- 1Nuffield Department of Women's and Reproductive Health, Oxford Endometriosis CaRe Centre, Nuffield University of Oxford, Oxford, United Kingdom
- 2Division of Biomedical Sciences, Warwick Medical School, University of Warwick, Coventry, United Kingdom
Endometriosis is an inflammatory disease that is defined as the growth of endometrium-like tissue outside the uterus, commonly on the lining of the pelvic cavity, visceral organs and in the ovaries. It affects around 190 million women of reproductive age worldwide and is associated with chronic pelvic pain and infertility, which greatly impairs health-related life quality. The symptoms of the disease are variable, this combined with a lack of diagnostic biomarkers and necessity of surgical visualisation to confirm disease, the prognosis can take an average timespan of 6–8 years. Accurate non-invasive diagnostic tests and the identification of effective therapeutic targets are essential for disease management. To achieve this, one of the priorities is to define the underlying pathophysiological mechanisms that contribute to endometriosis. Recently, immune dysregulation in the peritoneal cavity has been linked to endometriosis progression. Macrophages account for over 50% of immune cells in the peritoneal fluid and are critical for lesion growth, angiogenesis, innervation and immune regulation. Apart from the secretion of soluble factors like cytokines and chemokines, macrophages can communicate with other cells and prime disease microenvironments, such as the tumour microenvironment, via the secretion of small extracellular vesicles (sEVs). The sEV-mediated intracellular communication pathways between macrophages and other cells within the peritoneal microenvironment in endometriosis remain unclear. Here, we give an overview of peritoneal macrophage (pMΦ) phenotypes in endometriosis and discuss the role of sEVs in the intracellular communication within disease microenvironments and the impact they may have on endometriosis progression.
Introduction
Endometriosis is a chronic inflammatory disease that affects approximately 10% of women of reproductive age worldwide (1). It is characterized as the ectopic growth of endometrium-like tissue, most commonly along the mesothelial cell layer lining the peritoneal cavity, but also in the form of ovarian endometriosis cysts (endometrioma) or below the peritoneal surface as deep nodules (2). Clinical symptoms include cyclical and non-cyclical pelvic pain, dysmenorrhea, and pain during and after sexual intercourse, defecation and emptying the bladder (2). Around 30% to 50% of patients with endometriosis present with subfertility (3).
Depending on the location, depth and size of lesions and adhesions, endometriosis can be divided into stages I-IV using the rASRM (revised American Society for Reproductive Medicine) classification system (4); deeply infiltrating endometriosis can be further classified following the ENZIAN criteria (5) and pregnancy outcomes can be predicted using the Endometriosis Fertility Index (EFI) (6). With the improvement of medical technologies, imaging tools (MRI and ultrasound) have shown reasonable specificity and sensitivity to aid diagnosis of endometrioma and deep endometriosis (7). The definitive diagnosis of endometriosis, especially peritoneal endometriosis, still requires laparoscopy (1).
As an oestrogen-driven chronic inflammatory disease, endometriosis primarily affects women during reproductive age. Clinically, it often manifests itself as early as adolescence (1). Dependent on geographical locations and accessibility of health care, there exists a delay of 6–8 years between the onset of symptoms and diagnosis (8). Shortening this gap requires increased awareness both in the general population and in the medical community, improvement of positive and negative predicative value of current imaging modalities particularly for peritoneal endometriosis and development of clinically reliable biomarkers. Furthermore, both medical and surgical approaches are associated with high recurrence rates and significant side effects (9, 10). For many patients, therefore, the disease generates long-term impairment to their quality of life, and consequently it is a substantial burden to healthcare systems and within society (1).
Retrograde menstruation is the most widely accepted theory implicated in the aetiology of endometriosis (11). This theory proposes that endometriosis lesions develop from endometrial cells and tissue flowing backward from the uterine cavity during menses, via the Fallopian tubes, into the peritoneal cavity (11). However, other mechanisms involved in the regulation of cell adhesion and proliferation must exist, as this retrograde menstruation occurs in as many as 90% of females (12). Endometriosis lesion architecture is variable but is usually composed of endometrial stromal and epithelial cells, with immune cell infiltration, fibrogenesis, neovascularisation, and innervation (2, 13). Endometrial stromal cells are the most predominant cell population in ectopic lesions and are thought to be mostly responsible for lesion attachment to the peritoneum (14).
Immune dysfunction in the peritoneal microenvironment of endometriosis
Endometriotic lesions and the mesothelial cell layer are exposed to immune cells in the peritoneal fluid (PF). Mass cytometry (15) and single-cell RNA sequencing analysis (16) has revealed distinct immune cell profiles of PF between endometriosis patients and controls. Cellular profiling studies identified over 40 types of immune cells in the PF, including monocytes and macrophages (the most abundant cell population), natural killer (NK) cells and neutrophils from the innate immune system, as well as T and B cells from the adaptive immune system (15). Recent evidence has suggested that peritoneal immune dysregulation facilitates the growth of endometriotic lesions (17). For example, decreased NK cell cytotoxicity was observed in PF of women with endometriosis compared to control women (17). The T helper (CD4+) immune pattern in PF of endometriosis patients is shifted toward a Th2 anti-inflammatory immune response favouring lesion growth (18, 19). Recently regulatory T (Treg) cells have also been implicated in disease development, through interactions with endometrial stromal cells and macrophages (20–22).
The role of peritoneal macrophages in endometriosis
Macrophages are the most abundant immune cell population in PF, accounting for almost 50% (15). In addition to tissue-resident macrophages, monocyte-derived macrophages are recruited to the peritoneal cavity when local inflammation occurs (23). Elevated numbers of macrophage are found in PF of endometriosis patients (15). These peritoneal macrophages (pMΦ) are recruited and get activated under the influence of macrophage growth factors and chemokines, such as colony-stimulating factor-1 (CSF-1) and monocyte chemoattractant protein-1 (MCP-1/CCL2) (24, 25). Mesothelium, endometriotic stromal cells and nerve fibres participate in the chemotactic recruitment of macrophages to the pelvic cavity in an oestrogen-dependent manner (26, 27). Oestrogen acts on pMΦ and endometriotic lesions via the oestrogen receptors alpha (ERα) and beta (ERβ) (28, 29).
The activated pMΦ exhibit both pro-inflammatory and pro-repair phenotypes (15). They produce numerous cytokines and growth factors in the peritoneal microenvironment, such as interleukin-1 beta (IL-1β), interleukin-6 (IL-6), interleukin-8 (IL-8), interleukin-12 (IL-12), tumour necrosis factor-alpha (TNF-α), vascular epithelial growth factor (VEGF) and transforming growth factor-beta 1 (TGF-β1) to induce endometriosis lesion implantation, growth and angiogenesis (2, 17, 30). Notably, Treg cells can promote macrophage polarization with pro-repair phenotypes via the secretion of soluble fibrinogen-like protein 2 (22). In addition, pMΦ from endometriosis patients have impaired phagocytotic abilities caused by the downregulation of CD36 (31, 32). These may contribute to the survival and attachment of refluxed endometrial cells and tissue. Furthermore, increased pMΦ abundance is correlated with pelvic pain scores in endometriosis patients (33), but the severity of pain symptoms does not correlate with rASRM stages, suggesting complex mechanisms (34). Inflammatory responses in endometriosis modulate pain by activating and sensitising peripheral nerve fibres, and long-term peripheral nociceptive input leads to central sensitisation (35, 36). Macrophages are attracted to nerve fibres under the influence of CSF-1 and CCL-2 (27, 37), and the recruited macrophages secrete nerve growth factors such as insulin growth factor 1, and VEGF, promote neurogenesis and nerve sensitization mediated by oestrogen (29, 38).
These studies show that pMΦ are associated with endometriosis progression through intercellular communication with other cells in peritoneal microenvironments (Figure 1). Intercellular crosstalk is not only limited to soluble factors; small extracellular vesicles (sEVs) also mediate cell communication. sEVs, previously referred to as exosomes, are nanosized lipid-bilayer vesicles (30 nm to 150 nm) released by cells (39), which are present in almost all biological fluids, including PF (40). They are formed by the inward budding of multivesicular endosomes (MVEs) and secreted after the fusing of MVEs with the cell surface (39). sEVs are enriched in specific cargoes (proteins, lipids, nucleic acids and metabolites), reflecting their cell of origin, and they deliver these to recipient cells to modulate their activities (41). Noticeably, sEVs are elevated in several diseases where they display altered phenotypes (39). Studies characterising the role of sEVs have advanced our knowledge of the pathology of various diseases, including cardiovascular diseases (42), neurological diseases (43), autoimmune disorders (44) and cancer (45). It has become clear that sEVs are important mediators of intracellular communication in disease microenvironments and they have emerged as valuable biomarkers and potential therapeutic targets (46, 47).
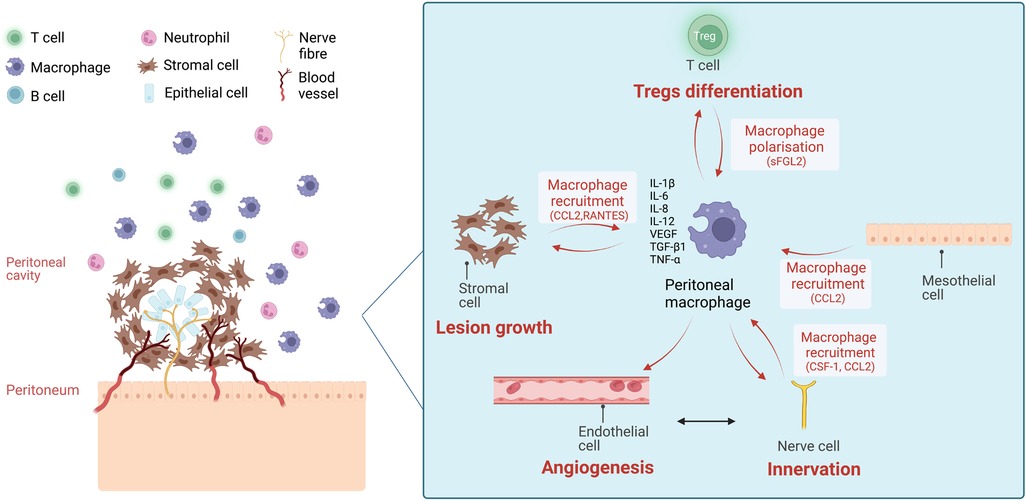
Figure 1. The chemokine-mediated intercellular communication of macrophages and other cells in the peritoneal microenvironment of endometriosis. Ectopic endometriotic lesions in the peritoneal cavity are composed of stromal cells and epithelial cells and infiltrated with blood vessels and nerve fibres. In the surrounding peritoneal fluid (PF), immune cells are present, including macrophages, neutrophils, T cells and B cells. The number of total peritoneal macrophages and CD206+/CD163+ macrophages are increased in PF of endometriosis patients. Stromal cells from the endometriotic lesions, mesothelial cells from peritoneum, nerve fibres attract macrophages to peritoneal cavity via the secretion of attractant factors like monocyte chemoattractant protein-1 (MCP-1/CCL2), colony-stimulating factor-1 (CSF-1), and RANTES (CCL5). Existing peritoneal macrophages also secrete MCP-1 to attract more macrophages. Treg cells induce macrophage polarisation into pro-repair subtypes via the secretion of soluble fibrinogen-like protein 2 (sFGL2), favouring lesion growth. On the other hand, peritoneal macrophages facilitate lesion growth, angiogenesis, neurogenesis and Treg differentiation via the secretion of cytokines and growth factors including interleukin-1β (IL-1β), interleukin-6 (IL-6), interleukin-8 (IL-8), interleukin-12 (IL-12), tumour necrosis factor alpha (TNF-α), vascular epithelial growth factor (VEGF) and transforming growth factor beta 1 (TGF-β1). (Created with biorender.com).
In this review, we will discuss the phenotypes of pMΦ and sEV-mediated intracellular crosstalk in several different disease microenvironments, and consider how these may contribute to endometriosis development.
Peritoneal macrophage phenotypes in endometriosis
pMΦ comprise a heterogenous population of immune cells originating from various locations (23). They are highly plastic cells that can differentiate into specific subtypes in response to local stimuli. Different subtypes of tissue-resident macrophages present various receptors, secrete distinct chemokines and cytokines, and consequently play specific functional roles (48). Flow cytometry studies have reported the heterogeneity within human pMΦ populations and various subsets are identified by assessing the expression of canonical markers, such as CD14, CD16 and HLA-DR (49) and distinguished by expression of Complement Receptor of the Immunoglobulin subfamily (CRIg) and CCR2 (50). In endometriosis patients, one study identified two subpopulations of pMΦ as HLA-DR+ CD14lo and HLA-DR+ CD14hi (33) and another study revealed two populations of pMΦ based on the expression of CD14 and CD68 (51). Recently, single cell RNA-Seq analysis identified seven distinct subtypes of macrophages in PF from an endometriosis patient (16) and reported five subpopulations of tissue resident and blood infiltrated macrophages in ectopic lesions (52). Validation of the characterisation and functional studies of these subtypes are now required to determine if the newly identified pMΦ subtypes have distinct roles in endometriosis progression.
In mouse models of experimental endometriosis, the origins and phenotypes of pMΦ have been thoroughly investigated. Tissue-resident macrophages are seeded from the embryo yolk sac and foetal liver, and monocyte-derived macrophages are seeded from the bone marrow during adulthood (23). In mice, pMΦ are characterised into large peritoneal macrophages (LpM; F4/80hi MHC IIlo) and monocyte-derived small peritoneal macrophages (SpM; F4/80lo MHC IIhi) (23). LpM are dominant in the peritoneal cavity and consist primarily of self-renewing embryonic-derived cells (53). They perform immunosurveillance in the peritoneal cavity (54). Under inflammatory conditions like endometriosis, monocytes infiltrate into pelvic cavity and transform into pro-inflammatory SpM, which eventually differentiate into LpM (55). LpM, therefore, consists of embryonic-derived and monocyte-derived cells (55). Notably, the tissue-resident, embryonic-derived LpM promote lesion growth, while monocyte-derived LpM appear to limit the growth of lesions in an endometriosis mouse model (56). These findings on the origins of pMΦ and SpM/LpM functions in the mouse model, cannot be directly translated to the human macrophage system, where pMΦ display a higher level of complexity and heterogeneity. Further research is critically required on the origins, phenotypes and functions of human pMΦ in homeostasis and disease states.
Small extracellular vesicle-mediated crosstalk in tissue-specific microenvironments
The uptake and functions of sEVs
sEVs are important cellular communicators in both physiological and pathological processes, through transferring functional proteins, lipids, and nucleic acids to recipient cells (39). While the cargo in sEVs generally reflects that of the parent cells, the RNA in sEVs tends to consist of small noncoding RNAs, like micro RNAs (miRNAs) and RNA fragments (57, 58). Altered miRNA expression profiles are observed in endometriosis patients and as miRNAs also function as epigenetic machinary, sEVs could contribute to the process (59). The uptake of sEVs can be local to the site of release or distant as they circulate in biological fluids (60). Some sEVs can be exclusively taken up by certain cell types, for example in the case of sEV mediated organ-specific metastasis in cancer (61). Cellular uptake is mediated by the surface composition of the vesicles (62). Once sEVs reach the recipient cells, they can either trigger signalling by directly interacting with surface receptors, fusing with the plasma membrane, or be internalised (60). For the functional use of sEV-encapsulated miRNAs and RNAs, sEVs need to get internalised, bypass degradation and release cargos targeted to endoplasmic reticulum for translation (63).
sEV-mediated crosstalk in healthy and diseased microenvironments
Endometriotic lesions share some clinical similarities with cancer. For instance, both exhibit a metastatic phenotype with adhesion, invasion and neuroangiogenesis, although the lack of driver mutations limit the malignant potential of most forms of endometriosis (2). There are a wealth of studies showing that tumour-associated macrophage (TAM) derived-sEVs (TAM-sEVs) interact with each other and other cells to promote tumour progression. TAMs are one of the most studied disease-associated macrophage populations. TAM-sEVs have been found to regulate tumourigenesis (64), metastasis (65, 66) and drug resistance (67), by transferring miRNAs and proteins to other cells in various tumour microenvironments. The regulations conferred are likely specific and dynamic to the cancer type. For example, tumour-derived sEV miR-934 induces macrophage polarisation into anti-inflammatory subtypes, promoting liver metastasis of colorectal cancer (68). Notably, the sEV-mediated regulation of TAMs is not limited to inducing anti-inflammatory polarisation. In oral squamous cell carcinoma, tumour-derived sEVs can activate pro-inflammatory TAMs to promote tumour migration (69). In addition, melanoma cell-derived sEVs can transform lipopolysaccharide (LPS) and interferon-gamma (IFN-γ) stimulated macrophages to pro-inflammatory and pro-angiogenic TAMs, which present strong differences in gene expression compared to macrophages stimulated by interleukin-14 (IL-4) + interleukin-13 (IL-13) and LPS + IFN- γ, and higher survival rates (70). These studies highlight the heterogeneity of TAMs and their complex roles in tumour microenvironments.
Importantly, sEV-mediated communication between TAMs and other cells within the tumour microenvironment is bilateral. For example, in gastric cancer, TAMs promote the migration of gastric cancer cells by transfer of functional Apolipoprotein E via sEVs to activate the PI3K-Akt signalling pathway (65). TAM-sEVs do not only directly regulate cancer cells—in cases of pancreatic ductal adenocarcinoma TAM-sEVs carrying miR-155–5p and miR-211–5p promote angiogenesis and tumour growth by suppressing E2F2 expression in endothelial cells (71). In epithelial ovarian cancer TAM-sEVs induce Treg/T helper 17 cell imbalance, contributing to tumour progression and metastasis (72). In summary, in the tumour microenvironment, TAMs can promote tumorigenesis and metastasis by directly regulating tumour cells, or by indirectly targeting endothelial and immune cells, via sEVs.
It is important to remember that sEVs also influence eutopic endometrium function in both physiological and pathological processes. Protein cargos of sEVs derived from endometrial epithelial cells enhance the adhesive capacity of trophoblast, potentially contributing to embryo implantation (73). Intriguingly, in patients with adenomyosis (a condition where endometrial-like cells grow into the myometrium causing heavy menstrual bleeding, pain and infertility, often in association with endometriosis) (74), sEVs secreted by endometrial organoids contain miRNAs associated with pregnancy complications and adenomyosis progression (75).
The sEV-mediated crosstalk in the peritoneal microenvironment of endometriosis
These findings from cancer and endometrium studies raise the possibility that macrophage-derived and endometriosis tissue-specific sEVs could have an impact on endometriosis progression through concerted cell targeting in the lesion microenvironment (76–78). Indeed, similar to TAM, pMΦ are also regulated by sEV mediated signalling networks. In an endometriosis mouse model, sEVs derived from stromal cells induced macrophage polarisation into an anti-inflammatory subtype with decreased phagocytotic abilities, leading to increased lesion size (79). One recent study found that ectopic stromal cells collected from recurrent ovarian endometriosis patients induced anti-inflammatory polarisation of macrophages via the secretion of sEV-derived Legumain pseudogene 1 (EV-LGMNP1), a newly identified pseudogene of LGMN (80). LGMN is highly expressed in many cancers and appears to promote cancer progression (81, 82). Intriguingly in the following retrospective clinical cohort study (n = 73) a higher serum EV-LGMNP was detected in recurrent endometriosis patients (80).
pMΦ-sEVs, on the other hand, have been shown to transfer miR-22–3p to endometrial stromal cells, enhancing cell proliferation, migration, and invasion through the regulation of the SIRT1/NF-κB signalling pathway (83). Another study revealed that pMΦ-sEVs induce proliferation and migration of ectopic stromal cells in vitro and promote lesion growth in an endometriosis mouse model via the transfer of the long non-coding RNA (lncRNA) CHL1-AS1 (84). lncRNA CHL1-AS1 is the antisense of the CHL1 gene, which can suppress or promote cancer development at different stages (85). Overexpression of the CHL1 gene and lncRNA CHL1-AS1 has been found in the ectopic endometrium from ovarian endometriosis patients (86). Interestingly, sEVs from LPS-induced macrophages can reduce endometriosis lesion growth by repolarising anti-inflammatory macrophages into pro-inflammatory subtypes in mice (87). These macrophage-derived sEVs also repress stromal cell migration and angiogenesis in vitro (87). These above-mentioned studies suggest that macrophage-derived sEVs could target various cells and pathways in endometriosis. Accordingly, these sEVs may promote or supress endometriosis progression, determined by the phenotypes of the macrophages that they are derived from. The majority of the literature has focussed on pMΦ-sEVs and, to date, there exists a lack of data on the role of lesion-resident macrophage-derived sEVs.
An additional source of sEVs relevant to endometriosis are those from stromal cells. Apart from regulating pMΦ, sEVs derived from endometrial stromal cells are found to induce neuroangiogenesis (88). Additionally, sEVs from endometrial stromal cells from endometriosis patients exhibit differential profiles of miR-21 and lncRNA antisense hypoxia inducible factor (aHIF), promoting proangiogenic properties of endothelial cells (89, 90). lncRNA aHIF derived from endometrial stromal cells target VEGF, a strong pro-angiogenic molecule, which is highly expressed in endometriosis lesions and PF of endometriosis patients (90). Of note, sEV shuttled miR-21, has been linked to tumour progression through targeting of cancer cells, endothelial cells and immune cells (like macrophages) as an apoptosis suppressor (91). Interestingly, stromal cell sEV secreted miR-214 and miR-214–3p are found to supress fibrosis of endometriosis lesions in murine models (92, 93); miR-214–3p was significantly downregulated and its target, connective tissue growth factor, is upregulated in ectopic lesions from endometriosis patients (93). Together, the evidence suggests endometriosis-specific stromal derived sEVs may contribute to disease progression. Recently, miR-30c encapsulated in sEVs derived from endometriotic epithelial cells was found to supress epithelial cell invasion and migration and attenuate endometriosis progression in a mouse model (94).
These in vitro and in vivo studies indicate that the peritoneal microenvironment will likely contain a variety of sEVs, and that these sEVs may carry key factors instrumental in the pathogenesis of endometriosis. Combined with the known miRNA and lncRNA, a distinct sEV protein profile has been identified in a mass spectroscopy proteomic study of PF-derived sEVs from endometriosis patients, compared to controls (39). Five proteins, peroxiredoxin-1, histone H2A type-2-C, annexin A2, inter-α-trypsin inhibitor heavy chain H4 and tubulin alpha-chain were exclusively present in sEVs in the PF from women with endometriosis (39). One of the proteins, Annexin A2 has been found to be highly expressed in ectopic stromal cell-derived sEVs, and promotes angiogenesis and stromal cell proliferation and migration by activating ERK1/2 STAT3 pathways (95). Future work is required to build on these data and establish key pathways that could be targeted for therapies, or function as biomarkers for disease severity.
Future perspectives
Macrophages play a central role in endometriosis establishment and progression and exhibit a high level of heterogeneity. Recent studies have identified various human pMΦ subpopulations using different experimental approaches. pMΦ achieve bilateral interactions with a broad range of cells in endometriosis (Figure 1). Along with soluble factors, pMΦ will likely secrete sEVs with functional moieties, and the pMΦ themselves are likely affected by sEVs from the microenvironment, akin to tumour microenvironments (Figure 2). Recent studies have revealed the significance of stromal cell-derived sEVs in several aspects of endometriosis progression including angiogenesis, neuroangiogenesis and macrophage polarisation by transferring RNAs and proteins. The research on macrophage-derived sEVs, on the other hand, is limited to the regulation of pMΦ-sEVs on cell proliferation, migration and invasion of stromal cells. We propose a succession of pathways in which pMΦ-sEVs may be involved in endometriosis: when ectopic endometrial tissue and cells enter the abdominal cavity during menstruation, pMΦ-sEVs regulate other immune cells (e.g.,: T cells) impairing immune surveillance, facilitating lesion implantation to peritoneal surfaces. Once the lesions are attached, the endometrial lesions will further release sEVs to transform pMΦ into lesion-favouring subtypes. sEVs secreted from these macrophages will then communicate with other immune cells, endothelial and nerve cells to sustain immune evasion, promote lesion proliferation, and induce fibrosis, angiogenesis and neurogenesis (38). Future work to investigate sEVs derived from endometriosis associated-macrophages including lesion-resident macrophages are critically required.
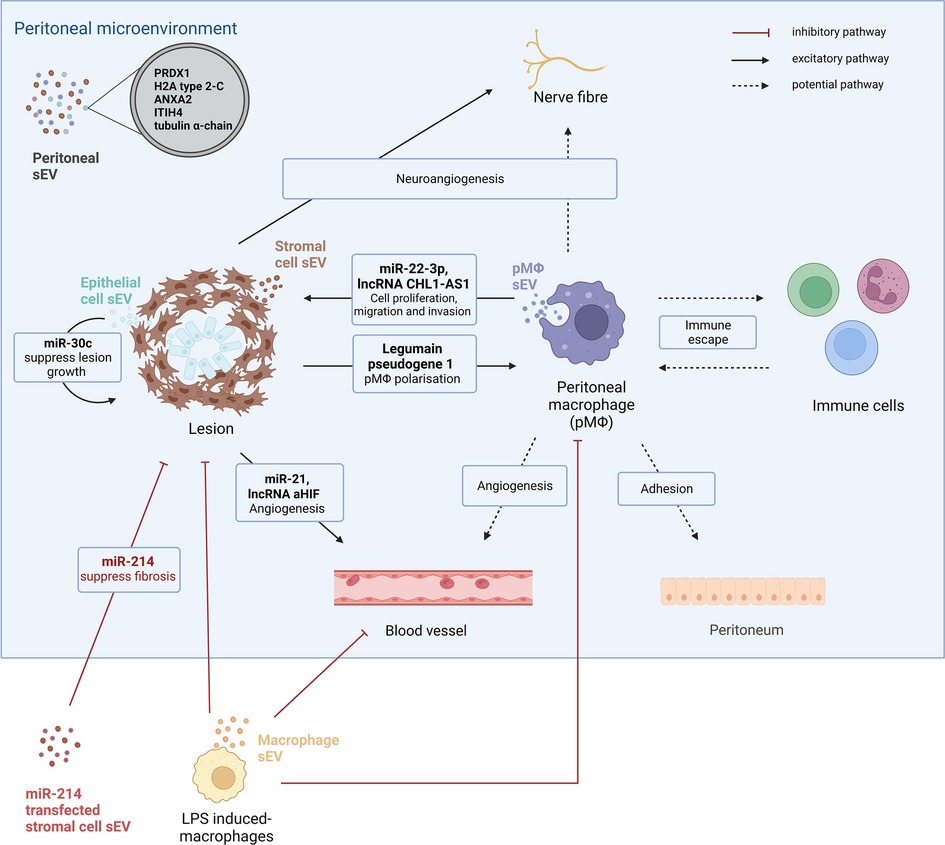
Figure 2. The small extracellular-mediated intracellular communication in endometriosis. Small extracellular vesicles (sEVs) are present in peritoneal fluid and distinct protein profiles are shown in peritoneal sEVs from endometriosis patients compared to controls. Five proteins, peroxiredoxin-1 (PRDX1), histone H2A (H2A) type-2-C, annexin A2 (ANXA2), inter-α-trypsin inhibitor heavy chain H4 (ITIH4) and tubulin alpha-chain are solely present in peritoneal sEVs from endometriosis patients. sEVs are important intracellular communicators between different types of cells in the peritoneal microenvironment of endometriosis. One study has revealed that stromal cell-derived sEVs regulate macrophage polarisation by delivering legumain pseudogene 1 and promote angiogenesis via miR-21 and lncRNA aHIF. Additionally, stromal cell-derived sEVs promote neuro-angiogenesis. Endometrial epithelial cell sEVs inhibit lesion growth by transferring miR-30c. Peritoneal macrophages also secrete sEVs. pMΦ -derived sEVs promote lesion growth by delivering miR-22-3p and lncRNA CHL1-AS1 to stromal cells. pMΦ could potentially regulate angiogenesis, neuroangiogenesis and immune escape in endometriosis progression via sEVs. As a future potential therapeutic approach, miR-24 transfected sEVs from stromal cells are found to supress fibrosis in an endometriosis mouse model. LPS induced-macrophage-derived sEVs could attenuate endometriosis progression by repolarising pMΦ, inhibiting angiogenesis and stromal cell proliferation.
One pivotal point when conducting sEVs characterisation studies in endometriosis is that many cellular and intracellular activities in the peritoneal microenvironment are oestrogen dependent (1, 29). Ectopic endometriotic lesions contain oestrogen receptors and enzymes such as P450−aromatase to convert androgens into potent 17β-oestradiol (27). Oestrogen receptors are overexpressed in pMΦ from endometriosis patients (96). In addition, oestrogen levels fluctuate across the menstrual cycle and are also affected by frequently used hormonal treatments, such as the combined contraceptive pill, progestogens or gonadotrophin-releasing hormone agonists and antagonists (2). Mismatches of the hormonal status may impair the reliability and reproducibility of the characterisations. Standardised protocols to collect clinical data and biological samples as well as their processing are the prerequisite for replicable studies and data validation. For endometriosis, these protocols exist and should be used (97–100).
Identification of non-invasive biomarkers is one of the ultimate goals of sEVs studies which requires research beyond the peritoneal microenvironment. Notably, sEV shuttled miR-22–3p, lncRNA aHIF which are identified as key intracellular communicators in the peritoneal microenvironment are significantly higher in the serum of endometriosis patients (90, 101). sEV packaged-miR-214–3p which were reported to have a protective role on endometriosis fibrosis are decreased in serum of women with endometriosis (93). The physiological and pathophysiological significance of these RNAs remains to be determined in endometriosis, but the finding raises hope that sEVs could be the source of a peripheral blood biomarker for diagnosis and assessing the efficacy of treatments of endometriosis. It is necessary to remember that the majority of sEVs studies in endometriosis are pilot studies, we need confirmation and validation of these results using independent and sufficiently powered studies before any conclusion can been drawn. Future work is also required to examine whether engineered-sEVs could become therapeutic modalities, to constrain lesion development and/or improve the quality of life for many women with endometriosis.
Author contributions
YW wrote the manuscript. RD, CMB, EG and JHS edited. All authors contributed to the article and approved the submitted version.
Conflict of interest
The authors declare that the research was conducted in the absence of any commercial or financial relationships that could be construed as a potential conflict of interest.
Publisher's note
All claims expressed in this article are solely those of the authors and do not necessarily represent those of their affiliated organizations, or those of the publisher, the editors and the reviewers. Any product that may be evaluated in this article, or claim that may be made by its manufacturer, is not guaranteed or endorsed by the publisher.
References
1. Zondervan KT, Becker CM, Koga K, Missmer SA, Taylor RN, Viganò P. Endometriosis. Nat Rev Dis Primers. (2018) 4(1):9. doi: 10.1038/s41572-018-0008-5
2. Zondervan KT, Becker CM, Missmer SA. Endometriosis. N Engl J Med. (2020) 382(13):1244–56. doi: 10.1056/NEJMra1810764
3. Prescott J, Farland LV, Tobias DK, Gaskins AJ, Spiegelman D, Chavarro JE, et al. A prospective cohort study of endometriosis and subsequent risk of infertility. Hum Reprod. (2016) 31(7):1475–82. doi: 10.1093/humrep/dew085
4. Canis M, Donnez JG, Guzick DS, Halme JK, Rock JA, Schenken RS, et al. Revised American society for reproductive medicine classification of endometriosis: 1996. Fertil & Steril. (1997) 67(5):817–21. doi: 10.1016/S0015-0282(97)81391-X
5. Tuttlies F, Keckstein J, Ulrich U, Possover M, Schweppe K, Wustlich M, et al. Enzian-Score, a classification of deep infiltrating endometriosis. Zentralbl Gynakol. (2005) 127(5):275–81. doi: 10.1055/s-2005-836904
6. Adamson GD, Pasta DJ. Endometriosis fertility Index: the new, validated endometriosis staging system. Fertility & Sterility. (2010) 94(5):1609–15. doi: 10.1016/j.fertnstert.2009.09.035
7. Nisenblat V, Bossuyt PM, Farquhar C, Johnson N, Hull ML. Imaging modalities for the non-invasive diagnosis of endometriosis. Cochrane Database Syst Rev. (2016) 2(2):CD009591. doi: 10.1002/14651858.CD009591.pub2
8. Nnoaham KE, Hummelshoj L, Webster P, d'Hooghe T, de Cicco Nardone F, de Cicco Nardone C, et al. Impact of endometriosis on quality of life and work productivity: a multicenter study across ten countries. Fertil & Steril. (2011) 96(2):366–73.e8. doi: 10.1016/j.fertnstert.2011.05.090
9. Becker CM, Gattrell WT, Gude K, Singh SS. Reevaluating response and failure of medical treatment of endometriosis: a systematic review. Fertil & Steril. (2017) 108(1):125–36. doi: 10.1016/j.fertnstert.2017.05.004
10. Agarwal SK, Chapron C, Giudice LC, Laufer MR, Leyland N, Missmer SA, et al. Clinical diagnosis of endometriosis: a call to action. Am J Obstet Gynecol. (2019) 220(4):354.e1–.e12. doi: 10.1016/j.ajog.2018.12.039
11. Sampson J. Peritoneal endometriosis due to menstrual dissemination of endometrial tissue into the peritoneal cavity. Am J Obstet Gynecol. (1927) 14:422–69. doi: 10.1016/S0002-9378(15)30003-X
12. Halme J, Hammond MG, Hulka JF, Raj SG, Talbert LM. Retrograde menstruation in healthy women and in patients with endometriosis. Obstet Gynecol. (1984) 64(2):151–4. 6234483.6234483
13. Vigano P, Candiani M, Monno A, Giacomini E, Vercellini P, Somigliana E. Time to redefine endometriosis including its pro-fibrotic nature. Hum Reprod. (2017) 33(3):347–52. doi: 10.1093/humrep/dex354
14. Maybin JA, Critchley HOD. Menstrual physiology: implications for endometrial pathology and beyond. Hum Reprod Update. (2015) 21(6):748–61. doi: 10.1093/humupd/dmv038
15. Guo M, Bafligil C, Tapmeier T, Hubbard C, Manek S, Shang C, et al. Mass cytometry analysis reveals a distinct immune environment in peritoneal fluid in endometriosis: a characterisation study. BMC Med. (2020) 18(1):3. doi: 10.1186/s12916-019-1470-y
16. Zou G, Wang J, Xu X, Xu P, Zhu L, Yu Q, et al. Cell subtypes and immune dysfunction in peritoneal fluid of endometriosis revealed by single-cell rna-sequencing. Cell Biosci. (2021) 11(1):1–17. doi: 10.1186/s13578-020-00515-y
17. Symons LK, Miller JE, Kay VR, Marks RM, Liblik K, Koti M, et al. The immunopathophysiology of endometriosis. Trends Mol Med. (2018) 24(9):748–62. doi: 10.1016/j.molmed.2018.07.004
18. Podgaec S, Abrao MS, Dias JA Jr, Rizzo LV, de Oliveira RM, Baracat EC. Endometriosis: an inflammatory disease with a Th2 immune response component. Hum Reprod. (2007) 22(5):1373–9. doi: 10.1093/humrep/del516
19. Takamura M, Koga K, Izumi G, Hirata T, Harada M, Hirota Y, et al. Simultaneous detection and evaluation of four subsets of Cd4+ T lymphocyte in lesions and peripheral blood in endometriosis. Am J Reprod Immunol. (2015) 74(6):480–6. doi: 10.1111/aji.12426
20. Wang X-Q, Zhou W-J, Luo X-Z, Tao Y, Li D-J. Synergistic effect of regulatory T cells and proinflammatory cytokines in angiogenesis in the endometriotic milieu. Hum Reprod. (2017) 32(6):1304–17. doi: 10.1093/humrep/dex067
21. Li M-Q, Wang Y, Chang K-K, Meng Y-H, Liu L, Mei J, et al. Cd4+ Foxp3+ regulatory T cell differentiation mediated by endometrial stromal cell-derived teck promotes the growth and invasion of endometriotic lesions. Cell Death Dis. (2014) 5(10):e1436-e. doi: 10.1038/cddis.2014.414
22. Hou X-X, Wang X-Q, Zhou W-J, Li D-J. Regulatory T cells induce polarization of pro-repair macrophages by secreting Sfgl2 into the endometriotic milieu. Commun Biol. (2021) 4(1):499. doi: 10.1038/s42003-021-02018-z
23. Hogg C, Horne AW, Greaves E. Endometriosis-Associated macrophages: origin, phenotype, and function. Front Endocrinol (Lausanne). (2020) 11:7. doi: 10.3389/fendo.2020.00007
24. Budrys NM, Nair HB, Liu Y-G, Kirma NB, Binkley PA, Kumar S, et al. Increased expression of macrophage colony–stimulating factor and its receptor in patients with endometriosis. Fertil & Steril. (2012) 97(5):1129–35.e1. doi: 10.1016/j.fertnstert.2012.02.007
25. Ueno T, Toi M, Saji H, Muta M, Bando H, Kuroi K, et al. Significance of macrophage chemoattractant protein-1 in macrophage recruitment, angiogenesis, and survival in human breast cancer. Clin Cancer Res. (2000) 6(8):3282–9. 10955814.10955814
26. Gou Y, Li X, Li P, Zhang H, Xu T, Wang H, et al. Estrogen receptor Β upregulates Ccl2 via nf-Κb signaling in endometriotic stromal cells and recruits macrophages to promote the pathogenesis of endometriosis. Hum Reprod. (2019) 34(4):646–58. doi: 10.1093/humrep/dez019
27. Greaves E, Temp J, Esnal-Zufiurre A, Mechsner S, Horne AW, Saunders PT. Estradiol is a critical mediator of macrophage-nerve cross talk in peritoneal endometriosis. Am J Pathol. (2015) 185(8):2286–97. doi: 10.1016/j.ajpath.2015.04.012
28. Kitawaki J, Kado N, Ishihara H, Koshiba H, Kitaoka Y, Honjo H. Endometriosis: the pathophysiology as an estrogen-dependent disease. J Steroid Biochem Bol Biol. (2002) 83(1-5):149–55. doi: 10.1016/S0960-0760(02)00260-1
29. Liang Y, Xie H, Wu J, Liu D, Yao S. Villainous role of estrogen in macrophage-nerve interaction in endometriosis. Reprod Biol Endocrinol. (2018) 16(1):122. doi: 10.1186/s12958-018-0441-z
30. Harada T, Iwabe T, Terakawa N. Role of cytokines in endometriosis. Fertil & Steril. (2001) 76(1):1–10. doi: 10.1016/S0015-0282(01)01816-7
31. Chuang PC, Wu MH, Shoji Y, Tsai SJ, Ireland. Downregulation of Cd36 results in reduced phagocytic ability of peritoneal macrophages of women with endometriosis. J Pathol. (2009) 219(2):232–41. doi: 10.1002/path.2588
32. Chuang P-C, Lin Y-J, Wu M-H, Wing L-YC, Shoji Y, Tsai S-J. Inhibition of Cd36-dependent phagocytosis by prostaglandin E2 contributes to the development of endometriosis. Am J Pathol. (2010) 176(2):850–60. doi: 10.2353/ajpath.2010.090551
33. Gibson DA, Collins F, De Leo B, Horne AW, Saunders PT. Pelvic pain correlates with peritoneal macrophage abundance not endometriosis. Reprod & Fertil. (2021) 2(1):47–57. doi: 10.1530/RAF-20-0072
34. Johnson NP, Hummelshoj L, Adamson GD, Keckstein J, Taylor HS, Abrao MS, et al. World endometriosis society consensus on the classification of endometriosis. Hum Reprod. (2017) 32(2):315–24. doi: 10.1093/humrep/dew293
35. Morotti M, Vincent K, Becker CM. Mechanisms of pain in endometriosis. Eur J Obstet Gynecol Reprod Biol. (2017) 209:8–13. doi: 10.1016/j.ejogrb.2016.07.497
36. Bajaj P, Bajaj P, Madsen H, Arendt-Nielsen L. Endometriosis is associated with central sensitization: a psychophysical controlled study. J Pain. (2003) 4(7):372–80. doi: 10.1016/S1526-5900(03)00720-X
37. Tran LVP, Tokushige N, Berbic M, Markham R, Fraser IS. Macrophages and nerve fibres in peritoneal endometriosis. Hum Reprod. (2009) 24(4):835–41. doi: 10.1093/humrep/den483
38. Forster R, Sarginson A, Velichkova A, Hogg C, Dorning A, Horne AW, et al. Macrophage-Derived insulin-like growth factor-1 is a key neurotrophic and nerve-sensitizing factor in pain associated with endometriosis. FASEB J. (2019) 33(10):11210–22. doi: 10.1096/fj.201900797R
39. Van Niel G, d'Angelo G, Raposo G. Shedding light on the cell biology of extracellular vesicles. Nat Rev Mol Cell Biol. (2018) 19(4):213–28. doi: 10.1038/nrm.2017.125
40. Nazri HM, Imran M, Fischer R, Heilig R, Manek S, Dragovic RA, et al. Characterization of exosomes in peritoneal fluid of endometriosis patients. Fertil Steril. (2020) 113(2):364–73.e2. doi: 10.1016/j.fertnstert.2019.09.032
41. Minciacchi VR, Freeman MR, Di Vizio D. Extracellular vesicles in cancer: exosomes, microvesicles and the emerging role of large oncosomes. Semin Cell Dev Biol. (2015) 40:41–51.
42. Fu S, Zhang Y, Li Y, Luo L, Zhao Y, Yao Y. Extracellular vesicles in cardiovascular diseases. Cell Death Discov. (2020) 6(1):1–9. doi: 10.1038/s41420-020-00305-y
43. Iranifar E, Seresht BM, Momeni F, Fadaei E, Mehr MH, Ebrahimi Z, et al. Exosomes and micrornas: new potential therapeutic candidates in Alzheimer disease therapy. J Cell Physiol. (2019) 234(3):2296–305. doi: 10.1002/jcp.27214
44. Tan L, Wu H, Liu Y, Zhao M, Li D, Lu Q. Recent advances of exosomes in immune modulation and autoimmune diseases. Autoimmunity. (2016) 49(6):357–65. doi: 10.1080/08916934.2016.1191477
45. Kalluri R. The biology and function of exosomes in cancer. J Clin Invest. (2016) 126(4):1208–15. doi: 10.1172/JCI81135
46. Raposo G, Stahl PD. Extracellular vesicles: a new communication paradigm? Nat Rev Mol Cell Biol. (2019) 20(9):509–10. doi: 10.1038/s41580-019-0158-7
47. Möller A, Lobb R. The evolving translational potential of small extracellular vesicles in cancer. Nat Rev Cancer. (2020) 20(12):697–709. doi: 10.1038/s41568-020-00299-w
48. Wynn TA, Chawla A, Pollard J. Macrophage biology in development, homeostasis and disease. Nature. (2013) 496(7446):445–55. doi: 10.1038/nature12034
49. Ruiz-Alcaraz AJ, Carmona-Martínez V, Tristán-Manzano M, Machado-Linde F, Sánchez-Ferrer ML, García-Peñarrubia P, et al. Characterization of human peritoneal monocyte/macrophage subsets in homeostasis: phenotype, Gata6, phagocytic/oxidative activities and cytokines expression. Sci Rep. (2018) 8(1):1–14. doi: 10.1038/s41598-018-30787-x
50. Irvine KM, Banh X, Gadd VL, Wojcik KK, Ariffin JK, Jose S, et al. Crig-Expressing peritoneal macrophages are associated with disease severity in patients with cirrhosis and ascites. JCI Insight. (2016) 1(8):e86914. doi: 10.1172/jci.insight.86914
51. Hudson QJ, Ashjaei K, Perricos A, Kuessel L, Husslein H, Wenzl R, et al. Endometriosis patients show an increased M2 response in the peritoneal Cd14 + low/Cd68 + low macrophage subpopulation coupled with an increase in the T-helper 2 and T-regulatory cells. Reprod Sci. (2020) 27(10):1920–31. doi: 10.1007/s43032-020-00211-9
52. Tan Y, Flynn WF, Sivajothi S, Luo D, Bozal SB, Davé M, et al. Single-Cell analysis of endometriosis reveals a coordinated transcriptional programme driving immunotolerance and angiogenesis across eutopic and ectopic tissues. Nat Cell Biol. (2022) 24(8):1306–18. doi: 10.1038/s41556-022-00961-5
53. Gomez Perdiguero E, Klapproth K, Schulz C, Busch K, Azzoni E, Crozet L, et al. Tissue-Resident macrophages originate from yolk-sac-derived erythro-myeloid progenitors. Nature. (2015) 518(7540):547–51. doi: 10.1038/nature13989
54. Ghosn EEB, Cassado AA, Govoni GR, Fukuhara T, Yang Y, Monack DM, et al. Two physically, functionally, and developmentally distinct peritoneal macrophage subsets. Proc Natl Acad Sci USA. (2010) 107(6):2568–73. doi: 10.1073/pnas.0915000107
55. Bain CC, Hawley CA, Garner H, Scott CL, Schridde A, Steers NJ, et al. Long-Lived self-renewing bone marrow-derived macrophages displace embryo-derived cells to inhabit adult serous cavities. Nat Commun. (2016) 7(1):1–14. doi: 10.1038/ncomms11852
56. Hogg C, Panir K, Dhami P, Rosser M, Mack M, Soong D, et al. Macrophages inhibit and enhance endometriosis depending on their origin. Proc Natl Acad Sci USA. (2021) 118(6):e2013776118. doi: 10.1073/pnas.2013776118
57. Taylor DD, Gercel-Taylor C. Microrna signatures of tumor-derived exosomes as diagnostic biomarkers of ovarian cancer. Gynecol Oncol. (2008) 110(1):13–21. doi: 10.1016/j.ygyno.2008.04.033
58. Huang X, Yuan T, Tschannen M, Sun Z, Jacob H, Du M, et al. Characterization of human plasma-derived exosomal rnas by deep sequencing. BMC genomics. (2013) 14:319. doi: 10.1186/1471-2164-14-319
59. Braza-Boïls A, Marí-Alexandre J, Gilabert J, Sanchez-Izquierdo D, Espana F, Estelles A, et al. Microrna expression profile in endometriosis: its relation to angiogenesis and fibrinolytic factors. Hum Reprod. (2014) 29(5):978–88. doi: 10.1093/humrep/deu019
60. Gurung S, Perocheau D, Touramanidou L, Baruteau J. The exosome journey: from biogenesis to uptake and intracellular signalling. Cell Commun Signal. (2021) 19(1):47. doi: 10.1186/s12964-021-00730-1
61. Hoshino A, Costa-Silva B, Shen T-L, Rodrigues G, Hashimoto A, Tesic Mark M, et al. Tumour exosome integrins determine organotropic metastasis. Nature. (2015) 527(7578):329–35. doi: 10.1038/nature15756
62. Alvarez-Erviti L, Seow Y, Yin H, Betts C, Lakhal S, Wood MJA. Delivery of sirna to the mouse brain by systemic injection of targeted exosomes. Nat Biotechnol. (2011) 29(4):341–5. doi: 10.1038/nbt.1807
63. Heusermann W, Hean J, Trojer D, Steib E, Von Bueren S, Graff-Meyer A, et al. Exosomes surf on filopodia to enter cells at endocytic hot spots, traffic within endosomes, and are targeted to the er. J Cell Biol. (2016) 213(2):173–84. doi: 10.1083/jcb.201506084
64. Guan H, Peng R, Fang F, Mao L, Chen Z, Yang S, et al. Tumor-Associated macrophages promote prostate cancer progression via exosome-mediated mir-95 transfer. J Cell Physiol. (2020) 235(12):9729–42. doi: 10.1002/jcp.29784
65. Li X, Chen Z, Ni Y, Bian C, Huang J, Chen L, et al. Tumor-Associated macrophages secret exosomal mir-155 and mir-196a-5p to promote metastasis of non-small-cell lung cancer. Transl Lung Cancer Res. (2021) 10(3):1338. doi: 10.21037/tlcr-20-1255
66. Zheng P, Luo Q, Wang W, Li J, Wang T, Wang P, et al. Tumor-Associated macrophages-derived exosomes promote the migration of gastric cancer cells by transfer of functional apolipoprotein E. Cell Death Dis. (2018) 9(4):1–14. doi: 10.1038/s41419-018-0465-5
67. Zheng P, Chen L, Yuan X, Luo Q, Liu Y, Xie G, et al. Exosomal transfer of tumor-associated macrophage-derived mir-21 confers cisplatin resistance in gastric cancer cells. J Exp Clin Cancer Res. (2017) 36(1):1–13. doi: 10.1186/s13046-016-0473-1
68. Zhao S, Mi Y, Guan B, Zheng B, Wei P, Gu Y, et al. Tumor-Derived exosomal mir-934 induces macrophage M2 polarization to promote liver metastasis of colorectal cancer. J Hematol Oncol. (2020) 13(1):1–19. doi: 10.1186/s13045-019-0838-y
69. Xiao M, Zhang J, Chen W, Chen W. M1-Like tumor-associated macrophages activated by exosome-transferred Thbs1 promote malignant migration in oral squamous cell carcinoma. J Exp Clin Cancer Res. (2018) 37(1):143. doi: 10.1186/s13046-018-0815-2
70. Gerloff D, Lützkendorf J, Moritz RKC, Wersig T, Mäder K, Müller LP, et al. Melanoma-Derived exosomal mir-125b-5p educates tumor associated macrophages (tams) by targeting lysosomal acid lipase a (lipa). Cancers (Basel). (2020) 12(2):464. doi: 10.3390/cancers12020464
71. Yang Y, Guo Z, Chen W, Wang X, Cao M, Han X, et al. M2 macrophage-derived exosomes promote angiogenesis and growth of pancreatic ductal adenocarcinoma by targeting E2f2. Mol Ther. (2021) 29(3):1226–38. doi: 10.1016/j.ymthe.2020.11.024
72. Zhou J, Li X, Wu X, Zhang T, Zhu Q, Wang X, et al. Exosomes released from tumor-associated macrophages transfer mirnas that induce a treg/Th17 cell imbalance in epithelial ovarian cancer. Cancer Immunol Res. (2018) 6(12):1578–92. doi: 10.1158/2326-6066.CIR-17-0479
73. Greening DW, Nguyen HPT, Elgass K, Simpson RJ, Salamonsen LA. Human endometrial exosomes contain hormone-specific cargo modulating trophoblast adhesive capacity: insights into endometrial-embryo Interactions1. Biol Reprod. (2016) 94(2):38, 1–15. doi: 10.1095/biolreprod.115.134890
74. Benagiano G, Brosens I, Habiba M. Structural and molecular features of the endomyometrium in endometriosis and adenomyosis. Hum Reprod Update. (2013) 20(3):386–402. doi: 10.1093/humupd/dmt052
75. Juárez-Barber E, Segura-Benítez M, Carbajo-García MC, Bas-Rivas A, Faus A, Vidal C, et al. Extracellular vesicles secreted by adenomyosis endometrial organoids contain mirnas involved in embryo implantation and pregnancy. Reprod Biomed Online. (2023) 46(3):470–81. doi: 10.1016/j.rbmo.2022.12.008
76. Schjenken JE, Panir K, Robertson SA, Hull ML. Exosome-Mediated intracellular signalling impacts the development of endometriosis—new avenues for endometriosis research. Mol Hum Reprod. (2018) 25(1):2–4. doi: 10.1093/molehr/gay050
77. Freger S, Leonardi M, Foster WG. Exosomes and their cargo are important regulators of cell function in endometriosis. Reprod Biomed Online. (2021) 43(3):370–8. doi: 10.1016/j.rbmo.2021.05.022
78. Esfandyari S, Elkafas H, Chugh RM, Park H-S, Navarro A, Al-Hendy A. Exosomes as biomarkers for female reproductive diseases diagnosis and therapy. Int J Mol Sci. (2021) 22(4):2165. doi: 10.3390/ijms22042165
79. Sun H, Li D, Yuan M, Li Q, Zhen Q, Li N, et al. Macrophages alternatively activated by endometriosis-exosomes contribute to the development of lesions in mice. Mol Hum Reprod. (2018) 25(1):5–16. doi: 10.1093/molehr/gay049
80. Sun SG, Guo JJ, Qu XY, Tang XY, Lin YY, Hua KQ, et al. The extracellular vesicular pseudogene Lgmnp1 induces M2-like macrophage polarization by upregulating lgmn and serves as a novel promising predictive biomarker for ovarian endometriosis recurrence. Hum Reprod. (2022) 37(3):447–65. doi: 10.1093/humrep/deab266
81. Liao K, Qian Z, Zhang S, Chen B, Li Z, Huang R, et al. The lgmn pseudogene promotes tumor progression by acting as a mir-495-3p sponge in glioblastoma. Cancer Lett. (2020) 490:111–23. doi: 10.1016/j.canlet.2020.07.012
82. Zhen Y, Chunlei G, Wenzhi S, Shuangtao Z, Na L, Rongrong W, et al. Clinicopathologic significance of legumain overexpression in cancer: a systematic review and meta-analysis. Sci Rep. (2015) 5(1):16599. doi: 10.1038/srep16599
83. Zhang L, Li H, Yuan M, Li D, Wang G. Exosomal mir-22-3p derived from peritoneal macrophages enhances proliferation, migration, and invasion of ectopic endometrial stromal cells through regulation of the Sirt1/nf-Κb signaling pathway. Eur Rev Med Pharmacol Sci. (2020) 24(2):571–80. doi: 10.26355/eurrev_202001_20033
84. Liu T, Liu M, Zheng C, Zhang D, Li M, Zhang L. Exosomal lncrna Chl1-As1 derived from peritoneal macrophages promotes the progression of endometriosis via the mir-610/Mdm2 axis. Int J Nanomedicine. (2021) 16:5451–64. doi: 10.2147/ijn.S323671
85. Senchenko VN, Krasnov GS, Dmitriev AA, Kudryavtseva AV, Anedchenko EA, Braga EA, et al. Differential expression of Chl1 gene during development of Major human cancers. PloS One. (2011) 6(3):e15612. doi: 10.1371/journal.pone.0015612
86. Zhang C, Wu W, Ye X, Ma R, Luo J, Zhu H, et al. Aberrant expression of Chl1 gene and long non-coding rna Chl1-As1, Chl1-As2 in ovarian endometriosis. Eur J Obstet & Gynecol and Reprod Biol. (2019) 236:177–82. doi: 10.1016/j.ejogrb.2019.03.020
87. Li Q, Yuan M, Jiao X, Huang Y, Li J, Li D, et al. M1 macrophage-derived nanovesicles repolarize M2 macrophages for inhibiting the development of endometriosis. Front Immunol. (2021) 12:707784. doi: 10.3389/fimmu.2021.707784
88. Sun H, Li D, Yuan M, Li Q, Li N, Wang G. Eutopic stromal cells of endometriosis promote neuroangiogenesis via exosome pathway. Biol Reprod. (2018) 100(3):649–59. doi: 10.1093/biolre/ioy212
89. Harp D, Driss A, Mehrabi S, Chowdhury I, Xu W, Liu D, et al. Exosomes derived from endometriotic stromal cells have enhanced angiogenic effects in vitro. Cell Tissue Research. (2016) 365(1):187–96. doi: 10.1007/s00441-016-2358-1
90. Qiu J-J, Lin X-J, Zheng T-T, Tang X-Y, Zhang Y, Hua K-Q. The exosomal long noncoding rna ahif is upregulated in Serum from patients with endometriosis and promotes angiogenesis in endometriosis. Reprod Sci. (2019) 26(12):1590–602. doi: 10.1177/1933719119831775
91. Wang S, Ma F, Feng Y, Liu T, He S. Role of exosomal mir-21 in the tumor microenvironment and osteosarcoma tumorigenesis and progression (review). Int J Oncol. (2020) 56(5):1055–63. doi: 10.3892/ijo.2020.4992
92. Wu D, Lu P, Mi X, Miao J. Exosomal mir-214 from endometrial stromal cells inhibits endometriosis fibrosis. MHR: Basic Sci of Reprod Med. (2018) 24(7):357–65. doi: 10.1093/molehr/gay019
93. Zhang Y, Chang X, Wu D, Deng M, Miao J, Jin Z. Down-Regulation of exosomal mir-214-3p targeting Ccn2 contributes to endometriosis fibrosis and the role of exosomes in the horizontal transfer of mir-214-3p. Reprod Sci. (2021) 28(3):715–27. doi: 10.1007/s43032-020-00350-z
94. Zhang M, Wang X, Xia X, Fang X, Zhang T, Huang F. Endometrial epithelial cells-derived exosomes deliver microrna-3°c to block the Bcl9/wnt/Cd44 signaling and inhibit cell invasion and migration in ovarian endometriosis. Cell Death Discov. (2022) 8(1):151. doi: 10.1038/s41420-022-00941-6
95. Hsu CY, Hsieh TH, Lin HY, Lu CY, Lo HW, Tsai CC, et al. Characterization and proteomic analysis of endometrial stromal cell-derived small extracellular vesicles. J Clin Endocrinol Metab. (2021) 106(5):1516–29. doi: 10.1210/clinem/dgab045
96. Montagna P, Capellino S, Villaggio B, Remorgida V, Ragni N, Cutolo M, et al. Peritoneal fluid macrophages in endometriosis: correlation between the expression of estrogen receptors and inflammation. Fertil & Steril. (2008) 90(1):156–64. doi: 10.1016/j.fertnstert.2006.11.200
97. Becker CM, Laufer MR, Stratton P, Hummelshoj L, Missmer SA, Zondervan KT, et al. World endometriosis research foundation endometriosis phenome and biobanking harmonisation project: i. Surgical phenotype data collection in endometriosis research. Fertil & Steril. (2014) 102(5):1213–22. doi: 10.1016/j.fertnstert.2014.07.709
98. Vitonis AF, Vincent K, Rahmioglu N, Fassbender A, Louis GMB, Hummelshoj L, et al. World endometriosis research foundation endometriosis phenome and biobanking harmonization project: ii. Clinical and covariate phenotype data collection in endometriosis research. Fertil & Steril. (2014) 102(5):1223–32. doi: 10.1016/j.fertnstert.2014.07.1244
99. Rahmioglu N, Fassbender A, Vitonis AF, Tworoger SS, Hummelshoj L, D'Hooghe TM, et al. World endometriosis research foundation endometriosis phenome and biobanking harmonization project: iii. Fluid biospecimen collection, processing, and storage in endometriosis research. Fertil & Steril. (2014) 102(5):1233–43. doi: 10.1016/j.fertnstert.2014.07.1208
100. Fassbender A, Rahmioglu N, Vitonis AF, Viganò P, Giudice LC, D’Hooghe TM, et al. World endometriosis research foundation endometriosis phenome and biobanking harmonisation project: iv. Tissue collection, processing, and storage in endometriosis research. Fertil & Steril. (2014) 102(5):1244–53. doi: 10.1016/j.fertnstert.2014.07.1209
Keywords: endometriosis, small extracellular vesicle, peritoneal microenvironment, endometriotic stromal cell, macrophage
Citation: Wang Y, Dragovic RA, Greaves E, Becker CM and Southcombe JH (2023) Macrophages and small extracellular vesicle mediated-intracellular communication in the peritoneal microenvironment: Impact on endometriosis development. Front. Reprod. Health 5:1130849. doi: 10.3389/frph.2023.1130849
Received: 23 December 2022; Accepted: 20 March 2023;
Published: 3 April 2023.
Edited by:
Elizabeth A. Stewart, Mayo Clinic, United StatesReviewed by:
Chandrakant Tayade, Queen's University, CanadaMary Louise Hull, University of Adelaide, Australia
© 2023 Wang, Dragovic, Greaves, Becker and Southcombe. This is an open-access article distributed under the terms of the Creative Commons Attribution License (CC BY). The use, distribution or reproduction in other forums is permitted, provided the original author(s) and the copyright owner(s) are credited and that the original publication in this journal is cited, in accordance with accepted academic practice. No use, distribution or reproduction is permitted which does not comply with these terms.
*Correspondence: Jennifer Southcombe amVuLnNvdXRoY29tYmVAd3JoLm94LmFjLnVr
Specialty Section: This article was submitted to Gynecology, a section of the journal Frontiers in Reproductive Health