- 1Sports Coaching College, Beijing Sports University, Beijing, China
- 2Big Ball Sports Center, Hebei Provincial Sports Bureau, Shijiazhuang, China
Background: Nowadays, many high-profile international sport events are often held in warm or hot environments, hence, it is inevitable for these elite athletes to be prepared for the challenges from the heat. Owing to internal cooling may cause gastrointestinal discomfort to athletes, external cooling technique seems to be a more applicable method to deal with thermal stress. Central cooling mainly refers to head, face, neck and torso cooling, can help to reduce skin temperature and relieve thermal perception. Peripheral cooling mainly refers to four limbs cooling, can help to mitigate metabolic heat from muscular contrac to effectively prevent the accumulation of body heat. Hence, we performed a meta-analysis to assess the effectiveness of different external cooling placements on athletic performance in the heat
Methods: A literatures search was conducted using Web of Science, MEDLINE and SPORTDiscus until September 2022. The quality and risk of bias in the studies were independently assessed by two researchers.
Results: 1,430 articles were initially identified (Web of Science = 775; MEDLINE = 358; SPORTDiscus = 271; Additional records identified through other sources = 26), 60 articles (82 experiments) met the inclusion criteria and were included in the final analysis, with overall article quality being deemed moderate. Central cooling (SMD = 0.43, 95% CI 0.27 to 0.58, p < 0.001) was most effective in improving athletic performance in the heat, followed by central and peripheral cooling (SMD = 0.38, 95% CI 0.23 to 0.54, p < 0.001), AND peripheral cooling (SMD = 0.32, 95% CI 0.07 to 0.57, p = 0.013). For the cooling-promotion effects on different sports types, the ranking order in central cooling was ETE (exercise to exhaustion), TT (time-trial), EWT (exercise within the fixed time or sets), IS (intermittent sprint); the ranking order in peripheral cooling was EWT, TT, ETE and IS; the ranking order in central and peripheral cooling was ETE, IS, EWT and TT.
Conclusion: Central cooling appears to be an more effective intervention to enhance performance in hot conditions through improvements of skin temperature and thermal sensation, compared to other external cooling strategies. The enhancement effects of peripheral cooling require sufficient re-warming, otherwise it will be trivial. Although, central and peripheral cooling seems to retain advantages from central cooling, as many factors may influence the effects of peripheral cooling to offset the positive effects from central cooling, the question about whether central and peripheral cooling method is better than an isolated cooling technique is still uncertain and needs more researchs to explore it.
Introduction
During the period of exercise, about more than 75% energy that is generated by skeletal muscle substrate oxidation is liberated as heat, which inevitably lead to an increase in body temperature (Wendt et al., 2007). However, The heat dissipation capacity of the human body would be impaired resulting in a further increase in body temperature, when coupled with hot environmental conditions (Berggren and Hohwu Christensen, 1950; Saltin and Hermansen, 1966). Nowadays, many high-profile international sport events are often held in warm or hot environments, such as 2022 Federation International Football Association (FIFA) World Championship in Qatar, 2023 International Association of Athletics Federations (IAAF) World Championships in Budapest, and 2024 Olympic Games in Paris. These hot environmental conditions would pose a major challenge to the physiological status and sport performance of athletes during the competition, and even lead to fatal incidents (Casa et al., 2005; Vanos et al., 2018). Hence, it is inevitable for these elite athletes to be prepared for the challenges from the heat.
Pre-cooling is considered as a practical on-field strategy for athletes to relieve the adverse effects from heat-stress-induced fatigue and enhance athletic performance, because it is easy to be implemented during the warm-up period (Hohenauer et al., 2018). According to the application of the cooling technique used, cooling strategies are categorized into internal cooling strategy (e.g., cold water ingestion, ice slurry ingestion, etc) and external cooling strategy (e.g., ice vest, ice packs, cold water immersion, etc) (Ross et al., 2013). However, while the effect of ice slurry ingestion on reducing core temperature is significant, it may cause gastrointestinal discomfort to athletes, especially those with gastrointestinal diseases (Bongers et al., 2017). For this reason, external cooling technique instead of internal cooling technique may be a relatively safer and more applicable method to deal with thermal stress. Because in addition to cutaneous thermoreception, thermoreceptive mechanisms exist in body core structures including the brain, spinal cord and abdomen (Morrison and Nakamura, 2011), and an elevated core body temperature is the critical limiting factor for exercise performance in the heat (Maughan, 2010). Hence, central cooling (face, neck, head and torso cooling) may have a relatively more direct effect on mitigating physiological strains and thermal perception to promote athletic performance in the heat (Cotter and Taylor, 2005). On the other hand, peripheral cooling (four limbs cooling) can help to mitigate metabolic heat from muscular contraction to effectively prevent the accumulation of body heat, and improve athletic performance in the heat by the maintenance of muscle recruitment (Randall et al., 2015). Thus, the question about the most effective external cooling placement (central? Or peripheral?) remains unclear.
This study thus aims to quantitatively examine the effects of different external cooling placements on athletic performance in the heat (≥ 28°C) in athlete by completing a systematic review and meta-analysis of the peer-reviewed publications. For practical-application considerations, we conducted subgroup analysis of exercise protocols to explore the influences of each external cooling intervention on various exercise types, which may provide valuable lessons for athletes to select targeted external cooling strategies when performing diverse exercise types (e.g., sustained endurance exercise, intermittent sprint exercise, etc) under hot thermal conditions. Finally, we also proposed some potential research issues about practical external cooling strategies in the future.
Materials and methods
This systematic review and meta-analysis was conducted using Preferred Reporting Items for Systematic Reviews and Meta-Analysis guidelines (PRISMA) (Page et al., 2021) and registered with PROSPERO (Registration ID CRD42022368476), an international prospective registry for systematic reviews.
Data sources and search strategy
Two authors (DJ and QY) independently searched Web of Science, MEDLINE and SPORTDiscus data bases from inception to September 25th 2022, using a comprehensive search strategy (Supplementary Table S1). Manual searches of the reference lists in the related publications were also performed.
A researcher (QY) imported all records into EndnoteX9 software and deleted any duplicates. Then, two researchers (DJ and JD) checked the title and abstract separately to exclude any unrelated articles, with the remaining full texts screened against the inclusion criteria. Discrepancies over article inclusion were settled through discussion with a third researcher (ML) until consensus was achieved. Only peer-reviewed parallel and crossover randomized controlled trials (RCTs) written in English were classifed as relevant. Based on the information within the full text, the inclusion and exclusion criteria were applied to select the trials eligible for inclusion in the review and meta-analysis. Conference papers and case studies were excluded, as were reviews, but their references were manually screened to ensure all appropriate citations were also considered for inclusion.
Eligibility criteria
A PICOS (Participants, Intervention, Comparators, Outcomes, and Study design) approach was used to rate studies for eligibility (Liberati et al., 2009). Studies were eligible if they met the following criteria: 1) the design of study was parallel or crossover RCT; 2) subjects included were healthy and non-injured humans without heat acclimation; 3) male and female study participants were included; 4) hot ambient conditions (≥ 28°C); 5) cooling was conducted prior to and during exercise, which may have included recovery cooling where subjects cooled after one bout of exercise (e.g., simulation of halftime) and before a second bout; 6) cooling interventions were limited to external cooling; 7) specific cooling placements were mentioned; 8) control interventions were passive non-cooling strategies; 9) measurement of athletic performance; 10) provided full data (mean and SD) that allowed effect sizes to be calculated; 11) the manuscripts were written in English and were published in a peer-reviewed journal. Articles were excluded if: 1) studies were incomplete (e.g., abstracts); 2) outcome measures were merely based on physiological parameters; 3) repeated publications.
Data extraction and variable categorisation
The two researchers (QY and JD) extracted the data from the selected literatures with a standard table. In case of disagreement, a third researcher (DJ) checked the data in the original study and agreement was sought by consensus. The following data were extracted: studies (author, year), sample size (number, sex), ambient conditions (temperature, relative humidity), cooling strategies (type, placement, method), exercise details (type, modality, protocol) and sport performance outcome measures. When one study consisted of multiple sport performance outcome measures, only one outcome measure was used and included in the analysis. Discrepancies over sport performance outcome were settled through discussion with a third researcher (ML) until consensus was achieved. When studies only reported standard errors, standard deviations were calculated by multiplying the standard error by the square root of the sample size (Altman and Bland, 2005). In addition, when studies only reported split results, the total results were were calculated with the following algorithms (Higgins et al., 2019):
In the event that relevant data were not available, the corresponding authors of the manuscripts were contacted to request it (Higgins et al., 2019). Studies with missing data that could not be retrieved or provided by the author were excluded from the meta-analysis.
The reviewed articles were divided into three groups depending on the different external cooling placements, namely: central cooling, peripheral cooling and central and peripheral cooling (Giesbrecht et al., 1995; Drinkwater, 2008; Rodríguez et al., 2020). Based on the basic characteristics of different exercise protocols, subsequent analysis was conducted on the external cooling data by dividing the data set into four groups: 1) intermittent sprint (IS); 2) exercise to exhaustion (ETE); 3) exercise within fixed the fixed time or sets (EWT); 4) time-trial (TT) (Alhadad et al., 2019).
Quality assessment
Quality assessment was performed using the Physiotherapy Evidence Database (PEDro) Scale, which is a valid measure of the methodological quality of clinical trials (De Morton, 2009). Two researchers (DJ and QY) assessed the quality of included studies independently according to 11 items on the PEDro scale. For each item whose criterion was met, one point was awarded, whereas no points were given if the item was not fulfilled (Maher et al., 2003). The item 1 (eligibility criteria) which refers to external validity was not included in the total score calculation, and the remaining 10 items which refers to internal validity and statistical reporting were included in the total score calculation. Studies were considered high quality if PEDro scores were greater than 7, medium quality if five to six, and low quality if four and below (Cashin and McAuley, 2019). Two researchers (DJ and QY) applied the PEDro scale to evaluated the 60 studies (82 experiments) independently, and any scoring discrepancies were discussed with a third researcher (ML) until aconsensus was achieved.
Data synthesis
To determine the effect size (ES) of the intervention, the standardized mean difference (SMD; Hedges’ g) of the outcomes was calculated, with a 95% confidence interval (CI). ES was classified as trivial (<0.2), small (0.2–.49), moderate (0.5–0.79), or large (> 0.8) (Cohen, 2013). Meta-analysis was performed in Stata 14.0 (STATA Corp., College Station, TX) using the inverse variance method. The heterogeneity was assessed by measuring the inconsistency (I2 statistic) of intervention effects among the trials. The level of heterogeneity was interpreted according to guidelines from the Cochrane Collaboration: trivial (< 25%), low (25–50%), moderate (50–75%), and high (> 75%) (Higgins et al., 2003). The random effect model was used if there was significant heterogeneity across studies (I2 > 50%). Otherwise, the fixed effect model was used. The publication bias was assessed by the funnel plot and Egger’s test. Subgroup analysis was used to analyze the possible sources of heterogeneity. If a significant asymmetry was detected, we used the Trim and Fill method for sensitivity analysis of the results (Duval and Tweedie, 2000). All the statistical significance was set at p < 0.05.
Results
Search results
The initial search resulted in 1,430 records (Web of Science = 775; MEDLINE = 358; SPORTDiscus = 271; Additional records identified through other sources = 26). After excluding 440 duplicates, 990 studies were selected to be screened by title and abstract. According to the inclusion criteria, 878 papers were excluded. Subsequently, the remaining 112 full-text articles were evaluated for eligibility, and 52 of them were excluded because the ambient temperature was less than 28°C (n = 17), the complete raw data was unavailable (n = 11), not including control groups with passive non-cooling strategies (n = 6), not mentioning specific cooling placements (n = 6), not including sport performance outcomes (n = 5), participants were acclimatized to heat (n = 3), cooling interventions were conducted after exercise (n = 3), cooling interventions included internal and external cooling (n = 1). Finally, 60 articles (82 experiments) meeting the eligibility criteria were included for qualitative analysis (Figure 1).
Quality assessment
In accordance with the PEDro, the scores of 82 in cluded experiments ranged from 5 to 7 (Supplementary Table S2), the mean score was 6.7. Hence, the overall quality of the included experiments was good. The majority of the experiments were rated as high-quality (n = 56), and the remaining experiments were rated as medium-quality (n = 26). 21 experiments did not clearly describe the specific eligibility criteria. Two experiments (Randall et al., 2015a; Randall et al., 2015b) did not get score in item eight and nine because that both only partial subjects (≤ 75%) completed all conditions to get sport performance outcome measures and not all results (≤ 75%) were included in analysis. Due to their crossover design, none of the studies could conceal allocations and blind participants from the interventions. Moreover, none of them blinded therapists or assessors (Rodríguez et al., 2020).
Characteristics of included studies
The basic informations of the participants, ambient conditions, cooling interventions and exercise-test protocol were included in Table 1. Overall, 595 subjects were included in the qualitative analysis. The ambient temperature in the included studies varied from 29°C to 42°C and relative humidity varied from 15% to 80%. The external cooling placements of included studies mainly referred to head, neck, torso, upper limbs and lower limps. According to different external cooling placements, all external cooling interventions can be categorized into central cooling, peripheral cooling and central and peripheral cooling. Among them, 34 experiments used central cooling, 14 experiments used peripheral cooling and 34 experiments used central and peripheral cooling. Regarding cooling methods, CWI and ice vest were the most commonly used means of external cooling, overall 54 experiments’ cooling strategies involved with them. Moreover, other external cooling strategies consisted of neck-cooling device, ice towels, ice packs, cooling garment, cooling jacket, neck-cooling collar, cooling glove, water-perfused cuffs, palm-cooling device, external-pouring cold water, cooling cap, cooling shirt, fan, wet sponge, hand-cooling device, head-cooling device, heat emergency kit, hybrid cooling vest, ice bath, ice headband, ice wraps and mixed external cooling methods. Among them, 65 experiments purely utilized a single external cooling method and 17 experiments used mixed external cooling methods. Exercise modality comprised of cycling (47 experiments), running (33 experiments), rowing (1 experiment) and running combined with bench press (1 experiment). Based on the basic characteristics of different exercise protocols, they can be classified as four various exercise types (IS, ETE, EWT and TT). Specifically, 25 experiments chose IS as sport performance test, 20 experiments chose ETE as sport performance test, 20 experiments chose EWT as sport performance test and 17 experiments chose TT as sport performance test. The sport performance outcome indicators of the 82 included experiments were various. Concretely speaking, the sequence of from high to low of usage frequency of outcome indicators is: distance (19 experiments), total work done (14 experiments), duration (18 experiments), MPO (11 experiments), time (15 experiments), RPO (2 experiments), PPO (1 experiment), speed at VO2max (1 experiment), total reps (1 experiment).
Quantitative analysis
The sport performance tests in included studies can be categorized into four subgroups, namely: IS, ETE, EWT and TT. On the basis of different external cooling placements (central cooling, peripheral cooling and central and peripheral cooling), quantitative analysing the effects of each cooling placement on four sport performance tests. In general, the effect of central cooling was relatively best (SMD = 0.43, 95% CI 0.27 to 0.58, p < 0.001), followed by central and peripheral cooling (SMD = 0.39, 95% CI 0.23 to .55, p < 0.001), and the effect of peripheral cooling was the relatively worst (SMD = 0.32, 95% CI 0.07 to 0.57, p = 0.013).
Effects of central cooling on athletic performance
In a meta-analysis including 34 experiments, we found that central cooling interventions significantly enhanced sport performance in the heat. The pooled effect size was significant with small ES (SMD = 0.43, 95% CI 0.27 to 0.58, p < 0.001, Figure 2) and no heterogeneity (I2 = 0.0%, p = 0.776). The funnel plot (Figures 3A) and Egger’s test (t = 2.61, p = 0.014) indicated that there was publication bias on these results. Processed by the Trim and Fill method, analysis results showed that seven studies were added and the pooled ES was robust (SMD = 0.527, 95% CI 0.384 to .671, p < 0.001) compared to before trim and fill.
Sub-group analysing for the four exercise protocols (IS, ETE, EWT and TT) showed that central cooling interventions had small, positive and statically insignificant effects on IS (SMD = 0.22, 95% CI -0.09 to 0.53, p = 0.165) without heterogeneity (I2 = 0.0%, p = 0.999); moderate, positive and statically significant effects on ETE (SMD = 0.80, 95% CI 0.42 to 1.18, p < 0.001) with low heterogeneity (I2 = 38.9%, p = 0.090); small, positive and statically insignificant effects on EWT (SMD = 0.31, 95% CI -0.00 to 0.61, p = 0.051) without heterogeneity (I2 = 0.0%, p = 0.991); small, positive and statically insignificant effects on TT (SMD = 0.34, 95% CI -0.05 to 0.74, p = 0.086) without heterogeneity (I2 = 0.0%, p = 0.989).
Effects of peripheral cooling on athletic performance
In a meta-analysis including 14 experiments, we found that peripheral cooling interventions significantly enhanced sport performance in the heat. The pooled effect size was significant with small ES (SMD = 0.32, 95% CI 0.07 to 0.57, p = 0.013, Figure 4) and no heterogeneity (I2 = 0.0%, p = 0.680). The funnel plot (Figures 3B) and Egger’s test (t = 4.37, p = 0.001) indicated that there was publication bias on these results. Processed by the Trim and Fill method, analysis results showed that one studies were added and the pooled ES was robust (SMD = 1.296, 95% CI 1.014 to 1.656, p = 0.038) compared to before trim and fill.
Sub-group analysing for the four exercise protocols (IS, ETE, EWT and TT) showed that peripheral cooling interventions had trivial, positive and statically insignificant effects on IS (SMD = 0.08, 95% CI -0.35 to 0.51, p = 0.714) without heterogeneity (I2 = 0.0%, p = 0.991); small, positive and statically insignificant effects on ETE (SMD = 0.37, 95% CI -0.28 to 1.02, p = 0.265) with low heterogeneity (I2 = 47.3%, p = 0.127); large, positive and statically significant effects on EWT (SMD = 0.86, 95% CI 0.13 to 1.59, p = 0.022) without heterogeneity (I2 = 0.0%, p = 0.894); small, positive and statically insignificant effects on TT (SMD = 0.41, 95% CI -0.09 to 0.91, p = 0.109) without heterogeneity (I2 = 0.0%, p = 0.815).
Effects of central and peripheral cooling on athletic performance
In a meta-analysis including 34 experiments, we found that central and peripheral cooling interventions significantly enhanced sport performance in the heat. The pooled effect size was significant with small ES (SMD = .39, 95% CI .23 to .55, p < 0.001, Figure 5) and low heterogeneity (I2 = 6.4%, p = 0.362). The funnel plot (Figures 3C) and Egger’s test (t = 2.87, p = 0.007) indicated that there was publication bias on these results. Processed by the Trim and Fill method, analysis results showed that no study was added and the pooled ES was robust (SMD = 1.468, 95% CI 1.260 to 1.709, p < 0.001) compared to before trim and fill.
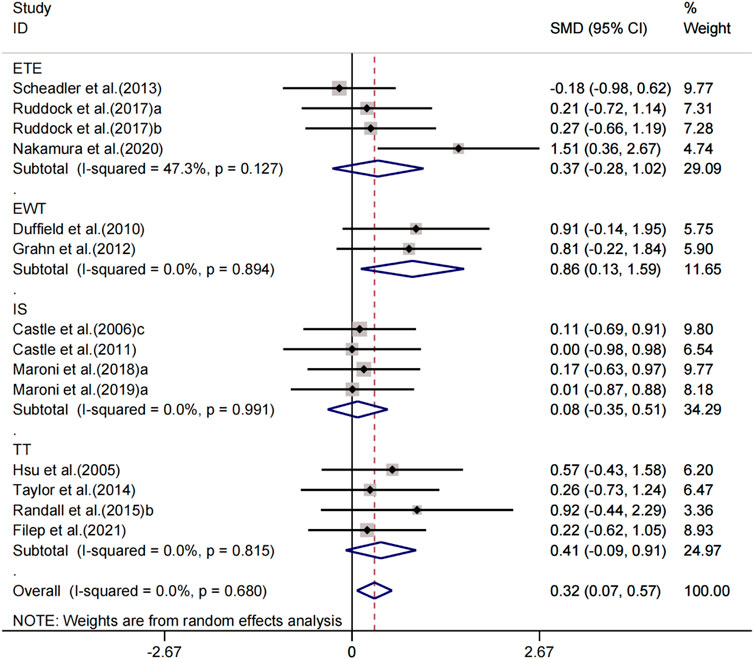
FIGURE 5. Forest plot regarding the effects of central and peripheral cooling on athletic performance
Sub-group analysing for the four exercise protocols (IS, ETE, EWT and TT) showed that central and peripheral cooling interventions had small, positive and statically significant effects on IS (SMD = 0.33, 95% CI 0.07 to 0.59, p = 0.012) without heterogeneity (I2 = 0.0%, p = 0.798); large, positive and statically significant effects on ETE (SMD = 0.86, 95% CI 0.40 to 1.32, p < 0.001) with low heterogeneity (I2 = 28.3%, p = 0.233); small, positive and statically significant effects on EWT (SMD = 0.35, 95% CI -0.05 to 0.74, p = 0.086) with low heterogeneity (I2 = 33.7%, p = 0.159); small, positive and statically insignificant effects on TT (SMD = 0.24, 95% CI -0.06 to 0.53, p = 0.115) without heterogeneity (I2 = 0.0%, p = 0.682).
Discussion
Effects of central cooling on athletic performance
Central cooling showed the optimal effects on the athletic performance, compared to the other two cooling strategies, on the basis of the research results of this study. Central cooling can be further divided into torso cooling and neck and head cooling by different functional characteristics. On the one hand, torso cooling, such as cooling vest, has a beneficial effect on skin temperatures, resulting in improved exercise performance (Ross et al., 2013; Chaen et al., 2019). During strenuous exercise in the heat, there exists a fierce competition for blood flow between the skin and muscles to the extent that muscle blood flow can be impaired (González-Alonso et al., 1999), which may induce negative effects on supply of energy substrates and removal of metabolic waste, and ultimately lead to premature fatigue. The reduction in torso skin temperature can decrease the peripheral skin blood flow and increase blood supply to the skeletal muscle (James et al., 2015) via superficial blood vessel constriction, and expand the heat gradient from the core to the skin to help promoting heat loss and reducing cardiovascular strain (Cheuvront et al., 2010). Mitigation of competition pressure between the skin and muscles, possibly allowing better maintenance of venous return, cardiac output and mean arterial pressure, and greater blood flow perfusion of the active muscle, thereby boosting oxygen delivery or metabolite removal, and increasing the contribution of aerobic metabolism to ATP re-phosphorylation (Marsh and Sleivert, 1999; Cotter et al., 2001). On the other hand, as neck and head are adjacent to the temperature-regulating centers and high sensory thermoreceptor, which dominate whole-body temperature perception (Cotter and Taylor, 2005; Kim et al., 2019), neck and head cooling could alter the sensory feedback and attenuate the inhibitory effect on neural drive (Watkins et al., 2018), thereby changing thermal sensation, mitigating central fatigue and improving exercise performance in the heat (Chaen et al., 2019; Cao et al., 2022). However, central cooling seems not to be able to significantly alter the physiological or peripheral neuroendocrinological responses to the exercise in the heat (Tyler et al., 2010). Based on above evidences, we concluded that central cooling could improve exercise performance with improvements of skin temperature and thermal sensation, which allowed the athletes to tolerate higher core temperature and HR induced by high performance in the heat (Tyler and Sunderland, 2011).
In terms of the effects on different sports types, the ranking as follows: ETE, TT, EWT and IS. Duffield and Marino (2007) reported that it is possible that the effects of pre-cooling (ice-vest and ice-bath) on IS performance are minor and only manifest when the thermal and exercise stress is sufficient to induce heat strain. Therefore, it seems that the extent of heat strain during exercise in the heat could influence the effects of central cooling. Because that ETE requires athletes exercise at a fixed load until exhaustion without interval recovery, stimulating the physiological limits of athletes, which may elicit greater thermoregulatory and physiological strain, ETE is more likely to gain more benefits from central cooling through improvements from skin temperature and thermal sensation. By contrast, as the intensity and duration of other three exercise type (TT, EWT and IS) are optional, it is difficult to guarantee that athletes suffer sufficient heat strain and even approach to their physiological limits. Moreover, different from the continuous exercise of ETE, IS allows athletes to have interval-recovery, which may further weaken the dependence on mitigating heat strain through central cooling. Hence, it is suggested that the implementation of central cooling depends on the situation, and this method can bring more benefits to the exercise with more heat strain, such as ETE.
Effects of peripheral cooling on athletic performance
Peripheral cooling showed the least effects on the athletic performance, compared to the other two cooling strategies, on the basis of the research results of this study. The critical limiting factor for exercise performance in the heat is an elevated core body temperature (Maughan, 2010), and accumulation of body heat mostly comes from metabolic heat production from working muscle contraction (Taylor et al., 2014). Furthermore, research also showed that significantly shorter endurance times were found for the heated muscle, because that the accumulation of hydrogen ions in muscle caused partial inhibition of the rate controlling enzyme phosphofructokinase (Edwards et al., 1972). Castle et al. (2006) and Duffield et al. (2010) found that the effect of reducing muscle temperature in peripheral cooling was better than central cooling (Castle et al., 2006; Duffield et al., 2010). In addition, after peripheral cooling (hands and forearms cooling), the cooler blood returned to the central circulation (Livingstone et al., 1989), which helped to decrease thermal perception, attenuate an increase in body temperature, limit heat storage and lower cardiorespiratory and skin blood flow demands (Ruddock et al., 2017). Nevertheless, the results of both Castle et al. (2006) and Maroni et al. (2018) showed that both central cooling and peripheral cooling could effectively diminish the core temperature compared with passive non-cooling intervention, and central cooling was even slightly better, but no significant difference between the cooling effects on two intervention methods. Ruddock et al. (2017) also pointed out that the majority of per-cooling methods had limited influence on body temperature and performance improvements might be attributed to a lower perception of heat strain, a mismatch between thermal perception and thermal strain might impair performance and as exercise continued accelerated hyperthermia-mediated fatigue. In addition, as the sites of peripheral cooling is far from the temperature-regulating centers and high sensory thermoreceptor, the effects of mitigating thermal perception may be weakened. Hence, we deduced that the major difference between peripheral cooling and central cooling might lie in the degree of improvements in thermal perception, which caused the difference in the effects on the athletic performance.
In terms of the effects on different sports types, the ranking as follows: EWT, TT, ETE and IS. Sleivert et al. (2001) found that thighs cooling impaired peak and short-term (45s) high-intensity power outputs, as a decreased muscle temperature made direct effects on contractile function or anaerobic metabolism. Anaerobic glycolysis was less active in cooled muscle, and glycogenolysis was increased in warmed muscle (Febbraio et al., 1996). Moreover, cooling leads to changes in the activation of motor units in the working muscle, some slower motor units are recruited to sustain a given level of power output in order to compensate for the reduced power of the cooled contractile elements, which impairs maximal power output (Sleivert et al., 2001). Whereas, when a short warm-up exercise and thighs cooling were combined, detrimental effects of a cold muscle on performance would be removed by increasing muscle temperature (Sleivert et al., 2001). Thus, it was suggested that direct cooling on the working muscle might influence the subsequent high-intensity exercise performance and sufficient re-warming was required (Chaen et al., 2019). Therefore, we deduced that enhancement effects of peripheral cooling required a threshold for muscle temperature, and long term sustained endurance exercises, such as ETE, with sufficient heat strains were more likely to be strengthen when combined with peripheral cooling. In the contrast, as IS allowed athletes to have a rest to relieve hyperthermia and had certain requirements for power output, the application of peripheral cooling in IS should be treated with caution, which was also consistent with the results of this study. Because that the intensity and duration of TT and EWT are optional, which requires different characteristics of energy metabolism, it is uncertain that the exact effects of peripheral cooling on them, so future research is needed to thoroughly investigate this issue. It is suggested that developing specific peripheral cooling and warm-up protocols to prompt performance, according to the intensity and duration of exercise.
Effects of central and peripheral cooling on athletic performance
The results of this study showed that central and peripheral cooling was better than peripheral cooling but not as good as central cooling. Minett et al. (2011) found that 20 min of whole-body pre-cooling was more effective than head refreshing alone in intermittent sprint bouts. However, Maroni et al. (2019) denoted that there were no differences in performance when comparing a hand-cooling glove technique with a cooling jacket or a combination of both during high intensity prolonged repeated-sprint efforts in cyclists. As a result, whether a mixed external cooling method is better than an isolated cooling technique is still uncertain. Nevertheless, we inferred that central and peripheral cooling seemed to retain central cooling’s advantages of reducing physiological stress and relieving thermal perception, because that both of them showed the better promotion effects on ETE than other exercise types. Based on the previous analysis, the effects of peripheral cooling seems to be variable and may be influenced by many factors, such as initial muscle temperature, cooling temperature and so on, thus some inappropriate operations can offset the positive effects from central cooling, which leads to the effects and mechanism of the central and peripheral cooling method is uncertain.
Limitations
The main limitation of our study is the lack of the changes in physiological parameters after different cooling interventions. This would help us to further understand how the improvements from nervous system, cardiovascular system, skeletal muscle system and other human systems or tissues transfer into athletic performance in the heat. The second limitation lies on the division of cooling placements is not detailed enough, central cooling could be further divided into face, neck, head or torso cooling, peripheral cooling also could be further divided into upper limbs or lower limbs cooling. However, because there are so many types of cooling placements, it is extremely difficult to explore the effectiveness of all types of external cooling, this article only divides external cooling into three categories and makes a rough comparison between them. Another limitation may be that the different intensity and duration of exercise need specific peripheral cooling and warm-up protocol, hence it is difficult to precisely investigate the cooling effects on these four exercise type, and even underlying mechanism behind them. In the future, the above aspects need to be further explored to provide meaningful suggestions for coaches and athletes.
Conclusion
On the basis of the results of this meta-analysis, central cooling appears to be an more effective intervention to enhance performance in hot conditions, compared to other external cooling strategies. Central cooling can improve exercise performance with improvements of skin temperature and thermal sensation, which allows the athletes to tolerate higher physiological strains induced by high performance in the heat, and shows the best enhancement effect on ETE. The effects of peripheral cooling on IS are trivial, and the enhancement effects of peripheral cooling require sufficient re-warming, hence it is suggested that developing specific peripheral cooling and warm-up protocols to prompt performance, according to the intensity and duration of exercise. The effect of central and peripheral cooling is better than peripheral cooling but not as good as central cooling. Although, central and peripheral cooling seems to retain central cooling’s advantages of reducing physiological stress and relieving thermal perception, and both of them show the better promotion effects on ETE, as many factors may influence the effects of peripheral cooling, thus some inappropriate operations can offset the positive effects from central cooling. Hence, in the future, more researchs are needed to further explore whether a mixed external cooling method is better than an isolated cooling technique.
Data availability statement
The original contributions presented in the study are included in the article/Supplementary Material, further inquiries can be directed to the corresponding author.
Ethics statement
The studies involving human participants were reviewed and approved by Ethics Committee of Beijing Sport University. The patients/participants provided their written informed consent to participate in this study. Written informed consent was obtained from the individual (s), and minor (s)’ legal guardian/next of kin, for the publication of any potentially identifiable images or data included in this article.
Author contributions
DJ and ML designed the study. DJ, QY, and JD performed literature searches, conducted study selection, data extraction, and study quality assessment. DJ and ML performed the statistical analysis, and all authors contributed to data interpretation. ML provided methodological insight and guidance throughout the process. DJ drafted the manuscript, while QY, ML, and JD critically revised the article and contributed to the final version of the manuscript. All authors gave their final approval of the version published.
Conflict of interest
The authors declare that the research was conducted in the absence of any commercial or financial relationships that could be construed as a potential conflict of interest.
Publisher’s note
All claims expressed in this article are solely those of the authors and do not necessarily represent those of their affiliated organizations, or those of the publisher, the editors and the reviewers. Any product that may be evaluated in this article, or claim that may be made by its manufacturer, is not guaranteed or endorsed by the publisher.
Supplementary material
The Supplementary Material for this article can be found online at: https://www.frontiersin.org/articles/10.3389/fphys.2022.1091228/full#supplementary-material
References
Abbass K., Qasim M. Z., Song H., Murshed M., Mahmood H., Younis I. (2022). A review of the global climate change impacts, adaptation, and sustainable mitigation measures. Environ. Sci. Pollut. Res. 29, 42539–42559. doi:10.1007/s11356-022-19718-6
Alhadad S. B., Tan P. M., Lee J. K. (2019). Efficacy of heat mitigation strategies on core temperature and endurance exercise: A meta-analysis. Front. physiology 10, 71. doi:10.3389/fphys.2019.00071
Altman D. G., Bland J. M. (2005). Standard deviations and standard errors. Bmj 331 (7521), 903. doi:10.1136/bmj.331.7521.903
Azad A., Mousavi M., Gorzi A., Ghasemnian A. (2016). The effect of precooling on exhaustive performance in the hot environment. Asian J. Sports Med. 7 (3), e33125. doi:10.5812/asjsm.33125
Berggren G., Hohwu Christensen E. (1950). Heart rate and body temperature as indices of metabolic rate during work. Arbeitsphysiologie 14, 255–260. doi:10.1007/bf00933843
Bogerd N., Perret C., Bogerd C. P., Rossi R. M., Daanen H. A. M. (2010). The effect of pre-cooling intensity on cooling efficiency and exercise performance. J. Sports Sci. 28 (7), 771–779. doi:10.1080/02640411003716942
Bongers C. C., Hopman M. T., Eijsvogels T. M. (2017). Cooling interventions for athletes: An overview of effectiveness, physiological mechanisms, and practical considerations. Temperature 4 (1), 60–78. doi:10.1080/23328940.2016.1277003
Borenstein M., Hedges L. V., Higgins J. P., Rothstein H. R. (2021). Introduction to meta-analysis. John Wiley & Sons.
Bouchama A., Knochel J. P. (2002). Heat stroke. N. Engl. J. Med. 346 (25), 1978–1988. doi:10.1056/nejmra011089
Brade C., Dawson B., Wallman K. (2014). Effects of different precooling techniques on repeat sprint ability in team sport athletes. Eur. J. Sport Sci. 14, S84–S91. doi:10.1080/17461391.2011.651491
Cao Y., Lei T. H., Wang F., Yang B., Mündel T. (2022). Head, face and neck cooling as per-cooling (cooling during exercise) modalities to improve exercise performance in the heat: A narrative review and practical applications. Sports Medicine-Open 8 (1), 16–17. doi:10.1186/s40798-022-00411-4
Casa D. J., Armstrong L. E., Ganio M. S., Yeargin S. W. (2005). Exertional heat stroke in competitive athletes. Curr. sports Med. Rep. 4 (6), 309–317. doi:10.1097/01.csmr.0000306292.64954.da
Cashin A. G., McAuley J. H. (2019). Clinimetrics: Physiotherapy evidence database (pedro) scale. J. Physiother. 66 (1), 59. doi:10.1016/j.jphys.2019.08.005
Castle P. C., Macdonald A. L., Philp A., Webborn A., Watt P. W., Maxwell N. S. (2006). Precooling leg muscle improves intermittent sprint exercise performance in hot, humid conditions. J. Appl. Physiol. 100 (4), 1377–1384. doi:10.1152/japplphysiol.00822.2005
Castle P., Mackenzie R. W., Maxwell N., Webborn A. D. J., Watt P. W. (2011). Heat acclimation improves intermittent sprinting in the heat but additional pre-cooling offers no further ergogenic effect. J. Sports Sci. 29 (11), 1125–1134. doi:10.1080/02640414.2011.583673
Chaen Y., Onitsuka S., Hasegawa H. (2019). Wearing a cooling vest during half-time improves intermittent exercise in the heat. Front. Physiology 10, 711. doi:10.3389/fphys.2019.00711
Chan A. P. C., Yang Y., Wong F. K. W., Yam M. C. H., Wong D. P., Song W. F. (2019). Reduction of physiological strain under a hot and humid environment by a hybrid cooling vest. J. Strength Cond. Res. 33 (5), 1429–1436. doi:10.1519/jsc.0000000000001837
Cheuvront S. N., Kenefick R. W., Montain S. J., Sawka M. N. (2010). Mechanisms of aerobic performance impairment with heat stress and dehydration. J. Appl. physiology 109 (6), 1989–1995. doi:10.1152/japplphysiol.00367.2010
Choo H. C., Peiffer J. J., Lopes-Silva J. P., Mesquita R. N. O., Amano T., Kondo N., et al. (2019). Effect of ice slushy ingestion and cold water immersion on thermoregulatory behavior. PLoS One 14 (2), e0212966. doi:10.1371/journal.pone.0212966
Clarke N. D., Maclaren D. P. M., Reilly T., Drust B. (2011). Carbohydrate ingestion and pre-cooling improves exercise capacity following soccer-specific intermittent exercise performed in the heat. Eur. J. Appl. Physiology 111 (7), 1447–1455. doi:10.1007/s00421-010-1771-5
Coelho L. G. M., Ferreira-Júnior J. B., Williams T. B., Maia-Lima A., Borba D. A., Silva C. D., et al. (2021). Head pre-cooling improves 5-km time-trial performance in male amateur runners in the heat. Scand. J. Med. Sci. Sports 31 (9), 1753–1763. doi:10.1111/sms.13985
Cotter J. D., Sleivert G. G., Roberts W. S., Febbraio M. A. (2001). Effect of pre-cooling, with and without thigh cooling, on strain and endurance exercise performance in the heat. Comp. Biochem. Physiology Part A Mol. Integr. Physiology 128 (4), 667–677. doi:10.1016/S1095-6433(01)00273-2
Cotter J. D., Taylor N. A. (2005). The distribution of cutaneous sudomotor and alliesthesial thermosensitivity in mildly heat-stressed humans: An open-loop approach. J. physiology 565 (1), 335–345. doi:10.1113/jphysiol.2004.081562
Cuttell S. A., Kiri V., Tyler C. (2016). A comparison of 2 practical cooling methods on cycling capacity in the heat. J. Athl. Train. 51 (7), 525–532. doi:10.4085/1062-6050-51.8.07
De Morton N. A. (2009). The PEDro scale is a valid measure of the methodological quality of clinical trials: A demographic study. Aust. J. Physiother. 55 (2), 129–133. doi:10.1016/S0004-9514(09)70043-1
De Pauw K., Roelands B., Vanparijs J., Meeusen R. (2014). Effect of recovery. Interventions on cycling performance and pacing strategy in the heat. Int. J. Sports Physiology Perform. 9 (2), 240–248. doi:10.1123/ijspp.2012-0366
Drinkwater E. (2008). Effects of peripheral cooling on characteristics of local muscle. Thermoregulation Hum. Perform. 53, 74–88. doi:10.1159/000151551
Duffield R., Bird S. P., Ballard R. J. (2011). Field-based pre-cooling for on-court tennis conditioning training in the heat. J. Sports Sci. Med. 10 (2), 376–384. https://www.jssm.org/jssm-10-376.xml%3EFulltext.
Duffield R., Green R., Castle P., Maxwell N. (2010). Precooling can prevent the reduction of self-paced exercise intensity in the heat. Med. Sci. Sports Exerc. 42 (3), 577–584. doi:10.1249/MSS.0b013e3181b675da
Duffield R., Marino F. E. (2007). Effects of pre-cooling procedures on intermittent-sprint exercise performance in warm conditions. Eur. J. Appl. physiology 100 (6), 727–735. doi:10.1007/s00421-007-0468-x
Duffield R., Steinbacher G., Fairchild T. J. (2009). The use of mixed-method, part-body pre-cooling procedures for team-sport athletes training in the heat. J. Strength Cond. Res. 23 (9), 2524–2532. doi:10.1519/JSC.0b013e3181bf7a4f
Duval S., Tweedie R. (2000). Trim and fill: A simple funnel-plot–based method of testing and adjusting for publication bias in meta-analysis. Biometrics 56 (2), 455–463. doi:10.1111/j.0006-341X.2000.00455.x
Edwards R. H. T., Harris R. C., Hultman E., Kaijser L., Koh D., Nordesjö L. O. (1972). Effect of temperature on muscle energy metabolism and endurance during successive isometric contractions, sustained to fatigue, of the quadriceps muscle in man. J. physiology 220 (2), 335–352. doi:10.1113/jphysiol.1972.sp009710
Faulkner S. H., Broekhuijzen I., Raccuglia M., Hupperets M., Hodder S. G., Havenith G. (2019). The threshold ambient temperature for the use of precooling to improve cycling time-trial performance. Int. J. Sports Physiology Perform. 14 (3), 323–330. doi:10.1123/ijspp.2018-0310
Faulkner S. H., Hupperets M., Hodder S. G., Havenith G. (2015). Conductive and evaporative precooling lowers mean skin temperature and improves time trial performance in the heat. Scand. J. Med. Sci. Sports 25, 183–189. doi:10.1111/sms.12373
Febbraio M. A., Carey M. F., Snow R. J., Stathis C. G., Hargreaves M. (1996). Influence of elevated muscle temperature on metabolism during intense, dynamic exercise. Am. J. Physiology-Regulatory, Integr. Comp. Physiology 271 (5), R1251–R1255. doi:10.1152/ajpregu.1996.271.5.R1251
Filep E. M., Szymanski M. R., Navarro J. S., Nye M., Dierickx E. E., Casa D. J. (2021). Effects of forearm cooling on thermal responses and exercise performance during intermittent exercise in the heat: 1076. Med. Sci. Sports Exerc. 53 (8S), 349. doi:10.1249/01.mss.0000763296.44189.53
Gonzales B. R., Hagin V., Guillot R., Placet V., Monnier-Benoit P., Groslambert A. (2014). Self-paced cycling performance and recovery under a hot and highly humid environment after cooling. J. Sports Med. Phys. Fit. 54 (1), 43–52. Available at: https://serval.unil.ch/resource/serval:BIB_2280B9E22E90.P001/REF.pdf.
González-Alonso J., Teller C., Andersen S. L., Jensen F. B., Hyldig T., Nielsen B. (1999). Influence of body temperature on the development of fatigue during prolonged exercise in the heat. J. Appl. physiology 86 (3), 1032–1039. doi:10.1152/jappl.1999.86.3.1032
Grahn D. A., Cao V. H., Nguyen C. M., Liu M. T., Heller H. C. (2012). Work volume and strength training responses to resistive exercise improve with periodic heat extraction from the palm. J. Strength Cond. Res. 26 (9), 2558–2569. doi:10.1519/JSC.0b013e31823f8c1a
Grissom R. J., Kim J. J. (2005). Effect sizes for research: A broad practical approach. Lawrence Erlbaum Associates Publishers.
Hasegawa H., Takatori T., Komura T., Yamasaki M. (2006). Combined effects of pre-cooling and water ingestion on thermoregulation and physical capacity during exercise in a hot environment. J. sports Sci. 24 (1), 3–9. doi:10.1080/02640410400022185
Hasegawa H., Takatori T., Komura T., Yamasaki M. (2005). Wearing a cooling jacket during exercise reduces thermal strain and improves endurance exercise performance in a warm environment. J. Strength Cond. Res. 19 (1), 122–128. doi:10.1519/14503.1
J. P. Higgins, J. Thomas, J. Chandler, M. Cumpston, T. Li, M. J. Pageet al. (Editors) (2019). Cochrane handbook for systematic reviews of interventions (John Wiley & Sons).
Higgins J. P., Thompson S. G., Deeks J. J., Altman D. G. (2003). Measuring inconsistency in meta-analyses. Bmj 327 (7414), 557–560. doi:10.1136/bmj.327.7414.557
Hohenauer E., Stoop R., Clarys P., Clijsen R., Deliens T., Taeymans J. (2018). The effect of pre-exercise cooling on performance characteristics: A systematic review and meta-analysis. Int. J. Clin. Med. 9 (3), 117–141. doi:10.4236/ijcm.2018.93012
Hsu A. R., Hagobian T. A., Jacobs K. A., Attallah H., Friedlander A. L. (2005). Effects of heat removal through the hand on metabolism and performance during cycling exercise in the heat. Can. J. Appl. physiology 30 (1), 87–104. doi:10.1139/h05-107
James C. A., Richardson A. J., Watt P. W., Gibson O. R., Maxwell N. S. (2015). Physiological responses to incremental exercise in the heat following internal and external precooling. Scand. J. Med. Sci. Sports 25, 190–199. doi:10.1111/sms.12376
James C. A., Richardson A. J., Watt P. W., Willmott A. G. B., Gibson O. R., Maxwell N. S. (2018). Short-term heat acclimation and precooling, independently and combined, improve 5-km time trial performance in the heat. J. Strength Cond. Res. 32 (5), 1366–1375. doi:10.1519/JSC.0000000000001979
Katica C. P., Wingo J. E., Herron R. L., Ryan G. A., Bishops S. H., Richardson M. (2018). Impact of upper body precooling during warm-up on subsequent time trial paced cycling in the heat. J. Sci. Med. Sport 21 (6), 621–625. doi:10.1016/j.jsams.2017.10.007
Kim J. H., Seo Y., Quinn T., Yorio P., Roberge R. (2019). Intersegmental differences in facial warmth sensitivity during rest, passive heat and exercise. Int. J. Hyperth. 36 (1), 654–659. doi:10.1080/02656736.2019.1627430
Liao K. F., Nassis G., Bishop C., Yang W., Bian C., Li Y. M. (2021). Effects of unilateral vs. bilateral resistance training interventions on measures of strength, jump, linear and change of direction speed: A systematic review and meta-analysis. Biol. Sport 39 (3), 485–497. doi:10.5114/biolsport.2022.107024
Liberati A., Altman D. G., Tetzlaff J., Mulrow C., Gøtzsche P. C., Ioannidis J. P., et al. (2009). The PRISMA statement for reporting systematic reviews and meta-analyses of studies that evaluate health care interventions: Explanation and elaboration. J. Clin. Epidemiol. 62 (10), e1–e34. doi:10.1016/j.jclinepi.2009.06.006
Livingstone S. D., Nolan R. W., Cattroll S. W. (1989). Heat loss caused by immersing the hands in water. Aviat. space, Environ. Med. 60 (12), 1166–1171. https://pubmed.ncbi.nlm.nih.gov/2604672/.
Luomala M. J., Oksa J., Salmi J. A., Linnamo V., Holmér I., Smolander J., et al. (2012). Adding a cooling vest during cycling improves performance in warm and humid conditions. J. Therm. Biol. 37 (1), 47–55. doi:10.1016/j.jtherbio.2011.10.009
Maher C. G., Sherrington C., Herbert R. D., Moseley A. M., Elkins M. (2003). Reliability of the PEDro scale for rating quality of randomized controlled trials. Phys. Ther. 83 (8), 713–721. doi:10.1093/ptj/83.8.713
Maia-Lima A., Ramos G. P., Moraes M. M., Pacheco D. A. S., de Oliveira G. G. A., de Barros C. L. M., et al. (2017). Effects of precooling on 30-km cycling performance and pacing in hot and temperate environments. Int. J. Sports Med. 38 (01), 48–54. doi:10.1055/s-0042-113465
Maroni T., Dawson B., Dennis M., Naylor L., Brade C., Wallman K. (2018). Effects of half-time cooling using A cooling glove and jacket on manual dexterity and repeated-sprint performance in heat. J. Sports Sci. Med. 17 (3), 485–491. https://www.jssm.org/jssm-17-485.xml%3EFulltext.
Maroni T., Dawson B., Landers G., Naylor L., Wallman K. (2020). Hand and torso pre-cooling does not enhance subsequent high-intensity cycling or cognitive performance in heat. Temperature 7 (2), 165–177. doi:10.1080/23328940.2019.1631731
Marsh D., Sleivert G. (1999). Effect of precooling on high intensity cycling performance. Br. J. sports Med. 33 (6), 393–397. doi:10.1136/bjsm.33.6.393
Maughan R. J. (2010). Distance running in hot environments: A thermal challenge to the elite runner. Scand. J. Med. Sci. sports 20, 95–102. doi:10.1111/j.1600-0838.2010.01214.x
Minett G. M., Duffield R., Marino F. E., Portus M. (2012). Duration-dependant response of mixed-method pre-cooling for intermittent-sprint exercise in the heat. Eur. J. Appl. Physiology 112 (10), 3655–3666. doi:10.1007/s00421-012-2348-2
Minett G. M., Duffield R., Marino F. E., Portus M. (2011). Volume-dependent response of precooling for intermittent-sprint exercise in the heat. Med. Sci. Sports Exerc. 43 (9), 1760–1769. doi:10.1249/MSS.0b013e318211be3e
Minniti A., Tyler C. J., Sunderland C. (2011). Effects of a cooling collar on affect, ratings of perceived exertion, and running performance in the heat. Eur. J. Sport Sci. 11 (6), 419–429. doi:10.1080/17461391.2010.536577
Morrison S. A., Cheung S., Cotter J. D. (2014). Importance of airflow for physiologic and ergogenic effects of precooling. J. Athl. Train. 49 (5), 632–639. doi:10.4085/1062-6050-49.3.27
Morrison S. F., Nakamura K. (2011). Central neural pathways for thermoregulation. Front. Biosci. a J. virtual Libr. 16, 74–104. doi:10.2741/3677
Moss J. N., Trangmar S. J., Mackenzie R. W. A., Tyler C. J. (2021). The effects of pre- and per-cooling interventions used in isolation and combination on subsequent 15-minute time-trial cycling performance in the heat. J. Sci. Med. Sport 24 (8), 800–805. doi:10.1016/j.jsams.2021.04.006
Munoz C. X., Carney K. R., Schick M. K., Coburn J. W., Becker A. J., Judelson D. A. (2012). Effects of oral rehydration and external cooling on physiology, perception, and performance in hot, dry climates. Scand. J. Med. Sci. Sports 22 (6), e115–e124. doi:10.1111/j.1600-0838.2012.01510.x
Naito T., Nakamura M., Muraishi K., Eda N., Ando K., Takemura A., et al. (2022). In-play optimal cooling for outdoor match-play tennis in the heat. Eur. J. Sport Sci. 22 (3), 326–335. doi:10.1080/17461391.2020.1870160
Nakamura D., Muraishi K., Hasegawa H., Yasumatsu M., Takahashi H. (2020). Effect of a cooling strategy combining forearm water immersion and a low dose of ice slurry ingestion on physiological response and subsequent exercise performance in the heat. J. Therm. Biol. 89, 102530. doi:10.1016/j.jtherbio.2020.102530
Osakabe J., Kajiki M., Kondo K., Matsumoto T., Umemura Y. (2021). Effects of half-time cooling using a fan with skin wetting on thermal response during intermittent cycling exercise in the heat. Sports Med. Int. Open 5 (03), E91–E98. doi:10.1055/a-1588-3126
Page M. J., McKenzie J. E., Bossuyt P. M., Boutron I., Hoffmann T. C., Mulrow C. D., et al. (2021). The PRISMA 2020 statement: An updated guideline for reporting systematic reviews. Syst. Rev. 10 (1), 89–11. doi:10.1186/s13643-021-01626-4
Peiffer J. J., Abbiss C. R., Watson G., Nosaka K., Laursen P. B. (2010). Effect of a 5-min cold-water immersion recovery on exercise performance in the heat. Br. J. sports Med. 44 (6), 461–465. doi:10.1136/bjsm.2008.048173
Randall C. A., Ross E. Z., Maxwell N. S. (2015). Effect of practical precooling on neuromuscular function and 5-km time-trial performance in hot, humid conditions among well-trained male runners. J. Strength Cond. Res. 29 (7), 1925–1936. doi:10.1519/jsc.0000000000000840
Rodríguez M. Á., Piedra J. V., Sánchez-Fernández M., Del Valle M., Crespo I., Olmedillas H. (2020). A matter of degrees: A systematic review of the ergogenic effect of pre-cooling in highly trained athletes. Int. J. Environ. Res. Public Health 17 (8), 2952. doi:10.3390/ijerph17082952
Ross M., Abbiss C., Laursen P., Martin D., Burke L. (2013). Precooling methods and their effects on athletic performance: A systematic review and practical applications. Sports Med. 43 (3), 207–225. doi:10.1007/s40279-012-0014-9
Ruddock A. D., Tew G. A., Purvis A. J. (2017). Effect of hand cooling on body temperature, cardiovascular and perceptual responses during recumbent cycling in a hot environment. J. Sports Sci. 35 (14), 1466–1474. doi:10.1080/02640414.2016.1215501
Saltin B., Hermansen L. (1966). Esophageal, rectal, and muscle temperature during exercise. J. Appl. physiology 21 (6), 1757–1762. doi:10.1152/jappl.1966.21.6.1757
Scheadler C. M., Saunders N. W., Hanson N. J., Devor S. T. (2013). Palm cooling does not improve running performance. Int. J. Sports Med. 34 (8), 732–735. doi:10.1055/s-0032-1327576
Schmit C., Le Meur Y., Duffield R., Robach P., Oussedik N., Coutts A. J., et al. (2017). Heat-acclimatization and pre-cooling: A further boost for endurance performance? Scand. J. Med. Sci. Sports 27 (1), 55–65. doi:10.1111/sms.12629
Skein M., Duffield R., Cannon J., Marino F. E. (2012). Self-paced intermittent-sprint performance and pacing strategies following respective pre-cooling and heating. Eur. J. Appl. Physiology 112 (1), 253–266. doi:10.1007/s00421-011-1972-6
Sleivert G. G., Cotter J. D., Roberts W. S., Febbraio M. A. (2001). The influence of whole-body vs. torso pre-cooling on physiological strain and performance of high-intensity exercise in the heat. Comp. Biochem. Physiology Part A Mol. Integr. Physiology 128 (4), 657–666. doi:10.1016/S1095-6433(01)00272-0
Smith C. R., Butts C. L., Adams J. D., Tucker M. A., Moyen N. E., Ganio M. S., et al. (2018). Effect of a cooling kit on physiology and performance following exercise in the heat. J. Sport Rehabilitation 27 (5), 413–418. doi:10.1123/jsr.2016-0116
Sunderland C., Stevens R., Everson B., Tyler C. J. (2015). Neck-cooling improves repeated sprint performance in the heat. Front. physiology 6, 314. doi:10.3389/fphys.2015.00314
Taylor L., Mauger A. R., Watkins S. L., Fitch N., Brewer J., Maxwell N. S., et al. (2014). Precooling does not improve 2, 000-m rowing performance of females in hot, humid conditions. J. Strength Cond. Res. 28 (12), 3416–3424. doi:10.1519/jsc.0000000000000558
Thomas G., Cullen T., Davies M., Hetherton C., Duncan B., Gerrett N. (2019). Independent or simultaneous lowering of core and skin temperature has no impact on self-paced intermittent running performancein hot conditions. Eur. J. Appl. Physiology 119 (8), 1841–1853. doi:10.1007/s00421-019-04173-y
Tyler C. J., Sunderland C. (2011). Cooling the neck region during exercise in the heat. J. Athl. Train. 46 (1), 61–68. doi:10.4085/1062-6050-46.1.61
Tyler C. J., Sunderland C. (2011). Neck cooling and running performance in the heat: Single versus repeated application. Med. Sci. Sports Exerc. 43 (12), 2388–2395. doi:10.1249/MSS.0b013e318222ef72
Tyler C. J., Wild P., Sunderland C. (2010). Practical neck cooling and time-trial running performance in a hot environment. Eur. J. Appl. Physiology 110 (5), 1063–1074. doi:10.1007/s00421-010-1567-7
Vaile J., Halson S., Gill N., Dawson B. (2008). Effect of cold water immersion on repeat cycling performance and thermoregulation in the heat. J. Sports Sci. 26 (5), 431–440. doi:10.1080/02640410701567425
Vaile J., O'Hagan C., Stefanovic B., Walker M., Gill N., Askew C. D. (2011). Effect of cold water immersion on repeated cycling performance and limb blood flow. Br. J. Sports Med. 45 (10), 825–829. doi:10.1136/bjsm.2009.067272
Vanos J. K., Kosaka E., Iida A., Yokohari M., Middel A., Scott-Fleming I., et al. (2019). Planning for spectator thermal comfort and health in the face of extreme heat: The Tokyo 2020 olympic marathons. Sci. Total Environ. 657, 904–917. doi:10.1016/j.scitotenv.2018.11.447
Vanos J., Kosaka E., Iida A., Yokohari M., Middel A., Scott-Fleming I., et al. (2018). Planning for spectator thermal comfort and health in the face of extreme heat: The Tokyo 2020 Olympic marathons. Sci. Total Environ. 657, 904–917. doi:10.1016/j.scitotenv.2018.11.447
Walters P., Thom N., Libby K., Edgren S., Azadian A., Tannous D., et al. (2017). The effect of intermittent head cooling on aerobic performance in the heat. J. Sports Sci. Med. 16 (1), 77–83. Available at: https://www.jssm.org/jssm-16-77.xml%3EFulltext.
Watkins E. R., Hayes M., Watt P., Richardson A. J. (2018). Practical pre-cooling methods for occupational heat exposure. Appl. Ergon. 70, 26–33. doi:10.1016/j.apergo.2018.01.011
Wendt D., Van Loon L. J., Marken Lichtenbelt W. D. (2007). Thermoregulation during exercise in the heat: Strategies for maintaining health and performance. Sports Med. 37 (8), 669–682. doi:10.2165/00007256-200737080-00002
Wohlfert T. M., Miller K. C. (2019). Precooling, exertional heatstroke risk factors, and postexercise cooling rates. Aerosp. Med. Hum. Perform. 90 (1), 12–17. doi:10.3357/amhp.5194.2019
Keywords: external cooling, cooling placements, athletic performance, heat, hot environment
Citation: Jiang D, Yu Q, Liu M and Dai J (2023) Effects of different external cooling placements prior to and during exercise on athletic performance in the heat: A systematic review and meta-analysis. Front. Physiol. 13:1091228. doi: 10.3389/fphys.2022.1091228
Received: 06 November 2022; Accepted: 28 December 2022;
Published: 10 January 2023.
Edited by:
Kazunobu Okazaki, Osaka City University, JapanReviewed by:
Masanobu Kajiki, University of Tsukuba, JapanAlex Buoite Stella, University of Trieste, Italy
Copyright © 2023 Jiang, Yu, Liu and Dai. This is an open-access article distributed under the terms of the Creative Commons Attribution License (CC BY). The use, distribution or reproduction in other forums is permitted, provided the original author(s) and the copyright owner(s) are credited and that the original publication in this journal is cited, in accordance with accepted academic practice. No use, distribution or reproduction is permitted which does not comply with these terms.
*Correspondence: Meng Liu, bGl1c2t5QGJzdS5lZHUuY24=
†These authors have contributed equally to this work and share first authorship