- 1Department of Water Sports, Faculty of Physical Education and Sport, University of Physical Education, Kraków, Poland
- 2Department of Sport Sciences and Physical Education, Instituto Politécnico de Bragança, Bragança, Portugal
- 3Department of Sports Sciences, Polytechnic of Guarda, Guarda, Portugal
- 4Research Center in Sports Sciences, Health and Human Development (CIDESD), Vila Real, Portugal
Background: The aim of this research was to examine the relationship between the fast component of oxygen consumption developed in 1-min
Methods: Forty-eight male swimmers (aged 13.5 ± 0.9 years old) participated in this study. Testing included 1) 1-min all-out front crawl tethered swimming while oxygen consumption (breath by breath) and tethered forces were measured, 2) 200-m front crawl race-like swimming featuring kinematic analysis, and 3) biological age (BA) examination.
Results: During the 1-min all-out tethered swimming test, a linear increase in oxygen consumption was observed. There were moderate to high partial correlations between particular periods of seconds in the 1-min
Conclusion: The ability of reaching a high level of
Introduction
The ability to increase energy production is considered crucial in various sports, even in swimming where high velocities cause relatively high energy cost of movement. Thus, it is necessary among athletes of different age groups to develop either aerobic or anaerobic metabolic pathways of energy production. This begins with proper and adequate training from early prepubertal age and continues further with aging, while controlling the maturation level of the swimmer (Balyi and Way, 2009; Lätt et al., 2009). The contribution of energy pathways in swimming events is varied and depends on the duration of the race (Olbrecht, 2000). The 200-m front crawl, for example, is a race which requires a high involvement of aerobic and anaerobic pathways of energy production (Gastin, 2001).
The aerobic energy system participates in the overall energy production right from the beginning of the all-out effort, and the oxygen uptake almost reaches its maximum level within 60 s of exercising (Gastin and Lawson, 1994; Serresse et al., 1988; Strzała and Tyka 2009). It has been stated that the maximal oxygen uptake
In swimming, the examination of specific strength abilities is deemed as a key factor when performing an evaluation. For this purpose, swimming tethered tests are often conducted in adults (Kjendlie and Thorsvald, 2006) and swimmers of other age groups (Amaro et al., 2014). Several studies have confirmed a strong relationship between tethered swimming tests (30–120 s) and short-to-middle distance swimming performances (Morouço et al., 2012; Santos et al., 2016).
Biomechanical indices such as stroke length (SL), stroke rate (SR), and stroke index (SI) are significant predictors of young swimmers’ performance (Lätt et al., 2009) and are directly related to swimming efficiency (Geladas et al., 2005). The literature reports that strength preparation and a well-developed oxygen system should cause better stroke kinematics in terms of the ability to maintain proper SR and SL along the race (Costill et al., 1985; Sokołowski et al., 2021). Given these premises, the aim of this research was threefold: 1) to examine the relationship between the fast component of oxygen consumption and tethered swimming force production, 2) to examine the relationship between the fast component of oxygen consumption and 200-m front crawl race kinematics, and 3) to assess the relationship between 200-m front crawl race swimming kinematics and performance. It is hypothesized that there would be a significant relationship between oxygen uptake, tethered swimming force, stroke kinematics, and the performance indices.
Materials and methods
Participants
Forty-eight young male swimmers [13.5 ± 0.9 years old; 14.55 ± 1.66 years of biological age (BA)] participated in this study. They were recruited as swimmers with the highest performance level in their age category from the Polish region of Krakow and were at the fifth threshold in the Ruiz-Navarro et al. (2022) classification of competitive level. Participants presented swimming levels which resulted in a mean value of 350.32 ± 60.22 FINA points for the 200-m front crawl race. All participants were clinically healthy and held a license from the Polish Swimming Federation. All swimmers had been through 4–5 years of systematic swimming at the time of conducting this research, encompassing at least 10 sessions per week and had taken part in national-level competitions and national swimming championships for their age group.
1-min Tethered swimming test
A tethered swimming test (Figure 1) in a laboratory-controlled environment (temperature and humidity) was conducted. The test consisted of a single bout of 1-min duration of all-out freestyle tethered swimming and was performed in a flume in still water. With due advance notice, the swimmers were asked to rest the day before the test and maintain their daily diet. Before entering the pool, they were informed about the testing procedure and then underwent a 1000-m in-water warm-up, as before any competition. After the warm-up and before the test, they swam for 1 min in the flume at a slow pace, fully equipped with the testing apparatus for adjusting to the testing conditions. At this time, they got the possibility to familiarize with the specific environment of the flume and potential inconveniences of using the breathing apparatus and tethered swimming. After the initial 1 min of familiarization, the scientist conducting the test received feedback from the participant. To signal the beginning and ending of the test, a whistle was used. For the last minutes of warm-up and the test itself, the swimmers were asked to breathe only through the mouthpiece and avoid losing their nose clip. This procedure is similar to their training sessions done using a snorkel. The swimmers were equipped with a respiratory valve system that featured an ergospirometer (Start 2000 MES, Poland). The valve system was attached to a rod-like construction just above the swimmer’s head. During the duration of the test, the expired air was analyzed continuously (breath by breath) (Ergo 2000M software MES, Poland) and data were saved for further analysis. This has been proved to be a reliable method of calculating oxygen uptake in swimming (Neiva et al., 2017; Ribeiro et al., 2015; Sousa et al., 2011).
From the collected data, the following indices were computed: 1) average oxygen consumption from the first 30 s of the test (1–30
Additionally, the participants wore a nylon waist belt, connected by a 3.7 m steel cable to a load cell (ZPS5-BTU-1kN, Poland) which was fixed on a steel pole (the fixing point is 0.49 m above the water surface). Data were recorded by the load cell at 100 Hz and transferred to a computer software program for further analysis (MAX6v0M software, Poland). Three parameters were calculated over a 60-s recording time: 1) maximum value of force (Fmax, N); 2) average value of force in the entire test (Fave, N) and in the first and second 30-s parts: Fave 0-30, Fave 30-60, N; and 3) average impulse per single cycle (Iave, N·s−1) which is defined as the integral of force over a period of time containing all full cycles divided by the number of completed cycles:
where t0 is the beginning of the first full cycle and t1 is the ending of the last full cycle in the 60-s period. Tethered swimming has been described as a reliable method to assess swimming force production (Kjendlie and Thorsvald, 2006; Psycharakis et al., 2011; Amaro et al., 2014).
200-m Front crawl race
The 200-m all-out test was carried out in a 25-m swimming pool that meets the International Swimming Federation (FINA) requirements. Before the race, the swimmers completed a 1000-m warm-up just like in competitions. Each trial was performed by three to four swimmers in order to mimic competition conditions. The final and split times of each trial were measured with an automatic timing device (Omega, Switzerland; OCP5, StartTime V). All trials were recorded with a camera at 50 Hz framing (GC-PX100BE, JVC, Japan).
The velocity of the part of the race containing the first 10-m start zone as well as start, turn, and finish (which resulted in 115 m) was calculated as VSTF (m·s−1). The surface swimming velocity, i.e., the velocity over the effective clean swimming distance (85 m) was deemed Vsurf (m·s−1). The times for separate sectors were measured when the swimmer's head crosses the imaginary line linking the markers at both sides of the pool. The 200-m front crawl velocity (Vtotal200, m·s−1) was defined as 200 divided by the final time of the race. The video footage, placement of the cameras and markers, video analysis, and computation of the basic kinematic parameters were performed analogically to the ones described in the literature (Sokołowski et al., 2021), but in this study, a swimming distance twice as long was considered.
Kinematic parameters
For the kinematic analysis, the stroke rate (SR), stroke length (SL), and stroke index were calculated. The SR was defined as the number of full stroke cycles performed within a unit of time (in cycles per minute) and was calculated by video analysis of three consecutive stroke cycles (intraclass correlation of 0.99, 95% CI = 0.960–0.997). The SL was defined as the horizontal distance that the body travels during a full stroke cycle and was calculated as
where SL (in m) is the stroke length, v is the swimming velocity, and SR is the stroke rate. Finally, the SI was deemed as an overall swimming efficiency estimator and computed as
where SI (in m2∙s−1) is the stroke index, SL is the stroke length, and v is the swimming velocity.
Biological age
Examination of the participants in terms of BA was conducted by an experienced anthropologist and calculated as
where BHage is the age obtained from the percentile charts based on the participant’s body height and BMage is the age obtained from the percentile charts based on the participant’s body mass. The growth charts by the Children’s Memorial Health Institute, which are standardized and validated for the Polish population, were used (the 50th percentile was used to align the height and mass with age). Additionally, pubertal development was assessed. The Tanner stages based on pubic hair scale were estimated (Bornstein, 2018). The great variety of biological maturation levels in the adolescent groups at the same calendar age causes great differences in muscle mass and aerobic and anaerobic capacities of swimmers. Because of differences in maturation specific water abilities of swimmers and specific testing could be less correlated with swimming performance than simple general tests as isometric force or counter movement jump (Garrido et al., 2012; Strzała et al., 2019). BA may cause bias in the statistical analysis and conclusions. The use of partial correlation statistics with age control helps limit the strong influence of BA in the effects of statistical calculations. The data used in biological age calculation are presented in Figure 2.
Statistical analysis
The values are presented as mean ± standard deviation. The normality of the data was checked with the Kolmogorov–Smirnov test. In oxygen consumption averaged per 10-s periods, the trend that was most suitable for the gathered data (Figure 3) was identified. The paired-sample t-test was used to compare the values of the average tethered swimming force of the first and second parts of the 1-min tethered swimming test. To identify the relationship between all the variables and swimming velocities in the 200-m front crawl, partial correlations controlled for BA were computed for
1) oxygen consumption and force indices;
2) oxygen consumption, swimming speed variables, and kinematic indices; and
3) swimming speed variables and kinematic and force indices.
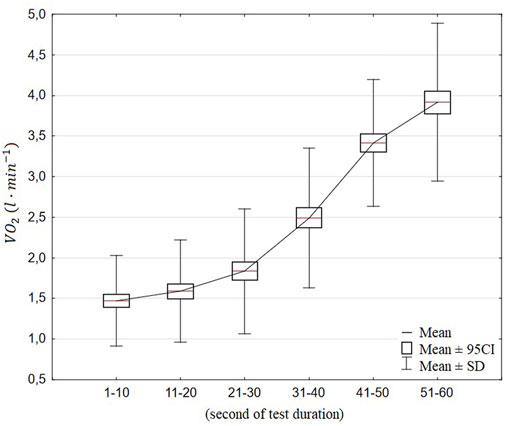
FIGURE 3. Average oxygen consumption of all participants, in 10-s periods, during the 1-min tethered swimming test.
The magnitude of the correlations was determined using the modified scale by Hopkins (2000)—trivial: r ≤ 0.1; low: 0.1 < r ≤ 0.3; moderate: 0.3 < r ≤ 0.5; high: 0.5 < r ≤ 0.7; very high: 0.7 < r ≤ 0.9; nearly perfect: r > 0.9; and perfect: r = 1.
Results
The data shown in Figure 3 represent the increase in oxygen consumption in the 1-min all-out tethered swimming test, in 10-s periods. The analysis of variance revealed significant differences between values measured every 10 s (F = 164,9, p < 0.01). Further trend analysis indicates the linear trend as the best adjusted to the collected data (F = 289,44, p < 0.01).
There were moderate to high correlations between 31–60
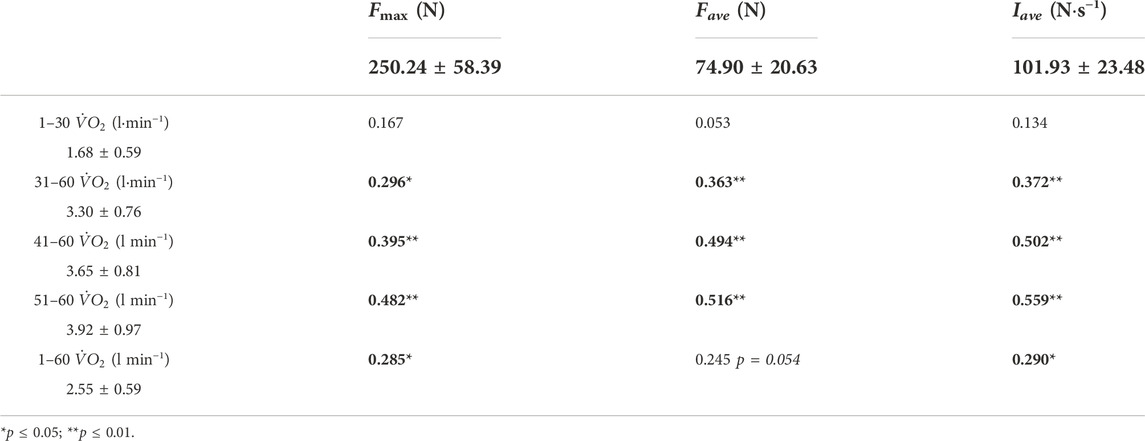
TABLE 1. Partial correlations controlled for BA between oxygen consumption and force indices from the tethered swimming test.
The 41–60
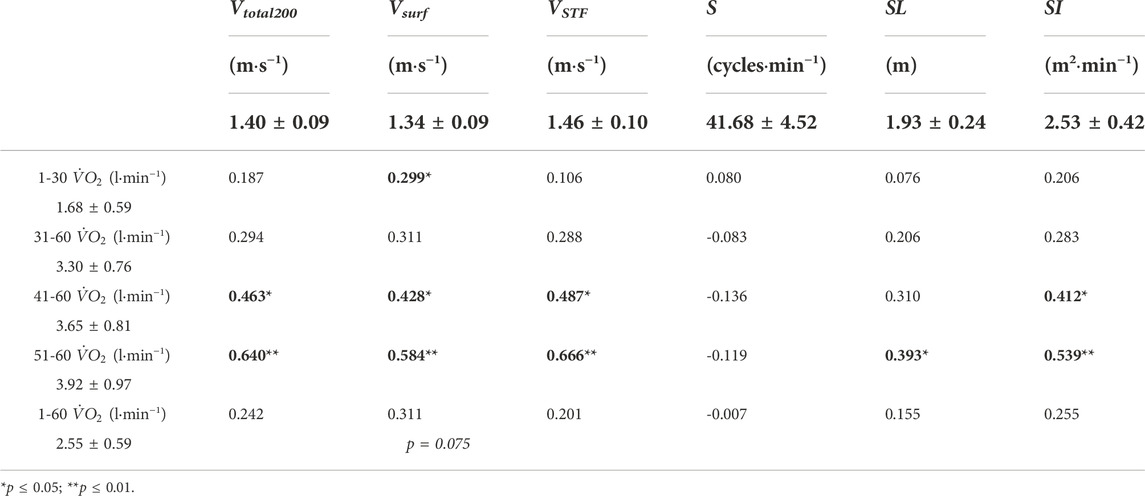
TABLE 2. Partial correlations controlled for BA between oxygen consumption indices from the tethered swimming test, and swimming speed variables and kinematic indices from the 200-m front crawl race.
Regarding the swimming speed and kinematic variables, the strongest relationships were observed between SI and Vtotal200 and Vsurf and VSTF. The swimming speed was also moderately correlated with SL, Fmax, Fave, and Iave (Table 3).
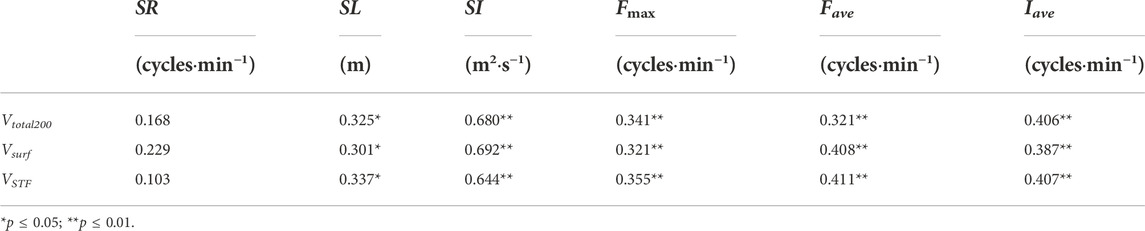
TABLE 3. Partial correlations controlled for BA between swimming speed variables and kinematic indices from the 200-m front crawl race and the force indices from the tethered swimming test.
As a supplement to the results, it was decided to present the level of selected oxygen uptake and strength indicators, measured in the 1-min test, followed by the kinematics of 200-m front crawl in relation to BA (Table 4). It could be observed that oxygen uptake and strength abilities continuously improve with higher BA. There was also a general increase in values of stroke kinematics through the years of BA.
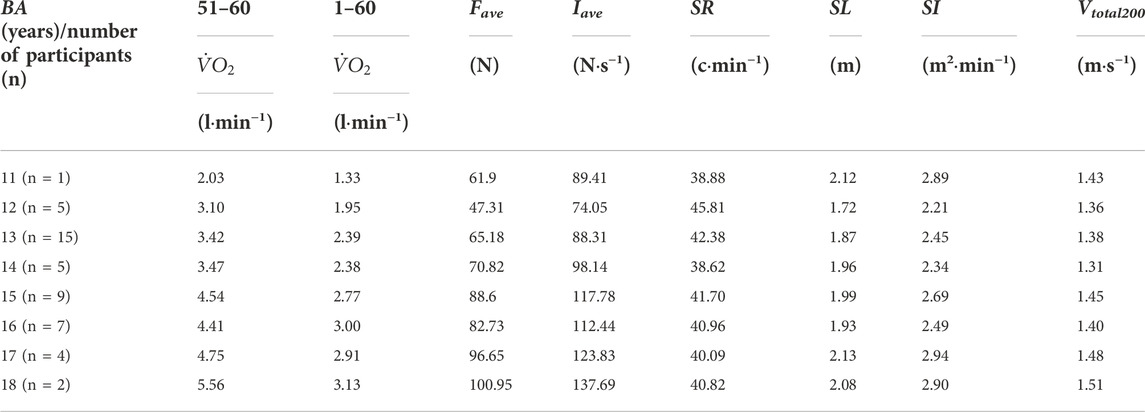
TABLE 4. Average values of oxygen uptake, tethered swimming, and kinematic indices of 200-m front crawl calculated for biological age.
Table 5 shows 200-m front crawl kinematics by each 50-m lap.
Discussion
Regarding the analysis of
This study noted a relationship between 51–60
It can be stated that for high aerobic capacity, the fast development of high level of
Regarding tethered force production, in the present study, a significant positive correlation was found between all indices and 200-m front crawl speed (0.321 ≤ r ≤ 0.411, p ≤ 0.01). Other authors have reported similar findings: Santos et al. (2016) have noted a positive correlation (0.61, p < 0.001) between the peak force of the 2-min tethered swimming test and clean velocity of 200-m front crawl race, while Morouço et al. (2012) showed a very strong relationship between average pulling force, peak force, and 200-m front crawl velocity (r = 0.94 and r = 0.93, respectively, p < 0.01). Again, controlling for BA and longer test duration could be the reasons for weaker correlations in our study.
Our study showed great diversity in BA (Figure 2; Table 4). It is therefore a practical example of emphasizing the need for each trainer to adapt their training in relation to the BA of their swimmers. If this is the case, even the most gifted swimmers with delays in relation to BA are often frustrated by worse athletic performance when compared to their calendar peers, and in consequence, they overtrain trying to catch up to the others, get disappointed, then quit their swimming training. On the other hand, swimmers more advanced in relation to BA have the potential to develop through more individualized, intense training.
Based on the high correlation between 51–60
The present study, analyzing the relationship between the aerobic conditioning level, force production, and stroke kinematics is in accordance with the one study found in the literature on this matter, where Costill et al. (1985) identified interrelationships between oxygen uptake, energy cost of swimming, and stroking economy (SI). In our study, we found moderate to high correlations between 31–60
Conclusion
In the 1-min all-out effort, a sudden increase in oxygen uptake was observed, with swimmers reaching high levels of
Data availability statement
The raw data supporting the conclusions of this article will be made available by the authors, without undue reservation.
Ethics statement
The studies involving human participants were reviewed and approved by the Regional Medical Chamber in Cracow; decision number: 94/KBL/OIL/2020. Written informed consent to participate in this study was provided by the participants' legal guardian/next of kin.
Author contributions
KS collected data, performed statistical analysis, and wrote the manuscript. RB cowrote the manuscript. TB reread and corrected the manuscript. MS cowrote the manuscript and collected data.
Funding
Article processing charge (open access) was funded within the framework of the programme of the Ministry of Science and Higher Education (Poland) under the name “Regional Initiative for Perfection” within the years 2019–2022, project No. 022/RID/2018/19 in the total of 11,919,908 PLN.
Conflict of interest
The authors declare that the research was conducted in the absence of any commercial or financial relationships that could be construed as a potential conflict of interest.
Publisher’s note
All claims expressed in this article are solely those of the authors and do not necessarily represent those of their affiliated organizations, or those of the publisher, editors, and reviewers. Any product that may be evaluated in this article, or claim that may be made by its manufacturer, is not guaranteed or endorsed by the publisher.
References
Alves F., Reis J. F., Alves F. B., Bruno P. M., Vleck V., Millet G. P. (2011). Oxygen uptake kinetics and middle distance swimming performance. J. Sci. Med. Sport 15, 58–63. doi:10.1016/j.jsams.2011.05.012
Amaro N., Marinho D. A., Batalha N., Marques M. C., Morouço P. (2014). Reliability of tethered swimming evaluation in age group swimmers. J. Hum. Kinet. 41, 155–162. doi:10.2478/hukin-2014-0043
Balyi I., Way R. (2009). The role of monitoring growth in long-term athlete development Canadian sport for life. Available at: http://lthd.fieldhockey.ca/files/CS4LDocs/Monitoring Growth.pdf (Accessed February 9, 2022).
Barbosa T., Costa M., Marinho D. (2013). Deterministic model-swimming performance. Available at: http://www.swimkinetics.isosc.org/ (Accessed June 17, 2022).
Bornstein M. H. (2018). The SAGE encyclopedia of lifespan human development. National Institute of Child Health & Human Development, Bethesda, Maryland. doi:10.4135/9781506307633
Costill D. L., Kovaleski J., Porter D., Kirwan J., Fielding R., King D. (1985). Energy expenditure during front crawl swimming: Predicting success in middle-distance events. Int. J. Sports Med. 6, 266–270. doi:10.1055/s-2008-1025849
Figueiredo P., Zamparo P., Sousa A., Vilas-Boas J. P., Fernandes R. J. (2011). An energy balance of the 200 m front crawl race. Eur. J. Appl. Physiol. 111, 767–777. doi:10.1007/s00421-010-1696-z
Garrido N. D., Silva A. J., Fernandes R. J., Barbosa T. M., Costa A. M., Marinho D., et al. (2012). HigH level swimming performance and its relation to non-specific parameters: A cross-sectional study on maximum handgrip isometric strengtH. Percept. Mot. Ski. 114, 936–948. doi:10.2466/05.10.25.PMS.114.3.936-948
Gastin P. B., Lawson D. L. (1994). Variable resistance all-out test to generate accumulated oxygen deficit and predict anaerobic capacity. Eur. J. Appl. Physiol. Occup. Physiol. 69, 331–336. doi:10.1007/BF00392039
Gastin P. (2001). Energy system interaction and relative contribution during maximal exercise. Sports Med. 31, 725–741. doi:10.2165/00007256-200131100-00003
Geladas N. D., Nassis G. P., Pavlicevic S. (2005). Somatic and physical traits affecting sprint swimming performance in young swimmers. Int. J. Sports Med. 26, 139–144. doi:10.1055/s-2004-817862
Greenwood J. D., Moses G. E., Bernardino F. M., Gaesser G. A., Weltman A. (2008). Intensity of exercise recovery, blood lactate disappearance, and subsequent swimming performance. J. Sports Sci. 26, 29–34. doi:10.1080/02640410701287263
Hellard P., Pla R., Rodríguez F. A., Simbana D., Pyne D. B. (2018). Dynamics of the metabolic response during a competitive 100-m freestyle in elite male swimmers. journals.humankinetics.Com. 13 (8):1011-20. Available at: https://journals.humankinetics.com/view/journals/ijspp/13/8/article-p1011.xml (Accessed November 4, 2022). doi:10.1123/ijspp.2017-0597
Hopkins W. G. (2000). Measures of reliability in sports medicine and science. Sports Med. 30, 1–15. doi:10.2165/00007256-200030010-00001
Kjendlie P. L., Thorsvald K. (2006). A tethered swimming power test is highly reliable. Portuguese J. Sport Sci. 6, 231–233. Available at: https://www.researchgate.net/publication/267548775 (Accessed April 25, 2022).
Lätt E., Jürimäe J., Haljaste K., Cicchella A., Purge P., Jürimäe T. (2009). Physical development and swimming performance during biological maturation in young female swimmers. Coll. Antropol. 33 (1), 117–122.
Mezzaroba P. V., Machado F. A. (2013). Effect of age, anthropometry, and distance in stroke parameters of young swimmers. Int. J. Sports Physiol. Perform. 9, 702–706. doi:10.1123/IJSPP.2013-0278
Morouço P. G., Vilas-Boas J. P., Fernandes R. J. (2012). Evaluation of adolescent swimmers through a 30-s tethered test. Pediatr. Exerc. Sci. 24, 312–321. doi:10.1123/pes.24.2.312
Nasirzade A., Sadeghi H., Sobhkhiz A., Mohammadian K., Nikouei A., Baghaiyan M., et al. (2015). Multivariate analysis of 200-m front crawl swimming performance in young male swimmers. Acta Bioeng. Biomech. 17, 137–143. paper 17. doi:10.5277/ABB-00160-2014-03
Neiva H. P., Marques M. C., Barbosa T. M., Izquierdo M., Viana J. L., Teixeira A. M., et al. (2017). Warm-up for sprint swimming: Race-pace or aerobic stimulation? A randomized study. The Journal of Strength and Conditioning Research, 31 (9), 2423–2431.
Olbrecht J. (2000). The science of winning: Planning, periodizing and optimizing swim training. F&G Partners, luton, England.
Peyrebrune M. C., Toubekis A. G., Lakomy H. K. A., Nevill M. E. (2014). Estimating the energy contribution during single and repeated sprint swimming. Scand. J. Med. Sci. Sports 24, 369–376. doi:10.1111/j.1600-0838.2012.01517.x
Psycharakis S. G., Paradisis G. P., Zacharogiannis E. (2011). Assessment of accuracy, reliability and force measurement errors for a tethered swimming apparatus. Int. J. Perform. Anal. Sport 11, 410–416. doi:10.1080/24748668.2011.11868560
Ribeiro J., Figueiredo P., Sousa A., Monteiro J., Pelarigo J., Vilas-Boas J. P., et al. (2015). VO2 kinetics and metabolic contributions during full and upper body extreme swimming intensity. Eur. J. Appl. Physiol. 115, 1117–1124. doi:10.1007/s00421-014-3093-5
Rodríguez F. A., Keskinen K. L., Malvela M. T., Keskinen O. P. (2003). Oxygen uptake kinetics during free swimming: A pilot study. Biomechanics Med. Swim. IX, University of Saint-Etienne, Saint-Etienne, France, 379–384. Available at: https://www.researchgate.net/publication/234015321 (Accessed May 16, 2022).
Ruiz-Navarro J. J., López-Belmonte Ó., Gay A., Cuenca-Fernández F., Arellano R. (2022). A new model of performance classification to standardize the research results in swimming. Eur. J. Sport Sci., 1–11. doi:10.1080/17461391.2022.2046174
Sánchez J., Arellano R. (2002). Stroke index values according to level, gender, swimming style and event race distance. Proc. XXth Int. symposium biomechanics sports 20, 56–59. Available at: https://ojs.ub.uni-konstanz.de/cpa/article/view/620 (Accessed June 17, 2022).
Santos K. B., Bento P. C. B., Pereira G., Rodacki A. L. F. (2016). The relationship between propulsive force in tethered swimming and 200-m front crawl performance. J. Strength Cond. Res. 30, 2500–2507. doi:10.1519/JSC.0000000000000410
Serresse O., Lortie G., Bouchard C., Boulay M. R. (1988). Estimation of the contribution of the various energy systems during maximal work of short duration. Int. J. Sports Med. 9, 456–460. doi:10.1055/s-2007-1025051
Sokołowski K., Strzała M., Stanula A., Kryst Ł., Radecki-Pawlik A., Krężałek P., et al. (2021). Biological age in relation to somatic, physiological, and swimming kinematic indices as predictors of 100 m front crawl performance in young female swimmers. Int. J. Environ. Res. Public Health 18, 6062. doi:10.3390/ijerph18116062
Sousa A. C., Figueiredo P., Oliveira N. L., Oliveira J., Silva A. J., Keskinen K. L., et al. (2011). VO2 kinetics in 200-m race-pace front crawl swimming. Int. J. Sports Med. 32, 765–770. doi:10.1055/s-0031-1279772
Strzała M., Stanula A., Krężałek P., Ostrowski A., Kaca M., Głąb G. (2019). Influence of morphology and strength on front crawl swimming speed in junior and youth age-group swimmers. J. Strength Cond. Res. 33, 2836–2845. doi:10.1519/JSC.0000000000002084
Strzała M., Tyka A. (2009). Physical endurance, somatic indices and swimming technique parameters as determinants of front crawl swimming speed at short distances in young swimmers. Med. Sport. 13, 99–107. doi:10.2478/v10036-009-0016-3
Keywords: adolescent swimming, oxygen uptake, tethered swimming, front crawl, biological age, kinematic indices
Citation: Sokołowski K, Bartolomeu RF, Barbosa TM and Strzała M (2022)
Received: 15 September 2022; Accepted: 07 November 2022;
Published: 24 November 2022.
Edited by:
Philippe Hellard, Ministry of Education and Sport, AlbaniaReviewed by:
Sebastian Weber, INSCYD, SwitzerlandSantiago Veiga, Universidad Politécnica de Madrid, Spain
Copyright © 2022 Sokołowski, Bartolomeu, Barbosa and Strzała. This is an open-access article distributed under the terms of the Creative Commons Attribution License (CC BY). The use, distribution or reproduction in other forums is permitted, provided the original author(s) and the copyright owner(s) are credited and that the original publication in this journal is cited, in accordance with accepted academic practice. No use, distribution or reproduction is permitted which does not comply with these terms.
*Correspondence: Kamil Sokołowski, kamil.sokolowski@awf.krakow.pl