Experimentalis Applicata 171, 56–67. doi: 10.1111/eea.v171.1
Nyberg G., Högberg P. (1995). Effects of young agroforestry trees on soils in on-farm situations in western Kenya. Agroforestry Syst. 32, 45–52. doi: 10.1007/BF00713847
Ong’ondo F. J., Fogarty F. A., Njoroge P., Johnson M. D. (2022). Bird abundance and diversity in shade coffee and natural forest in Kenya. Global Ecol. Conserv. 39, e02296. doi: 10.1016/j.gecco.2022.e02296
Otieno N. E., Gichuki N., Farwig N., Kiboi S. (2011). The role of farm structure on bird assemblages around a Kenyan tropical rainforest. Afr. J. Ecol. 49, 410–417. doi: 10.1111/j.1365-2028.2011.01273.x
Perfecto I., Rice R. A., Greenberg R., Van der Voort M. E. (1996). Shade coffee: A disappearing refuge for biodiversity. BioScience 46, 598–608. doi: 10.2307/1312989
Perfecto I., Vandermeer J. (2015). Coffee agroecology: A new approach to understanding agricultural biodiversity, ecosystem services, and sustainable development (London: Routledge).
Perfecto I., Vandermeer J. H., Bautista G. L., Nuñez G. I., Greenberg R., Bichier P., et al. (2004). Greater predation in shaded coffee farms: The role of resident Neotropical birds. Ecology 85, 2677–2681. doi: 10.1890/03-3145
Philpott S. M., Arendt W. J., Armbrecht I., Bichier P., Diestch T. V., Gordon C., et al. (2008). Biodiversity loss in Latin American coffee landscapes: Review of the evidence on ants, birds, and trees. Conserv. Biol. 22, 1093–1105. doi: 10.1111/j.1523-1739.2008.01029.x
Philpott S. M., Perfecto I., Vandermeer J. (2006). Effects of management intensity and season on arboreal ant diversity and abundance in coffee agroecosystems. Biodiversity Conserv. 15, 139–155. doi: 10.1007/s10531-004-4247-2
Pinard F., Boffa J. M., Rwakagara E. (2014). Scattered shade trees improve low-input smallholder Arabica coffee productivity in the northern Lake Kivu region of Rwanda. Agroforestry Syst. 88, 707–718. doi: 10.1007/s10457-014-9712-7
Posa M. R. C., Sodhi N. S., Koh L. P. (2007). Predation on artificial nests and caterpillar models across a disturbance gradient in Subic Bay, Philippines. J. Trop. Ecol. 23, 27–33. doi: 10.1017/S0266467406003671
Powell L. L., Cordeiro N. J., Stratford J. A. (2015). Ecology and conservation of avian insectivores of the rainforest understory: A pantropical perspective. Biol. Conserv. 188, 1–10. doi: 10.1016/j.biocon.2015.03.025
Railsback S. F., Johnson M. D. (2014). Effects of land use on bird populations and pest control services on coffee farms. Proc. Natl. Acad. Sci. 111, 6109–6114. doi: 10.1073/pnas.1320957111
Romero-Alvarado Y., Soto-Pinto L., Garcia-Barrios L., Barrera-Gaytán J. F. (2000). Coffee yields and soil nutrients under the shades of Inga sp. vs. multiple species in Chiapas, Mexico. Agroforestry Syst. 54, 215–224.
Roslin T., Hardwick B., Novotny V., Petry W. K., Andrew N. R., Asmus A., et al. (2017). Higher predation risk for insect prey at low latitudes and elevations. Science 356, 742–744. doi: 10.1126/science.aaj1631
Sam K., Remmel T., Molleman F. (2015). Material affects attack rates on dummy caterpillars in tropical forest where arthropod predators dominate: An experiment using clay and dough dummies with green colorants on various plant species. Entomologia Experimentalis Applicata 157, 317–324. doi: 10.1111/eea.2015.157.issue-3
Schemske D. W., Mittelbach G. G., Cornell H. V., Sobel J. M., Roy K. (2009). Is there a latitudinal gradient in the importance of biotic interactions? Annu. Rev. Ecology Evolution Systematics 40, 245–269. doi: 10.1146/annurev.ecolsys.39.110707.173430
Schooler S. L., Johnson M. D., Njoroge P., Bean W. T. (2020). Shade trees preserve avian insectivore biodiversity on coffee farms in a warming climate. Ecol. Evol. 10, 1–13. doi: 10.1002/ece3.v10.23
Seifert C., Lehner L., Adams M.-O., Fiedler K. (2015). Predation on artificial caterpillars is higher in countryside than near-natural forest habitat in lowland south-western Costa Rica. J. Trop. Ecol. 31, 281–284. doi: 10.1017/S0266467415000012
Sinu P. A., Viswan G., Fahira P. P., Rajesh T. P., Manoj K., Hariraveendra M., et al. (2021). Shade tree diversity may not drive prey-predator interaction in coffee agroforests of the Western Ghats biodiversity hotspot, India. Biol. Control 160, 104674. doi: 10.1016/j.biocontrol.2021.104674
Smith C., Barton D., Johnson M. D., Wendt C., Milligan M. C., Njoroge P., et al. (2015). Bird communities in sun and shade coffee farms in Kenya. Global Ecology and Conservation 4, 479–490.
Soto-Pinto L., Perfecto I., Castillo-Hernandez J., Caballero-Nieto J. (2000). Shade effect on coffee production at the northern Tzeltal zone of the state of Chiapas, Mexico. Agriculture Ecosyst. Environ. 80, 61–69. doi: 10.1016/S0167-8809(00)00134-1
Southwood T. R. E., Moran V. C., Kennedy C. E. J. (1982). The richness, abundance, and biomass of the arthropod communities on trees. J. Anim. Ecol. 51, 635–649. doi: 10.2307/3988
Tadesse Z., Nemomissa S., Lemessa D. (2023). Insect pest predation by arthropods and birds in different land use types with varying woody vegetation composition in agroecosystems of central Oromia, Ethiopia. Acta Oecologica 121, 103954.
Takaoka S. (2008). Long-term growth performance of Cordia africana and Grevillea robusta trees in the Mount Kenya region. Agroforestry Syst. 72, 169–172. doi: 10.1007/s10457-007-9056-7
Tallamy D. W., Shropshire K. J. (2009). Ranking lepidopteran use of native versus introduced plants. Conserv. Biol. 23, 941–947. doi: 10.1111/j.1523-1739.2009.01202.x
Tilman D., Clark M., Williams D. R., Kimmel K., Polasky S., Packer C. (2017). Future threats to biodiversity and pathways to their prevention. Nature 546, 73–81. doi: 10.1038/nature22900
Tvardikova K., Novotny V. (2012). Predation on exposed and leaf-rolling artificial caterpillars in tropical forests of Papua New Guinea. J. Trop. Ecol. 28, 331–341. doi: 10.1017/S0266467412000235
Vandermeer J., Perfecto I., Ibarra Nuñez G., Philpott S., Garcia Ballinas A. (2002). Ants (Azteca sp.) as potential biological control agents in shade coffee production in Chiapas, Mexico. Agroforestry Syst. 56, 271–276. doi: 10.1023/A:1021328820123
Wagner D. L., Hoyt A. C. (2022). “On Being a caterpillar: Structure, function, ecology, and behavior,” in Caterpillars in the Middle: Tritrophic Interactions in a Changing World (Springer International Publishing, Cham), 11–62.
Wainwright C. M., Finney D. L., Kilavi M., Black E., Marsham J. H. (2020). Extreme rainfall in East Africa, October 2019 – January 2020 and context under future climate change. R. Meteorological Soc. 76, 26–31. doi: 10.1002/wea.3824
Withaningsih S., Rabbany M. B. (2019). Correlation between some landscape metrics and insect species richness in coffee agroforests in Pangalengan Subdistrict, Bandung District, West Java, Indonesia. Biodiversitas 20, 3075–3085. doi: 10.13057/biodiv/d201042
Yadessa A., Burkhardt J., Denich M., Woldemariam T., Bekele E., Goldbach H. (2008). “Effect of different indigenous shade trees on the quality of wild Arabica coffee in the Afromontane rainforests of Ethiopia,” in Poster presented at the 22nd International Conference on Coffee Sciences (ASIC), Campinas SP, Brazil, 14–19 Sept. 2008.
Keywords: pest control, ecosystem service, coffee, birds, Africa, sentinel pest, predation
Citation: Moura XO, Jedlicka JA, Oliveira SL and Johnson MD (2025) Effects of native and non-native shade trees on insect predation pressure on Kenyan coffee farms. Front. Conserv. Sci. 6:1529450. doi: 10.3389/fcosc.2025.1529450
Received: 16 November 2024; Accepted: 25 March 2025;
Published: 22 April 2025.
Edited by:
Nickson E. Otieno, National Museums of Kenya, KenyaReviewed by:
Gabor L. Lovei, Aarhus University, DenmarkMateus Santos, Universidade Estadual do Sudoeste da Bahia, Brazil
Copyright © 2025 Moura, Jedlicka, Oliveira and Johnson. This is an open-access article distributed under the terms of the Creative Commons Attribution License (CC BY). The use, distribution or reproduction in other forums is permitted, provided the original author(s) and the copyright owner(s) are credited and that the original publication in this journal is cited, in accordance with accepted academic practice. No use, distribution or reproduction is permitted which does not comply with these terms.
*Correspondence: Matthew D. Johnson, bWF0dC5qb2huc29uQGh1bWJvbGR0LmVkdQ==