- 1Hubei Insect Resources Utilization and Sustainable Pest Management Key Laboratory, College of Plant Science and Technology, Huazhong Agricultural University, Wuhan, China
- 2Department of Entomology, University of Kentucky, Lexington, KY, United States
Nestmate discrimination allows social insects to recognize nestmates from non-nestmates using colony-specific chemosensory cues, which typically evoke aggressive behavior toward non-nestmates. Functional analysis of genes associated with nestmate discrimination has been primarily focused on inter-colonial discrimination in Hymenopterans, and parallel studies in termites, however, are grossly lacking. To fill this gap, we investigated the role of two genes, Orco and 5-HTT, associated with chemosensation and neurotransmission respectively, in nestmate discrimination in a highly eusocial subterranean termite, Odontotermes formosanus (Shiraki). We hypothesized that knocking down of these genes will compromise the nestmate recognition and lead to the antagonistic behavior. To test this hypothesis, we carried out (1) an in vivo RNAi to suppress the expression of Orco and 5-HTT, respectively, (2) a validation study to examine the knockdown efficiency, and finally, (3) a behavioral assay to document the phenotypic impacts/behavioral consequences. As expected, the suppression of either of these two genes elevated stress level (e.g., vibrations and retreats), and led to aggressive behaviors (e.g., biting) in O. formosanus workers toward their nestmates, suggesting both Orco and 5-HTT can modulate nestmate discrimination in termites. This research links chemosensation and neurotransmission with nestmate discrimination at the genetic basis, and lays the foundation for functional analyses of nestmate discrimination in termites.
Introduction
Nestmate discrimination is the ability of social insects to recognize colony members using colony-specific recognition cues (Wilson, 1971). Depending on the species, recognition cues can be derived from a variety of biotic and abiotic sources (Hölldobler and Wilson, 1977). The process of nestmate discrimination involves two steps: recognition, where individuals that encounter one another assess whether the other is a nestmate on the basis of their recognition cues (Mateo, 2010); and action, where they adjust their behavior according to the chemical information perceived during recognition (Reeve, 1989; Baracchi et al., 2013). In the case of nestmates, individuals usually exhibit altruistic behaviors; otherwise they may exhibit stressful or aggressive behaviors (Hölldobler and Michener, 1980). Nestmate discrimination plays a crucial role in maintaining the stability of eusocial societies, ensuring that altruistic behaviors are directed only toward related individuals (Wilson, 1971).
Recognition and communication in social insects rely primarily on the olfactory system (Nehring and Steiger, 2018), which uses olfactory receptors (ORs) to convert olfactory cues into neural signals, which then elicit neuroendocrinological outputs and corresponding behavioral responses (Touhara and Vosshall, 2009). Eusocial insects must effectively discriminate recognition cues, and this capacity is reflected at the genetic level: the numbers of olfactory and gustatory receptor genes in the genomes of social insects are significantly greater than those in non-social insects (Terrapon et al., 2014; Harrison et al., 2018). The olfactory receptor co-receptor (Orco) is highly conserved across insect species (Nakagawa et al., 2012; Zhou et al., 2012), and is considered to form a tetramer which is arranged around specific pore and bound together by a small cytoplasmic anchor domain (Butterwick et al., 2018). Orco can form Orco-OR heterotetramers with all insect tuning ORs, the minimal sequence conservation among which maps largely to the pore and anchor domain (Butterwick et al., 2018). In solitary insects, the disruption of Orco can cause dramatic reductions in olfactory sensitivity, abolishing the behavioral, and electrophysiological responses to a number of general odorants (DeGennaro et al., 2013; Yang et al., 2016). In social insects, Orco mutant ants display a lack of social interactions, abnormal social behaviors, and reduced fitness (Trible et al., 2017; Yan et al., 2017). However, whether disruption of Orco expression can alter the process of nestmate discrimination is still unclear.
When individuals of social insects encounter non-nestmates, one common behavioral response is aggression (Liebert and Starks, 2004). Animal aggression may be affected and shaped by a large number of factors (Archer, 1988). In particular, serotonin (5-HT) has been implicated to be positively associated with aggression in a wide range of insect species, from solitary insects such as fruit flies (Drosophila melanogaster) and stalk-eyed flies (Teleopsis dalmanni) (Dierick and Greenspan, 2007; Bubak et al., 2014b) to social insects such as ants (Formica rufa and Tetramorium caespitum) (Kostowski and Tarchalska, 1972; Bubak et al., 2016). Moreover, the 5-HT levels of individuals that serve a defensive role in the colony are higher than those of their nestmates (Bubak et al., 2016; Ishikawa et al., 2016; Ohkawara and Aonuma, 2016). The serotonin transporter (5-HTT) is a key regulator of central serotonergic activity and can reuptake 5-HT from the synaptic cleft to the presynaptic neuron, thereby terminating its action at the synapse (Owens and Nemeroff, 1998). Mammal species show reduced aggression levels after treatment with 5-HT reuptake inhibitors (Miczek and Fish, 2005; Chichinadze et al., 2011), and knockdown of the 5-HTT gene resulted in a reduction of aggression and home cage activity in mice (Holmes et al., 2002, 2003). However, the role of 5-HTT in insects, specifically in the process of nestmate discrimination by social insects, has rarely been documented.
In the past few years, screening and functional analyses of genes associated with inter-colonial discrimination have focused primarily on Hymenoptera (Kravitz and Huber, 2003; Li-Byarlay et al., 2014; Toth et al., 2014; Chandrasekaran et al., 2015), and parallel studies in termites, however, are grossly lacking. To fill this gap, in this study, we investigated the role of two genes, Orco and 5-HTT, associated with chemosensation and neurotransmission respectively, in nestmate discrimination in a highly eusocial subterranean termite, Odontotermes formosanus (Shiraki). Workers make up the majority of O. formosanus colonies and are responsible for foraging and colony maitantenance, i.e., frequently interacting with predators, competitors, and other biological stressors (Huang et al., 2006, 2007). Here, we hypothesized that knocking down of these genes will compromise the nestmate recognition and lead to the antagonistic behavior. To test this hypothesis, we carried out (1) an in vivo RNAi to suppress the expression of Orco and 5-HTT, respectively, (2) a validation study to examine the knockdown efficiency, and finally, (3) a behavioral assay to document the phenotypic impacts/behavioral consequences.
Materials and Methods
Odontotermes Formosanus Colony Maintenance
Colonies of O. formosanus were collected from Wuhan city in Hubei province, China. A total of 16 colonies were used in this study (Table S1). Termites were maintained in sealed plastic containers in complete darkness (L:D = 0:24), at 25 ± 1°C and 75 ± 1% RH. All colonies were maintained under laboratory conditions without soil and with moist filter paper for 1 d before the subsequent experiments. After that, whole body samples of workers from 3 colonies were collected and stored at −80°C for tissue-specific gene expression analysis, and workers from the remaining 13 colonies were used for the RNAi experiments.
Molecular Cloning
Total RNA from the whole bodies of 10 O. formosanus workers were extracted using TRIzol reagent (TaKaRa) according to the manufacturer's protocol, which was then treated with DNase I (TaKaRa) to remove genomic DNA. RNA quality was calculated and checked using a NanoDrop 2000 spectrophotometer (Thermo). The SMARTer RACE cDNA Amplification Kit (Clontech) was used to obtain the full-length sequences of Orco and 5-HTT by amplifying both the 5′ and 3′ cDNA ends. Gene-specific primers (GSPs) for 5′- and 3′-RACE of Orco and 5-HTT were designed based on the partial sequences of Unigene 55916 and Unigene 49370 (Table S2), respectively, which were obtained from transcriptome data of O. formosanus worker heads (Huang et al., 2012b). The amplification reactions were carried out as follows: 98°C for 3 min; 40 cycles of 98°C for 10 s, 60°C (touchdown to 55°C) for 10 s and 72°C for 30 s; and one cycle at 72°C for 7 min. The PCR products were purified using Wizard SV Gel Purification Kit (Promega). Purified PCR products were cloned into the pMD18-T vector (TaKaRa) followed by transformation in Trans1-T1 Phage Resistant Chemically Competent Cell (Transgen Biotech) according to the manufacturer's protocol. The plasmids were isolated from bacteria, and sequenced in both directions by Tsingke Biological Technology (Wuhan).
Phylogenetic Analysis
Protein prediction was performed using ExPASy (http://web.expasy.org/translate/). cDNA and amino acid sequence similarity searches were performed using BLAST (https://blast.ncbi.nlm.nih.gov/Blast.cgi). Signal peptide and domain organization were predicted with SMART (http://smart.embl-heidelberg.de/). Protein multiple alignment analyses were performed using MEGA 6.0 and GenDoc 2.0 software. Phylogenetic analyses were performed using MEGA 6.0 software (Tamura et al., 2013). Amino acid sequences obtained from the NCBI database (Table S3) were transformed to a FASTA formatted file and uploaded to MEGA 6.0 software. Followed performing protein alignments using the ClustalW (Thompson et al., 1994), the phylogenetic relationships of Orco/5-HTT and their homolog genes in other species were analyzed using the neighbor-joining (NJ) method, with 1,000 bootstrap replications. MEGA 6.0 software was used to generate graphic of the sequence alignment.
Tissue-Specific Expression Profile
Antenna, leg, head, and abdomen-thorax tissues of O. formosanus workers were dissected on an ice-cold plate. Per replicate, antennae of 100 individuals, legs of 30 individuals, and heads and abdomen-thoraxes of 15 individuals were used. Extraction and quality identification of total RNA were performed as described in “Molecular cloning and sequencing of Orco and 5-HTT.” Approximately 1 μg of RNA was converted to cDNA using the PrimeScriptTM RT Reagent Kit with gDNA Eraser (Perfect Real Time) (TaKaRa). The RT-qPCR assay was performed using the My IQTM Color Real-time PCR Detection System (Bio-Rad) with cDNA as the template. Relative expression levels of Orco and 5-HTT among the four tissue types were calculated using the 2−ΔΔCt method (Van Hiel et al., 2009) with Ribosomal protein S18 (RPS18) and glyceraldehyde-3-phosphate dehydrogenase (GAPDH) as reference genes. Five and six biological replicates were set for RT-qPCR of Orco and 5-HTT, respectively. The primers used for RT-qPCR are listed in Table S2.
In vivo Dietary RNAi Experiment
In order to construct a plasmid expressing dsRNA, fragments of Orco and 5-HTT were amplified by RT-PCR using specific primers (Table S2), which were designed based on the full-length sequences of Orco and 5-HTT. The restriction enzyme cutting sites of KpnI (Fermentas) and EcoRI (Fermentas) were added to the 5′ ends of the primers. PCR products were cloned to the L4440 plasmid, which has two T7 promoters in inverted orientation flanking the multiple cloning sites. The recombinant L4440-Orco and L4440-5-HTT plasmids were transformed to an HT115 (DE3) competent cell. Single colonies of HT115 (DE3) were shake-cultured in LB medium supplemented with 75 mg/mL ampicillin and 12.5 mg/mL tetracycline at 37°C overnight. The culture was diluted to 100-fold in 800 ml LB medium supplemented with 75 mg/mL ampicillin and 12.5 mg/mL tetracycline and shake-cultured at 37°C to OD600 = 0.5. Synthesis of dsRNA was induced by 0.4 mM IPTG, and then the bacteria were further incubated for 4 h at 37°C. The dsRNA of Orco and 5-HTT were purified following the method described by Timmons et al. (2001). The quality of dsRNA was calculated and checked using a NanoDrop 2000 spectrophotometer (Thermo).
The dietary RNAi experiment was carried out by placing groups of 30 O. formosanus workers into 55 mm diameter petri dishes which were covered with treated filter paper. For both dsOrco and ds5-HTT feeding behavioral assays, the experimental design included one treatment [workers were fed using filter paper treated with 40 μL dsOrco/ds5-HTT (1.5 μg/μL) and Nile blue (0.5% w/v)], and one control [workers were fed using filter paper treated with 40 μL dsRNA for green fluorescent protein (dsGFP, 1.5 μg/μL) and Nile blue (0.5% w/v)] group. The workers used in dietary RNAi experiment were maintained in complete darkness (L:D = 0:24), at 25 ± 1°C and 75 ± 1% RH. The O. formosanus workers were maintained for 24 h, and then workers whose guts were colored by Nile blue were used in the gene silencing validation and behavioral assays.
qRT-PCR Validation Study
After the dietary RNAi experiment, whole bodies of 5 O. formosanus workers were pooled and crushed in 1.5 mL centrifuge tubes with liquid nitrogen using sterilized grinding pestles. Total RNA extraction and cDNA synthesis of the samples were completed as described in “Molecular cloning and sequencing of Orco and 5-HTT” and “Tissue-specific expression profiles of Orco and 5-HTT.” The RT-qPCR assay was performed using the My IQ™ Color Real-time PCR Detection System (Bio-Rad) with cDNA as the template. Relative expression levels of Orco and 5-HTT between workers fed with dsOrco/ds5-HTT and dsGFP were calculated using the 2−ΔΔCt method (Van Hiel et al., 2009) with RPS18 and GAPDH as reference genes. Gene silencing validation of Orco and 5-HTT included three colonies with 8 and 7 replicates, respectively. The primers used for RT-qPCR are listed in Table S2.
Behavioral Assay
After the dietary RNAi experiment, behavioral assays were carried out in a 35 mm diameter petri dish lined with moist filter papers. The behavioral assay for each gene included three groups, including (1) 1 target termite treated with dsOrco/ds5-HTT vs. 5 non-target termites treated with dsGFP (treatment group); (2) 1 target termite treated with dsOrco/ds5-HTT vs. 5 non-target termites treated with dsOrco/ds5-HTT (treatment group); and (3) 1 target termite treated with dsGFP vs. 5 non-target termites treated with dsGFP (control group). In each test group, the 5 non-target termites were first placed in the Petri dish to adapt to the environment for 10 min, and then the target termite, marked with red color on the pronotum, was added. Behavioral phenotypes of the termites in the Petri dish were recorded immediately with a digital camera (HDR-XR550, SONY). All Petri dishes were kept under laboratory conditions (25 ± 1°C, 75% ± 1% RH) and illuminated by a ceiling-mounted fluorescent lamp, which was necessary for the video-recording.
At the beginning of the behavioral assay and another three timepoints thereafter (30 min, 1 h, 2 h), we analyzed a 10 min section of video for the frequency and duration of five behaviors (biting, vibrating, retreat, grooming, trophallaxis) between the target and non-target workers, but did not analyze behaviors among the non-target termites. The five behaviors are shown in Figure 1 and Video S1 and described as follows: biting is an aggressive behavior in which one termite bites the body parts of another termite with the maxillae after the encounter (Tanner and Adler, 2009); vibrating is a distinctive behavior in which termites move repeatedly backwards and forwards after the encounter (Reinhard and Clément, 2002); retreat is a behavior in which termites move quickly in the opposite direction to avoid contact after the encounter (Martin et al., 2009); grooming is a behavior in which one termite licks the cuticle of a nestmate with its mouthpart after the encounter (Konrad et al., 2018); and trophallaxis is a behavior in which one termite transfers food or other fluids to a nestmate through mouth-to-mouth contact (Konrad et al., 2018). The Orco and 5-HTT silencing behavioral assays included 10 colonies with 28 and 26 replicates, respectively.
Results
The Role of Orco in Nestmate Discrimination
Bioinformatic Analysis of O. formosanus Orco
Based on the partial sequence of Unigene 55916 derived from transcriptome data of O. formosanus worker heads, a 1,737 bp nucleotide sequence representing the complete cDNA sequence of Orco was amplified. The cDNA of Orco included a 199 bp 5′ untranslated region (UTR) and a 119 bp 3′ UTR with a poly (A) tail. The open reading frame (ORF) of Orco was 1,419 bp and encoded a predicted protein of 472 amino acid (aa), with predicted molecular mass of 53.64 kDa and an isoelectric point (pI) of 6.89. SMART analysis showed that Orco contained a 20 aa transmembrane region and a 393 aa 7tm_6 domain (Figure 2A).
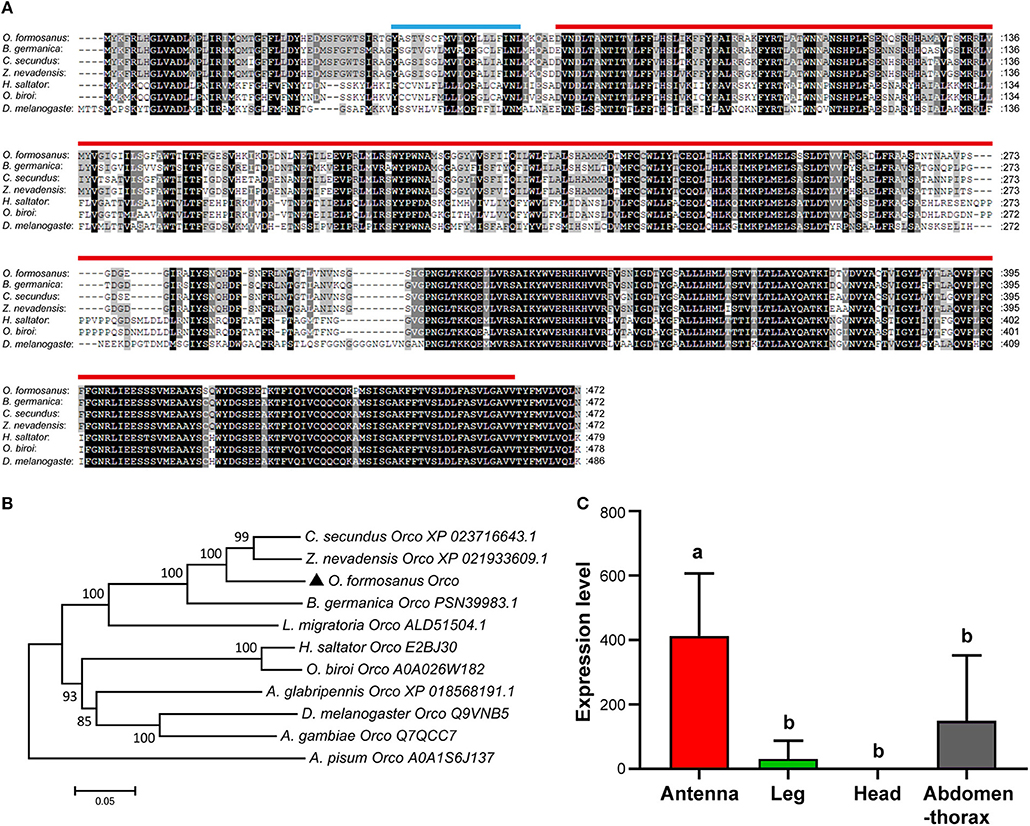
Figure 2. The structure, phylogeny and spatial expression of O. formosanus Orco. (A) Protein alignment of the Orco gene; the transmembrane domain is marked with a blue line, and the 7tm_6 domain is marked with a red line. (B) Phylogenetic tree of Orco in O. formosanus with homologs in other 10 species. (C) Expression patterns of Orco in antenna, leg, head, and abdomen-thorax tissue of workers (Tukey's HSD test, P < 0.05).
Multiple amino acid sequence alignments showed that Orco from O. formosanus shared the highest sequence identity with Orco genes from the termite Zootermopsis nevadensis (88%), the termite Cryptotermes secundus (87%), and the cockroach Blattella germanica (80%) (Figure 2A). Evolutionary analysis showed that Orco from O. formosanus was clustered with Orco from Z. nevadensis, C. secundus, B. germanica, and the locust Locusta migratoria, while Orco from two ant species (Harpegnathos saltator and Ooceraea biroi) was clustered in a different branch together with Orco from D. melanogaster, the longhorn beetle Anoplophora glabripennis, and the mosquito Anopheles gambiae (Figure 2B).
Tissue Distribution of O. formosanus Orco
The spatial distribution of gene expression may inform an initial understanding of the gene's function, therefore we performed qRT-PCR to profile the expression level of Orco in different tissue types (antenna, leg, head, and abdomen-thorax) of O. formosanus. The results showed that Orco exhibited the highest expression level in antennae, and there were no significant differences in the Orco expression among leg, head, and abdomen-thorax tissues (Figure 2C).
Behavioral Phenotype of Orco Knockdown
To investigate the potential role of Orco in O. formosanus nestmate discrimination, RNAi-mediated silencing of Orco was performed in workers. The expression level of Orco was significantly suppressed in workers fed with dsOrco compared to workers fed with dsGFP 24 h after the dietary RNAi experiment (df = 7, P < 0.05; Figure 3A). This result indicates that RNAi effectively suppressed the expression of Orco in workers 24 h after the dietary RNAi experiment.
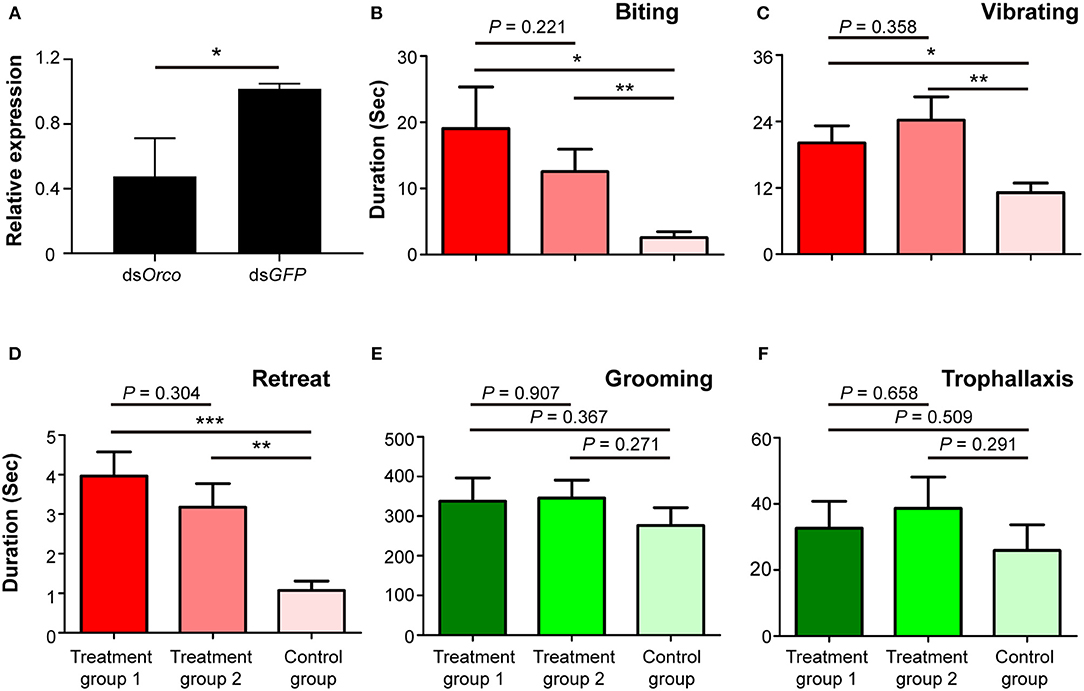
Figure 3. Phenotypic impact of Orco knockdown on nestmate discrimination. (A) The expression level of Orco after RNAi treatment. (B) Biting, (C) vibrating, (D) retreat, (E) grooming, and (F) trophallaxis behaviors modulated by silencing of Orco. The red columns represent social behaviors triggered by non-nestmate discrimination, including biting, vibrating, and retreat; the green columns represent social behaviors triggered by nestmate discrimination, including grooming and trophallaxis. Treatment group 1 consisted of 1 termite treated with dsOrco and 5 termites treated with dsGFP, treatment group 2 consisted of 1 termite treated with dsOrco and 5 termites treated with dsOrco, and the control group consisted of 1 termite treated with dsGFP and 5 additional termites treated with dsGFP. Data are shown as the means ± S.E.M. (paired t-test; *P < 0.05; **P < 0.01; ***P < 0.001).
Behavioral assays were performed 24 h after the dietary RNAi experiment. The durations of stressful and aggressive behaviors between target and non-target workers were significantly higher in treatment groups 1 and 2 than in control groups. These behaviors were biting (treatment group 1: t = 2.553, df = 27, P < 0.05; treatment group 2: t = 2.931, df = 27, P < 0.01; Figure 3B), vibrating (treatment group 1: t = 2.757, df = 27, P < 0.05; treatment group 2: t = 3.151, df = 27, P < 0.01; Figure 3C), and retreat (treatment group 1: t = 4.649, df = 27, P < 0.001; treatment group 2: t = 3.483, df = 27, P < 0.01; Figure 3D). The duration of these behaviors between target and non-target workers in treatment groups 1 and 2 was not significantly different (Figures 3B–D). The durations of grooming and trophallaxis behaviors between target and non-target workers were not significantly different among the three groups (Figures 3E,F). The behavioral assays indicated that RNAi-mediated Orco silencing significantly altered social behaviors associated with non-nestmate discrimination (biting, vibrating, retreat) of workers in O. formosanus, but did not significantly influence social behaviors associated with nestmate discrimination (grooming and trophallaxis).
The Role of 5-HTT in Nestmate Discrimination of O. formosanus
Bioinformatic Analysis of O. formosanus 5-HTT
Based on the partial sequence of Unigene 49370 derived from transcriptome data of O. formosanus worker heads, a 2,595 bp nucleotide sequence representing the complete cDNA sequence of 5-HTT was amplified. The cDNA of 5-HTT included a 728 bp 5′ UTR and a 343 bp 3′ UTR with a poly (A) tail. The ORF of 5-HTT was 1,524 bp and encoded a predicted protein of 618 aa, with predicted molecular mass of 59.65 kDa and a pI of 6.71. SMART analysis showed that 5-HTT contained a 44 aa Coiled coil domain and a 507 aa SNF domain (Figure 4A).
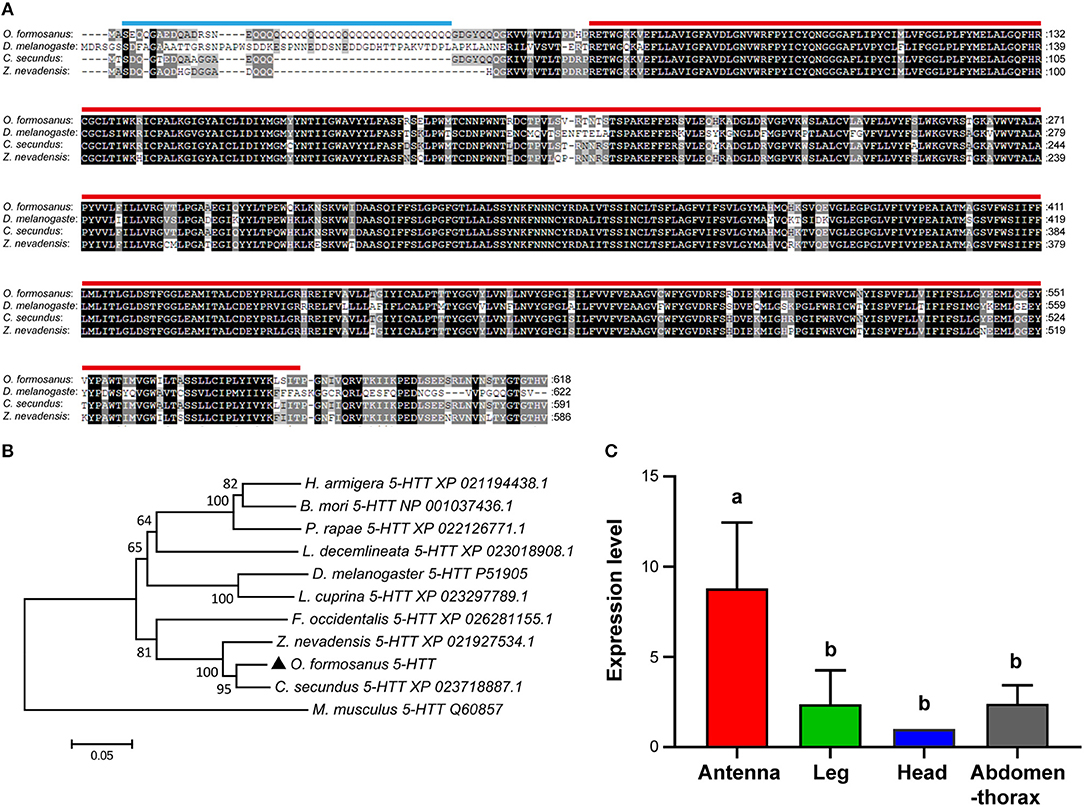
Figure 4. The structure, phylogeny, and spatial expression of O. formosanus 5-HTT. (A) Protein alignment of the 5-HTT gene, the coiled coil domain is marked with a blue line, and the SNF domain is marked with a red line. (B) Phylogenetic tree of 5-HTT in O. formosanus with homologs in 10 other species. (C) Expression patterns of 5-HTT in antenna, leg, head and abdomen-thorax tissue of workers (Tukey's HSD test, P < 0.05).
Multiple amino acid sequence alignments showed that 5-HTT from O. formosanus shared the highest sequence identity with 5-HTT genes from the termite C. secundus (94%), the termite Z. nevadensis (91%), and the thrips Frankliniella occidentalis (83%) (Figure 4A). Evolutionary analysis showed that 5-HTT from O. formosanus was clustered with 5-HTT from Z. nevadensis, C. secundus, and F. occidentalis. 5-HTT from the mouse Mus musculus was clustered in a distinct branch apart from the 5-HTT of insect species (Figure 4B).
Tissue Distribution of O. formosanus 5-HTT
Spatial analysis of 5-HTT expression in different tissue types (antenna, leg, head, and abdomen-thorax) of O. formosanus showed that 5-HTT exhibited the highest expression level in antennae, and there were no significant differences in the 5-HTT expression among leg, head, and abdomen-thorax tissues (Figure 4C).
Behavioral Phenotype of 5-HTT Knockdown
The expression level of 5-HTT was significantly suppressed in workers fed with ds5-HTT compared to workers fed with dsGFP 24 h after the dietary RNAi experiment (df = 6, P < 0.05; Figure 5A). This result indicates that RNAi effectively suppressed the expression of 5-HTT in workers 24 h after the dietary RNAi experiment.
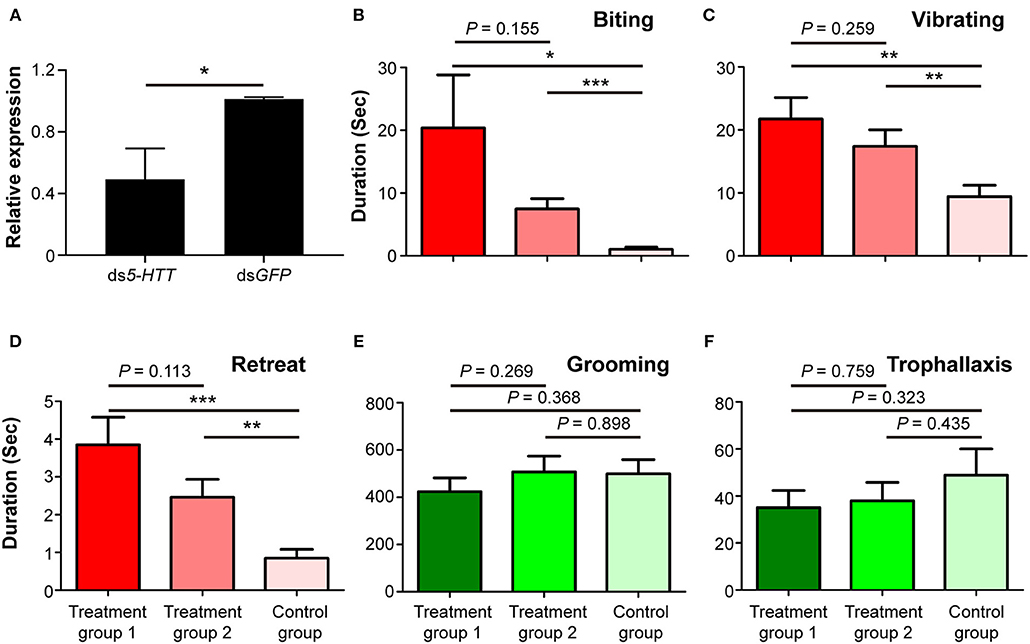
Figure 5. Phenotypic impact of 5-HTT knockdown on nestmate discrimination. (A) The expression level of 5-HTT after RNAi treatment. (B) Biting, (C) vibrating, (D) retreat, (E) grooming, and (F) trophallaxis behaviors modulated by silencing 5-HTT. The red columns represent social behaviors triggered by non-nestmate discrimination, including biting, vibrating, and retreat; the green columns represent social behaviors triggered by nestmate discrimination, including grooming and trophallaxis. Treatment group 1 consisted of 1 termite treated with ds5-HTT and 5 termites treated with dsGFP, treatment group 2 consisted of 1 termite treated with ds5-HTT and 5 termites treated with ds5-HTT, and control group consisted of 1 termite treated with dsGFP and 5 additional termites treated with dsGFP. Data are shown as the means ± S.E.M. (paired t-test; *P < 0.05; **P < 0.01; ***P < 0.001).
The results of behavioral assays following RNAi-mediated 5-HTT silencing showed that the durations of stressful and aggressive behaviors between target and non-target workers were significantly higher in treatment groups 1 and 2 than in control groups. These behaviors were biting (treatment group 1: t = 2.303, df = 25, P < 0.05; treatment group 2: t = 4.122, df = 25, P < 0.001; Figure 5B), vibrating (treatment group 1: t = 3.509, df = 25, P < 0.01; treatment group 2: t = 3.369, df = 25, P < 0.01; Figure 5C), and retreat (treatment group 1: t = 4.316, df = 25, P < 0.001; treatment group 2: t = 3.139, df = 25, P < 0.01; Figure 5D). The duration of these behaviors between target and non-target workers in treatment groups 1 and 2 was not significantly different (Figures 5B–D). The durations of grooming and trophallaxis behaviors between target and non-target workers were not significantly different among the three groups (Figures 5E,F). The behavioral assays indicated that RNAi-mediated 5-HTT silencing induced similar behavioral alterations in workers of O. formosanus as with Orco silencing, suggesting that the expression level of 5-HTT can also affect the discrimination among O. formosanus workers from the same colony.
Discussion
Nestmate discrimination of social insects is characterized by altruistic behaviors toward nestmates and stressful or aggressive behaviors toward non-nestmates (Huang et al., 2007, 2012a; Wenseleers et al., 2011; Konrad et al., 2018). Behaviors linked to nestmate discrimination vary across different social insect species (Reinhard and Clément, 2002). In termites, altruistic behaviors toward nestmates include grooming and trophallaxis (Nalepa, 2015), while stressful or aggressive behaviors toward non-nestmates mainly include biting, vibrating, and retreat behaviors (Reinhard and Clément, 2002; Martin et al., 2009; Tanner and Adler, 2009). Interactions between termites are direct reflections of the results of nestmate discrimination. In this study, we used O. formosanus to investigate the role of the Orco and 5-HTT genes in nestmate discrimination through observation of the above-mentioned five social behaviors (biting, vibrating, retreat, grooming, and trophallaxis).
Phenotypic Impact of Orco Knockdown
The Orco protein of O. formosanus contains a transmembrane region, which might aid in dimerization (Mukunda et al., 2014) and a 7tm_6 domain, which belongs to the insect chemoreceptor family (Goodstein et al., 2012; Benton, 2015). The results of our multiple amino acid sequence alignments and evolutionary analysis provide support for the idea that Orco is highly conserved across all insect species (Vosshall et al., 2000; Nakagawa et al., 2012; Zhou et al., 2012; Yan et al., 2017). In O. formosanus, Orco is predominantly expressed in the antennae. This expression pattern is similar to that of Orco orthologs in many other insect species, and further supports the idea that Orco plays critical roles in chemosensation (Malpel et al., 2008; Liu et al., 2013; Zhou et al., 2014; Franco et al., 2016).
The Orco protein is considered to form heterotetramer with all insect ORs for the purpose of chemosensation (Nakagawa et al., 2012; Zhou et al., 2012; Butterwick et al., 2018). Similar to observations in ant Orco mutants (Trible et al., 2017; Yan et al., 2017), our behavioral assays showed that the incidence of stressful behaviors (vibrating and retreat) between nestmates increased when Orco in one or both of them was knocked down, which was most likely due to a defect in sensing nestmate recognition cues (Yan et al., 2017). Furthermore, these results suggest that olfaction is important for accurate nestmate discrimination in termites, as in ants, although termites may have a limited ability to discriminate odors when compared to the eusocial Hymenoptera (Terrapon et al., 2014; Harrison et al., 2018). Knockdown of Orco increased the duration of biting behavior between nestmates, a behavioral phenotype that was more extreme than that observed in ant Orco mutants (Trible et al., 2017; Yan et al., 2017). These results might be due to a tolerance to non-nestmate odors, as in some ant or termite species that can peacefully coexist with heterospecific or non-nestmate conspecific competitors (Su and Haverty, 1991; Lenoir et al., 2001; Kaib et al., 2002; Boulay et al., 2004). O. formosanus individuals construct underground colonies in which foraging individuals are protected by mud shelters, and where a king-queen reproductive pair always resides (Huang et al., 2006). Colonies may be entrained to a relatively simple odor and show high sensitivity to dissimilar odors, so that the knockdown of Orco triggers aggressive behaviors (biting) among nestmates. Overall, these results suggest that the knockdown of Orco may cause a disruption of chemosensation in O. formosanus, which can degrade the ability to discriminate nestmates and non-nestmates, and ultimately trigger stressful (vibrating and retreat) and aggressive behaviors (biting) between nestmates.
Phenotypic Impact of 5-HTT Knockdown
The 5-HTT protein of O. formosanus contains a coiled coil domain and an SNF domain, which belongs to the neurotransmitter transport system responsible for the removal of released neurotransmitters from the extracellular space (Attwell and Bouvier, 1992). Multiple amino acid sequence alignments and evolutionary analysis suggested that the 5-HTT gene was highly conserved across different insect species but was distinct from that in mammal species. The antennae of insects contain an abundance of sensory neurons to process olfactory, gustatory, mechanosensory, hygrosensory, and thermosensory information (Watanabe et al., 2014; Versteven et al., 2017). 5-HT is secreted into the antennal hemolymph to modulate the responses of sensory neurons (Dolzer et al., 2001; Grosmaitre et al., 2001). Thus, the high expression of 5-HTT in the antennae of O. formosanus might be responsible for the reuptake of 5-HT.
5-HT has been demonstrated to be positively associated with aggression in many insect species, such as fruit flies (D. melanogaster), stalk-eyed flies (Teleopsis dalmanni), and ants (F. rufa and T. caespitum) (Kostowski and Tarchalska, 1972; Dierick and Greenspan, 2007; Bubak et al., 2014b; Williams et al., 2014). However, this same association has not been observed in crickets (Gryllus bimaculatus) or ants (Oecophylla smaragdina) (Kamhi et al., 2015; Rillich and Stevenson, 2015). Our behavioral studies showed that incidence of stressful (vibrating and retreat) and aggressive behaviors (biting) among nestmates was significantly elevated following 5-HTT knockdown, supporting the existence of species-specific relationships between 5-HT and aggression (Bubak et al., 2014a). These results were most likely due to the fact that 5-HTT knockdown disrupts reuptake of 5-HT, which leads to an elevation of 5-HT in the synaptic cleft (Owens and Nemeroff, 1998), and therefore an increase in aggressive behavior. Moreover, 5-HT plays important role in the process of chemosensation by acting on peripheral neurons and brain centers. 5-HT can secret into the hemolymph to modulate the sensitivity of olfactory receptor neurons in insects' antennae (Dolzer et al., 2001; Grosmaitre et al., 2001). In the olfactory center of Drosophila melanogaster brain, 5-HT can enhance projection neuron responses by increasing the sensitivity of projection neuron (Dacks et al., 2009). In our study, there may have been slight differences in the odors of target and non-target workers which were separately maintained before the behavioral assays. Therefore, the enhanced sensitivity to different odors caused by 5-HTT knockdown might be another interpretation of the elevated biting, vibrating, and retreat behaviors between target and non-target workers.
Collectively, we analyzed the primary sequence and spatial expression pattern of Orco and 5-HTT in O. formosanus workers, which are respectively, associated with chemosensation and neurotransmission in insects. Our behavioral assays demonstrated that both Orco and 5-HTT knockdown could trigger aggressive or stress responses (biting, vibrating, and retreat) among nestmates. These results suggest that downregulation of Orco and 5-HTT expression can alter the nestmate discrimination of eusocial termites. Future studies on the neural circuit and nerve impulse transmission related to Orco and 5-HTT is warranted to better understand the mechanism of nestmate discrimination in social animals.
Data Availability
All datasets generated for this study are included in the manuscript and/or the Supplementary Files.
Author Contributions
PS, XZ, and QH conceived and designed the experiments. PS and SY performed the experiments. PS, SY, and QH analyzed the data. PS, AM, CL, XZ, and QH drafted and revised the manuscript. All authors discussed the results and commented on the manuscript. All authors approved the final manuscript.
Funding
This research was funded by the Fundamental Research Funds for the Central Universities (grant number: 2662016PY062 and 2013PY007) and the National Natural Science Foundation of China (grant number: 31772516).
Conflict of Interest Statement
The authors declare that the research was conducted in the absence of any commercial or financial relationships that could be construed as a potential conflict of interest.
Acknowledgments
We thank Huan Xu, Yongyong Gao, Wenjie Li, and Yutong Liu for helping with the sample collection and the video observation of the behavior assays. Also, we are grateful for the comments and suggestions provided by the reviewers.
Supplementary Material
The Supplementary Material for this article can be found online at: https://www.frontiersin.org/articles/10.3389/fphys.2019.00714/full#supplementary-material
Video S1. Social interactions between one Odontotermes formosanus worker (marked in red) and five nestmates.
Abbreviations
5-HT, serotonin; 5-HTT, serotonin transporter; aa, amino acid; GFP, green fluorescent protein; GSPs, gene-specific primers; NJ, neighbor-joining; Orco, olfactory receptor co-receptor; ORF, open reading frame; ORs, olfactory receptors; pI, isoelectric point; UTR, untranslated region.
References
Attwell, D., and Bouvier, M. (1992). Cloners quick on the uptake. Curr. Biol. 2 , 541–543. doi: 10.1016/0960-9822(92)90024-5
Baracchi, D., Petrocelli, I., Cusseau, G., Pizzocaro, L., Teseo, S., et al. (2013). Facial markings in the hover wasps: quality signals and familiar recognition cues in two species of Stenogastrinae. Anim. Behav. 85, 203–212. doi: 10.1016/j.anbehav.2012.10.027
Benton, R. (2015). Multigene family evolution: perspectives from insect chemoreceptors. Trends. Ecol. Evol. 30 , 590–600. doi: 10.1016/j.tree.2015.07.009
Boulay, R., Katzav-Gozansky, T., Hefetz, A., and Lenoir, A. (2004). Odour convergence and tolerance between nestmates through trophallaxis and grooming in the ant Camponotus fellah (Dalla Torre). Insect. Soc. 51, 55–61. doi: 10.1007/s00040-003-0706-0
Bubak, A. N., Grace, J. L., Watt, M. J., Renner, K. J., and Swallow, J. G. (2014a). Neurochemistry as a bridge between morphology and behavior: Perspectives on aggression in insects. Curr. Zool. 60, 778–790. doi: 10.1093/czoolo/60.6.778
Bubak, A. N., Renner, K. J., and Swallow, J. G. (2014b). Heightened serotonin influences contest outcome and enhances expression of high-intensity aggressive behaviors. Behav. Brain Res. 259, 137–142. doi: 10.1016/j.bbr.2013.10.050
Bubak, A. N., Yaeger, J. D., Renner, K. J., Swallow, J. G., and Greene, M. J. (2016). Neuromodulation of nestmate recognition decisions by pavement ants. PLoS ONE 11:e0166417. doi: 10.1371/journal.pone.0166417
Butterwick, J. A., del Mármol, J., Kim, K. H., Kahlson, M. A., Rogow, J. A., Walz, T., et al. (2018). Cryo-EM structure of the insect olfactory receptor Orco. Nature 560, 447–452. doi: 10.1038/s41586-018-0420-8
Chandrasekaran, S., Rittschof, C. C., Djukovic, D., Gu, H., Raftery, D., et al. (2015). Aggression is associated with aerobic glycolysis in the honey bee brain. Genes Brain Behav. 14, 158–166. doi: 10.1111/gbb.12201
Chichinadze, K., Chichinadze, N., and Lazarashvili, A. (2011). Hormonal and neurochemical mechanisms of aggression and a new classification of aggressive behavior. Aggress. Violent. Behav. 16, 461–471. doi: 10.1016/j.avb.2011.03.002
Dacks, A. M., Green, D. S., Root, C. M., Nighorn, A. J., and Wang, J. W. (2009). Serotonin modulates olfactory processing in the antennal lobe of Drosophila. J. Neurogenet. 23, 366–377. doi: 10.1080/01677060903085722
DeGennaro, M., McBride, C. S., Seeholzer, L., Nakagawa, T., Dennis, E. J., et al. (2013). orco mutant mosquitoes lose strong preference for humans and are not repelled by volatile DEET. Nature 498:487. doi: 10.1038/nature12206
Dierick, H. A., and Greenspan, R. J. (2007). Serotonin and neuropeptide F have opposite modulatory effects on fly aggression. Nat. Genet. 39, 678–682. doi: 10.1038/ng2029
Dolzer, J., Krannich, S., Fischer, K., and Stengl, M. (2001). Oscillations of the transepithelial potential of moth olfactory sensilla are influenced by octopamine and serotonin. J. Exp. Biol. 204, 2781–2794.
Franco, T. A., Oliveira, D. S., Moreira, M. F., Leal, W. S., and Melo, A. C. (2016). Silencing the odorant receptor co-receptor RproOrco affects the physiology and behavior of the Chagas disease vector Rhodnius prolixus. Insect Biochem. Molec. 69, 82–90. doi: 10.1016/j.ibmb.2015.02.012
Goodstein, D. M., Shu, S., Howson, R., Neupane, R., Hayes, R. D., et al. (2012). Phytozome: a comparative platform for green plant genomics. Nucleic. Acids. Res. 40, D1178–D1186. doi: 10.1093/nar/gkr944
Grosmaitre, X., Marion-Poll, F., and Renou, M. (2001). Biogenic amines modulate olfactory receptor neurons firing activity in Mamestra brassicae. Chem. Senses 26, 653–661. doi: 10.1093/chemse/26.6.653
Harrison, M. C., Jongepier, E., Robertson, H. M., Arning, N., Bitard-Feildel, T., et al. (2018). Hemimetabolous genomes reveal molecular basis of termite eusociality. Nat. Ecol. Evol. 2, 557–566. doi: 10.1038/s41559-017-0459-1
Hölldobler, B., and Michener, C. D. (1980). “Mechanisms of identification and discrimination in social Hymenoptera,” in Evolution of Social Behavior: Hypotheses and Empirical Tests, ed H. Markl (Weinheim:VC), 35–58.
Hölldobler, B., and Wilson, E. O. (1977). The number of queens: an important trait in ant evolution. Naturwissenschaften 64, 8–15. doi: 10.1111/evo.12010
Holmes, A., Murphy, D. L., and Crawley, J. N. (2002). Reduced aggression in mice lacking the serotonin transporter. Psychopharmacology 161, 160–167. doi: 10.1007/s00213-002-1024-3
Holmes, A., Murphy, D. L., and Crawley, J. N. (2003). Abnormal behavioral phenotypes of serotonin transporter knockout mice: parallels with human anxiety and depression. Biol. Psychiat. 54, 953–959. doi: 10.1016/j.biopsych.2003.09.003
Huang, Q., Guan, C., Shen, Q., Hu, C., and Zhu, B. (2012a). Aggressive behavior and the role of antennal sensillae in the termite Reticulitermes chinensis (Isoptera: Rhinotermitidae). Sociobiology 59, 1239–1251.
Huang, Q., Sun, P., Zhou, X., and Lei, C. (2012b). Characterization of head transcriptome and analysis of gene expression involved in caste differentiation and aggression in Odontotermes formosanus (Shiraki). PLoS ONE 7:e50383. doi: 10.1371/journal.pone.0050383
Huang, Q. Y., Chen, Y., Li, J. H., and Lei, C. L. (2007). Intercolony agonism in the subterranean termite Odontotermes formosanus (Isoptera: Termitidae). Sociobiology 50, 867–880.
Huang, Q. Y., Lei, C. L., and Xue, D. (2006). Field evaluation of a fipronil bait against subterranean termite Odontotermes formosanus (Isoptera: Termitidae). J. Econ. Entomol. 99, 455–461. doi: 10.1603/0022-0493-99.2.455
Ishikawa, Y., Aonuma, H., Sasaki, K., and Miura, T. (2016). Tyraminergic and octopaminergic modulation of defensive behavior in termite soldier. PLoS ONE 11:e0154230. doi: 10.1371/journal.pone.0154230
Kaib, M., Franke, S., Francke, W., and Brandl, R. (2002). Cuticular hydrocarbons in a termite: phenotypes and a neighbour-stranger effect. Physiol. Entomol. 27, 189–198. doi: 10.1046/j.1365-3032.2002.00292.x
Kamhi, J. F., Nunn, K., Robson, S. K., and Traniello, J. F. (2015). Polymorphism and division of labour in a socially complex ant: neuromodulation of aggression in the Australian weaver ant, Oecophylla smaragdina. Proc. Biol. Sci. 282:1811. doi: 10.1098/rspb.2015.0704
Konrad, M., Pull, C. D., Metzler, S., Seif, K., Naderlinger, E., et al. (2018). Ants avoid superinfections by performing risk-adjusted sanitary care. Proc. Natl. Acad. Sci. U.S.A. 115, 2782–2787. doi: 10.1073/pnas.1713501115
Kostowski, W., and Tarchalska, B. (1972). The effects of some drugs affecting brain 5-HT on the aggressive behaviour and spontaneous electrical activity of the central nervous system of the ant, Formica rufa. Brain Res. 38, 143–149. doi: 10.1016/0006-8993(72)90595-1
Kravitz, E. A., and Huber, R. (2003). Aggression in invertebrates. Curr. Opin. Neurobiol. 13, 736–743. doi: 10.1016/j.conb.2003.10.003
Lenoir, A., D'Ettorre, P., Errard, C., and Hefetz, A. (2001). Chemical ecology and social parasitism in ants. Annu. Rev. Entomol. 46, 573–599. doi: 10.1146/annurev.ento.46.1.573
Li-Byarlay, H., Rittschof, C. C., Massey, J. H., Pittendrigh, B. R., and Robinson, G. E. (2014). Socially responsive effects of brain oxidative metabolism on aggression. Proc. Natl. Acad. Sci. U.S.A. 111:12533–12537. doi: 10.1073/pnas.1412306111
Liebert, A. E., and Starks, P. T. (2004). The action component of recognition systems: a focus on the response. Ann. Zool. Fenn. 41, 747–764.
Liu, S., Huang, Y. J., Qiao, F., Zhou, W. W., Gong, Z. J., et al. (2013). Cloning, tissue distribution, and transmembrane orientation of the olfactory co-receptor Orco from two important Lepidopteran rice pests, the leaffolder (Cnaphalocrocis medinalis) and the striped stem borer (Chilo suppressalis). J. Integr. Agr. 12, 1816–1825. doi: 10.1016/S2095-3119(13)60501-8
Malpel, S., Merlin, C., François, M. C., and Jacquin-Joly, E. (2008). Molecular identification and characterization of two new Lepidoptera chemoreceptors belonging to the Drosophila melanogaster OR83b family. Insect Mol. Biol. 17, 587–596. doi: 10.1111/j.1365-2583.2008.00830.x
Martin, S. J., Helanterä, H., Kiss, K., Lee, Y. R., and Drijfhout, F. P. (2009). Polygyny reduces rather than increases nestmate discrimination cue diversity in Formica exsecta ants. Insect. Soc. 56, 375–383. doi: 10.1007/s00040-009-0035-z
Mateo, J. M. (2010). Self-referent phenotype matching and long-term maintenance of kin recognition. Anim. Behav. 80, 929–935. doi: 10.1016/j.anbehav.2010.08.019
Miczek, K. A., and Fish, E. W. (2005). “Monoamines, GABA, glutamate, and aggression”, in Biology of Aggression, ed R. J. Nelson (New York, NY: Oxford University Press), 114–149.
Mukunda, L., Lavista-Llanos, S., Hansson, B. S., and Wicher, D. (2014). Dimerisation of the Drosophila odorant coreceptor Orco. Front. Cell Neurosci. 8:261. doi: 10.3389/fncel.2014.00261
Nakagawa, T., Pellegrino, M., Sato, K., Vosshall, L. B., and Touhara, K. (2012). Amino acid residues contributing to function of the heteromeric insect olfactory receptor complex. PLoS ONE 7:9. doi: 10.1371/journal.pone.0032372
Nalepa, C. A. (2015). Origin of termite eusociality: trophallaxis integrates the social, nutritional, and microbial environments. Ecol. Entomol. 40, 323–335. doi: 10.1111/een.12197
Nehring, V., and Steiger, S. (2018). Sociality and communicative complexity: insights from the other insect societies. Curr. Opin. Insect Sci. 28, 19–25. doi: 10.1016/j.cois.2018.04.002
Ohkawara, K., and Aonuma, H. (2016). Changes in the levels of biogenic amines associated with aggressive behavior of queen in the social parasite ant Vollenhovia nipponica. Insect. Soc. 63, 257–264. doi: 10.1007/s00040-016-0461-7
Owens, M. J., and Nemeroff, C. B. (1998). The serotonin transporter and depression. Depress. Anxiety. 8(Suppl. 1), 5–12.
Reeve, H. K. (1989). The evolution of conspecific acceptance thresholds. Am. Nat. 133, 407–435. doi: 10.1007/s10071-007-0071-x
Reinhard, J., and Clément, J. L. (2002). Alarm reaction of European Reticulitermes termites to soldier head capsule volatiles (Isoptera, Rhinotermitidae). J. Insect Behav. 15, 95–107. doi: 10.1023/A:1014436313710
Rillich, J., and Stevenson, P. A. (2015). Releasing stimuli and aggression in crickets: octopamine promotes escalation and maintenance but not initiation. Front. Behav. Neurosci. 9:95. doi: 10.3389/fnbeh.2015.00095
Su, N. Y., and Haverty, M. I. (1991). Agonistic behavior among colonies of the Formosan subterranean termite, Coptotermes formosanus Shiraki (Isoptera: Rhinotermitidae), from Florida and Hawaii: lack of correlation with cuticular hydrocarbon composition. J. Insect Behav. 4, 115–128. doi: 10.1007/BF01092555
Tamura, K., Stecher, G., Peterson, D., Filipski, A., and Kumar, S. (2013). MEGA6: molecular evolutionary genetics analysis version 6.0. Mol. Biol. Evol. 30, 2725–2729. doi: 10.1093/molbev/mst197
Tanner, C. J., and Adler, F. R. (2009). To fight or not to fight: context-dependent interspecific aggression in competing ants. Anim. Behav. 77, 297–305. doi: 10.1016/j.anbehav.2008.10.016
Terrapon, N., Li, C., Robertson, H. M., Ji, L., Meng, X., et al. (2014). Molecular traces of alternative social organization in a termite genome. Nat. Commun. 5:3636. doi: 10.1038/ncomms4636
Thompson, J. D., Higgins, D. G., and Gibson, T. J. (1994). Clustal-W - improving the sensitivity of progressive multiple sequence alignment through sequence weighting, position-specific gap penalties and weight matrix choice. Nucleic. Acids Res. 22, 4673–4680. doi: 10.1007/978-1-4020-6754-9_3188
Timmons, L., Court, D. L., and Fire, A. (2001). Ingestion of bacterially expressed dsRNAs can produce specific and potent genetic interference in Caenorhabditis elegans. Gene 263, 103–112. doi: 10.1016/S0378-1119(00)00579-5
Toth, A. L., Tooker, J. F., Radhakrishnan, S., Minard, R., Henshaw, M. T., et al. (2014). Shared genes related to aggression, rather than chemical communication, are associated with reproductive dominance in paper wasps (Polistes metricus). BMC Genomics 15:75. doi: 10.1186/1471-2164-15-75
Touhara, K., and Vosshall, L. B. (2009). Sensing odorants and pheromones with chemosensory receptors. Annu. Rev. Physiol. 71, 307–332. doi: 10.1146/annurev.physiol.010908.163209
Trible, W., Olivos-Cisneros, L., McKenzie, S. K., Saragosti, J., Chang, N. C., et al. (2017). Orco mutagenesis causes loss of antennal lobe glomeruli and impaired social behavior in ants. Cell 170, 727–735 e710. doi: 10.1016/j.cell.2017.07.001
Van Hiel, M. B., Van Wielendaele, P., Temmerman, L., Soest, S., Vuerinckx, K., et al. (2009). Identification and validation of housekeeping genes in brains of the desert locust Schistocerca gregaria under different developmental conditions. BMC Mol. Biol. 10:10. doi: 10.1186/1471-2199-10-56
Versteven, M., Vanden Broeck, L., Geurten, B., Zwarts, L., Decraecker, L., et al. (2017). Hearing regulates Drosophila aggression. Proc. Natl. Acad. Sci. U.S.A 114, 1958–1963. doi: 10.1073/pnas.1605946114
Vosshall, L. B., Wong, A. M., and Axel, R. (2000). An olfactory sensory map in the fly brain. Cell 102, 147–159. doi: 10.1016/S0092-8674(00)00021-0
Watanabe, H., Shimohigashi, M., and Yokohari, F. (2014). Serotonin-immunoreactive sensory neurons in the antenna of the cockroach Periplaneta americana. J. Comp. Neurol. 522, 414–434. doi: 10.1002/cne.23419
Wenseleers, T., Alves, D. A., Francoy, T. M., Billen, J., and Imperatriz-Fonseca, V. L. (2011). Intraspecific queen parasitism in a highly eusocial bee. Biol. Lett. 7, 173–176. doi: 10.1098/rsbl.2010.0819
Williams, M. J., Goergen, P., Phad, G., Fredriksson, R., and Schiöth, H. B. (2014). The Drosophila Kctd-family homologue Kctd12-like modulates male aggression and mating behaviour. Eur. J. Neurosci. 40, 2513–2526. doi: 10.1111/ejn.12619
Yan, H., Opachaloemphan, C., Mancini, G., Yang, H., Gallitto, M., et al. (2017). An engineered orco mutation produces aberrant social behavior and defective neural development in ants. Cell 170, 736–747.e9. doi: 10.1016/j.cell.2017.06.051
Yang, B., Fujii, T., Ishikawa, Y., and Matsuo, T. (2016). Targeted mutagenesis of an odorant receptor co-receptor using TALEN in Ostrinia furnacalis. Insect Biochem. Molec. 70, 53–59. doi: 10.1016/j.ibmb.2015.12.003
Zhou, X., Slone, J. D., Rokas, A., Berger, S. L., Liebig, J., et al. (2012). Phylogenetic and transcriptomic analysis of chemosensory receptors in a pair of divergent ant species reveals sex-specific signatures of odor coding. PLoS Genet. 8:e1002930. doi: 10.1371/journal.pgen.1002930
Keywords: nestmate discrimination, termites, chemosensation, neurotransmission, in vivo RNAi
Citation: Sun P, Yu S, Merchant A, Lei C, Zhou X and Huang Q (2019) Downregulation of Orco and 5-HTT Alters Nestmate Discrimination in the Subterranean Termite Odontotermes formosanus (Shiraki). Front. Physiol. 10:714. doi: 10.3389/fphys.2019.00714
Received: 08 March 2019; Accepted: 23 May 2019;
Published: 11 June 2019.
Edited by:
Peng He, Guizhou University, ChinaReviewed by:
Xiao Hong Su, Northwest University, ChinaLiang Sun, Tea Research Institute, Chinese Academy of Agricultural Sciences, China
Hao Guo, Chinese Academy of Sciences, China
Copyright © 2019 Sun, Yu, Merchant, Lei, Zhou and Huang. This is an open-access article distributed under the terms of the Creative Commons Attribution License (CC BY). The use, distribution or reproduction in other forums is permitted, provided the original author(s) and the copyright owner(s) are credited and that the original publication in this journal is cited, in accordance with accepted academic practice. No use, distribution or reproduction is permitted which does not comply with these terms.
*Correspondence: Xuguo Zhou, xuguozhou@uky.edu; Qiuying Huang, qyhuang2006@mail.hzau.edu.cn