- 1Department of Biomedical Sciences and Center for Craniofacial Research and Diagnosis, Texas A&M University College of Dentistry, Dallas, TX, United States
- 2Department of Periodontics and Center for Craniofacial Research and Diagnosis, Texas A&M University College of Dentistry, Dallas, TX, United States
Dentin sialophosphoprotein (DSPP) is an extracellular matrix protein that is highly expressed in odontoblasts, but only transiently expressed in presecretory ameloblasts during tooth development. We previously generated a knockin mouse model expressing a mouse equivalent (DSPP, p.P19L) of human mutant DSPP (p.P17L; referred to as “DsppP19L/+”), and reported that DsppP19L/+ and DsppP19L/P19L mice manifested a dentin phenotype resembling human dentinogenesis imperfecta (DGI). In this study, we analyzed pathogenic effects of mutant P19L-DSPP on enamel development in DsppP19L/+ and DsppP19L/P19L mice. Micro-Computed Tomography (μCT) analyses of 7-week-old mouse mandibular incisors showed that DsppP19L/P19L mice had significantly decreased enamel volume and/or enamel density at different stages of amelogenesis examined. Acid-etched scanning electron microscopy (SEM) analyses of mouse incisors demonstrated that, at the mid-late maturation stage of amelogenesis, the enamel of wild-type mice already had apparent decussating pattern of enamel rods, whereas only minute particulates were found in DsppP19L/+ mice, and no discernible structures in DsppP19L/P19L mouse enamel. However, by the time that incisor enamel was about to erupt into oral cavity, distinct decussating enamel rods were evident in DsppP19L/+ mice, but only poorly-defined enamel rods were revealed in DsppP19L/P19L mice. Moreover, μCT analyses of the mandibular first molars showed that DsppP19L/+ and DsppP19L/P19L mice had a significant reduction in enamel volume and enamel density at the ages of 2, 3, and 24weeks after birth. Backscattered and acid-etched SEM analyses revealed that while 3-week-old DsppP19L/+ mice had similar pattern of enamel rods in the mandibular first molars as age-matched wild-type mice, no distinct enamel rods were observed in DsppP19L/P19L mice. Yet neither DsppP19L/+ nor DsppP19L/P19L mice showed well-defined enamel rods in the mandibular first molars by the age of 24weeks, as judged by backscattered and acid-etched SEM. In situ hybridization showed that DSPP mRNA level was markedly reduced in the presecretory ameloblasts, but immunohistochemistry revealed that DSP/DSPP immunostaining signals were much stronger within the presecretory ameloblasts in Dspp mutant mice than in wild-type mice. These results suggest that mutant P19L-DSPP protein caused developmental enamel defects in mice, which may be associated with intracellular retention of mutant DSPP in the presecretory ameloblasts.
Introduction
Tooth development involves a series of sequential interactions that occur between dental epithelium and underlying mesenchyme (Thesleff, 2003; Balic and Thesleff, 2015). The dental epithelium differentiates into ameloblasts, which form enamel. Enamel formation occurs in three major stages, including presecretory, secretory, and maturation stages (Warshawsky and Smith, 1974; Hu et al., 2007; Moradian-Oldak, 2012; Balic and Thesleff, 2015). During the presecretory stage, the dental epithelium differentiates into presecretory ameloblasts. As the presecretory ameloblasts differentiate into secretory ameloblasts, they acquire a special apical structure known as “Tomes’ process.” The secretory ameloblasts with Tomes’ processes produce and secrete a specific set of proteins to form organic enamel matrix, which is then partially replaced by minerals during the secretory stage. The full thickness of enamel is achieved at the end of the secretory stage, and the organic matrix is completely removed and replaced by minerals during the maturation stage. The underlying mesenchyme differentiates into odontoblasts, which form dentin located directly underneath enamel in the crown of a tooth (Balic and Thesleff, 2015).
Mutations in the genes encoding enamel matrix proteins as well as other proteins involved in enamel formation result in a group of inherited enamel defects, known as “Amelogenesis Imperfecta (AI; Smith et al., 2017).” Based on the phenotypic defects of enamel, AI may be classified into hypoplastic and hypomineralized forms (Hu et al., 2007; Smith et al., 2017). Hypoplastic AI is characterized by the formation of a thin but mineralized layer of enamel that results from an insufficient apposition of enamel during the secretory stage of amelogenesis. In contrast, hypomineralized AI is distinguished by the formation of a full thickness but soft layer of enamel caused by a failure in enamel maturation. Hypomineralized AI can be further subclassified into hypomaturation and hypocalcified forms (Hu et al., 2007; Smith et al., 2017). Hypomaturation AI is due to an incomplete removal of the organic enamel matrix proteins that separate adjacent enamel crystals, whereas hypocalcified AI is caused by an insufficient deposition of minerals, particularly calcium ions.
Dentin sialophosphoprotein (DSPP) is a non-collagenous extracellular matrix protein and is a member of the SIBLING (Small integrin-binding ligand N-linked glycoprotein) family (Fisher et al., 2001; Fisher and Fedarko, 2003). It is highly expressed in odontoblasts, but transiently expressed in differentiating ameloblasts, as its transcripts are only observed in presecretory ameloblasts and early secretory ameloblasts during tooth development (D'Souza et al., 1997; Ritchie et al., 1997; Begue-Kirn et al., 1998; MacDougall et al., 1998; Bleicher et al., 1999). DSPP is synthesized as a single large protein, which is subsequently processed into an amino-terminal fragment called dentin sialoprotein (DSP) and a carboxyl-terminal fragment known as dentin phosphoprotein (DPP; MacDougall et al., 1997; Sun et al., 2010; von Marschall and Fisher, 2010; Zhu et al., 2012). DSP is a proteoglycan containing two glycosaminoglycan chains (Ritchie et al., 1994; Zhu et al., 2010; Yamakoshi et al., 2011), whereas DPP is a highly phosphorylated and very acidic protein (Butler et al., 1983; George et al., 1996; Ritchie and Wang, 1996). In addition, a third cleaved fragment, named “dentin glycoprotein (DGP),” is identified in porcine and is derived from the middle region of DSPP (Yamakoshi et al., 2005).
Mutations in the DSPP gene in humans affect tooth development, resulting in an inheritable autosomal dominant dental disorder. Consistent with its high level of expression in odontoblasts, the primary dental defect associated with DSPP mutations in humans is the formation of various types of abnormal dentin (Kim and Simmer, 2007). Based on the phenotypic differences, the dental defects caused by DSPP mutations may be classified into dentinogenesis imperfecta (DGI) Type II (OMIM #125490), characterized by pulp chamber obliteration, DGI Type III (OMIM #125500), featured by pulp chamber enlargement and thinner dentin, and dentin dysplasia (DD) Type II (OMIM #125420), which manifests relatively mild dental defects (Shields et al., 1973; MacDougall et al., 2006; Kim and Simmer, 2007). Similarly, Dspp ablation in mice causes a defective dentin mineralization, resulting in a tooth phenotype similar to that observed in human DGI type III patients (Sreenath et al., 2003). In addition to dentin, enamel formation may be affected by DSPP mutations in DGI patients (Lee et al., 2011; Wang et al., 2011; Bloch-Zupan et al., 2016; Taleb et al., 2018). However, very limited studies have been done to understand how mutant DSPP proteins interfere with amelogenesis to date.
We previously generated a mouse model (referred to as “DsppP19L/+” mice), that expressed a mutant DSPP, in which the proline residue at position 19 was replaced by a leucine residue (p.P19L; Liang et al., 2019). The proline residue at position 19 in mouse DSPP is the second amino acid residue from the signal peptide cleavage site, and it corresponds to the proline residue P17 in human DSPP. Our findings demonstrated that DsppP19L/+ and DsppP19L/P19L mice manifested a DGI Type III-like phenotype at younger age, and acquired a DGI Type II-like defect as they grew older, which is similar to the dental phenotype of human patients carrying the corresponding p.P17L mutation (Li et al., 2012; Lee et al., 2013; Porntaveetus et al., 2019). In this study, we reported the ultrastructural changes in enamel as well as the molecular changes in differentiating ameloblasts in DsppP19L/+ and DsppP19L/P19L mice. We showed that the Dspp mutant mice had reduced enamel formation, delayed enamel maturation, and ultrastructural enamel defects; and we demonstrated that such enamel defects may be associated with an accumulation of mutant DSPP protein within the presecretory ameloblasts.
Materials and Methods
Generation of DsppP19L/+ and DsppP19L/P19L Mice
Generation and genotyping of DsppP19L/+ and DsppP19L/P19L mice was described in our previous report (Liang et al., 2019). All mice were maintained on a C57BL/6 genetic background on a 12h light/dark cycle with free access to water and standard pelleted food. Both male and female mice were used for analyses of the enamel phenotypes, as there was no phenotypic difference between sexes for each genotype. All animal procedures were approved by the Institutional Animal Care and Use Committee of Texas A&M University College of Dentistry (Dallas, TX, United States).
Plain X-Ray Radiography and Micro-Computed Tomography
The left mandibles from 2-, 3-, 7-, and 24-week-old Dspp+/+, DsppP19L/+, and DsppP19L/P19L mice were dissected and processed for plain x-ray radiography and/or μCT analyses, as previously described (Bouxsein et al., 2010; Liang et al., 2019). Seven-week-old mouse mandibles were first evaluated using a plain X-ray radiography system (Faxitron MX-20DC 12; Tucson, AZ, United States) to obtain an overall morphological assessment of the mandibular incisors; they were then scanned with a Scanco μCT35 imaging system (Scanco Medical, Brüttisellen, Switzerland) at three regions that contain the mandibular incisor enamel segment 1 (ES1), ES2, and ES3, respectively (Figure 1A). ES1 is a 1-mm-long incisor enamel segment located immediately distal to the distal aspect of the third molar crown, representing the late secretory stage amelogenesis; ES2 is a 1-mm-long incisor enamel segment located right underneath the proximal root of first molar, representing the mid-late maturation stage amelogenesis; ES3 is a 1-mm-long incisor enamel segment that is located below the labial alveolar crest, that is about to erupt into oral cavity, and that has undergone extensive maturation (enamel mineral density reaches its peak). For the morphometric analyses of ES1, ES2, and ES3, the enamel layer was manually delineated to separate enamel from the subjacent dentin; and a threshold of 120 was then chosen to exclude any soft tissues/ameloblasts. The left mandibles from 2-, 3-, and 24-week-old Dspp+/+, DsppP19L/+, and DsppP19L/P19L mice were scanned for mandibular first molars. For three-dimensional (3D) construction and morphometric analyses of mandibular first molars, the whole teeth were outlined, and an appropriate threshold was determined for each age (a threshold of 515 for 2-week-old mice, 563 for 3-week-old mice, and 600 for 24-week-old mice), based on the visual comparisons to include enamel but not adjacent tissues. All morphometric parameters were evaluated using the μCT built-in software (Bouxsein et al., 2010). Data obtained from 3 to 5 independent mice for each group were used for quantitative analysis.
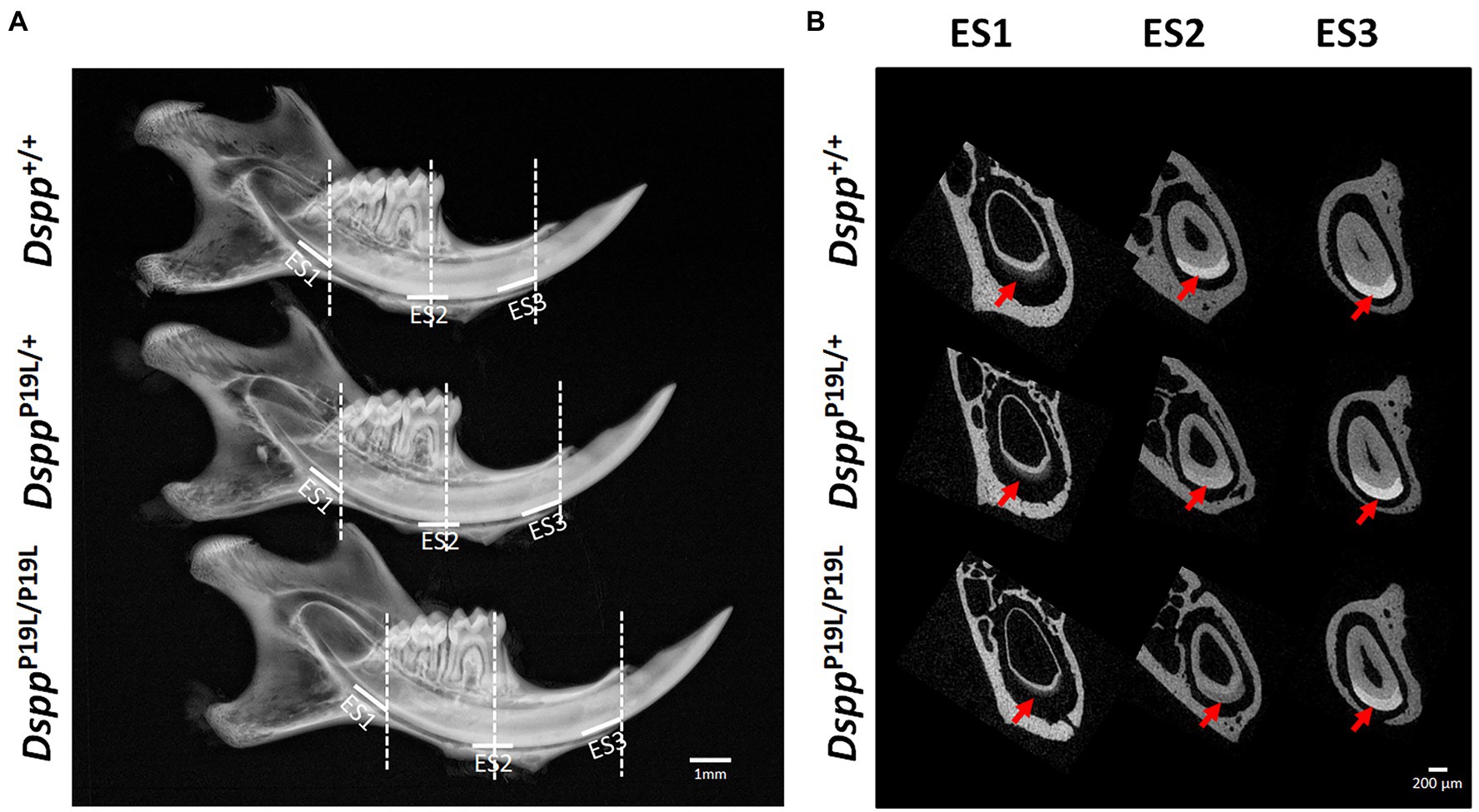
Figure 1. Gross overview of the tooth phenotype of DsppP19L/+ and DsppP19L/P19L mice. (A) Representative plain x-ray radiographic images of the mandibles of 7-week-old wild-type Dspp+/+, heterozygous DsppP19L/+ and homozygous DsppP19L/P19L mice. The white horizontal lines denote the enamel segments, ES1, ES2, and ES3, that were quantified by μCT analyses. ES1 corresponds to the late secretory stage amelogenesis; ES2 corresponds to mid-late maturation stage amelogenesis; and ES3 is the enamel segment that has undergone extensive maturation and is about to erupt. The dashed vertical white lines in (A) mark the locations of reconstructed trans-axial μCT images shown in (B). Red arrows indicate the mandibular incisor enamel in the trans-axial images. Scale bars: 1mm in A; 200μm in B.
Resin-Casted Backscattered and Acid-Etched Scanning Electron Microscopy
The 3-, 7-, and 24-week-old left mandible samples were processed for resin-casted backscattered and acid-etched SEM analysis, as described previously (Gibson et al., 2013; Zhang et al., 2018; Liang et al., 2019). Briefly, the mandible samples were dehydrated in gradient ethanol (from 70 to 100%) and xylene before embedded in methyl methacrylate (MMA). For resin-casted backscattered SEM analysis of the molars, the buccal-lingual sections crossing the middle of the proximal root of mandibular first molars were chosen. The cut surface was polished and dehydrated, followed by gold coating. Scanning was performed in backscattered electronic shadow (BES) mode in a JEOL JSM-6010 LA SEM (JEOL, Japan). Following resin-casted backscattered SEM analysis, the molar sections were further processed for acid-etched SEM analysis. The coating particles were removed, and sample surface was re-polished and etched with 10% phosphoric acid for 7–14s, followed by immersing in 5% sodium hypochlorite for 20min twice. The samples were gold-coated and scanned in secondary electron image (SEI) mode in the same SEM. For acid-etched SEM analysis of 7-week-old mandibular incisors, the mandibles were cross sectioned to obtain sections at the levels of ES2 and ES3, which were subsequently processed for acid-etched SEM analysis as the molar sections. Two independent mice were analyzed for molars or incisors for each genotype of 3-, 7-, and 24-week-old mice.
Sample Processing and Histological Analysis
The right mandibles from 1-week-old Dspp+/+, DsppP19L/+, and DsppP19L/P19L mice were harvested and fixed in freshly prepared 4% paraformaldehyde in diethyl pyrocarbonate (DEPC)-treated 0.1M phosphate-buffered saline (pH 7.4) at 4°C overnight and then decalcified in 15% ethylenediaminetetraacetate (EDTA) solution (pH 7.4) at 4°C for 3days. The decalcified samples were dehydrated, and embedded in paraffin following standard histological procedures. The tissue blocks were cut into serial sagittal sections at a thickness of 5μm, which were used for Hematoxylin and Eosin (H&E) staining, and other histological analyses, as previously described (Zhang et al., 2018; Liang et al., 2019).
In situ Hybridization
In situ hybridization (ISH) was performed to detect DSPP transcripts, as previously described (Meng et al., 2015; Liang et al., 2019). Briefly, RNA probes for mouse DSPP were labeled with digoxigenin (DIG) by using an RNA Labeling Kit (Roche, Indianapolis, IN, United States), according to the manufacturer’s instruction. The DIG-labeled RNA probes were used to hybridize with the DSPP transcripts in the mandibular first molars. After hybridization, the hybridized DIG-labeled RNA probes were detected by an enzyme-linked immunoassay with an alkaline phosphatase-conjugated anti-DIG antibody (1:2000; Roche), and an NBT/BCIP (nitro blue tetrazolium/5-bromo-4-chloro-3-indolyl-phosphate) substrate (Roche, Mannheim, Germany). The sections were counterstained with nuclear fast red (Sigma, Saint Louis, MO, United States). At least three individual samples were analyzed.
Immunohistochemistry
Immunohistochemistry (IHC) was carried out to assess DSP/DSPP, amelogenin (AMEL), and ameloblastin (AMBN) proteins, as previously described (Meng et al., 2015; Li et al., 2019; Liang et al., 2019). Briefly, sections were incubated with a rabbit anti-DSP polyclonal antibody (recognizing both DSP and full-length DSPP), a mouse monoclonal antibody raised against the full-length human AMEL (Santa Cruz Biotechnology; 1:500), or a rabbit polyclonal antibody generated against the C-terminus of mouse AMBN (Santa Cruz Biotechnology; 1:800), followed by incubating in a biotinylated anti-rabbit IgG or anti-mouse IgG secondary antibody (1:200, Vector Laboratories, Burlingame, CA, United States). The immunostaining signals were visualized using the DAB (3,3′-diaminobenzidine) kit (Vector Laboratories), according to the manufacturer’s instructions. The sections were counterstained with methyl green (Sigma). At least three individual samples were analyzed. The same concentrations of nonimmune rabbit IgG or mouse IgG were used to replace the polyclonal or monoclonal antibodies as negative controls.
Statistical Analysis
One-way ANOVA was conducted to compare the differences among three groups. If significant differences were found by One-way ANOVA, Bonferroni method was used as post hoc. The quantified results were represented as mean±SD. p<0.05 was considered statistically significant.
Results
Delayed Incisor Enamel Maturation in DsppP19L/+ and DsppP19L/P19L Mice
Rodent incisors continuously grow and erupt, so that different stages of amelogenesis can be evaluated in the same incisor (Robinson et al., 1977; Smith and Nanci, 1989; Smith et al., 2011; Schmitz et al., 2014). We first examined the mandibular incisor phenotype of 7-week-old DsppP19L/+ and DsppP19L/P19L mice. Plain x-ray radiography demonstrated that DsppP19L/+ and DsppP19L/P19L mice had no apparent difference in the overall appearance of the mandibular incisors, compared to the age-matched Dspp+/+ control mice (Figure 1A). The trans-axial μCT images demonstrated that DsppP19L/+ and DsppP19L/P19L mice had reduced radiopacity in enamel, compared to Dspp+/+ mice at the late secretory stage (ES1) and at the mid-late maturation stage (ES2; Figure 1B). Such reduced radiopacity was more prominent at the mid-late maturation stage and was also more severe in DsppP19L/P19L mice (Figure 1B). However, for the enamel segment (ES3), which was immediately before eruption, DsppP19L/+ and DsppP19L/P19L mice achieved a radiopacity that was very close to that of Dspp+/+ mice (Figure 1B). Quantitative μCT analyses showed that DsppP19L/P19L mice had a significant reduction in enamel volume at all three stages of amelogenesis examined, whereas DsppP19L/+ mice only displayed reduced enamel volume at the level of ES2, compared to Dspp+/+ control mice (Table 1). In addition, both DsppP19L/+ and DsppP19L/P19L mice had significantly reduced enamel density at the level of ES2, compared to Dspp+/+ mice (Table 1). We also analyzed the ultrastructural changes in the mandibular incisor enamel. Acid-etched scanning electron microscopy (SEM) analyses demonstrated that, at the mid-late maturation stage of amelogenesis, the wild-type mice already had apparent decussating pattern of enamel rods, whereas only minute particulates were found in DsppP19L/+ mice, and no discernible structures in DsppP19L/P19L mice (Figure 2A). However, by the stage that the incisor enamel is about to erupt into the oral cavity, distinct decussating enamel rods were evident in DsppP19L/+ mice, but only poorly-defined enamel rods were revealed in DsppP19L/P19L mice (Figure 2B). Taken together, these results demonstrated that DsppP19L/+ and DsppP19L/P19L mice showed reduced incisor enamel formation, and delayed enamel maturation.
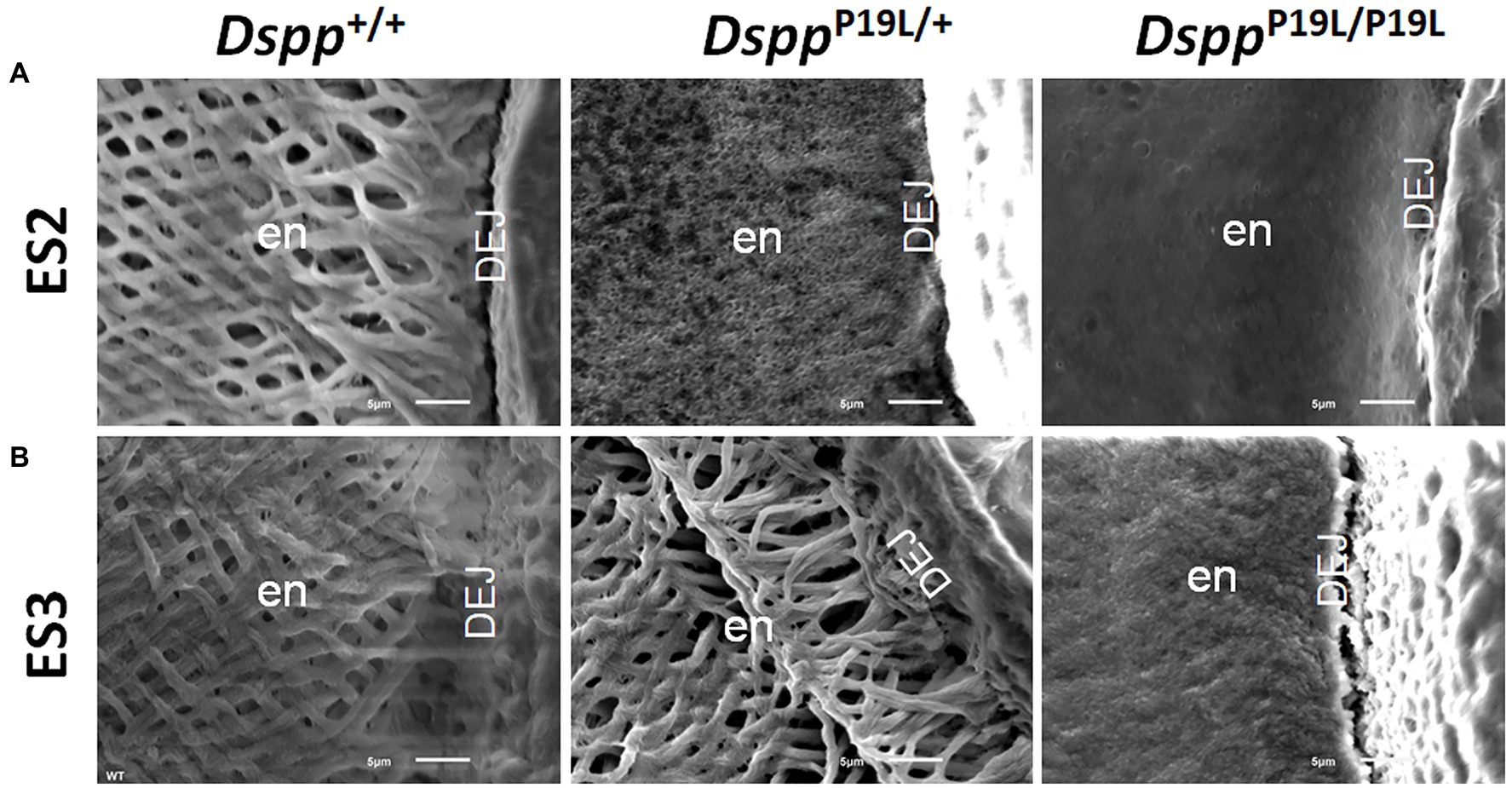
Figure 2. Acid-etched scanning electron microscopy (SEM) analyses of the mandibular incisors. Shown are the acid-etched SEM images of the mandibular incisors of 7-week-old wild-type Dspp+/+, heterozygous DsppP19L/+ and homozygous DsppP19L/P19L mice. All images are the cross sections obtained at the levels of ES2 (A) and ES3 (B). Note that the enamel from Dspp+/+ mice showed decussating pattern of enamel rods at both ES2 and ES3 levels. In DsppP19L/+ mouse incisors, even though only minute particulates were observed at the level of ES2, distinct decussating enamel rods were present at the level of ES3. In the DsppP19L/P19L mouse incisors, there was a complete absence of any discernible structures at the level of ES2, and only poorly-defined enamel rods were found at the level of ES3. en, enamel and DEJ, dentinoenamel junction. Scale bars: 5μm.
Reduced Molar Enamel Formation and Accelerated Molar Enamel Attrition in DsppP19L/+ and DsppP19L/P19L Mice
Next, we analyzed the mandibular first molars (M1) of DsppP19L/+ and DsppP19L/P19L mice at postnatal 2weeks (M1 at the beginning of eruption phase before it is exposed to oral cavity; Komiyama et al., 2013), 3weeks (M1 at the beginning of post-eruptive phase when it reaches the occlusal plane; Komiyama et al., 2013), and 24weeks (when M1 has undergone substantial attrition). In contrast to incisors, rodent molars have limited growth as no more enamel will be formed once enamel formation is complete. Reconstructed 3D μCT images showed that the enamel was poorly formed in DsppP19L/+ and DsppP19L/P19L mice, particularly in DsppP19L/P19L mice, at the age of 2weeks before the teeth were exposed to oral cavity (Figure 3A). Moreover, DsppP19L/P19L mice showed a more severe loss of enamel with age after tooth eruption, compared with age-matched Dspp+/+ and DsppP19L/+ mice (Figures 3B,C). Quantitative μCT analyses confirmed that DsppP19L/+ and DsppP19L/P19L mice had a significant reduction in both enamel volume and enamel density of the mandibular first molars at the age of 2weeks, compared to Dspp+/+ mice (Table 2). The enamel volumes and densities of DsppP19L/+ and DsppP19L/P19L mouse molars remained significantly lower than those of age-matched Dspp+/+ mice when the teeth reached occlusion at the age of 3weeks (Table 2). Even though all three groups of mice showed reduced molar enamel volumes with age due to attrition, DsppP19L/+ and DsppP19L/P19L mouse molars apparently showed a more severe loss of enamel volume (with a loss of 15.74 and 33.48% total enamel volume, respectively) from the age of 3 to 24weeks, compared to the Dspp+/+ mice (with a loss of 7.48% total enamel volume; Table 2). In contrast to enamel volume, the molar enamel densities increased with age among all three groups of mice; however, the enamel densities of DsppP19L/+ and DsppP19L/P19L mouse molars remained significantly lower, compared to that of Dspp+/+ mice at the age 24weeks (Table 2). These data suggest that the molar enamel was less formed and hypomineralized in DsppP19L/+ and DsppP19L/P19L mice and was subject to rapid attrition with age after teeth reach occlusion.
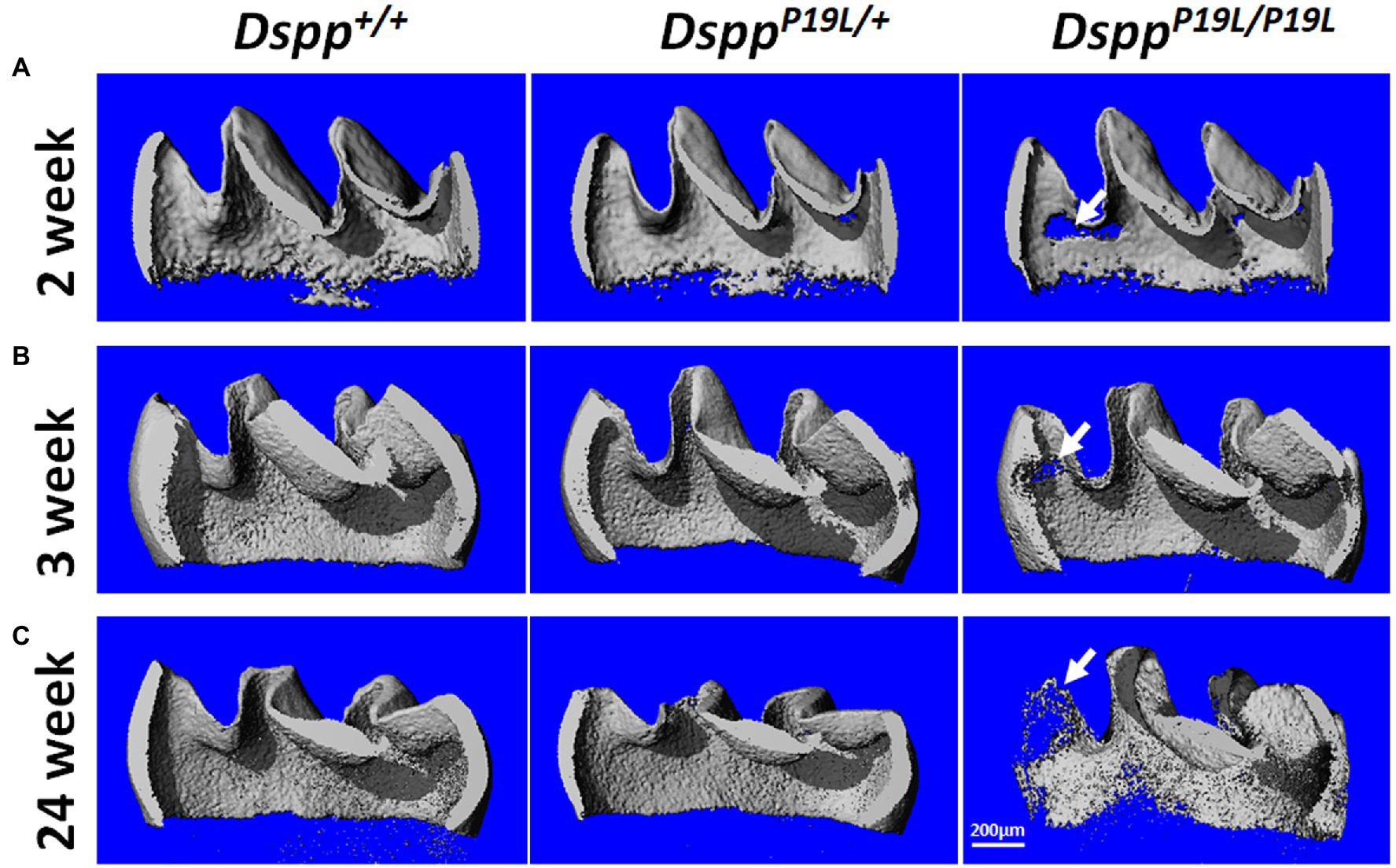
Figure 3. Micro-Computed Tomography (μCT) analyses of the mandibular first molars. Shown are the representative 3D reconstructed μCT images (sagittal sections) of enamel of the mandibular first molars of 2-week-old (A), 3-week-old (B), and 24-week-old (C) wild-type Dspp+/+, heterozygous DsppP19L/+, and homozygous DsppP19L/P19L mice. The enamel was poorly formed in DsppP19L/+ and DsppP19L/P19L mice, particularly in DsppP19L/P19L mice (arrows), at the age of 2weeks. There was also a severe loss of enamel in the DsppP19L/P19L mice (arrows) with age, in comparison to age-matched Dspp+/+ and DsppP19L/ mice. Scale bar: 200μm.
Ultrastructural Changes in Molar Enamel in DsppP19L/+ and DsppP19L/P19L Mice
We then examined the ultrastructural changes in the molar enamel by backscattered and acid-etched SEM analyses, as previously described (Liang et al., 2019). Backscattered SEM analysis demonstrated that 3-week-old Dspp+/+ mice showed a decussating pattern of enamel rods in the inner enamel, a parallel pattern in the outer enamel and were rod-free in the superficial enamel (Figure 4A). Three-week-old DsppP19L/+ mice showed a similar organization of enamel rods as age-matched Dspp+/+ mice, but there was a complete lack of clearly defined enamel rods in 3-week-old DsppP19L/P19L mice (Figure 4A). By 24weeks, the decussating pattern enamel rods remained in Dspp+/+ mice (Figure 4B). However, the enamel rod boundaries became blurred in DsppP19L/+ mice, whereas only poorly-defined enamel rods were observed in DsppP19L/P19L mice (Figure 4B). Acid-etched SEM analyses revealed that DsppP19L/+ mice showed an acid-etching pattern in enamel that was similar to that of the wild-type mice, at the age of 3weeks (Figure 5A). At the age of 24weeks, Dspp+/+ mice presented an acid-etching pattern, with the cross-sectioned enamel rods preferentially removed by acid (Figure 5B). Although DsppP19L/+ mice displayed a similar pattern, the cross-sectioned enamel rods were not as well-defined as the wild-type mice (Figure 5B). There were no recognizable enamel rods in DsppP19L/P19L mice at both ages (Figures 5A,B). In addition, the dentinoenamel junction (DEJ) appeared to be altered in DsppP19L/P19L mice, compared to age-matched DsppP19L/+ and DsppP19L/+ mice (Figures 5A,B). Taken together, these findings support that the molar enamel was poorly formed in DsppP19L/+ and DsppP19L/P19L mice, particularly in DsppP19L/P19L mice.
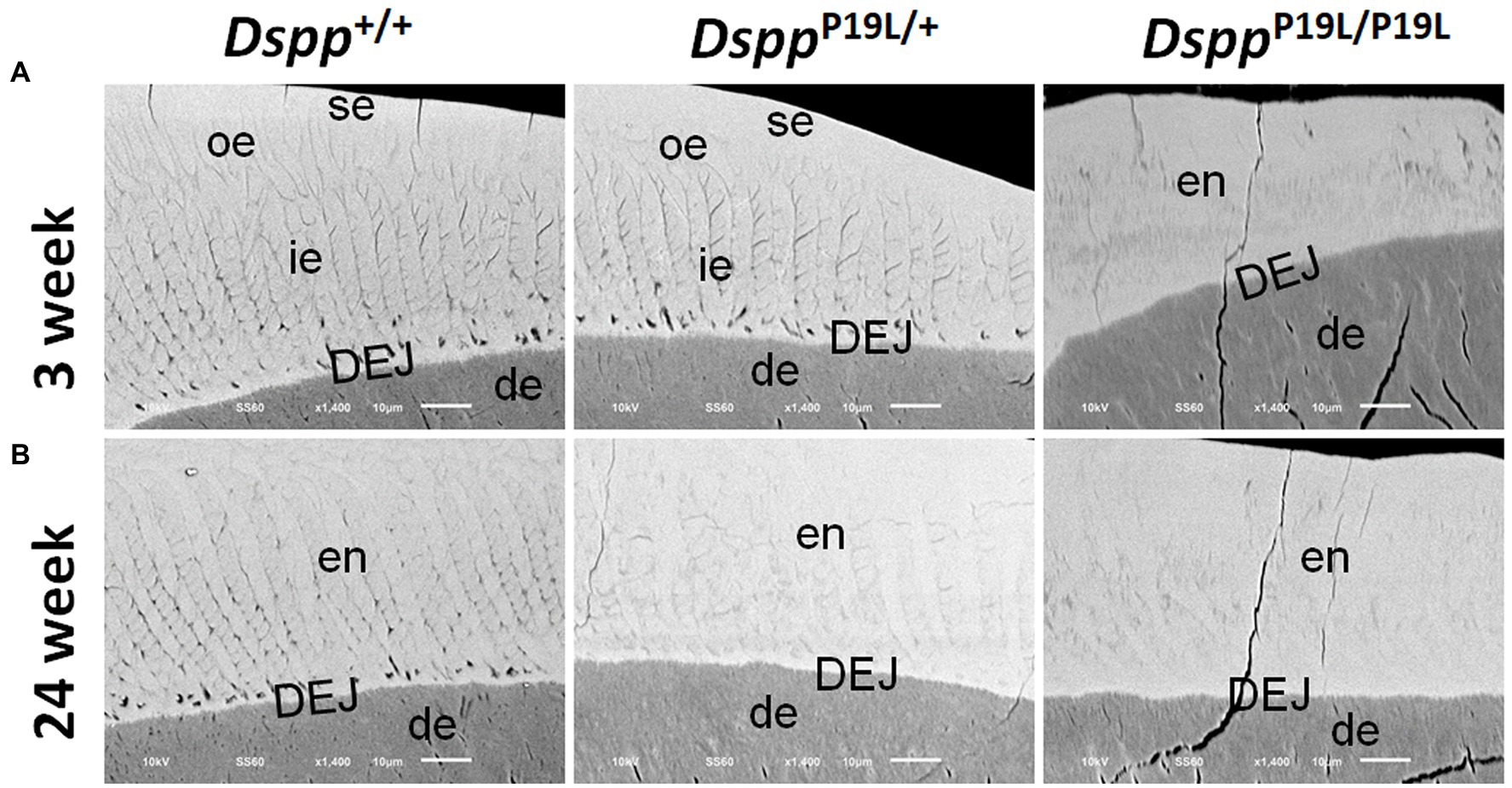
Figure 4. Backscattered SEM analyses of the mandibular first molars. Shown are the backscattered SEM images of the mandibular first molars of 3- (A) and 24- (B) week-old wild-type Dspp+/+, heterozygous DsppP19L/+ and homozygous DsppP19L/P19L mice. All images are the longitudinal sections and buccal sides of the mandibular first molars. Three-week-old Dspp+/+ mice showed a decussating pattern of enamel rods in the inner enamel (ie), a parallel pattern in the outer enamel (ore), and were rod-free in the superficial enamel (se). Three-week-old DsppP19L/+ mice showed a similar arrangement of enamel rods as the age-matched wild-type mice, but there were no discernable enamel rods in 3-week-old DsppP19L/P19L mice. By 24weeks, the decussating enamel rods remain in Dspp+/+ mice. However, the enamel rod boundaries became blurred in DsppP19L/+ mice, whereas only poorly-defined enamel rods were observed in DsppP19L/P19L mice. ie, inner enamel; oe, outer enamel; en, enamel; se, superficial enamel; de, dentin; and DEJ, dentinoenamel junction. Scale bars: 10μm.
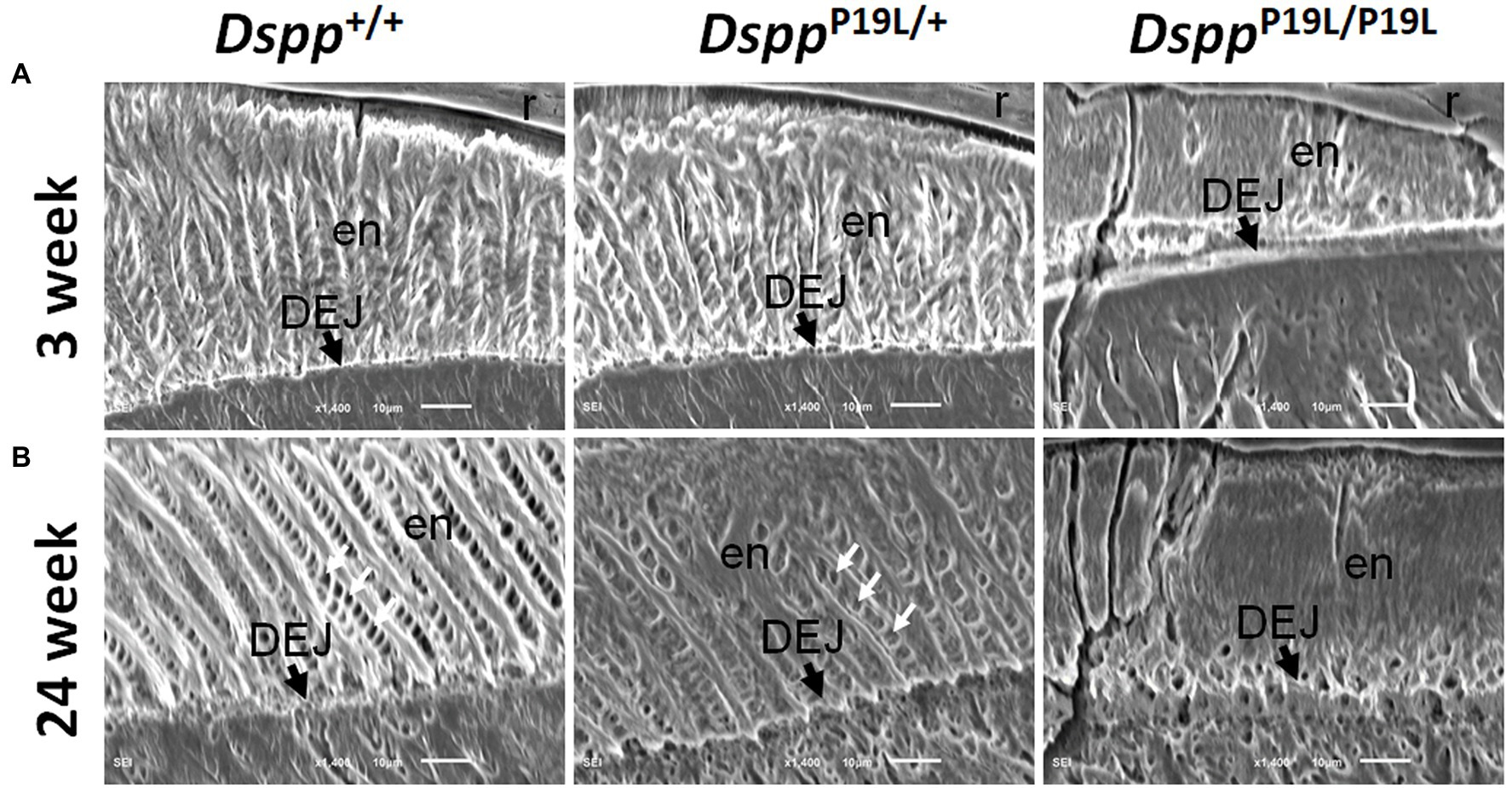
Figure 5. Acid-etched SEM analyses of the mandibular first molars. Shown are the acid-etched SEM images of the mandibular first molars of 3- (A) and 24- (B) week-old wild-type Dspp+/+, heterozygous DsppP19L/+ and homozygous DsppP19L/P19L mice. All images are the longitudinal sections and buccal sides of the mandibular first molars. At the age of 3weeks, DsppP19L/+ mice showed a similar acid-etching pattern in enamel (en) as the wild-type mice. At the age of 24weeks, Dspp+/+ mice presented an acid-etching pattern, with the cross-sectioned enamel rods (pointed by white arrows) preferentially removed by acid; although DsppP19L/+ mice displayed a similar pattern, the cross-sectioned enamel rods (pointed by white arrows) were not as well-defined as the wild-type mice. There were no recognizable enamel rods in DsppP19L/P19L mice at both ages. The dentinoenamel junction (DEJ) appeared to be different in DsppP19L/P19L mice, compared to age-matched Dspp+/+ and DsppP19L/+ mice. en, enamel; DEJ, dentinoenamel junction; and r, resin. Scale bars: 10μm.
An Accumulation of DSPP Protein Within the Presecretory Ameloblasts in DsppP19L/+ and DsppP19L/P19L Mice
To determine the molecular changes that occurred in the differentiating ameloblasts, we examined the expression of the Dspp gene at both mRNA and protein levels in the mandibular first molars (pre-eruptive phase) of 7-day-old DsppP19L/+ and DsppP19L/P19L mice. Histologic analyses showed that the secretory ameloblasts in DsppP19L/+ and DsppP19L/P19L mice appeared to be similar in morphology to those in the Dspp+/+ mice (Figure 6A). In situ hybridization demonstrated that a low level of DSPP mRNAs was observed in the presecretory ameloblasts in Dspp+/+ mice (Figure 6B). Unlike the strong and sustained Dspp expression in the opposing odontoblasts, the Dspp expression abruptly diminished as the presecretory ameloblasts differentiated into secretory ameloblasts (Figure 6B). Compared to Dspp+/+ mice, there was a moderate decrease in DSPP mRNA signal in both presecretory ameloblasts and odontoblasts in DsppP19L/+ mice and a great decrease in DsppP19L/P19L mice (Figure 6B). In contrast to the changes in DSPP mRNA, immunohistochemical signals for DSP/DSPP protein were weakly detected in the presecretory ameloblasts and odontoblasts in Dspp+/+ mice (Figure 6C). There was a moderate increase in the DSP/DSPP signals in both presecretory ameloblasts and odontoblasts in DsppP19L/+ mice, and a strong increase in DsppP19L/P19L mice (Figure 6C). Nevertheless, immunohisto chemistry showed that there was no obvious difference in the level and distribution of two secretory stage ameloblast markers, AMEL and AMBN, in the enamel matrices among all three groups of mice (Figures 7A,B). These results suggest that the defective enamel may be associated with an accumulation of the mutant P19L-DSPP protein within the presecretory ameloblasts in DsppP19L/+ and DsppP19L/P19L mice.
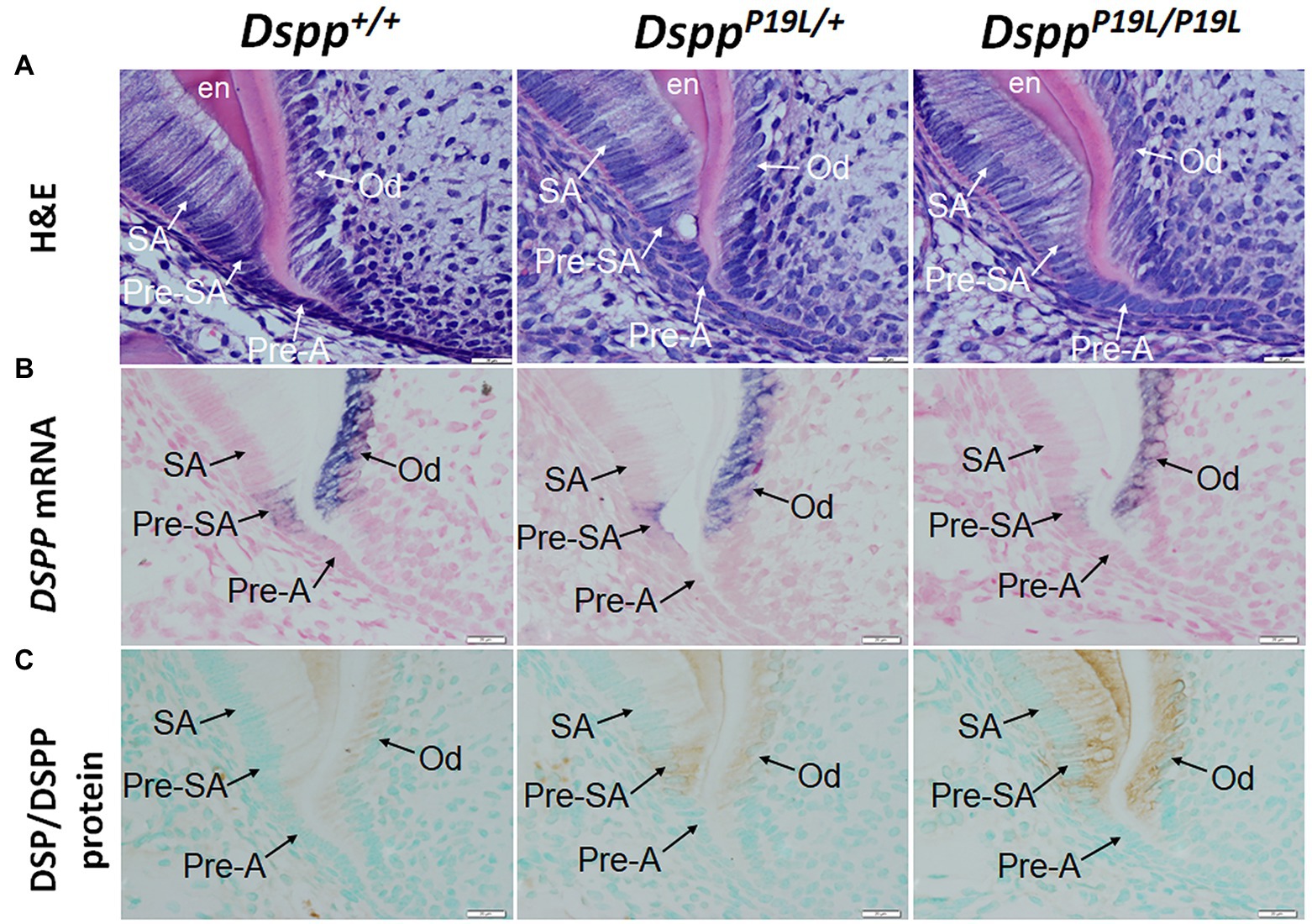
Figure 6. Intracellular accumulation of dentin sialophosphoprotein (DSPP) protein in presecretory ameloblasts. All images are the sagittal section and distal cervical region of the mandibular first molars of 1-week-old wild-type Dspp+/+, heterozygous DsppP19L/+ and homozygous DsppP19L/P19L mice. (A) H&E staining. (B) In situ hybridization analysis of DSPP mRNA (signal in purple). DsppP19L/+ mice had a moderate decrease, whereas homozygous DsppP19L/P19L mice showed a marked decrease in DSPP mRNA level in both odontoblasts and presecretory ameloblasts, compared to the wild-type mice. (C) Immunohistochemical staining of DSP/DSPP protein (signal in brown). The immunohistochemical staining signals for DSP/DSPP protein were weakly detected in the presecretory ameloblasts and odontoblasts in the wild-type mice. There was a moderate increase in the DSP/DSPP signals in both presecretory ameloblasts and odontoblasts in DsppP19L/+ mice, and a strong increase in DsppP19L/P19L mice. SA, secretory ameloblasts; Pre-SA, presecretory ameloblast, Pre-A, preameloblasts; OD, odontoblasts; and en, enamel. Scale bars: 20μm in A–I.
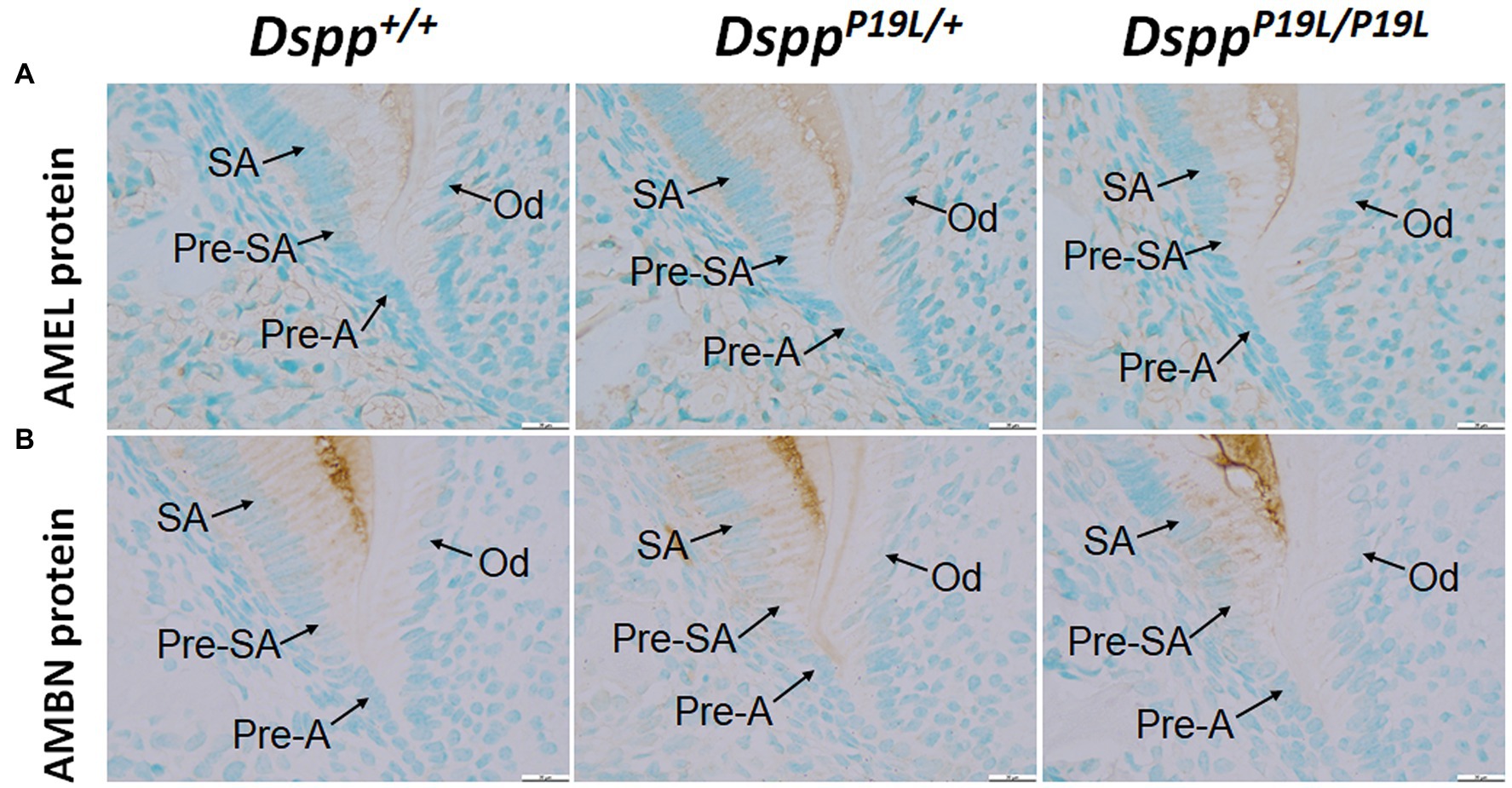
Figure 7. Immunohistochemical staining of amelogenin (AMEL) and ameloblastin (AMBN). All images are the sagittal section and distal cervical region of the mandibular first molars of 1-week-old wild-type Dspp+/+, heterozygous DsppP19L/+ and homozygous DsppP19L/P19L mice. (A) Immunohistochemical staining of AMEL (signal in brown). (B) Immunohistochemical staining of AMBN (signal in brown). All three groups of mice showed a similar distribution and intensity of AMEL and AMBN immunostaining signals in the enamel matrices. SA, secretory ameloblasts; Pre-SA, presecretory ameloblast, Pre-A, preameloblasts; and OD, odontoblasts. Scale bars: 20μm in A–F.
Discussion
The enamel defects are less-well studied in human DGI patients associated with DSPP mutations because of rapid enamel attrition in human patients and lack of an appropriate animal model. We previously generated a mouse model that expresses P19L-DSPP – a mouse equivalent of human mutant P17L-DSPP, and reported the dentin/pulp phenotypes of DsppP19L/+ and DsppP19L/P19L mice. In this study, we presented the ultrastructural enamel defects of these mice as well as the molecular changes in the differentiating ameloblasts and demonstrated that the enamel defects may be associated with an accumulation of the mutant P19L-DSPP protein within the presecretory ameloblasts.
The teeth often undergo rapid and severe attrition in DGI patients associated with DSPP mutations. It is generally believed that enamel readily chips off from the underlying defective dentin and/or abnormal DEJ after tooth eruption, thereby exposing the softer and malformed dentin to rapid attrition (Wright and Gantt, 1985; Kim et al., 2004; MacDougall et al., 2006; Hart and Hart, 2007; Kida et al., 2009; Min et al., 2014; Porntaveetus et al., 2018). However, it has also become evident that in some cases, the enamel itself may have intrinsic developmental defects (Lee et al., 2011; Wang et al., 2011; Bloch-Zupan et al., 2016; Taleb et al., 2018), which may be a direct contributing factor to rapid tooth wear observed in DGI patients. Consistently, we previously reported that both DsppP19L/+ and DsppP19L/P19L mice showed significantly reduced thickness of dental pulp chamber roof dentin and reduced dentin mineral densities (Liang et al., 2019), suggesting that the defective dentin may contribute to the accelerated tooth attrition as it cannot provide adequate support to the overlying enamel. In this study, we further demonstrated that DsppP19L/+ and DsppP19L/P19L mice displayed delayed enamel maturation and reduced enamel mineral densities, corroborating the intrinsic defects in the enamel. Similar to human DGI patients, the malformed enamel experienced accelerated attrition after the teeth erupted in DsppP19L/+ and DsppP19L/P19L mice. These human and animal genetic studies strongly demonstrate that DSPP mutations can cause both enamel and dentin defects, which may together contribute to the severe tooth attrition seen in DGI patients. However, it is of note that some DSPP mutations may have no or negligible effects on enamel formation (McKnight et al., 2008; Nieminen et al., 2011; Verdelis et al., 2016; Yang et al., 2016; Park et al., 2020).
During tooth development, Dspp is transiently expressed by the presecretory ameloblasts (D'Souza et al., 1997; Ritchie et al., 1997; Begue-Kirn et al., 1998; MacDougall et al., 1998; Bleicher et al., 1999). Thereby, it has been speculated that the enamel defects may be due to ameloblast pathology caused by mutant DSPP proteins (Lee et al., 2011; Wang et al., 2011). Indeed, we found that even though the level of DSPP mRNA was markedly reduced in the presecretory ameloblasts, and the mutant P19L-DSPP protein was accumulated within the cells in both DsppP19L/+ and DsppP19L/P19L mice. We have previously reported that the mutant P19L-DSPP protein was accumulated in the endoplasmic reticulum when it was transiently expressed in in vitro cultured odontoblast-like cells (Liang et al., 2019). Therefore, it is most likely that the mutant P19L-DSPP protein was also retained in the ER within the presecretory ameloblasts. DSPP is highly acidic as it contains a large number of aspartate and glutamate residues (Prasad et al., 2010). If the full-length DSPP was accumulated in the ER, the highly acidic DSPP could incur pathogenic ER stress. It has been shown that ER stress and its associated unfolded protein response (UPR) is involved in AI caused by mutations in the genes that encode enamel matrix proteins (Brookes et al., 2014, 2017a,b; Hetz et al., 2020). Therefore, it is reasonable to postulate that the enamel defects observed in DsppP19L/+ and DsppP19L/P19L mice may be associated with ER stress and its activated UPR caused by mutant P19L-DSPP protein.
It is important to note that even though the mutant DsppP19L allele, like the wild-type Dspp allele, was only transiently expressed in the presecretory ameloblasts, it caused an irreversible damage on ameloblast differentiation and function. First, we demonstrated that the function of the secretory-stage ameloblasts was affected as evidenced by reduced enamel formation, even though the levels of AMEL and AMBN in the enamel matrices appeared to be normal in DsppP19L/+ and DsppP19L/P19L mice. Secondly, the function of the maturation-stage ameloblasts was also compromised as reflected by delayed enamel maturation in DsppP19L/+ and DsppP19L/P19L mice. These findings further emphasize the notion that the presecretory ameloblasts are very sensitive to their intracellular and extracellular disturbances so that any pathological conditions may affect their function and continual differentiation and cause developmental enamel defects (Lee et al., 2011; Wang et al., 2011). Further studies are needed to determine how the mutant P19L-DSPP protein exerted its negative effect on the presecretory ameloblasts, and their subsequent differentiation into secretory stage ameloblasts and maturation stage ameloblasts.
DsppP19L/+ and DsppP19L/P19L mice manifested an enamel phenotype that is quite different from Dspp-null mice. It has been reported that even though Dspp-null mice had earlier onset of enamel matrix deposition in mandibular incisors, they did not exhibit any major phenotypic abnormalities in mature enamel with regards to enamel structural organization, mineral density, or hardness (Verdelis et al., 2016). Nevertheless, Dspp-null mice did show a defect in DEJ, which might be due to either the loss of DSPP function in the formation of this dentin and enamel interface or the earlier onset of enamel deposition (Verdelis et al., 2016). In contrast, DsppP19L/+ and DsppP19L/P19L mice not only displayed an abnormal DEJ as evidenced by acid-etched SEM analysis, they also had reduced enamel formation as well as intrinsic structural defects in enamel. Moreover, unlike Dspp heterozygous mice who did not show any apparent dental phonotype (Sreenath et al., 2003; Suzuki et al., 2009; Verdelis et al., 2016), our current and previous studies showed that the DsppP19L/+ mice developed a similar, though less severe, tooth phenotype as DsppP19L/P19L mice (Liang et al., 2019). The phenotypic difference between the Dspp mutant mice and Dspp-null mice support that the enamel phenotypes of DsppP19L/+ and DsppP19L/P19L mice were not simply caused by a loss of DSPP function in the presecretory ameloblasts.
Moreover, previous studies have shown that ectopic overexpression of DSP in secretory ameloblasts in mice resulted in an increase in enamel hardness, whereas similar overexpression of DPP weakened enamel and made it more prone to wear (Paine et al., 2005; White et al., 2007). Yet, it is very unlikely that the enamel phenotypes of DsppP19L/+ and DsppP19L/P19L mice were caused by ectopic overexpression of DSP or DPP. First, we have demonstrated that the mutant DsppP19L allele, like the wild-type Dspp allele, was only transiently expressed in the presecretory ameloblasts. Secondly, our previous studies have demonstrated that although the secretion of P19L-DSPP was impaired, Western-blotting analysis of the total proteins extracted from the dental pulps and dentin matrices of first molars of DsppP19L/P19L mice as well as the proteins from the conditioned media harvested from cells transfected with the mutant P19L-DSPP-expressing construct revealed that the mutant P19L-DSP/DSPP proteins showed a similar migrating pattern as the normal DSP/DSPP proteins, suggesting that the secreted mutant and normal DSPP proteins were subject to similar posttranslational modifications and proteolytic processing (Liang et al., 2019). Therefore, it is reasonable to exclude the possibility that the enamel phenotypes of the Dspp mutant mice were caused by a gain of DSP or DPP function in the presecretory/secretory ameloblasts.
In summary, we have demonstrated that DSPP mutations could affect enamel formation and cause severe intrinsic enamel defects. Future studies are warranted to determine the molecular pathogenesis underlying the enamel defects associated with the mutant P19L-DSPP protein.
Data Availability Statement
The original contributions presented in this study are included in the article/supplementary material; further inquiries can be directed to the corresponding author.
Ethics Statement
The animal study was reviewed and approved by the Institutional Animal Care and Use Committee of Texas A&M University College of Dentistry.
Author Contributions
TL and QX contributed to design, data acquisition, analysis and interpretation, drafted, and critically revised the manuscript. HZ and SW contributed to data acquisition, analysis, interpretation, drafted, and critically revised the manuscript. TD and CQ contributed to interpretation, drafted, and critically revised the manuscript. YL contributed to conception, design, interpretation, drafted, and critically revised the manuscript. All authors approved the final version of the submitted manuscript and agree to be accountable for all aspects of the work.
Funding
This work was supported by National Institute of Dental & Craniofacial Research (NIDCR) grant DE027345.
Conflict of Interest
The authors declare that the research was conducted in the absence of any commercial or financial relationships that could be construed as a potential conflict of interest.
Publisher’s Note
All claims expressed in this article are solely those of the authors and do not necessarily represent those of their affiliated organizations, or those of the publisher, the editors and the reviewers. Any product that may be evaluated in this article, or claim that may be made by its manufacturer, is not guaranteed or endorsed by the publisher.
References
Balic, A., and Thesleff, I. (2015). Tissue interactions regulating tooth development and renewal. Curr. Top. Dev. Biol. 115, 157–186. doi: 10.1016/bs.ctdb.2015.07.006
Begue-Kirn, C., Krebsbach, P. H., Bartlett, J. D., and Butler, W. T. (1998). Dentin sialoprotein, dentin phosphoprotein, enamelysin and ameloblastin: tooth-specific molecules that are distinctively expressed during murine dental differentiation. Eur. J. Oral Sci. 106, 963–970. doi: 10.1046/j.0909-8836.1998.eos106510.x
Bleicher, F., Couble, M. L., Farges, J. C., Couble, P., and Magloire, H. (1999). Sequential expression of matrix protein genes in developing rat teeth. Matrix Biol. 18, 133–143. doi: 10.1016/S0945-053X(99)00007-4
Bloch-Zupan, A., Huckert, M., Stoetzel, C., Meyer, J., Geoffroy, V., Razafindrakoto, R. W., et al. (2016). Detection of a novel DSPP mutation by NGS in a population isolate in Madagascar. Front. Physiol. 7:70. doi: 10.3389/fphys.2016.00304
Bouxsein, M. L., Boyd, S. K., Christiansen, B. A., Guldberg, R. E., Jepsen, K. J., and Muller, R. (2010). Guidelines for assessment of bone microstructure in rodents using micro-computed tomography. J. Bone Miner. Res. 25, 1468–1486. doi: 10.1002/jbmr.141
Brookes, S. J., Barron, M. J., Boot-Handford, R., Kirkham, J., and Dixon, M. J. (2014). Endoplasmic reticulum stress in amelogenesis imperfecta and phenotypic rescue using 4-phenylbutyrate. Hum. Mol. Genet. 23, 2468–2480. doi: 10.1093/hmg/ddt642
Brookes, S. J., Barron, M. J., Dixon, M. J., and Kirkham, J. (2017a). The unfolded protein response in amelogenesis and enamel pathologies. Front. Physiol. 8:653. doi: 10.3389/fphys.2017.00653
Brookes, S. J., Barron, M. J., Smith, C. E. L., Poulter, J. A., Mighell, A. J., Inglehearn, C. F., et al. (2017b). Amelogenesis imperfecta caused by N-terminal enamelin point mutations in mice and men is driven by endoplasmic reticulum stress. Hum. Mol. Genet. 26, 1863–1876. doi: 10.1093/hmg/ddx090
Butler, W. T., Bhown, M., Dimuzio, M. T., Cothran, W. C., and Linde, A. (1983). Multiple forms of rat dentin phosphoproteins. Arch. Biochem. Biophys. 225, 178–186. doi: 10.1016/0003-9861(83)90021-8
D'Souza, R. N., Cavender, A., Sunavala, G., Alvarez, J., Ohshima, T., Kulkarni, A. B., et al. (1997). Gene expression patterns of murine dentin matrix protein 1 (Dmp1) and dentin sialophosphoprotein (DSPP) suggest distinct developmental functions in vivo. J. Bone Miner. Res. 12, 2040–2049. doi: 10.1359/jbmr.1997.12.12.2040
Fisher, L. W., and Fedarko, N. S. (2003). Six genes expressed in bones and teeth encode the current members of the SIBLING family of proteins. Connect. Tissue Res. 44: (Suppl. 1), 33–40. doi: 10.1080/03008200390152061
Fisher, L. W., Torchia, D. A., Fohr, B., Young, M. F., and Fedarko, N. S. (2001). Flexible structures of SIBLING proteins, bone sialoprotein, and osteopontin. Biochem. Biophys. Res. Commun. 280: 460–465. doi: 10.1006/bbrc.2000.4146
George, A., Bannon, L., Sabsay, B., Dillon, J. W., Malone, J., Veis, A., et al. (1996). The carboxyl-terminal domain of phosphophoryn contains unique extended triplet amino acid repeat sequences forming ordered carboxyl-phosphate interaction ridges that may be essential in the biomineralization process. J. Biol. Chem. 271, 32869–32873. doi: 10.1074/jbc.271.51.32869
Gibson, M. P., Zhu, Q., Wang, S., Liu, Q., Liu, Y., Wang, X., et al. (2013). The rescue of dentin matrix protein 1 (DMP1)-deficient tooth defects by the transgenic expression of dentin sialophosphoprotein (DSPP) indicates that DSPP is a downstream effector molecule of DMP1 in dentinogenesis. J. Biol. Chem. 288, 7204–7214. doi: 10.1074/jbc.M112.445775
Hart, P. S., and Hart, T. C. (2007). Disorders of human dentin. Cells Tissues Organs 186, 70–77. doi: 10.1159/000102682
Hetz, C., Zhang, K., and Kaufman, R. J. (2020). Mechanisms, regulation and functions of the unfolded protein response. Nat. Rev. Mol. Cell Biol. 21, 421–438. doi: 10.1038/s41580-020-0250-z
Hu, J. C., Chun, Y. H., Al Hazzazzi, T., and Simmer, J. P. (2007). Enamel formation and amelogenesis imperfecta. Cells Tissues Organs 186, 78–85. doi: 10.1159/000102683
Kida, M., Tsutsumi, T., Shindoh, M., Ikeda, H., and Ariga, T. (2009). De novo mutation in the DSPP gene associated with dentinogenesis imperfecta type II in a Japanese family. Eur. J. Oral Sci. 117, 691–694. doi: 10.1111/j.1600-0722.2009.00683.x
Kim, J. W., Nam, S. H., Jang, K. T., Lee, S. H., Kim, C. C., Hahn, S. H., et al. (2004). A novel splice acceptor mutation in the DSPP gene causing dentinogenesis imperfecta type II. Hum. Genet. 115, 248–254. doi: 10.1007/s00439-004-1143-5
Kim, J. W., and Simmer, J. P. (2007). Hereditary dentin defects. J. Dent. Res. 86, 392–399. doi: 10.1177/154405910708600502
Komiyama, Y., Ohba, S., Shimohata, N., Nakajima, K., Hojo, H., Yano, F., et al. (2013). Tenomodulin expression in the periodontal ligament enhances cellular adhesion. PLoS One 8:e60203. doi: 10.1371/journal.pone.0060203
Lee, S. K., Lee, K. E., Hwang, Y. H., Kida, M., Tsutsumi, T., Ariga, T., et al. (2011). Identification of the DSPP mutation in a new kindred and phenotype-genotype correlation. Oral Dis. 17, 314–319. doi: 10.1111/j.1601-0825.2010.01760.x
Lee, S. K., Lee, K. E., Song, S. J., Hyun, H. K., Lee, S. H., and Kim, J. W. (2013). A DSPP mutation causing dentinogenesis imperfecta and characterization of the mutational effect. Biomed. Res. Int. 2013:948181. doi: 10.1155/2013/948181
Li, D., Du, X., Zhang, R., Shen, B., Huang, Y., Valenzuela, R. K., et al. (2012). Mutation identification of the DSPP in a Chinese family with DGI-II and an up-to-date bioinformatic analysis. Genomics 99, 220–226. doi: 10.1016/j.ygeno.2012.01.006
Li, L., Saiyin, W., Zhang, H., Wang, S., Xu, Q., Qin, C., et al. (2019). FAM20A is essential for amelogenesis, but is dispensable for dentinogenesis. J. Mol. Histol. 50, 581–591. doi: 10.1007/s10735-019-09851-x
Liang, T., Zhang, H., Xu, Q., Wang, S., Qin, C., and Lu, Y. (2019). Mutant dentin sialophosphoprotein causes dentinogenesis imperfecta. J. Dent. Res. 98, 912–919. doi: 10.1177/0022034519854029
MacDougall, M., Dong, J., and Acevedo, A. C. (2006). Molecular basis of human dentin diseases. Am. J. Med. Genet. A 140, 2536–2546. doi: 10.1002/ajmg.a.31359
MacDougall, M., Nydegger, J., Gu, T. T., Simmons, D., Luan, X., Cavender, A., et al. (1998). Developmental regulation of dentin sialophosphoprotein during ameloblast differentiation: a potential enamel matrix nucleator. Connect. Tissue Res. 39, 25–37. doi: 10.3109/03008209809023909
MacDougall, M., Simmons, D., Luan, X., Nydegger, J., Feng, J., and Gu, T. T. (1997). Dentin phosphoprotein and dentin sialoprotein are cleavage products expressed from a single transcript coded by a gene on human chromosome 4. Dentin phosphoprotein DNA sequence determination. J. Biol. Chem. 272, 835–842. doi: 10.1074/jbc.272.2.835
McKnight, D. A., Simmer, J. P., Hart, P. S., Hart, T. C., and Fisher, L. W. (2008). Overlapping DSPP mutations cause dentin dysplasia and dentinogenesis imperfecta. J. Dent. Res. 87, 1108–1111. doi: 10.1177/154405910808701217
Meng, T., Huang, Y., Wang, S., Zhang, H., Dechow, P. C., Wang, X., et al. (2015). Twist1 is essential for tooth morphogenesis and odontoblast differentiation. J. Biol. Chem. 290, 29593–29602. doi: 10.1074/jbc.M115.680546
Min, B., Song, J. S., Lee, J. H., Choi, B. J., Kim, K. M., and Kim, S. O. (2014). Multiple teeth fractures in dentinogenesis imperfecta: a case report. J. Clin. Pediatr. Dent. 38, 362–365. doi: 10.17796/jcpd.38.4.q523456j733642r2
Moradian-Oldak, J. (2012). Protein-mediated enamel mineralization. Front. Biosci. 17, 1996–2023. doi: 10.2741/4034
Nieminen, P., Papagiannoulis-Lascarides, L., Waltimo-Siren, J., Ollila, P., Karjalainen, S., Arte, S., et al. (2011). Frameshift mutations in dentin phosphoprotein and dependence of dentin disease phenotype on mutation location. J. Bone Miner. Res. 26, 873–880. doi: 10.1002/jbmr.276
Paine, M. L., Luo, W., Wang, H. J., Bringas, P. Jr., Ngan, A. Y., Miklus, V. G., et al. (2005). Dentin sialoprotein and dentin phosphoprotein overexpression during amelogenesis. J. Biol. Chem. 280, 31991–31998. doi: 10.1074/jbc.M502991200
Park, H., Hyun, H. K., Woo, K. M., and Kim, J. W. (2020). Physicochemical properties of dentinogenesis imperfecta with a known DSPP mutation. Arch. Oral Biol. 117:104815. doi: 10.1016/j.archoralbio.2020.104815
Porntaveetus, T., Nowwarote, N., Osathanon, T., Theerapanon, T., Pavasant, P., Boonprakong, L., et al. (2019). Compromised alveolar bone cells in a patient with dentinogenesis imperfecta caused by DSPP mutation. Clin. Oral Investig. 23, 303–313. doi: 10.1007/s00784-018-2437-7
Porntaveetus, T., Osathanon, T., Nowwarote, N., Pavasant, P., Srichomthong, C., Suphapeetiporn, K., et al. (2018). Dental properties, ultrastructure, and pulp cells associated with a novel DSPP mutation. Oral Dis. 24, 619–627. doi: 10.1111/odi.12801
Prasad, M., Butler, W. T., and Qin, C. (2010). Dentin sialophosphoprotein in biomineralization. Connect. Tissue Res. 51, 404–417. doi: 10.3109/03008200903329789
Ritchie, H. H., Berry, J. E., Somerman, M. J., Hanks, C. T., Bronckers, A. L., Hotton, D., et al. (1997). Dentin sialoprotein (DSP) transcripts: developmentally-sustained expression in odontoblasts and transient expression in pre-ameloblasts. Eur. J. Oral Sci. 105, 405–413. doi: 10.1111/j.1600-0722.1997.tb02137.x
Ritchie, H. H., Hou, H., Veis, A., and Butler, W. T. (1994). Cloning and sequence determination of rat dentin sialoprotein, a novel dentin protein. J. Biol. Chem. 269, 3698–3702. doi: 10.1016/S0021-9258(17)41916-8
Ritchie, H. H., and Wang, L. H. (1996). Sequence determination of an extremely acidic rat dentin phosphoprotein. J. Biol. Chem. 271, 21695–21698. doi: 10.1074/jbc.271.36.21695
Robinson, C., Lowe, N. R., and Weatherell, J. A. (1977). Changes in amino-acid composition of developing rat incisor enamel. Calcif. Tissue Res. 23, 19–31. doi: 10.1007/BF02012762
Schmitz, J. E., Teepe, J. D., Hu, Y., Smith, C. E., Fajardo, R. J., and Chun, Y. H. (2014). Estimating mineral changes in enamel formation by ashing/BSE and microCT. J. Dent. Res. 93, 256–262. doi: 10.1177/0022034513520548
Shields, E. D., Bixler, D., and El-Kafrawy, A. M. (1973). A proposed classification for heritable human dentine defects with a description of a new entity. Arch. Oral Biol. 18, 543–553. doi: 10.1016/0003-9969(73)90075-7
Smith, C. E., Hu, Y., Richardson, A. S., Bartlett, J. D., Hu, J. C., and Simmer, J. P. (2011). Relationships between protein and mineral during enamel development in normal and genetically altered mice. Eur. J. Oral Sci. 119: (Suppl 1), 125–135. doi: 10.1111/j.1600-0722.2011.00871.x
Smith, C. E., and Nanci, A. (1989). A method for sampling the stages of amelogenesis on mandibular rat incisors using the molars as a reference for dissection. Anat. Rec. 225: 257–266. doi: 10.1002/ar.1092250312
Smith, C. E. L., Poulter, J. A., Antanaviciute, A., Kirkham, J., Brookes, S. J., Inglehearn, C. F., et al. (2017). Amelogenesis imperfecta; genes, proteins, and pathways. Front. Physiol. 8:435. doi: 10.3389/fphys.2017.00435
Sreenath, T., Thyagarajan, T., Hall, B., Longenecker, G., D'Souza, R., Hong, S., et al. (2003). Dentin sialophosphoprotein knockout mouse teeth display widened predentin zone and develop defective dentin mineralization similar to human dentinogenesis imperfecta type III. J. Biol. Chem. 278, 24874–24880. doi: 10.1074/jbc.M303908200
Sun, Y., Lu, Y., Chen, S., Prasad, M., Wang, X., Zhu, Q., et al. (2010). Key proteolytic cleavage site and full-length form of DSPP. J. Dent. Res. 89, 498–503. doi: 10.1177/0022034510363109
Suzuki, S., Sreenath, T., Haruyama, N., Honeycutt, C., Terse, A., Cho, A., et al. (2009). Dentin sialoprotein and dentin phosphoprotein have distinct roles in dentin mineralization. Matrix Biol. 28, 221–229. doi: 10.1016/j.matbio.2009.03.006
Taleb, K., Lauridsen, E., Daugaard-Jensen, J., Nieminen, P., and Kreiborg, S. (2018). Dentinogenesis imperfecta type II- genotype and phenotype analyses in three Danish families. Mol. Genet. Genomic Med. 6, 339–349. doi: 10.1002/mgg3.375
Thesleff, I. (2003). Epithelial-mesenchymal signalling regulating tooth morphogenesis. J. Cell Sci. 116, 1647–1648. doi: 10.1242/jcs.00410
Verdelis, K., Szabo-Rogers, H. L., Xu, Y., Chong, R., Kang, R., Cusack, B. J., et al. (2016). Accelerated enamel mineralization in Dspp mutant mice. Matrix Biol. 52-54, 246–259. doi: 10.1016/j.matbio.2016.01.003
von Marschall, Z., and Fisher, L. W. (2010). Dentin sialophosphoprotein (DSPP) is cleaved into its two natural dentin matrix products by three isoforms of bone morphogenetic protein-1 (BMP1). Matrix Biol. 29, 295–303. doi: 10.1016/j.matbio.2010.01.002
Wang, S. K., Chan, H. C., Rajderkar, S., Milkovich, R. N., Uston, K. A., Kim, J. W., et al. (2011). Enamel malformations associated with a defined dentin sialophosphoprotein mutation in two families. Eur. J. Oral Sci. 119: (Suppl. 1), 158–167. doi: 10.1111/j.1600-0722.2011.00874.x
Warshawsky, H., and Smith, C. E. (1974). Morphological classification of rat incisor ameloblasts. Anat. Rec. 179: 423–446. doi: 10.1002/ar.1091790403
White, S. N., Paine, M. L., Ngan, A. Y., Miklus, V. G., Luo, W., Wang, H., et al. (2007). Ectopic expression of dentin sialoprotein during amelogenesis hardens bulk enamel. J. Biol. Chem. 282, 5340–5345. doi: 10.1074/jbc.M604814200
Wright, J. T., and Gantt, D. G. (1985). The ultrastructure of the dental tissues in dentinogenesis imperfecta in man. Arch. Oral Biol. 30, 201–206. doi: 10.1016/0003-9969(85)90116-5
Yamakoshi, Y., Hu, J. C., Fukae, M., Zhang, H., and Simmer, J. P. (2005). Dentin glycoprotein: the protein in the middle of the dentin sialophosphoprotein chimera. J. Biol. Chem. 280, 17472–17479. doi: 10.1074/jbc.M413220200
Yamakoshi, Y., Nagano, T., Hu, J. C., Yamakoshi, F., and Simmer, J. P. (2011). Porcine dentin sialoprotein glycosylation and glycosaminoglycan attachments. BMC Biochem. 12:6. doi: 10.1186/1471-2091-12-6
Yang, J., Kawasaki, K., Lee, M., Reid, B. M., Nunez, S. M., Choi, M., et al. (2016). The dentin phosphoprotein repeat region and inherited defects of dentin. Mol. Genet. Genomic Med. 4, 28–38. doi: 10.1002/mgg3.176
Zhang, H., Xie, X., Liu, P., Liang, T., Lu, Y., and Qin, C. (2018). Transgenic expression of dentin phosphoprotein (DPP) partially rescued the dentin defects of DSPP-null mice. PLoS One 13:e0195854. doi: 10.1371/journal.pone.0209862
Zhu, Q., Gibson, M. P., Liu, Q., Liu, Y., Lu, Y., Wang, X., et al. (2012). Proteolytic processing of dentin sialophosphoprotein (DSPP) is essential to dentinogenesis. J. Biol. Chem. 287, 30426–30435. doi: 10.1074/jbc.M112.388587
Keywords: tooth development, enamel, amelogenesis, ameloblasts, cell differentiation, mineralization, dentin sialophosphoprotein
Citation: Liang T, Xu Q, Zhang H, Wang S, Diekwisch TGH, Qin C and Lu Y (2021) Enamel Defects Associated With Dentin Sialophosphoprotein Mutation in Mice. Front. Physiol. 12:724098. doi: 10.3389/fphys.2021.724098
Edited by:
Shuo Chen, The University of Texas Health Science Center at San Antonio, United StatesReviewed by:
Angela Quispe-Salcedo, Niigata University, JapanJanet Moradian-Oldak, University of Southern California, United States
Copyright © 2021 Liang, Xu, Zhang, Wang, Diekwisch, Qin and Lu. This is an open-access article distributed under the terms of the Creative Commons Attribution License (CC BY). The use, distribution or reproduction in other forums is permitted, provided the original author(s) and the copyright owner(s) are credited and that the original publication in this journal is cited, in accordance with accepted academic practice. No use, distribution or reproduction is permitted which does not comply with these terms.
*Correspondence: Yongbo Lu, ylu@tamu.edu
†Present address: Tian Liang, Department of Biologic and Materials Sciences, University of Michigan School of Dentistry, Ann Arbor, MI, United States
‡These authors have contributed equally to this work and share first authorship