- 1Department of Medical Microbiology, Cihan University-Erbil, Erbil, Iraq
- 2School of Biomedicine, Faculty of Health and Medical Sciences, The University of Adelaide, Adelaide, SA, Australia
- 3Institute of Evolutionary Medicine, University of Zurich, Zurich, Switzerland
- 4Department of Medical Microbiology, College of Science, Knowledge University, Erbil, Iraq
Although evolutionary medicine has produced several novel insights for explaining prevalent health issues, it has yet to sufficiently address possible adverse mental health effects of humans during long-term space missions While evolutionary applications to medicine have increased over the past 20 years, there is scope for the integration of evolutionary applications in the new branch of space medicine called bioastronautics, which analyses the effects on human bodies when in outer space. Evolutionary principles may explain what kinds of space environments increase mental health risks to astronauts, both in the short and long term; secondly, evolutionary principles may provide a more informed understanding of the evolutionary mismatch between terrestrial and space environments in which astronauts exist. This information may assist in developing frameworks for improving mental health of astronauts and future space colonists. Consequently, this paper will focus on some of the major evolutionary mismatches currently confronting astronauts’ mental health, with an aim to improve medical knowledge. It will also provide possible therapeutic countermeasures based on evolutionary principles for reducing adverse mental effects on astronauts.
1 Introduction
Evolutionary medicine is a growing discipline integrating the principles of evolutionary biology, medicine, genetics and psychology, providing a range of concepts and insights for explaining human vulnerability to disease. (Beeson and Kosal, 2023; Nesse and Williams, 2012; Stearns et al., 2010; Stearns, 2012; Dolgova, 2021). Evolutionary medicine speculates that the human body has evolved under various conflicts of evolutionary forces, trade-offs, mismatches and constraints intrinsic to biological organisms under the influence of natural selection and non-directional forces of evolution such as the genetic drift, gene flow and inbreeding (Nesse and Williams, 2012; Dolgova, 2021). An understanding of these evolutionary phenomena provides a feasible framework for classifying and understanding the complex interaction between genes, environment and history in relation to disease (Dolgova, 2021). Next, the human genome has undergone various adaptations over evolutionary history in response to ancestral environments, disease load and cultural behaviours, which inform current susceptibility to disease (Perry, 2021). Notwithstanding its growth in the English speaking world and in Europe, evolutionary medicine has yet to be established within global medical curricula (Natterson-Horowitz et al., 2023).
Even though evolution has been traditionally considered integral to biology, medical practitioners and public health experts have generally overlooked evolution’s role in human susceptibility to disease. Recent medical science developments such as the Human Genome Project, recombinant DNA, and the Haplotype Map have reaffirmed the significance of genes for human health and disease. Concomitantly, evolutionary psychology examines the gene/environmental interplay and its enduring effect on traits of extant humans.
Thus far, evolutionary medicine has produced several novel insights for explaining prevalent health issues such as cancer, antimicrobial resistance, autoimmune disorders, diabetes, heart disease, depression/anxiety disorders, dementia and the advent of new anatomical variations. Evolutionary medicine also addresses the role of medical and public health interventions in reducing natural selection, which has been proposed to have led to an increase in fitness-reducing alleles with subsequential genetic load (Saniotis et al., 2021; Saniotis et al., 2024). For example, recent studies of 190 countries have suggested a correlation between type-1 diabetes and cancer incidence and the proliferation of harmful genes due to relaxed natural selection (You and Henneberg, 2016; 2017). Alterations in gene patterns due to relaxed selection could be important in mapping out possible future trajectories in human population health.
However, evolutionary medicine has yet to sufficiently address possible adverse mental health effects of humans during long-term space missions (LTSM). “Long term” can be referred to as being in outer space for a couple of months to years (Criscuolo et al., 2020). It will also apply eventually to life in human colonies on celestial bodies (Moon, Mars, etc.). While evolutionary applications to medicine have increased over the past 20 years, there is scope for the integration of evolutionary applications in the new branch of space medicine called bioastronautics, which analyses the effects on human bodies when in outer space (Arone et al., 2021). For example, evolutionary principles may explain what kinds of space environments increase mental health risks to astronauts, both in the short and long term; secondly, evolutionary principles may provide a more informed understanding of the evolutionary mismatch between terrestrial and space environments in which astronauts transit. This information may assist in developing frameworks for improving human mental health for future space colonists (Saniotis et al., 2016).
Consequently, this paper will focus on some of the major evolutionary mismatches currently confronting astronauts’ mental health, with an aim to improve bioastronautical knowledge. It will also provide possible therapeutic countermeasures based on evolutionary principles for reducing adverse mental effects to astronauts.
2 Evolutionary mismatch, adaptation and outer space
A central concept in evolutionary medicine is “evolutionary mismatch,” which refers to the discordance between the ancestral environments in which hominins lived and the novel societies’ extant human habitat (Trevathan, 2007), resulting from cultural evolution outpacing biological evolution. This is consistent with the rapid rate of allele substitution during the Holocene Epoch, which is thought to be 100 times higher than for most of the evolution of Homo (Hawks et al., 2007; Keller, 2008).
It has been speculated that accelerated cultural evolution has left extant humans susceptible to a plethora of diseases and disorders. Like other organisms, the human genetic profile has been shaped by the complex interplay of various evolutionary forces (i.e., natural selection, mutation, gene flow, genetic drift) and sexual selection.
Notwithstanding the evolutionary discordance between ancestral and modern environments, human evolution is on-going, even though, natural selection has been relaxed in our species (Saniotis and Henneberg, 2011b; 2023). Some studies have highlighted rapid human micro-evolution during the Holocene (10,000 ka-present) (Rühli and Henneberg, 2013). Recent microevolution has occurred via lactase persistence (Itan et al., 2009), amylase gene copy variation [Zuk, 2013; Perry et al., 2007) malarial resistance, high altitude adaptation, brain volume reduction (Henneberg, 1988; Henneberg and Steyn, 1993)) decreasing musculoskeletal robusticity (Ruff, 2002), and ongoing Abnormal Spindle-like, Microcephaly-associated (ASPM) gene adaptation [Mekel-Bobrov et al., 2005).
Humans have not evolved to live in the outer space environment. This is evident by the numerous studies on the Soviet Mir space station (1986–2001), and American Shuttle and ISS missions, which have shown that even short-term space flight may cause deleterious changes to muscles and skeletal function (Fitts et al., 2001; Adams et al., 2003), vestibular system (Ross and Tomko, 1998), cardiopulmonary system, immunological system, motor system (Kozlovskaya et al., 1981), neuro-behavioural and cognitive function (Kanas and Manzey, 2008; De La Torre et al., 2012).
While understanding of the effect of space flight on the human body and mind has improved in the last two decades, there is still a great deal of medical research needed on mid (1–5 years) to long duration (>5 years) consequences of space flight. The proposed permanent Moon base will provide a basis for monitoring the human body in conditions beyond low Earth orbit. Furthermore, NASA’s intention to send a manned space mission to Mars in the next-generation (the return trip will take approximately 21 months) will further identify health risks due to high exposure to radiation (astronauts could be exposed to ∼1 Sievert (Sv) radiation during the trip) (Cucinotta and Durante, 2006; Wilson et al., 1995), as well as potential neuro-behavioural issues due to the extended duration of the mission.
Evolutionary history indicates that humans exhibit significant plasticity and adaptability across various environments. In the context of evolution, outer space represents a distinct environment that necessitates a range of new adaptations. A key focus is how humans adjust to ensure neuro-behavioural allostasis, which involves sustaining neurohormonal balance for prolonged survival in space. This paper will further explore mental health challenges in bioastronautics, considering the implications of evolutionary mismatch.
3 Stress and glucocorticoid dysregulation in outer space
A major area of space medicine is the role of stress during long-duration space missions. Stress has been shown to affect astronaut behaviours and their ability to perform multiple tasks (Kanas, 1985; 1987). Space flight stressors include microgravity, living in small and enclosed confines, isolation, arduous workload, boredom, disconnection from the natural world, circadian disruption, unpredictable events, etc. These issues have received various levels of attention from space researchers.
Human stress response (HSR) is mediated by the Hypothalamus-Pituitary-Adrenal axis (HPA). The HPA axis evolved in our pre-hominin ancestors as an adaptive mechanism for triggering the sympathetic nervous system (SNS) in response to threats (de Kloet et al., 2005). Second, the HSR evolved to deal mainly with acute stressors (a few hours) and not with sub-acute (several days) or chronic stress (de Kloet, 2004). Prolonged exposure to chronic stress (several weeks/months to years) has been shown to play a role in depressive disorder and psychotic illness (de Kloet, 2004). In the hippocampus, long term glucocorticoid hormonal insults lead to impaired declarative and spatial memory [Ortiz and Conrad 2018), with diminished fear learning. Although dendritic recovery is possible in the hippocampal and pre-frontal cortex, this is only possible with the discontinuation of chronic stress (Conrad et al., 1999; Vyas et al., 2004). However, continuing dendritic retraction has a potential for damaging the hippocampus if persisting for months to years–referred to as the Glucocorticoid Vulnerability Hypothesis (Conrad, 2008).
Due to the various major stressors encountered by astronauts during LTSM, outer space presents an evolutionary mismatch with consequential risk for increasing neurotoxic challenges to emotional, learning and memory functions of the CNS. This is problematic since astronauts will be space-bound and isolated for a long duration when travelling to Mars.
4 Spaceflight-associated neuro-ocular syndrome (SANS)
From an ocular perspective the space environment presents critical challenges to human eyes and the visual system. In the absence of gravity, which has been a major evolutionary force in informing the structure, function and health of the human eyes, these organs are especially prone to visual pathologies such as spaceflight-associated neuro-ocular syndrome (SANS) (Mehare et al., 2024). This syndrome has been well documented in space medical literature given its relevance to neurological and mental health issues in astronauts. SANS presents as a collection of neuro-ocular symptoms due to a combination of factors such as exposure to micro-gravity, radiation, as well as, genetic factors (Ong et al., 2023; Mehare et al., 2024; Huang et al., 2019; Yang et al., 2022). Environmental increase in CO2, onboard lighting, high sodium intake and poor diet may also contribute to SANS (Mehare et al., 2024). However, microgravity plays a major role in the development of SANS, since micro-gravity may alter the distribution of CSF leading to increased cranial pressure (ICF), compression to the optic nerve and optic disc edema (Mehare et al., 2024; Mader et al., 2011). SANS may also induce intra/extraocular muscle atrophy with subsequent ocular impairments (i.e., blurred vision, hyperopic shift) (Paez et al., 2020). Importantly, there is a strong correlation between duration of flight and the development of SANS. Medical reports reveal that even short-term spaceflight (<2–3 weeks) may trigger visual disturbances (Paez et al., 2020), with increasing SANS related symptoms during LTSM (Mehare et al., 2024). Although, various potential treatments have been proposed (i.e., diuretics, nutritional supplements, anti-inflammatory drugs, neuroprotective agents, low vision aids) (Hartmann et al., 1977; Ghlichloo and Gerriets, 2023; Nucci et al., 2018) to mitigate SANS induced damage, astronauts will further need extensive monitoring and behavioural counselling due to the psychological impact of possible long-term visual alterations in space (Mehare et al., 2024). Moreover, more studies need to examine psychological stressors due to microgravity (Marsh, 2007).
5 Enteric gut microbiome and outer space: dysbiosis increases the risk of psychiatric diseases
The human intestinal tract, primarily the colon, is the site of the enteric gut microbiome (EGM) which consists of large communities of bacteria (numbering ∼ 1014) (Ley et al., 2006), comprising 150–400 species (Lloyd-Price et al., 2016), that differ significantly between individuals (The Human Microbiome Project Consortium, 2012), and which can alter over lifetime (Davenport et al., 2017; Odamaki et al., 2016; Aleman and Valenzano, 2019). The EGM is an important source of gene encoding as it contains a vast number of genes (>100 times greater than in the human genome) (O’Hara and Shanahan, 2006).
The EGM is involved in several key functions such as digestion, immune response (Shahab and Shahab, 2022), skeletal integrity, as well as neuro-behavioural and psychological functions (i.e., neuro-hormonal regulation, cognition (Davidson et al., 2018; Saniotis et al., 2020) and mood regulation (Desbonnet et al., 2008). Consequently, dysbiosis of the EGM can have a deleterious health outcome for individuals in several of these mentioned areas.
For millions of years hominins have co-evolved with microbial communities in the EGM. The EGM would have undergone continual evolutionary changes along with hominin adaptations to their environments and diets. For example, probably during the Pliocene/Pleistocene transition (∼3.2–2.6 Ma), the GIT of hominins underwent size reduction due to the introduction of meat and, later on, cooked food (Aiello and Wheeler, 1995; Henneberg et al., 1998; Mann, 2007). These two elements would have also contributed to alterations in the microbiome, which selected bacteria that favoured omnivorous diets (Shahab and Shahab, 2022).
Although, the human EGM is less diverse than that of the great apes, (Moeller et al., 2014; Davenport et al., 2017), intestinal biodiversity has further been altered by several bio-cultural changes from the Neolithic revolution (circa 10,000 ka) onwards. This trend was accelerated in the 20th century with global socio-cultural changes such as the introduction of anti-biotics, industrialised food products, increasing sedentism and chronic stress, and shifts to “western” style diets with significantly high omega-6 and inflammatory profiles (Bednarik et al., 2022), which have contributed to the “disappearing microbiome” (Blaser and Falkow, 2009).
Outer space represents a radical evolutionary mismatch for the EGM. It is well known that the EGM of astronauts is affected during outer space missions. Many stressors contribute to EGM dysbiosis during manned space missions which include microgravity, radiation, circadian desynchronisation cloistered living conditions and monotonous diets (Garrett-Bakelman et al., 2019; Voorhies et al., 2019; Marazziti et al., 2022; Arone et al., 2021; Siddiqui et al., 2021a; Siddiqui et al., 2021b; Mortazavi et al., 2024). The spacecraft/station environment does not contain many organisms that on Earth are sources of bacteria that may enrich the EGM. The EGM dysbiosis is significant since there are complex bidirectional interactions between the EGM and central nervous system (Kim et al., 2018; Saniotis et al., 2020), which also include microbiotic modulation of the vagus nerve via the microbiota-gut-vagus-brain-axis which may modify human behaviour (Montiel-Castro et al., 2013; Alcock et al., 2014). Interesting is that the probiotic influence of the EGM is annulled with either partial excision of the vagus nerve or vagotomy (Cryan and Dinan, 2012; Saniotis et al., 2020). Besides vagus transmitting sensations resulting from EGM’s activity (Liao et al., 2016) this may suggest that EGM produced neurometabolites are reliant on vagus nerve stimulation (Cryan and Dinan, 2012). Alterations to the microbiota-gut-brain-axis are associated with the pathogenesis of neurologic and psychiatric disorders (Cryan and Dinan, 2012; Kim et al., 2015; Mohajeri et al., 2018; Peterson, 2020).
Several types of microorganisms inhibit space-ship environments (Siddiqui et al., 2021a). Earlier microbiome studies found that astronauts were passing Staphylococcus aureus to each other (Pierson et al., 1996). A more recent International Space Ship (ISS) found that alteration to the EGM of astronauts increased inflammatory markers (Fusicatenibacter was negatively associated with “pro-inflammatory cytokines IL-8, IL-1b, IL4, and TNFa,” while genus Dorea was negatively correlated with cytokine IL-1b (Voorhies et al., 2019). It should be noted that studies have identified pro-inflammatory cytokines IL-8 and IL-1b as being implicated in higher risk of psychiatric pathologies (Tsai, 2021; Liu et al., 2022). In conclusion, the space environment increases the likelihood of EGM dysbiosis with subsequent risk of psychiatric disorders.
6 Circadian disruption during outer space missions and evolutionary mismatch
Like in other animals, human neurohormonal regulation is modulated by circadian rhythms. In humans, circadian rhythms regulate sleep/wake cycles, temperature, endocrine mechanisms, mental health, and wellbeing. Circadian synchronisation is an ancient adaptation to evolutionary environments in which the visceral neuroendocrine system (NS) was attuned to natural light fluctuations. In humans, the NS is regulated by a master biological clock (orchestrated by retinal, hypothalamic and reticular pathways), based on a 24 h cycle with light as the key zeitgeber (Anderson and Bradley, 2013). Any alteration of this circadian cycle (i.e., jetlag or shift workers) often leads to sleep disturbances or interruptions with subsequent reduced cognitive performance, tiredness, as well as higher risk for the onset of mental health problems (Anderson and Bradley, 2013).
Outer space represents a major mismatch for astronauts since they are removed from their evolutionary circadian environment. Astronautic sleep studies have identified that astronauts undergo sleep alteration due to circadian desychronisation, bright lighting, noisy internal spaceship environment, interior temperature, and sleeping bag discomfort (Arone et al., 2021; Marazziti et al., 2022). Even microgravity may diminish sleep duration (Gonfalone, 2016).
Analysis of sleep patterns of Shuttle crew members reported a 15% sleep reduction (Kelly et al., 2005), and 20 min less sleep during a 2-week mission relative to ground-based sleep (Barger et al., 2014). Consequently, due to insufficient additional light exposure in space, the 90-min light/dark cycle is disrupted eliciting homeostatic imbalance (Guo et al., 2014; Flynn-Evans et al., 2016; Wu et al., 2018). Despite NASA scheduling 8.5 h of sleep per night for crew members in space flight the average duration of sleep during space flight is just over 6 hours on Shuttle and ISS missions (Barger et al., 2014). Despite being allowed to sleep more hours astronauts still may have unproductive sleep quality (Flynn-Evans et al., 2016). Sleep alterations in astronauts are associated with increasing glucocorticoid secretion and inflammatory markers, memory and cognitive impairments, mood changes, altered relationship quality with crew members and reduced work performance (Chaumet et al., 2009).
Thus far, astronauts’ major recourse for improved sleep has been taking sleeping drugs and melatonin. However, long term use of benzodiazepine derivative sleeping medications (e.g., zolpidem midazolam, flurazepam, temazepam) that are regularly prescribed to astronauts are associated with various adverse side effects such as reduced cognitive and motor performance, drowsiness, etc. (Maust et al., 2019). Despite this, one study found that 71% of spaceship crew members were using sleep medications to promote or preserve sleep (Wotring, 2015).
7 “Earth-out-of-view-phenomenon”: neuro-behavioural risks of loss of connection with the natural world
Homo evolved in the natural world under intricate and on-going evolution. For tens of thousands of generations Homo evolved and adapted to various evolutionary environments which shaped its genome. The human body is an artefact of nature and exemplifies the complex gene/environment interplay. The entire emotional complex in Homo, both its neuro-hormonal substrates and psychosocial phenotypes were shaped and contoured by interaction with nature. In addition, the sensory perceptions which have been shaped by the natural world mediate our embodied awareness, which is intrinsically intertwined with multiple life forms “whether we are conscious of this connection or not” (Abram, 1996). The idea that natural environments promote human wellbeing has received considerable scientific attention over the last 3 decades. Many of these studies suggest that immersion in the natural world has neuro-behavioural and psychosocial benefits, such as reduced blood pressure and stress, shorter post-operative hospital period, lower mental fatigue and fewer sick days taken off work (Bowler et al., 2010; Velarde et al., 2007). Other cited psychological benefits from natural environments are promotion of calmness (Heerwagen, 2009), mental health improvement (Gullone, 2000), reduction in anger and aggression (Groenewegen et al., 2006) and enhanced natural killer (NK) immune response (Li et al., 2008). The theoretical frameworks of many of the afore-mentioned studies are informed mainly by E.O. Wilson’s “Biophilia Hypothesis” (2010), which argues that Homo has an innate preference for natural environments as a consequence of evolutionary history (Grinde and Patil, 2009).
The question remains that if psycho-somatic homeostasis is in some ways contingent on human/nature connection, what may be the mid to long term health consequences of astronauts stay in space? Will the evolutionary mismatch between the Gaian habitat and space lead to unknown health risks for future astronauts? Regarding physical health, some of these concerns have been addressed, and thus now regular exercise on board, recommendations for a balanced diet are common among astronauts, as well as sporadic leisure activities. However, much work still needs to be done on the psychological domain with long duration missions in perspective. On this note, some authors indicate that astronauts’ psychological status could be disrupted as the vision of planet Earth becomes increasingly difficult (Kanas and Manzey, 2008; Ihle et al., 2006). Moreover, astronauts from previous space missions stated that observing the Earth was meaningful and a positive factor of space travel (Kanas, 2011). Many interviewed astronauts and cosmonauts stated that being in space endowed them with a feeling of unity with nature, awe and a sense of transcendence–synonymous with “the overview effect” (Opsina et al., 2007). Since the so called “Earth-out-of-view-phenomenon” is new to the human psyche (Kanas and Manzey, 2008), it could well exacerbate feelings of homesickness, isolation and monotony (Kanas, 2011), with possible adverse mental health risks during LTSM.
8 Reduced natural selection in outer space and potential psychiatric disorders
Evolutionary medicine makes special reference to mechanisms operating within natural selection in ancestral hominins, and how alterations in selection processes are having health consequences in extant humans. Homo evolved on Earth and has adapted to the terrestrial environment. During most of hominin history, our ancestors were subjected to predation, parasitic and infectious diseases, trauma and environmental changes which shaped our genotypes (Saniotis and Henneberg, 2011b). Natural selection controlled the ratio between fitness enhancing/fitness compromising genes via differential reproductive success, which favoured traits that gave adaptive and reproductive advantages (Saniotis and Henneberg, 2011b). It is only with the advent of the Industrial Revolution of the 19th century that natural selection became relaxed due to improvements in public sanitation, nutrition and medicine (Saniotis and Henneberg, 2011b). Although this meant that most individuals in developed countries could reach reproductive adulthood, greater dependence on human interventions meant that natural selection had less ability to eradicate harmful alleles from the human gene pool. (Saniotis et al., 2020; Henneberg, 2025). It has been speculated that natural selection in the developed world is currently operating at only <1% compared with ∼50% prior to the Industrial Revolution (Saniotis and Henneberg, 2011b; Saniotis et al., 2020). Consequently, the proliferation of deleterious mutations may be leading to constrained fitness at a macro scale (Agrawal and Whitlock, 2012).
In outer space, humans are not only removed from the natural environment where natural selection operates–an evolutionary mismatch, but are entirely dependent on human technology and medical interventions to sustain them (Saniotis et al., 2016). (Figure 1).
Given that there is a strong correlation between relaxed selection and mutation load, we can anticipate that this process may continue in humans during LTSM and in permanent space colonies on the moon and Mars. Additionally, long term radiation exposure could increase the incidence of deleterious mutations (Horneck et al., 2010).
On this theme, we must consider the possibility of potential alterations in gene expression regarding neuro-hormonal regulation in outer space. Popova et al. (2020) state that we have just begun to examine the association between risk neurogenes and behavioural disorders during LTSM. Limited animal studies during spaceflight identify that serotonergic and dopaminergic metabolic pathways, as well as neurotrophic factors (GDNF, CDNF) - key regulators of neuroplasticity are all altered (Popova et al., 2020; Rappaport and Corbally, 2023). Serotonin and dopamine are involved in emotion, motivation, memory, learning, sleep and motor skills. Neuroanatomical changes to the substantia nigra, hypothalamus and striatum have also been identified–brain areas involved in endocrine regulation, mood, cognition and movement are also vulnerable during spaceflight (Popova et al., 2020). These preliminary results indicate characteristics that correspond with Spaceflight Neuroplastic Syndrome (Rappaport and Corbally, 2023). It should be noted that the reported changes in rodent brain neuroanatomy and neurohormonal regulation occurred after only 1 month in space (Popova et al., 2020). Rappaport and Corbally (2023) argue that, although hundreds of astronauts seem to have been shielded from similar deleterious neurological deficits (possibly due to a higher level of neuroplasticity), this does not discount the effect of relaxed natural selection conferring greater probability for risk neurogenes, especially to future permanent space colonies.
9 Evolutionary based countermeasures for reducing psychopathologies during LTSM
For several decades, a plethora of space research has been accumulated regarding the physiological/psychological responses of astronauts during outer space missions. Thus far, scientists have been able to assess the health impact of astronauts, as well as the types of pre-mission acclimatising training necessary for alleviating exposure to the outer space environment (Criscuolo et al., 2020). Although humans have not adapted to living in outer space, it does not exclude that in the future, humans could be genetically engineered to better adapt to living in outer space for the long term. Human medical interventions have not only led to an unprecedented reduction in premature mortality since the 19th century, but are now preoccupied in developing techniques for artificially modifying the body at genetic and phenotypic levels (Saniotis and Henneberg, 2011b). We concur with Criscuolo et al. (2020) who point out that gene expression in outer space will have a major significance in the future evolution of permanent space colonists as we have suggested earlier regarding relaxation of natural selection. Due to psychiatric health issues that astronauts face, evolutionary medicine can provide various countermeasures for reducing the onset of psychopathologies and assisting astronauts’ mental health during LTSM. We anticipate that the following countermeasures may benefit astronauts since they are based on evolutionary antecedents (Figure 2).
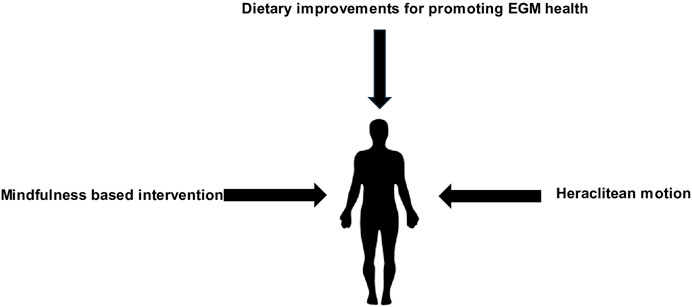
Figure 2. Evolutionary based countermeasures for reducing adverse mental health effects during LTSM.
9.1 Use of mindfulness based intervention for astronauts
Various authors have proposed the use of yoga and mindfulness based intervention (MBI) as a therapeutic countermeasure reducing psychological side effects of astronauts during space missions (Vernikos, 2012; Saniotis et al., 2023; Le Roy et al., 2023). These techniques have been used for millennia in various cultures and function in stimulating parasympathetic response while reducing sympathetic induced stress reaction. It has been argued that meditational techniques are based on evolutionary antecedents such as neuro-hormonally regulated auto-hypnotic response to threat, which had been positively selected due to its fitness value (McClenon, 1997; Krippner, 2000; Winkelman, 2000; 2004; Saniotis and Henneberg, 2011b). It has also been noted that Upper Palaeolithic shamans may have used altered states of consciousness in order to reduce individual ailments, improve fertility and increase group cohesion (Winkelman, 2000; 2004). Since relaxation inducing techniques may reduce an individual’s overall stress, this is important since astronauts have reported to have increasing stressors during LTSM (Marsh, 2007).
Mindfulness based intervention has been used in analog environments (Isolated and Confined Environments (ICE), Extreme and Unusual Environments (EUE)) with promising results in (i.e., better eating and sleeping habits, higher positive emotions states and lower negative emotions, reduced stress reactions) (Trousselard et al., 2010). Currently, more research needs to be conducted on whether MBI increases group cohesion among LTSM crew members or whether improving individual mindfulness has corresponding benefits at a group level (Le Roy et al., 2023). According to Saniotis et al. (2023), MBI is an inexpensive therapeutic technique that could be employed during pre-flight preparation and post-flight readaptation in order to assist astronauts’ mental health.
9.2 New strategies for addressing EGM in LTSM
Given that the EGM is altered during space conditions (i.e., radiation, microgravity, reduced biodiversity) it is difficult to develop new strategies in order to maintain its “normal” function. We encourage the further use of Lactobacillus and Bifidobacterium strains in probiotic form due to their anti-depressant mechanisms (Yong et al., 2020; Johnson et al., 2021; Dicks, 2022). Consequently, reduction of these species in the EGM have been linked to psychiatric disorders (Johnson et al., 2023). These bacteria can be freeze-dried and used, as has been the case of the Lactobacillus casei strain, which was used for 1 month by astronauts on the International Space Station (ISS) with positive results in improving intestinal microbial balance and innate immunity (Bharindwal et al., 2023). Future research needs to be conducted on developing probiotics that enhance mental health in astronauts, including bacterial strains not mentioned in this paper.
Second, we suggest the administration of faecal microbiota transplantation (FMT) to astronauts in order to recolonise beneficial gut bacteria where ordinary probiotic use is not efficacious due to the effects of antibiotic induced gut dysbiosis, microgravity, stress and radiation. Faecal microbiota transplantation provides a potential therapy in treating gut dysbiosis and restoring beneficial microbiota with subsequent mental wellbeing in astronauts during LTSM (Gupta et al., 2016). However, long term clinical trials need to be conducted on astronauts during space flight in order to verify the feasibility of FMT.
Third, we endorse the prioritising of omega-3 polyunsaturated fatty acids (PUFAs) in astronauts’ diets since they are critical to proper brain development and regulation. Human brain weight consists of approximately 50%–60% lipids, of which PUFAs account for 35% (Dighriri et al., 2022; Bos et al., 2016). Docosahexaenoic acid (DHA) is especially found in grey matter. It is well known that PUFAs are involved in numerous neuro-hormonal and cognitive processes (i.e., neurogenesis, neurotransmitter modulation, neuronal plasticity regulation, synthesis of neurotrophic factor BDNF) (Bednarik et al., 2022). Hominin intake of PUFAs increased during the Paleolithic period where the estimated ratio between omega-3 (α-linoleic acid) and omega-6 (linoleic acid) intake was ∼1:1 (Roccisano et al., 2016). In contrast, the ratio between omega-6/omega-3 in the western diet is ∼20:1. This difference is sufficient to increase the risk of psychiatric and neurological pathologies over time. For this reason, it is vital that astronauts maintain a healthy intake of PUFAs during LTSM.
9.3 Human space omics and personalised medicine
It is becoming clear that outer space has the potential to change gene expression which could affect metabolite synthesis and function across several neuro-physiological domains. As diverse human populations enter space natural selection will be further relaxed due to medical interventions and artificial conditions that will be necessary for humans to survive. We can only speculate to what extent gene-environment interaction may have in future gene expression of space travellers and colonists. For example, the enzyme Cytochrome P450 (CYP450), which is involved in ̴ 75% of drug metabolism and is considerably polymorphic, has shown alterations in some astronaut gene profiles (Rutter et al., 2024). Additionally, a contemporary pharmacogenetics study found that 24 out of 78 standard drugs available to astronauts on the ISS were not effectively metabolised in polymorphic enzymes due to gene variants (Stingl et al., 2015; Rutter et al., 2024). These findings point to possible “omic derived molecular changes” in astronauts during prolonged space flight (Cope et al., 2022; Willis et al., 2021), Other studies have examined proteomic alterations in astronauts post LTSM (Brzhozovskiy et al., 2019; Pastushkova et al., 2021). Consequently, omic profiling has been proposed as a medical countermeasure in storing and using genetic data of astronauts (Cope et al., 2022). Such genetic data sets could lead to the development of personalised medicine which would facilitate metagenomic based health monitoring of astronauts in future LTSM (Danko et al., 2020).
9.4 Incorporating Heraclitean motion and attention restoration for promoting mental wellbeing in astronauts
In an earlier section, it was noted that humans have an inherent connection with nature as a result of their evolutionary history. It is an indisputable fact that human corporeality is rooted in sensuous engagement with the natural world, the latter operating as the inherent template for human biological and social processes (Saniotis et al., 2016). The loss of interaction with the natural world in outer space which has been referred to as “Earth-out-of-view-phenomenon” may have possible consequences in increasing psychopathologies especially during LTSM. We propose that developing relevant therapies to counter this problem should be based on the “Attention Restoration Hypothesis” which postulates that natural environments may provide temporary psychological relief from demanding cognitive routines that require high attention levels, such as various tasks performed by astronauts. Thus, short-term involvement in natural environments may reduce “depleted attentional resources” (Atchley et al., 2012). It has been identified that restoration occurs in the pre-frontal cortex, which regulates executive functions while also modulating hypothalamic-pituitary-adrenal axis (HPA) stress response (Winkelman, 2000; Saniotis et al., 2016).
Linked to attention restoration is the idea of “Heraclitean motion” which refers to environmental phenomena that are both static yet changing (i.e., fire, clouds, ocean, fish in an aquarium, fountains) (Katcher and Wilkins, 1993). According to some authors, the act of viewing phenomena that manifest Heraclitean motion produces a self-hypnotic state in the viewer after a few minutes (Saniotis et al., 2016). The relatively easy ability for humans to enter into self-hypnotic states was probably positively selected by natural selection since such states conferred ancestral hominins with reduced stress response to threat and induced relaxation which increased fertility and emotional wellbeing (McClenon, 1997; Saniotis and Henneberg, 2011a). Moreover, ancestral hominins may have gazed into fires which may have contributed to the evolution of cognitive abilities such as increased attention span, which is a hallmark of Homo. On this note, fire gazing was probably one of several behavioural and morphological characteristics which ancestral hominins were undergoing that were a result of self-amplifying feedback mechanisms probably starting already in the Miocene (Henneberg and Eckhardt, 2022; Henneberg, 2025). For example, the extant practice of fire gazing (tatraka) that has been practiced for centuries in India has been suggested to increase focus and attention, cognitive performance and mental relaxation (Kumar, 2010; Raghavendra and Singh, 2016; Chundawat and Panda, 2023).
We posit that effects of Heraclitean motion via prolonged exposure to the natural world may induce attention restoration. As Saniotis et al. (2016) note:
While more empirical studies are needed in testing the viability of Heraclitean motion in relation to astronauts, it seems likely that Heraclitean motion may improve individual resilience, especially in sensorially monotonous and enclosed environments such as spaceships.
1. We speculate that Heraclitean motion can be employed via the use of virtual reality (VR) technology that would provide images of natural environments to astronauts. Virtual reality technology could be employed at specified times during astronauts’work cycles as a way of restoring attentional resources, as well as countering the “Earth-out-of-view-phenomenon” in “home sick’ individuals. The use of VR as a countermeasure for the “Earth-out-of-view-phenomenon” has been identified by Gushin et al. (2021).
2. In the near future, holographic technology (HT) may also incorporate 3D natural environments in different parts of the space craft in order to restore attention resources and foster connection with the Earth. Thus far, holographic teleportation has been successfully employed in teleporting members that have been projected virtually on the International Space Station (Llaca and Davidson, 2023). It is anticipated that as HT improves, specific natural environments could be projected in various areas of spacecraft accompanied by sounds and smells of the respective nature-based holographs. The combination of visual, auditory and olfactory-based holographic natural environments would provide a more realistic experience of natural scenes, as well as necessary sensorial stimulation for countering the claustrophobic and tedious spaceship ambience. It is also feasible to suggest that holographs combining the sights, sounds and smells of nature could act as:
1) an important sleeping aid for astronauts
2) increase psychological wellbeing by reducing stress induced glucocorticoids and maintaining healthy neuro-hormonal regulation in astronauts.
3. Due to the significant musculoskeletal deterioration effects of microgravity, astronauts are required to exercise for approximately 2 hours per day using stationary bicycles or treadmills (Braddock, 2018). Although exercise fills a mandatory part in astronauts’ waking hours, this regime can be monotonous and boring. In the future, VR or HT can be employed during exercise programs where astronauts move through nature simulations (including sounds and smells of natural landscapes as well as flora and fauna). Such simulations would be designed to integrate executive, motor, sensory and limbic regions, thus providing cognitive, sensorial and emotional experiences for astronauts with subsequential neuroprotective and mental health benefits.
9.5 The problem of consciousness
Astronauts are highly educated and very intelligent people. Therefore, they may realise that their exposure to technologically created Earth environments is artificial. This realisation may interfere with them relaxing and restoring their attention. They may become stressed by what they will perceive as “fake” natural environments, especially that those may present them with repeated, like a broken record, experiences. During a space flight, there are real opportunities for observing Heraclitean motions–the slowly rotating view of the surrounding universe and of pondering details of the actual living environment that, although being a technological construct, is comprised of natural chemical compounds–plastics, metals, textiles, etc. Introducing astronauts to the acceptance of their immediate environment as a part of the nature may help in their personal adaptation to their unusual lifestyle. A spaceship consists of atoms linked in various ways to produce its structure. The ship moves through the space that contains the Earth and other objects. These objects move in regular ways that inform a rhythm of observing them. The schedule of human activities aboard a spaceship also has its rhythm, that, though artificial, is also informed by the biological requirements of human bodies–sleep, meals, exercise, relaxation, work. A spaceflight is a natural portion of the universal world where feedbacks between human needs and activities and their surroundings occur in ways somewhat different from the past evolutionary experience, but essentially the same systemic interactions among humans and their surroundings.
9.6 Peri anthropon: natural selection and humans in outer space
It is more than likely that human beings born in space stations over several generations will undergo changes in their genome resulting from altered mutation/selection balance. Some space environments may be mutagenic due to different from the Earth levels of radiation. Spontaneous mutations occur on Earth at high rates. The human germline mutation rate is approximately 1.2 × 10−8 per nucleotide per generation (Wood and Goriely, 2022).
Operation of natural selection in space stations/colonies will be strongly relaxed due to the availability of medical technologies and artificial living conditions. Thus, the accumulation of even mildly deleterious mutations over generations may lead to genetic load, which is evident in extant human populations (Saniotis et al., 2020; You and Henneberg, 2016; 2017; You et al., 2022). Allelic changes may occur faster in more mutagenic environments.
Although the operation of natural selection will be largely relaxed, some extreme effects of changed environments may affect the reproductive fitness of some colonists, thus leading to microevolutionary adaptations to particular space environments. Possible effects of inbreeding resulting from small effective population size, pressures of gravity, radiation and magnetic fields may trigger mutational variants causing neuro-neurobehavioural changes in ways that we do not yet fully understand.
Notwithstanding medical and dietary interventions in ensuring healthy gut microbiomes in astronauts and future space station colonists, relaxed natural selection will probably reduce microbiota variability with subsequent alterations in neuro-hormonal/neuro-trophic regulation and the growth of gut derived neurotransmitters. On this theme, Silva et al. (2023) note that changes to the gut microbiome will likely occur in response to the lack of exposure to widely varied microbiota colonies found in terrestrial environments (Silva et al., 2023).
As noted in numerous space studies, changes in gravity during space missions exert fundamental changes to astronauts’ bodies. The influence of gravity is significantly marked in growing offspring. Growth plates of human bones respond to mechanical forces acting on them (Alonso et al., 2022). Thus, children growing in an environment of lower force of gravity may have their growth disturbed in one way, e.g., growing very tall, while those growing under greater than Earth’s force of gravity will have growth disturbed in a different way, e.g., being very short and stocky. Concomitantly, gravity also affects muscle growth that is dependent on mechanical loads during their use. This, in turn contributes to proprioceptive sensory nerve signals from the musculoskeletal apparatus and motor signals to muscles which probably influence cognition. On this point, it has been speculated that motor neural circuits are implicated in thinking and cognition (Pilacinski et al., 2025; Baladron and Hamker, 2024). Therefore, voluntary muscular control, involuntary reflexes and cognitive abilities in inter-generational space station colonists could evolve differently from humans on Earth.
10 Conclusion
This paper has aimed to improve our understanding of the neuro-behavioural challenges which current astronauts face, as well as future astronauts and space colonists, from an evolutionary perspective. Evolutionary medicine has yet to be used as an explanatory model for addressing possible adverse mental health effects of humans during LTSM. This oversight needs to be corrected due to the increasing investment by space agencies and national governments in outer space exploration. Due to the evolutionary mismatches currently confronting astronauts’ mental health, this paper has discussed novel therapeutic countermeasures based on evolutionary principles for reducing adverse mental effects on astronauts and future space colonists. As we are still at a pioneering stage in space exploration the development of mental health strategies which privilege evolutionary principles will improve our current knowledge of bioastronautics.
Data availability statement
The original contributions presented in the study are included in the article/supplementary material, further inquiries can be directed to the corresponding author.
Author contributions
AS: Conceptualization, Validation, Writing – original draft, Writing – review and editing. MH: Validation, Writing – original draft, Writing – review and editing. KM: Writing – original draft, Writing – review and editing.
Funding
The author(s) declare that no financial support was received for the research and/or publication of this article.
Conflict of interest
The authors declare that the research was conducted in the absence of any commercial or financial relationships that could be construed as a potential conflict of interest.
Generative AI statement
The author(s) declare that no Generative AI was used in the creation of this manuscript.
Publisher’s note
All claims expressed in this article are solely those of the authors and do not necessarily represent those of their affiliated organizations, or those of the publisher, the editors and the reviewers. Any product that may be evaluated in this article, or claim that may be made by its manufacturer, is not guaranteed or endorsed by the publisher.
References
Abram D. (1996). The spell of the sensuous: perception and language in a more-than-human world. 1st ed. New York: Pantheon Books.
Adams G. R., Caiozzo V. J., Baldwin K. M. (2003). Skeletal muscle unweighting: spaceflight and ground-based models. J. Appl. Physiol. 95 (6), 2185–2201. doi:10.1152/japplphysiol.00346.2003
Agrawal A. F., Whitlock M. C. (2012). Mutation load: the fitness of individuals in populations where deleterious alleles are abundant. Annu. Rev. Ecol. Evol. Syst. 43 (1), 115–135. doi:10.1146/annurev-ecolsys-110411-160257
Aiello L. C., Wheeler P. (1995). The expensive-tissue hypothesis: the brain and the digestive system in human and primate evolution. Curr. Anthropol. 36 (2), 199–221. doi:10.1086/204350
Alcock J., Maley C. C., Aktipis C. A. Is eating behavior manipulated by the gastrointestinal microbiota? Evolutionary pressures and potential mechanisms. BioEssays (2014) 36, 940–949. doi:10.1002/bies.201400071
Aleman F. D. D., Valenzano D. R. (2019). Microbiome evolution during host aging. PLoS Pathog. 15 (7), e1007727. doi:10.1371/journal.ppat.1007727
Alonso G., Yawny A., Bertolino G. (2022). How do bones grow? A mathematical description of the mechanobiological behavior of the epiphyseal plate. Biomechanics Model. Mechanobiol. 21 (5), 1585–1601. doi:10.1007/s10237-022-01608-y
Anderson K., Bradley A. J. (2013). Sleep disturbance in mental health problems and neurodegenerative disease. Nat. Sci. Sleep 5, 61–75. doi:10.2147/NSS.S34842
Arone A., Ivaldi T., Loganovsky K., Palermo S., Parra E., Flamini W., et al. (2021). The burden of space exploration on the mental health of astronauts: a narrative review. Clin. Neuropsychi 18 (5), 237–246. doi:10.36131/cnfioritieditore20210502
Atchley R. A., Strayer D. L., Atchley P. (2012). Creativity in the wild: improving creative reasoning through immersion in natural settings. PLoS One 7 (12), e51474. doi:10.1371/journal.pone.0051474
Baladron J., Hamker F. H. (2024). Re-thinking the organization of cortico-basal ganglia-thalamo-cortical loops. Cogn. Comput. 16 (5), 2405–2410. doi:10.1007/s12559-023-10140-9
Barger L. K., Flynn-Evans E. E., Kubey A., Walsh L., Ronda J. M., Wang W., et al. (2014). Prevalence of sleep deficiency and use of hypnotic drugs in astronauts before, during, and after spaceflight: an observational study. Lancet Neurol. 13 (9), 904–912. doi:10.1016/S1474-4422(14)70122-X
Bednarik R. G., Saniotis A., Henneberg M. (2022). Auto-domestication hypothesis and the rise in mental disorders in modern humans. Med. Hypothe. 164, 110874. doi:10.1016/j.mehy.2022.110874
Beeson C., Kosal E. (2023). Evolutionary medicine. Am. Biol. Teach. 85 (2), 80–84. doi:10.1525/abt.2023.85.2.80
Bharindwal S., Goswami N., Jha P., Pandey S., Jobby R. (2023). Prospective use of probiotics to maintain astronaut health during spaceflight. Life 13 (3), 727. doi:10.3390/life13030727
Blaser M. J., Falkow S. (2009). What are the consequences of the disappearing human microbiota? Nat. Rev. Microbiol. 7 (12), 887–894. doi:10.1038/nrmicro2245
Bos D. J., van Montfort S. J., Oranje B., Durston S., Smeets P. A. (2016). Effects of omega-3 polyunsaturated fatty acids on human brain morphology and function: what is the evidence? Eur. Neuropsychopharmacol. 26 (3), 546–561. doi:10.1016/j.euroneuro.2015.12.031
Bowler D. E., Buyung-Ali L. M., Knight T. M., Pullin A. S. (2010). A systematic review of evidence for the added benefits to health of exposure to natural environments. BMC Public Health 10 (1), 456. doi:10.1186/1471-2458-10-456
Braddock M. (2018). Exercise and ergonomics on the international apace station and orion spacecraft. J Ergon Res 1, 2. doi:10.4172/JEOR.1001104
Brzhozovskiy A. G., Kononikhin A. S., Pastushkova L. C., Kashirina D. N., Indeykina M. I., Popov I. A., et al. (2019). The effects of spaceflight factors on the human plasma proteome, including both real space missions and ground-based experiments. Int. J. Mol. Sci. 20, 3194. doi:10.3390/ijms20133194
Chaumet G., Taillard J., Sagaspe P., Pagani M., Dinges D. F., Pavy-Le-Traon A., et al. (2009). Confinement and sleep deprivation effects on propensity to take risks. Aviat. Space, Environ. Med. 80 (2), 73–80. doi:10.3357/ASEM.2366.2009
Chundawat A., Panda S. K. (2023). Tratak and its benefits: a review. J. Ayurveda Integr. Med. Sci. 8 (5), 61–65. doi:10.21760/jaims.8.5.10
Conrad C. D. (2008). Chronic stress-induced hippocampal vulnerability: the glucocorticoid vulnerability hypothesis. Rev. Neurosci. 19 (6), 395–411. doi:10.1515/REVNEURO.2008.19.6.395
Conrad C. D., LeDoux J. E., Magariños A. M., McEwen B. S. (1999). Repeated restraint stress facilitates fear conditioning independently of causing hippocampal CA3 dendritic atrophy. Behav. Neurosci. 113 (5), 902–913. doi:10.1037/0735-7044.113.5.902
Cope H., Willis C. R. G., MacKay M. J., Rutter L. A., Toh L. S., Williams P. M., et al. (2022). Routine omics collection is a golden opportunity for European human research in space and analog environments. Patterns (N Y) 3 (10), 100550. doi:10.1016/j.patter.2022.100550
Criscuolo F., Sueur C., Bergouignan A. (2020). Human adaptation to deep space environment: an evolutionary perspective of the foreseen interplanetary exploration. Front. Public Health 8, 119. doi:10.3389/fpubh.2020.00119
Cryan J. F., Dinan T. G. (2012). Mind-altering microorganisms: the impact of the gut microbiota on brain and behaviour. Nat. Rev. Neurosci. 13 (10), 701–712. doi:10.1038/nrn3346
Cucinotta F. A., Durante M. (2006). Cancer risk from exposure to galactic cosmic rays: implications for space exploration by human beings. Lancet Oncol. 7 (5), 431–435. doi:10.1016/S1470-2045(06)70695-7
Danko D. C., Singh N., Butler D. J., Mozsary C., Jiang P., Keshavarzian A., et al. (2020). Genetic and immunological evidence for microbial transfer between the international space station and an astronaut. bioRxiv, 1–24. doi:10.1101/2020.11.10.376954
Davenport E. R., Sanders J. G., Song S. J., Amato K. R., Clark A. G., Knight R. (2017). The human microbiome in evolution. BMC Biol. 15 (1), 127. doi:10.1186/s12915-017-0454-7
Davidson G. L., Cooke A. C., Johnson C. N., Quinn J. L. (2018). The gut microbiome as a driver of individual variation in cognition and functional behaviour. Philosoph. Trans. R. Soc. B Biol. Sci. 373 (1756), 20170286. doi:10.1098/rstb.2017.0286
De Kloet E. R. (2004). Hormones and the stressed brain. Ann. N. Y. Acad. Sci. 1018 (1), 1–15. doi:10.1196/annals.1296.001
De Kloet E. R., Joëls M., Holsboer F. (2005). Stress and the brain: from adaptation to disease. Nat. Rev. Neurosci. 6 (6), 463–475. doi:10.1038/nrn1683
De La Torre G. G., van Baarsen B., Ferlazzo F., Kanas N., Weiss K., Schneider S., et al. (2012). Future perspectives on space psychology: recommendations on psychosocial and neurobehavioural aspects of human spaceflight. Acta Astronaut. 81 (2), 587–599. doi:10.1016/j.actaastro.2012.08.013
Desbonnet L., Garrett L., Clarke G., Bienenstock J., Dinan T. G. (2008). The probiotic Bifidobacteria infantis: an assessment of potential antidepressant properties in the rat properties in the rat. J. Psychiatr. Res. 43, 164–174. doi:10.1016/j.jpsychires
Dicks L. M. T. (2022). Gut bacteria and neurotransmitters. Microorganisms 10 (9), 1838. doi:10.3390/microorganisms10091838
Dighriri I. M., Alsubaie A. M., Hakami F. M., Hamithi D. M., Alshekh M. M., Khobrani F. A., et al. (2022). Effects of omega-3 polyunsaturated fatty acids on brain functions: a systematic review. Cureus 14, e30091. [Preprint]. doi:10.7759/cureus.30091
Fitts R. H., Riley D. R., Widrick J. J. (2001). Functional and structural adaptations of skeletal muscle to microgravity. J. Exp. Biol. 204 (18), 3201–3208. doi:10.1242/jeb.204.18.3201
Flynn-Evans E. E., Barger L. K., Kubey A. A., Sullivan J. P., Czeisler C. A. (2016). Circadian misalignment affects sleep and medication use before and during spaceflight. NPJ Microgr. 2 (1), 15019. doi:10.1038/npjmgrav.2015.19
Garrett-Bakelman F. E., Darshi M., Green S. J., Gur R. C., Lin L., Macias B. R., et al. (2019). The NASA Twins Study: a multidimensional analysis of a year-long human spaceflight. Science 364 (6436), eaau8650. doi:10.1126/science.aau8650
Ghlichloo I., Gerriets V. (2023). “Nonsteroidal anti-inflammatory drugs (NSAIDs),” in StatPearls [Internet]. Treasure Island, FL: StatPearls Publishing.
Gonfalone A. (2016). Sleep on manned space flights: zero gravity reduces sleep duration. Pathophysiology 23 (4), 259–263. doi:10.1016/j.pathophys.2016.08.003
Grinde B., Patil G. G. (2009). Biophilia: does visual contact with nature impact on health and well-being? Int. J. Environ. Res. Public Health 6 (9), 2332–2343. doi:10.3390/ijerph6092332
Groenewegen P. P., van den, Berg A. E., de Vries S., Verheij R. A. (2006). Vitamin G: effects of green space on health, well-being, and social safety. BMC Public Health 6 (1), 149. doi:10.1186/1471-2458-6-149
Gullone E. (2000). The biophilia hypothesis and life in the 21st century: increasing mental health or increasing pathology? J. Happiness Stud. 1 (3), 293–322. doi:10.1023/A:1010043827986
Guo J.-H., Qu W. M., Chen S. G., Chen X. P., Lv K., Huang Z. L., et al. (2014). Keeping the right time in space: importance of circadian clock and sleep for physiology and performance of astronauts. Mil. Med. Res. 1 (1), 23. doi:10.1186/2054-9369-1-23
Gupta S., Allen-Vercoe E., Petrof E. O. (2016). Fecal microbiota transplantation: in perspective. Ther. Adv. Gastroenterol. 9 (2), 229–239. doi:10.1177/1756283X15607414
Gushin V., Ryumin O., Karpova O., Rozanov I., Shved D., Yusupova A. (2021). Prospects for psychological supportin interplanetary expeditions. Front. Physiol. 12, 750414. doi:10.3389/fphys.2021.750414
Hartmann A., Schütz H. J., Alberti E., Schreckenberger F., Loew D., Dýcka J. (1977). Effects of a new diuretic on cerebrospinal fluid pressure in patients with supratentorial tumors. Arch. Psychiatr. Nervenkr 224, 351–360. doi:10.1007/BF00341617
Hawks J., Wang E. T., Cochran G. M., Harpending H. C., Moyzis R. K. (2007). Recent acceleration of human adaptive evolution. Proc. Natl. Acad. Sci. U. S. A. 104 (52), 20753–20758. doi:10.1073/pnas.0707650104
Heerwagen J.(2009). Biophilia, health and well-being. In: restorative commons: Creating health and well-being through urban landscapes, 39–57.
Henneberg M. (1998). Evolution of the human brain: is bigger better. Exp. Clin. Pharmacol. 25, 745–749. doi:10.1111/j.1440-1681.1998.tb02289.x
Henneberg M. (2025). Our one human family. A story of continuing evolution. New York: NOVA Publishers.
Henneberg M., Eckhardt R. B. (2022). Evolution of modern humans is a result of self-amplifying feedbacks beginning in the Miocene and continuing without interruption until now. Anthropol. Rev. 85 (1), 77–83. doi:10.18778/1898-6773.85.1.05
Henneberg M., Sarafis V., Mathers K. (1998). Human adaptations to meat eating. Hum. Evol. 13 (3), 229–234. Available online at: https://www.jstor.org/stable/41464021. doi:10.1007/BF02436507
Henneberg M., Steyn M. (1993). Trends in cranial capacity and cranial index in Subsaharan Africa during the Holocene. Am. J. Hum. Biol. 5 (4), 473–479. doi:10.1002/ajhb.1310050411
Horneck G., Klaus D. M., Mancinelli R. L. (2010). Space microbiology. Microbiol. Mol. Biol. Rev. 74 (1), 121–156. doi:10.1128/MMBR.00016-09
Huang A. S., Stenger M. B., Macias B. R. (2019). Gravitational influence on intraocular pressure: implications for spaceflight and disease. J. Glaucoma 28, 756–764. doi:10.1097/IJG.0000000000001293
Ihle E. C., Ritsher J. B., Kanas N. (2006). Positive psychological outcomes of spaceflight: an empirical study. Aviat. Space, Environ. Med. 77, 93–101.
Itan Y., Powell A., Beaumont M. A., Burger J., Thomas M. G. (2009). The origins of lactase persistence in Europe. PLoS Comput. Biol. 5 (8), e1000491. doi:10.1371/journal.pcbi.1000491
Johnson D., Letchumanan V., Thum C. C., Thurairajasingam S., Lee L. H. (2023). A microbial-based approach to mental health: the potential of probiotics in the treatment of depression. Nutrients 15 (6), 1382. doi:10.3390/nu15061382
Johnson D., Thurairajasingam S., Letchumanan V., Chan K. G., Lee L. H. (2021). Exploring the role and potential of probiotics in the field of mental health: major depressive disorder. Nutrients 13 (5), 1728. doi:10.3390/nu13051728
Kanas N. (1985). Psychosocial factors affecting simulated and actual space missions. Aviat. Space, Environ. Med. 56, 806–811.
Kanas N. (1987). Psychological and interpersonal issues in space. Am. J. Psychiatry 144 (6), 703–709. doi:10.1176/ajp.144.6.703
Kanas N. (2011). From Earth’s orbit to the outer planets and beyond: psychological issues in space. Acta Astronaut. 68 (5–6), 576–581. doi:10.1016/j.actaastro.2010.04.012
Kanas N., Manzey D. (2008). Space psychology and psychiatry. 2nd ed. El Segundo, California, and Springer Dordrecht, Netherlands: Microcosm Press.
Katcher A., Wilkins G. (1993). “Dialogue with animals: its nature and culture,” in The biophilia hypothesis. Editors S. R. Kellert, and E. O. Wilson (Covelo, California: Island Press), 173–197.
Keller M. C. (2008). The evolutionary persistence of genes that increase mental disorders risk. Curr. Dir. Psychol. Sci. 17 (6), 395–399. doi:10.1111/j.1467-8721.2008.00613.x
Kelly T. H., Hienz R. D., Zarcone T. J., Wurster R. M., Brady J. V. (2005). Crewmember performance before, during, and after spaceflight. J. Exp. Analysis Behav. 84 (2), 227–241. doi:10.1901/jeab.2005.77-04
Kim E. J., Pellman B., Kim J. J. (2015). Stress effects on the hippocampus: a critical review. Learn. Mem. 22 (9), 411–416. doi:10.1101/lm.037291.114
Kim N., Yun M., Oh Y. J., Choi H. J. (2018). Mind-altering with the gut: modulation of the gut-brain axis with probiotics. J. Microbiol. 56 (3), 172–182. doi:10.1007/s12275-018-8032-4
Kozlovskaya I. B., Kreidich Y. V., Oganov V. S., Koserenko O. P. (1981). Pathophysiology of motor functions in prolonged manned space flights. Acta Astronaut. 8 (9–10), 1059–1072. doi:10.1016/0094-5765(81)90079-5
Krippner S. (2000). The epistemology and technologies of shamanic states of consciousness. J. Conscious. Stud. 7 (11–12), 93–118.
Le Roy B., Martin-Krumm C., Trousselard M. (2023). Mindfulness for adaptation to analog and new technologies emergence for long-term space missions. Front. Space Technol. 4, 1109556. doi:10.3389/frspt.2023.1109556
Ley R. E., Peterson D. A., Gordon J. I. (2006). Ecological and evolutionary forces shaping microbial diversity in the human intestine. Cell 124 (4), 837–848. doi:10.1016/j.cell.2006.02.017
Li J., Mookerjee B., Singh P., Wagner J. L. (2008). Generation of bkv-specific t cells for adoptive therapy against bkv nephropathy. Virology Res. Treat. 1. VRT.S942. doi:10.4137/VRT.S942
Liao W. H., Henneberg M., Langhans W. (2016)Immunity-based evolutionary interpretation of diet-induced thermogenesis, Cell Metab. 23: 971–979. doi:10.1016/j.cmet.2016.05.002
Liu X., Huang J., Jiang Y., Cao Z., Wu M., Sun R., et al. (2022). IL-6 and IL-8 are likely associated with psychological status in treatment naïve general population. J. Affect. Disord. 298, 337–344. doi:10.1016/j.jad.2021.10.042
Llaca F. D. L. P., Davidson P. L. (2023). Holographic teleportation in space and astronauts’ stress: a Delphi study. Sci. Talks 6 (2), 100228. doi:10.1016/j.sctalk.2023.100228
Lloyd-Price J., Abu-Ali G., Huttenhower C. (2016). The healthy human microbiome. Genome Med. 8, 51. doi:10.1186/s13073-016-0307-y
Mader T. H., Gibson C. R., Pass A. F., Kramer L. A., Lee A. G., Fogarty J., et al. (2011). Optic disc edema, globe flattening, choroidal folds, and hyperopic shifts observed in astronauts after long-duration space flight. Ophthalmology 118 (10), 2058–2069. doi:10.1016/j.ophtha.2011.06.021
Mann N. (2007). Meat in the human diet: an anthropological perspective. Nutr. Dietet. 64 (s4). doi:10.1111/j.1747-0080.2007.00194.x
Marazziti D., Arone A., Ivaldi T., Kut s K., Loganovsky K. (2022). Space missions: psychological and psychopathological issues. CNS Spectrums 27 (5), 536–540. doi:10.1017/S1092852921000535
Marsh M. S. (2007). Identification of psychological stressors for long duration space missions: psychological stressors among five astronauts and cosmonauts. Theses Diss. 754. Available online at: https://commons.und.edu/theses/754.
Maust D. T., Lin L. A., Blow F. C. (2019). Benzodiazepine use and misuse among adults in the United States. Psychiatr. Serv. 70 (2), 97–106. doi:10.1176/appi.ps.201800321
McClenon J. (1997). Shamanic healing, human evolution, and the origin of religion. J. Sci. Study Relig. 36 (3), 345. doi:10.2307/1387852
Mehare A., Chakole S., Wandile B. (2024). Navigating the unknown: a comprehensive review of spaceflight-associated neuro-ocular syndrome. Cureus 16 (2), e53380. doi:10.7759/cureus.53380
Mekel-Bobrov N., Gilbert S. L., Evans P. D., Vallender E. J., Anderson J. R., Hudson R. R., et al. (2005). Ongoing adaptive evolution of aspm, a brain size determinant in homo sapiens. Science 309 (5741), 1720–1722. doi:10.1126/science.1116815
Moeller A. H., Li Y., Mpoudi Ngole E., Ahuka-Mundeke S., Lonsdorf E. V., Pusey A. E., et al. (2014). Rapid changes in the gut microbiome during human evolution. Proc. Natl. Acad. Sci. U. S. A. 111 (46), 16431–16435. doi:10.1073/pnas.1419136111
Mohajeri M. H., La Fata G., Steinert R. E., Weber P. (2018). Relationship between the gut microbiome and brain function. Nutr. Rev. 76, 481–496. doi:10.1093/nutrit/nuy009
Montiel-Castro A. J., González-Cervantes R. M., Bravo-Ruiseco G., Pacheco-López G. (2013). The microbiota-gut-brain axis: neurobehavioral correlates, health and sociality. Front. Integr. Neurosci. 7, 70. doi:10.3389/fnint.2013.00070
Mortazavi S. M. J., Said-Salman I., Mortazavi A. R., El Khatib S., Sihver L. (2024). How the adaptation of the human microbiome to harsh space environment can determine the chances of success for a space mission to Mars and beyond. Front. Microbiol. 14, 1237564. doi:10.3389/fmicb.2023.1237564
Natterson-Horowitz B., Aktipis A., Fox M., Gluckman P. D., Low F. M., Mace R., et al. (2023). The future of evolutionary medicine: sparking innovation in biomedicine and public health. Front. Sci. 1, 997136. doi:10.3389/fsci.2023.997136
Nesse R. M., Williams G. C. (2012). Why we get sick: the new science of Darwinian medicine. New York: Vintage Books.
Nucci C., Martucci A., Giannini C., Morrone L. A., Bagetta G., Mancino R. (2018). Neuroprotective agents in the management of glaucoma. Eye 32, 938–945. doi:10.1038/s41433-018-0050-2
Odamaki T., Kato K., Sugahara H., Hashikura N., Takahash S., Xiao J. Z., et al. (2016). Age-related changes in gut microbiota composition from newborn to centenarian: a cross-sectional study. BMC Microbiol. 16 (1), 90. doi:10.1186/s12866-016-0708-5
O’Hara A. M., Shanahan F. (2006). The gut flora as a forgotten organ. EMBO Rep. 7 (7), 688–693. doi:10.1038/sj.embor.7400731
Ong J., Tarver W., Brunstetter T., Mader T. H., Gibson C. R., Mason S. S., et al. (2023). Spaceflight associated neuro-ocular syndrome: proposed pathogenesis, terrestrial analogues, and emerging countermeasures. Br. J. Ophthalmol. 107 (7), 895–900. doi:10.1136/bjo-2022-322892
Opsina M. B., Bond K., Karkhaneh M., Tjosvold L., Vandermeer B., Yuanyuan Liang Y., et al. (2007). Meditation practices for health: state of the research. Evid. Rep. Technol. Assess. (Full Rep.) 155, 1–263.
Ortiz J. B., Conrad C. D. (2018). The impact from the aftermath of chronic stress on hippocampal structure and function: is there a recovery? Front. Neuroendocrinol. 49, 114–123. doi:10.1016/j.yfrne.2018.02.005
Paez M. Y., Mudie L. I., Subramanian P. S. (2020). Spaceflight associated neuro-ocular syndrome (SANS): a systematic review and future directions. Eye Brain 19 (12), 105–117. doi:10.2147/EB.S234076
Pastushkova L. K., Rusanov V. B., Goncharova A. G., Nosovskiy A. M., Luchitskaya E. S., Kashirina D. N., et al. (2021). Blood plasma proteins associated with heart rate variability in cosmonauts who have completed long-duration space missions. Front. Physiol. 12, 760875. doi:10.3389/fphys.2021.760875
Perry G. H., Dominy N. J., Claw K. G., Lee A. S., Fiegler H., Redon R., et al. (2007). Diet and the evolution of human amylase gene copy number variation. Nat. Genet. 39 (10), 1256–1260. doi:10.1038/ng2123
Peterson C. T. (2020). Dysfunction of the microbiota-gut-brain axis in neurodegenerative disease: the promise of therapeutic modulation with prebiotics, medicinal herbs, probiotics, and synbiotics. J. Evidence-Based Integr. Med. 25, 2515690X20957225. doi:10.1177/2515690X20957225
Pierson D. L., Chidambaram M., Heath J. D., Mallary L., Mishra S. K., Sharma B., et al. (1996). Epidemiology of Staphylococcus aureus during space flight. FEMS Immunol. Med. Microbiol. 16 (3–4), 273–281. doi:10.1111/j.1574-695X.1996.tb00146.x
Pilacinski A., Wagner L., Besson G., Matos M. J., Araujo E., Klaes C. (2025). Spontaneous eye movements reveal that premotor cortex is involved in human thinking. bioRxiv, 1–25. doi:10.1101/2025.03.11.642662
Popova N. K., Kulikov A. V., Naumenko V. S. (2020). Spaceflight and brain plasticity: spaceflight effects on regional expression of neurotransmitter systems and neurotrophic factors encoding genes. Neurosci. Biobehav. Rev. 119, 396–405. doi:10.1016/j.neubiorev.2020.10.010
Raghavendra B. R., Singh P. (2016). Immediate effect of yogic visual concentration on cognitive performance. J. Tradit. Complem. Med. 6 (1), 34–36. doi:10.1016/j.jtcme.2014.11.030
Rappaport M. B., Corbally C. J. (2023). Toward an etiology of spaceflight neuroplastic syndrome: evolutionary science leads to new hypotheses and program priorities. NeuroSci 4 (4), 247–262. doi:10.3390/neurosci4040021
Roccisano D., Kumaratilake J., Saniotis A., Henneberg M. (2016). Dietary fats and oils: some evolutionary and historical perspectives concerning edible lipids for human consumption. Food Nutr. Sci. 07 (08), 689–702. doi:10.4236/fns.2016.78070
Ross M. D., Tomko D. L. (1998). Effect of gravity on vestibular neural development. Brain Res. Rev. 28 (1–2), 44–51. doi:10.1016/S0165-0173(98)00025-3
Ruff C. (2002). Variation in human body size and shape. Annu. Rev. Anthropol. 31 (1), 211–232. doi:10.1146/annurev.anthro.31.040402.085407
Rühli F. J., Henneberg M. (2013). New perspectives on evolutionary medicine: the relevance of microevolution for human health and disease. BMC Med. 11 (1), 115. doi:10.1186/1741-7015-11-115
Rutter L. A., Cope H., MacKay M. J., Herranz R., Das S., Ponomarev S. A., et al. (2024). Astronaut omics and the impact of space on the human body at scale. Nat. Commun. 15 (1), 4952. doi:10.1038/s41467-024-47237-0
Saniotis A., De la Torre G. G., Galassi F. M., Henneberg M., Mohammadi K. (2023). Mindfulness meditation and spaceflight: a potential adjunct therapy for astronauts. MIND Bull. Mind-Body Med. Res. 3, 4–5. doi:10.61936/themind/202312222
Saniotis A., Galassi F. M., Henneberg M. (2024). Possible future evolutionary consequences to Homo as a result of the implementation of biotechnology. Anthropol. Rev. 87 (1), 69–80. doi:10.18778/1898-6773.87.1.05
Saniotis A., Grantham J. P., Kumaratilake J., Henneberg M. (2020). Neuro-hormonal regulation is a better indicator of human cognitive abilities than brain anatomy: the need for a new paradigm. Front. Neuroanat. 13, 101. doi:10.3389/fnana.2019.00101
Saniotis A., Henneberg M. (2011a). An evolutionary approach toward exploring altered states of consciousness, mind–body techniques, and non-local mind. World Futur. 67 (3), 182–200. doi:10.1080/02604027.2011.555250
Saniotis A., Henneberg M. (2011b). Medicine could be constructing human bodies in the future. Med. Hypothe. 77 (4), 560–564. doi:10.1016/j.mehy.2011.06.031
Saniotis A., Henneberg M., Ebrahimi G. (2016). Human microevolution in outer space. J. Futur. Stud. 20 (4), 61–78. doi:10.6531/JFS.2016.20(4).A61
Saniotis A., Henneberg M., Mohammadi K. (2021). Genetic load and biological changes to extant humans. J. Biosoc. Sci. 53 (4), 639–642. doi:10.1017/S0021932020000413
Shahab M., Shahab N.(2022). Coevolution of the human host and gut microbiome: Metagenomics of microbiota. Cureus 14(6), e26310. doi:10.7759/cureus.26310
Siddiqui R., Akbar N., Khan N. A. (2021b). Gut microbiome and human health under the space environment. J. Appl. Microbiol. 130 (1), 14–24. doi:10.1111/jam.14789
Siddiqui R., Qaisar R., Goswami N., Khan N. A., Elmoselhi A. (2021a). Effect of microgravity environment on gut microbiome and angiogenesis. Life 11 (10), 1008. doi:10.3390/life11101008
Silva I. D. C. E., Russomano T., Ferreira R. A., Cupertino M. D. C., Alcântara F. A., Geller M., et al. (2023). Physiological adaptations to life in space: an update. J. Aerosp. Technol. Manag. 15, 1–14. doi:10.1590/jatm.v15.1319
Stearns S. C. (2012). Evolutionary medicine: its scope, interest and potential. Proc. R. Soc. B Biol. Sci. 279 (1746), 4305–4321. doi:10.1098/rspb.2012.1326
Stearns S. C., Nesse R. M., Govindaraju D. R., Ellison P. T. (2010). Evolutionary perspectives on health and medicine. Proc. Natl. Acad. Sci. 107 (Suppl. l_1), 1691–1695. doi:10.1073/pnas.0914475107
Stingl J. C., Welker S., Hartmann G., Damann V., Gerzer R. (2015). Where failure is not an option—personalized medicine in astronauts. PLoS One 10, e0140764. doi:10.1371/journal.pone.0140764
The Human Microbiome Project Consortium (2012). Structure, function and diversity of the healthy human microbiome. Nature 486 (7402), 207–214. doi:10.1038/nature11234
Trevathan W. (2007). Evolutionary medicine. Annu. Rev. Anthropol. 36, 139–154. doi:10.1146/annurev.anthro.36.081406.094321
Trousselard M., Steiler D., Raphel C., Cian C., Duymedjian R., Claverie D., et al. (2010). Validation of a French version of the Freiburg Mindfulness Inventory - short version: relationships between mindfulness and stress in an adult population. Biopsychosoc. Med. 4 (1), 8. doi:10.1186/1751-0759-4-8
Tsai S.-J. (2021). Role of interleukin 8 in depression and other psychiatric disorders. Prog. Neuro-Psychopharmacol. Biol. Psychi. 106, 110173. doi:10.1016/j.pnpbp.2020.110173
Velarde Ma.D., Fry G., Tveit M. (2007). Health effects of viewing landscapes – landscape types in environmental psychology. Urban For. Urban Green. 6 (4), 199–212. doi:10.1016/j.ufug.2007.07.001
Vernikos J., Deepak A., Sarkar D. K., Rickards C. A., Convertina V. A. (2012). Yoga therapy as a complement to astronaut health and emotional fitness – stress reduction and countermeasure effectiveness before, during, and in post-flight rehabilitation: a hypothesis. Gravitational Space Biol. 26 (1), 65–76.
Voorhies A. A., Mark Ott C., Mehta S., Pierson D. L., Crucian B. E., Feiveson A., et al. (2019). Study of the impact of long-duration space missions at the International Space Station on the astronaut microbiome. Sci. Rep. 9 (1), 9911. doi:10.1038/s41598-019-46303-8
Vyas A., Pillai A. G., Chattarji S. (2004). Recovery after chronic stress fails to reverse amygdaloid neuronal hypertrophy and enhanced anxiety-like behavior. Neuroscience 128 (4), 667–673. doi:10.1016/j.neuroscience.2004.07.013
Willis C. R. G., Gallagher I. J., Wilkinson D. J., Brook M. S., Bass J. J., Phillips B. E., et al. (2021). Transcriptomic links to muscle mass loss and declines in cumulative muscle protein synthesis during short-term disuse in healthy younger humans. FASEB J. 35, e21830. doi:10.1096/fj.202100276RR
Wilson J. W., Thibeault S. A., Cucinotta F. A., Shinn J. L., Kim M., Kiefer R., et al. (1995). Issues in protection from galactic cosmic rays. Radiat. Environ. Biophys. 34 (4), 217–222. doi:10.1007/BF01209745
Winkelman M. (2004). Shamanism as the original neurotheology. Zygon 39 (1), 193–217. doi:10.1111/j.1467-9744.2004.00566.x
Winkelman M. J. (2000). Shamanism: the neural ecology of consciousness and healing. 1st ed. Westport, CT: Praeger. doi:10.5040/9798216986232
Wood K. A., Goriely A. (2022). The impact of paternal age on new mutations and disease in the next generation. Fertil. Steril. 118 (6), 1001–1012. doi:10.1016/j.fertnstert.2022.10.017
Wotring V. E. (2015). Medication use by u. S. Crewmembers on the international space station. FASEB J. 29 (11), 4417–4423. doi:10.1096/fj.14-264838
Wu B., Wang Y., Wu X., Liu D., Xu D., Wang F. (2018). On-orbit sleep problems of astronauts and countermeasures. Mil. Med. Res. 5 (1), 17. doi:10.1186/s40779-018-0165-6
Yang J. W., Song Q. Y., Zhang M. X., Ai J. L., Wang F., Kan G. H., et al. (2022). Spaceflight-associated neuro-ocular syndrome: a review of potential pathogenesis and intervention. Int. J. Ophthalmol. 15, 336–341. doi:10.18240/ijo.2022.02.21
Yong S. J., Tong T., Chew J., Lim W. L. (2020). Antidepressive mechanisms of probiotics and their therapeutic potential. Front. Neurosci. 13, 1361. doi:10.3389/fnins.2019.01361
You W., Henneberg M. (2017). Cancer incidence increasing globally: the role of relaxed natural selection. Evol. Appl. 11 (2), 140–152. doi:10.1111/eva.12523
You W., Henneberg R., Henneberg M. (2022). Healthcare services relaxing natural selection may contribute to increase of dementia incidence. Sci. Rep. 12, 8873. doi:10.1038/s41598-022-12678-4
You W.-P., Henneberg M. (2016). Type 1 diabetes prevalence increasing globally and regionally: the role of natural selection and life expectancy at birth. BMJ Open Diabetes Res. Care 4 (1), e000161. doi:10.1136/bmjdrc-2015-000161
Keywords: evolutionary mismatch, hormonal dysregulation, spaceflight-associated neuro-ocular syndrome, spaceflight-associated dysbiosis, circadian disruption, Earth-out-of-view-phenomenon, relaxed natural selection, evolutionary based countermeasures
Citation: Saniotis A, Henneberg M and Mohammadi K (2025) Evolutionary medicine and bioastronautics: an innovative approach in addressing adverse mental health effects to astronauts during long term space missions. Front. Physiol. 16:1558625. doi: 10.3389/fphys.2025.1558625
Received: 19 January 2025; Accepted: 08 April 2025;
Published: 24 April 2025.
Edited by:
Lidia Strigari, IRCCS Azienda Ospedaliero-Universitaria di Bologna, ItalyReviewed by:
Adel B Elmoselhi, University of Sharjah, United Arab EmiratesMelvin S. Marsh, Alabama State University, United States
Copyright © 2025 Saniotis, Henneberg and Mohammadi. This is an open-access article distributed under the terms of the Creative Commons Attribution License (CC BY). The use, distribution or reproduction in other forums is permitted, provided the original author(s) and the copyright owner(s) are credited and that the original publication in this journal is cited, in accordance with accepted academic practice. No use, distribution or reproduction is permitted which does not comply with these terms.
*Correspondence: Arthur Saniotis, YXJ0aHVyLnNhbmlvdGlzQGNpaGFudW5pdmVyc2l0eS5lZHUuaXE=