- 1Department of Combat Sports, Gdansk University of Physical Education and Sport, Gdansk, Poland
- 2Department of Athletics, Gdansk University of Physical Education and Sport, Gdansk, Poland
- 3University WSB Merito, Wrocław, Poland
- 4Department of Cognitive and Behavioral Sciences and Technology in Sport, Shahid Beheshti University, Teheran, Iran
- 5Department of Swimming, Gdansk University of Physical Education and Sport, Gdansk, Poland
- 6Department of Biomechanics and Sport Engineering, Gdansk University of Physical Education and Sport, Gdansk, Poland
- 7Department of Paralympic Sport, Wrocław University of Health and Sport Sciences, Wrocław, Poland
The main goal was to investigate the effectiveness of cryosauna in preventing the development of delayed onset muscle soreness and to analyze the regenerative changes within muscles after acute fatigue-induced exercises. Thirty-one volunteers were assigned into two groups: 1) an intervention group that participated in cryostimulation after fatigue-induced exercise protocol (CRYO, n = 16) and a control group that performed fatigue-induced exercise protocol, but without any intervention (CONT, n = 15). Main outcome measures include at baseline: blood sample testing (leukocyte content, myoglobin concentration, and creatine kinase activity) and muscle stiffness of lower extremity; immediately after (stiffness), and 24-48-72-96 h post-exercise (blood samples and stiffness). Both groups performed an exercise-induced muscle damage protocol based on repeated countermovement jumps (10 sets, 10 repetitions). The CRYO group underwent a cryosauna (temperature: −110°C, time: 1.5 min per session) intervention during four sessions (i.e., immediately after, 24-48-72 h post-exercise). Leukocyte content was significantly greater 24-48-72 h after exercise in CONT, compared with the CRYO group (p ≤ 0.05 for all), while creatine kinase activity was greater 24-48-96 h in CONT, compared with the CRYO group (p ≤ 0.05 for all). Muscle stiffness increased significantly in rectus femoris, tibialis anterior, and fibula muscle after 48 h post-exercise (p ≤ 0.05 for all), as well as in tibialis anterior and fibula after 72 h post-exercise (p ≤ 0.05 for all) in the CRYO group. Multiple cryosauna was an effective recovery strategy that reduced blood biomarkers and muscle stiffness after exercise-induced muscle damage. Moreover, the development of delayed onset muscle soreness, expressed by a greater muscle stiffness post-exercise, was attenuated to the first 48 h.
Introduction
Delayed onset muscle soreness (DOMS) has been defined as the initial type 1) of muscle strain occurring with accompanying pain sensations (Gulick and Kimura, 1996) and characterized by additional stiffness related to increased inflammation (MacIntyre et al., 2001). DOMS appears as early as 8 h after exercise in the form of muscle soreness, peaking between 24 and 48 h after exercises (Kawczyński et al., 2012; Argus et al., 2017), and then gradually relieves and disappears in about 5–7 days due to physiological changes in the muscle fibers. During the last several decades many theories were created to explain the mechanisms of DOMS, however the most recent focused on inflammation and enzyme efflux (Gulick and Kimura, 1996; Cheung et al., 2003). Currently, models integrate different theories together and form a consistent description of different physiological processes (Cheung et al., 2003). The exercise-induced muscle fiber damage may results in disruption of protein structures of a fiber followed by calcium imbalance. During the next few hours neutrophils increases their concentration causing inflammation (Smith, 1991). The increase in creatine kinase (CK), an marker of connective tissue and muscle damage, causes increase in mast cells and histamine within the next 6–12 h. Moreover, macrophages reach the maximal number within 48 h and produce prostaglandin that affects mechanical and chemical stimulation of type III and IV nerve). Finally, these processes may result in an increase of temperature, which might be an additional symptom of inflammation (Cheung et al., 2003). This leads to muscle stiffness and consequently causes pain that can alter the neuromuscular response during movement and impair the athlete’s performance and training program (Hotfiel et al., 2018; Seco-Calvo et al., 2020).
Muscle damage and soreness are common conditions experienced by athletes and individuals who engage in strenuous exercise. Therefore, several interventions have been suggested to prevent the effects of DOMS. Recently, cryotherapy has emerged as a promising method for reducing DOMS and enhancing post-exercise recovery. There are several cold therapy methods used by athletes: cold water immersion (CWI), contrast water therapy (CWT), whole-body cryotherapy (WBC), and cryosauna (Jajtner et al., 2015; Argus et al., 2017; Lombardi et al., 2017; Seco-Calvo et al., 2020; Miranda et al., 2021). Cryotherapy involves exposing the body to cold temperatures to induce physiological responses that promote healing and regeneration by reducing inflammation, increasing blood flow, and, finally, enhancing muscle recovery (Banfi et al., 2009; Pournot et al., 2011; Siqueira et al., 2018). One of the most significant effects of cryogenic temperatures is the analgesic effect connected with influence on the endocrine system, such as increased secretion of 𝛽-endorphins, and metabolic action manifested by decreased concentrations of histamine and lactate in changed inflammatory tissues. These effects are thought to be mediated by the activation of various cellular and molecular pathways, including the release of anti-inflammatory cytokines and growth factors, as well as the impact of cryogenic temperatures on the prooxidant-antioxidant balance and stabilization of lysosome membranes and subsequent inhibition of release of active enzymes from lysosomes (Rose et al., 2017; Miranda et al., 2021). The physiological mechanism of action of cryogenic temperatures might be related to the effect on the vasomotor response of the small blood vessels in the skin during and after cryostimulation (Olson and Stravino, 1972) and the mechanism of ischemia and reperfusion (Pumtel et al., 2013). Previous studies have demonstrated the beneficial effect of different cryotherapy methods (e.g., ice massage, CWI, CWT, and WBC) on DOMS, especially in the reduction of post-exercise pressure pain threshold (Klich et al., 2018) and blood biomarkers concentration and activity (Eston and Peters, 1999; Goodall et al., 2008; Machado et al., 2017; Siqueira et al., 2018). Moreover, previous studies reported that a multiple-session intervention of cryotherapy during 72–168 h post-exercise may significantly lead to a decrease in creatine kinase, C-reactive protein, and inflammatory cytokines concentration compared to a non-intervention group (Eston and Peters, 1999; Goodall et al., 2008; Siqueira et al., 2018). However, it should be noted that some studies have not shown any beneficial effects on inflammation, muscle soreness, and stiffness after local cryotherapy (Behringer et al., 2018) and CWI (Pinto et al., 2020).
Despite its growing popularity, the effectiveness of cryotherapy and cryosauna for DOMS relief is still a topic of debate in the scientific community. Cryosauna is a type of partial-body cryotherapy that might be used in sports regeneration because of a lower temperature (up to −160°C) at the initial stage compared with WBC. The lower temperature is caused by a direct contact between the medium and the user. Additionally, it should be noted that cryosauna has no vestibule chamber (Piotrowska, 2017). Furthermore, the mechanisms by which cryotherapy exerts its effects on DOMS are not fully understood (Lombardi et al., 2017). There are a few studies that have investigated the effect of a series of whole-body cryotherapy for athletes using a cryo-chamber or cryosauna based on changes in biochemical blood indices and pain evaluation (Hausswirth et al., 2011; Pournot et al., 2011; Kozłowska-Flis et al., 2021).
To our knowledge, no study has addressed the effectiveness of cryosauna in the prevention of DOMS by the determination of changes in the levels of leukocyte content, myoglobin concentration, creatine kinase activity with the quantified measurements of muscle stiffness. Therefore, in the present study, we have focused not only on changes in biochemical blood indices but also on the properties of the muscles themselves, as an increase in muscle stiffness is one of the main symptoms of DOMS, which can increase the risk of injury and reduce exercise capacity. Moreover, our research has been extended to 96 h long, and we looked at biochemical indices and muscle stiffness at 24, 48,72, and 96 h after exercise, when most studies describing the effects of cold therapy after exercise monitored its effectiveness for up to 48 h (Lombardi et al., 2017). Although, as recommended in the literature, all cryotherapy sessions were performed up to 72 h after a counter-movement jumps (CMJ) series due to the duration of the destruction phase of muscle regeneration (Rose et al., 2017). Therefore our goals were to investigate the effectiveness of cryosauna in preventing the development of DOMS and to analyze the regenerative changes within muscles after acute fatigue-induced exercises. We hypothesized that multiple cryosauna sessions would reduce the effect of DOMS within 96 h post-exercise by a decrease of blood biomarkers of muscle damage and optimization of muscle stiffness (Eston and Peters, 1999; Goodall et al., 2008; Point et al., 2018; Siqueira et al., 2018).
Materials and methods
Participants
The eligible population of thirty-six healthy and active students who specialized in martial arts volunteered in this study. A detailed description of participant demographics and performance parameters is included in Table 1. All the participants had training experience in martial arts (judo and karate) of 12 ± 4 years and a training duration of 10 ± 2 h per week. The weekly training routine included aerobic, strength, and specific martial arts training. The participants were asked to resign from training and avoid strenuous physical activity 48 h before data collection. Inclusion criteria were: 1) no previous experience with cryostimulation using a cryosauna, 2) no supplementation or prescribe drugs, and 3) recreational lifestyle. The exclusion criteria included: 1) current or prior pain or injury in the lower extremity; 2) prior history of surgery in the lower extremity, and 3) cardiovascular diseases. All participants were fully informed about the procedures, read and signed written informed consent, and agreed to participate in this study.
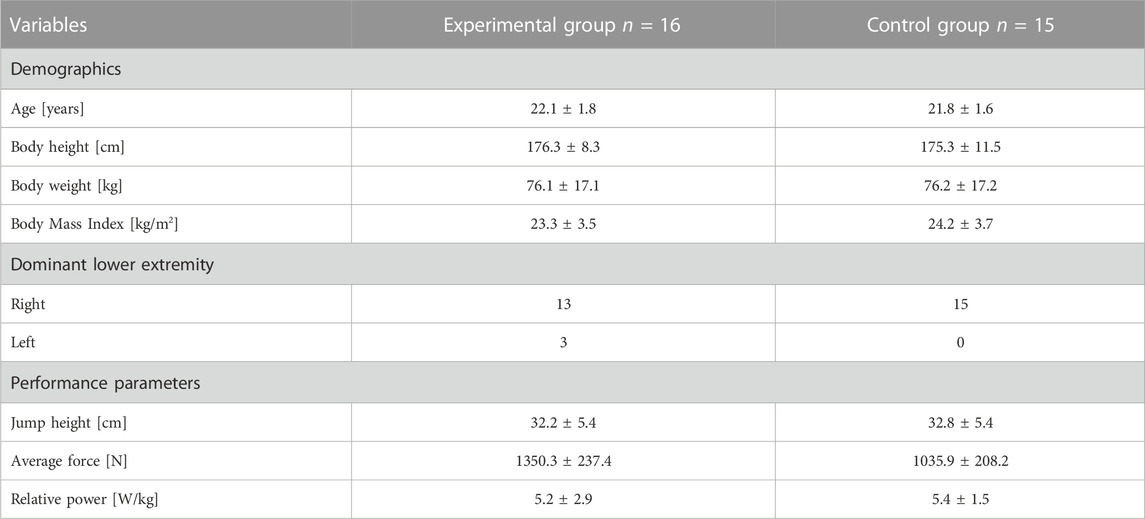
TABLE 1. Participant demographics and performance parameters of mean and standard deviation in both groups.
Study design
This study design was a randomized, controlled, single-blind trial with repeated measures. Moreover, the design was prepared according to the Consolidated Standards of Reporting Trials (CONSORT) for pragmatic trials. During the randomization, one participant was excluded from this study because have not met the inclusion criteria. The allocation of groups was randomly generated using Research Randomizer v.4. Individual and sequentially numbered cards containing the random assignment were folded in sealed opaque envelopes, and then an external researcher selected the envelope to allocate the participants. Then, the assignment was revealed after baseline data collection to the participant but not to the examiner and rater.
Participants were assigned into two groups: 1) an intervention group that participated in cryostimulation after fatigue-induced exercise protocol (CRYO, n = 18), and a control group performing fatigue-induced exercise protocol, but without any intervention (CONT, n = 17). However, during the process, four participants (two in CRYO and two in the CONT group) resigned from participation in the further part of the study for health reasons. Finally, the analysis was performed on thirty-one participants, including sixteen in CRYO and fifteen in the CONT group (Figure 1).
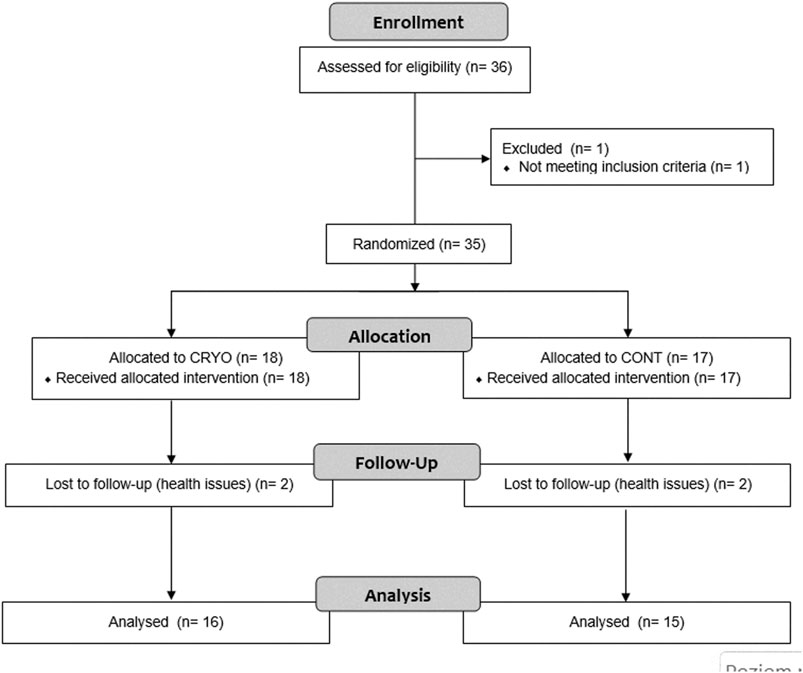
FIGURE 1. Experimental procedures. Fatigue-induced protocol consisting of CMJ (10 sets for 10 repetitions). After the fatigue exercises, only CRYO underwent a cryosauna intervention including entry immediately after, 24-48-72 h post-exercise. Measure outcomes at baseline: blood sample testing (leukocyte content, myoglobin concentration, and creatine kinase activity) and muscle stiffness of the dominant lower extremity; immediately after (stiffness), and 24-48-72-96 h post-exercise (blood samples and stiffness).
A familiarization session was performed 1 week before the main experiment, where all participants were informed about the study design and signed the informed consent. Furthermore, during this session, participants were instructed to exclude criteria, avoid strenuous exercise during the study, and follow safety rules in cryosauna. Next, participants were instructed to a proper counter-movement jumps (CMJ) using a Kistler force plate (model: 9286BA; Winterthur, Switzerland). During the test, all participants performed two CMJs from a bounce with both feet and a standing position with their hands placed on their hips. This performance measure was used for the detailed description.
The G*Power software (version 3.1.9.2; Kiel University, Kiel, Germany) was used to estimate the required sample size. A sample size estimated with a repeated measure ANOVA within and between factors, an α of 0.05, set a minimum expected effect size (Cohen’s f) of 0.25, β of 0.95, and correlation among repeated measures of 0.5. The number of measurements for blood samples was five, while for stiffness was six. The procedure included a minimum number of 32 participants required for blood sample testing, however 28 participants for stiffness measurements. Therefore, four extra participants were recruited to account for potential drop-out.
Experimental protocol
Research procedures and data collection were conducted in the Exercise Physiology Laboratory at Gdansk University of Physical Education and Sport. The laboratory has special facilities for blood sample taking and testing and monitoring exercise outcomes. The room temperature and humidity are 21°C (69.8°F) and 60%, respectively. The order of measurements included at baseline: blood sample testing (leukocyte content, myoglobin concentration, and creatine kinase activity) and muscle stiffness of the dominant lower extremity; immediately after (stiffness), and 24-48-72-96 h post-exercise (blood samples and stiffness). The examiners responsible for blood sample tests and stiffness were blinded and thus were not informed about the order of participants and being assigned to one of the groups. After baseline measurements, all participants performed 10-min warm-up exercises based on global exercises of the lower extremity and trunk joints and muscles. Following a 5-min passive rest, each participant performed repeated CMJ jumps (10 sets of 10 repetitions within a 1-min rest between sets) (Kons et al., 2020).
Intervention
After the fatigue exercises, only CRYO participated in a cryostimulation intervention during four sessions (i.e., immediately after, 24-48-72 h post-exercise) of cryo-cabin where the head was not exposed (cryosauna) (JUKA Standards: EN ISO 9001:2009–EC Certificate of Conformity to Directive 93/42/EEC/Annex VI on Medical Devices– ISO 13485: 2003). All sessions took place between 10–11 a.m. from Monday to Thursday under the supervision of a qualified staff member. Each session lasted 1.5 min. Before entering the cryosauna, participants had their blood pressure measured. The temperature in the cryosauna was −110°C. During this intervention, participants wore only shorts, socks, gloves, and hats (Figure 2).
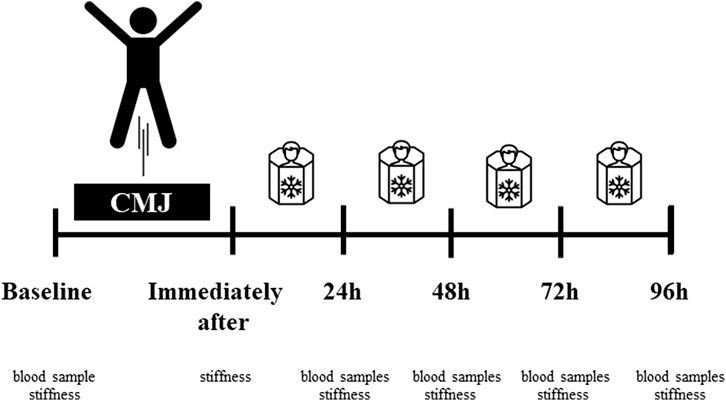
FIGURE 2. Experimental procedures. Fatigue-induced protocol consisting of CMJ (10 sets for 10 repetitions). After the fatigue exercises, only CRYO underwent a cryosauna intervention including entry immediately after, 24-48-72 h post-exercise. Measure outcomes at baseline: blood sample testing (leukocyte content, myoglobin concentration, and creatine kinase activity) and muscle stiffness of the dominant lower extremity; immediately after (stiffness), and 24-48-72-96 h post-exercise (blood samples and stiffness).
Outcomes
Blood biomarkers of muscle damage
Blood samples were collected by standard intravenous puncture by a qualified nurse before the fatigue procedure and also 24-48-72-96 h post-exercise. The test consisted of drawing 5 mL of blood from a vein (in the ulnar fossa) into a tube containing EDTA (Becton Dickinson and Co., Franklin Lakes, NJ, United States). Blood samples were centrifuged twice (1,600× g, 10 min) within 2 h after collection (prior stored at 4°C). Clear plasma samples were transferred to fresh Eppendorf tubes and stored at −80°C until further analysis. For the data analysis, leukocyte content [109/L], myoglobin concentration [ng/mL], and creatine kinase activity [U/L] were measured. Leukocyte content was analyzed using an automated hematologic analyzer (SYSMEX XN 2000; SYSMEX Corporation, Kobe, Japan). This measurement was based on fluorescence flow cytometry using a semiconductor laser that measures cells at a wavelength of 663 nm. The measurement detailed characteristics, i.e., analysis range: 0.0-440 G/L and coefficient of variation (95% confidence) when analyzing peripheral blood −3.0% or less (4.00 × 103/ul or less). Enzyme-linked immunosorbent assays were used to assess changes in myoglobin concentration (Human Myoglobin Matched Antibody Pair Kit AB215407m Cambridge, United Kingdom) and creatine kinase activity was analyzed with an EC 2.7.3.2 Assay Kit (Biosystems, Barcelona, Spain). Normal value range for leukocytes concent was 4.5–11.0 × 109/L; myoglobin concentration was 25–72 ng/mL, as well as creatine kinase activity was 30–135 [U/L] (Chernecky and Berger, 2013).
Muscle stiffness
A hand-held myotonometer (MyotonPro, Myoton Ltd., Estonia) was used to measure the stiffness of the lower extremity muscles. The myotonometer measurements were performed on the dominant lower limb (right) at 23 reference points, covering the muscles of the anterior thigh (points 1–7): posterior thigh (points 8–15), shin muscles (points 16–18), and calf muscles (points 19–23). The specific muscles analyzed in this study were as follows: rectus femoris (points 1–2), tensor fasciae latae (point 3); vastus lateralis (points 4–6); vastus medialis (point 7); external extensor muscles (points 8–11); internal extensor muscles (points 12–15); tibialis anterior (point 16); peroneus (points 17–18); external gastrocnemius muscles (points 19–20); internal gastrocnemius (points 21–22); and soleus muscles (point 23) (Klich et al., 2018; Klich et al., 2020). In this study, three measurements were collected at each reference point, and the average was calculated. The measurement for one reference point took about 5 s, while the total test time was less than 2 min.
The relative intra-reliability revealed excellent reliability and acceptable measurement error in a pilot study of n = 15 participants for a single point (no. 2) of the quadriceps muscle stiffness (ICC2, 1 = 0.91). The absolute reliability showed SEM was 12 N/m, while MDC90% was 27 N/m.
Statistical analysis
SPSS statistical software (version 18., SPSS Inc., Chicago, Illinois, United States) was used for all statistical tests and data analysis. Mean values ±standard deviation with confidence interval (CI 95%) were reported in this study. The normality of the data distribution was applied through the Shapiro–Wilk tests, while homogeneity of variance was analyzed by Levene’s test. The analyzed data were normally distributed for all parameters, while the variances for all parameters were equal. A two-way repeated measure analysis of variance (RM-ANOVA) with Time (baseline, 24-48-72-96 h post-exercise) and Group (CRYO and CONT) was conducted for blood fatigue tests (leukocyte content, myoglobin concentration, and creatine kinase activity). Additionally, a three-way RMANOVA with Time (baseline, post-exercise, and 24-48-72-96 h post-exercise), Group (CRYO and CONT), and Muscle (RF-TFL-VL-VM-TIB-FIB-HAM-CALF) were conducted for muscle stiffness. The Bonferroni adjustment for multiple comparisons was used for post hoc tests (p = 0.05) if a significant interaction between variables was found. The effect size was estimated using partial eta square (η2), classified as small (η2 = .0.01), medium (η2 = .06), or large (η2 = .14) (Cohen, 1988). A p-value ≤0.05 was considered significant.
Results
Blood biomarkers of muscle damage
Figure 3 showed the mean ± SD of blood sample tests, including leukocyte content, myoglobin concentration, and creatine kinase activity at baseline, 24-48-72-96-h after fatigue-induced jumping protocol in both groups (experimental and control). The two-way RM-ANOVA revealed a statistically significant main effect of Group (F1,29 = 13.2, p = 0.001, η2 = 0.31) for leukocyte content; Time (F4,116 = 7.4, p ≤ 0.001, η2 = 0.20) for myoglobin concentration; as well as Time (F4,116 = 9.6, p ≤ 0.001, η2 = 0.25) and Group (F1,29 = 18.9, p = 0.001, η2 = 0.49) for creatine kinase activity. The analysis revealed a statistically significant interaction effect between Time and Group (F4,116 = 3.0, p = 0.025, η2 = 0.10) for leukocyte content and (F4,116 = 2.6, p = 0.04, η2 = 0.08) for creatine kinase activity.
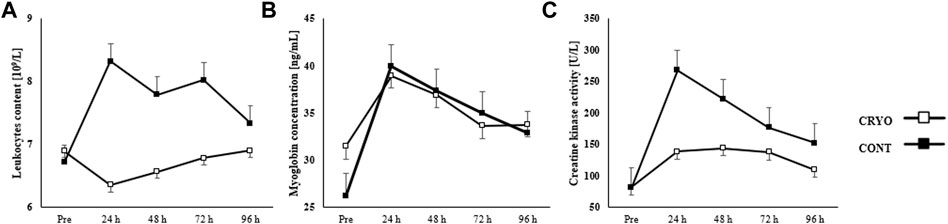
FIGURE 3. Mean ± SD from pre-test (Pre) and time course (24-48-72-96 h) values for the experimental (CRYO) and control (CON) group for (A) leukocyte content, (B) myoglobin concentration, and (C) serum kinase creatinine (CK) activity after fatigue-induced CMJ protocol. Significant differences *- within-group differences between Pre and 24-48-72-96 h after exercise, and **- between-group differences between CRYO and CONT (p ≤ 0.05).
Leukocyte content increased significantly 24 h after CMJ protocol (p = 0.008), while myoglobin concentration after 24-48-72 h (p = 0.001 for all), and creatine kinase activity after 24-48-72-96 h post-exercise (p ≤ 0.001 for all) in CONT group. However, we found a significant increase in myoglobin concentration after 24 h (p = 0.001) in the CRYO group. Finally, the leukocyte content was significantly greater 24-48-72 h after exercise in CONT, compared with the CRYO group (p ≤ 0.05 for all), while creatine kinase activity was greater 24-48-96 h in CONT, compared with the CRYO group (p ≤ 0.05 for all) (Figure 3).
Muscle stiffness
Figure 4 demonstrates the mean ± SD of the lower extremity muscle stiffness at baseline, post-exercise, and 24-48-72-96-h after CMJ protocol in the experimental and control group. The three-way RM-ANOVA revealed a statistically significant main effect of Time (F5,1080 = 257.1, p ≤ 0.001, η2 = 0.54), Group (F1,216 = 430.0, p ≤ 0.001, η2 = 0.67) and Muscle (F35,1080 = 7.3, p ≤ 0.001, η2 = 0.19). The analysis revealed a statistically significant interaction effect between Time, Group, and Muscle (F35,1080 = 7.3, p ≤ 0.001, η2 = 0.19).
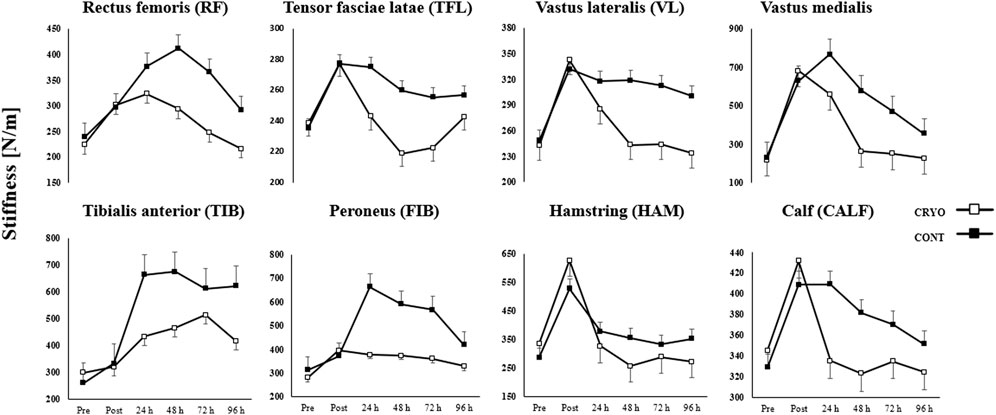
FIGURE 4. Mean ± SD from pre-test (Pre), immediately after (Post) and time course (24-48-72-96 h) values for the experimental (CRYO) and control (CON) group for lower extremity muscle stiffness: rectus femoris (RF), tensor fasciae latae (TFL); vastus lateralis (VL); vastus medialis (VM); tibialis anterior (TIB); peroneus (FIB); hamstring (HAM); and calf (CALF) muscles after fatigue-induced CMJ protocol. Significant differences *- within-group differences between Pre, Post, and 24-48-72–96 h after exercise, and **- between-group differences between CRYO and CONT (p ≤ 0.05).
Muscle stiffness increased significantly in all examined muscles (except TFL) post-exercise (p ≤ 0.05 for all) and in RF, VM, TIB, and FIB after 24 h post-exercise (p ≤ 0.05 for all) in the CRYO group. Moreover, an increase was found in RF, TIB, and FIB after 48 h post-exercise (p ≤ 0.05 for all), as well as in TIB and FIB after 72 h post-exercise (p ≤ 0.05 for all) in the CRYO group. The TIB and FIB muscle stiffness increased after 96 h post-exercise (p ≤ 0.05 for all) in the CRYO group (Figure 4).
Moreover, only HAM muscle stiffness decreased after 48 and 96 h post-exercise (p ≤ 0.05 for both). For the CON group, muscle stiffness increased significantly in VM and TIB after all time courses (i.e., post-exercise, 24-48-72-96 h) (p ≤ 0.05 for both), while VL and FIB expect 96 h after CMJ protocol (p ≤ 0.05 for both). The HAM and CALF muscle stiffness increased significantly post-exercise (p = 0.001 for both) and after 24 h (p ≤ 0.05 for both), while RF post-exercise (p = 0.015) (Figure 4).
An increase was found in HAM after 48 h post-exercise (p = 0.001), as well as in RF, VL, and FIB after 72 h post-exercise (p ≤ 0.05 for all) in the CONT group. Finally, VM, TIB, FIB, and HAM muscle stiffness increased after 96 h post-exercise (p ≤ 0.05 for all) in the CONT group. The post hoc analysis also showed a significantly greater stiffness post-exercise and lower after 48 h of CMJ protocol in HAM, compared with CALF (p = 0.001 and p = 0.002, respectively), while greater stiffness post-exercise in HAM, compared with RF and VL (p ≤ 0.001 for both) in CRYO group (Figure 4).
However, a lower stiffness post-exercise and after 24 h was found in HAM and RF, compared with VM (p ≤ 0.001 for all) in the CRYO group. For the CON group, a greater stiffness was observed post-exercise, while a lower stiffness after 24, 72 h post-exercise in HAM, compared with CALF (p ≤ 0.001 for all). Moreover, a greater stiffness was found in VM post-exercise, after 24 and 48 h, compared with RF, VL, and HAM (p ≤ 0.05 for all) in the CONT group. Finally, the stiffness of RF, VL, VM, TIB, FIB, and HAM was significantly greater post-exercise in CONT compared with the CRYO group (p ≤ 0.05 for all) (Figure 4).
Discussion
This study investigated the effectiveness of multiple cryosauna sessions on the reduction of DOMS in lower extremity muscles. Our results showed a recovery effect in muscle stiffness after exercise-induced muscle damage. This finding may indicate changes in blood biomarkers of muscle damage and muscle stiffness. Moreover, we have confirmed our hypothesis that multiple cryosauna during the 96 h post-exercise would reduce the post-exercise DOMS, expressed by muscle damage indicators, and support the recovery effect of specific kinds of cryotherapy sessions. Specifically, we observed a statistically significant increase in blood biomarkers of muscle damage after 24 h post-exercise (myoglobin concentration) in the CRYO group. However, a significant increase in leukocyte content after 24 h, myoglobin concentration after 72 h, and creatine kinase activity after 96 h post-exercise were found in the CON group. Muscle stiffness showed a significant increase in stiffness of VM after 24 h post-exercise; RF after 48 h post-exercise; FIB after 72 h post-exercise, as well as TIB after 96 h post-exercise, however a decrease in muscle stiffness was found in HAM after 48 and 96 h post-exercise. In the COM group, we observed a significant increase in muscle stiffness of CALF after 24 h post-exercise; HAM after 48 h post-exercise; RF and VL after 72 h post-exercise, as well as VM, FIB, and TIB after all time courses (up to 96 h post-exercise).
The current study design was constructed to apply four cryosauna sessions during a 96 h post-exercise period. To the best of our knowledge, this is the first study that used cryosauna to evaluate DOMS following exercise-induced muscle damage. Previous studies used a similar intervention based on four cryotherapy sessions (using cold water immersion–CWI) during 72–168 h post-exercise (Eston and Peters, 1999; Goodall et al., 2008; Machado et al., 2017; Siqueira et al., 2018), however, Vaile et al. (2008) compared three different interventions, e.g., cold water immersion, hot water immersion, and contrast water therapy. The results of our study have demonstrated that multiple cryosauna sessions may influence the recovery of lower extremity muscles by reducing the blood biomarkers and muscle stiffness after exercise-induced muscle damage. The intervention group (CRYO) was characterized by significantly lower blood biomarkers after 24–72 h post-exercise (leukocyte content) and 24, 48, and 96 h post-exercise (creatine kinase activity), compared to the CON group. Moreover, in VL, VM, HAM, and CALF, we observed a decrease in muscle stiffness post-exercise (after 48 h) compared to baseline; however, only in HAM, the decrease was statistically significant. In previous studies, authors have not found CWI as much effective in improving the recovery process by reducing post-exercise blood biomarkers concentration and increasing muscle functional performance (e.g., range of motion and strength) (Eston and Peters, 1999; Goodall et al., 2008; Machado et al., 2017; Siqueira et al., 2018). Probably, the reason for this low effectiveness might be related to water temperature and the duration of a single session. Fonda and Sarabon (2013) reported that whole-body cryotherapy with temperatures at −140 and −195°C (with a 3-min duration) might reduce DOMS. In our study, we used multiple cryosauna recovery strategies (four sessions), including a 1.5 min duration of cryostimulation at −110°C.
Blood biomarkers of muscle damage
The observations from our study have confirmed our expectations about the development of DOMS post-exercise. We observed an increase in blood biomarkers concentration after 24 h post-exercise, while in the lower extremity muscles stiffness immediately after, as well as in some cases a continuous increase until 24 h post-exercise in the CON group. However, the stiffness of RF increased to the first 48 h post-exercise in the CON group. Muscle stiffness in most of the analyzed muscles increased immediately after exercise and decreased after the first session of cryosauna.
Previous studies have shown an ineffective role of a single cryotherapy session on creatine kinase activity and C-reactive protein concentration in the blood after exercise (Lombardi et al., 2017). The use of multiple cryostimulation sessions after exercise or training may decrease creatine kinase activity (30%–40%) (Wozniak et al., 2007; Banfi et al., 2009). Siqueira et al. (2018) investigated other inflammatory blood biomarkers, e.g., interleukin (IL)-6, IL-1a, IL-10, and tumor necrosis factor (TNF)-α. Multiple CWI sessions after exercise-induced muscle damage showed a decrease in these blood biomarkers after 72 h post-exercise. In contrast to the CON group, the tendency of increase after 24 h post-exercise in creatine kinase activity was consistent with the tendency of muscle stiffness. According to previous studies that investigated the effect of multiple CWI on reduce of blood biomarkers, we observed also a decrease in creatine kinase activity after the first cryostimulation. Eston and Peters (1999) demonstrated a decrease in creatine kinase activity after 48 and 72 h post-exercise (eccentric exercise on the elbow flexors), while Vaile et al. (2008) observed after 24 and 72 h post-exercise (eccentric program for lower extremities). In contrast, Siqueira et al. (2018) have not observed a significant decrease in creatine kinase activity post-exercise (drop jump protocol), but only after 168 h post-exercise. In our study, we used exercise-induced muscle damage consisting of CMJ jumps. It should be noted that some studies, e.g., Eston and Peters (1999), investigated DOMS after upper extremity exercises, which might be more susceptible to damage. Finally, the effect of cryotherapy or CWI on leukocyte content and myoglobin concentration have not been evaluated for multiple cryostimulation session post-exercise. Stacey et al. (2010) did not find any positive effect of repeated cycling bouts on leukocyte content after CWI, as well as Krueger et al. (2020) found no reduction in myoglobin concentration after whole-body cryotherapy (at −110°C) followed by high-intensity intermittent exercise. Rodenburg et al. (1995) reported acute biochemical outcomes in creatine kinase activity and myoglobin concentration after eccentric exercise. This study showed an increase in creatin kinase activity after 24 h post-exercise, however, myoglobin concentration increased after 1 h post-exercise. These observations may suggest that myoglobin concentration is increasing immediately after exercise.
Muscle stiffness
The evaluation of muscle stiffness after exercise-induced muscle damage was shown for the first time in our study. As we expected, muscle stiffness has changed in muscles mainly involved in CMJ. During the stretch-shortening cycle in CMJ, mostly vastus lateralis and medialis, hamstring, and calf muscles are activated (Donohue, 2021). As expected, these muscles, as well as the tibialis anterior and peroneus, reached a higher stiffness immediately after repeated CMJ protocol. For all tested muscles there was a significant decrease in stiffness in all timepoints, for the CRYO group in relation to CON group. The effectiveness of multiple cryosauna on the reduction of muscle stiffness might be related to the alterations in blood biomarkers concentration, which is also in line with the results of our research. The underlining mechanism could be due to the transfer of creatine kinase in the interstitial fluid and the lymphatic system to the bloodstream (Clarkson and Hubal, 2002). Previous studies demonstrated a reduction in muscle and muscle-tendon stiffness after stretch-shortening cycle exercises (Pousson et a., 1991; Avela and Komi, 1998; Toumi et al., 2006; Kubo and Ikebukuro, 2019) using different biomechanical evaluation (surface electromyography), however, there is a lack of use devices based on mechanical properties (myotonometry and elastography). The eccentric phase of stretch-shortening cycle exercise may cause alterations due to muscle damage. According to Harrison and Gaffney (2004), mechanisms responsible for the reduction of muscle stiffness during the stretch-shortening cycle are unknown. However, eccentric exercises may cause DOMS by provoking muscle damage and increasing pain (Kubo and Ikebukuro, 2019), thus the mechanisms theoretically might be related to mechanical and/or metabolic responses (Harrison and Gaffney, 2004). Yanagisawa et al. (2015) considered an increase in creatine kinase activity with alterations in muscle mechanical properties, which is consistent with the results of our study. In general we showed a significant decrease in the level of muscle stiffness, corresponding to a decrease in the level of creatine kinase activity for parallel time points for the CRYO group with respect to the control group.
Acute mechanisms of DOMS
The acute effect of exercise-induced muscle damage is related to the inflammation process. Acute inflammation requires vascular and cellular response due to vasoconstriction during the initial phase (approximately between 5 and 10 min) and followed by vasodilation and vascular permeability. However, the cellular response uses neutrophils and monocytes. During the next 4 h post-exercise neutrophils increase their concentration and next decreasing concentration, however, monocytes may migrate within the next couple hours. Moreover, the monocytes transfer from blood to tissue during 48 h, increasing their concentration because of an additional transport of plasma proteins to the tissue (Smith, 1991; Stozer et al., 2020). The acute increase in muscle stiffness might be related to alterations in extracellular water volume (Yanagisawa et al., 2015).
Limitations
Some of the potential limitations should be pointed out to consider in future studies. First, we recruited a group of active participants specialized in martial arts. Future studies should include semi-profession and/or professional athletes to indicate the level of development of DOMS and the effectiveness of recovery strategies. Second, future studies should also include other indicators, such as muscle strength, power and morphological properties assessed by ultrasonography imaging. Third, when calculating the number of participants in future trials, more participants may be required than in the original calculation due to the higher number of potential withdrawals due to blood samples testing and for health reasons.
Conclusion
Multiple cryosauna was an effective recovery strategy that resulted in a reduction of blood biomarkers of muscle damage (especially creatine kinase activity) and muscle stiffness after repeated CMJ protocol that led to exercise-induced muscle damage. Moreover, the development of DOMS, expressed by greater muscle stiffness post-exercise, was attenuated to the first 48 h. Thus, these multiple recovery strategies should be recommended for sports medicine professionals, such as physical therapists, athletic trainers, and strength and conditioning coaches, to apply after training, competition, and matches.
Data availability statement
The raw data supporting the conclusion of this article will be made available by the authors, without undue reservation.
Ethics statement
The studies involving humans were approved by the Bioethical Committee of the Regional Medical Society. The studies were conducted in accordance with the local legislation and institutional requirements. The participants provided their written informed consent to participate in this study. The study was conducted following the Declaration of Helsinki and was approved by the Bioethical Committee of the Regional Medical Society (KB-62/22) and registered in ClinicalTrials.gov (first date registration: 07.03.2023; ID: NCT05757518).
Author contributions
BW, ŁD, PM, and SK participated in study design; BW, ŁD, AKa, and SK conducted the study and drafted the paper; BW, ŁD, and SK participated in fieldwork; AKi, HH, and AKa revised the paper. All authors contributed to the article and approved the submitted version.
Acknowledgments
We thank the volunteers for their participation in the study.
Conflict of interest
The authors declare that the research was conducted in the absence of any commercial or financial relationships that could be construed as a potential conflict of interest.
Publisher’s note
All claims expressed in this article are solely those of the authors and do not necessarily represent those of their affiliated organizations, or those of the publisher, the editors and the reviewers. Any product that may be evaluated in this article, or claim that may be made by its manufacturer, is not guaranteed or endorsed by the publisher.
References
Argus C. K., Broatch J. R., Petersen A. C., Polman R., Bishop D. J., Halson S. (2017). Cold-water immersion and contrast water therapy: no improvement of short-term recovery after resistance training. Int. J. Sports Physiol. Perform. 12, 886–892. doi:10.1123/ijspp.2016-0127
Avela J., Komi P. V. (1998). Reduced stretch reflex sensitivity and muscle stiffness after long-lasting stretch-shortening cycle exercise in humans. Eur. J. Appl. Physiol. Occup. Physiol. 78 (5), 403–410. doi:10.1007/s004210050438
Banfi G., Melegati G., Barassi A., Dogliotti G., D’eril G. M., Dugué B., et al. (2009). Effects of whole-body cryotherapy on serum mediators of inflammation and serum muscle enzymes in athletes. J. Therm. Biol. 34, 55–59. doi:10.1016/j.jtherbio.2008.10.003
Behringer M., Jedlicka D., Mester J. (2018). Effects of lymphatic drainage and cryotherapy on indirect markers of muscle damage. J. Sports Med. Phys. Fit. 58 (6), 903–909. doi:10.23736/S0022-4707.17.07261-9
Chernecky C. C., Berger B. J. (2013). Laboratory tests and diagnostic procedures. 6th ed. St Louis, MO: Elsevier Saunders.
Cheung K., Hume P., Maxwell L. (2003). Delayed onset muscle soreness: treatment strategies and performance factors. Sports Med. 33 (2), 145–164. doi:10.2165/00007256-200333020-00005
Clarkson P. M., Hubal M. J. (2002). Exercise-induced muscle damage in humans. Am. J. Phys. Med. Rehabil 81 (11), S52–69. doi:10.1097/00002060-200211001-00007
Cohen J. (1988). Statistical power analysis for the behavioral sciences. 2nd edition. New York: Academic Press.
Donohue M. (2021). Quantification of Legionella pneumophila by qPCR and culture in tap water with different concentrations of residual disinfectants and heterotrophic bacteria. Sci. Total Environ. 774, 145142. doi:10.1016/j.scitotenv.2021.145142
Eston R., Peters D. (1999). Effects of cold water immersion on the symptoms of exercise-induced muscle damage. J. Sports Sci. 17, 231–238. doi:10.1080/026404199366136
Fonda B., Sarabon N. (2013). Effects of whole-body cryotherapy on recovery after hamstring damaging exercise: A crossover study. Scand. J. Med. Sci. Sports 23, e270–e278. doi:10.1111/sms.12074
Goodall S., Howatson G. (2008). The effects of multiple cold water immersions on indices of muscle damage. J. Sports Sci. Med. 7, 235–241.
Gulick D. T., Kimura I. F. (1996). Delayed onset muscle soreness: what is it and how do we treat it? J. Sport Rehab 5, 234–243. doi:10.1123/jsr.5.3.234
Harrison A. J., Gaffney S. D. (2004). Effects of muscle damage on stretch-shortening cycle function and muscle stiffness control. J. Strength Cond. Res. 18 (4), 771–776. doi:10.1519/14343.1
Hausswirth C., Louis J., Bieuzen F., Pournot H., Fournier J., Filliard J. R. (2011). Effects of whole-body cryotherapy vs. far-infrared vs. passive modalities on recovery from exercise-induced muscle damage in highly-trained runners. PLoS One 612, e27749. doi:10.1371/journal.pone.0027749
Hotfiel T., Freiwald J., Hoppe M. W., Lutter C., Forst R., Grim C., et al. (2018). Advances in delayed-onset muscle soreness (DOMS): part I: pathogenesis and diagnostics. Sportverletz Sportschaden 32, 243–250. doi:10.1055/a-0753-1884
Jajtner A. R., Hoffman J. R., Gonzalez A. M., Worts P. R., Fragala M. S., Stout J. R. (2015). Comparison of the effects of electrical stimulation and cold-water immersion on muscle soreness after resistance exercise. J. Sport Rehabil. 24, 99–108. doi:10.1123/jsr.2013-0113
Kawczyński A., Samani A., Fernández-De-Las-Peñas C., Chmura J., Madeleine P. (2012). Sensory mapping of the upper trapezius muscle in relation to consecutive sessions of eccentric exercise. J. Strength Cond. Res. 26, 1577–1583. doi:10.1519/JSC.0b013e318234e589
Klich S., Ficek K., Krymski I., Klimek A., Kawczyński A., Madeleine P., et al. (2020). Quadriceps and patellar tendon thickness and stiffness in elite track cyclists: an ultrasonographic and myotonometric evaluation. Front. Physiol. 14 (11), 607208. doi:10.3389/fphys.2020.607208
Klich S., Krymski I., Michalik K., Kawczynski A. (2018). Effect of short-term cold-water immersion on muscle pain sensitivity in elite track cyclists. Phys. Ther. Sport 32, 42–47. doi:10.1016/j.ptsp.2018.04.022
Kons R. L., Orssatto L., Sakugawa R. L., Da Silva Junior J. N., Diefenthaeler F., Detanico D. (2020). Effects of stretch-shortening cycle fatigue protocol on lower limb asymmetry and muscle soreness in judo athletes. Sports Biomech. 22, 1079–1094. doi:10.1080/14763141.2020.1779335
Kozłowska-Flis M., Rodziewicz-Flis E., Micielska K., Kortas J., Jaworska J., Borkowska A., et al. (2021). Short and long-term effects of high-intensity interval training applied alone or with whole-body cryostimulation on glucose homeostasis and myokine levels in overweight to obese subjects. Front. Biosci. 26, 1132–1146. doi:10.52586/5015
Krueger M., Costello J. T., Stenzel M., Mester J., Wahl P. (2020). The physiological effects of daily cold-water immersion on 5-day tournament performance in international standard youth field-hockey players. Eur. J. Appl. Physiol. 120, 295–305. doi:10.1007/s00421-019-04274-8
Kubo K., Ikebukuro T. (2019). Changes in joint, muscle, and tendon stiffness following repeated hopping exercise. Physiol. Rep. 7 (19), e14237. doi:10.14814/phy2.14237
Lombardi G., Ziemann E., Banfi G. (2017). Whole-Body cryotherapy in athletes: from therapy to stimulation. an updated review of the literature. Front. Physiol. 2, 258. doi:10.3389/fphys.2017.00258
Machado A., Almeida A., Micheletti J., Vanderlei F., Tribst M., Netto Junior J., et al. (2017). Dosages of cold-water immersion post exercise on functional and clinical responses: A randomized controlled trial. Scand. J. Med. Sci. Sports 27, 1356–1363. doi:10.1111/sms.12734
MacIntyre D. L., Sorichter S., Mair J., Berg A., McKenzie D. C. (2001). Markers of inflammation and myofibrillar proteins following eccentric exercise in humans. Eur. J. Appl. Physiol. 84 (3), 180–186. doi:10.1007/s004210170002
Miranda J. P., Silva W. T., Silva H. J., Mascarenhas R. O., Oliveira V. C. (2021). Effectiveness of cryotherapy on pain intensity, swelling, range of motion, function and recurrence in acute ankle sprain: A systematic review of randomized controlled trials. Phys. Ther. Sport 49, 243–249. doi:10.1016/j.ptsp.2021.03.011
Olson J. E., Stravino V. D. (1972). A review of cryotherapy. Phys. Ther. 52 (8), 840–853. doi:10.1093/ptj/52.8.840
Pinto J., Rocha P., Torres R. (2020). Cold-water immersion has No effect on muscle stiffness after exercise-induced muscle damage. Clin. J. Sport Med. 30 (6), 533–538. doi:10.1097/JSM.0000000000000682
Piotrowska A. (2017). Safety aspects of whole-body cryochamber and cryosauna operation. IOP Conf. Ser. Mater. Sci. Eng. 278, 012125. doi:10.1088/1757-899x/278/1/012125
Point M., Guilhem G., Hug F., Nordez A., Frey A., Lacourpaille L. (2018). Cryotherapy induces an increase in muscle stiffness. Scand. J. Med. Sci. Sports 28 (1), 260–266. doi:10.1111/sms.12872
Pournot H., Bieuzen F., Louis J., Mounier R., Fillard J. R., Barbiche E., et al. (2011). Time-course of changes in inflammatory response after whole-body cryotherapy multi exposures following severe exercise. PLoS One 6, e22748. doi:10.1371/journal.pone.0022748
Pousson M., Pérot C., Goubel F. (1991). Stiffness changes and fibre type transitions in rat soleus muscle produced by jumping training. Pflugers Arch. 419 (2), 127–130. doi:10.1007/BF00372997
Puntel G. O., Carvalho N. R., Dobrachinski F., Salgueiro A. C., Puntel R. L., Folmer V., et al. (2013). Cryotherapy reduces skeletal muscle damage after ischemia/reperfusion in rats. J. Anat. 222 (2), 223–230. doi:10.1111/joa.12009
Rodenburg J. B., Bär P. R., De Boer R. W. (1985). Relations between muscle soreness and biochemical and functional outcomes of eccentric exercise. J. Appl. Physiol. 74 (6), 2976–2983. doi:10.1152/jappl.1993.74.6.2976
Rose C., Edwards K. M., Siegler J., Graham K., Caillaud C. (2017). Whole-body cryotherapy as a recovery technique after exercise: A review of the literature. Int. J. Sports Med. 38, 1049–1060. doi:10.1055/s-0043-114861
Seco-Calvo J., Mielgo-Ayuso J., Calvo-Lobo C., Córdova A. (2020). Cold water immersion as a strategy for muscle recovery in professional basketball players during the competitive season. J. Sport Rehabil. 29, 301–309. doi:10.1123/jsr.2018-0301
Siqueira A. F., Vieira A., Bottaro M., Ferreira-Junior J. B., Nobrega O. D. T., De Souza V. C., et al. (2018). Multiple cold-water immersions attenuate muscle damage but not alter systemic inflammation and muscle function recovery: A parallel randomized controlled trial. Sci. Rep. 8, 10961–11012. doi:10.1038/s41598-018-28942-5
Smith L. L. (1991). Acute inflammation: the underlying mechanism in delayed onset muscle soreness? Med. Sci. Sports Exerc 23 (5), 542–551. doi:10.1249/00005768-199105000-00006
Stacey D. L., Gibala M. J., Martin Ginis K. A., Timmons B. W. (2010). Effects of recovery method after exercise on performance, immune changes, and psychological outcomes. J. Orthop. Sports Phys. Ther. 40, 656–665. doi:10.2519/jospt.2010.3224
Stožer A., Vodopivc P., Križančić Bombek L. (2020). Pathophysiology of exercise-induced muscle damage and its structural, functional, metabolic, and clinical consequences. Physiol. Res. 69 (4), 565–598. doi:10.33549/physiolres.934371
Toumi H., Poumarat G., Best T. M., Martin A., Fairclough J., Benjamin M. (2006). Fatigue and muscle-tendon stiffness after stretch-shortening cycle and isometric exercise. Appl. Physiol. Nutr. Metab. 31 (5), 565–572. doi:10.1139/h06-034
Vaile J., Halson S., Gill N., Dawson B. J. J. O. S. S. (2008). Effect of cold water immersion on repeat cycling performance and thermoregulation in the heat. J. Sports Sci. 26, 431–440. doi:10.1080/02640410701567425
Wozniak A., Wozniak B., Drewa G., Mila-Kierzenkowska C. (2007). The effect of whole-body cryostimulation on the prooxidant–antioxidant balance in blood of elite kayakers after training. Eur. J. Appl. Physiol. 101, 533–537. doi:10.1007/s00421-007-0524-6
Keywords: cryotherapy, muscle damage, countermovement jump, myotonometry, recovery
Citation: Wolska B, Domagała Ł, Kisilewicz A, Hassanlouei H, Makar P, Kawczyński A and Klich S (2023) Multiple cryosauna sessions for post-exercise recovery of delayed onset muscle soreness (DOMS): a randomized control trial. Front. Physiol. 14:1253140. doi: 10.3389/fphys.2023.1253140
Received: 04 July 2023; Accepted: 30 August 2023;
Published: 12 September 2023.
Edited by:
J. Derek Kingsley, Kent State University, United StatesReviewed by:
Riku Yoshida, Niigata University of Health and Welfare, JapanMagdalena Wiecek, University School of Physical Education in Krakow, Poland
Copyright © 2023 Wolska, Domagała, Kisilewicz, Hassanlouei, Makar, Kawczyński and Klich. This is an open-access article distributed under the terms of the Creative Commons Attribution License (CC BY). The use, distribution or reproduction in other forums is permitted, provided the original author(s) and the copyright owner(s) are credited and that the original publication in this journal is cited, in accordance with accepted academic practice. No use, distribution or reproduction is permitted which does not comply with these terms.
*Correspondence: Sebastian Klich, c2ViYXN0aWFuLmtsaWNoQGF3Zi53cm9jLnBs