- Cardioprotection Group, Hatter Institute for Cardiovascular Research in Africa, Department of Medicine, Faculty of Health Sciences, University of Cape Town, Cape Town, South Africa
The role of the diet as well as the impact of the dietary habits on human health and disease is well established. Apart from its sleep regulatory effect, the indoleamine melatonin is a well-established antioxidant molecule with multiple health benefits. Convincing evidence supports the presence of melatonin in plants and foods with the intake of such foods affecting circulating melatonin levels in humans. While numerous actions of both endogenous melatonin and melatonin supplementation are well described, little is known about the influence of the dietary melatonin intake on human health. In the present review, evidence for the cardiovascular health benefits of melatonin supplementation and dietary melatonin is discussed. Current knowledge on the biological significance as well as the underlying physiological mechanism of action of the dietary melatonin is also summarized. Whether dietary melatonin constitutes an alternative preventive treatment for cardiovascular disease is addressed.
Introduction
The role of the diet as well as the impact of the dietary habits on human health and disease has been described since the antiquity (Skiadas and Lascaratos, 2001). In ancient Greece, excess food intake was considered as unhealthy and a cause of illness, whereas a moderate diet made of cereals, legumes, fruits, milk, honey and fish with a modest consumption of meat, confectionery and wine, as currently found in the common Mediterranean diet, was recommended as healthy (Skiadas and Lascaratos, 2001). Today, the health benefits of the Mediterranean diet are well established (Estruch et al., 2013; Afshin et al., 2014). Accordingly, supplementation of the Mediterranean diet with extra-virgin olive oil or nuts is associated with a reduction in the risk of major cardiovascular events among high-risk persons (Estruch et al., 2013). The health-promoting properties of the Mediterranean diet are attributed to its various food ingredients including, amongst others, flavonoids (Kruger et al., 2014) and, more recently, melatonin (Lecour and Lamont, 2011), therefore supporting the current growing interest in the dietary sources and bioactivities of melatonin (Peuhkuri et al., 2012; Johns et al., 2013; Sae-Teaw et al., 2013; Tan et al., 2014; Iriti and Varoni, 2015; Meng et al., 2017b).
Melatonin or N-acetyl-5-methoxytryptamine is a highly conserved indoleamine molecule found in all microorganisms (Hardeland and Fuhrberg, 1996), plants and animals (Reiter et al., 2001; Tan et al., 2012). Originally identified in the bovine pineal gland (Lerner et al., 1958), melatonin is also produced by a wide range of tissues including the retina, thymus, spleen, heart, muscle, liver, stomach, pancreas, intestine, placenta, testis, ovaries, bone marrow, skin and hair follicle, cerebral cortex, and striatum (Stefulj et al., 2001; Venegas et al., 2012; Acuna-Castroviejo et al., 2014). The content of melatonin in these tissues varies and decreases with age to a similar extent as its pineal production (Sanchez-Hidalgo et al., 2009; Scholtens et al., 2016). After biosynthesis, pineal melatonin is immediately released into the circulatory system and reaches all biological fluids including cerebrospinal fluid, bile, saliva, synovial fluid, semen, ovarian follicular fluid, amniotic fluid, breast milk and tears (Illnerova et al., 1993; Acuna-Castroviejo et al., 2014; Carracedo et al., 2017). In physiological conditions, the extra-pineal melatonin does not seem to significantly contribute to the circulating levels of melatonin (Acuna-Castroviejo et al., 2014).
Historically, the isolation and identification of melatonin in the bovine pineal gland in 1958 (Lerner et al., 1958) was motivated by the potential dermatological effects of the pineal gland extracts reported early in 1917 with the hypothesis that melatonin could play a major role in the skin lightening (McCord and Allen, 1917). Unfortunately, the skin lightening properties could not be further demonstrated (Lerner et al., 1958; McElhinney et al., 1994) and the project was then abandoned. In the early 1990s, the research conducted on melatonin received popular attention with the media to the point that its multiple actions including its potential anti-ageing activities were at some extent described as “a miracle” or “a mystery” due to a lack of scientific evidence (Reppert and Weaver, 1995). Also, the role of melatonin in the physiological regulation of seasonal and circadian rhythms (Arendt, 1998), its antioxidant properties (Tan et al., 1993) and the presence of its specific receptors (Hattori et al., 1995) were progressively established in various experimental models. Since then, numerous studies have demonstrated additional properties of melatonin (for review, see Acuna-Castroviejo et al., 2014; Reiter et al., 2016; Favero et al., 2017), making the wide range of actions of melatonin a reality and not a myth. It is now well established that endogenous melatonin induces multiple physiological responses in humans including, amongst others, synchronizing the circadian rhythms of the body, regulation of the sleep-wakefulness cycle, antioxidant capacity, modulation of the immune system and the cardiovascular system (Hardeland et al., 2011; Reiter et al., 2016).
The health benefits of melatonin as a nutritional supplement are widely accepted [EFSA Panel on Dietetic Products, Nutrition and Allergies (NDA), 2011]. Currently, melatonin is only prescribed for the regulation of sleeping patterns such as in the jet lag (Herxheimer and Petrie, 2002) and adult sleep disorders (Auld et al., 2017). However, growing evidence supports the multi-organ effects of melatonin (Opie and Lecour, 2016) with a therapeutic potential in cardiovascular pathologies (Sun et al., 2016; Pandi-Perumal et al., 2017), neurodegenerative diseases (Trotti and Karroum, 2016), reproductive diseases (Reiter et al., 2009), bone diseases (osteopenia, osteoporosis, and periodontal disease) (Maria and Witt-Enderby, 2014), various cancers (Reiter et al., 2017), skin diseases (Fischer et al., 2013) and metabolic disorders (Nduhirabandi et al., 2012; Navarro-Alarcon et al., 2014). Interestingly, melatonin is present in edible plants, meats, fruits, beverage and other food stuffs (Dubbels et al., 1995; Hattori et al., 1995; Hardeland and Pandi-Perumal, 2005; Stürtz et al., 2011; Tan et al., 2014; Herrera et al., 2018). Although the levels of melatonin in foods are much lower than those of melatonin given as a nutritional supplement, consumption of foods rich in melatonin significantly increases circulating melatonin levels in the range of the physiological concentrations (Maldonado et al., 2009; Johns et al., 2013; Sae-Teaw et al., 2013). However, little is known about the influence of the dietary melatonin intake on human health. In this review, evidence for cardiovascular health benefits of endogenous melatonin and melatonin supplementation as a pharmacological agent or from the diet is discussed.
Evidence for Cardiovascular Benefits of Endogenous Melatonin and Melatonin Supplementation
A strong inverse relationship exists between endogenous melatonin levels and cardiovascular disease (Dominguez-Rodriguez et al., 2010). Epidemiological studies report that both nocturnal melatonin synthesis and circulating levels are reduced in patients with coronary heart disease (Brugger et al., 1995; Altun et al., 2002; Dominguez-Rodriguez et al., 2002), hypertension (Kozirog et al., 2011; Dominguez-Rodriguez et al., 2014), heart failure (Girotti et al., 2003; Dzida et al., 2013; Kimak et al., 2014; Dominguez-Rodriguez et al., 2016) and cardiovascular risk conditions such as diabetes (McMullan et al., 2013) and obesity (Mantele et al., 2012). Incidence for adverse cardiac events, including myocardial infarction (Dominguez-Rodriguez et al., 2002), sudden cardiac death (Muller et al., 1987) and cardiac arrhythmias (Siegel et al., 1992) increases in the early morning, when circulating melatonin levels are considerably lower (Altun et al., 2002). Similarly, low melatonin secretion levels are associated with a greater risk of incidence for myocardial infarction in women with increased body mass index (McMullan et al., 2017), supporting the crucial role of endogenous melatonin in cardiovascular pathologies.
Solid evidence supports the beneficial effects of melatonin supplementation in various cardiovascular pathologies (for review, see Paulis and Simko, 2007; Reiter et al., 2010; Sun et al., 2016). Since the cardiovascular benefits of melatonin have recently been reviewed elsewhere (Sun et al., 2016), only the evidence for the benefits of melatonin supplementation in hypertension, pulmonary hypertension and ischemic heart disease is summarized below.
Melatonin and Hypertension and Other Vascular Pathologies
Endogenous and exogenous melatonin play an important role in hypertension and other vascular pathologies (Grossman et al., 2006; Paulis and Simko, 2007; Mozdzan et al., 2014; Simko et al., 2016). In animal studies, continuous light exposure or pinealectomy with a subsequent melatonin deficiency (Brown et al., 1991; Iigo et al., 1995) results in an increase in blood pressure (BP), a condition which is reversed by melatonin supplementation (Simko et al., 2014b). A similar finding is also reported in spontaneous hypertensive (Tain et al., 2010) and metabolic syndrome (Kantar et al., 2015) rats supplemented with melatonin, thereby confirming its therapeutic potential in hypertension.
In human studies, night time melatonin administration (2–5 mg/day for 3–4 weeks) reduces BP values of hypertensive men (Scheer et al., 2004; Grossman et al., 2006) or women (Cagnacci et al., 2005) as well as normotensive women (Cagnacci et al., 2005). In patients with metabolic syndrome, melatonin supplementation (5 mg/day, 2 h before bedtime) for 2 months reduces the systolic and diastolic BP, low-density lipoprotein cholesterol (LDL-C), thiobarbituric acid reactive substrates (TBARS, a marker of oxidative stress) and increases antioxidant defense (catalase activity) (Kozirog et al., 2011). These beneficial effects are also observed in patients with essential hypertension receiving medical treatment where melatonin (3 mg or 5 mg for 4 weeks) restores the normal circadian rhythm of BP (Mozdzan et al., 2014). However, in patients with postural tachycardia syndrome (characterized by an excessive increase in heart rate with upright posture accompanied by palpitations), melatonin does not affect systolic BP despite a modest decrease in standing tachycardia (Green et al., 2014).
Figure 1 summarizes the mechanisms of actions of melatonin in hypertension and vascular pathologies. These mechanisms involve the indirect regulation of blood pressure via the central nervous system and the modulation of catecholamine secretion, the direct antioxidative and anti-inflammatory activities, the relaxation of the smooth muscle in blood vessels via α1-adrenergic receptors, nitric oxide production and calcium signaling, and the improvement of insulin signaling in insulin resistance states (Paulis and Simko, 2007; Paulis et al., 2010; Kantar et al., 2015; Agabiti-Rosei et al., 2017). In high-fat-fed rabbits, melatonin ameliorates vascular endothelial dysfunction and inflammation (Hu et al., 2013), the major contributing factors of the initiation and progression of atherosclerosis. Melatonin also improves endothelial vascular function and oxidative stress in type 2 diabetic rats (Salmanoglu et al., 2016) and insulin-resistant mice (Sartori et al., 2009). In a senescence-accelerated prone mice (SAMP8, a model of age-related vascular dysfunction), a 10-month melatonin treatment increases the expression of adiponectin and adiponectin receptor 1 in the visceral adipose tissue, the markers of vasoprotection (upregulation of eNOS and sirtuin 1 (SIRT1) and downregulation of endothelin-1 and iNOS) and inhibits aorta hypertrophy (by reducing oxidative stress and inflammation) while restoring the anticontractile effect of the perivascular adipose tissue (Agabiti-Rosei et al., 2017).
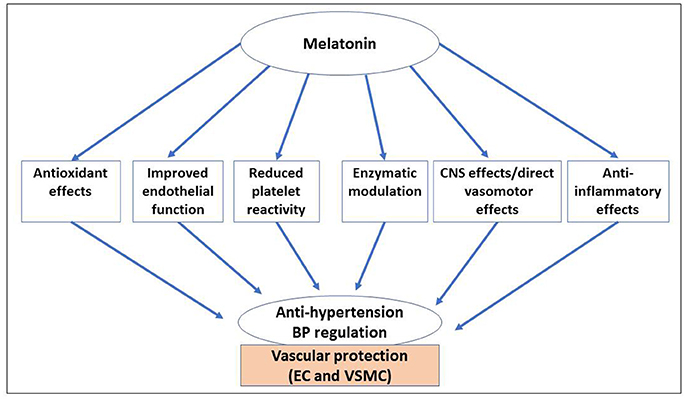
Figure 1. Benefits of melatonin in hypertension and vascular pathologies. Melatonin positively affects vascular function (endothelial and smooth vascular muscle cells) via its direct and indirect regulatory effects associated with its strong antioxidant, anti-inflammatory, anti-lipidemic and vasomotor properties (vasodilation), all contributing to BP regulation (anti-hypertensive effects). These effects are also associated with enzymatic modulation, improved endothelial function and a reduced platelet reactivity. BP, blood pressure; CNS, central nervous system; EC, endothelial cells; CSMC, vascular smooth muscle cells.
As a potential therapy in vascular disease, melatonin improves vascular function by decreasing the expression of platelets, endothelial cells adhesion molecule-1 (CD31), intercellular adhesion molecule-1 (ICAM-1), vascular cell adhesion molecule-1 (VCAM-1) and endothelin-1 (ET-1) while it increases endothelial nitric oxide synthase (eNOS), nuclear erythroid 2-related factor 2 (Nrf2), NAD(P)H quinone oxidoreductase 1 (NQO-1), catalytic glutamate cysteine ligase (GCLC) and heme oxygenase-1 (HO-1) in a rat model of a smoke-induced vascular injury (Wang et al., 2016). Similarly, in a population smoking more than 10 cigarettes per day for at least 1 year, supplementation of melatonin (3 mg/day for 2 weeks) reduces the concentration of fibrinogen and free fatty acids, the expression of ICAM-1, VCAM-1 and ET-1, and increases the expressions of Nrf2 and HO-1 (Wang et al., 2016).
Melatonin and Pulmonary Hypertension
Hypoxia-induced inflammation and excessive proliferation of pulmonary artery smooth muscle cells (PASMCs) play an important role in the pathological process of pulmonary hypertension and subsequent heart failure (Jin et al., 2014). Melatonin improves hypoxia-induced pulmonary hypertension by suppressing the hypoxia-induced high expression of proliferating cell nuclear antigen (PCNA), hypoxia-inducible factor-1α (HIF-1α), and nuclear factor-κB (NF-κB) (Jin et al., 2014). These effects are associated with inhibition of proliferation of PASMCs, the levels of phosphorylation of protein kinase B (PKB/Akt) and extracellular signal-regulated kinases1/2 (ERK1/2) caused by hypoxia (Jin et al., 2014), supporting the preventive activities of melatonin via its anti-inflammatory and anti-proliferative mechanisms. Similar findings are also reported in a new born sheep model of pulmonary hypertension (Torres et al., 2015). In this model, melatonin improves the vasodilator function of small pulmonary arteries, enhancing the endothelial-and muscular-dependent pathways associated with enhanced nitric oxide-dependent and independent vasodilator components and increased bioavailability of nitric oxide in lung tissue (Torres et al., 2015).
Melatonin and Cardiac Diseases
Melatonin induces multiple actions in various cardiac pathologies (Reiter et al., 2010; Lochner et al., 2013; Yang et al., 2014; Sun et al., 2016; Favero et al., 2017). In this paper, only relevant evidence in myocardial infarction, myocardial ischemia/reperfusion (I/R) injury and heart failure is included (Tables 1, 2).
Melatonin supplementation protects the heart in several experimental models of myocardial infarction and myocardial I/R injuries (Lochner et al., 2013; Yang et al., 2014). Tan and co-workers were first to demonstrate the beneficial effects of melatonin in isolated rat hearts subjected to cardiac I/R-induced arrhythmias: infusion of melatonin (during the period of ischemia and reperfusion or reperfusion only) reduces premature ventricular contraction and ventricular fibrillation due to occlusion and reopening of the anterior descending coronary artery (Tan et al., 1998). This finding is also reported in other animal models of cardiac arrhythmias (Lagneux et al., 2000; Sahna et al., 2002; Diez et al., 2013), confirming the therapeutic potential for melatonin in reducing the incidence of sudden cardiac death (Sahna et al., 2002). Additional studies show that short-and long-term melatonin supplementation is cardioprotective in various in vivo and ex vivo myocardial models of I/R injury (Lagneux et al., 2000; Lochner et al., 2006; Lamont et al., 2011; Nduhirabandi et al., 2011, 2014, 2016; Maarman et al., 2015; Zhai et al., 2017a,b). In these studies, administration of melatonin before or after ischemia preserves the microstructure of the cardiomyocyte and reduced myocardial I/R injury as indicated by a reduction in myocardial infarct size (for review, see Lochner et al., 2013).
The mechanisms of cardioprotection induced with melatonin against myocardial infarction or I/R injury are complex and not well understood. As presented in the Figure 2, melatonin may directly and indirectly affect cardiac pathologies via multiple mechanisms including amongst others, antihypertensive, antilipidemic, antiadrenergic, and immunomodulatory activities. It also reduces oxidative stress, apoptosis, necrosis, mitochondrial permeability transition pore opening, lipid peroxidation and inflammation (for more details, see Yang et al., 2014). Recent findings in the mechanisms of melatonin-induced cardioprotection suggest the involvement of the intracellular survival signaling pathways including, mainly, the activation of the survivor activating factor enhancement (SAFE), the reperfusion injury salvage kinase risk (RISK), Notch1 and sirtuins (SIRT1 and SIRT 3) signaling pathways (Lochner et al., 2013; Nduhirabandi et al., 2014, 2016; Yu et al., 2017b; Zhai et al., 2017a) as well as the crucial role of the mitochondria in cell death and survival (necrosis, apoptosis, autophagy, mitophagy) (Petrosillo et al., 2009; Pei et al., 2016; Hu et al., 2017). The description of these cardioprotective mechanisms is beyond the focus of the present paper (for details, see Lochner et al., 2013; Yang et al., 2014; Sun et al., 2016).
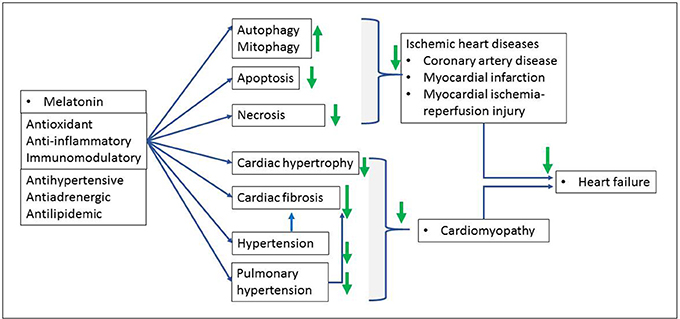
Figure 2. Benefits of melatonin in cardiac pathologies. Melatonin, via its antioxidant, anti-inflammatory and immunomodulatory properties protects against ischemic heart disease as well as subsequent ischemic heart failure characterized by myocardial cell death (necrosis, apoptosis, autophagy) and cardiac dysfunction. Hypertension and pulmonary hypertension induce both cardiac fibrosis and pathological hypertrophy (cardiomyopathy) and subsequent heart failure. Melatonin reverses these effects as indicated with green arrows (↓: increase, ↑: reduce).
Melatonin supplementation is also beneficial for the treatment of pathological cardiac remodeling and heart failure (Chua et al., 2016; Hu et al., 2017; Zhai et al., 2017b). For example, in a mouse model of myocardial infarction, melatonin significantly reduces adverse left ventricle remodeling and post-myocardial infarction remodeling and dysfunction by increasing autophagy, reducing apoptosis, and reversing mitochondrial dysfunction (Hu et al., 2017). As underlying mechanisms, these effects are associated with a significant activation of adenosine monophosphate-activated protein kinase (AMPK) and an inhibition of macrophage-stimulating 1 (MST) phosphorylation while increasing the expression of SIRT1 and SIRT3, peroxisome proliferator-activated receptor gamma (PPARγ) co-activator 1-protein (PGC-1α), the translocase of the outer membrane 70 (Tom 70), a receptor for translocases in the outer mitochondrial membrane complex (Hu et al., 2017; Pei et al., 2017; Yu et al., 2017b). Furthermore, in a murine model of pathological cardiac hypertrophy induced by transverse aortic constriction (TAC), melatonin supplementation for 4–8 weeks reverses the pathological hypertrophy via the reduction of the pulmonary congestion (Zhai et al., 2017b). These effects are associated with an upregulation of the level of α-myosin heavy chain expression, a downregulation of the levels of β-myosin heavy chain and atrial natriuretic peptide expression, an inhibition of oxidative stress (as expressed by the levels of malondialdehyde (MDA) and superoxide dismutase (SOD) activity), and the activation of PGC-1β as well as the reduction of the cardiac fibrosis (Zhai et al., 2017b). Similar protective effects of melatonin with its antifibrotic properties are also reported in a rat model of cardiorenal syndrome (Chua et al., 2016) and in other animal models of heart failure such as myocardial damage induced by chronic intermittent hypoxia (Yeung et al., 2015), isoproterenol (Patel et al., 2010) or epinephrine (Vazan and Ravingerova, 2015), chemotherapy drugs (Liu et al., 2002), sepsis-induced myocardial injury (An et al., 2016), and diabetic cardiomyopathy (Zhang et al., 2017).
In view of the above benefits in animal studies, melatonin supplementation is highly regarded as an effective therapy in cardiac diseases (Yang et al., 2014; Opie and Lecour, 2016; Sun et al., 2016). Unfortunately, very few clinical trials have investigated the effects of exogenous melatonin in cardiac diseases (Dominguez-Rodriguez et al., 2007; Gogenur et al., 2014; Ekelof et al., 2016) (Table 2). In patients undergoing surgery for abdominal aortic aneurisms, an infusion of 50 mg melatonin over a 2 h period followed by oral administration of 10 mg melatonin for the first three nights after surgery, protects the heart against reperfusion injury by reducing cardiac morbidity as well as the occurrence of myocardial ischemia after abdominal aortic aneurism repair (Gogenur et al., 2014). In patients undergoing coronary artery bypass grafting surgery, melatonin supplementation (10 or 20 mg capsule once a day) increases significantly the ejection fraction and the outcomes together with a remarkable decrease in pro-inflammatory and apoptotic markers, supporting its promising benefits in myocardial I/R injury (Dwaich et al., 2016).
The therapeutic potential of melatonin in cardiac diseases has recently received controversial comments with the publication of the study of melatonin as an adjunct in patients with acute myocardial infarction undergoing primary angioplasty (MARIA trial) (Dominguez-Rodriguez et al., 2007, 2017a,b; Hausenloy et al., 2017). Despite the acceptable safety and tolerability, the MARIA trial reports a lack of cardioprotection in patients treated with formulation of melatonin in polyethylene glycol solution (51.7 μmoles given 60 min before intervention and a bolus of 8.6 μmoles 60 min after intervention) (Dominguez-Rodriguez et al., 2017b). A lack of beneficial effects of melatonin is also reported in other clinical study (Ekeloef et al., 2017). Interestingly, preclinical testing showed that melatonin fails to protect the heart in a closed-chest porcine model of acute myocardial infarction (Halladin et al., 2014; Ekelof et al., 2016) and in a rabbit model of myocardial I/R injury (Dave et al., 1998). It is possible that the intracoronary and intravenous administrations, as opposed to the preferred oral administration, may contribute to the neutral outcomes as suggested by other studies (Dwaich et al., 2016). In addition, ischemic duration and other methodological issues (for review, see Heusch, 2017) may also play a role in the outcomes of above clinical trials, suggesting a re-evaluation of the therapeutic effects of melatonin in a well-planed study.
In the light of the very low physiological concentrations of melatonin, it remains unknown whether consumption of melatonin-rich foods, which increases circulating melatonin concentration into physiological range, may be more effective for cardioprotection.
Overview of Cardiovascular Benefits of Melatonin-Rich Food
The presence of melatonin in edible plants and other types of food is well established (Tan et al., 2012, 2014). Melatonin-rich foods include various food components from both animal and plant origins such as chicken, lamb, pork, cow milk, strawberries, tomatoes, olives, grapes, wines, cereals and cherries (for review, see Iriti et al., 2010; Tan et al., 2014) (see Table 3). Interestingly, melatonin concentrations are significantly higher in plants than in animals (Byeon et al., 2014). This is most likely due to differences between the biosynthetic pathways of melatonin in plants and animals (see Figure 3). Plants synthesize tryptophan themselves via the shikimic acid pathway, which increases their melatonin synthetic capacity (Byeon et al., 2014). Animals produce melatonin from tryptophan (essential amino acid from the food) (Byeon et al., 2014). For more details on the biosynthesis of melatonin as well as the dietary source of melatonin, see reviews (Park et al., 2013; Byeon et al., 2014; Tan et al., 2016).
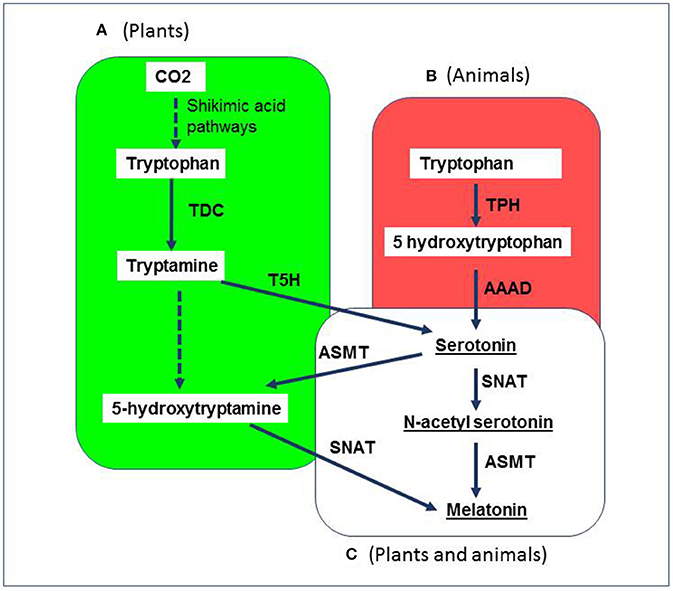
Figure 3. Simplified representation of the biosynthetic pathways of melatonin in plants and animals. Tryptophan is the common precursor of melatonin in all species. (A) In plants (green), melatonin is synthesized under two pathways: (1) tryptophan-tryptamine-serotonin-N-acetyl serotonin-melatonin pathway (under normal growth conditions); (2) tryptophan-tryptamine-serotonin-5-methoxytryptamine-melatonin pathway (upon senescence, when plants produce large amounts of serotonin); (B) In animals (red); tryptophan is converted in serotonin via hydroxytryptophan; (C) In both plants and animals (white), melatonin production from serotonin is the same two-step process and includes the conversion of serotonin to N-acetylserotonin by the rate-limiting enzyme AA-NAT (arylalkylamine N-acetyltransferase) also called as serotonin N-acetyltransferase followed by the conversion of N-acetylserotonin to melatonin by acetylserotonin O-methyltransferase. CO2, carbon dioxide; TPH, tryptophan hydroxylase; AAAD, aromatic amino acid decarboxylase; SNAT, serotonin N-acetyltransferase; ASMT, N-acetylserotonin O-methyltransferase; TDC, tryptophan decarboxylase; T5H, tryptamine 5-hydroxylase.
It is likely that the consumption of melatonin-rich food influences endogenous melatonin production (for review, see Peuhkuri et al., 2012). For example, in chickens, circulating melatonin levels increase more than 3.5-folds 1.5 h after the intake of chick food composed of corn, milo, beans, and rice (3 ng/g melatonin) (Hattori et al., 1995). A similar observation is reported in other studies after the consumption of melatonin-rich foods such as walnuts, olive oil, wine, fruits and legumes as well as germinated kidney beans (Peuhkuri et al., 2012; Johns et al., 2013; Sae-Teaw et al., 2013; Tan et al., 2014; Aguilera et al., 2016). However, it remains unclear whether the acute variation of circulating melatonin levels induced by the consumption of melatonin-rich foods correlates with their influence on the cardiovascular system (Bazzano et al., 2001; Al Abdrabalnabi et al., 2017; Aune et al., 2017; Micha et al., 2017). The evidence for cardiovascular benefits of some melatonin containing foods including, walnuts, tropical fruits and grape products are reviewed below.
Walnuts Consumption
Long and short-term observational and intervention studies show that regular consumption of walnuts reduces the risk of CVDs (Kris-Etherton, 2014; Al Abdrabalnabi et al., 2017). In an elderly population, a daily consumption of walnuts improves blood lipids and BP after a one-year trial (Al Abdrabalnabi et al., 2017). In rats fed walnuts an increase in the blood concentration of melatonin and antioxidant capacity is observed (Reiter et al., 2005) However, the correlation between the variation of melatonin levels and the cardiovascular benefits of walnuts remains to be established.
Tropical Fruits Consumption
Fruits consumption is associated with reduced CVDs (Aune et al., 2017; Micha et al., 2017). Melatonin is present in tropical fruits, namely banana, pineapple, orange, papaya, mango (Johns et al., 2013). Apart from papaya and mango, the consumption of these fruits increases the circulating melatonin as measured by 6-sulphatoxymelatonin in healthy volunteers (Johns et al., 2013). Interestingly, the rise in serum melatonin levels is positively associated with the antioxidant capacity but not the melatonin content in fruits (Johns et al., 2013; Sae-Teaw et al., 2013). However, it remains unknown whether increasing circulating melatonin levels with fruits consumption is beneficial for patients with reduced levels of endogenous melatonin or CVDs and other illnesses involving oxidative damage.
Grapes and Wine Consumption
Chronic and moderate consumption of red wine is associated with a reduced risk of CVDs and other diseases such as diabetes and neurological diseases (Opie and Lecour, 2007). Melatonin is present in grapes and wines; and consumption of grape products may affect endogenous melatonin levels (Iriti, 2009; Murch et al., 2010; Meng et al., 2017a). In young, middle-aged and elderly individuals, intake of 200 mL of grape juice twice a day significantly increases urinary 6-sulfatoxymelatonin and total antioxidant capacity (Gonzalez-Flores et al., 2012). However, whether grape juice-induced elevated circulating melatonin is associated with improved disease conditions is still unknown.
Interestingly, despite the presence of melatonin in grape, melatonin found in wine is mainly synthesized by yeast during alcoholic fermentation (Rodriguez-Naranjo et al., 2011). The concentrations of melatonin in human serum significantly increase after drinking alcoholic beer (Maldonado et al., 2009). Conversely, other studies report a decrease in endogenous melatonin production following alcohol consumption (Peuhkuri et al., 2012) thereby making the influence of alcohol found in wine on melatonin levels inconsistent. Nevertheless, as described below, melatonin present in red wine significantly induces cardioprotective effects (Lamont et al., 2015).
Role of Dietary Melatonin in CVDs
Dietary melatonin refers commonly to melatonin content that is present in diet or melatonin-rich food (Iriti and Varoni, 2015; Meng et al., 2017b). Very few studies have investigated the role of dietary melatonin in CVD. These are limited to the exploration of the cardioprotective effect of melatonin in red wine against myocardial I/R injury (Lamont et al., 2011, 2012, 2015; Nduhirabandi et al., 2016) and pulmonary hypertension (Maarman et al., 2015).
Dietary Melatonin and Myocardial I/R Injury
Using both in vivo and in vitro models of myocardial I/R, studies from our laboratory demonstrate that the presence of melatonin in red wine may contributes to the protective effect of red wine against lethal I/R damage (Lamont et al., 2011, 2015). This is supported by the findings that: (1) lowering the alcohol content of red wine does not alter its cardioprotective properties (Lamont et al., 2012); and (2) melatonin, given acutely and directly to the isolated heart at the concentration found in wine (75 ng/L) protects the isolated mouse and rat hearts against I/R injury by reducing myocardial infarct size and improving functional recovery (Lamont et al., 2011). (3) The cardioprotective effect of the consumption chronic and moderate red wine against I/R damage is partially abolished in the presence of prazosin, an inhibitor of melatonin receptor type 3 (M3) (Lamont et al., 2015). Further findings from our laboratory show that drinking water supplemented daily with a moderate amount of red wine or melatonin given at the concentration found in the red wine (75 pg/mL) for 14 days protects the rat and mouse hearts subjected to in vivo or ex vivo I/R by reducing their infarct size (Lamont et al., 2015).
The mechanism of cardioprotection induced with dietary melatonin is complex and still under investigation. As indicated above, melatonin exhibits its physiological functions through its antioxidant, anti-inflammatory, immune-modulatory and vasomotor activities (Pandi-Perumal et al., 2006). Acute (Lamont et al., 2011; Nduhirabandi et al., 2016) and chronic (Lamont et al., 2015) physiological concentrations of melatonin at the concentration found in red wine protect the heart against I/R injury via its membrane receptors related cell survival signaling (Lamont et al., 2015; Nduhirabandi et al., 2016). This is associated with an increased level of activation of myocardial signal transducer and activator of transcription 3 (STAT3) prior to ischemic insult by 79% (Lamont et al., 2011). The importance of STAT3 activation is that it is associated with the activation of SAFE pathway, a well-known powerful cardioprotective signaling pathway (Lamont et al., 2015; Nduhirabandi et al., 2016). Interestingly, luzindole, a specific inhibitor of membrane melatonin receptors 1 and 2 (MT1 and MT 2) (2.3 mg/kg/day, intraperitoneally) does not affect wine treatment, while prazosin, an inhibitor of M3 receptor (2.5 mg/kg/day, intraperitoneally) abolishes wine-induced cardioprotection, therefore suggesting the crucial role of both melatonin and M3 receptor in the cardioprotective effect of red wine (Lamont et al., 2015).
However, how moderate red wine consumption or dietary melatonin affects the SAFE pathway is still unclear. Our recent data suggest that melatonin confers cardioprotection via toll-like receptor 4 (TLR4) which, in turn, activates tumor necrosis factor-α (TNFα)/STAT3 pathway (Nduhirabandi et al., 2016). In view of the pro-inflammatory effects of TLR4 stimulation (Yang et al., 2000), this finding is surprising considering the well-known anti-inflammatory activities of melatonin with its TLR4 suppressing activities (Hu et al., 2013; Mauriz et al., 2013); but it is consistent with the current view of melatonin as an immune system buffer acting as a stimulant under basal or immunosuppressive conditions or as an anti-inflammatory compound in the presence of exacerbated immune responses (for review, see Carrillo-Vico et al., 2013).
Dietary Melatonin and Pulmonary Hypertension
Pulmonary hypertension is associated with an increased oxidative stress and leads to right ventricle (RV) hypertrophy and cardiac fibrosis with the hallmarks of the heart failure (Maarman et al., 2015). A single subcutaneous injection of monocrotaline (80 mg/kg) induces pulmonary hypertension with RV hypertrophy and dysfunction, increase in interstitial fibrosis and plasma oxidative stress (Maarman et al., 2015). In this model, a chronic dietary melatonin treatment (75 ng/L) reduces RV hypertrophy, improves RV function, reduces plasma oxidative stress and reduces cardiac interstitial fibrosis, therefore supporting the beneficial effects of curative and preventive treatments of dietary melatonin in pulmonary hypertension (Maarman et al., 2015). These effects of melatonin are associated with a reduction in oxidative stress and an increase in enzymatic and non-enzymatic antioxidant capacity (Maarman et al., 2015; Torres et al., 2015).
Current Challenges, Limitations and New Perspectives for the Use of Melatonin in CVDs
The dietary supplementation of melatonin appears as an effective option to compensate the physiological decline in the production of pineal gland melatonin with ageing; however, the role of dietary melatonin in health and disease is still not well established (Kennaway, 2017; Meng et al., 2017b). The strong limitation for the studies of dietary melatonin is the lack of standardized methods to determine melatonin concentration in foods with adequate sample treatment to obtain accurate results, thus making some of its proposed benefits hard to swallow (Kennaway, 2017). In addition, most of the studies on dietary melatonin measure melatonin content in food, but very few evaluate the bioavailability of melatonin after melatonin-rich food consumption. It is well established that the bioavailability of melatonin after oral and intravenous melatonin administration in human is very low (approximately 15%) with a maximal half-life of 10–60 min (Harpsoe et al., 2015). Although other factors (such as age, disease conditions, specific drugs, cigarette smoking and caffeine intake) may also influence the bioavailability of melatonin which is mostly metabolized by the enzymatic catabolism in the liver (Ma et al., 2005). As shown in the Figure 4, melatonin is principally metabolized in the liver by cytochrome P-450 isoforms (CYP1A1, CYP1A2, and CYP1B1) in 6-hydroxylation to yield 6-hydroxymelatonin which is thereafter conjugated with sulfates to 6-sulfatoxymelatonin and eliminated in urine (Ma et al., 2005; Pandi-Perumal et al., 2006). Melatonin may also be transformed to a less extend by CYP2C19 and CYP1A2 mediated O-demethylation in N-acetylserotonin (N-acetyl-5-hydroxytryptamine) or by deacetylation to 5-methoxytryptamine which can be reconverted to melatonin (Ma et al., 2005).
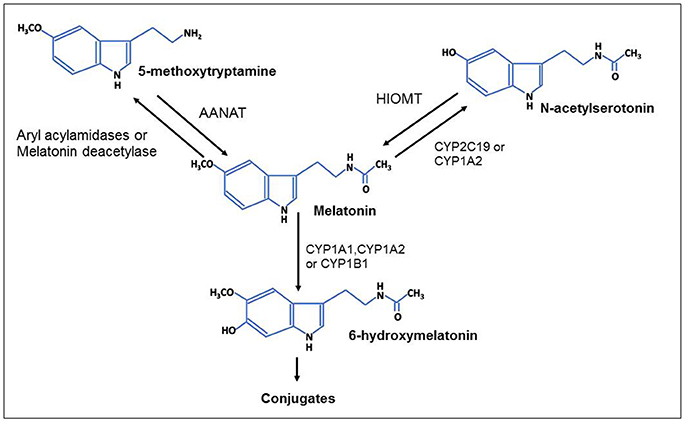
Figure 4. Metabolism of melatonin: enzymatic pathways. Melatonin given orally is principally metabolized in the liver by cytochrome P-450 isoforms (CYP1A1, CYP1A2, and CYP1B1) in 6-hydroxylation to yield 6-hydroxymelatonin which is thereafter conjugated with sulfates to 6-sulfatoxymelatonin and eliminated in urine. Melatonin may also be transformed by deacetylation or by CYP2C19 and CYP1A2 mediated O-demethylation in 5-methoxytryptamine or N-acetylserotonin, respectively. N-acetylserotonin and 5-methoxytryptamine can be converted in melatonin by hydroxyindole-O-methyltransferase (HIOMT) and arylalkylamine N-acetyltransferase (AANAT).
Furthermore, how melatonin-rich food contributes to the overall endogenous melatonin production is complex and still unclear. Besides the melatonin content in the food, food intake may also influence endogenous melatonin concentration, suggesting that the overall beneficial effect could be a result of a combined action of more components found in these food stuffs. Indeed, an increase in blood melatonin levels may be a result of other components found in food that may stimulate endogenous melatonin production such as in the case of ingestion of tryptophan (Huether et al., 1992; Bravo et al., 2013) or serotonin (Esteban et al., 2004). These possible effects make more challenging the delimitation of the contribution of the dietary melatonin to the overall cardiovascular benefits of melatonin-rich foods. Moreover, to avoid bias due to drug interaction, the drugs that are metabolized by cytochrome P-450 such as fluvoxamine, caffeine, and oral contraceptives need to be considered (Harpsoe et al., 2015). Since these drugs compete with melatonin for the same enzyme, they may increase the plasma levels of melatonin after exogenous melatonin administration (Harpsoe et al., 2015).
Due to the very low bioavailability of melatonin after oral or intravenous administration, new developments to optimize the intake of melatonin as supplement or food consider various alternative administrative routes, namely: intranasal, transdermal, subcutaneous, and oral transmucosal (such as sublingual and trans buccal) administration or other forms of preparations (spray, elastic liposomes, gels, pastes) (for review, see Zetner et al., 2016). These alternative routes are very important because they: (1) bypass the liver metabolism, (2) are painless, and (3) provide possible sustained release with a subsequent increase in the bioavailability of melatonin (Zetner et al., 2016).
Although melatonin supplementation is safe in patients with myocardial infarction (Dominguez-Rodriguez et al., 2017b) and nocturnal hypertension (Grossman et al., 2011; Simko et al., 2016), melatonin is contra-indicated for patients with high normal BP to avoid the danger of the diurnal arterial hypertension (Rechcinski et al., 2010). In addition, in children with age of 1-year, increased serum melatonin levels may be associated with severe heart failure (Wu et al., 2017), raising the alarming issue of a high dose of melatonin supplementation in children at this age. While this association is surprising in view of low circulating melatonin levels in adult patients with heart failure, it may be explained by either compensatory mechanisms (of heart failure) or potential detrimental effects of high dose of melatonin in infants (Wu et al., 2017).
Thus far, there is no evidence for the dose-dependent effect of melatonin in the context of cardiovascular diseases. Current data show that both high and low doses of short-term melatonin treatment confer cardiovascular protection in experimental animal models. According to recent clinical trials, although the overall melatonin treatment is safe, it may cause mild adverse effects from transient sedation, daytime sleepiness, mild headache to worsening of dyspnea, and combining melatonin with any drug associated with these effects is contra-indicated (for review, see Andersen et al., 2016). However, further investigation is needed to determine the optimal dose of melatonin and the effects of long-term of melatonin supplementation in humans.
Recent data from clinical studies using melatonin in cardiac diseases show more inconsistencies regarding its cardioprotective effects (Andersen et al., 2016; Dominguez-Rodriguez et al., 2017b; Ekeloef et al., 2017). Apart from dosage issues and mode of administration, previous failures could be partially explained by the use of young and healthy animals with eventual lack of various cardiovascular risk factors, comorbidities and comedications which are characteristics of patients suffering an acute myocardial infarction or undergoing cardiovascular surgery (Heusch, 2017). Considering the current disappointment, further well-planned preclinical and clinical studies are needed to better delineate the cardiovascular benefits of melatonin.
Conclusion
In summary, preclinical studies clearly suggest the cardiovascular health benefit of both endogenous and supplementary melatonin. Melatonin is an important safe molecule with a wide range of physiological functions in animals and humans with a strong therapeutic potential in CVDs. Despite the current difficulties to translate the basic research findings into a clinical setting, cardiovascular protective action of melatonin supplementation is promising but a better understanding of this action is needed. Most importantly, consumption of melatonin-rich foods such as grape juice, wine, cereals, tropical fruits and walnuts increases circulating melatonin levels and antioxidant capacity. Preclinical studies suggest that melatonin, given at dietary levels, confers cardioprotection. However, a better understanding of the mechanisms involved in this effect are required before it can be considered as an adjuvant for effective preventive and curative therapy in CVDs.
Author Contributions
ZJ: wrote the first draft of the manuscript; SL: conception, critical comments on the different drafts of the manuscript (content review); FN: conception, literature choice, figures and tables, revised the final draft manuscript.
Conflict of Interest Statement
The authors declare that the research was conducted in the absence of any commercial or financial relationships that could be construed as a potential conflict of interest.
Acknowledgments
This work was supported by the South African National Research Foundation (NRF), the University of Cape Town and the Wine Industry Network for Expertise and Technology (Winetech) (SL). FN received a National Research Foundation (NRF) Postdoctoral Research Fellowship and European Research Council (ERC)/NRF partnership award.
References
Acuna-Castroviejo, D., Escames, G., Venegas, C., Diaz-Casado, M. E., Lima-Cabello, E., Lopez, L. C., et al. (2014). Extrapineal melatonin: sources, regulation, and potential functions. Cell Mol. Life Sci. 71, 2997–3025. doi: 10.1007/s00018-014-1579-2
Afshin, A., Micha, R., Khatibzadeh, S., and Mozaffarian, D. (2014). Consumption of nuts and legumes and risk of incident ischemic heart disease, stroke, and diabetes: a systematic review and meta-analysis. Am. J. Clin. Nutr. 100, 278–288. doi: 10.3945/ajcn.113.076901
Agabiti-Rosei, C., Favero, G., De Ciuceis, C., Rossini, C., Porteri, E., Rodella, L. F., et al. (2017). Effect of long-term treatment with melatonin on vascular markers of oxidative stress/inflammation and on the anticontractile activity of perivascular fat in aging mice. Hypertens. Res. 40, 41–50. doi: 10.1038/hr.2016.103
Aguilera, Y., Rebollo-Hernanz, M., Herrera, T., Cayuelas, L. T., Rodríguez-Rodríguez, P., de Pablo, Á. L., et al. (2016). Intake of bean sprouts influences melatonin and antioxidant capacity biomarker levels in rats. Food Funct. 7, 1438–1445. doi: 10.1039/C5FO01538C
Al Abdrabalnabi, A., Bitok, E., Rajaram, S., Rizzo, N., and Sabaté, J. (2017). A year-long trial to examine the effects of a daily dose of walnuts on cardiometabolic risk markers in an elderly population. FASEB J. 31:447.1.
Altun, A., Yaprak, M., Aktoz, M., Vardar, A., Betul, U. A., and Ozbay, G. (2002). Impaired nocturnal synthesis of melatonin in patients with cardiac syndrome X. Neurosci. Lett. 327, 143–145. doi: 10.1016/S0304-3940(02)00368-3
An, R., Zhao, L., Xi, C., Li, H., Shen, G., Liu, H., et al. (2016). Melatonin attenuates sepsis-induced cardiac dysfunction via a PI3K/Akt-dependent mechanism. Basic Res. Cardiol. 111:8-015-0526-1. doi: 10.1007/s00395-015-0526-1
Andersen, L. P., Gogenur, I., Rosenberg, J., and Reiter, R. J. (2016). The safety of melatonin in humans. Clin. Drug Investig. 36, 169–175. doi: 10.1007/s40261-015-0368-5
Arendt, J. (1998). Melatonin and the pineal gland: influence on mammalian seasonal and circadian physiology. Rev. Reprod. 3, 13–22. doi: 10.1530/ror.0.0030013
Auld, F., Maschauer, E. L., Morrison, I., Skene, D. J., and Riha, R. L. (2017). Evidence for the efficacy of melatonin in the treatment of primary adult sleep disorders. Sleep Med. Rev. 34, 10–22. doi: 10.1016/j.smrv.2016.06.005
Aune, D., Giovannucci, E., Boffetta, P., Fadnes, L. T., Keum, N., Norat, T., et al. (2017). Fruit and vegetable intake and the risk of cardiovascular disease, total cancer and all-cause mortality-a systematic review and dose-response meta-analysis of prospective studies. Int. J. Epidemiol. 46, 1029–1056. doi: 10.1093/ije/dyw319
Bazzano, L. A., He, J., Ogden, L. G., Loria, C., Vupputuri, S., Myers, L., et al. (2001). Legume consumption and risk of coronary heart disease in US men and women: NHANES I Epidemiologic Follow-up Study. Arch. Intern. Med. 161, 2573–2578. doi: 10.1001/archinte.161.21.2573
Bravo, R., Matito, S., Cubero, J., Paredes, S., Franco, L., Rivero, M., et al. (2013). Tryptophan-enriched cereal intake improves nocturnal sleep, melatonin, serotonin, and total antioxidant capacity levels and mood in elderly humans. Age (Dordr). 35, 1277–1285. doi: 10.1007/s11357-012-9419-5
Brown, G. M., Bar-Or, A., Grossi, D., Kashur, S., Johannson, E., and Yie, S. M. (1991). Urinary 6-sulphatoxymelatonin, an index of pineal function in the rat. J. Pineal Res. 10, 141–147. doi: 10.1111/j.1600-079X.1991.tb00831.x
Brugger, P., Marktl, W., and Herold, M. (1995). Impaired nocturnal secretion of melatonin in coronary heart disease. Lancet 345:1408.
Byeon, Y., Lee, H. Y., Lee, K., Park, S., and Back, K. (2014). Cellular localization and kinetics of the rice melatonin biosynthetic enzymes SNAT and ASMT. J. Pineal Res. 56, 107–114. doi: 10.1111/jpi.12103
Cagnacci, A., Cannoletta, M., Renzi, A., Baldassari, F., Arangino, S., and Volpe, A. (2005). Prolonged melatonin administration decreases nocturnal blood pressure in women. Am. J. Hypertens. 18, 1614–1618. doi: 10.1016/j.amjhyper.2005.05.008
Carracedo, G., Carpena, C., Concepción, P., Díaz, V., García-García, M., Jemni, N., et al. (2017). Presence of melatonin in human tears. J. Optom. 10, 3–4. doi: 10.1016/j.optom.2016.03.002
Carrillo-Vico, A., Lardone, P. J., Alvarez-Sanchez, N., Rodriguez-Rodriguez, A., and Guerrero, J. M. (2013). Melatonin: buffering the immune system. Int. J. Mol. Sci. 14, 8638–8683. doi: 10.3390/ijms14048638
Chua, S., Lee, F. Y., Chiang, H. J., Chen, K. H., Lu, H. I., Chen, Y. T., et al. (2016). The cardioprotective effect of melatonin and exendin-4 treatment in a rat model of cardiorenal syndrome. J. Pineal Res. 61, 438–456. doi: 10.1111/jpi.12357
Dave, R. H., Hale, S. L., and Kloner, R. A. (1998). The effect of melatonin on hemodynamics, blood flow, and myocardial infarct size in a rabbit model of ischemia-reperfusion. J. Cardiovasc. Pharmacol. Ther. 3, 153–160. doi: 10.1177/107424849800300208
de la Puerta, C., Carrascosa-Salmoral, M. P., García-Luna, P. P., Lardone, P. J., Herrera, J. L., Fernández-Montesinos, R., et al. (2007). Melatonin is a phytochemical in olive oil. Food Chem. 104, 609–612. doi: 10.1016/j.foodchem.2006.12.010
Diez, E. R., Renna, N. F., Prado, N. J., Lembo, C., Ponce Zumino, A. Z., Vazquez-Prieto, M., et al. (2013). Melatonin, given at the time of reperfusion, prevents ventricular arrhythmias in isolated hearts from fructose-fed rats and spontaneously hypertensive rats. J. Pineal Res. 55, 166–173. doi: 10.1111/jpi.12059
Dominguez-Rodriguez, A., Abreu-Gonzalez, P., de la Torre-Hernandez, J. M., Consuegra-Sanchez, L., Piccolo, R., Gonzalez-Gonzalez, J., et al. (2017a). Usefulness of early treatment with melatonin to reduce infarct size in patients with ST-segment elevation myocardial infarction receiving percutaneous coronary intervention (from the melatonin adjunct in the acute myocardial infarction treated with angioplasty trial). Am. J. Cardiol. 120, 522–526. doi: 10.1016/j.amjcard.2017.05.018
Dominguez-Rodriguez, A., Abreu-Gonzalez, P., de la Torre-Hernandez, J. M., Gonzalez-Gonzalez, J., Garcia-Camarero, T., Consuegra-Sanchez, L., et al. (2017b). Effect of intravenous and intracoronary melatonin as an adjunct to primary percutaneous coronary intervention for acute ST-elevation myocardial infarction: results of the Melatonin Adjunct in the acute myocaRdial Infarction treated with Angioplasty trial. J. Pineal Res. 62:e12374. doi: 10.1111/jpi.12374
Dominguez-Rodriguez, A., Abreu-Gonzalez, P., Garcia, M. J., Sanchez, J., Marrero, F., and de Armas-Trujillo, D. (2002). Decreased nocturnal melatonin levels during acute myocardial infarction. J. Pineal Res. 33, 248–252. doi: 10.1034/j.1600-079X.2002.02938.x
Dominguez-Rodriguez, A., Abreu-Gonzalez, P., Garcia-Gonzalez, M. J., Kaski, J. C., Reiter, R. J., and Jimenez-Sosa, A. (2007). A unicenter, randomized, double-blind, parallel-group, placebo-controlled study of Melatonin as an Adjunct in patients with acute myocaRdial Infarction undergoing primary Angioplasty The Melatonin Adjunct in the acute myocaRdial Infarction treated with Angioplasty (MARIA) trial: study design and rationale. Contemp. Clin. Trials 28, 532–539. doi: 10.1016/j.cct.2006.10.007
Dominguez-Rodriguez, A., Abreu-Gonzalez, P., Piccolo, R., Galasso, G., and Reiter, R. J. (2016). Melatonin is associated with reverse remodeling after cardiac resynchronization therapy in patients with heart failure and ventricular dyssynchrony. Int. J. Cardiol. 221, 359–363. doi: 10.1016/j.ijcard.2016.07.056
Dominguez-Rodriguez, A., Abreu-Gonzalez, P., and Reiter, R. J. (2014). The potential usefulness of serum melatonin level to predict heart failure in patients with hypertensive cardiomyopathy. Int. J. Cardiol. 174, 415–417. doi: 10.1016/j.ijcard.2014.04.044
Dominguez-Rodriguez, A., Abreu-Gonzalez, P., Sanchez-Sanchez, J. J., Kaski, J. C., and Reiter, R. J. (2010). Melatonin and circadian biology in human cardiovascular disease. J. Pineal Res. 49, 14–22. doi: 10.1111/j.1600-079X.2010.00773.x
Dubbels, R., Reiter, R. J., Klenke, E., Goebel, A., Schnakenberg, E., Ehlers, C., et al. (1995). Melatonin in edible plants identified by radioimmunoassay and by high performance liquid chromatography-mass spectrometry. J. Pineal Res. 18, 28–31. doi: 10.1111/j.1600-079X.1995.tb00136.x
Dwaich, K. H., Al-Amran, F. G., Al-Sheibani, B. I., and Al-Aubaidy, H. A. (2016). Melatonin effects on myocardial ischemia–reperfusion injury: impact on the outcome in patients undergoing coronary artery bypass grafting surgery. Int. J. Cardiol. 221, 977–986. doi: 10.1016/j.ijcard.2016.07.108
Dzida, G., Prystupa, A., Lachowska-Kotowska, P., Kadas, T., Kamienski, P., Kimak, E., et al. (2013). Alteration in diurnal and nocturnal melatonin serum level in patients with chronic heart failure. Ann. Agric. Environ. Med. 20, 745–748.
EFSA (European Food Safety Authority), Panel on Dietetic Products, Nutrition and Allergies (NDA). (2011). Scientific Opinion on the substantiation of a health claim related to melatonin and reduction of sleep onset latency (ID 1698, 1780, 4080) pursuant to Article 13(1) of Regulation (EC) No 1924/2006. EFSA J. 9:2241. doi: 10.2903/j.efsa.2011.2241
Ekeloef, S., Halladin, N., Fonnes, S., Jensen, S. E., Zaremba, T., Rosenberg, J., et al. (2017). Effect of intracoronary and intravenous melatonin on myocardial salvage index in patients with ST-elevation myocardial infarction: a randomized placebo controlled trial. J. Cardiovasc. Transl. Res. 10, 470–479. doi: 10.1007/s12265-017-9768-7
Ekelof, S. V., Halladin, N. L., Jensen, S. E., Zaremba, T., Aaroe, J., Kjaergaard, B., et al. (2016). Effects of intracoronary melatonin on ischemia-reperfusion injury in ST-elevation myocardial infarction. Heart Vessels 31, 88–95. doi: 10.1007/s00380-014-0589-1
Esteban, S., Nicolaus, C., Garmundi, A., Rial, R. V., Rodriguez, A. B., Ortega, E., et al. (2004). Effect of orally administered L-tryptophan on serotonin, melatonin, and the innate immune response in the rat. Mol. Cell. Biochem. 267, 39–46. doi: 10.1023/B:MCBI.0000049363.97713.74
Estruch, R., Ros, E., Salas-Salvado, J., Covas, M. I., Corella, D., and Aros, F. (2013). Primary prevention of cardiovascular disease with a Mediterranean diet. N. Engl. J. Med. 368, 1279–1290. doi: 10.1056/NEJMoa1200303
Favero, G., Franceschetti, L., Buffoli, B., Moghadasian, M. H., Reiter, R. J., Rodella, L. F., et al. (2017). Melatonin: protection against age-related cardiac pathology. Ageing Res. Rev. 35, 336–349. doi: 10.1016/j.arr.2016.11.007
Fischer, T. W., Kleszczynski, K., Hardkop, L. H., Kruse, N., and Zillikens, D. (2013). Melatonin enhances antioxidative enzyme gene expression (CAT, GPx, SOD), prevents their UVR-induced depletion, and protects against the formation of DNA damage (8-hydroxy-2′-deoxyguanosine) in ex vivo human skin. J. Pineal Res. 54, 303–312. doi: 10.1111/jpi.12018
Girotti, L., Lago, M., Ianovsky, O., Elizari, M. V., Dini, A., Perez Lloret, S., et al. (2003). Low urinary 6-sulfatoxymelatonin levels in patients with severe congestive heart failure. Endocrine 22, 245–248. doi: 10.1385/ENDO:22:3:245
Gogenur, I., Kucukakin, B., Panduro Jensen, L., Reiter, R. J., and Rosenberg, J. (2014). Melatonin reduces cardiac morbidity and markers of myocardial ischemia after elective abdominal aortic aneurism repair: a randomized, placebo-controlled, clinical trial. J. Pineal Res. 57, 10–15. doi: 10.1111/jpi.12138
Gonzalez-Flores, D., Gamero, E., Garrido, M., Ramirez, R., Moreno, D., Delgado, J., et al. (2012). Urinary 6-sulfatoxymelatonin and total antioxidant capacity increase after the intake of a grape juice cv. Tempranillo stabilized with HHP. Food Funct. 3, 34–39. doi: 10.1039/C1FO10146C
Green, E. A., Black, B. K., Biaggioni, I., Paranjape, S. Y., Bagai, K., Shibao, C., et al. (2014). Melatonin reduces tachycardia in postural tachycardia syndrome: a randomized, crossover trial. Cardiovasc. Ther. 32, 105–112. doi: 10.1111/1755-5922.12067
Grossman, E., Laudon, M., Yalcin, R., Zengil, H., Peleg, E., Sharabi, Y., et al. (2006). Melatonin reduces night blood pressure in patients with nocturnal hypertension. Am. J. Med. 119, 898–902. doi: 10.1016/j.amjmed.2006.02.002
Grossman, E., Laudon, M., and Zisapel, N. (2011). Effect of melatonin on nocturnal blood pressure: meta-analysis of randomized controlled trials. Vasc. Health. Risk Manag. 7, 577–584. doi: 10.2147/VHRM.S24603
Haghjooy-Javanmard, S., Ziaei, A., Ziaei, S., Ziaei, E., and Mirmohammad-Sadeghi, M. (2013). The effect of preoperative melatonin on nuclear erythroid 2-related factor 2 activation in patients undergoing coronary artery bypass grafting surgery. Oxid Med. Cell. Longev 2013:676829. doi: 10.1155/2013/676829
Halladin, N. L., Ekelof, S., Jensen, S. E., Aaroe, J., Kjaergaard, B., Heegaard, P. M., et al. (2014). Melatonin does not affect oxidative/inflammatory biomarkers in a closed-chest porcine model of acute myocardial infarction. In Vivo 28, 483–488.
Hardeland, R., and Fuhrberg, B. (1996). Ubiquitous melatonin–presence and effects in unicells, plants and animals. Trends Comp. Biochem. Physiol. 2, 25–45.
Hardeland, R., Cardinali, D. P., Srinivasan, V., Spence, D. W., Brown, G. M., and Pandi-Perumal, S. R. (2011). Melatonin–a pleiotropic, orchestrating regulator molecule. Prog. Neurobiol. 93, 350–384. doi: 10.1016/j.pneurobio.2010.12.004
Hardeland, R., and Pandi-Perumal, S. R. (2005). Melatonin, a potent agent in antioxidative defense: actions as a natural food constituent, gastrointestinal factor, drug and prodrug. Nutr. Metab. (Lond) 2:22. doi: 10.1186/1743-7075-2-22
Harpsoe, N. G., Andersen, L. P., Gogenur, I., and Rosenberg, J. (2015). Clinical pharmacokinetics of melatonin: a systematic review. Eur. J. Clin. Pharmacol. 71, 901–909. doi: 10.1007/s00228-015-1873-4
Hattori, A., Migitaka, H., Iigo, M., Itoh, M., Yamamoto, K., Ohtani-Kaneko, R., et al. (1995). Identification of melatonin in plants and its effects on plasma melatonin levels and binding to melatonin receptors in vertebrates. Biochem. Mol. Biol. Int. 35, 627–634.
Hausenloy, D. J., Garcia-Dorado, D., Erik Botker, H., Davidson, S. M., Downey, J., Engel, F. B., et al. (2017). Melatonin as a cardioprotective therapy following ST-segment elevation myocardial infarction: is it really promising? Reply. Cardiovasc. Res. 113, 1418–1419. doi: 10.1093/cvr/cvx137
Herrera, T., Aguilera, Y., Rebollo-Hernanz, M., Bravo, E., Benítez, V., Martínez-Sáez, N., et al. (2018). Teas and herbal infusions as sources of melatonin and other bioactive non-nutrient components. Food Sci. Technol. 89, 65–73. doi: 10.1016/j.lwt.2017.10.031
Herxheimer, A., and Petrie, K. J. (2002). Melatonin for the prevention and treatment of jet lag. Cochrane Database Syst. Rev. 2:CD001520. doi: 10.1002/14651858.CD001520
Heusch, G. (2017). Critical issues for the translation of cardioprotection. Circ. Res. 120, 1477–1486. doi: 10.1161/CIRCRESAHA.117.310820
Hu, J., Zhang, L., Yang, Y., Guo, Y., Fan, Y., Zhang, M., et al. (2017). Melatonin alleviates postinfarction cardiac remodeling and dysfunction by inhibiting Mst1. J. Pineal Res. 62:e12368. doi: 10.1111/jpi.12368
Hu, Z. P., Fang, X. L., Fang, N., Wang, X. B., Qian, H. Y., Cao, Z., et al. (2013). Melatonin ameliorates vascular endothelial dysfunction, inflammation, and atherosclerosis by suppressing the TLR4/NF-kappaB system in high-fat-fed rabbits. J. Pineal Res. 55, 388–398. doi: 10.1111/jpi.12085
Huether, G., Poeggeler, B., Reimer, A., and George, A. (1992). Effect of tryptophan administration on circulating melatonin levels in chicks and rats: evidence for stimulation of melatonin synthesis and release in the gastrointestinal tract. Life Sci. 51, 945–953. doi: 10.1016/0024-3205(92)90402-B
Iigo, M., Furukawa, K., Hattori, A., Hara, M., Ohtani-Kaneko, R., Suzuki, T., et al. (1995). Effects of pinealectomy and constant light exposure on day-night changes of melatonin binding sites in the goldfish brain. Neurosci. Lett. 197, 61–64. doi: 10.1016/0304-3940(95)11903-A
Illnerova, H., Buresova, M., and Presl, J. (1993). Melatonin rhythm in human milk. J. Clin. Endocrinol. Metab. 77, 838–841.
Iriti, M. (2009). Melatonin in grape, not just a myth, maybe a panacea. J. Pineal Res. 46, 353–353. doi: 10.1111/j.1600-079X.2008.00616.x
Iriti, M., and Varoni, E. M. (2015). Melatonin in Mediterranean diet, a new perspective. J. Sci. Food Agric. 95, 2355–2359. doi: 10.1002/jsfa.7051
Iriti, M., Varoni, E. M., and Vitalini, S. (2010). Melatonin in traditional Mediterranean diets. J. Pineal Res. 49, 101–105. doi: 10.1111/j.1600-079X.2010.00777.x
Jin, H., Wang, Y., Zhou, L., Liu, L., Zhang, P., Deng, W., et al. (2014). Melatonin attenuates hypoxic pulmonary hypertension by inhibiting the inflammation and the proliferation of pulmonary arterial smooth muscle cells. J. Pineal Res. 57, 442–450. doi: 10.1111/jpi.12184
Johns, N. P., Johns, J., Porasuphatana, S., Plaimee, P., and Sae-Teaw, M. (2013). Dietary intake of melatonin from tropical fruit altered urinary excretion of 6-sulfatoxymelatonin in healthy volunteers. J. Agric. Food Chem. 61, 913–919. doi: 10.1021/jf300359a
Kantar, S., Turkozkan, N., Bircan, F. S., and Pasaoglu, O. T. (2015). Beneficial effects of melatonin on serum nitric oxide, homocysteine, and ADMA levels in fructose-fed rats. Pharm. Biol. 53, 1035–1041. doi: 10.3109/13880209.2014.957782
Kennaway, D. J. (2017). Are the proposed benefits of melatonin-rich foods too hard to swallow? Crit. Rev. Food Sci. Nutr. 57, 958–962. doi: 10.1080/10408398.2014.962686
Kimak, E., Dzida, G., Duma, D., Prystupa, A., Halabis, M., Kimak, A., et al. (2014). Association between concentration of melatonin, and lipoproteins, LPO, hsCRP, NTproBNP in chronic heart failure patients. Curr. Issues Pharm. Med. Sci. 27, 159–164. doi: 10.1515/cipms-2015-0007
Kozirog, M., Poliwczak, A. R., Duchnowicz, P., Koter-Michalak, M., Sikora, J., and Broncel, M. (2011). Melatonin treatment improves blood pressure, lipid profile, and parameters of oxidative stress in patients with metabolic syndrome. J. Pineal Res. 50, 261–266. doi: 10.1111/j.1600-079X.2010.00835.x
Kris-Etherton, P. M. (2014). Walnuts decrease risk of cardiovascular disease: a summary of efficacy and biologic mechanisms. J. Nutr. 144, 547S–554S. doi: 10.3945/jn.113.182907
Kruger, M. J., Davies, N., Myburgh, K. H., and Lecour, S. (2014). Proanthocyanidins, anthocyanins and cardiovascular diseases. Food. Res. Int. 59, 41–52. doi: 10.1016/j.foodres.2014.01.046
Lagneux, C., Joyeux, M., Demenge, P., Ribuot, C., and Godin-Ribuot, D. (2000). Protective effects of melatonin against ischemia-reperfusion injury in the isolated rat heart. Life Sci. 66, 503–509. doi: 10.1016/S0024-3205(99)00620-7
Lamont, K., Blackhurst, D., Albertyn, Z., Marais, D., and Lecour, S. (2012). Lowering the alcohol content of red wine does not alter its cardioprotective properties. S. Afr. Med. J. 102, 565–567. doi: 10.7196/SAMJ.5733
Lamont, K., Nduhirabandi, F., Adam, T., Thomas, D. P., Opie, L. H., and Lecour, S. (2015). Role of melatonin, melatonin receptors and STAT3 in the cardioprotective effect of chronic and moderate consumption of red wine. Biochem. Biophys. Res. Commun. 465, 719–724. doi: 10.1016/j.bbrc.2015.08.064
Lamont, K. T., Somers, S., Lacerda, L., Opie, L. H., and Lecour, S. (2011). Is red wine a SAFE sip away from cardioprotection? Mechanisms involved in resveratrol- and melatonin-induced cardioprotection. J. Pineal Res. 50, 374–380. doi: 10.1111/j.1600-079X.2010.00853.x
Lecour, S., and Lamont, K. T. (2011). Natural polyphenols and cardioprotection. Mini Rev. Med. Chem. 11, 1191–1199. doi: 10.2174/13895575111091191
Lerner, A. B., Case, J. D., Takahashi, Y., Lee, T. H., and Mori, W. (1958). Isolation of melatonin, the pineal gland factor that lightens melanocyteS1. J. Am. Chem. Soc. 80, 2587–2587. doi: 10.1021/ja01543a060
Liu, X., Chen, Z., Chua, C. C., Ma, Y. S., Youngberg, G. A., Hamdy, R., et al. (2002). Melatonin as an effective protector against doxorubicin-induced cardiotoxicity. Am. J. Physiol. Heart Circ. Physiol. 283, H254–H263. doi: 10.1152/ajpheart.01023.2001
Lochner, A., Genade, S., Davids, A., Ytrehus, K., and Moolman, J. A. (2006). Short- and long-term effects of melatonin on myocardial post-ischemic recovery. J. Pineal Res. 40, 56–63. doi: 10.1111/j.1600-079X.2005.00280.x
Lochner, A., Huisamen, B., and Nduhirabandi, F. (2013). Cardioprotective effect of melatonin against ischaemia/reperfusion damage. Front. Biosci. (Elite Ed) 5, 305–315. doi: 10.2741/E617
Ma, X., Idle, J. R., Krausz, K. W., and Gonzalez, F. J. (2005). Metabolism of melatonin by human cytochromes p450. Drug Metab. Dispos. 33, 489–494. doi: 10.1124/dmd.104.002410
Maarman, G., Blackhurst, D., Thienemann, F., Blauwet, L., Butrous, G., Davies, N., et al. (2015). Melatonin as a preventive and curative therapy against pulmonary hypertension. J. Pineal Res. 59, 343–353. doi: 10.1111/jpi.12263
Májovský, M., Rezáčová, L., Sumová, A., Pospíšilová, L., Netuka, D., Bradáč, O., et al. (2017). Melatonin and cortisol secretion profile in patients with pineal cyst before and after pineal cyst resection. J. Clin. Neurosci. 39, 155–163. doi: 10.1016/j.jocn.2017.01.022
Maldonado, M. D., Moreno, H., and Calvo, J. R. (2009). Melatonin present in beer contributes to increase the levels of melatonin and antioxidant capacity of the human serum. Clin. Nutr. 28, 188–191. doi: 10.1016/j.clnu.2009.02.001
Mantele, S., Otway, D. T., Middleton, B., Bretschneider, S., Wright, J., Robertson, M. D., et al. (2012). Daily rhythms of plasma melatonin, but not plasma leptin or leptin mRNA, vary between lean, obese and type 2 diabetic men. PLoS ONE 7:e37123. doi: 10.1371/journal.pone.0037123
Maria, S., and Witt-Enderby, P. A. (2014). Melatonin effects on bone: potential use for the prevention and treatment for osteopenia, osteoporosis, and periodontal disease and for use in bone-grafting procedures. J. Pineal Res. 56, 115–125. doi: 10.1111/jpi.12116
Mauriz, J. L., Collado, P. S., Veneroso, C., Reiter, R. J., and Gonzalez-Gallego, J. (2013). A review of the molecular aspects of melatonin's anti-inflammatory actions: recent insights and new perspectives. J. Pineal Res. 54, 1–14. doi: 10.1111/j.1600-079X.2012.01014.x
McCord, C. P., and Allen, F. P. (1917). Evidences associating pineal gland function with alterations in pigmentation. J. Exp. Zool. 23, 207–224. doi: 10.1002/jez.1400230108
McElhinney, D. B., Hoffman, S. J., Robinson, W. A., and Ferguson, J. (1994). Effect of melatonin on human skin color. J. Invest. Dermatol. 102, 258–259. doi: 10.1111/1523-1747.ep12371773
McMullan, C. J., Rimm, E. B., Schernhammer, E. S., and Forman, J. P. (2017). A nested case-control study of the association between melatonin secretion and incident myocardial infarction. Heart 103, 694–701. doi: 10.1136/heartjnl-2016-310098
McMullan, C. J., Schernhammer, E. S., Rimm, E. B., Hu, F. B., and Forman, J. P. (2013). Melatonin secretion and the incidence of type 2 diabetes. JAMA 309, 1388–1396. doi: 10.1001/jama.2013.2710
Meng, J., Shi, T., Song, S., Zhang, Z., and Fang, Y. (2017a). Melatonin in grapes and grape-related foodstuffs: a review. Food Chem. 231, 185–191. doi: 10.1016/j.foodchem.2017.03.137
Meng, X., Li, Y., Li, S., Zhou, Y., Gan, R. Y., Xu, D. P., et al. (2017b). Dietary sources and bioactivities of melatonin. Nutrients 9:367. doi: 10.3390/nu9040367
Micha, R., Penalvo, J. L., Cudhea, F., Imamura, F., Rehm, C. D., and Mozaffarian, D. (2017). Association between dietary factors and mortality from heart disease, stroke, and type 2 diabetes in the United States. JAMA 317, 912–924. doi: 10.1001/jama.2017.0947
Mozdzan, M., Mozdzan, M., Chalubinski, M., Wojdan, K., and Broncel, M. (2014). The effect of melatonin on circadian blood pressure in patients with type 2 diabetes and essential hypertension. Arch. Med. Sci. 10, 669–675. doi: 10.5114/aoms.2014.44858
Muller, J. E., Ludmer, P. L., Willich, S. N., Tofler, G. H., Aylmer, G., Klangos, I., et al. (1987). Circadian variation in the frequency of sudden cardiac death. Circulation 75, 131–138. doi: 10.1161/01.CIR.75.1.131
Murch, S. J., Hall, B. A., Le, C. H., and Saxena, P. K. (2010). Changes in the levels of indoleamine phytochemicals during véraison and ripening of wine grapes. J. Pineal Res. 49, 95–100. doi: 10.1111/j.1600-079X.2010.00774.x
Navarro-Alarcon, M., Ruiz-Ojeda, F. J., Blanca-Herrera, R. M., Serrano, M. M., Acuna-Castroviejo, D., Fernandez-Vazquez, G., et al. (2014). Melatonin and metabolic regulation: a review. Food Funct. 5, 2806–2832. doi: 10.1039/C4FO00317A
Nduhirabandi, F., Du Toit, E. F., Blackhurst, D., Marais, D., and Lochner, A. (2011). Chronic melatonin consumption prevents obesity-related metabolic abnormalities and protects the heart against myocardial ischemia and reperfusion injury in a prediabetic model of diet-induced obesity. J. Pineal Res. 50, 171–182. doi: 10.1111/j.1600-079X.2010.00826.x
Nduhirabandi, F., du Toit, E. F., and Lochner, A. (2012). Melatonin and the metabolic syndrome: a tool for effective therapy in obesity-associated abnormalities? Acta Physiol. (Oxf) 205, 209–223. doi: 10.1111/j.1748-1716.2012.02410.x
Nduhirabandi, F., Huisamen, B., Strijdom, H., Blackhurst, D., and Lochner, A. (2014). Short-term melatonin consumption protects the heart of obese rats independent of body weight change and visceral adiposity. J. Pineal Res. 57, 317–332. doi: 10.1111/jpi.12171
Nduhirabandi, F., Lamont, K., Albertyn, Z., Opie, L. H., and Lecour, S. (2016). Role of toll-like receptor 4 in melatonin-induced cardioprotection. J. Pineal Res. 60, 39–47. doi: 10.1111/jpi.12286
Opie, L. H., and Lecour, S. (2016). Melatonin has multiorgan effects. Eur. Heart J. Cardiovasc. Pharmacother. 2, 258–265. doi: 10.1093/ehjcvp/pvv037
Opie, L. H., and Lecour, S. (2007). The red wine hypothesis: from concepts to protective signalling molecules. Eur. Heart J. 28, 1683–1693. doi: 10.1093/eurheartj/ehm149
Pandi-Perumal, S., BaHammam, A. S., Ojike, N. I., Akinseye, O. A., Kendzerska, T., Buttoo, K., et al. (2017). Melatonin and human cardiovascular disease. J. Cardiovasc. Pharmacol. Ther. 22, 122–132. doi: 10.1177/1074248416660622
Pandi-Perumal, S. R., Srinivasan, V., Maestroni, G. J., Cardinali, D. P., Poeggeler, B., and Hardeland, R. (2006). Melatonin: nature's most versatile biological signal? FEBS J. 273, 2813–2838. doi: 10.1111/j.1742-4658.2006.05322.x
Park, S., Byeon, Y., and Back, K. (2013). Transcriptional suppression of tryptamine 5-hydroxylase, a terminal serotonin biosynthetic gene, induces melatonin biosynthesis in rice (Oryza sativa L.). J. Pineal Res. 55, 131–137. doi: 10.1111/jpi.12053
Patel, V., Upaganlawar, A., Zalawadia, R., and Balaraman, R. (2010). Cardioprotective effect of melatonin against isoproterenol induced myocardial infarction in rats: a biochemical, electrocardiographic and histoarchitectural evaluation. Eur. J. Pharmacol. 644, 160–168. doi: 10.1016/j.ejphar.2010.06.065
Paulis, L., Pechanova, O., Zicha, J., Barta, A., Gardlik, R., Celec, P., et al. (2010). Melatonin interactions with blood pressure and vascular function during L-NAME-induced hypertension. J. Pineal Res. 48, 102–108. doi: 10.1111/j.1600-079X.2009.00732.x
Paulis, L., and Simko, F. (2007). Blood pressure modulation and cardiovascular protection by melatonin: potential mechanisms behind. Physiol. Res. 56, 671–684.
Pei, H., Du, J., Song, X., He, L., Zhang, Y., Li, X., et al. (2016). Melatonin prevents adverse myocardial infarction remodeling via Notch1/Mfn2 pathway. Free Radic. Biol. Med. 97:e1237. 408–417. doi: 10.1016/j.freeradbiomed.2016.06.015
Pei, H. F., Hou, J. N., Wei, F. P., Xue, Q., Zhang, F., Peng, C. F., et al. (2017). Melatonin attenuates postmyocardial infarction injury via increasing Tom70 expression. J. Pineal Res. 62:e12371. doi: 10.1111/jpi.12371
Petrosillo, G., Colantuono, G., Moro, N., Ruggiero, F. M., Tiravanti, E., Di Venosa, N., et al. (2009). Melatonin protects against heart ischemia-reperfusion injury by inhibiting mitochondrial permeability transition pore opening. Am. J. Physiol. Heart Circ. Physiol. 297, H1487–H1493. doi: 10.1152/ajpheart.00163.2009
Peuhkuri, K., Sihvola, N., and Korpela, R. (2012). Dietary factors and fluctuating levels of melatonin. Food Nutr. Res. 56:17252. doi: 10.3402/fnr.v56i0.17252
Rechcinski, T., Trzos, E., Wierzbowska-Drabik, K., Krzeminska-Pakula, M., and Kurpesa, M. (2010). Melatonin for nondippers with coronary artery disease: assessment of blood pressure profile and heart rate variability. Hypertens. Res. 33, 56–61. doi: 10.1038/hr.2009.174
Reiter, R. J., Manchester, L. C., and Tan, D. X. (2005). Melatonin in walnuts: influence on levels of melatonin and total antioxidant capacity of blood. Nutrition 21, 920–924. doi: 10.1016/j.nut.2005.02.005
Reiter, R. J., Mayo, J. C., Tan, D. X., Sainz, R. M., Alatorre-Jimenez, M., and Qin, L. (2016). Melatonin as an antioxidant: under promises but over delivers. J. Pineal Res. 61, 253–278. doi: 10.1111/jpi.12360
Reiter, R. J., Rosales-Corral, S. A., Tan, D. X., Acuna-Castroviejo, D., Qin, L., Yang, S. F., et al. (2017). Melatonin, a full service anti-cancer agent: inhibition of initiation, progression and metastasis. Int. J. Mol. Sci. 18:843. doi: 10.3390/ijms18040843
Reiter, R. J., Tan, D. X., Burkhardt, S., and Manchester, L. C. (2001). Melatonin in plants. Nutr. Rev. 59, 286–290. doi: 10.1111/j.1753-4887.2001.tb07018.x
Reiter, R. J., Tan, D. X., Manchester, L. C., Paredes, S. D., Mayo, J. C., and Sainz, R. M. (2009). Melatonin and reproduction revisited. Biol. Reprod. 81, 445–456. doi: 10.1095/biolreprod.108.075655
Reiter, R. J., Tan, D. X., Paredes, S. D., and Fuentes-Broto, L. (2010). Beneficial effects of melatonin in cardiovascular disease. Ann. Med. 42, 276–285. doi: 10.3109/07853890903485748
Reppert, S. M., and Weaver, D. R. (1995). Melatonin madness. Cell 83, 1059–1062. doi: 10.1016/0092-8674(95)90131-0
Rodriguez-Naranjo, M. I., Gil-Izquierdo, A., Troncoso, A. M., Cantos-Villar, E., and Garcia-Parrilla, M. C. (2011). Melatonin is synthesised by yeast during alcoholic fermentation in wines. Food Chem. 126, 1608–1613. doi: 10.1016/j.foodchem.2010.12.038
Sae-Teaw, M., Johns, J., Johns, N. P., and Subongkot, S. (2013). Serum melatonin levels and antioxidant capacities after consumption of pineapple, orange, or banana by healthy male volunteers. J. Pineal Res. 55, 58–64. doi: 10.1111/jpi.12025
Sahna, E., Olmez, E., and Acet, A. (2002). Effects of physiological and pharmacological concentrations of melatonin on ischemia-reperfusion arrhythmias in rats: can the incidence of sudden cardiac death be reduced? J. Pineal Res. 32, 194–198. doi: 10.1034/j.1600-079x.2002.1o853.x
Salmanoglu, D. S., Gurpinar, T., Vural, K., Ekerbicer, N., Dariverenli, E., and Var, A. (2016). Melatonin and L-carnitin improves endothelial disfunction and oxidative stress in Type 2 diabetic rats. Redox Biol. 8, 199–204. doi: 10.1016/j.redox.2015.11.007
Sanchez-Hidalgo, M., Guerrero Montavez, J. M., Carrascosa-Salmoral Mdel, P., Naranjo Gutierrez Mdel, C., Lardone, P. J., and de la Lastra Romero, C. A. (2009). Decreased MT1 and MT2 melatonin receptor expression in extrapineal tissues of the rat during physiological aging. J. Pineal Res. 46, 29–35. doi: 10.1111/j.1600-079X.2008.00604.x
Sartori, C., Dessen, P., Mathieu, C., Monney, A., Bloch, J., Nicod, P., et al. (2009). Melatonin improves glucose homeostasis and endothelial vascular function in high-fat diet-fed insulin-resistant mice. Endocrinology 150, 5311–5317. doi: 10.1210/en.2009-0425
Scheer, F. A., Van Montfrans, G. A., van Someren, E. J., Mairuhu, G., and Buijs, R. M. (2004). Daily nighttime melatonin reduces blood pressure in male patients with essential hypertension. Hypertension 43, 192–197. doi: 10.1161/01.HYP.0000113293.15186.3b
Scholtens, R. M., van Munster, B. C., van Kempen, M. F., and de Rooij, S. E. (2016). Physiological melatonin levels in healthy older people: a systematic review. J. Psychosom. Res. 86, 20–27. doi: 10.1016/j.jpsychores.2016.05.005
Sehirli, A. O., Koyun, D., Tetik, S., Ozsavci, D., Yiginer, O., Cetinel, S., et al. (2013). Melatonin protects against ischemic heart failure in rats. J. Pineal Res. 55, 138–148. doi: 10.1111/jpi.12054
Siegel, D., Black, D. M., Seeley, D. G., and Hulley, S. B. (1992). Circadian variation in ventricular arrhythmias in hypertensive men. Am. J. Cardiol. 69, 344–347. doi: 10.1016/0002-9149(92)90231-M
Simko, F., Baka, T., Paulis, L., and Reiter, R. J. (2016). Elevated heart rate and nondipping heart rate as potential targets for melatonin: a review. J. Pineal Res. 61, 127–137. doi: 10.1111/jpi.12348
Simko, F., Bednarova, K. R., Krajcirovicova, K., Hrenak, J., Celec, P., Kamodyova, N., et al. (2014a). Melatonin reduces cardiac remodeling and improves survival in rats with isoproterenol-induced heart failure. J. Pineal Res. 57, 177–184. doi: 10.1111/jpi.12154
Simko, F., Pechanova, O., Repova Bednarova, K., Krajcirovicova, K., Celec, P., Kamodyova, N., et al. (2014b). Hypertension and cardiovascular remodelling in rats exposed to continuous light: protection by ACE-inhibition and melatonin. Mediators Inflamm. 2014:703175. doi: 10.1155/2014/703175
Skiadas, P. K., and Lascaratos, J. G. (2001). Dietetics in ancient Greek philosophy: plato's concepts of healthy diet. Eur. J. Clin. Nutr. 55, 532–537. doi: 10.1038/sj.ejcn.1601179
Stefulj, J., Hortner, M., Ghosh, M., Schauenstein, K., Rinner, I., Wolfler, A., et al. (2001). Gene expression of the key enzymes of melatonin synthesis in extrapineal tissues of the rat. J. Pineal Res. 30, 243–247. doi: 10.1034/j.1600-079X.2001.300408.x
Stürtz, M., Cerezo, A. B., Cantos-Villar, E., and Garcia-Parrilla, M. C. (2011). Determination of the melatonin content of different varieties of tomatoes (Lycopersicon esculentum) and strawberries (Fragaria ananassa). Food Chem. 127, 1329–1334. doi: 10.1016/j.foodchem.2011.01.093
Sun, H., Gusdon, A. M., and Qu, S. (2016). Effects of melatonin on cardiovascular diseases: progress in the past year. Curr. Opin. Lipidol. 27, 408–413. doi: 10.1097/MOL.0000000000000314
Tain, Y. L., Huang, L. T., Lin, I. C., Lau, Y. T., and Lin, C. Y. (2010). Melatonin prevents hypertension and increased asymmetric dimethylarginine in young spontaneous hypertensive rats. J. Pineal Res. 49, 390–398. doi: 10.1111/j.1600-079X.2010.00806.x
Tan, D. X., Chen, L. D., Poeggeler, B., Manchester, L. C., and Reiter, R. J. (1993). Melatonin: a potent, endogenous hydroxyl radical scavenger. Endocr. J. 1, 57–60.
Tan, D., Manchester, L. C., Reiter, R. J., Qi, W., Kim, S. J., and El-Sokkary, G. H. (1998). Ischemia/reperfusion-induced arrhythmias in the isolated rat heart: prevention by melatonin. J. Pineal Res. 25, 184–191. doi: 10.1111/j.1600-079X.1998.tb00558.x
Tan, D. X., Hardeland, R., Back, K., Manchester, L. C., Alatorre-Jimenez, M. A., and Reiter, R. J. (2016). On the significance of an alternate pathway of melatonin synthesis via 5-methoxytryptamine: comparisons across species. J. Pineal Res. 61, 27–40. doi: 10.1111/jpi.12336
Tan, D. X., Hardeland, R., Manchester, L. C., Korkmaz, A., Ma, S., Rosales-Corral, S., et al. (2012). Functional roles of melatonin in plants, and perspectives in nutritional and agricultural science. J. Exp. Bot. 63, 577–597. doi: 10.1093/jxb/err256
Tan, D. X., Zanghi, B. M., Manchester, L. C., and Reiter, R. J. (2014). Melatonin identified in meats and other food stuffs: potentially nutritional impact. J. Pineal Res. 57, 213–218. doi: 10.1111/jpi.12152
Torres, F., Gonzalez-Candia, A., Montt, C., Ebensperger, G., Chubretovic, M., Seron-Ferre, M., et al. (2015). Melatonin reduces oxidative stress and improves vascular function in pulmonary hypertensive newborn sheep. J. Pineal Res. 58, 362–373. doi: 10.1111/jpi.12222
Trotti, L. M., and Karroum, E. G. (2016). Melatonin for sleep disorders in patients with neurodegenerative diseases. Curr. Neurol. Neurosci. Rep. 16:63. doi: 10.1007/s11910-016-0664-3
Vazan, R., and Ravingerova, T. (2015). Protective effect of melatonin against myocardial injury induced by epinephrine. J. Physiol. Biochem. 71, 43–49. doi: 10.1007/s13105-014-0377-5
Venegas, C., Garcia, J. A., Escames, G., Ortiz, F., Lopez, A., Doerrier, C., et al. (2012). Extrapineal melatonin: analysis of its subcellular distribution and daily fluctuations. J. Pineal Res. 52, 217–227. doi: 10.1111/j.1600-079X.2011.00931.x
Wang, Z., Ni, L., Wang, J., Lu, C., Ren, M., Han, W., et al. (2016). The protective effect of melatonin on smoke-induced vascular injury in rats and humans: a randomized controlled trial. J. Pineal Res. 60, 217–227. doi: 10.1111/jpi.12305
Wu, Y., Si, F., Luo, L., and Yi, Q. (2017). Serum levels of melatonin may contribute to the pathogenesis of heart failure in children with median age of 1 year. J. Pediatr. doi: 10.1016/j.jped.2017.06.023. [Epub ahead of print].
Yang, H., Young, D. W., Gusovsky, F., and Chow, J. C. (2000). Cellular events mediated by lipopolysaccharide-stimulated toll-like receptor 4. MD-2 is required for activation of mitogen-activated protein kinases and Elk-1. J. Biol. Chem. 275, 20861–20866. doi: 10.1074/jbc.M002896200
Yang, Y., Sun, Y., Yi, W., Li, Y., Fan, C., Xin, Z., et al. (2014). A review of melatonin as a suitable antioxidant against myocardial ischemia-reperfusion injury and clinical heart diseases. J. Pineal Res. 57, 357–366. doi: 10.1111/jpi.12175
Yeung, H. M., Hung, M. W., Lau, C. F., and Fung, M. L. (2015). Cardioprotective effects of melatonin against myocardial injuries induced by chronic intermittent hypoxia in rats. J. Pineal Res. 58, 12–25. doi: 10.1111/jpi.12190
Yu, L., Fan, C., Li, Z., Zhang, J., Xue, X., Xu, Y., et al. (2017a). Melatonin rescues cardiac thioredoxin system during ischemia-reperfusion injury in acute hyperglycemic state by restoring Notch1/Hes1/Akt signaling in a membrane receptor-dependent manner. J. Pineal Res. 62:e12375. doi: 10.1111/jpi.12375
Yu, L., Gong, B., Duan, W., Fan, C., Zhang, J., Li, Z., et al. (2017b). Melatonin ameliorates myocardial ischemia/reperfusion injury in type 1 diabetic rats by preserving mitochondrial function: role of AMPK-PGC-1alpha-SIRT3 signaling. Sci. Rep. 7:41337. doi: 10.1038/srep41337
Zetner, D., Andersen, L. P., and Rosenberg, J. (2016). Pharmacokinetics of alternative administration routes of melatonin: a systematic review. Drug Res. (Stuttg) 66, 169–173. doi: 10.1055/s-0035-1565083
Zhai, M., Li, B., Duan, W., Jing, L., Zhang, B., Zhang, M., et al. (2017a). Melatonin ameliorates myocardial ischemia reperfusion injury through SIRT3-dependent regulation of oxidative stress and apoptosis. J. Pineal Res. 63:e12419. doi: 10.1111/jpi.12419
Zhai, M., Liu, Z., Zhang, B., Jing, L., Li, B., Li, K., et al. (2017b). Melatonin protects against the pathological cardiac hypertrophy induced by transverse aortic constriction through activating PGC-1beta: in vivo and in vitro studies. J. Pineal Res. 63:3. doi: 10.1111/jpi.12433
Keywords: antioxidant, cardiovascular diseases, hypertension, melatonin, myocardial infarction
Citation: Jiki Z, Lecour S and Nduhirabandi F (2018) Cardiovascular Benefits of Dietary Melatonin: A Myth or a Reality? Front. Physiol. 9:528. doi: 10.3389/fphys.2018.00528
Received: 29 January 2018; Accepted: 24 April 2018;
Published: 17 May 2018.
Edited by:
Pasquale Pagliaro, Università degli Studi di Torino, ItalyReviewed by:
Vincenzo Lionetti, Scuola Sant'Anna di Studi Avanzati, ItalyAntonio Colantuoni, University of Naples Federico II, Italy
Copyright © 2018 Jiki, Lecour and Nduhirabandi. This is an open-access article distributed under the terms of the Creative Commons Attribution License (CC BY). The use, distribution or reproduction in other forums is permitted, provided the original author(s) and the copyright owner are credited and that the original publication in this journal is cited, in accordance with accepted academic practice. No use, distribution or reproduction is permitted which does not comply with these terms.
*Correspondence: Frederic Nduhirabandi, ZnJlZGVyaWMubmR1aGlyYWJhbmRpQHVjdC5hYy56YQ==