- Department of Biology, University of Saskatchewan, Saskatoon, SK, Canada
Understanding species occupancy and habitat selection is fundamental to ecology and provides critical information for management. In the Rocky Mountain Foothills of western Canada, feral horses (Equus ferus caballus) are now sympatric with many native species and free-ranging cattle. From 2018–2020 we assessed the seasonal habitat selection of GPS-tracked feral horses in Alberta’s Sundre Equine Management Zone; and compared summer probability of occupancy of horses, domestic cattle (Bos taurus; not present in winter), and elk (Cervus elaphus) using a 120-unit array of trail-camera data. GPS-tracked horses varied in selection for vegetation type and counter to expectations horses tended to avoid native rangeland in summer compared to greater selection for forestry cutblocks. In winter, horses were closer to native rangelands and selected areas closer to roads, areas of lower terrain ruggedness, and areas of higher solar radiation farther from forests, suggesting that forage, habitat accessibility, and thermoregulation are important drivers of winter habitat use. GPS-tracking results were supported by trail-camera occupancy analyses that pointed to the presence of cattle as a potential modulator of horse habitat use. Summer probability of occupancy for horses was highest with increasing coverage of cutblocks in contrast to cattle where occupancy probability decreased strongly with the latter. Cattle occupancy was also negatively influenced by terrain, though positively influenced by the presence of linear features and reduced distance to roads. Elk summer occupancy increased with decreasing distance to conifer forest and increasing native rangeland, though spatial coverage of elk was low compared to cattle and horses. Our results suggest that human-caused landscape changes are important drivers of feral horse occupancy in this northern ecosystem. While cattle may displace horses from native rangeland in summer, horses appear to seasonally adjust their foraging strategy to focus on forestry cutblocks and clearings that are less used by cattle, until cattle are removed from the system for winter. Horse populations can be expected to respond favorably to increasing access to cutblocks in this ecosystem, and their presence can be predicted by increasing anthropogenic activity.
1 Introduction
The evolution and success of Equus in the grasslands of North America facilitated the expansion of the genus across diverse ecosystems worldwide (MacFadden, 1994; Shoemaker and Clauset, 2014). Hindgut fermentation enables equids to efficiently process large amounts of low-quality forage compared to ruminants (Slade et al., 1970), allowing populations to thrive in ecosystems where poor-quality forage is relatively abundant (Schoenecker et al., 2016). A notable example is Sable Island, Canada, where horses persist on a low-protein, high-fiber diet dominated by beachgrass, while all introduced ruminants (cattle, sheep, and goats) have failed to establish long-term populations (Frasier et al., 2016). This advantage has contributed to the historical survival and expansion of equids into varied ecosystems, including the contemporary introduction of feral horses. However, understanding the circumstances under which horses gain a biological or ecological advantage over native or introduced species, such as cattle, remains limited. This knowledge gap persists despite increasing overlap between feral horses and priority species in ecologically sensitive environments, raising concerns about potential competition (Bonacic et al., 2019; Boyce and McLoughlin, 2021). Competition may be particularly pronounced in the context of diminishing native grasslands (Zapisocki et al., 2022), where the scarcity of data on feral horses complicates already challenging management decisions (Scasta et al., 2018). Investigating the resource use of feral horses is therefore essential for understanding the ecology of this widespread equid and for informing management strategies aimed at maintaining the ecological integrity of the landscapes they inhabit.
As predominant grazers, feral horses are often assumed to select habitat based on grass abundance (Salter and Hudson, 1979). However, habitat selection is complex and influenced by multiple factors, particularly in seasonally variable ecosystems and at higher latitudes (Girard et al., 2013a). While horses can subsist on abundant low-quality forage, they consistently select higher-quality forage when available (Salter, 1978), suggesting that reliance on lower-quality resources may occur primarily when population densities are high or resources are limited (van Beest et al., 2014a). In northern temperate ecosystems, peak biomass production and forage quality occur during summer (Girard, 2012; Hebblewhite, 2006a; Kaufmann et al., 2013). However, competition with domestic cattle and elk (Cervus elaphus) during this period may drive horses to select suboptimal habitats as relative grassland biomass declines (Salter and Hudson, 1980). Seasonal habitat selection may also be influenced by climatic conditions, with temperature and precipitation fluctuating widely across annual cycles (Ganskopp and Vavra, 1986). Snow cover (Berger, 1986), temperature (Girard et al., 2013b), and grazing pressure from previous summer months (Salter, 1978) can all shape winter habitat use. Furthermore, anthropogenic disturbance, such as clearcut logging, may provide alternative forage when winter resources are scarce or difficult to access (Irving, 2001; Salter, 1978). Thus, feral horse habitat selection is inherently complex, and patterns may be difficult to discern in highly disturbed and seasonally dynamic ecosystems.
In western Canada, feral horses inhabit the Rocky Mountain Foothills, where landscapes comprise a mosaic of native and anthropogenic grasslands (Figure 1; Thistle, 2008), forestry cutblocks (i.e., areas where trees have been clearcut as part of timber harvesting operations), intensive recreational use), intensive recreational use (McFarlane and Boxall, 1996), and linear features with dense grass cover (Dickie et al., 2020; Irving, 2001). In Alberta, feral horse populations have persisted since the 1700s following the escape and release of domestic horses (McKnight, 1959). Competition between feral horses, domestic cattle, and native grazers for rangeland resources is a primary management concern (Girard et al., 2013a; Hebblewhite, 2006a; Kaufmann et al., 2013), yet few studies have investigated habitat selection in forested northern environments (e.g., Salter, 1978; Girard, 2012).
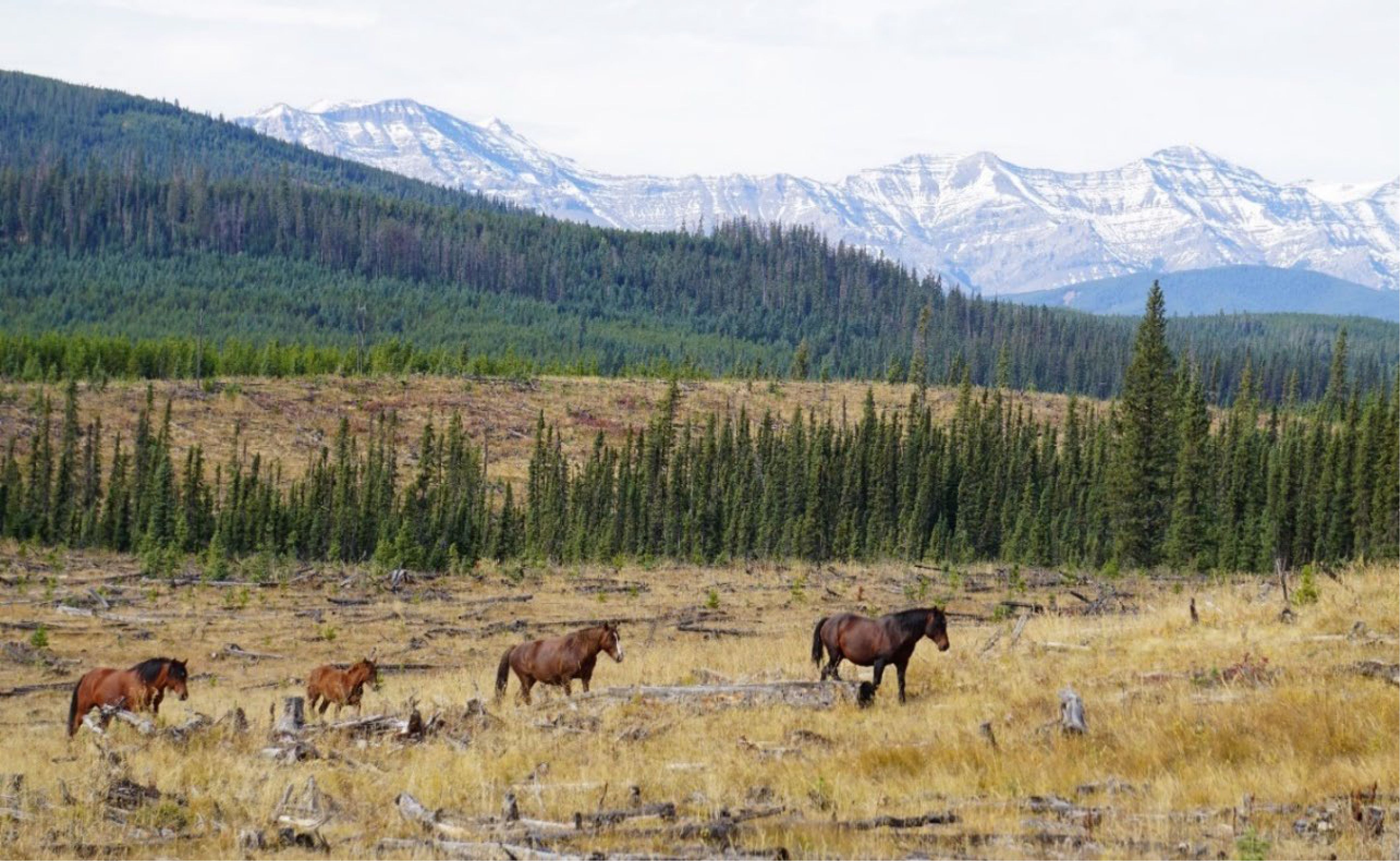
Figure 1. Feral horses in the Williams Creek area in the eastern region of the Sundre Equine Management Zone, Alberta, Canada, showing typical use of cutblocks and summer grazing conditions. The Rocky Mountains of Banff National Park are visible in background. Photography © Paul Boyce, 2019.
Much of the research on feral horse ecology has focused on arid environments; hence, extrapolating findings to western Canada’s forested landscapes may be inappropriate. For example, while water availability often strongly influences habitat selection in feral horses (Ganskopp and Vavra, 1986) and is a common source of competition between horses, cattle, and native ungulates (Hall et al., 2018), this relationship appears weaker in the Alberta Foothills, where water sources are widespread (Girard et al., 2013a, Girard et al., 2013b). Additionally, the region’s relatively abundant and diverse forage throughout the growing season may allow horses to reduce direct competition by selecting alternative, though still productive, habitats as grazing pressure increases (Salter, 1978; Salter and Hudson, 1979). Industrial land use is extensive across the Foothills (Schneider, 2002), and certain disturbances may enhance forage availability for feral horses. Forestry cutblocks, for example, produce high forage biomass, second only to native grasslands and shrublands (Girard et al., 2013b). Given the ubiquity of cutblocks throughout the Foothills (McFarlane and Boxall, 1996), these disturbed areas may significantly influence feral horse distribution, particularly when competition for native grasslands is high.
Seasonal changes in forage availability also coincide with shifts in grazing communities. Free-ranging cattle in Alberta are present only during summer (Kaufmann et al., 2013), while partially migratory elk populations may reduce their spatial overlap with horses in summer by moving westward, outside of general feral horse range (Hebblewhite et al., 2006b). Consequently, competition for preferred resources is likely to vary temporally, potentially peaking when all three species co-occur. Additionally, several biotic and abiotic factors influence large herbivore habitat use, including terrain, distance to water, linear features, forest fragmentation, and human disturbance. These factors mediate habitat selection through their effects on predation risk (DeCesare et al., 2014; Hebblewhite et al., 2005), mobility and habitat accessibility (Dickie et al., 2020; Trombulak and Frissell, 2000), and behavioral avoidance of humans (Leblond et al., 2013). As a result, competition between species extends beyond forage availability and is shaped by multiple ecological and landscape variables. The interaction of these factors, coupled with species-specific differences in foraging strategies and digestive physiology (Janis, 1976; Preston, 1984), is likely to drive varying habitat use among species. However, due to limited empirical evidence on feral horse habitat selection in these ecosystems, directly comparing habitat use among species remains challenging without further research.
Understanding the habitat use and spatial dynamics of feral horses is increasingly important for conservation planning in multi-use landscapes (Ostermann-Kelm et al., 2009). In regions such as the Rocky Mountain Foothills—where native ungulates, domestic livestock, and feral horses coexist—maintaining ecological integrity requires insight into how these species interact across space and time. To address this, we investigated landscape-scale habitat selection and occupancy of feral horses in the Sundre (Alberta) Equine Management Zone (EMZ; Figure 2), a temperate montane ecosystem. Our first objective was to use resource selection analyses to quantify the relative importance of natural and anthropogenic landscape features across seasons, generating spatially explicit predictions of habitat use. Second, we assessed single-season occupancy of horses, cattle, and elk in summer, evaluating how key habitat features influenced occupancy and comparing species-specific habitat associations. These comparisons are ecologically meaningful because the three species differ markedly in foraging strategies and digestive physiology: horses are hindgut fermenters capable of rapidly consuming large volumes of low-quality forage, while cattle and elk are ruminants that selectively graze on higher-quality forage (Ménard et al., 2002). Such differences influence the degree of spatial overlap and the potential for interspecific competition, especially under conditions of forage limitation or habitat disturbance. We predicted that feral horse selection for grasslands would peak in summer when forage productivity is highest, and that selection for disturbed areas with lower-quality forage would increase in winter (Girard et al., 2013b). We also predicted that occupancy for all three species would be positively associated with the availability of native rangelands, and that human disturbance features would negatively affect predicted occupancy for horses and elk (DeMars and Boutin, 2018; Leblond et al., 2013).
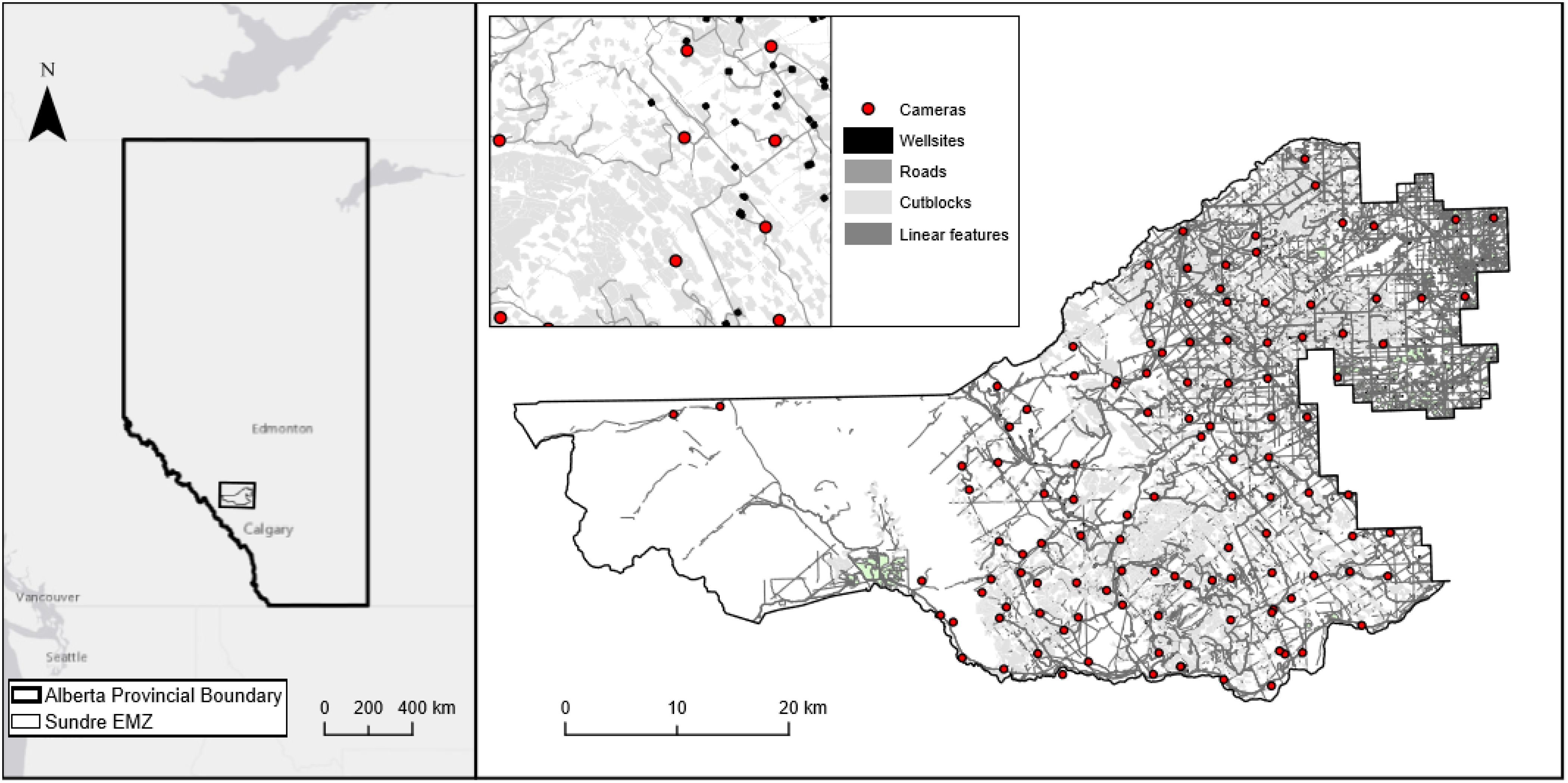
Figure 2. The Sundre Equine Management Zone (EMZ) is situated in the south-western corner of the province of Alberta, Canada. Industrial features predominate in the east. The features in grey are composed of relevant human footprint features as identified in the Alberta Biological Monitoring Institute (ABMI). Camera locations include all deployments and redeployments.
2 Materials and methods
2.1 Study area
The Sundre EMZ is one of six equine management zones in the Alberta foothills, located approximately 100 km northwest of Calgary, Alberta (Figure 2). The zone is approximately 2205 km2 and is bordered by the Clearwater River to the north, the Red Deer River to the south, and Banff National Park to the west. The eastern boundary of the zone is comprised of a mix of grazing leases and fenced private land, with topography generally rolling or flat. Approaching the west of the study area, terrain ruggedness increases, with the eastern ranges of the Rocky Mountains bordering the western administrative boundary (Figure 1). Abundant creeks, muskegs, ponds and sloughs are present throughout the study area, many of which are frozen in winter, while snow generally remains on the ground from November through to March, particularly at higher elevation towards the western end of the EMZ (Hebblewhite, 2006a; Salter, 1978). Temperatures vary by season with minimum hourly temperatures dropping below -29°C in February (mean = -7°C) and maximum temperatures exceeding 31°C in July (mean = 16°C). Precipitation is highest in May (mean = 70mm), June (mean = 114mm), and July (mean = 82mm) with mean annual precipitation approximately 550 mm (data from 2017–2019 inclusive; temperature and precipitation data from the Coalcamp Creek weather station in the south of the EMZ). Botanical species in the Sundre EMZ include pine (Pinus spp.), fir (Abies and Psuedostuga spp.), spruce (Picea spp.), tamarack (Larix sp.), poplar (Populus spp.), and birch (Betula spp.) with (willow [Salix spp.], alder [Alnus spp.], bog birch [Betula pumila]), herbaceous forbs (non-graminoid dominant) and grasses/sedges (graminoid dominant [Festuca spp.; Poa spp.; Carex spp.) present in non-forested areas including native rangeland and cutblocks (Alberta Vegetation Inventory, 2016). Forage quality for herbivores in cutblocks is generally lower than in native rangelands, with reduced crude protein and higher fiber content, particularly in older regenerating stands in Alberta’s foothills (Kaufmann, 2011; Girard, 2012; Girard et al., 2013b).
The Sundre EMZ is managed under the publicly owned “Green Area” of Alberta’s Land-use Framework (Government of Alberta, 2008), which emphasizes balancing timber production, oil and gas development, tourism and recreation, conservation of natural spaces, watershed protection, and fish and wildlife habitat. Within the EMZ, elk and cattle are priority species for management. Elk are native wildlife of high ecological and cultural importance, and are managed for sustainable harvest and population persistence. Cattle grazing is permitted under regulated tenures with seasonal grazing. As an unmanaged, free-ranging population, feral horses exist outside conventional regulatory frameworks and raise unique challenges for conservation, land-use planning, and ecosystem monitoring (Boyce and McLoughlin, 2021; Boyce et al., 2021). Ecosystem management within the EMZ seeks to maintain biodiversity, ecosystem function, and sustainable land use across sectors, and understanding how horses interact with managed species is essential to achieving these objectives.
Horse density is typically highest in the eastern extent of the EMZ which has been subject to substantial modification (Figure 2). Approximately 21% of the total area of the EMZ has been logged at some time, while the combined human footprint (e.g., roads, oil and gas infrastructure, transmission lines, forestry) in the EMZ is approximately 25% based on Alberta Biodiversity Monitoring Institute (ABMI) data. Vegetation inventories on public land are maintained by Alberta Environment and Parks (AEP) in the Alberta Vegetation Inventory (AVI), which is a composition of vector polygons classified from aerial photography (Alberta Agriculture and Forestry, 2016). In locations where forestry management agreements (FMA) are in place, the inventory is maintained by agreement holders. Within the Sundre EMZ, two such FMAs exist in the eastern portion of the study area, covering approximately 51% of the total EMZ. Thus, vegetation and landcover data used to characterize the study area were compiled from three separate AVI datasets: crown AVI data; and two AVI datasets provided on request from agreement holders. Aerial photograph dates within each dataset were dated to 2011/12, and changes in areal coverage of some classes (e.g., forest harvest data) since these dates were updated using 2018 data from the ABMI human footprint layer (Alberta Biodiversity Monitoring Institute, 2018).
2.2 Trail cameras
120 trail cameras (BlazeVideo© model A252; Stealthcam© model PX14) (Blaze Video Canada, 2022; Stealth Cam, 2022) were deployed from 2017/18–2020 across the Sundre EMZ using 4-km grid spacing across the known distribution of feral horses based on minimum count observations from 2001-2016 (Alberta Environment and Parks, 2021). Cameras were set to motion-triggered detection, on the highest sensitivity setting, with 32-GB SanDisk® memory cards and alkaline batteries exchanged at approximately 6-monthly intervals. Cameras were mounted at waist height (1–1.5 m) relative to animal movement, oriented to maximize detection of both feet and facial markings of horses. Given the ubiquitous nature of linear features and trails, cameras located adjacent to, or, on trails were oriented towards animal movement to maximize detection of animals when present in the area (Stewart et al., 2019). Several cameras and SD cards were stolen, damaged, or otherwise rendered inoperable (e.g., flipped against trees) leaving approximately 79% of the grid functioning over the study period.
Images were classified in Timelapse2 Image Classification Software (Greenberg et al., 2019) using both manual classification and machine learning image recognition techniques. Approximately 1/3 of all images were classified to species level manually by the authors and undergraduate students from the University of Saskatchewan, with the remaining 2/3 of images classified using Microsoft’s Megadetector algorithm (Beery et al., 2019; Microsoft Corporation, Redmond, WA, United States). Following classification, we reviewed all classifications for large-mammal species including those by both students and the Megadetector algorithm and corrected any misclassifications. The equations should be inserted in editable format from the equation editor.
2.3 GPS collars
To assess resource selection, 5 GPS collars were deployed on female horses in the 2018/19 winter using free-darting protocols and drug combinations (Stover and Caulkett, 2021) under University of Saskatchewan Animal Use Protocol 20170117, and Alberta Environment and Parks Horse Capture License RDNS 003 2018 (TFAs: 182578, 185269). Collars were a combination of Lotek 7000 series (2; 12 hourly fixes) and Vectronic SURVEY 2-D (3; 3-hourly fixes) models (Lotek Wireless Inc., 2022; Vectronic Aerospace GmbH, 2022). Collars recorded date and time, elevation, and temperature. All collars were initially set to record animal locations every 3 hours, though Lotek brand collars reverted to 12 hourly fixes following deployment from previous study schedules, with one Lotek collar going offline without further satellite communication near the end of our study. We targeted adult females for GPS collaring because they are reliably associated with stable family bands, unlike males which may be solitary or form transient bachelor groups. This approach allowed us to track the movements of five distinct bands—our primary ecological unit—representing approximately 50 individual horses.
2.4 Landcover and covariates
Variables used in resource selection and occupancy analyses were selected based on evidence from previous feral horse habitat selection studies (Table 1) including vegetation classes, distance to water, terrain ruggedness, and disturbance features. To generate vegetation classes, AVI data were combined into broad categories of conifer and deciduous dominant forest (i.e., mixed-wood), non-forested land such as grasslands and cut blocks, and disturbance features such as roads, trails, and transmission lines (anthropogenic linear features). Non-forested land within the AVI encapsulates areas with ≤6% tree cover, but ≥6% vegetated cover and includes herbaceous graminoids and forbs, closed and open shrub, or bryophyte dominant classified polygons. Conifer and deciduous classes refer to the dominant tree species present as identified in the AVI, though not the absence of deciduous or conifer trees, respectively. Non-forested land previously logged (i.e., current or historic non-regenerated clear-cuts) was grouped as cleared non-forested land (cutblocks) distinct from native rangeland. Unmodified grasslands and shrublands were grouped together as native rangeland, as both classes have been shown to have high herbage production and similar patterns of seasonal horse selection within the temporal scales investigated here (Girard et al., 2013a, Girard et al., 2013b; Salter and Hudson, 1979). Other cleared features directly seeded with grass such as transmission lines and areas associated with geophysical exploration or extraction (e.g., pipelines) were grouped as linear features. All water features including rivers, lakes and ponds, and named streams from both the AVI, and the Alberta hydrography database (www.AltaLIS.com) were combined into a single “water” class, and road vector data was sourced from both ABMI data, and open source AltaLIS feature layers, and included both paved and unpaved roads and trails within the study area. Proportional areal coverage of landscape covariates throughout the Sundre EMZ was as follows: conifer forest (~60%) ≫ mixed-wood forest (~10%) > native rangeland (grasslands and shrublands; 7%) > forestry cutblocks (7%) ≫ linear features (areal coverage of ~1%). For analysis of GPS-tracked bands (i.e., Step-Selection Function (SSF) analyses; §2.5), individual Euclidean distance raster datasets were created for each vegetation covariate, linear features, and water; with all classes scaled and centered prior to analysis.
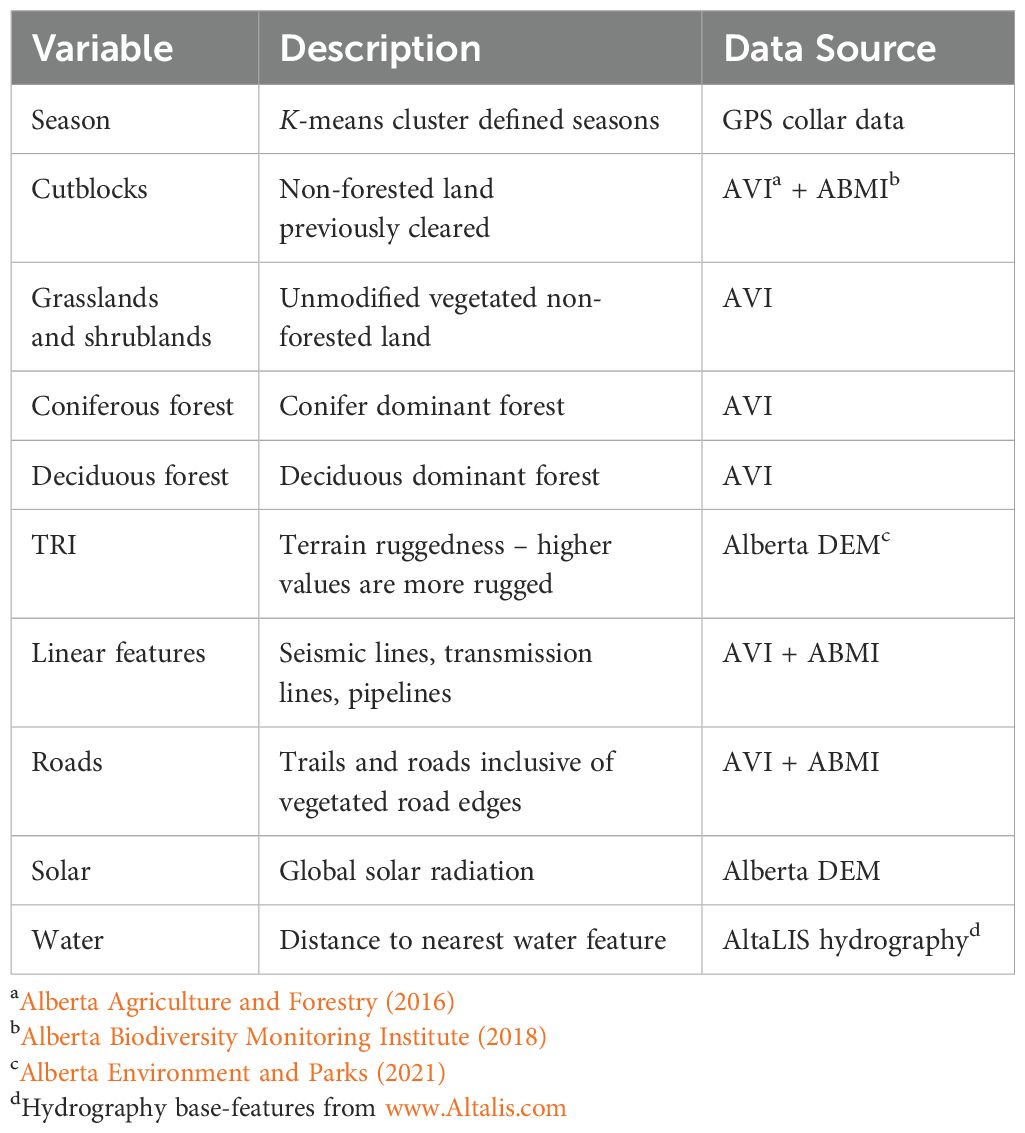
Table 1. Data sources of variables used in the habitat selection analysis for GPS-tracked horses in the Sundre Equine Management Zone, Alberta, Canada (2018–2020).
In ArcMap (ESRI, 2011) two additional covariates were derived from an Alberta provincial 25-m digital elevation model (DEM) (Alberta Environment and Parks, 2017). A terrain ruggedness index (TRI) characterizing topography was calculated following Riley et al. (1999), while global solar radiation (GSR) was calculated following equations derived by (Fu and Rich, 2002) in the Solar Radiation toolset in ArcMAP (ESRI, 2011). All covariates for SSF model analyses and selection were examined for collinearity with variables correlated at r ≥ 0.70 not used in the same model.
For camera-trap occupancy analyses (§2.6), 1000-m radius buffers (Fisher et al., 2011, Fisher et al., 2021) were created around each camera site and the percent areal coverage of vegetation classes (above) were calculated to characterize habitat. For covariates such as GSR and TRI, both the range and the mean value within the buffer were calculated, with top-ranking variables (based on Akaike’s Information Criterion, AIC; Anderson and Burnham, 2004) from univariate models used in subsequent models (see below). For roads and distance to water data, Euclidean distance from the camera location to the nearest feature was calculated. Covariates were scaled and checked for collinearity as with raster datasets, with correlated variables (r ≥ 0.7) not included in models together.
Biological seasons were defined based on the presence or absence of cattle grazing, which corresponds closely with snow-free (summer) and snow-covered (winter) periods in the region, and reflects seasonal shifts in forage availability and habitat accessibility. K-means clustering (Zeller et al., 2019) was first used to identify different statistical clusters in mean daily movement pooled across horses and years to determine movement-based biological seasons (Zeller et al., 2019). Clusters were then visually compared to key phenological dates as identified by Girard (2012) in a similar Foothills ecosystem (i.e., Bragg Creek, AB). Two clear seasons based on movement data were defined (see Boyce, 2022) compared to the four vegetation-derived seasons in Girard (2012). Seasons based on movement data matched closely with the winter-spring seasonal transition (May 14th), and the summer-fall transition (October 31st) described from plant phenology and snow cover in Girard (2012), and these transition dates were used to define seasons thereafter.
2.5 Resource selection
We assessed landscape-scale selection of feral horses (i.e., second-order selection [Johnson, 1980]) using step-selection functions. Step-selection functions are an adaptation of tradition resource-selection functions (Manly, 2002) and assess segments (steps) of animal movement as sampling units rather than static locations (as in point-location resource-selection functions), allowing the modeling of conditional selection, as a function of covariates along an animals’ projected path (step) (Fortin et al., 2005). Step-selection functions are useful in highly heterogeneous environments compared to static use-available analyses (Prokopenko et al., 2017), which was an important consideration as much of the Sundre zone is a highly heterogenous complex of industrial linear features and habitat patches.
Individual step-selection functions (SSF) were derived from trajectories for each horse fitted with a GPS collar using the amt package in R (Core Team, 2022) and following the equation from Fortin et al. (2005):
where coefficients β1 to βp derived from conditional logistic regression associated with landscape covariates x1 to xp, estimate conditional selection of steps, with steps with higher ŵ(x) values more likely (higher odds) to be selected (Fortin et al., 2005). Step length, log step length, and cosine of turn angle were used to generate available steps but were not included as covariates in the conditional logistic regression models, consistent with standard SSF practice (Fortin et al., 2005). Sample frequencies between collars were first regularized and fifty available points per used point, were randomly generated from distributions of observed step lengths and turn angles for each horse where two successive steps could be calculated based on sample frequency (i.e., minimum of three locations not longer than sample frequency; Prokopenko et al., 2017). Conditional logistic models were then fitted to location data using the survival package in R (Core Team, 2022). Univariate selection models for covariates predicted to influence feral horse habitat selection (Table 1) were initially assessed and compared to a global model (i.e., unconstrained) using AIC. The global model including all variables and a season interaction received full model weight when compared to univariate models and was subsequently used to describe feral horse selection. Mean beta coefficients across individuals based on the global model were used to describe “population” level selection characteristics within the Sundre EMZ. Spatial predictions of habitat suitability based on mean selection coefficients for each covariate were then used to generate predicted habitat use maps across the Sundre EMZ by season.
Spatial prediction surfaces were generated by applying the averaged β coefficients from the global model across rasterized covariate layers, using a log-linear form to produce a relative index of habitat suitability. While we did not perform formal k-fold cross-validation due to the small number of individuals, we assessed prediction consistency by comparing mapped suitability to known high-use areas such as Ya Ha Tinda and Harrison Flats.
2.6 Occupancy modeling
Occupancy models are robust to variable detection rates of species and are an effective means to assess broad patterns of species distribution in relation to landscape covariates (MacKenzie et al., 2002). Combined with camera-trap data, they can provide robust inference about multiple species within ecological communities (Kays et al., 2020). We assessed occupancy of horses, cattle, and elk using an information-theoretic approach to test the relative influence of landscape covariates on summer occupancy of each species. We focused on the summer growing period of June–September, as all three species are present within the Sundre EMZ (i.e., cattle are removed in late summer/fall); with winter defining the remaining season. The former also lies within peak rangeland biomass production (Girard, 2012; Hebblewhite, 2006a) and was short enough (12 weeks) that colonization or extinction across sites could reasonably be assumed to absent (MacKenzie and Bailey, 2004), while long enough to provide robust estimates of occupancy (Kays et al., 2020).
Single-season occupancy models (MacKenzie et al., 2002) were fit using the unmarked package in R (Core Team, 2022) with detection data discretized to weekly samples creating a detection history for each species. We first assessed global models for each species for goodness-of-fit using the Mackenzie-Baily (MB) goodness-of-fit (GOF) test (MacKenzie and Bailey, 2004). The MB goodness-of-fit test calculates a Pearson’s chi-squared statistic (ĉ) to assess model dispersion using parametric bootstrapping of observed occupancy compared with occupancy fit to randomly generated detection histories. Values of ĉ approximating unity denote adequate model fit. Where significant (P< 0.05) lack-of-fit and ĉ > 1.0 or<1.0 is identified, model variance (SE) can be inflated based on the chi-squared statistic to facilitate more robust inference during model selection (Burnham and Anderson, 2002; MacKenzie and Bailey, 2004). We estimated variance inflation factors (ĉ) for each species’ global model using 1000 simulated bootstrap samples to assess global fit prior to assessment of model hypotheses and assumed fit of subsequent nested models was adequate where ĉ was close to 1.0 and lack-of-fit estimates were not significant (Grant et al., 2009). Following model testing, we fitted multiple single-season occupancy models within a structured candidate model set (Table 2). Detection probability in all models was modeled as a function of effort (continuous; total trap days) and whether the camera was adjacent to a trail or not (factor; 1, 0). We then compared models within species using AIC and where multiple models within species had ΔAIC< 2.0, we used model averaging to estimate the relative influence of each covariate from top models on occupancy, to reduce potential inferential bias based on a single top-ranked model (Burnham et al, 2002; Anderson and Burnham, 2004). We interpret occupancy here as an index of site use rather than true presence–absence occupancy (MacKenzie et al., 2002), consistent with other camera-trap based studies in large mammal systems. While we used model-averaging to incorporate model uncertainty, we acknowledge limitations of this approach when covariates vary in inclusion and scale among models (Cade, 2015). Finally, we calculated evidence ratios (ER) comparing top models with second-ranked models for each species, assessing relative influence of omission or inclusion of variables in top models.
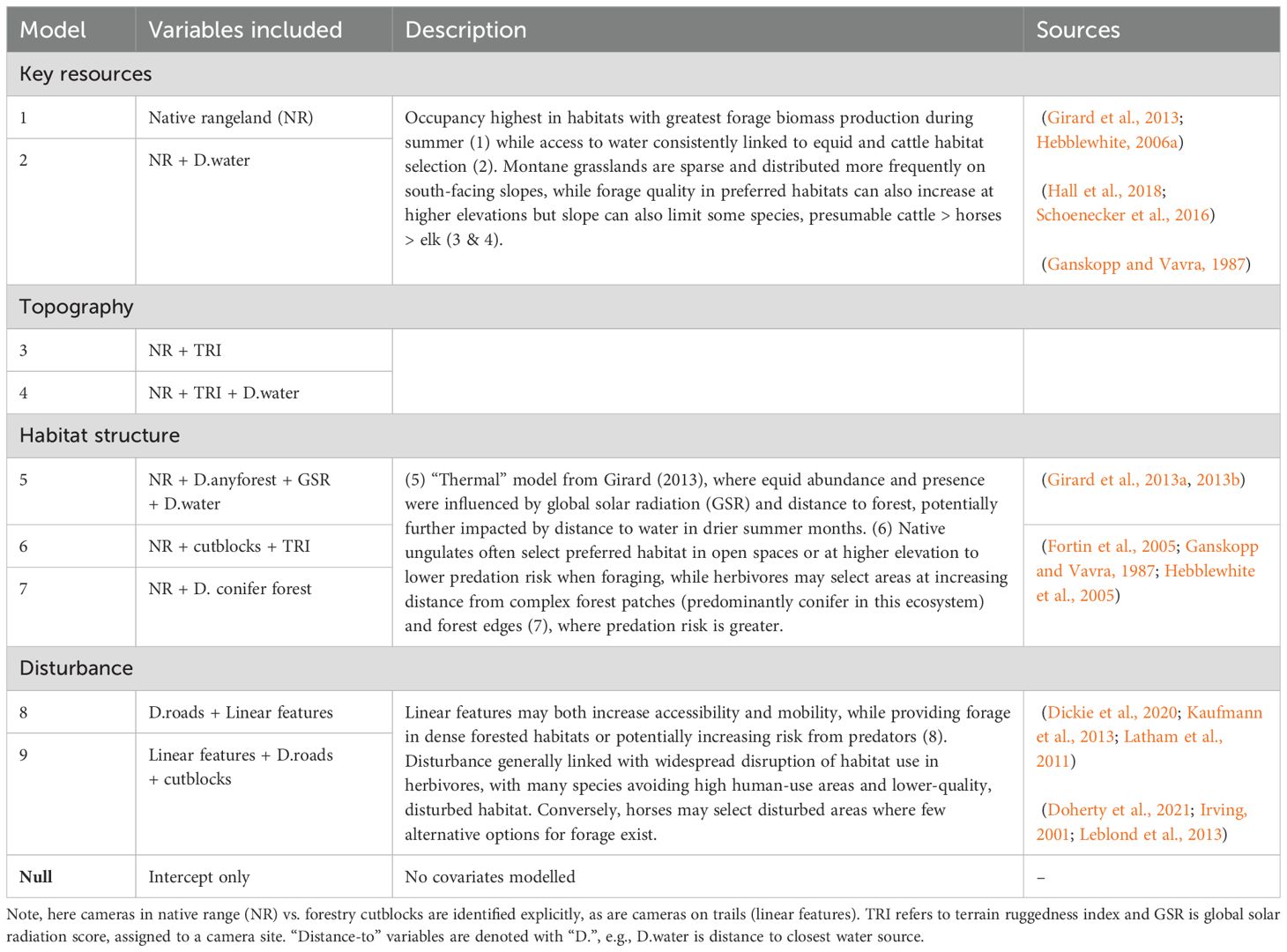
Table 2. Candidate models used in occupancy modeling for horses, elk, and cattle in the Sundre Equine Management Zone, Alberta, Canada (2018–2020).
We developed nine candidate models based on a priori hypotheses nested within four broad predictions characterizing comparative habitat use of horses, cattle, and elk based on A. key resources, B. topography, C. habitat structure, and D. disturbance (Table 2). Our first hypothesis predicted that occupancy would be greatest for all species in habitats with the greatest forage biomass production (native rangeland including grasslands and shrublands), and that distance to water would be important for more water-dependent species such as equids (Ganskopp and Vavra, 1986; Schoenecker et al., 2016). Our second hypothesis predicted that forage biomass and terrain would best describe occupancy, as Montane grasslands are often sparse and dispersed at both high elevation and slope (Girard, 2012) yet cattle are often limited by slope and elevation compared to horses and elk (Kaufmann et al., 2013). Our third hypothesis predicted that habitat structure would best explain occupancy, as both elk and horses have been shown to select and avoid open habitats, potentially to reduce predation risk, or for thermoregulation purposes where solar radiation is high, respectively (Girard et al., 2013b; Hebblewhite, 2006a). Our final hypothesis predicted that disturbance would best explain occupancy, where linear features and distance to roads strongly influence large herbivore movement and habitat selection (Dickie et al., 2020; Leblond et al., 2013), while disturbances in the Sundre EMZ that potentially increase forage biomass (i.e., cutblocks, linear features) may result in additional and more accessible forage when more preferred habitat is limiting, particularly at high species overlap in summer (Irving, 2001).
3 Results
3.1 Locations and landscape data
We obtained 23,821 locations from 5 adult female horses (all from separate breeding groups) to evaluate step-selection functions across movement-based biological seasons (summer, winter; 2018– 2020). One band (collared female) travelled outside the southern boundary of the Sundre EMZ, crossing the Red Deer River in winter in multiple years. All other animals remained within the administrative boundaries of the EMZ.
3.2 Resource selection
The global model including all variables predicted to influence habitat selection in feral horses received the total weight (AICwt = 1.0) compared to univariate selection models for each of the above vegetation covariates, and distance to water or roads, terrain ruggedness, or solar radiation. Habitat selection of feral horses showed clear seasonal differences. Selection for areas farther from native rangeland in summer (i.e., selection for increasing distance from rangeland) was strong (Figure 3). Selection for areas of lower solar radiation in summer relative to winter was also apparent (Figure 3), while areas closer to cutblocks appeared to be selected in summer relative to winter (i.e., decreasing distance to cutblocks; Figure 3). Selection patterns in winter appeared to be stronger and non-overlapping with zero for several covariates compared with summer selection patterns. In winter, areas closer to roads (i.e., less distance from) and with lower terrain ruggedness values were selected, while areas with greater solar radiation were selected strongly. Areas farther from cutblocks and conifer forest were selected more in winter relative to summer, reflecting a trade-off with native rangeland which was clearly avoided in summer (when cattle were present) but yet likely then used by horses in winter (Figure 3). Selection for all other covariates appeared weak, neutral, or were variable among individuals (Table 3), with greater general consistency in selection patterns in winter relative to summer.
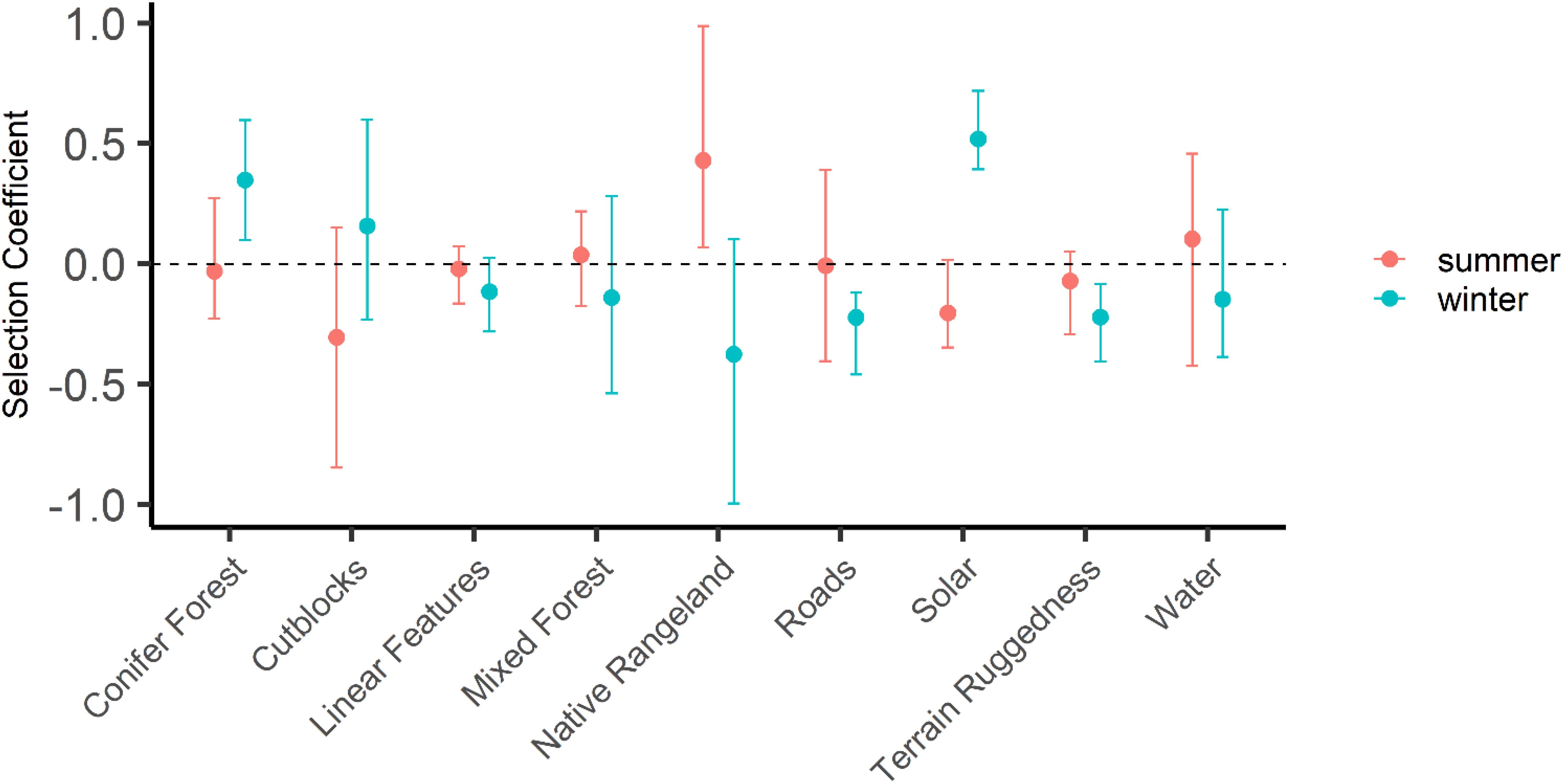
Figure 3. Relative strength of selection coefficients and 95% confidence intervals reflecting use of areas relative to increasing distance from conifer forest, forestry cutblocks, linear anthropogenic features, mixed (conifer-deciduous) forest, native grassland or rangeland, roads and water (variables defined in Table 1) for summer and winter in the Sundre Equine Management Zone, Alberta, Canada (2018–2020). Coefficients for modifiers of extent of solar radiation and terrain ruggedness are interpreted not as “distance to” but rather with respect to increasing amount (i.e., winter selection is for sites with higher solar radiation). Data are from five bands of GPS-tracked feral horses (collared adult females of separate breeding groups). A higher selection coefficient depicts increased relative probability of selecting for greater distance from a feature (e.g., summer selection is for areas farther from native rangeland, compared to winter selection).
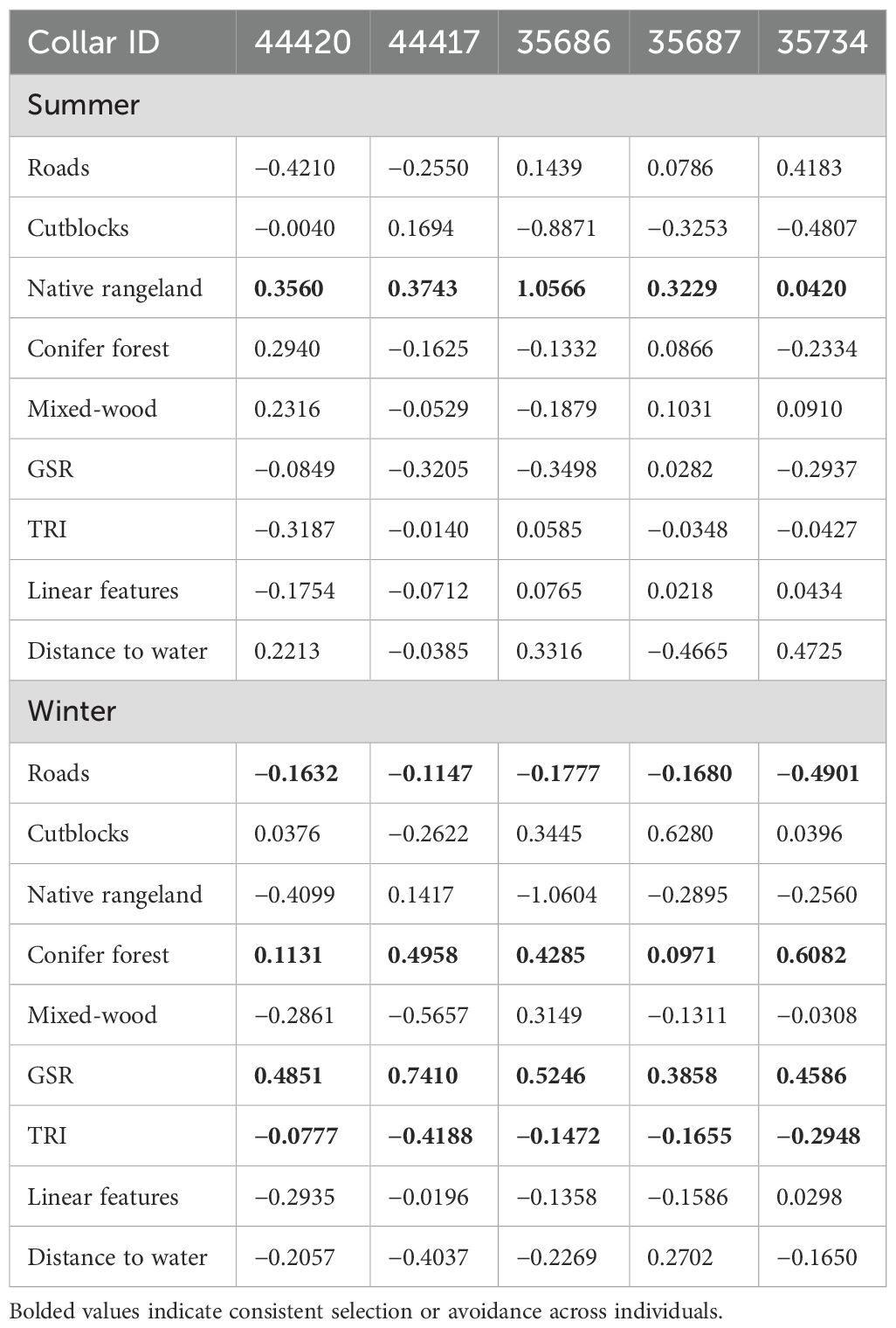
Table 3. Individual selection coefficients (conditional step-wise selection) for five adult females of separate bands (GPS-tracked horses, each indicated by a Collar ID) in the Sundre Equine Management Zone, Alberta, Canada (2018–2020).
Spatially explicit predictions of habitat suitability from occupancy modeling showed a more widespread distribution of suitable habitats in the eastern extent of the Sundre EMZ in summer compared to winter (Figure 4). Winter selection was more limited, with medium-use areas by horses in the eastern extent of the study area reflected in the general distribution of remaining rangeland and areas of highest solar radiation highlighted towards the mountains in the west, compared to the wider distribution of cutblocks throughout the EMZ in the east (see Figure 2). Both summer and winter predictions of suitable habitat were relatively high for areas of flat, native rangeland (note the high predicted use of the expansive Ya Ha Tinda ranch grasslands in the south-west), while the western regions characterized by higher elevation and more rugged terrain, and greater forest coverage (i.e., approaching the Rocky Mountains), indicated low habitat suitability (note the greater relative suitability within this more mountainous habitat of the grassland areas known as Harrison flats in the north-west of the Sundre EMZ [Figure 4]).
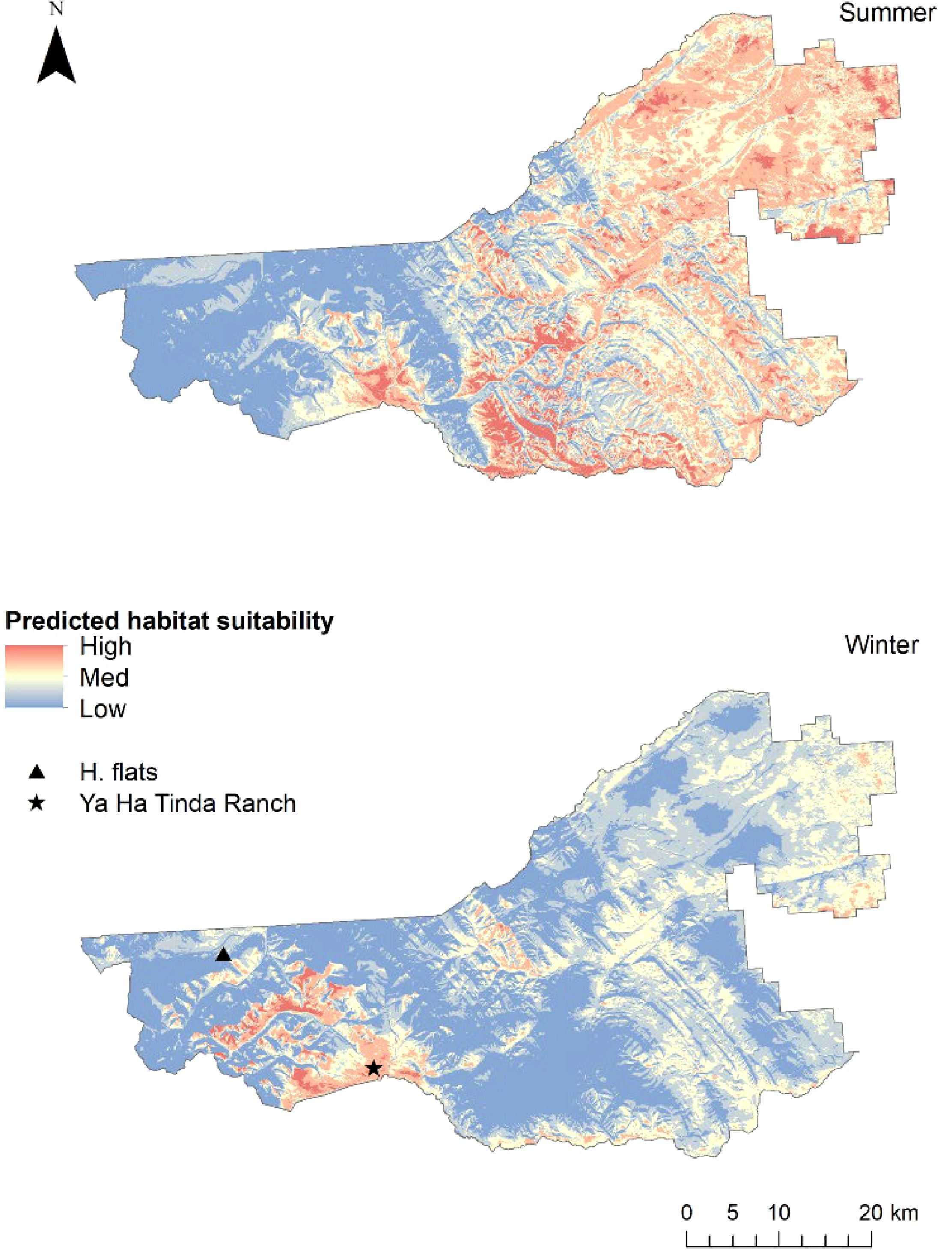
Figure 4. Spatially explicit habitat suitability for feral horses derived from step-selection functions from five GPS-collared adult females from separate breeding groups in the Sundre Equine Management Zone, Alberta, Canada (2018–2020). Harrison flats (H. flats) are native grasslands. The Ya Ha Tinda ranch includes associated native grasslands and pastures managed by Parks Canada Agency.
3.3 Occupancy
There was no evidence of lack-of-fit for global occupancy models as assessed by our goodness-of-fit test. Estimates of ĉ were close to one for each species and lack-of-fit tests were non-significant (horses = 1.02, χ2 = 4193.7, P= 0.30; cattle = 1.07, χ2 = 4375.2, P = 0.15; elk = 1.03, χ2 = 4201.2, P = 0.23 [MacKenzie and Bailey, 2004]). Naïve occupancy probability (i.e., occupancy assuming detection probability = 1.0; MacKenzie et al., 2002) across all sites during summer was 0.80 (0.65–0.89 [95% CI]) for horses, 0.46 (0.36–0.60) for cattle, and 0.19 (0.088–0.36) for elk. Detection probability (assuming occupancy at a site =1.0) was 0.44 (0.39–0.48), 0.43 (0.38–0.49), and 0.14 (0.073–0.25) for horses, cattle, and elk, respectively. Proportion of native rangeland-only models were the top-ranked occupancy models for both horses and elk; whereas disturbance (from cutblocks or linear features) was the top-ranked model for cattle (Table 4). ΔAIC values for all species showed multiple models explained occupancy reasonably (cattle n = 2; elk n = 3; horses n = 5) and evidence ratios between top and second-ranked models were relatively low. For horses in particular, several models had ΔAIC< 2.0.
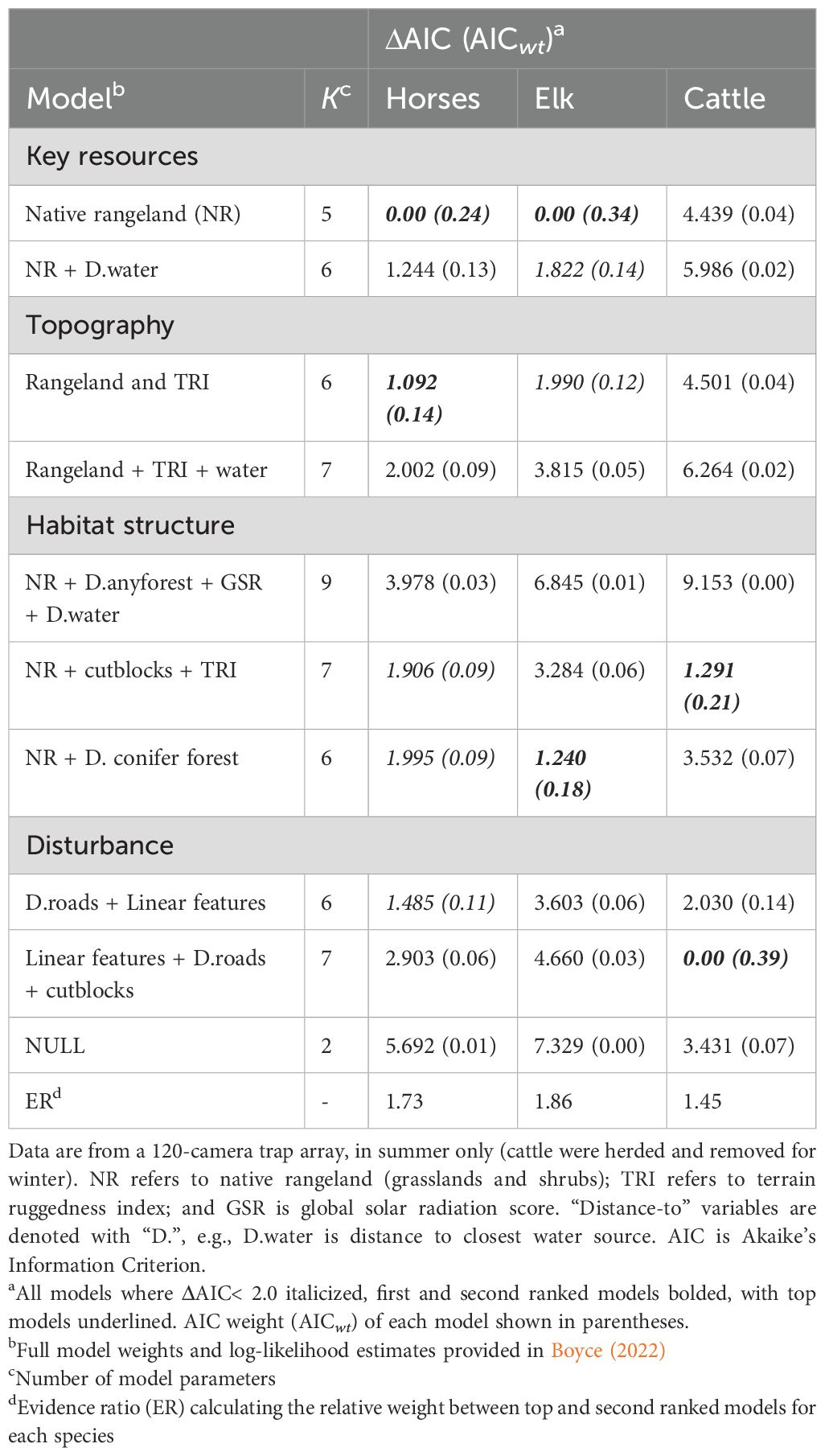
Table 4. Relative support for models estimating occupancy of horses, cattle and elk in the Sundre Equine Management Zone, Alberta, Canada (2018–2020).
Model-averaged occupancy coefficients showed proportion of rangeland had a positive effect on occupancy for all species although this was weak for cattle compared to elk and horses (Table 5; Figure 5). Predicted occupancy decreased with increasing terrain ruggedness (−0.858) and distance from roads (−0.484) for cattle, and the proportion of cutblocks has a similarly negative effect (−0.768); i.e., cattle occupancy was not strongly associated with cutblocks in summer. Proportion of cutblocks had an opposite, positive effect for horses (Figure 5), and was the largest effect across all covariates for horses (0.471). Occupancy was higher with greater areal coverage of linear features for cattle (0.726), although this had the opposite effect for horses (−0.324), while elk occupancy was lower at sites farther from conifer forest patches (−0.858; Table 5). Distance to water and terrain ruggedness had a positive effect on horse predicted occupancy, suggesting these are not limiting factors, while global solar radiation and distance to roads had minor effects. Interestingly, distance to any forest, which included mixed-wood forest, had a negative effect on horse occupancy (−0.330), while distance to conifer forest had a weak positive effect (0.022).
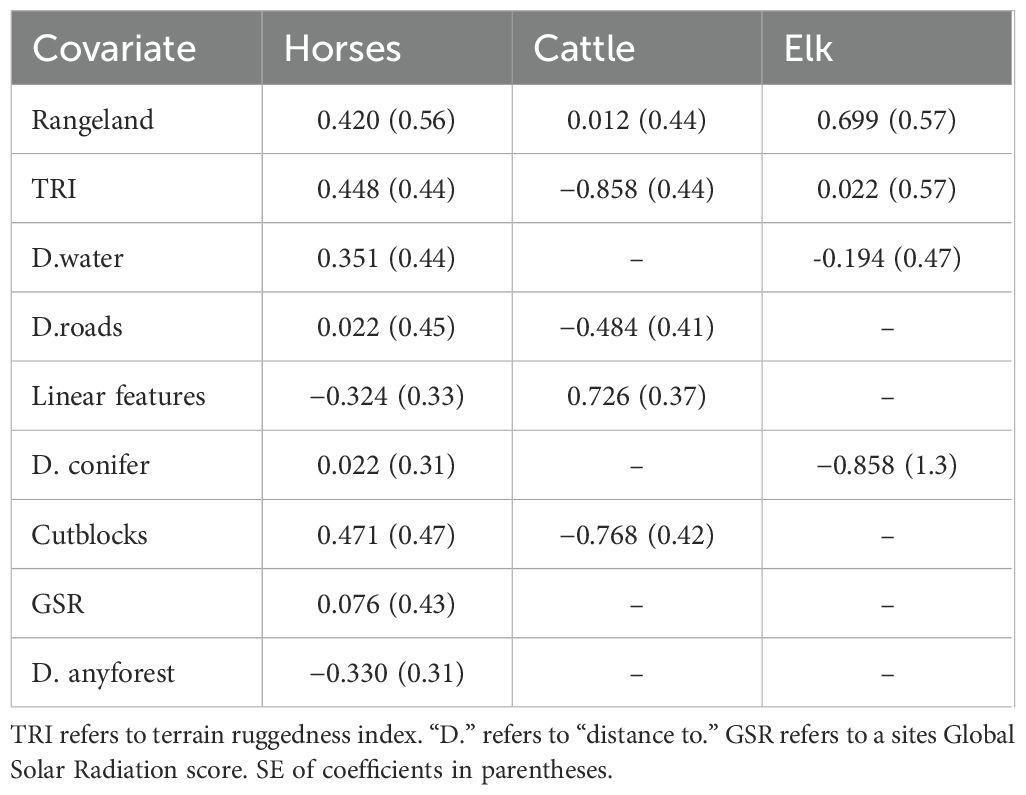
Table 5. Model averaged coefficients of species occupancy from top ranked (ΔAIC< 2.0) models for horses, cattle and elk in Sundre Equine Management Zone, Alberta, Canada (2018–2020).
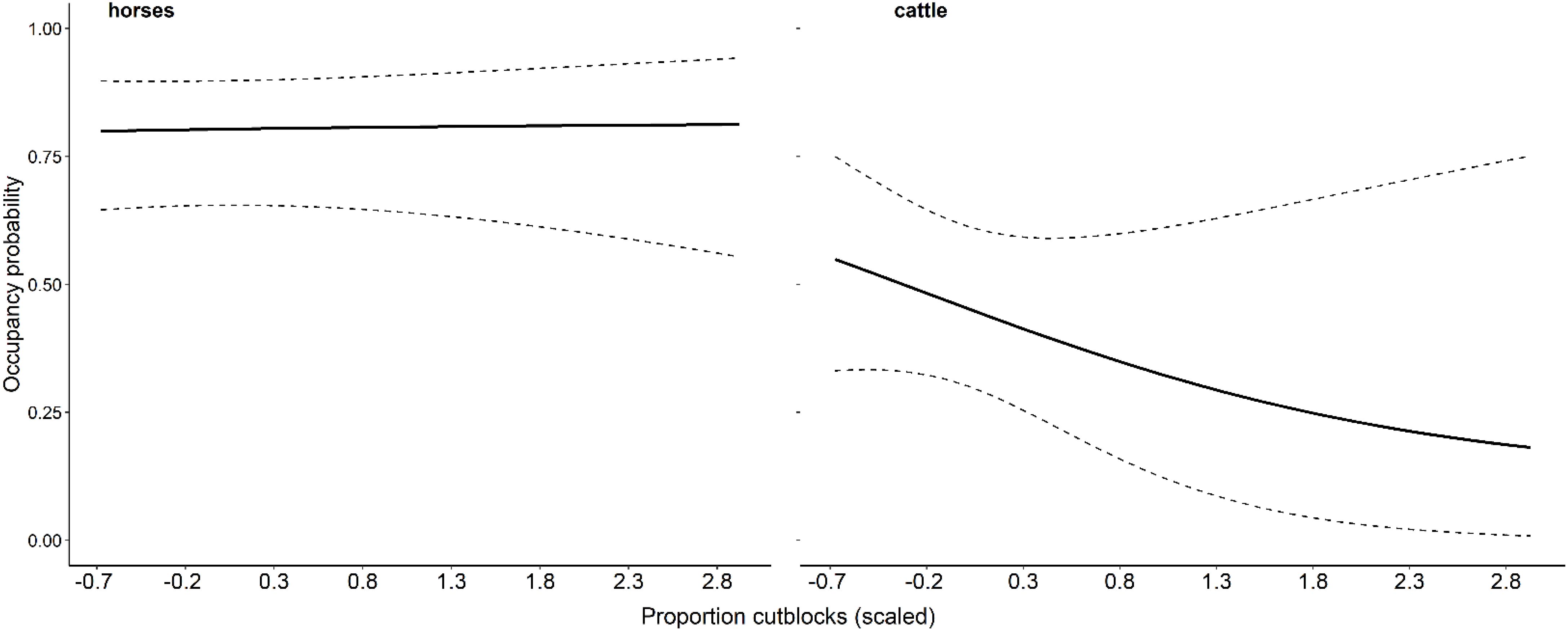
Figure 5. Model-averaged occupancy probability as a function of the proportion of cutblocks for horses and cattle in the Sundre Equine Management Zone, Alberta, Canada (2018–2020). Model selection based on top-ranked (ΔAIC< 2.0). Dashed lines are 95% confidence intervals, and predictions are calculated with all other model covariates held constant.
4 Discussion
We found that feral horses in the Sundre EMZ exhibit distinct seasonal habitat preferences influenced by anthropogenic landscape features, aligning with summer competition with free-ranging cattle. In summer, horses favored forestry cutblocks over native rangelands; in winter, when cattle were absent, their preference shifted back to native grasslands and areas with higher solar radiation, lower terrain ruggedness, and closer proximity to roads, likely due to thermoregulation and mobility considerations. Camera-trap data supported that horses avoided rangelands occupied by cattle in summer and were more associated with cutblocks. Predation risk and human disturbance also appeared to influence habitat use, as horses avoided conifer forests and linear features in winter but showed reduced occupancy in areas with high linear feature coverage in summer, suggesting complex interactions between resource availability, competition, and environmental pressures.
Forage biomass production in our study area is greatest in native rangelands (Girard et al., 2013b; Hebblewhite, 2006a), and feral horses typically select habitats with the highest forage availability (Schoenecker et al., 2016). Girard (2012) found that grassland and rangeland habitats were preferred over more abundant forested types, while Salter (1978) study of the Sundre population similarly reported year-round selection for grasslands, though noted important shifts in habitat use when cattle were present. Despite high dietary overlap (64%), horses and cattle exhibited only 2% contemporaneous overlap in habitat use, suggesting spatial partitioning under conditions of competition. Cutblocks also offer relatively high forage biomass compared to conifer or mixed-wood forests (Girard et al., 2013b; Kaufmann, 2011; Kaufmann et al., 2013), and in our study, horses appeared to select cutblocks more frequently in summer—when cattle are present—despite individual variation (Figure 3). This seasonal pattern likely reflects the management regime within the EMZ, where cattle are removed each fall. Their absence in winter reduces competition for native rangeland, allowing horses to reoccupy these preferred habitats. Although summer occupancy of horses was similar in cutblocks and native rangelands (Table 5), the stronger avoidance of cutblocks by cattle (Figure 5) suggests that horse use of these areas may be driven in part by competitive exclusion.
Cattle use of cutblocks in montane ecosystems is generally low (Kaufmann et al., 2013), whereas the generalist foraging strategy and greater mobility of feral horses may facilitate their use of these areas when spatial overlap with cattle is high (Janis, 1976; Ménard et al., 2002; van Beest et al., 2014b). Despite a general preference for native rangeland (Girard et al., 2013a; Salter, 1978), horses appeared to use cutblocks more frequently in summer, likely as a strategy to reduce competition. In contrast, their use of rangelands increased in winter when cattle were absent (Figure 3), a pattern also reflected in our spatial predictions of winter habitat suitability (Figure 4). Salter (1978) similarly identified dry grasslands as important winter habitat for feral horses.
Digestive physiology likely underpins these seasonal patterns of habitat use. As hindgut fermenters, horses can process large volumes of low-quality forage quickly, allowing them to exploit biomass-rich but nutritionally poor environments such as cutblocks. In contrast, ruminants like cattle and elk rely on foregut fermentation, which enables efficient nutrient extraction from higher-quality forage but limits their use of lower-quality habitats. This functional divergence may reduce direct dietary overlap yet promote spatial partitioning under conditions of high competition. It also helps explain why horses shift toward marginal habitats in summer, while returning to preferred rangelands in winter when forage competition declines (Ménard et al., 2002). Seasonal migration for horses warrant additional research.
Suitable habitat in winter is more limited, and horses appeared to select habitats that potentially mitigate harsh winter conditions (Berger, 1986; Girard et al., 2013b). For example, areas with greater solar radiation and those farther from forests were selected, supporting hypotheses that thermoregulation via sun exposure may drive winter habitat selection (Girard et al., 2013b). However, as noted by Girard et al. (2013b), sites with greater solar radiation may also experience increased herbage growth (Willoughby et al., 1998) or reduced snow cover, thereby improving access to limited winter forage (Salter, 1978). Horses also selected areas closer to roads and with less rugged terrain in winter, likely due to reduced habitat accessibility and mobility constraints when snow cover is at its peak (Whittington et al., 2005). While animals often avoid roads to reduce the risk of human encounters, particularly when human activity is high (Leblond et al., 2013), human activity in the Sundre EMZ peaks in summer. Accordingly, selection and occupancy were neutral or only weakly influenced by distance to roads during this season. In winter, when human activity is low, roads may serve as movement corridors, especially in complex terrain (Underhill and Angold, 1999; Whittington et al., 2005), enhancing mobility between habitat patches where alternative movement corridors are limited. Roadsides may also provide important forage resources for grazers (Fahrig and Rytwinski, 2009). In ecosystems with limited grassland availability, feral horses may utilize disturbed areas where grass production is relatively high (Irving, 2001). Salter (1978) reported similar findings, with horses using roadside forage extensively in winter, as snow depth and litter cover were lower compared to other habitats. Thus, roads may represent an important winter habitat for horses, providing both forage and access to other habitats under severe conditions.
Habitat structure, mediated by seasonality, is a key determinant of habitat use in large mammal communities (Garrott et al., 2008; Kuijper et al., 2015; Sutherland et al., 2015), particularly in landscapes where predators impose variable risk (DeMars and Boutin, 2018) or human activity is high (Treves et al., 2006). While horses selected areas closer to roads in winter, summer occupancy was lower in areas with greater coverage of linear features (Table 5), which may reflect avoidance of potential human encounters when activity is high (Girard et al., 2013a). Large predators are also attracted to linear features (DeMars and Boutin, 2018; Whittington et al., 2005), and although predation on horses in this system is poorly quantified, cougars (Felis concolor) and wolves (Canis lupus) are known to kill horses (Knopff, 2010; Webb, 2009). Grizzly bears (Ursus arctos) may represent a third predator influencing horse behavior, potentially leading to avoidance of linear features (Dickie et al., 2020). Consistent with this, Girard et al. (2013b) found that feral horses selected areas farther from conifer forests in winter, likely reducing the ambush risk from cougars, which predominantly kill horses during this season (Knopff, 2010). These results suggest that horses may structure their habitat use, in part, based on predation risk. In contrast, cattle summer occupancy increased in areas closer to roads and with greater linear feature coverage (Table 5). While cattle generally exhibit reduced anti-predator behavior compared to wild ungulates (Clutton-Brock, 1981; Kluever et al., 2008), they are also less mobile than horses (Ganskopp and Vavra, 1987; Kaufmann et al., 2013) and may simply be constrained to areas with higher accessibility, including linear features and relatively flat terrain (Table 5).
Interestingly, elk summer occupancy was also higher in rangeland, similar to horses and cattle, though distance to conifer forest had the largest effect on elk occupancy (Table 5). Elk may avoid risky predator habitat near forests (Hebblewhite et al., 2005; Laundre et al., 2001), preferring to graze in open areas while utilizing forest edges and cover to reduce predation risk from pursuit predators such as wolves (Mysterud and Ostbye, 1999; Hernandez and Laundre, 2005). Conifer and deciduous browse are also important for elk in this system (Salter, 1978), and occupancy of rangelands close to forests may provide an optimal balance between foraging opportunities and predator avoidance.
Previous studies in the Foothills ecosystem have found water not to be a limiting factor for horses, despite their strong water dependence (Schoenecker et al., 2016), and our results further support this. Distance to water had little impact on feral horse selection in either winter or summer and did not significantly influence occupancy (Tables 4 and 5). Similarly, while flatter areas were preferred in winter, likely due to accessibility constraints, terrain did not appear to be a limiting factor in summer (Table 5; Figure 3). This may further reflect avoidance of spatial overlap with cattle, which are more restricted to lowland areas (Table 5; Kaufmann et al., 2013).
These findings have important implications for management, particularly given the rapid decline of native grassland habitats across the province (Zapisocki et al., 2022). Overgrazing of rangelands and competition among horses, cattle, and elk remain contentious issues in the Sundre EMZ (Girard et al., 2013; Hebblewhite, 2006a; McInenly, 2004). The widespread presence of cutblocks and their use by horses suggest a potential mechanism for reducing competition when grazing pressure is high (van Beest et al., 2014a). Given that feral horse populations are closely tied to bottom-up processes, further study is needed to assess population-level responses to shifting resource availability. Human-caused landscape changes, particularly forestry activities, are key drivers of feral horse selection and occupancy, warranting further consideration in management strategies.
5 Conclusion
Our study highlights the complex and seasonally variable habitat use of feral horses in the Rocky Mountain Foothills of Alberta, influenced by both natural features and human land use. Horses shifted their spatial strategies in response to competition with cattle, changing forage availability, and environmental conditions, with forestry cutblocks emerging as an important summer resource. These patterns suggest that horses use cutblocks as alternative forage areas during periods of high grazing pressure, while in winter they select habitats that enhance thermoregulation and mobility. The challenges of managing feral horses in multi-use landscapes (Boyce et al., 2021; Scasta et al., 2018) underscore the need for continued research to better understand their ecology and support evidence-based management. Given ongoing land-use change in this ecosystem, integrating seasonal dynamics and the role of anthropogenic disturbance into management strategies will be essential for balancing conservation goals and mitigating competition among horses, cattle, elk, and other native species.
Data availability statement
The raw data supporting the conclusions of this article will be made available by the authors, without undue reservation.
Ethics statement
This research was approved by the University of Saskatchewan Animal Research and Ethics Board, Animal Use Protocol 20170117. The study was conducted in accordance with local legislation and institutional requirements.
Author contributions
PB: Conceptualization, Funding acquisition, Methodology, Supervision, Writing – original draft, Writing – review & editing. PM: Conceptualization, Formal Analysis, Methodology, Writing – original draft, Writing – review & editing.
Funding
The author(s) declare that financial support was received for the research and/or publication of this article. PB was funded by the University of Saskatchewan, Alberta Conservation Association, and Wild Horses of Alberta Society. Additional funding for field work provided by a Discovery Grant to PDM from the Natural Sciences and Engineering Research Council (Canada), Award No: 2016–06459 and 2019-04388; and Alberta Environment and Parks, Office of the Chief Scientist (Jonathan Thompson).
Acknowledgments
We thank Bruce Stover, Jocelyn Poissant, Nigel Caulkett, Todd Shury, Wayne Linklater, Melanie Boudreau, Erick Lundgren, Jack Nichol, and Klara Johannesson for intellectual contributions and field work. Special thanks to the Wild Horses of Alberta Society and Alberta Environment and Parks for assisting us with logistics and funding associated with the project.
Conflict of interest
The authors declare that the research was conducted in the absence of any commercial or financial relationships that could be construed as a potential conflict of interest.
Generative AI statement
The author(s) declare that no Generative AI was used in the creation of this manuscript.
Publisher’s note
All claims expressed in this article are solely those of the authors and do not necessarily represent those of their affiliated organizations, or those of the publisher, the editors and the reviewers. Any product that may be evaluated in this article, or claim that may be made by its manufacturer, is not guaranteed or endorsed by the publisher.
References
Alberta Agriculture and Forestry. (2016). Alberta vegetation inventory standards - V2.1.5. (Edmonton, AB: Government of Alberta).
Alberta Biodiversity Monitoring Institute. (2018) “ABMI human footprint inventory: wall-to-wall human footprint inventory,” in Alberta biodiversity monitoring institute and alberta human footprint monitoring program. (Edmonton, AB: Government of Alberta).
Alberta Environment and Parks. (2017). Alberta provincial digital elevation model (Government of Alberta).
Alberta Environment and Parks. (2021). “Feral horses in alberta,” in Land - land management. Ed. Parks A. E. (Edmonton, AB: Government of Alberta). Available at: http://aep.alberta.ca/land/land-management/feral-horses/default.aspx.
Anderson D. and Burnham K. (2004). Model selection and multi-model inference. Second. NY: Springer-Verlag 63, 10.
Blaze video Canada (2022). User manual. Available online at: http://www.blazevideo.ca/collections (Accessed August 25, 2022).
Beery S., Morris D., and Yang S. (2019). Efficient pipeline for camera trap image review. ArXiv Preprint ArXiv 1907, 06772. doi: 10.48550/arXiv.1907.06772
Berger J. (1986). “Wild horses of the great basin: social competition and population size,” in Books on demand (Chicago, IL: University of Chicago Press). Available at: https://books.google.ca/books?id=RmQzAAAACAAJ.
Bonacic C., Almuna R., and Ibarra J. T. (2019). Biodiversity conservation requires management of feral domestic animals. Trends Ecol. Evol. 34, 683–686. doi: 10.1016/j.tree.2019.05.002
Boyce P. (2022). Feral horse ecology in the Rocky Mountain Foothills of Alberta, Canada. Dissertation (Saskatoon, Canada: Department of Biology, University of Saskatchewan).
Boyce P. N., Hennig J. D., Brook R. K., and McLoughlin P. D. (2021). Causes and consequences of lags in basic and applied research into feral wildlife ecology: the case for feral horses. Basic Appl. Ecol. 53, 154–163. doi: 10.1016/j.baae.2021.03.011
Boyce P. N. and McLoughlin P. D. (2021). Ecological interactions involving feral horses and predators: review with implications for biodiversity conservation. J. Wildlife Manage. 85, 1091–1103. doi: 10.1002/jwmg.21995
Burnham K. P. and Anderson D. R. (2002). Model selection and multimodel inference : a practical information-theoretic approach, 2nd ed. (New York: Springer-Verlag).
Cade B. S. (2015). Model averaging and muddled multimodel inferences. Ecology 96, 2370–2382. doi: 10.1890/14-1639.1
Clutton-Brock J. (1981). Domesticated animals from early times (1st Univer). Austin : University of Texas Press (London : British Museum Natural History).
Core Team R. (2022). R: A language and environment for statistical computing (Vienna, Austria: R Foundation for Statistical Computing). Available at: https://www.r-project.org. (Accessed December 30, 2024).
DeCesare N. J., Hebblewhite M., Bradley M., Hervieux D., Neufeld L., and Musiani M. (2014). Linking habitat selection and predation risk to spatial variation in survival. J. Anim. Ecol. doi: 10.1111/1365-2656.12144
DeMars C. A. and Boutin S. (2018). Nowhere to hide: effects of linear features on predator–prey dynamics in a large mammal system. J. Anim. Ecol. 87, 274–284. doi: 10.1111/jane.2018.87.issue-1
Dickie M., McNay S. R., Sutherland G. D., Cody M., and Avgar T. (2020). Corridors or risk? Movement along, and use of, linear features varies predictably among large mammal predator and prey species. J. Anim. Ecol. 89, 623–634. doi: 10.1111/1365-2656.13130
Doherty T. S., Hays G. C., and Driscoll D. A. (2021). Human disturbance causes widespread disruption of animal movement. Nat. Ecol. Evol. 5, 513–519. doi: 10.1038/s41559-020-01380-1
Fahrig L. and Rytwinski T. (2009). Effects of roads on animal abundance: an empirical review and synthesis. Ecol. Soc. 14, 21. doi: 10.5751/ES-02815-140121
Fisher J. T., Anholt B., and Volpe J. P. (2011). Body mass explains characteristic scales of habitat selection in terrestrial mammals. Ecol. Evol. 1, 517–528. doi: 10.1002/ece3.45
Fortin D., Beyer H. L., Boyce M. S., Smith D. W., Duchesne T., and Mao J. S. (2005). Wolves influence elk movements: Behavior shapes a trophic cascade in Yellowstone National Park. Ecology 86, 1320–1330. doi: 10.1890/04-0953
Frasier T.R., Lucas Z., McLeod B.A., and McLoughlin P.D.. (2016) “The horses of Sable Island (Ch. 16),” in Sable Island: Explorations in Ecology & Biodiversity. Ed. Freedman B.(Markham, ON: Fitzhenry & Whiteside), 271–299.
Fu P. and Rich P. M. (2002). A geometric solar radiation model with applications in agriculture and forestry. Comput. Electron. Agric. 37, 25–35. doi: 10.1016/S0168-1699(02)00115-1
Ganskopp D. and Vavra M. (1986). Habitat use by feral horses in the northern sagebrush steppe. J. Range Manage. 39, 207–212.
Ganskopp D. and Vavra M. (1987). Slope use by cattle, feral horses, deer, and bighorn sheep. Northwest Sci. 61, 74–81.
Garrott R. A., White P. J., and Watson F. G. R. (2008). The ecology of large mammals in central Yellowstone: sixteen years of integrated field studies (London, UK: Academic Press).
Girard T. (2012). Habitat selection by feral horses in the alberta foothills. Ed. Bork E. (ProQuest Dissertations Publishing).
Girard T., Bork E., Neilsen S., and Alexander M. (2013a). Landscape-scale factors affecting feral horse habitat use during summer within the rocky mountain foothills. Environ. Manage. 51, 435–447. doi: 10.1007/s00267-012-9987-2
Girard T. L., Bork E. W., Nielsen S. E., and Alexander M. J. (2013b). Seasonal variation in habitat selection by free-ranging feral horses within alberta’s forest reserve. Rangeland Ecol. Manage. 66, 428–437. doi: 10.2111/REM-D-12-00081.1
Government of Alberta (2008). Alberta land-use framework. Available online at: https://open.alberta.ca/publications/9780778566976 (Accessed December 30, 2024).
Grant E. H. C., Green L. E., and Lowe W. H. (2009). Salamander occupancy in headwater stream networks. Freshwater Biol. 54, 1370–1378. doi: 10.1111/j.1365-2427.2009.02166.x
Greenberg S., Godin T., and Whittington J. (2019). Design patterns for wildlife-related camera trap image analysis. Ecology and Evolution 9(24), 13706–13730. doi: 10.1002/ece3.5767
Hall L. K., Larsen R. T., Knight R. N., and McMillan B. R. (2018). Feral horses influence both spatial and temporal patterns of water use by native ungulates in a semi-arid environment. Ecosphere 9, e02096. doi: 10.1002/ecs2.2096
Hebblewhite M. (2006a). Linking predation risk and forage to ungulate population dynamics (University of Alberta, Edmonton, Canada: Dissertation).
Hebblewhite M., Merrill E. H., and McDonald T. L. (2005). Spatial decomposition of predation risk using resource selection functions: an example in a wolf–elk predator–prey system. Oikos 111, 101–111. doi: 10.1111/j.0030-1299.2005.13858.x
Hebblewhite M., Merrill E. H., Morgantini L. E., White C. A., Allen J. R., Bruns E., et al. (2006b). Is the migratory behavior of montane elk herds in peril? The case of alberta’s ya ha tinda elk herd. Wildlife Soc. Bull. 34, 1280–1294. doi: 10.2193/0091-7648(2006)34[1280:ITMBOM]2.0.CO;2
Hernandez L. and Laundre J. W. (2005). Foraging in the “landscape of fear” and its implications for habitat use and diet quality of elk Cervus elaphus and bison. Wildlife Biol. 11, 215–220. doi: 10.2981/0909-6396(2005)11[215:fitlof]2.0.co;2
Irving B. (2001). impacts of horse grazing on conifer regeneration in west-central Alberta (Edmonton, AB: University of Alberta).
Janis C. (1976). The evolutionary strategy of the equidae and the origins of rumen and cecal digestion. Evolution 30, 757–774. doi: 10.2307/2407816
Johnson D. H. (1980). The comparison of usage and availability measurements for evaluating resource preference. Ecology 61, 65–71. doi: 10.2307/1937156
Kaufmann J. (2011). Interactions between cattle grazing and forestry on Alberta’s public lands. (Edmonton, AB: M.Sc. Thesis).
Kaufmann J., Bork E. W., Blenis P. V, and Alexander M. J. (2013). Cattle habitat selection and associated habitat characteristics under free-range grazing within heterogeneous Montane rangelands of Alberta. Appl. Anim. Behav. Sci. 146, 1–10. doi: 10.1016/j.applanim.2013.03.014
Kays R., Arbogast B. S., Baker-Whatton M., Beirne C., Boone H. M., Bowler M., et al. (2020). An empirical evaluation of camera trap study design: How many, how long and when? Methods Ecol. Evol. 11, 700–713. doi: 10.1111/2041-210X.13370
Kluever B. M., Breck S. W., Howery L. D., Krausman P. R., and Bergman D. L. (2008). Vigilance in cattle: the influence of predation, social interactions, and environmental factors. Rangeland Ecol. Manage. 61, 321–328. doi: 10.2111/07-087.1
Knopff K. H. (2010). “Cougar kill rate in a multi-prey System in West-Central Alberta,” Dissertation (University of Alberta).
Kuijper D. P. J., Bubnicki J. W., Churski M., Mols B., and van Hooft P. (2015). Context dependence of risk effects: wolves and tree logs create patches of fear in an old-growth forest. Behav. Ecol. 26, 1558–1568. doi: 10.1093/beheco/arv107
Latham A. D. M., Latham M. C., Boyce M. S., and Boutin S. (2011). Movement responses by wolves to industrial linear features and their effect on woodland caribou in northeastern Alberta. Ecol. Appl. 21, 2854–2865. doi: 10.1890/11-0666.1
Laundre J. W., Hernandez L., and Altendorf K. B. (2001). Wolves, elk, and bison: reestablishing the “landscape of fear” in Yellowstone National Park, USA. Can. J. Zoology-Revue Can. Zoologie 79, 1401–1409. doi: 10.1139/cjz-79-8-1401
Leblond M., Dussault C., and Ouellet J. (2013). Avoidance of roads by large herbivores and its relation to disturbance intensity. J. Zoology 289, 32–40. doi: 10.1111/j.1469-7998.2012.00959.x
Lotek Wireless Inc. (2022). Lotek terrestrial GPS wildlife collars. Available online at: http://www.lotek.com/products (Accessed August 25, 2022).
MacFadden B. J. (1994). Fossil horses: systematics, paleobiology, and evolution of the family Equidae (Cambridge University Press).
MacKenzie D. I. and Bailey L. L. (2004). Assessing the fit of site-occupancy models. J. Agricultural Biological Environ. Stat 9, 300–318.
MacKenzie D. I., Nichols J. D., Lachman G. B., Droege S., Royle J. A., and Langtimm C. A. (2002). Estimating site occupancy rates when detection probabilities are less than one. Ecology 83, 2248–2255. doi: 10.2307/3072056
Manly B. F. J. (2002). Resource selection by animals : statistical design and analysis for field studies. 2nd ed (Dordrecht Boston : Kluwer Academic Publishers).
McFarlane B. L. and Boxall P. C. (1996). Exploring forest and recreation management preferences of forest recreationists in Alberta. Forestry Chronicle 72, 623–629. doi: 10.5558/tfc72623-6
McInenly L. E. (2004). Seasonal effects of defoliation on montane rough fescue, festuca campestris (Rydb.) (Ottawa: National Library of Canada= Bibliotheque nationale du Canada).
McKnight T. L. (1959). The feral horse in Anglo-America. Geographical Rev. 49, 506–525. doi: 10.2307/212210
Ménard C., Duncan P., Fleurance G., Georges J.-Y., and Lila M. (2002). Comparative foraging and nutrition of horses and cattle in European wetlands. J. Appl. Ecol. 39, 120–133. doi: 10.1046/j.1365-2664.2002.00693.x
Mysterud A. and Ostbye E. (1999). Cover as a habitat element for temperate ungulates: effects on habitat selection and demography. Wildlife Soc. Bull. 27, 385–394.
Ostermann-Kelm S., Atwill E. R., Rubin E. S., Jorgensen M. C., and Boyce W. M. (2009). Impacts of feral horses on a desert environment. BMC Ecol. 9, 22. doi: 10.1186/1472-6785-9-22
Preston K. B. (1984). A habitat-use and dietary analysis of a monogastric versus a ruminant herbivore, on forested range (Master’s thesis) (Vancouver, Canada: University of British Columbia).
Prokopenko C. M., Boyce M. S., and Avgar T. (2017). Characterizing wildlife behavioural responses to roads using integrated step selection analysis. J. Appl. Ecol. 54, 470–479. doi: 10.1111/1365-2664.12768
Riley S. J., DeGloria S. D., and Elliot R. (1999). Index that quantifies topographic heterogeneity. Intermountain J. Sci. 5, 23–27.
Salter R. E. and Hudson R. J. (1979). Feeding ecology of feral horses in western Alberta. J. Range Manage. 32, 221–225. doi: 10.2307/3897127
Salter R. E. and Hudson R. J. (1980). Range relationships of feral horses with wild ungulates and cattle in western Alberta. J. Range Manage. 33, 266–271. doi: 10.2307/3898070
Scasta J. D., Hennig J., and Beck J. L. (2018). Framing contemporary US wild horse and burro management processes in a dynamic ecological, sociological, and political environment. Human–Wildlife Interact. 12, 6. doi: 10.26077/2fhw-fz24
Schneider R. R. (2002). Alternative futures: Alberta’s boreal forest at the crossroads (Federation of Alberta Naturalists Edmonton).
Schoenecker K. A., King S. R. B., Nordquist M. K., Nandintsetseg D., and Cao Q. (2016). Habitat and diet of equids. Wild Equids—Ecology Management Conserv. Johns Hopkins Univ. Press Baltimore U.S.A., 41–55.
Shoemaker L. and Clauset A. (2014). Body mass evolution and diversification within horses (family Equidae). Ecol. Lett. 17, 211–220. doi: 10.1111/ele.12221
Slade L. M., Robinson D. W., and Casey K. E. (1970). Nitrogen metabolism in nonruminant herbivores. I. The influence of nonprotein nitrogen and protein quality on the nitrogen retention of adult mares. J. Anim. Sci. 30, 753–760. doi: 10.2527/jas1970.305753x
Stealth Cam. (2022). Trail cameras (Edmonton, AB: Government of Alberta). Available online at: http://www.stealthcam.com/cameras (Accessed August 25, 2022).
Stewart F. E. C., Volpe J. P., and Fisher J. T. (2019). The debate about bait: a red herring in wildlife research. J. Wildlife Manage. 83, 985–992. doi: 10.1002/jwmg.21657
Stover B. C. and Caulkett N. A. (2021). Anesthesia techniques used for field castration of 10 intractab le horses. Can. Veterinary J. 62, 501.
Sutherland C., Fuller A. K., and Royle J. A. (2015). Modelling non-Euclidean movement and landscape connectivity in highly structured ecological networks. Methods Ecol. Evol. 6, 169–177. doi: 10.1111/2041-210x.12316
Thistle J. (2008). Accommodating cattle: British Columbia’s” Wars” with Grasshoppers and” Wild Horses. Bc Stud. 160, 67. doi: 10.14288/bcs.v0i160.569
Treves A., Wallace R. B., Naughton-Treves L., and Morales A. (2006). Co-managing human–wildlife conflicts: A review. Hum. Dimensions Wildlife 11, 383–396. doi: 10.1080/10871200600984265
Trombulak S. C. and Frissell C. A. (2000). Review of ecological effects of roads on terrestrial and aquatic communities. Conserv. Biol. 14, 18–30. doi: 10.1046/j.1523-1739.2000.99084.x
Underhill J. E. and Angold P. G. (1999). Effects of roads on wildlife in an intensively modified landscape. Environ. Rev. 8, 21–39. doi: 10.1139/a00-003
van Beest F. M., McLoughlin P. D., Vander Wal E., and Brook R. K. (2014a). Density-dependent habitat selection and partitioning between two sympatric ungulates. Oecologia 175, 1155–1165. doi: 10.1007/s00442-014-2978-7
Vectronic Aerospace GmbH. (2022). SURVEY collars. Available online at: https://www.vectronic-aerospace.com/vertex-plus-collar/ (Accessed August 25, 2022).
Webb N. F. (2009). “Density, demography, and functional response of a harvested wolf population in west-central Alberta, Canada,” Dissertation (University of Alberta).
Whittington J., St. Clair C. C., and Mercer G. (2005). Spatial responses of wolves to roads and trails in mountain valleys. Ecol. Appl. 15, 543–553. doi: 10.1890/03-5317
Willoughby M. G., Alexander M. J., and Sundquist K. M. (1998). “Range plant community types and carrying capacity for the montane subregion,” in Environmental protection lands and forest service,(Edmonton, Alberta, Canada).
Zapisocki Z., de Assis Murillo R., and Wagner V. (2022). Non-native plant invasions in prairie grasslands of alberta, Canada. Rangeland Ecol. Manage. 83, 20–30. doi: 10.1016/j.rama.2022.02.011
Keywords: camera trap, cattle, disturbance, ELK, feral horse, grazing ecology, occupancy modeling, resource selection
Citation: Boyce PM and McLoughlin PD (2025) Habitat selection and occupancy of feral horses in comparison to cattle and elk in the Rocky Mountain Foothills of Canada. Front. Conserv. Sci. 6:1585546. doi: 10.3389/fcosc.2025.1585546
Received: 28 February 2025; Accepted: 30 April 2025;
Published: 23 May 2025.
Edited by:
Saeideh Esmaeili, Colorado State University, United StatesReviewed by:
Mateusz Rozmiarek, Poznan University of Physical Education, PolandTamao Maeda, Kyoto University, Japan
Jacob Hennig, University of Arizona, United States
Copyright © 2025 Boyce and McLoughlin. This is an open-access article distributed under the terms of the Creative Commons Attribution License (CC BY). The use, distribution or reproduction in other forums is permitted, provided the original author(s) and the copyright owner(s) are credited and that the original publication in this journal is cited, in accordance with accepted academic practice. No use, distribution or reproduction is permitted which does not comply with these terms.
*Correspondence: Philip D. McLoughlin, cGhpbGlwLm1jbG91Z2hsaW5AdXNhc2suY2E=