- 1Department of Sport, Exercise and Health, Faculty of Medicine, University of Basel, Basel, Switzerland
- 2Department Medicine, Training and Health, Philipps-University Marburg, Marburg, Germany
- 3Centre for Chronobiology, Psychiatric Hospital of the University of Basel, Basel, Switzerland
- 4Transfaculty Research Platform Molecular and Cognitive Neurosciences, University of Basel, Basel, Switzerland
In competitive sports any substantial individual differences in diurnal variations in maximal performance are highly relevant. Previous studies have exclusively focused on how the time of day affects performance and disregarded the maximal individual diurnal variation of performance. Thus, the aims of this study were (1) to investigate the maximum diurnal variation in maximum oxygen uptake (VO2max), (2) to compare the diurnal variation of VO2max during the day to the day-to-day variation in VO2max, and (3) to investigate if there is a time-of-day effect on VO2max. Ten male and seven female athletes (mean VO2max: 58.2 ± 6.9 ml/kg/min) performed six maximal cardiopulmonary exercise tests including a verification-phase at six different times of the day (i.e., diurnal variation) and a seventh test at the same time the sixth test took place (i.e., day-to-day variation). The test times were 7:00, 10:00, 13:00, 16:00, 19:00, and 21:00. The order of exercise tests was the same for all participants to ensure sufficient recovery but the time of day of the first exercise test was randomized. We used paired t-tests to compare the nadir and peak of diurnal variations, day-to-day variations and the difference between diurnal and day-to-day variations. The mean difference in VO2max was 5.0 ± 1.9 ml/kg/min (95% CI: 4.1, 6.0) for the diurnal variation and 2.0 ± 1.0 ml/kg/min (95% CI: 1.5, 2.5) for the day-to-day variation. The diurnal variation was significantly higher than the day-to-day variation with a mean difference of 3.0 ± 2.1 ml/kg/min (95% CI: 1.9, 4.1). The linear mixed effects model revealed no significant differences in VO2max for any pairwise comparison between the different times of the day (all p > 0.11). This absence of a time-of-day effect is explained by the fact that peak VO2max was achieved at different times of the day by different athletes. The diurnal variations have meaningful implications for competitive sports and need to be considered by athletes. However, the results are also relevant to research. To increase signal-to-noise-ratio in intervention studies it is necessary to conduct cardiopulmonary exercise testing at the same time of the day for pre- and post-intervention exercise tests.
Introduction
In competitive sports any substantial individual differences in diurnal variations in maximal performance are highly relevant. As shown by several reviews (Chtourou and Souissi, 2012), the diurnal variation of short-duration performance has been examined considerably more extensively than that of long-duration endurance performance. Our systematic literature search (see Supplemental Methods) in Pubmed and Web of Science revealed 15 studies (Reilly and Baxter, 1983; Hill et al., 1988; Burgoon et al., 1992; Dalton et al., 1997; Deschenes et al., 1998; Reilly and Garrett, 1998; Atkinson et al., 2005; Brown et al., 2008; Chtourou et al., 2012; Souissi et al., 2012; Hill, 2014; Chin et al., 2015; Facer-Childs and Brandstaetter, 2015; Rae et al., 2015; Aloui et al., 2017), that have examined the effect of the time of day on endurance performance with inconclusive results (Table 1). In detail, five studies showed significant differences (Atkinson et al., 2005; Chtourou et al., 2012; Hill, 2014; Facer-Childs and Brandstaetter, 2015; Aloui et al., 2017), three studies showed differences in subgroups (i.e., different chronotypes) (Hill et al., 1988; Brown et al., 2008; Chin et al., 2015), and seven studies showed no significant differences (Reilly and Baxter, 1983; Burgoon et al., 1992; Dalton et al., 1997; Deschenes et al., 1998; Reilly and Garrett, 1998; Souissi et al., 2012; Rae et al., 2015) in maximum performance at different times of the day.
However, the main issue is that most studies measured performance only at two times of the day (Reilly and Baxter, 1983; Hill et al., 1988; Burgoon et al., 1992; Reilly and Garrett, 1998; Atkinson et al., 2005; Brown et al., 2008; Chtourou et al., 2012; Souissi et al., 2012; Hill, 2014; Rae et al., 2015; Aloui et al., 2017) with 07:00 and 17:00 being the most frequently used times. Such a large measurement interval carries a high risk of missing the peak and nadir of performance during the day. Consequently, these studies are unable to describe the full extent of diurnal variation in performance. Furthermore, all studies show several methodological shortcomings, including missing sample size calculations in all studies, sample sizes ≤ 12 in half of the studies (Reilly and Baxter, 1983; Dalton et al., 1997; Deschenes et al., 1998; Reilly and Garrett, 1998; Atkinson et al., 2005; Souissi et al., 2012; Aloui et al., 2017), not reporting the absolute data for the primary outcome (Brown et al., 2008; Souissi et al., 2012; Chin et al., 2015; Facer-Childs and Brandstaetter, 2015), and no information regarding the sequence of the investigated times of day or regarding a performed randomization. Additionally, some studies tested untrained or moderately trained participants (Hill et al., 1988; Burgoon et al., 1992; Deschenes et al., 1998), making the results not generalizable for athletes. Moreover, the majority of studies used more time efficient but also less precise methods such as shuttle-run tests. The measurement of maximum oxygen uptake (VO2max) in a cardiopulmonary exercise test (CPET), which is supposed to represent the gold standard to determine aerobic performance, was only measured in studies with a small sample size (Deschenes et al., 1998) or with large measurement intervals (Hill et al., 1988; Burgoon et al., 1992; Hill, 2014). Previous studies also do not consider exhaustion criteria, leaving room for speculation whether lower performance at a certain time of day is due to physiological (i.e., no higher performance possible) or psychophysiological (i.e., not motivated to perform with maximum effort) reasons.
In addition to the study design, data analysis in previous studies is debatable as these studies solely investigated the effects of daytime on maximum performance by comparing performances achieved at different times of the day on a group level. Because several factors such as habitual training time (Torii et al., 1992; Rae et al., 2015) or chronotype (Hill et al., 1988; Brown et al., 2008; Facer-Childs and Brandstaetter, 2015) seem to influence the time of day when peak performance is achieved, we expected it to be unlikely that all participants reach their peak and nadir of performance at the same time of day. If an athlete performs better in the morning and another athlete performs better in the afternoon, the differences would be canceled out on a group level leading to the false conclusion that there are no diurnal variations in maximum performance. Therefore, we additionally compared each participant's VO2max at the peak and nadir of the day to describe the diurnal variations in VO2max. Furthermore, we aimed to determine the day-to-day variation in athletes' VO2max to be able to put the possible diurnal variation into context.
The aims of this study, therefore, were to (1) investigate the maximum diurnal variation in VO2max, (2) to compare the diurnal variation of VO2max during the day to the day-to-day variation in VO2max, and (3) to investigate if there is a time of peak VO2max on a group level. A further aim was (4) to investigate to which extent chronotype and habitual training time contribute to the diurnal variation of VO2max during the day.
To address methodological shortcomings from previous studies, we used a measurement interval of 3 h (i.e., six times of the day) in our study, tested an adequate number of trained athletes in a randomized sequence using the gold standard for cardiopulmonary exercise testing, and considered exhaustion criteria.
MaterialS and Methods
Study Design
This study was conducted between December 2016 and May 2018 in the laboratory of the Department of Sport, Exercise and Health of the University of Basel, Switzerland. Participants gave written informed consent before inclusion, and the study was approved by the local ethics committee “Ethikkommission Nordwest- und Zentralschweiz” (EKNZ 2016-01572). To investigate the diurnal variation, participants performed CPET at 7:00, 10:00, 13:00, 16:00, 19:00, and 21:00. The order of CPET was the same for all participants to ensure sufficient recovery of at least 26 h. However, the time of day of the first CPET was randomized. To investigate the day-to-day variation, we performed a seventh CPET at the same time of day as the sixth CPET. 21:00 instead of 22:00 was chosen because this time represented the dimmed light melatonin onset in a study with athletes of comparable age (Knaier et al., 2017).
Participants
Inclusion criteria were physical health, age between 18 and 40 years, no shift-work in the last 3 months, and no travel across time zones in the 4 weeks prior to the study. To avoid training effects resulting from performing multiple CPET and to be able to generalize the results for athletes, the inclusion criterion for VO2max was ≥ 50 ml/kg/min for males and ≥45 ml/kg/min for females achieved during the first CPET. This criterion was based on the 95th percentile of The American College of Sports Medicine reference values for VO2max (i.e., 56 ml/kg/min for males and 50 ml/kg/min for females). Because it may be possible that a participant performs his/her first CPET at the nadir of performance, we reduced the criterion for VO2max by 10% based on the expected maximum diurnal variation of VO2max during the day.
Testing Procedure
The Munich Chronotype Questionnaire (Roenneberg et al., 2004) was used to determine individuals' midpoint of sleep on free days corrected for oversleeping due to sleep debt on workdays (MSFsc). Further, participants filled out a questionnaire about their habitual training times, and a questionnaire asking the athletes at which of the six times of the day they would expect to have the best VO2max. The same questionnaire was filled out again after the sixth CPET when participants had performed one CPET at each of the predefined times of the day. Subsequently, sex and age were recorded and body height and blood pressure were measured. Then, participants filled out the Physical Activity Readiness Questionnaire (Shephard, 1988) and underwent a physical examination by a physician that included 12-channel resting electrocardiography and assessment of medical history.
Cardiopulmonary Exercise Test
Immediately before each CPET, body mass (kg) and body fat mass (kg) were measured with four-segment bioelectrical impedance analyses (Inbody 720, Biospace, Seoul, South Korea). Subjective sleepiness was assessed before CPET with the Karolinska Sleepiness Scale (Kaida et al., 2006) ranging from 1 (“extremely alert”) to 9 (“very sleepy, great effort to keep alert”). CPET was performed on a bicycle ergometer (Sport Excalibur, Lode Medical Technology, Groningen, The Netherlands) under standardized laboratory conditions (air humidity 40–55%, room temperature 20–22°C). For male/female participants the exercise protocol consisted of a warm-up of 5 min at 75/50 W, a linear increase of workload with 25/20 W/min up to exhaustion, and a 10 min cool-down phase at 75/50 W. Throughout the entire CPET, participants were verbally encouraged by the test supervisors to perform with maximum effort in all tests. Gas exchange was measured breath-by-breath (MetaMax 3B, Cortex Biophysik GmbH, Leipzig, Germany) with the highest 30 consecutive seconds of VO2 being determined as VO2max. After the cool-down phase a VO2max verification test was performed. Therefore, workload was set to 50% of maximum power output achieved during CPET for 2 min, then increased to 70% for 1 min and in the final stage of the verification test to 105% of maximum power output until exhaustion. VO2max verification was accepted if the verification-VO2 was ± 3% of the initially measured VO2max (Nolan et al., 2014). Because VO2-plateau was not reached in all tests and VO2-verification data from this study showed low agreement for diurnal variations (data not shown—under review), secondary exhaustion criteria were used to verify VO2max. During the first CPET, heart rate was measured with 12-channel electrocardiography and in the subsequent CPETs via 3-channel electrocardiography (Custo med GmbH, Ottobrunn, Germany). In all tests, heart rate was additionally measured with a heart rate belt (Polar T-34, Polar Electro Europe AG, Zug, Switzerland). Maximum heart rate was determined by the values recorded with the heart rate belt. Ratings of perceived exertion was assessed according to the 6–20 Borg scale (Borg, 1982). Blood lactate concentration was measured at rest, immediately after exhaustion, and at minutes one, three, and five of the cool-down phase. Blood samples were analyzed immediately after the exercise test (SuperGL Ambulance, Hitado Diagnostic Systems, Moehnesee, Germany). All participants reached all of the following exhaustion criteria in every test: respiratory exchange ratio ≥1.1, heart rate ≥95% of predicted maximum heart rate [210—age (years)], ratings of perceived exertion ≥19, and blood lactate concentration ≥8 mmol/l. These cut-off values were based on a previous study with athletes (Knaier et al., 2017) and proofed to strongly reduce type I errors without increasing the chance of type II errors (Knaier et al., 2018).
Observational Phase
Participants were advised to restrain from alcohol and sport during the entire study duration and to keep a constant sleeping routine. To monitor the compliance, participants filled out a diary recording bedtime, sleep time, wake-up time, and subjective sleep quality. Compliance to restrain from sport was monitored objectively by wGT3X+ ActiGraphs (Pensacola, United States) throughout the entire study.
Statistical Analysis
The primary outcome of this study was the difference in VO2max (in ml/kg/min) between the CPET with the lowest (i.e., nadir) and the CPET with the highest (i.e., peak) VO2max (i.e., maximum diurnal variation). We used paired t-tests to compare the nadir and peak of diurnal variations, day-to-day variations and the difference between diurnal and day-to-day variations. To calculate the day-to-day variations we compared the highest and lowest value from the sixth and the seventh test irrespective of which of these values was recorded in the first or second test. To investigate if there is a time of peak VO2max on a group level, we used descriptive statistics (i.e., boxplots) and a linear mixed effects model with post-hoc tests to compare the VO2max achieved at different times of the day. Habitual training time was coded as four different dummy variables, which were set to “1” if a participant trained during one of the predefined timeframes on any day during a usual week and to “0” if not. The timeframes were (Table 2): 04:00–08:59 (morning), 09:00–13:59 (noon), 14:00–18:59 (afternoon/evening), and 19:00–23:59 (evening/night). We used proportional odds logistic regression models to model the probability of achieving the peak VO2max in a certain time frame (Hosmer et al., 2013). The midpoint of sleep (MSFcs) and the dummy variables for the habitual training times were included as predictors. Likelihood ratio tests were used to assess statistical significance. Furthermore, we used descriptive statistics (i.e., histograms) to show the percentage of participants reaching their peak VO2max earlier during the day (i.e., at 16:00 and earlier), and reaching it later during the day (i.e., at 19:00 or later) for the two groups “morning types” and “evening types.” Median split of MSFsc was used to allocate participants to the groups. Furthermore, we performed a sensitivity analysis to investigate if there were learning (i.e., increase in performance) or fatigue effects (i.e., decrease in performance) from the first to the last CPET using a linear mixed effects model with test times in chronological order as continuous predictors. For each analysis, we report the estimated differences with 95% confidence intervals (95% CI) in outcome between the groups. For our analyses and graphics, we used IBM SPSS Statistics for Windows, version 24 (IBM Corp., Armonk, N.Y., USA) and R version 3.5.0 (R Foundation for Statistical Computing, Vienna, Austria), respectively.
Sample Size Calculation
For the sample size calculation, we assumed that the VO2max achieved at the time of peak VO2max was 60 ± 6 ml/kg/min (Knaier et al., 2017). Based on the previous reported difference of 10% in intermediate chronotypes and of 11.6% in all participants, we expected the VO2max achieved at the time of nadir VO2max to be 54 ± 6 ml/kg/min. Furthermore, we expected to reduce error variability by conservatively assuming a correlation of 0.5 between the VO2max from the peak and VO2max from the nadir of the day. With a 2-sided significance level of 0.05, the sample size needed to attain a targeted power of 90% to show a significant difference between the peak and nadir of VO2max was 13 participants. We increased this sample size to 18 participants to ensure an equal number of three participants in each of the six groups (i.e., the six different starting times for the first CPET). For our sample size calculation, we used G*Power (University of Kiel, Kiel, Germany).
Randomization
We used Friedman's Urn Model [UD(1, 0, 2)] to allocate the participants to the different starting times (Wei, 1978; Smith, 2014). This randomization procedure is known to lead to more balanced groups if the number of groups is large compared to the number of participants while still being free of selection or accidental bias (Wei, 1978). Participant recruitment, therefore, continued until three participants were allocated to each of the six starting times for CPET.
Results
Participant Flow and Characteristics
Twenty-seven participants were assessed for eligibility, whereby six participants did not meet the inclusion criteria for VO2max. One participant was excluded due to medical reasons. The remaining 20 participants were randomly allocated to the six different starting times (Figure 1). Two participants were excluded due to technical problems of measuring VO2 and one participant due to medical reasons. Participants' characteristics from the CPET with the highest VO2max are presented in Table 2. None of the participants was an exceptionally early or late chorotype. Participants followed the instructions regarding sleeping routine and physical activity.
Diurnal and Day-to-Day Variation in VO2max
Mean and standard deviation of VO2max in ml/kg/min, VO2max in L/min and Pmax at the time of the peak and the time of the nadir are presented in Table 3. There were significant diurnal variations for all three parameters as indicated by the mean differences. Furthermore, for all parameters there were significant correlations between the values from the peak and the nadir of the day. These correlations were higher than the conservatively assumed 0.5 from the sample size calculation.
There were also significant day-to-day variations for all three performance related parameters (Table 3).
For all three performance parameters the diurnal variations were significantly higher than the day-to-day variations with a mean difference of 3.0 ± 2.1 ml/kg/min (95% CI: 1.9, 4.1), 0.21 ± 0.17 L/min (95% CI: 0.12, 0.30), and 15.1 ± 11.8 W (95% CI: 9.0, 21.1), respectively. The diurnal variations were 2.5 to 4 times greater than the day-to-day variations for all three parameters. Figure 2 shows the contrast in diurnal and day-to-day variations in VO2max in ml/kg/min. Pearson's correlation showed no relationship between the diurnal variation in VO2max and the day-to-day variation in VO2max between the participants (r = 0.050, p = 0.848).
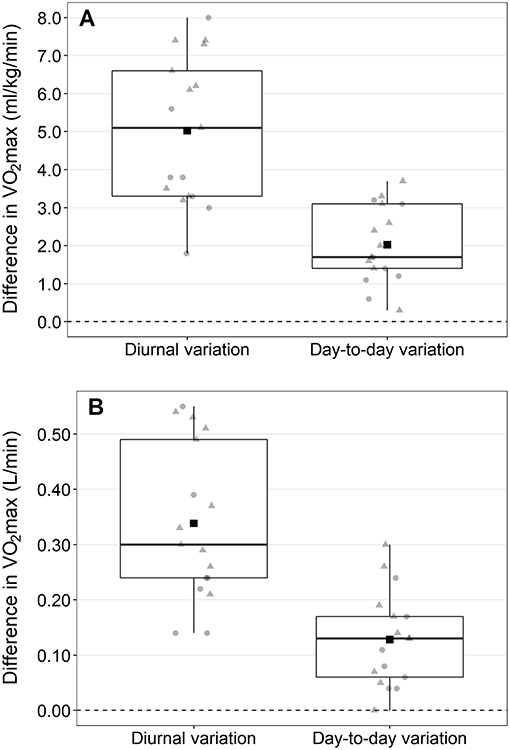
Figure 2. Differences between the cardiopulmonary exercise test with the highest and the exercise test with the lowest maximum oxygen uptake (VO2max) during the day (i.e., diurnal variation) and between the two exercise test taking place at the same time of the day (i.e., day-to-day variation). Values are presented for VO2max in ml/kg/min (A) and l/min (B). Square, mean difference; triangles, males; circles, females.
Influence of Time of Day on VO2max
The linear mixed effects model revealed no significant differences in VO2max between the different times of day (Figure 3). Neither the fixed effect of time ( = 8.00, p = 0.156) nor any pairwise comparison between the time points reached statistical significance (all p > 0.11). Time of day when peak VO2max was achieved was nearly equally distributed between the times 10:00 (n = 4), 16:00 (n = 3), 19:00 (n = 5), and 21:00 (n = 4). Interestingly, no participant achieved his/her peak VO2max at 13:00. The individual profiles for the diurnal variation differed between participants (Figure 4).
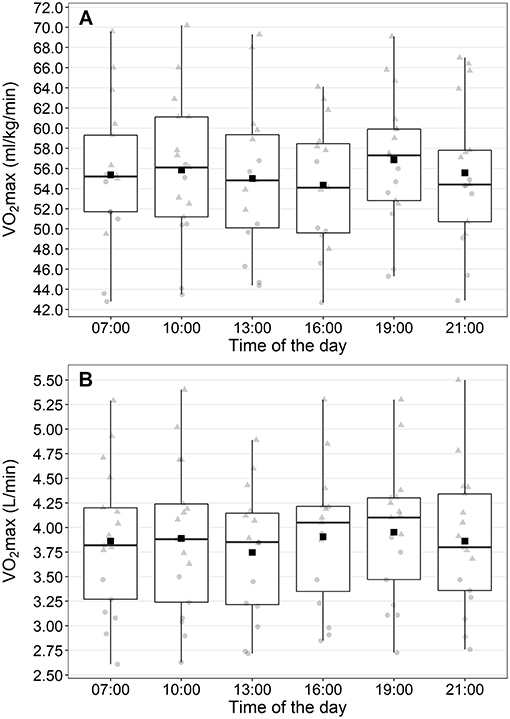
Figure 3. Maximum oxygen uptake (VO2max) at the different times of the day. Values are presented for VO2max in ml/kg/min (A) and l/min (B). Square, mean values; triangles, males; circles, females.
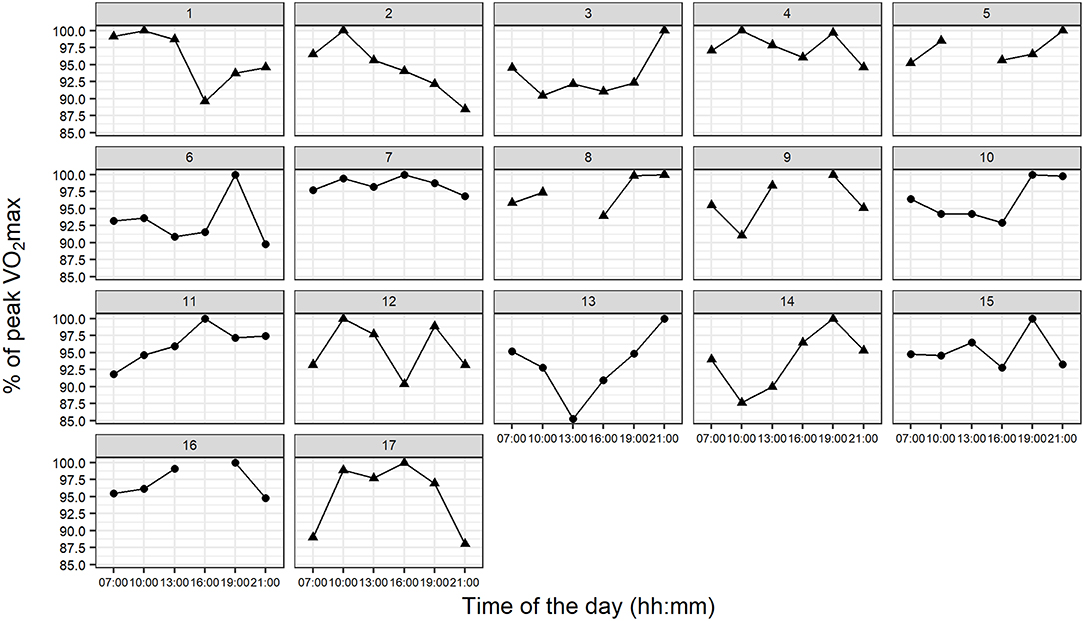
Figure 4. Individual profiles for the diurnal variation in percentage of peak VO2max from all participants. Triangles, males; circles, females.
Influence of Chronotype and Habitual Training Time on the Time of Peak VO2max
There was little evidence (p = 0.129) that the habitual training time influenced the time of day at which participants reached their peak VO2max since no effect reached statistical significance. Chronotype had a significant influence on the time of day when peak VO2max was achieved (OR = 0.34, 95%-CI: 0.11, 0.88, p = 0.035). Participants with later MSFsc had a higher probability to reach their peak VO2max earlier during the day than participants with earlier MSFsc. Figure 5 shows the percentage of participants reaching their peak VO2max earlier during the day (i.e., at 16:00 and earlier) and reaching it later during the day (i.e., at 19:00 or later) for the two groups “morning types” and “evening types.”
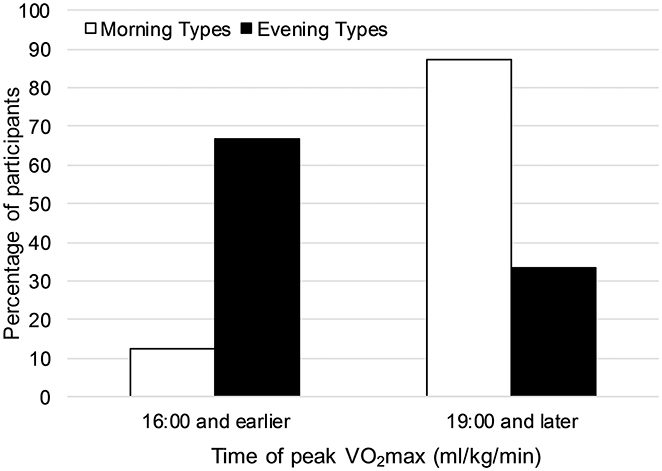
Figure 5. Percentage of participants reaching their peak VO2max (ml/kg/min) earlier during the day (i.e., at 16:00 and earlier) and reaching it later during the day (i.e., at 19:00 or later) for the two groups “morning types” and “evening types”.
Training Effects, Participants' Expectation, Adverse Events, and Exhaustion Criteria
The trial when peak VO2max (ml/kg/min) was achieved was nearly equally distributed between the trials: T1 (n = 1), T2 (n = 2), T3 (n = 4), T4 (n = 3), T5 (n = 3), T6 (n = 4). The linear regression analysis showed little evidence for training effects from the first to the last CPET ( 1.80, p = 0.179). None of the participants expected to reach their peak VO2max at 07:00, which proved to be true. Seven out of 17 participants anticipated their time of peak VO2max correctly. Except for one participant that was excluded because of cramps during the CPET, no further severe adverse events occurred during the study. The median (IQR) for the secondary exhaustion criteria at the time of peak VO2max (ml/kg/min) were maximum respiratory exchange ratio 1.23 (1.18, 1.26), maximum heart rate 187 (183, 197) and maximum rating of perceived exertion 20 (20, 20), respectively. At the time of the nadir of VO2max the values were maximum respiratory exchange ratio 1.23 (1.16, 1.26), maximum heart rate 186 (180, 192) and maximum rating of perceived exertion 20 (20, 20), respectively.
Discussion
This is the first study showing that diurnal variations in VO2max can be present without time-of-day effects. In line with several previous studies (Reilly and Baxter, 1983; Hill et al., 1988; Burgoon et al., 1992; Dalton et al., 1997; Deschenes et al., 1998; Reilly and Garrett, 1998; Brown et al., 2008; Souissi et al., 2012; Rae et al., 2015), we found no significant effect of the time of day on peak aerobic power. However, this was not due to the absence of diurnal variations, but rather to the fact that peak VO2max is achieved at different times of the day by different athletes and therefore masks the time-of-day effect. Furthermore, we were able to show that the presented diurnal variation in VO2max of 5.0 ± 1.9 ml/kg/min is 2.5 times greater than the day-to-day variation in VO2max of 2.0 ± 1.0 ml/kg/min, which is highly likely to have a striking impact on athletes. Similarly, for VO2max in L/min and Pmax the diurnal variations were 2.5 and 4 times greater than the day-to-day variations. Interestingly, only three participants (18%) showed their nadir of VO2max at 07:00—the time of day of the fewest competitions. Thus, the reported relevant diurnal variation is present at times when most competitions take place.
Previous studies reported an influence of habitual training time on the time of day when peak performance is achieved (Rae et al., 2015) and that training at a specific time of day can reduce diurnal variations (Torii et al., 1992). However, we chose a realistic free-living setting with trained athletes and found that most endurance athletes train at several times during the day to balance their training load with other obligations. Because athletes already train at different times of the day, this, therefore, may reduce the relevance of training habits on the time of peak VO2max and diurnal variations.
In contrast to Facer-Childs and Brandstaetter (2015), we did not find the association of early chronotypes reaching peak performance earlier during the day and late chronotypes reaching it later during the day. In fact, we found the opposite. Participants with later MSFsc had a higher probability to achieve peak VO2max at 10:00 or 16:00 than 19:00 or 21:00 (Figure 5). Several methodological differences between the two studies may explain the inconclusive results. Facer-Childs and Brandstaetter (2015) did not use MSFsc as a continuous measure for chronotype, but grouped athletes into the three categories early, intermediate, and late chronotype. This grouping resulted in an inadequate sample size of five participants in both the early and the late group. Furthermore, in Facer-Childs and Brandstaetter (2015), participants had to perform only five tests out of the six tests taking place at the different times of the day and the sequence of test times was not reported to be randomized. Finally, the authors did not explain the high differences in time of day when peak performance was reached between the three chronotype groups. Early and intermediate chronotypes were reported to reach peak performance 5.6 and 6.5 h after an entrained wake-up time, respectively, while late chronotypes needed 11.2 h to reach peak performance.
We are aware that this study was not powered to show the association between chronotype and diurnal variation. However, the results from our well-controlled study suggest that the association between time of peak VO2max and habitual training time or chronotype seems to be more complicated and not as simple and linear as reported by Facer-Childs and Brandstaetter (2015). Similarly, it is most likely that physiological factors, which are supposed to explain the time of day effects in physical performance, are also not simple and linear. Changes in core body temperature is the most frequently used explanation for the peak of performance in the late afternoon and early evening. However, Atkinson et al. (2005) demonstrated more than a decade ago that body temperature before an exercise test cannot solely explain the time of day differences in performance. In detail, athletes still performed better in a time trial at 17:30 following a short warm-up than at 07:30 even after a vigorous warm-up for 25 min, although post warm-up temperatures did not differ between the two times of the day. Our study was planned as a proof of concept to show actual diurnal variations independent of time of day effects, and we, therefore, refrained to measure any physiological parameters such as body temperature or melatonin. However, to explain the underlying physiological mechanism of diurnal variation in performance, further studies are necessary. These studies should include the measurement of a broad range of possible and expected confounders such as body temperature, melatonin, testosterone, chronotype, training time, and sleep times. The knowledge about the interaction of these factors with the time of peak VO2max might help to predict the time of peak performance and shift it to the time of competition. Furthermore, we observed high differences in VO2max between two neighboring measurement points in some athletes indicating a rapid increase or decrease in performance. These athletes seem to be most vulnerable to changing competition times. In a previous study, evening bright light exposure showed a trend to increase maximum cycling performance through reduced melatonin levels (Knaier et al., 2017). This method might be most beneficial for athletes with rapid decreases in performance in the evening.
Conclusions
Athletes show significant diurnal variations in VO2max, which are more than twice as large as the day-to-day variations. In competitive sports, these diurnal variations are highly relevant, because there are substantial differences in the time of the day when individuals achieve their peak performance. However, the direct application of our results for competitive sports is limited. We were able to show that some athletes have clear disadvantages if their time of peak performance does not comply with the time of competition, but further studies are required to investigate the underlying physiological mechanism causing these diurnal variations and to demonstrate methods to shift an athlete's time of peak performance. However, the results are directly applicable to exercise testing in research, athletes, and in a clinical setting. We could clearly show that it is necessary to conduct cardiopulmonary exercise testing at the same time of the day for pre- and post-intervention exercise tests to increase signal-to-noise-ratio. VO2max was not significantly higher at a specific time of the day compared to other times of the day. This absence of a time-of-day effect is explained by the fact that peak VO2max was achieved at different times of the day by different athletes. Habitual training times seem to have no influence on the time of day when peak VO2max is achieved, whereas the participants' chronotype may have an impact.
Data Availability
The datasets generated for this study are available on request to the corresponding author.
Author Contributions
RK, CC, AS-T: concept and design; RK: data acquisition; RK, DI, and MN: data analysis and interpretation; DI: statistical expertise; RK: writing manuscript; DI, MN, CC, and AS-T: writing—review and editing.
Funding
This work was supported by the Department of Sport Exercise and Health, University of Basel.
Conflict of Interest Statement
The authors declare that the research was conducted in the absence of any commercial or financial relationships that could be construed as a potential conflict of interest.
Acknowledgments
We thank all athletes who made this study possible by their participation. We further thank Karsten Königstein, Christopher Klenk, and Timo Hinrichs for examining the participants and Sabrina Frutig For Her Administrative Support and her support on data acquisition.
Supplementary Material
The Supplementary Material for this article can be found online at: https://www.frontiersin.org/articles/10.3389/fphys.2019.00219/full#supplementary-material
Abbreviations
VO2max, maximum oxygen uptake; CPET, cardiopulmonary exercise test; MSFsc, midpoint of sleep on free days corrected for oversleeping due to sleep debt on workdays.
References
Aloui, K., Abedelmalek, S., Chtourou, H., Wong, D. P., Boussetta, N., and Souissi, N. (2017). Effects of time-of-day on oxidative stress, cardiovascular parameters, biochemical markers, and hormonal response following level-1 Yo-Yo intermittent recovery test. Physiol. Int. 104, 77–90. doi: 10.1556/2060.104.2017.1.6
Atkinson, G., Todd, C., Reilly, T., and Waterhouse, J. (2005). Diurnal variation in cycling performance: influence of warm-up. J. Sports Sci. 23, 321–329. doi: 10.1080/02640410410001729919
Borg, G. (1982). Ratings of perceived exertion and heart rates during short-term cycle exercise and their use in a new cycling strength test. Int. J. Sports Med. 03, 153–158. doi: 10.1055/s-2008-1026080
Brown, F. M., Neft, E. E., and LaJambe, C. M. (2008). Collegiate rowing crew performance varies by morningness-eveningness. J. Strength Cond. Res. 22, 1894–1900. doi: 10.1519/JSC.0b013e318187534c
Burgoon, P., Holland, G. J., Loy, S. F., and Vincent, W. J. (1992). A comparison of morning and evening “types” during maximum exercise. J. Strength Cond. Res. 6, 115–119.
Chin, C.-Y., Chow, G. C.-C., Hung, K.-C., Kam, L.-H., Chan, K.-C., Mok, Y.-T., et al. (2015). The diurnal variation on cardiovascular endurance performance of secondary school athlete student. Asian J. Sports Med. 6:e22697. doi: 10.5812/asjsm.6(2)2015.22697
Chtourou, H., Hammouda, O., Souissi, H., Chamari, K., Chaouachi, A., and Souissi, N. (2012). Diurnal variations in physical performances related to football in young soccer players. Asian J. Sports Med. 3, 139–144.
Chtourou, H., and Souissi, N. (2012). The effect of training at a specific time of day: a review. J. Strength Cond. Res. 26, 1984–2005. doi: 10.1519/JSC.0b013e31825770a7
Dalton, B., McNaughton, L., and Davoren, B. (1997). Circadian rhythms have no effect on cycling performance. Int. J. Sports Med. 18, 538–542. doi: 10.1055/s-2007-972678
Deschenes, M. R., Sharma, J. V., Brittingham, K. T., Casa, D. J., Armstrong, L. E., and Maresh, C. M. (1998). Chronobiological effects on exercise performance and selected physiological responses. Eur. J. Appl. Physiol. 77, 249–256. doi: 10.1007/s004210050329
Facer-Childs, E., and Brandstaetter, R. (2015). The impact of circadian phenotype and time since awakening on diurnal performance in athletes. Curr. Biol. 25, 518–522. doi: 10.1016/j.cub.2014.12.036
Hill, D. W. (2014). Morning–evening differences in response to exhaustive severe-intensity exercise. Appl. Physiol. Nutr. Metab. 39, 248–254. doi: 10.1139/apnm-2013-0140
Hill, D. W., Cureton, K. J., Collins, M. A., and Grisham, S. C. (1988). Diurnal variations in responses to exercise of “morning types” and “evening types.” J. Sports Med. Phys. Fitness 28, 213–219.
Hosmer, D. W. Jr., Lemeshow, S., and Sturdivant, R. X. (2013). Applied Logistic Regression, 3rd Edn. John Wiley and Sons. Available online at: https://www.wiley.com/en-gb/Applied+Logistic+Regression%2C+3rd+Edition-p-9780470582473
Kaida, K., Takahashi, M., Åkerstedt, T., Nakata, A., Otsuka, Y., Haratani, T., et al. (2006). Validation of the Karolinska sleepiness scale against performance and EEG variables. Clin. Neurophysiol. 117, 1574–1581. doi: 10.1016/j.clinph.2006.03.011
Knaier, R., Niemeyer, M., Wagner, J., Infanger, D., Hinrichs, T., Klenk, C., et al. (2018). Which cut-offs for secondary VO2max criteria are robust to diurnal variations? Med. Sci. Sports Exerc. doi: 10.1249/MSS.0000000000001869. [Epub ahead of print].
Knaier, R., Schäfer, J., Rossmeissl, A., Klenk, C., Hanssen, H., Höchsmann, C., et al. (2017). Prime time light exposures do not seem to improve maximal physical performance in male elite athletes, but enhance end-spurt performance. Front. Physiol. 8:264. doi: 10.3389/fphys.2017.00264
Nolan, P. B., Beaven, M. L., and Dalleck, L. (2014). Comparison of intensities and rest periods for VO2max verification testing procedures. Int. J. Sports Med. 35, 1024–1029. doi: 10.1055/s-0034-1367065
Rae, D. E., Stephenson, K. J., and Roden, L. C. (2015). Factors to consider when assessing diurnal variation in sports performance: the influence of chronotype and habitual training time-of-day. Eur. J. Appl. Physiol. 115, 1339–1349. doi: 10.1007/s00421-015-3109-9
Reilly, T., and Baxter, C. (1983). Influence of time of day on reactions to cycling at a fixed high intensity. Br. J. Sports Med. 17, 128–130.
Reilly, T., and Garrett, R. (1998). Investigation of diurnal variation in sustained exercise performance. Ergonomics 41, 1085–1094. doi: 10.1080/001401398186397
Roenneberg, T., Kuehnle, T., Pramstaller, P., Ricken, J., Havel, M., Guth, A., et al. (2004). A marker for the end of adolescence. Curr. Biol. 14, R1038–R1039. doi: 10.1016/j.cub.2004.11.039
Shephard, R. J. (1988). PAR-Q, Canadian home fitness test and exercise screening alternatives. Sports Med. 5, 185–195. doi: 10.2165/00007256-198805030-00005
Smith, M. D. (2014). “Biased-Coin Randomization,” in Wiley StatsRef: Statistics Reference Online (Wiley). Available at: https://doi/pdf/10.1002/9780471462422.eoct370
Souissi, M., Chtourou, H., Zrane, A., Cheikh, R. B., Dogui, M., Tabka, Z., et al. (2012). Effect of time-of-day of aerobic maximal exercise on the sleep quality of trained subjects. Biol. Rhythm Res. 43, 323–330. doi: 10.1080/09291016.2011.589159
Torii, J., Shinkai, S., Hino, S., Kurokawa, Y., Tomita, N., Hirose, M., et al. (1992). Effect of time of day on adaptive response to a 4-week aerobic exercise program. J. Sports Med. Phys. Fitness 32, 348–352.
Keywords: circadian, chronotype, time of day, habitual, performance
Citation: Knaier R, Infanger D, Niemeyer M, Cajochen C and Schmidt-Trucksäss A (2019) In Athletes, the Diurnal Variations in Maximum Oxygen Uptake Are More Than Twice as Large as the Day-to-Day Variations. Front. Physiol. 10:219. doi: 10.3389/fphys.2019.00219
Received: 12 October 2018; Accepted: 20 February 2019;
Published: 18 March 2019.
Edited by:
Gary Iwamoto, University of Illinois at Urbana-Champaign, United StatesReviewed by:
Shelley Tischkau, Southern Ilinois University School of Medicine, United StatesHeiko T. Jansen, Washington State University, United States
Copyright © 2019 Knaier, Infanger, Niemeyer, Cajochen and Schmidt-Trucksäss. This is an open-access article distributed under the terms of the Creative Commons Attribution License (CC BY). The use, distribution or reproduction in other forums is permitted, provided the original author(s) and the copyright owner(s) are credited and that the original publication in this journal is cited, in accordance with accepted academic practice. No use, distribution or reproduction is permitted which does not comply with these terms.
*Correspondence: Raphael Knaier, raphael.knaier@unibas.ch