- 1Cancer Biology and Epigenetics Group, IPO Porto Research Center (CI-IPOP), Portuguese Oncology Institute of Porto (IPO Porto), Porto, Portugal
- 2Master in Oncology, Institute of Biomedical Sciences Abel Salazar–University of Porto (ICBAS-UP), Porto, Portugal
- 3Department of Pathology, Portuguese Oncology Institute of Porto, Porto, Portugal
- 4Department of Pathology and Molecular Immunology, Institute of Biomedical Sciences Abel Salazar–University of Porto (ICBAS-UP), Porto, Portugal
- 5Department of Medical Oncology, Portuguese Oncology Institute of Porto, Porto, Portugal
- 6Department of Epidemiology, Portuguese Oncology Institute of Porto, Porto, Portugal
Breast cancer (BrC) is the most frequent malignancy and the leading cause of cancer death among women worldwide. Approximately 70% of BrC are classified as luminal-like subtype, expressing the estrogen receptor. One of the most common and effective adjuvant therapies for this BrC subtype is endocrine therapy. However, its effectiveness is limited, with relapse occurring in up to 40% of patients. Because microRNAs have been associated with several mechanisms underlying endocrine resistance and sensitivity, they may serve as predictive and/or prognostic biomarkers in this setting. Hence, the main goal of this study was to investigate whether miRNAs deregulated in endocrine-resistant BrC may be clinically relevant as prognostic and predictive biomarkers in patients treated with adjuvant endocrine therapy. A global expression assay allowed for the identification of microRNAs differentially expressed between luminal BrC patients with or without recurrence after endocrine adjuvant therapy. Then, six microRNAs were chosen for validation using quantitative reverse transcription polymerase chain reaction in a larger set of tissue samples. Thus, miR-30c-5p, miR-30b-5p, miR-182-5p, and miR-200b-3p were found to be independent predictors of clinical benefit from endocrine therapy. Moreover, miR-182-5p and miR-200b-3p displayed independent prognostic value for disease recurrence in luminal BrC patients after endocrine therapy. Our results indicate that selected miRNAs’ panels may constitute clinically useful ancillary tools for management of luminal BrC patients. Nevertheless, additional validation, ideally in a multicentric setting, is required to confirm our findings.
Introduction
Breast cancer (BrC) is the second most common cancer worldwide and the most frequent cancer among women. Despite advances in screening, early diagnosis, and treatment strategies, BrC still constitutes the leading cause of cancer-related death among women (Bray et al., 2018). BrC is a highly heterogeneous disease with distinct biological features and clinical outcomes. Based on gene expression profiling, BrC is often classified into four well-established intrinsic subtypes (Table 1) (Sørlie, 2004; Parker et al., 2009). However, due to logistic and economical constraints, surrogate approaches have been developed for routine clinical practice, using widely available immunohistochemistry (IHC) assays for estrogen receptor (ER), progesterone receptor (PR), and Ki-67 index, together with IHC and/or in situ hybridization for human epidermal growth factor 2 receptor (HER2) overexpression/amplification (Senkus et al., 2015).
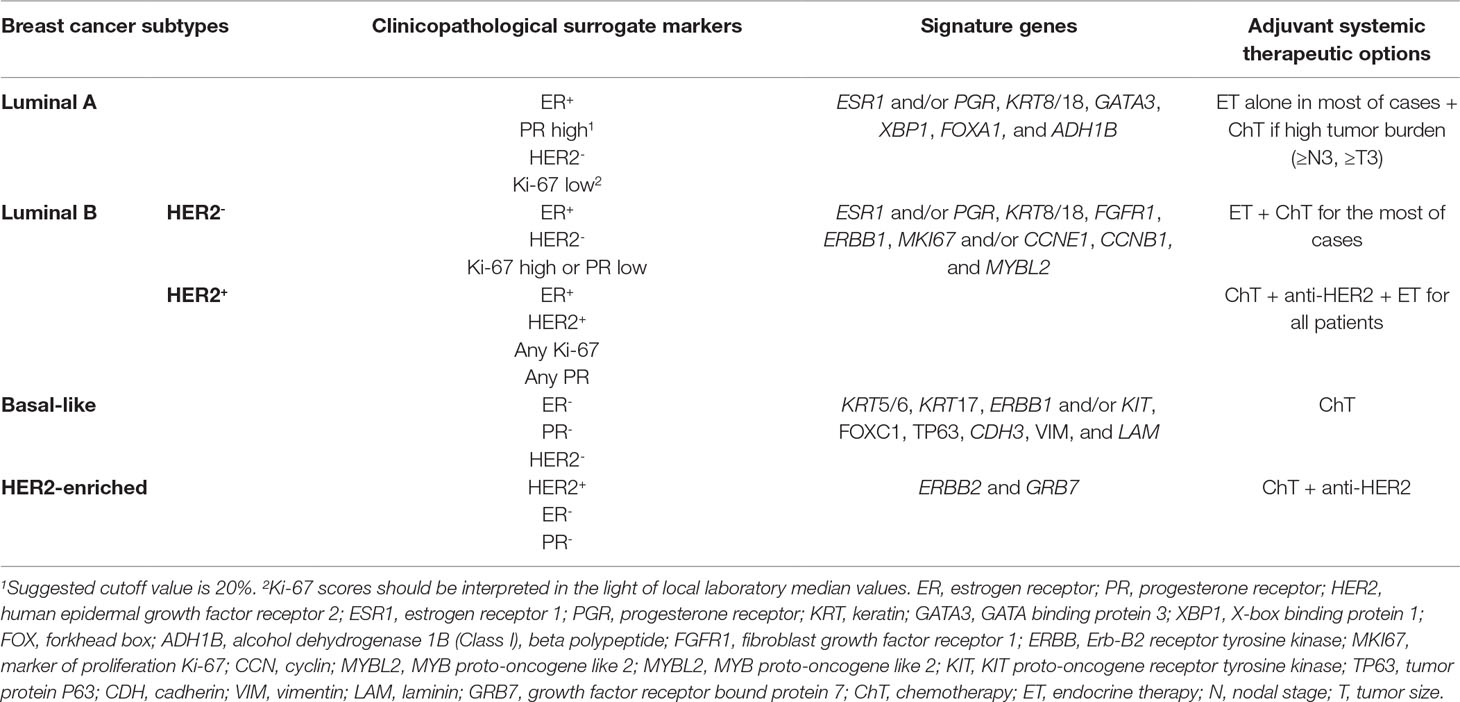
Table 1 Breast cancer molecular subtypes characterization (Perou et al., 2000; Sørlie et al., 2001; Oh et al., 2006; Eroles et al., 2012; Haque et al., 2012; Network, 2012; Howell, 2013; Zhang et al., 2014a; Senkus et al., 2015).
In addition to surgery, therapeutic strategies for BrC patients include neoadjuvant, adjuvant, and palliative treatments. Adjuvant systemic therapy, aiming to prevent BrC recurrence by eradicating micrometastases present at diagnosis, includes three modalities: chemotherapy, anti-HER2 therapy (e.g., trastuzumab), and endocrine therapy (ET). ER and HER2 status are used as predictive factors to select patients for specific adjuvant therapies (Table 1). ET, which blocks ER activation, is recommended for patients with ER-positive disease, to stop or slow the growth of hormone-sensitive BrC (Curigliano et al., 2017). Most luminal A BrC tumors do not require adjuvant chemotherapy, except those with the highest risk of relapse, whereas most luminal B tumors, especially those with HER2 overexpression, benefit from chemotherapy in addition to trastuzumab (Slamon et al., 2011). Although ET results in substantial improvement of patients’ outcome, resistance to treatment is a major hurdle (Zhang et al., 2014a), affecting 30–40% of ER-positive BrC patients, with all those treated in the metastatic setting eventually progressing (Normanno et al., 2005; Murphy and Dickler, 2016). According to the 3rd ESO–ESMO International Consensus Guidelines, endocrine resistance may be defined as primary endocrine resistance, when patients relapse within the first 2 years of adjuvant ET, or as secondary (acquired) endocrine resistance, when patients relapse while on adjuvant ET after the first 2 years of treatment or within 12 months after completing treatment (Cardoso et al., 2017).
MicroRNAs (miRNAs), a class of small (∼22 nucleotides) non-coding single-stranded RNAs, have shown promise for assisting in clinical management of BrC as diagnostic, prognostic, or predictive biomarkers (Amorim et al., 2016), namely, through assessment in liquid biopsies (plasma, serum, and urine) (Schwarzenbach et al., 2014). Indeed, several studies have associated miRNAs deregulation with endocrine resistance and prognosis in luminal BrC (Rodriguez-Gonzalez et al., 2011; Muluhngwi and Klinge, 2015; Barbano et al., 2017; Muluhngwi and Klinge, 2017). Whereas decreased ER expression and endocrine resistance may be due to miR-221/222 overexpression (Zhao et al., 2008; Rao et al., 2011; Wei et al., 2014; Song et al., 2017), miR-342-3p expression positively correlated with ER mRNA transcript levels, being downregulated in tamoxifen-refractory BrC (Cittelly et al., 2010). Moreover, miRNAs regulating growth, survival, and apoptosis of BrC cells may also be implicated in loss of responsiveness to ET by endowing tumor cells with alternative proliferative and survival stimuli (Thiantanawat et al., 2003). Indeed, miR-519a associated with worse prognosis in luminal BrC patients, directly targeting the transcripts of cyclin dependent kinase inhibitor 1A (CDKN1A) and phosphatase and tensin homolog (PTEN), allowing for enhanced signaling of the phosphoinositide3-kinase (PI3K) growth and survival pathway (Ward et al., 2014) and reducing sensitivity and tumor cell apoptosis in response to apoptotic stimuli (Breunig et al., 2017). Furthermore, miRNA-mediated endocrine resistance might be related with epithelial-to-mesenchymal transition (EMT) and metastatic potential of BrC cells, as members of the miR-200family(miR-200f), which act as major regulators of EMT, were found downregulated in endocrine-resistant BrC vs. endocrine-sensitive cell lines (Burk et al., 2008; Manavalan et al., 2013).
Herein, we aimed to identify miRNAs that might predict endocrine resistance in luminal BrC patients undergoing ET, by comparing expression levels between BrC samples of patients that developed endocrine resistance with those that did not, after long-term follow-up. Expression levels of the miRNAs identified might allow for stratification of luminal BrC cases into a low-risk patient subgroup, for which additional adjuvant systemic treatment can be safely omitted, and a high-risk group comprising patients at high risk for recurrence, allowing for detection of resistance to ET at an early stage.
Materials and Methods
Patients and Samples Collection
For this study, 139 BrC tissue samples were prospectively collected, after informed consent, from patients with luminal BrC and without metastasis at diagnosis, aged between 41 and 75 years, submitted to adjuvant ET (with or without other adjuvant modalities), after first-line surgical treatment, from 1995 to 2002 at the Portuguese Oncology Institute of Porto (IPO-Porto). Furthermore, 26 normal breast tissue samples were collected from reduction mammoplasties of contralateral breast from BrC patients. All these specimens were obtained from patients without BrC hereditary syndrome and no evidence of preneoplastic/neoplastic lesions. After surgical resection, samples were immediately frozen at −80°C. Relevant clinical and pathological data were retrieved from patients’ charts. Five-micrometer frozen sections were cut and stained with hematoxylin–eosin (H&E) staining for confirmation of BrC by an experienced pathologist, ensuring that samples contained at least 70% of tumor cells, and confirm that tissues obtained from reduction mammoplasties harbored normal epithelial cells. This study was approved by institutional ethical committee (CES-IPOFG-120/015).
BrC Subtyping
IHC was performed to identify the molecular subtype of each tumor tissue included in this study. Commercially available antibodies were used for ER (Clone 6F11, mouse, Leica), PR (Clone 16, mouse, Leica), HER2 (Clone 4B5, rabbit, Roche), and Ki-67 (Clone MIB-1, mouse, Dako). IHC was carried out in BenchMark ULTRA (Ventana, Roche) using ultraView Universal DAB Detection Kit (Ventana, Roche) according to the manufacturer’s instructions. Each case was evaluated by an experienced pathologist; it was classified according to the College of American Pathologists recommendations (Fitzgibbons et al., 2014) and categorized according to ESMO guidelines (Senkus et al., 2015). Cutoffs for Ki-67 and PR expression were set at 15% and 25% of positive cells, respectively, according to the optimized protocols of Department of Pathology.
RNA Extraction From Fresh Frozen Tissues
Total RNA was extracted from fresh frozen tissues using the TRIzol® Reagent (Invitrogen, Carlsbad, CA, USA) according to the manufacturer’s recommendations. RNA concentrations and purity ratios were ascertained using a NanoDrop Lite spectrophotometer (NanoDrop Technologies, Wilmington, DE, USA), and RNA samples were stored at −80ºC.
MiRNA cDNA Synthesis
cDNA synthesis was performed in a Veriti® Thermal Cycler (Applied Biosystems, Foster City, CA, USA) using miRCURY LNA™ Universal RT microRNA PCR (Exiqon, Vedbaek, Denmark) following the manufacturer’s instructions. cDNA samples were then stored at −20ºC.
Global Focus MiRNA PCR Panel
Global miRNAs’ expression was evaluated using a Cancer Focus microRNA PCR Panel, 384-well (V4.R) (Exiqon). Each plate, besides containing 80 lyophilized LNA™ miRNA primer sets focusing on cancer-relevant human miRNAs, also contained interplate calibrators, candidate reference genes [miRNAs and small nuclear RNAs (snRNAs)], and one water blank. In each well, 0.05 μl of cDNA previously synthesized, 5 μl of SYBR® Green master mix (Exiqon), and 4.95 μl of nuclease-free water (Exiqon) were added. Quantitative reverse transcription polymerase chain reactions (RT-qPCR) were performed in the LightCycler 480 instrument (Roche Diagnostics, Manheim, Germany) according to the following conditions: 95ºC for 10 min and 45 cycles at 95ºC for 10 s and 60ºC for 1 min.
The median values of miR-103a-3p, miR-107, miR-191-5p, and SNORD38B were used for normalization, as these genes were the most stably expressed candidate reference genes (Supplementary Figure 1). Differences in expression values for target miRNAs were calculated using the 2−ΔΔCT method. The selection of deregulated miRNAs for further validation was performed considering prominent fold change, good sensitivity for qRT-PCR detection (Ct values, in general, below 30), and novelty.
Individual Assays
Initially, cDNA samples were diluted 80× in sterile distilled water (B. Braun, Melsungen, Germany). Then, on ice, per each well of a 384-well plate, the following were added: 5 μl of NZYSpeedy qPCR Green Master Mix (2×) (NZYTECH, Portugal), 1 μl of miRNA specific primer mix (microRNA LNA™ PCR primer set, Exiqon), and 4 μl of previously diluted cDNA. Each amplification reaction was performed in triplicate on a LightCycler 480 instrument (Roche Diagnostics, Manheim, Germany). Each plate also contained two negative template controls. RT-qPCR protocol consisted of a denaturation step at 95ºC for 2 min, followed by 40 amplification cycles at 95ºC for 5 s and 60ºC for 20 s. Melting curve analysis was performed according to the instrument’s manufacturer’s recommendations.
SNORD38B was used as a reference gene for data normalization, as this gene was the most stably expressed over the whole range of the samples used for the global expression assay. Notwithstanding, the stability of SNORD38B expression was empirically validated in additional samples. Relative miRNA expression in each sample was calculated by the 2−ΔΔCT method (the target sequences of mature miRNAs analyzed are provided in Supplementary Table 1).
Statistical Analysis
Statistical analysis was performed using SPSS software (SPSS Version 24.0, Chicago, IL), and two-tailed p values were considered statistically significant when p < 0.05. Graphs were constructed using GraphPad 6 Prism (GraphPad Software, USA).
MiRNA Expression Analysis
Fold changes for single miRNAs were calculated using the 2−ΔΔCT method (Livak and Schmittgen, 2001).
Association Between MiRNA Expression and Clinicopathological Features
To ascertain statistical significance for continuous variables, comparisons were made between independent samples and non-parametric Mann–Whitney U tests were performed. Spearman nonparametric correlation test was performed to assess the association between continuous variables. Chi-square test or Fisher’s exact test were used as appropriate to compare proportions between two groups.
Survival Analysis
Some clinicopathological features were grouped, including pT stage (T1 and T2 and T3 and T4), pN stage (N0 and N1 and N2 and N3), and grade [grade (G)1 and G2 and G3] (Lakhani, 2012). Age was categorized into four groups (≤44, 45–64, 65–74, and ≥75), and miRNA expression levels were categorized according to 25th or 75th percentile. All survival analyses were restricted to 15 years of follow-up. Cox regression univariable and multivariable models were computed to assess standard clinicopathological variables and miRNA prognostic value. Hazard ratios (HRs) along with respective 95% confidence interval (95% CIs) were reported. Multivariable Cox models only included the statistically significant variables. Kaplan–Meier with log rank test was used to construct and compare survival curves according to categorized miRNA expression levels. Endocrine resistance-free survival (ERFS) was defined as the time between surgery and the recurrence dates. Recurrences occurring after 12 months of completing ET were not considered events for this analysis. Disease-free survival (DFS) was defined as the time between surgery date and recurrence date. Distant metastasis-free survival (DMFS) was defined as the time between surgery and the development of distant metastases. For prognostic assessment of miRNAs combined in panels, the miRNAs that remained statistically significant in multivariable analysis were differently combined, considering the same categories used in previous survival analysis (expression above or below P25). The best panels were selected based on the individual markers value in the Cox model: better HR, smaller 95% CI and p value, as well as value in stratified analysis.
Results
Characteristics of Study Populations
The discovery cohort (n = 16), used for global expression assay analysis, consisted of four luminal A and four luminal B tumors from BrC patients who relapsed, and the same number of patients who did not relapse after adjuvant ET. Patients who relapsed during adjuvant ET or within the first 12 months of completing adjuvant ET were considered endocrine-resistant (Table 2).
The validation cohort was composed of a total of 149 subjects, comprising 123 luminal BrC and 26 normal breast tissues. Among 34 cancer patients that recurred during follow-up time, 20 were considered endocrine-resistant. Clinical and pathological characteristics of patients and controls included in this study are shown in Table 3. Endocrine-sensitive and endocrine-resistant groups did not significantly differ concerning age distribution (p = 0.136). As expected, most of the endocrine-resistant cases were classified as luminal B (p = 0.011) and depicted high Ki-67 index (p = 0.001). Moreover, this group also showed a higher number of high-grade (G3) cases (p = 0.027). For the remaining clinicopathological features or treatment modalities, no significant differences were depicted.
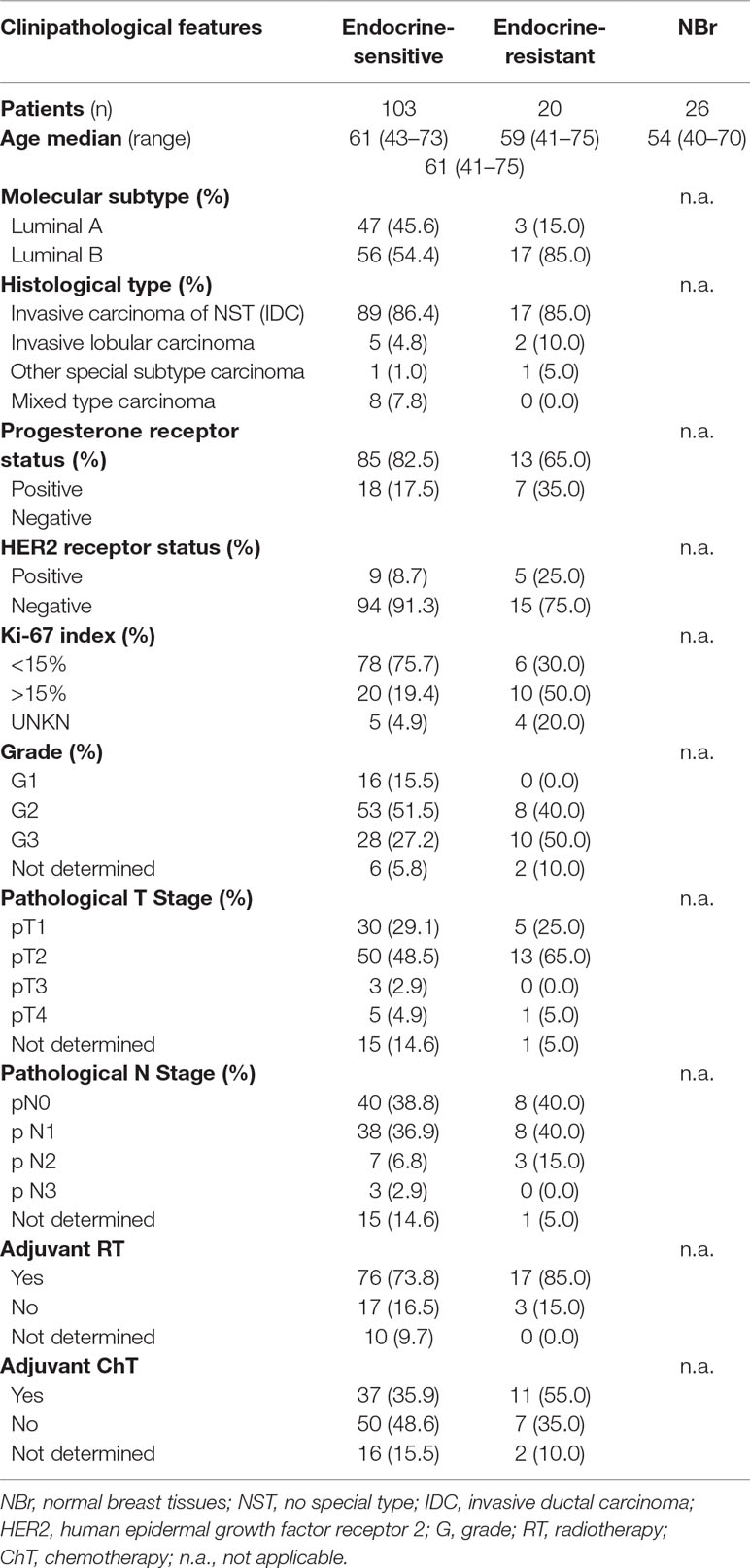
Table 3 Clinical and pathological data of luminal tumors and normal breast samples included in the validation cohort.
Global Focus MiRNA PCR Panel Analysis
In the global expression assay, one luminal A case with recurrence was excluded from the analysis, due to low RT-qPCR success rate (25% of the miRNAs did not amplify, and the remaining showed Ct values higher than 30). Likewise, 3 (miR-202-3p, -206, and -20b-5p) out of the 80 miRNAs were excluded due to low real-time PCR success rates. MiRNAs with fold variation values higher than 1 were selected, resulting in a panel comprising 56 miRNAs (Table 4).
Gene-Specific Assays
From the global expression assay analysis, miR-30b-5p, miR-30c-5p, miR-181a-5p, miR-182-5p, miR-200b-3p, and miR-205-5p were selected for further validation. All these miRNAs disclosed prominent fold change and good sensitivity for qRT-PCR detection, with different ranges of expression. MiR-30b-5p was chosen because several studies focused on other members of the miR-30 family (miR-30f) and, to the best of our knowledge, its predictive potential for ET had not been assessed previously ( Cheng et al., 2012; Bockhorn et al., 2013; Zhang et al., 2014b; D’aiuto et al., 2015; Yang et al., 2017). MiR-181a-5p and miR-200b-3p were selected to confirm the reported association with endocrine resistance in in vitro studies (Hiscox et al., 2006; Maillot et al., 2009; Manavalan et al., 2011; Vesuna et al., 2012; Manavalan et al., 2013). Furthermore, miR-182-5p was also selected to better ascertain its role in endocrine resistance due to controversial results in global focus miRNA PCR panel, since it was overexpressed in luminal B tumors from recurrent patients and downregulated in luminal A tumors from recurrent patients. Finally, miR-30c-5p was chosen as a positive control since higher expression levels of this miRNA had been positively associated with benefit of ET, in multivariate analysis, in advanced ER-positive BrC (Rodriguez-Gonzalez et al., 2011).
Except for miR-205-5p expression (p = 0.001), miR-181a-5p (p = 0.004), miR-182-5p (p < 0.001), and miR-200b-3p (p < 0.001), expression levels were significantly higher in luminal BrC tissues than in normal breast tissues (Figure 1), whereas no differences were depicted for the levels of the remaining miRNAs. Nonetheless, miR-30b-5p (p = 0.031), miR-30c-5p (p = 0.002), and miR-200b-3p (p = 0.021) were significantly downregulated in endocrine-resistant BrC samples compared to endocrine-sensitive tumors (Figure 2).
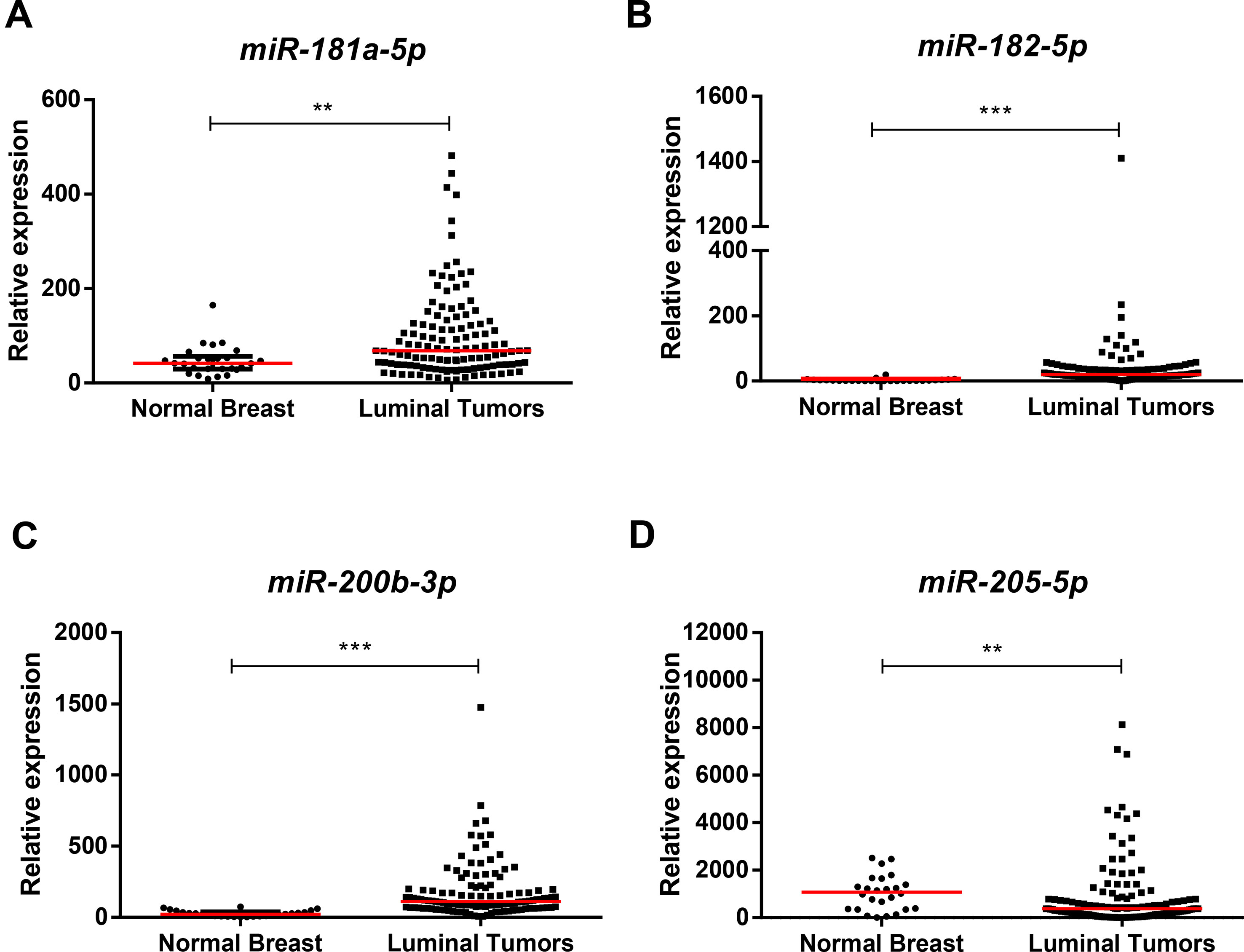
Figure 1 Scatterplots of miR-181a-5p (A), miR-182-5p (B), miR-200b-3p (C), and miR-205-5p (D) relative expression levels in luminal tumor tissues and normal breast tissues. A ** denotes p value <0.01 and a *** denotes p value <0.001 by non-parametric Mann–Whitney U test. Y-axis denotes 2−ΔΔCT values multiplied by 1000. Red horizontal lines represent median value.
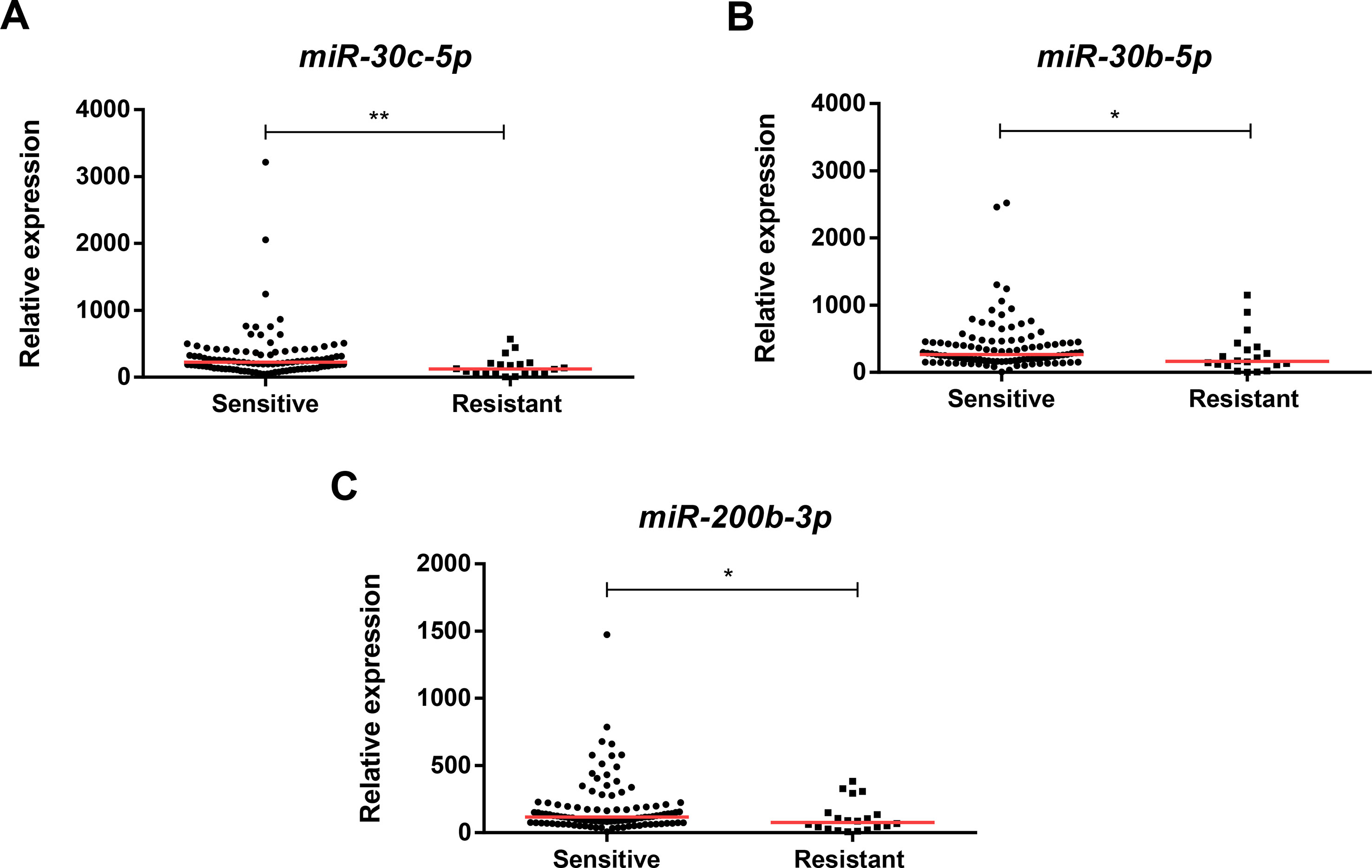
Figure 2 Scatterplots of miR-30c-5p (A), miR-30b-5p (B), and miR-200b-3p (C) relative expression levels in tumor tissues from endocrine-sensitive and -resistant patients. A * denotes p value <0.05 and a ** denotes p value <0.01 by non-parametric Mann–Whitney U test. Y-axis denotes 2−ΔΔCT values multiplied by 1000. Red horizontal lines represent median value.
Association Between MiRNA Expression and Clinicopathological Features
Higher miR-30b-5p and miR-30c-5p expression levels were found in tumors lacking HER2 overexpression (HER2-negative) (p = 0.010, p = 0.014, respectively). Conversely, lower miR-205-5p expression levels were found in high grade (G3) BrC (p = 0.009) compared to G1/G2 BrC (Figure 3). Moreover, miR-205-5p expression levels inversely correlated with patients’ age (R = −0.200, p = 0.027).
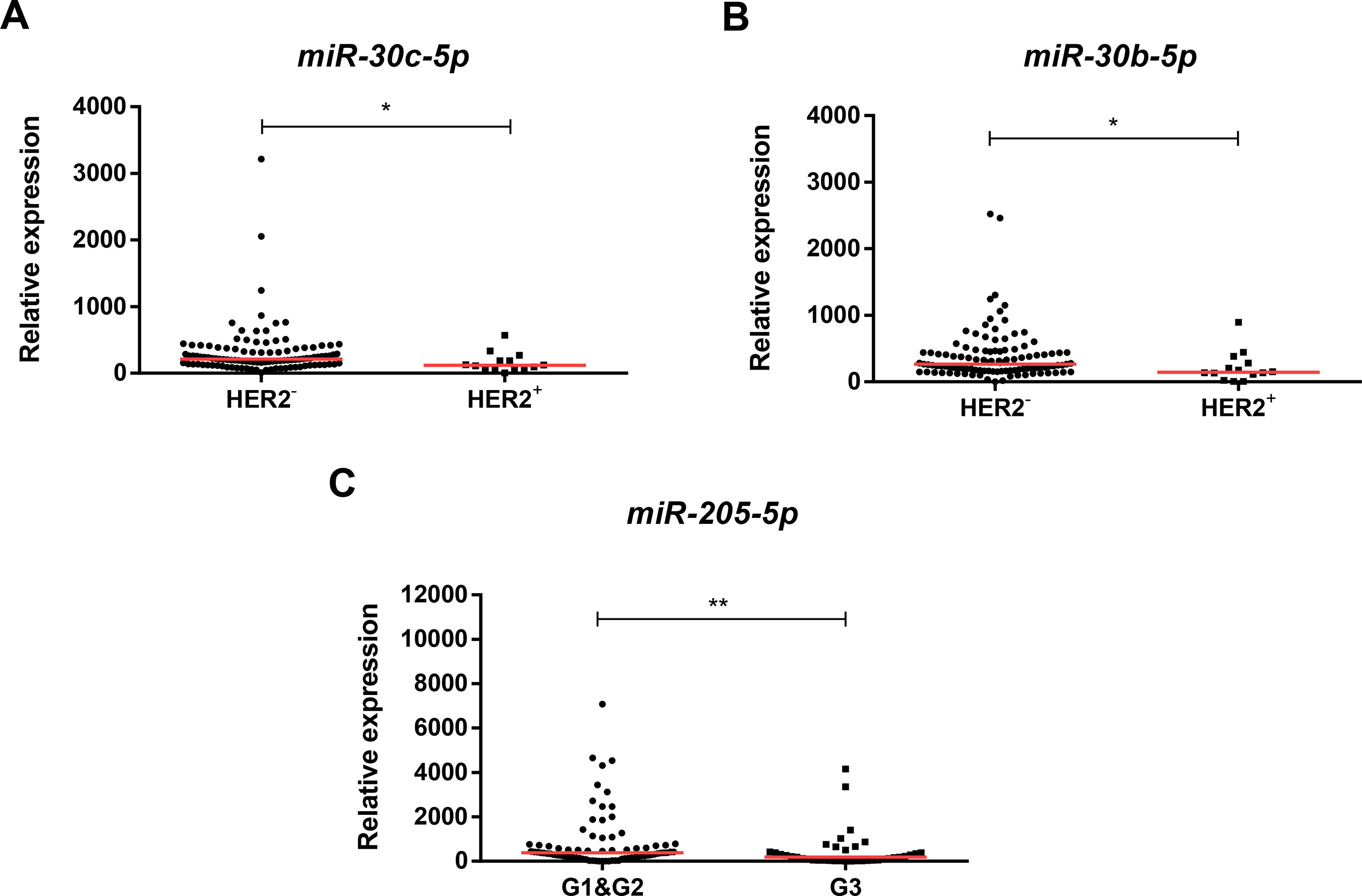
Figure 3 Scatterplots of miR-30c-5p relative expression levels according to HER2 status (A), miR-30b-5p relative expression according to HER2 status (B), and miR-205-5p relative expression according to grade (C). A * denotes p value <0.05 and a ** denotes p value <0.01 by non-parametric Mann–Whitney U test. Y-axis denotes 2−ΔΔCT values multiplied by 1000. Red horizontal lines represent median value.
Survival Analyses
The median follow-up time was 180 months (17.4–180 months). At 15 years of follow-up, 70 (56.9% of total) patients were alive, of whom 66 (53.7% of total) had no evidence of cancer. Moreover, from the 53 patients (43.1% of total) who died, death was due to BrC in 30 (24.4% of total).
Overall, in univariable analysis, most standard clinicopathological parameters were significantly associated with ERFS. Specifically, patients with HER2 positivity (HR = 2.91, p = 0.039), high Ki-67 index (HR = 5.59, p = 0.001), high grade (G3) (HR = 2.84, p = 0.028), and luminal B subtype (HR = 4.48, p = 0.017) disclosed shorter ERFS. Importantly, the same was observed for patients with lower miR-30c-5p, miR-30b-5p, miR-182-5p, and miR-200b-3p levels (Table 5, Figure 4). In multivariable analysis, all miRNAs remained independent predictors of ERFS adjusted for Ki-67 index (Table 5). After stratification for Ki-67 index, miR-30c-5p, miR-182-5p, and miR-200b-3p only independently predicted shorter ERFS in highly proliferative tumors, whereas miR-30b-5p was significant in tumors with low proliferative (Table 6).
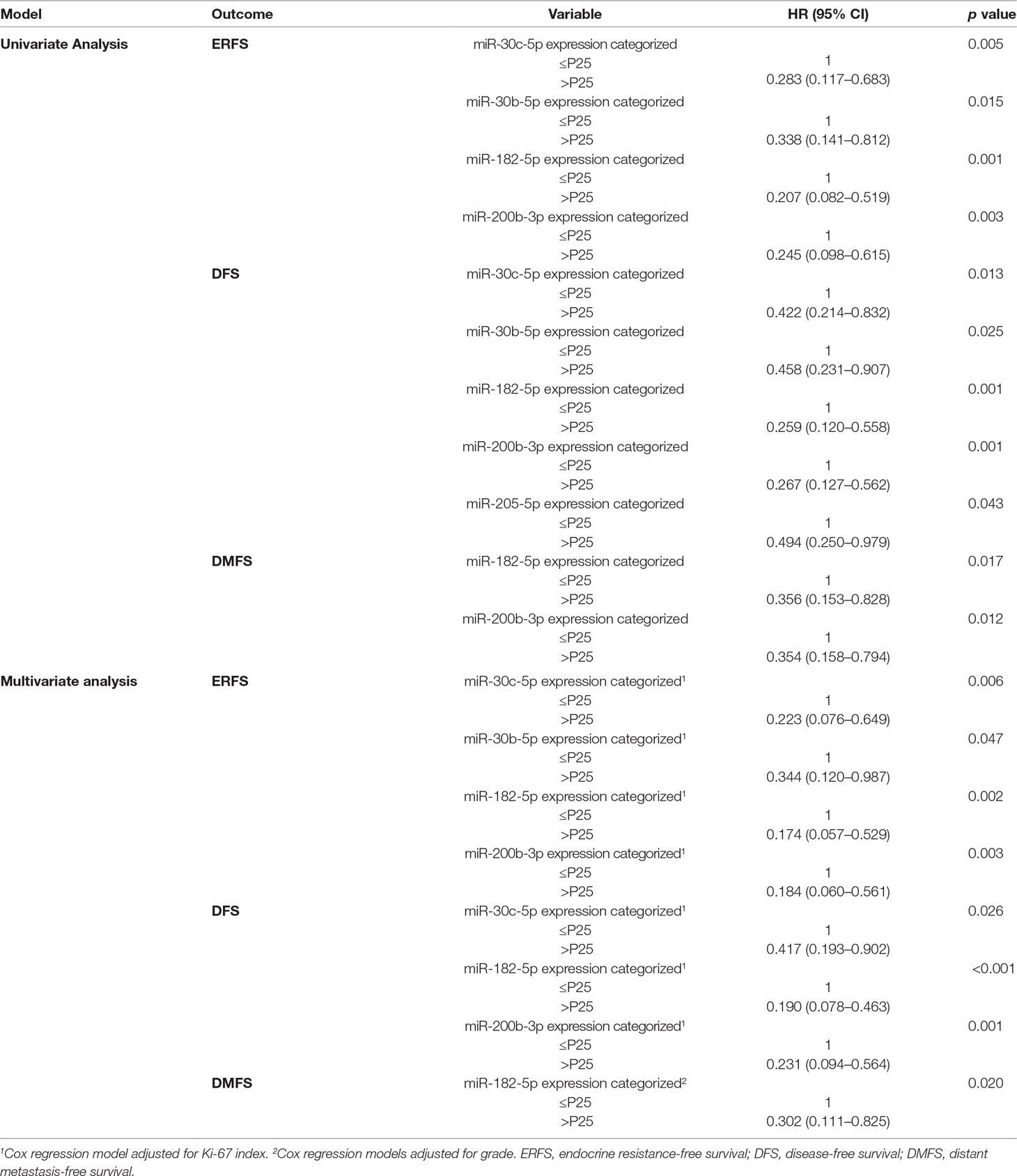
Table 5 Univariable and multivariable Cox regression models assessing the association between microRNAs expression levels and clinical outcome.
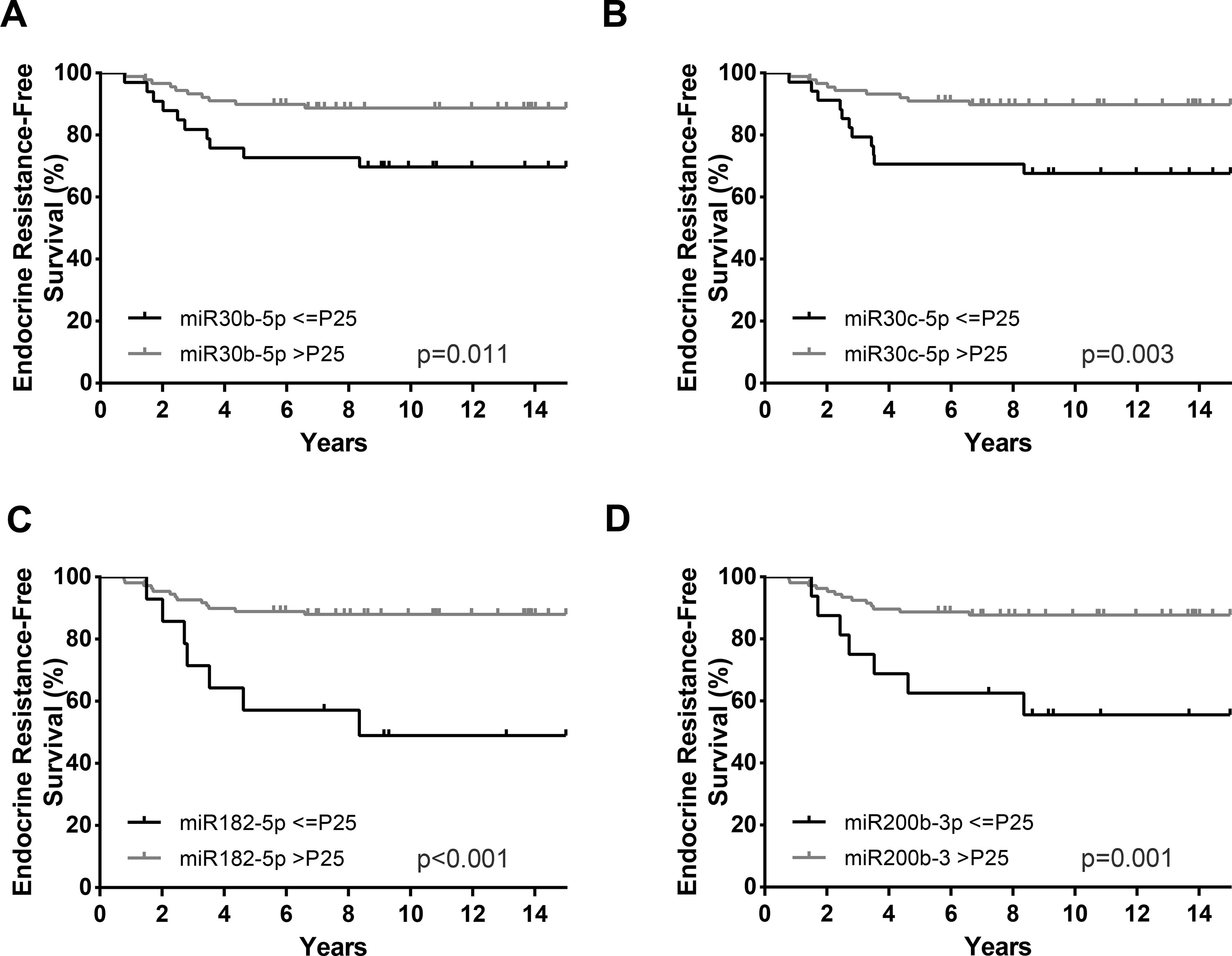
Figure 4 Endocrine resistance-free survival (ERFS) curves (Kaplan–Meier with log rank test) of miR-30b-5p (A), miR-30c-5p (B), miR-182-5p (C), and miR-200b-3p (D). P25, Percentile 25.
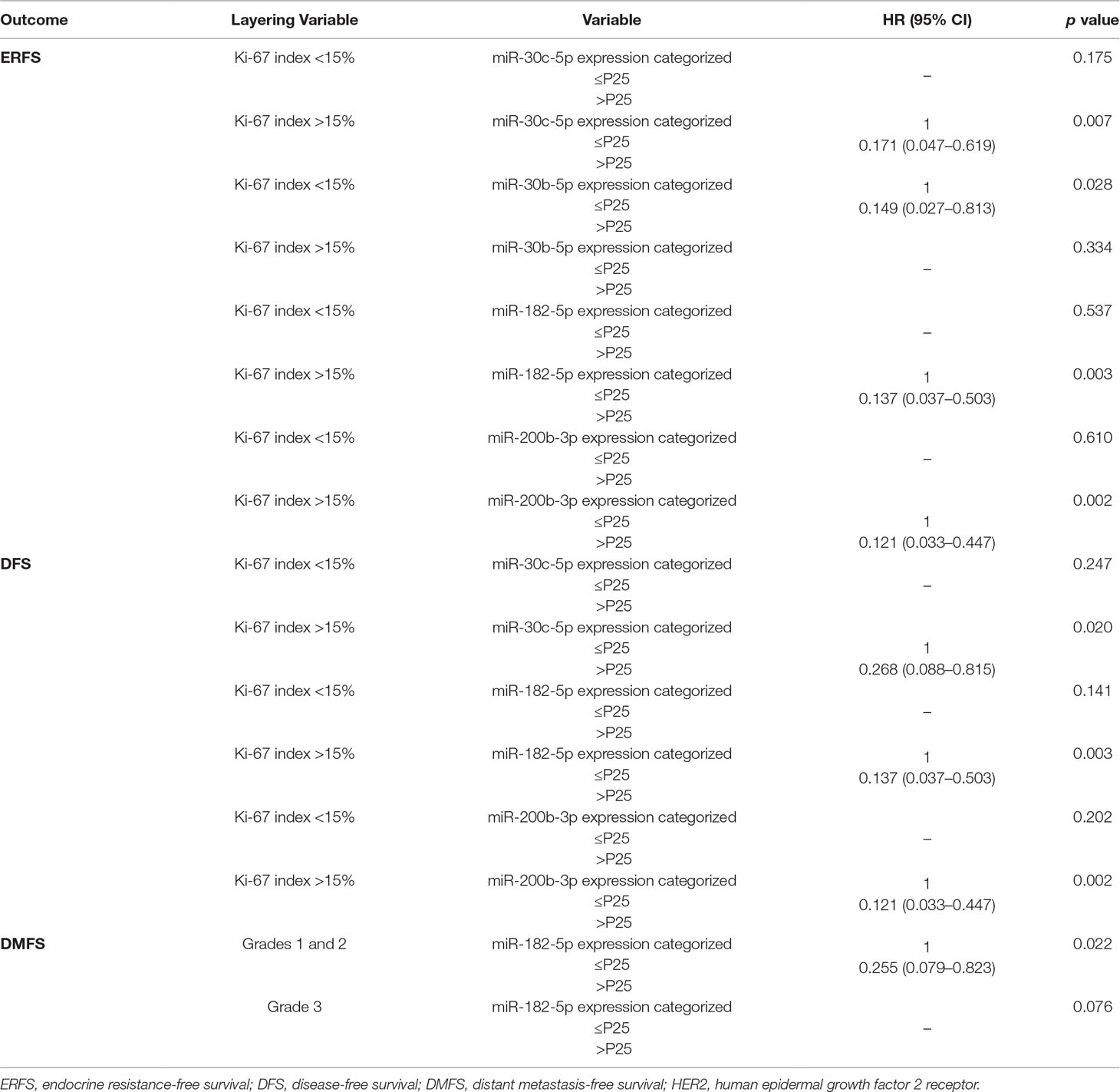
Table 6 Cox regression models stratified according to the clinicopathological features with statistical significance in the multivariate analysis.
Regarding DFS, in addition to HER2 positivity (HR = 2.40, p = 0.039), high Ki-67 index (HR = 3.01, p = 0.003), and high grade (G3) (HR = 2.65, p = 0.006), lower miR-30c-5p, miR-30b-5p, miR-182-5p, miR-200b-3p, and miR-205-5p expression levels associated with decreased DFS in univariable analysis (Table 5, Figure 5). Nonetheless, in the multivariable model, only miR-30c-5p, miR-200b-3p, and miR-182-5p were disclosed as independent prognostic predictors adjusted for Ki-67 index (Table 5), and after stratification according for Ki-67 index, all miRNAs retained statistical significance in high Ki-67 index BrC patients (Table 6). Similarly, HER2 positivity (HR = 2.63, p = 0.024), high Ki-67 index (HR = 2.48, p = 0.021), and high grade (G3) (HR = 2.69, p = 0.007) associated with worse DMFS, along with lower miR-182-5p and miR-200b-3p expression levels, in univariate analysis (Table 5). However, only miR-182-5p retained statistical significance when adjusted for tumor grade in multivariable analysis (Table 5). After stratification by tumor grade, miR-182-5p showed prognostic value in patients harboring low/intermediate-grade tumors (Table 6).
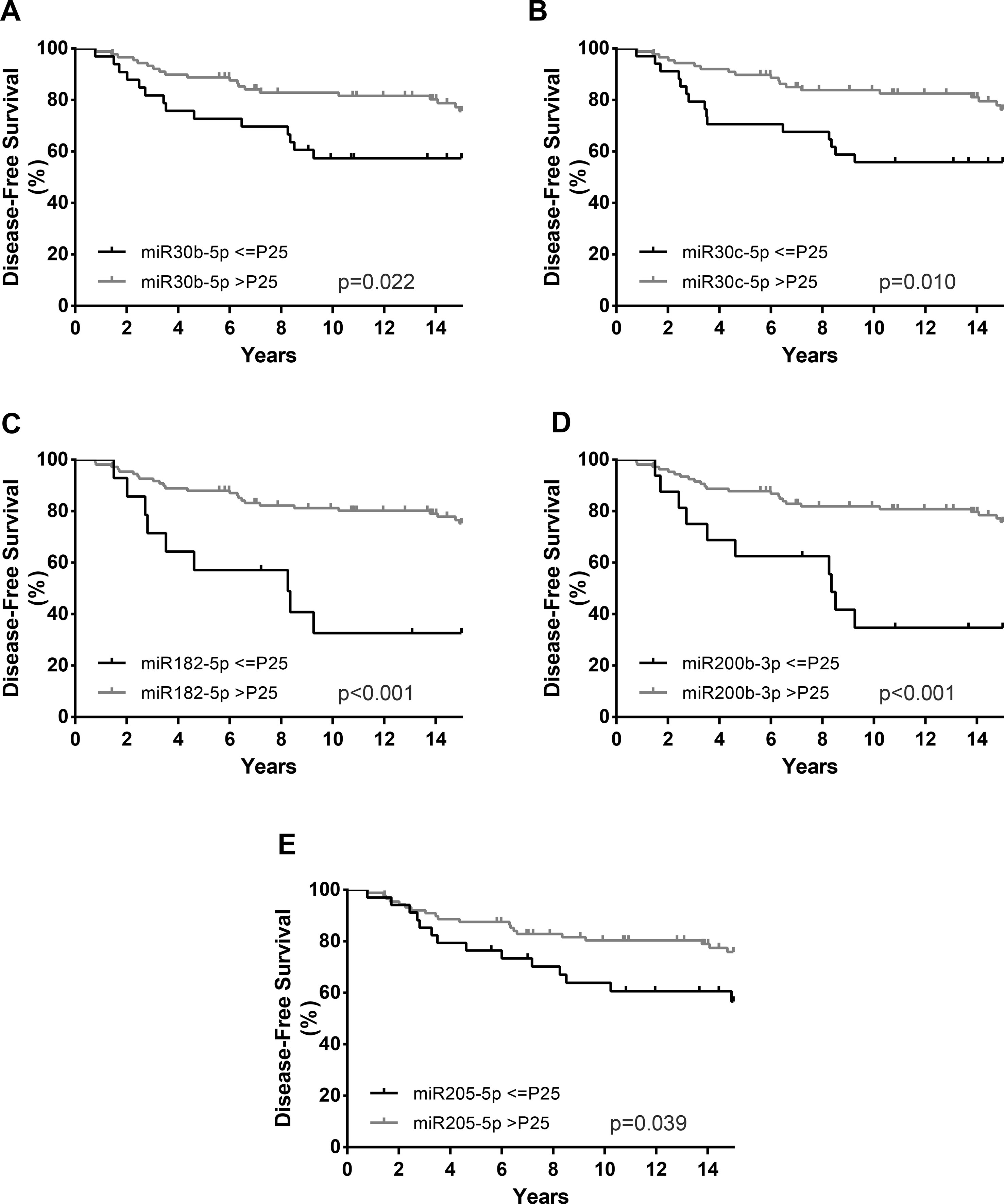
Figure 5 Disease-free survival (DFS) curves (Kaplan–Meier with log rank test) of miR-30b-5p (A), miR-30c-5p (B), miR-182-5p (C), miR-200b-3p (D), and miR-205-5p (E). P25, Percentile 25.
Furthermore, the prognostic value of the miRNAs that individually predicted ERFS and DFS was assessed when combined in panels. For ERFS, the patients were grouped as expression above P25 for 3 or 4 miRNAs versus expression below P25 for 2 or more miRNAs. Thus, the combination of miR-30c-5p, miR-30b-5p, miR-182-5p, and miR-200b-3p was shown as the best predictors of ERFS. Patients with miRNAs’ expression below P25 displayed a shorter ERFS (p < 0.001), paralleling the results obtained in single miRNAs analysis (Figure 6, Table 7). In multivariable analysis, miRNAs combined in panel were found to be independent ERFS predictors after Ki-67 index stratification (Table 7). Regarding DFS, the best predictive panel was composed of miR-182-5p and miR-200b-3p. The patients were grouped as expression above P25 for both miRNAs versus expression below P25 for at least one miRNA. Patients with both miRNAs’ expression levels above P25 showed longer DFS (p < 0.001) (Figure 6, Table 7). In multivariable analysis, miRNAs combined in panel remained independent DFS predictors, although only in cases with high Ki-67 index (Table 7).
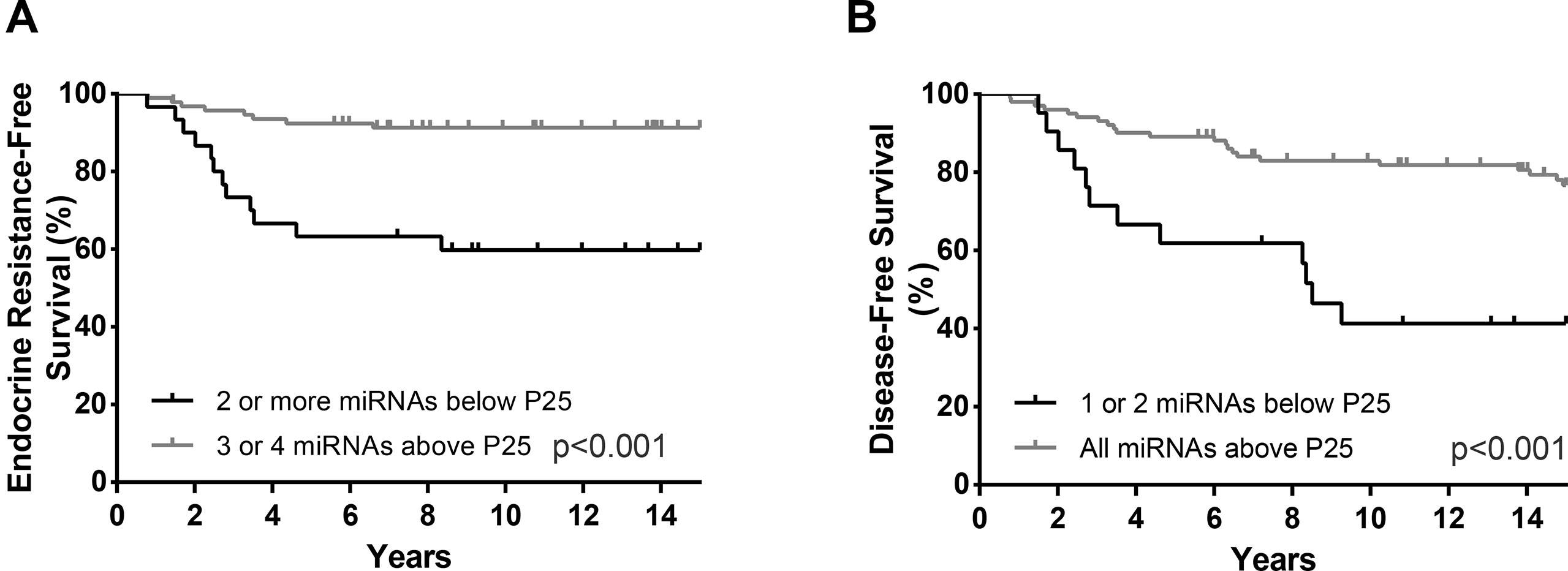
Figure 6 ERFS curves (Kaplan–Meier with log rank test) of combined miR-30b-5p, miR-30c-5p, miR-182-5p and miR200b-3p (A) and DFS curves (Kaplan–Meier with log rank test) of combined miR-182-5p and miR-200b-3p panel (B).
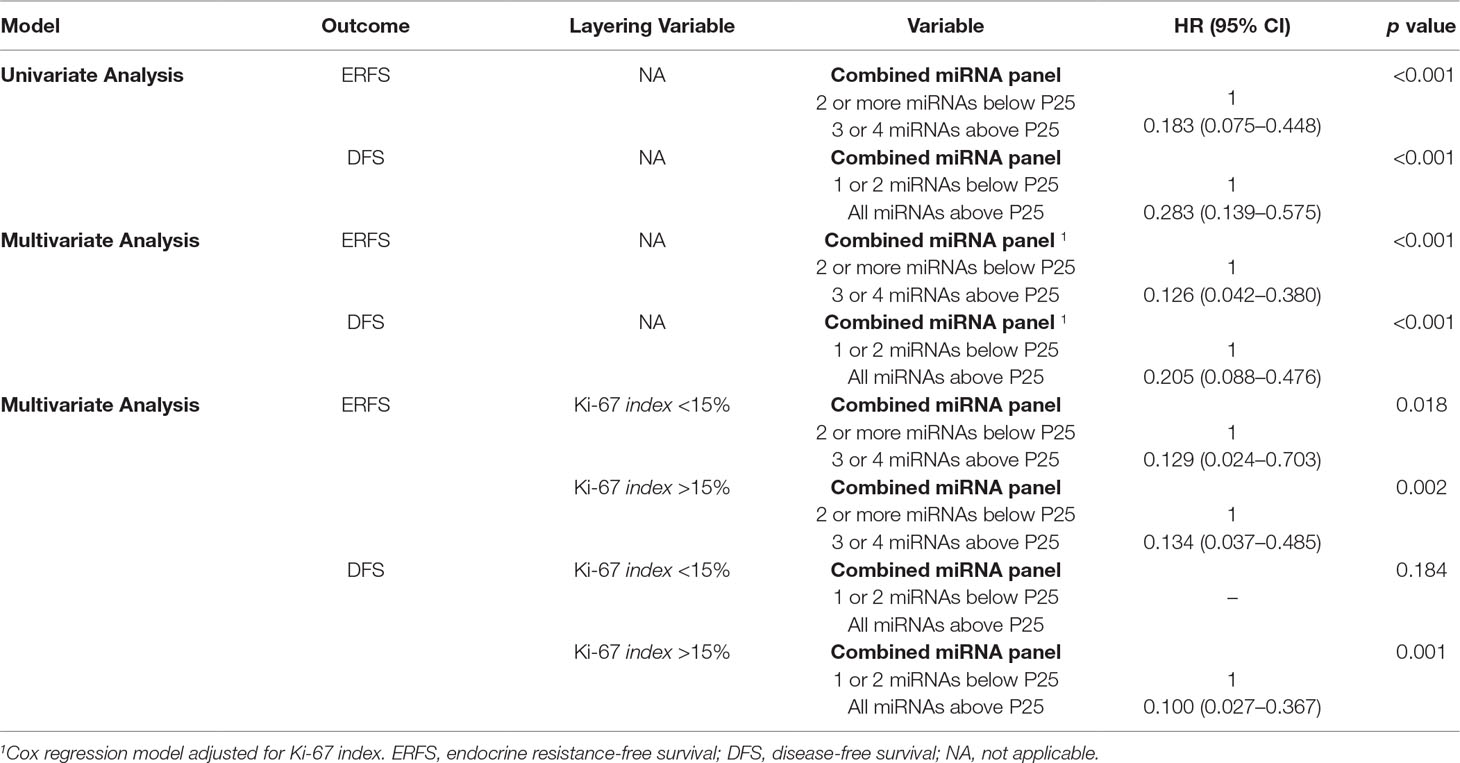
Table 7 Univariable and multivariable Cox regression models assessing the association between combined microRNAs expression panel and clinical outcome.
Discussion
BrC remains the most common malignancy in women and a major cause of morbidity and mortality (Bray et al., 2018). De-escalation of both systemic and local adjuvant treatment, paralleling trends in surgery, is critical to provide patient-tailored treatment and avoid harmful side effects (Hwang, 2014; Senkus et al., 2015). Indeed, identification of luminal BrC patients with low recurrence risk after or while on ET, for which additional adjuvant systemic treatment can be safely omitted, is very important. On the other hand, identification of high-risk luminal BrC patients requiring more aggressive treatment regimens is critical to avoid recurrence and subsequent metastatic disease, which currently affects approximately 40% of luminal BrC patients after adjuvant ET (Guarneri and Conte, 2004; Normanno et al., 2005; Murphy and Dickler, 2016). Thus, identification of biomarkers providing predictive and prognostic information in this group of patients is clinically relevant. Assessment of specific miRNAs’ expression deregulation, which has been associated with several mechanisms underlying endocrine resistance and sensitivity (Muluhngwi and Klinge, 2015; Muluhngwi and Klinge, 2017), might provide such kind of information. Nonetheless, most of those studies have been performed in cancer cell lines and display several limitations, including absence of epithelial–stromal and tumor–host interactions, that could modulate sensitivity in vivo (Shekhar et al., 2003). Conversely, tissue analysis from patients treated with ET may allow for broader insight into biologically and clinically relevant miRNAs that may serve as markers of response or resistance to ET. Thus, we focused on the identification of aberrantly expressed miRNAs in endocrine-resistant BrC, exploring its predictive and prognostic value in luminal BrC patients treated with adjuvant ET.
The first step of this study consisted on the profiling of miRNAs’ expression patterns, looking for differences between endocrine-sensitive and endocrine-resistant luminal BrC. Hence, miR-30c-5p, miR-30b-5p, miR-181a-5p, miR-182-5p, miR-200b-3p, and miR-205-5p were selected for validation in a larger set of luminal BrC and normal breast tissues. Upregulation of miR-181a-5p and miR-182-5p and downregulation of miR-205-5p in this BrC tissue cohort was consistent with previous reports (Hui et al., 2009; Li et al., 2014a; Zhang and Fan, 2015), providing indirect validation of our methodological approach. However, miR-200b-3p downregulation in tumor compared to normal tissues has been previously reported (Ye et al., 2014; Yao et al., 2015). Nevertheless, these studies have used non-cancerous tissues from breasts harboring carcinoma as controls, which may not represent truly normal breast tissues. Our results also confirm the biomarker potential of miR-30c-5p, which was found downregulated in endocrine-resistant BrC patients and independently predicted ERFS in luminal BrC patients, particularly in highly proliferative tumors. Moreover, miR-30c-5p expression correlated with HER2 status, one of the most important predictive factors for ET sensitivity (Konecny et al., 2003). In fact, HER2 signaling activation has been widely implicated in endocrine resistance (Moon et al., 2011; AlFakeeh and Brezden-Masley, 2018). Moreover, miR-200b-3p expression levels displayed the same trend and, together with miR-30b-5p and miR-182-5p, also independently predicted ERFS in luminal BrC patients. Importantly, we were able to validate in primary BrC the association between miR-200b-3p and endocrine-resistance, which was previously reported only in in vitro models (Manavalan et al., 2013). Interestingly, several members of miR-30f have been reported as markers of favorable prognosis in BrC (Cheng et al., 2012; Bockhorn et al., 2013; Zhang et al., 2014b; D’aiuto et al., 2015; Croset et al., 2018) and our study also revealed that miR-30b-5p might be predictive of response to ET. Finally, concerning miR-182-5p, our results extended previous observations on the correlation with clinical benefit from therapy with tamoxifen in advanced-stage BrC, only previously demonstrated in univariable analysis (Rodriguez-Gonzalez et al., 2011).
In addition to their predictive value, miR-30b-5p and miR-30c-5p lower expression levels also associated with decreased DFS, although in univariable analysis only. Indeed, the role of miR-30f members as tumor suppressors in BrC has been previously reported (Bockhorn et al., 2013; Zhang et al., 2014b). Furthermore, decreased levels of miR-30f members in BrC patients have been associated with poor relapse-free survival (Croset et al., 2018). Importantly, lower miR-182-5p and miR-200b-3p expression levels independently associated with decreased DFS in highly proliferative tumors. The role of miR-200b-3p as a prognostic marker in BrC is not a novelty (Ye et al., 2014; Yao et al., 2015). Indeed, members of miR-200f are known to act as enforcers of epithelial phenotype through either Zinc finger E-box-binding homeobox (ZEB)-dependent or -independent pathways (Li et al., 2014b). Intriguingly, most in vitro studies consistently attributed an oncogenic role to miR-182-5p (Chiang et al., 2013; Zhan et al., 2017). Nonetheless, higher miR-182-5p expression levels were associated with poor clinical outcome in BrC patients (Song et al., 2016), contrarily to our findings. It should be recalled, however, that miR-182-5p is a member of a miRNA family comprising three homologous, coordinately expressed, miRNAs (miR-183, miR-182, and miR-196), which are clustered in chromosome 7q32.2 and that members of this cluster have been associated with both pro- and anti-metastatic behavior in BrC, suggesting that miR-183/96/182 cluster members may have divergent functions that are regulated in a context- and tissue-dependent manner (Lowery et al., 2010; Li et al., 2014a; Hong et al., 2016). Furthermore, the 7q32.2 locus has been considered a metastasis suppressor locus, enduring genetic copy number losses in BrC progression (Png et al., 2011). Thus, the association between miR-182-5p downregulation and worse prognosis probably results from a complex molecular scenario and additional studies are required to discriminate which members of the miR-183/96/182 cluster might contribute and to which extent to BrC prognosis.
BrC tissues displayed higher miR-182-5p and miR-200b-3p levels compared to normal breast, although miR-182-5p and miR-200b-3p downregulation associated with shorter DMFS. Because development of solid neoplasms results from multiple sequential steps in which malignant cells undergo widespread modifications allowing for successful migration and colonization of other organs, we are tempted to speculate whether a context-dependent role of these miRNAs might contribute to the emergence of a malignant phenotype. Indeed, decreased expression of miR-200f members might be associated with EMT initiation, enabling cells with invasive capabilities, whereas subsequent upregulation might be associated with mesenchymal-to-epithelial transition, facilitating colonization (Gravgaard et al., 2012; Hilmarsdottir et al., 2014).
Combined expression levels of miR-30c-5p, miR-30b-5p, miR-182-5p, and miR-200b-3p independently predicted ERFS, when adjusted for confounding factors (Ki-67 index). In fact, this combined miRNA panel was associated with ERFS in both low and highly proliferative tumors. In parallel, the miR-182-5p/miR-200b-3p panel was shown to independently predict DFS in highly proliferative tumors. As previously reported in different tumor models, the combination of miRNAs in a panel might enable a more efficient diagnostic, predictive, and prognostic model overcoming the questionable value of single miRNAs (Sahlberg et al., 2015; Chen et al., 2018).
Although the retrospective design of the study and the relatively small number of samples of the discovery cohort constitute important limitations, our results suggest that a panel of miRNAs might be tested in primary tumor tissues to assess the likelihood of recurrence and resistance to ET in newly diagnosed luminal BrC. Nevertheless, these miRNAs need to be carefully validated, ideally in multicenter studies, to generate more conclusive results. Furthermore, in vitro studies, including gain- and loss-of-function assays following in vitro treatment with ET, are also critical to functionally characterize the role of these miRNAs. As a future perspective, we intend to evaluate the putative role of these miRNAs in tumor progression and dissemination. Additionally, we also intend to evaluate the potential role of these miRNAs in liquid biopsies, evaluating their potential as non-invasive biomarkers. Indeed, miRNAs in circulation would enable the repeated noninvasive monitoring of miRNA expression profile changes during treatment’s course, which could allow for early detection of ET resistance and/or recurrence, potentially improving the management and care of luminal BrC patients.
Ethics Statement
This study was carried out in accordance with the recommendations of Comissão de ética para a Saúde of Portuguese Oncology Institute of Porto, Portugal (CES-IPOFG-120/015) with written informed consent from all subjects. All subjects gave written informed consent in accordance with the Declaration of Helsinki. The protocol was approved by the Comissão de ética para a Saúde of Portuguese Oncology Institute of Porto, Portugal.
Author Contributions
MA prepared tissues for molecular analyses, including RNA extraction and cDNA synthesis, performed RT-qPCR assays, analyzed data, and drafted the manuscript. JL and NC collected normal breast tissues from reductive mammoplasty and assisted in histopathological evaluation of tissue samples. HE-P and SS contributed in data analysis and in the manuscript preparation. MF-S and SPS collected clinical follow-up data. PL performed IHC of all cases. LA assisted in the statistical analyses. RH performed histopathological evaluation of fresh frozen sections stained by H&E. RH and CJ designed and supervised the study and revised the manuscript. All the authors read and approved the final manuscript.
Funding
This work was supported by a grant from the Research Center of Portuguese Oncology Institute—Porto (PI 74-CI-IPOP-19-2016) and the Portuguese Society of Oncology—YOuR Project. SS is supported by a PhD fellowship IPO/ESTIMA-1 NORTE-01-0145-FEDER-000027. JL is supported by a PhD fellowship from FCT—Fundação para a Ciência e Tecnologia (SFRH/BD/132751/2017).
Conflict of Interest Statement
The authors declare that the research was conducted in the absence of any commercial or financial relationships that could be construed as a potential conflict of interest.
Acknowledgments
The authors would like to acknowledge the IPO Porto’s patients for their generous collaboration in providing the samples used in this study as well as to the Breast Cancer Clinic staff for their assistance.
Supplementary Material
The Supplementary Material for this article can be found online at: https://www.frontiersin.org/articles/10.3389/fgene.2019.00815/full#supplementary-material
References
AlFakeeh, A., Brezden-Masley, C. (2018). Overcoming endocrine resistance in hormone receptor–positive breast cancer. Curr. Oncol. 25 (Suppl 1), S18. doi: 10.3747/co.25.3752
Amorim, M., Salta, S., Henrique, R., Jerónimo, C. (2016). Decoding the usefulness of non-coding RNAs as breast cancer markers. J. Transl. Med. 14 (1), 265. doi: 10.1186/s12967-016-1025-3
Barbano, R., Pasculli, B., Rendina, M., Fontana, A., Fusilli, C., Copetti, M., et al. (2017). Stepwise analysis of MIR9 loci identifies miR-9-5p to be involved in oestrogen regulated pathways in breast cancer patients. Sci. Rep. 7, 45283. doi: 10.1038/srep45283
Bockhorn, J., Dalton, R., Nwachukwu, C., Huang, S., Prat, A., Yee, K., et al. (2013). MicroRNA-30c inhibits human breast tumour chemotherapy resistance by regulating TWF1 and IL-11. Nat. Commun. 4, 1393. doi: 10.1038/ncomms2393
Bray, F., Ferlay, J., Soerjomataram, I., Siegel, R. L., Torre, L. A., Jemal, A. (2018). Global cancer statistics 2018: GLOBOCAN estimates of incidence and mortality worldwide for 36 cancers in 185 countries. CA Cancer J. Clin. 68 (6), 394–424. doi: 10.3322/caac.21492
Breunig, C., Pahl, J., Küblbeck, M., Miller, M., Antonelli, D., Erdem, N., et al. (2017). MicroRNA-519a-3p mediates apoptosis resistance in breast cancer cells and their escape from recognition by natural killer cells. Cell Death Dis. 8 (8), e2973. doi: 10.1038/cddis.2017.364
Burk, U., Schubert, J., Wellner, U., Schmalhofer, O., Vincan, E., Spaderna, S., et al. (2008). A reciprocal repression between ZEB1 and members of the miR-200 family promotes EMT and invasion in cancer cells. EMBO Rep. 9 (6), 582–589. doi: 10.1038/embor.2008.74
Cardoso, F., Costa, A., Senkus, E., Aapro, M., André, F., Barrios, C., et al. (2017). 3rd ESO–ESMO international consensus guidelines for Advanced Breast Cancer (ABC 3). Breast 31, 244–259. doi: 10.1016/j.breast.2016.10.001
Chen, Z., Zhan, Y., Chi, J., Guo, S., Zhong, X., He, A., et al. (2018). Using microRNAs as novel predictors of urologic cancer survival: an integrated analysis. EBioMedicine 34, 94–107. doi: 10.1016/j.ebiom.2018.07.014
Cheng, C.-W., Wang, H.-W., Chang, C.-W., Chu, H.-W., Chen, C.-Y., Yu, J.-C., et al. (2012). MicroRNA-30a inhibits cell migration and invasion by downregulating vimentin expression and is a potential prognostic marker in breast cancer. Breast Cancer Res. Treat. 134 (3), 1081–1093. doi: 10.1007/s10549-012-2034-4
Chiang, C. H., Hou, M. F., Hung, W. C. (2013). Up-regulation of miR-182 by beta-catenin in breast cancer increases tumorigenicity and invasiveness by targeting the matrix metalloproteinase inhibitor RECK. Biochim. Biophys. Acta. 1830 (4), 3067–3076. doi: 10.1016/j.bbagen.2013.01.009
Cittelly, D. M., Das, P. M., Spoelstra, N. S., Edgerton, S. M., Richer, J. K., Thor, A. D., et al. (2010). Downregulation of miR-342 is associated with tamoxifen resistant breast tumors. Mol. Cancer 9 (1), 1. doi: 10.1186/1476-4598-9-317
Croset, M., Pantano, F., Kan, C. W. S., Bonnelye, E., Descotes, F., Alix-Panabieres, C., et al. (2018). miRNA-30 family members inhibit breast cancer invasion, osteomimicry, and bone destruction by directly targeting multiple bone metastasis-associated genes. Cancer Res. 78 (18), 5259–5273. doi: 10.1158/0008-5472.CAN-17-3058
Curigliano, G., Burstein, H. J., Winer, E. P., Gnant, M., Dubsky, P., Loibl, S., et al. (2017). De-escalating and escalating treatments for early-stage breast cancer: The St. Gallen International Expert Consensus Conference on the Primary Therapy of Early Breast Cancer 2017. Ann. Oncol. 28 (8), 1700–1712. doi: 10.1093/annonc/mdx308
D’aiuto, F., Callari, M., Dugo, M., Merlino, G., Musella, V., Miodini, P., et al. (2015). miR-30e* is an independent subtype-specific prognostic marker in breast cancer. Br. J. Cancer 113 (2), 290–298. doi: 10.1038/bjc.2015.206
Eroles, P., Bosch, A., Perez-Fidalgo, J. A., Lluch, A. (2012). Molecular biology in breast cancer: intrinsic subtypes and signaling pathways. Cancer Treat. Rev. 38 (6), 698–707. doi: 10.1016/j.ctrv.2011.11.005
Fitzgibbons, P. L., Bradley, L. A., Fatheree, L. A., Alsabeh, R., Fulton, R. S., Goldsmith, J. D., et al. (2014). Principles of analytic validation of immunohistochemical assays: guideline from the college of american pathologists pathology and laboratory quality center. Arch. Pathol. Lab. Med. 138 (11), 1432–1443. doi: 10.5858/arpa.2013-0610-CP
Gravgaard, K. H., Lyng, M. B., Laenkholm, A. V., Sokilde, R., Nielsen, B. S., Litman, T., et al. (2012). The miRNA-200 family and miRNA-9 exhibit differential expression in primary versus corresponding metastatic tissue in breast cancer. Breast Cancer Res. Treat. 134 (1), 207–217. doi: 10.1007/s10549-012-1969-9
Guarneri, V., Conte, P. F. (2004). The curability of breast cancer and the treatment of advanced disease. Eur. J. Nucl. Med. Mol. Imaging 31 Suppl 1 (1), S149–S161. doi: 10.1007/s00259-004-1538-5
Haque, R., Ahmed, S. A., Inzhakova, G., Shi, J., Avila, C., Polikoff, J., et al. (2012). Impact of breast cancer subtypes and treatment on survival: an analysis spanning two decades. Cancer Epidemiol. Biomarkers Prev. 21 (10), 1848–1855. doi: 10.1158/1055-9965.EPI-12-0474
Hilmarsdottir, B., Briem, E., Bergthorsson, J. T., Magnusson, M. K., Gudjonsson, T. (2014). Functional role of the microRNA-200 family in breast morphogenesis and neoplasia. Genes (Basel) 5 (3), 804–820. doi: 10.3390/genes5030804
Hiscox, S., Jiang, W. G., Obermeier, K., Taylor, K., Morgan, L., Burmi, R., et al. (2006). Tamoxifen resistance in MCF7 cells promotes EMT-like behaviour and involves modulation of beta-catenin phosphorylation. Int. J. Cancer 118 (2), 290–301. doi: 10.1002/ijc.21355
Hong, Y., Liang, H., Uzair Ur, R., Wang, Y., Zhang, W., Zhou, Y., et al. (2016). miR-96 promotes cell proliferation, migration and invasion by targeting PTPN9 in breast cancer. Sci. Rep. 6, 37421. doi: 10.1038/srep37421
Howell, S. J. (2013). Advances in the treatment of luminal breast cancer. Curr. Opin. Obstet. Gynecol. 25 (1), 49–54. doi: 10.1097/GCO.0b013e32835c0410
Hui, A. B., Shi, W., Boutros, P. C., Miller, N., Pintilie, M., Fyles, T., et al. (2009). Robust global micro-RNA profiling with formalin-fixed paraffin-embedded breast cancer tissues. Lab. Invest. 89 (5), 597–606. doi: 10.1038/labinvest.2009.12
Hwang, E. S. (2014). Breast conservation: is the survival better for mastectomy? J. Surg. Oncol. 110 (1), 58–61. doi: 10.1002/jso.23622
Konecny, G., Pauletti, G., Pegram, M., Untch, M., Dandekar, S., Aguilar, Z., et al. (2003). Quantitative association between HER-2/neu and steroid hormone receptors in hormone receptor-positive primary breast cancer. J. Natl. Cancer Inst. 95 (2), 142–153. doi: 10.1093/jnci/95.2.142
Lakhani, S. R. (2012). WHO classification of tumours of the breast. International Agency for Research on Cancer, Lyon.
Li, P., Sheng, C., Huang, L., Zhang, H., Huang, L., Cheng, Z., et al. (2014a). MiR-183/-96/-182 cluster is up-regulated in most breast cancers and increases cell proliferation and migration. Breast Cancer Res. 16 (6), 1. doi: 10.1186/s13058-014-0473-z
Li, X., Roslan, S., Johnstone, C., Wright, J., Bracken, C., Anderson, M., et al. (2014b). MiR-200 can repress breast cancer metastasis through ZEB1-independent but moesin-dependent pathways. Oncogene 33 (31), 4077–4088. doi: 10.1038/onc.2013.370
Livak, K. J., Schmittgen, T. D. (2001). Analysis of relative gene expression data using real-time quantitative PCR and the 2–DDCT method. Methods 25 (4), 402–408. doi: 10.1006/meth.2001.1262
Lowery, A. J., Miller, N., Dwyer, R. M., Kerin, M. J. (2010). Dysregulated miR-183 inhibits migration in breast cancer cells. BMC Cancer 10 (1), 502. doi: 10.1186/1471-2407-10-502
Maillot, G., Lacroix-Triki, M., Pierredon, S., Gratadou, L., Schmidt, S., Benes, V., et al. (2009). Widespread estrogen-dependent repression of micrornas involved in breast tumor cell growth. Cancer Res. 69 (21), 8332–8340. doi: 10.1158/0008-5472.CAN-09-2206
Manavalan, T. T., Teng, Y., Appana, S. N., Datta, S., Kalbfleisch, T. S., Li, Y., et al. (2011). Differential expression of microRNA expression in tamoxifen-sensitive MCF-7 versus tamoxifen-resistant LY2 human breast cancer cells. Cancer Lett. 313 (1), 26–43. doi: 10.1016/j.canlet.2011.08.018
Manavalan, T. T., Teng, Y., Litchfield, L. M., Muluhngwi, P., Al-Rayyan, N., Klinge, C. M. (2013). Reduced expression of miR-200 family members contributes to antiestrogen resistance in LY2 human breast cancer cells. PLoS One 8 (4), e62334. doi: 10.1371/journal.pone.0062334
Moon, Y. W., Park, S., Sohn, J. H., Kang, D. R., Koo, J. S., Park, H. S., et al. (2011). Clinical significance of progesterone receptor and HER2 status in estrogen receptor-positive, operable breast cancer with adjuvant tamoxifen. J. Cancer Res. Clin. Oncol. 137 (7), 1123–1130. doi: 10.1007/s00432-011-0976-2
Muluhngwi, P., Klinge, C. M. (2015). Roles for miRNAs in endocrine resistance in breast cancer. Endocr. Relat. Cancer 22 (5), R279–R300. doi: 10.1530/ERC-15-0355
Muluhngwi, P., Klinge, C. M. (2017). Identification of miRNAs as biomarkers for acquired endocrine resistance in breast cancer. Mol. Cell. Endocrinol. 456, 76–86. doi: 10.1016/j.mce.2017.02.004
Murphy, C. G., Dickler, M. N. (2016). Endocrine resistance in hormone-responsive breast cancer: mechanisms and therapeutic strategies. Endocr. Relat. Cancer 23 (8), R337–R352. doi: 10.1530/ERC-16-0121
Network C.G.A. (2012). Comprehensive molecular portraits of human breast tumours. Nature 490 (7418), 61–70. doi: 10.1038/nature11412
Normanno, N., Di Maio, M., De Maio, E., De Luca, A., de Matteis, A., Giordano, A., et al. (2005). Mechanisms of endocrine resistance and novel therapeutic strategies in breast cancer. Endocr. Relat. Cancer 12 (4), 721–747. doi: 10.1677/erc.1.00857
Oh, D. S., Troester, M. A., Usary, J., Hu, Z., He, X., Fan, C., et al. (2006). Estrogen-regulated genes predict survival in hormone receptor–positive breast cancers. J. Clin. Oncol. 24 (11), 1656–1664. doi: 10.1200/JCO.2005.03.2755
Parker, J. S., Mullins, M., Cheang, M. C., Leung, S., Voduc, D., Vickery, T., et al. (2009). Supervised risk predictor of breast cancer based on intrinsic subtypes. J. Clin. Oncol. 27 (8), 1160–1167. doi: 10.1200/JCO.2008.18.1370
Perou, C. M., Sørlie, T., Eisen, M. B., de Rijn, M., Jeffrey, S. S., Rees, C. A., et al. (2000). Molecular portraits of human breast tumours. Nature 406 (6797), 747–752. doi: 10.1038/35021093
Png, K. J., Yoshida, M., Zhang, X. H.-F., Shu, W., Lee, H., Rimner, A., et al. (2011). MicroRNA-335 inhibits tumor reinitiation and is silenced through genetic and epigenetic mechanisms in human breast cancer. Genes Dev. 25 (3), 226–231. doi: 10.1101/gad.1974211
Rao, X., Di Leva, G., Li, M., Fang, F., Devlin, C., Hartman-Frey, C., et al. (2011). MicroRNA-221/222 confers breast cancer fulvestrant resistance by regulating multiple signaling pathways. Oncogene 30 (9), 1082–1097. doi: 10.1038/onc.2010.487
Rodriguez-Gonzalez, F. G., Sieuwerts, A. M., Smid, M., Look, M. P., Meijer-van Gelder, M. E., de Weerd, V., et al. (2011). MicroRNA-30c expression level is an independent predictor of clinical benefit of endocrine therapy in advanced estrogen receptor positive breast cancer. Breast Cancer Res. Treat. 127 (1), 43–51. doi: 10.1007/s10549-010-0940-x
Sahlberg, K. K., Bottai, G., Naume, B., Burwinkel, B., Calin, G. A., Børresen-Dale, A.-L., et al. (2015). A serum microRNA signature predicts tumor relapse and survival in triple-negative breast cancer patients. Clin. Cancer Res. 21 (5), 1207–1214. doi: 10.1158/1078-0432.CCR-14-2011
Schwarzenbach, H., Nishida, N., Calin, G. A., Pantel, K. (2014). Clinical relevance of circulating cell-free microRNAs in cancer. Nat. Rev. Clin. Oncol. 11 (3), 145–156. doi: 10.1038/nrclinonc.2014.5
Senkus, E., Kyriakides, S., Ohno, S., Penault-Llorca, F., Poortmans, P., Rutgers, E., et al. (2015). Primary breast cancer: ESMO clinical practice guidelines for diagnosis, treatment and follow-up. Ann. Oncol. 26 Suppl 5, v8–30. doi: 10.1093/annonc/mdv298
Shekhar, M. P., Pauley, R., Heppner, G. (2003). Host microenvironment in breast cancer development: extracellular matrix-stromal cell contribution to neoplastic phenotype of epithelial cells in the breast. Breast Cancer Res. 5 (3), 130–135. doi: 10.1186/bcr580
Slamon, D., Eiermann, W., Robert, N., Pienkowski, T., Martin, M., Press, M., et al. (2011). Adjuvant trastuzumab in HER2-positive breast cancer. N. Engl. J. Med. 365 (14), 1273–1283. doi: 10.1056/NEJMoa0910383
Song, C., Zhang, L., Wang, J., Huang, Z., Li, X., Wu, M., et al. (2016). High expression of microRNA-183/182/96 cluster as a prognostic biomarker for breast cancer. Sci. Rep. 6, 24502. doi: 10.1038/srep24502
Song, J., Ouyang, Y., Che, J., Li, X., Zhao, Y., Yang, K., et al. (2017). Potential value of miR-221/222 as diagnostic, prognostic, and therapeutic biomarkers for diseases. Front. Immunol. 8, 56. doi: 10.3389/fimmu.2017.00056
Sørlie, T. (2004). Molecular portraits of breast cancer: tumour subtypes as distinct disease entities. Eur. J. Cancer 40 (18), 2667–2675. doi: 10.1016/j.ejca.2004.08.021
Sørlie, T., Perou, C. M., Tibshirani, R., Aas, T., Geisler, S., Johnsen, H., et al. (2001). Gene expression patterns of breast carcinomas distinguish tumor subclasses with clinical implications. Proc. Natl. Acad. Sci. 98 (19), 10869–10874. doi: 10.1073/pnas.191367098
Thiantanawat, A., Long, B. J., Brodie, A. M. (2003). Signaling pathways of apoptosis activated by aromatase inhibitors and antiestrogens. Cancer Res. 63 (22), 8037–8050.
Vesuna, F., Lisok, A., Kimble, B., Domek, J., Kato, Y., van der Groep, P., et al. (2012). Twist contributes to hormone resistance in breast cancer by downregulating estrogen receptor-alpha. Oncogene 31 (27), 3223–3234. doi: 10.1038/onc.2011.483
Ward, A., Shukla, K., Balwierz, A., Soons, Z., König, R., Sahin, Ö., et al. (2014). MicroRNA-519a is a novel oncomir conferring tamoxifen resistance by targeting a network of tumour-suppressor genes in ER+ breast cancer. J. Pathol. 233 (4), 368–379. doi: 10.1002/path.4363
Wei, Y., Lai, X., Yu, S., Chen, S., Ma, Y., Zhang, Y., et al. (2014). Exosomal miR-221/222 enhances tamoxifen resistance in recipient ER-positive breast cancer cells. Breast Cancer Res. Treat. 147 (2), 423–431. doi: 10.1007/s10549-014-3037-0
Yang, S. J., Yang, S. Y., Wang, D. D., Chen, X., Shen, H. Y., Zhang, X. H., et al. (2017). The miR-30 family: versatile players in breast cancer. Tumour Biol. 39 (3), 1–13. doi: 10.1177/1010428317692204
Yao, Y., Hu, J., Shen, Z., Yao, R., Liu, S., Li, Y., et al. (2015). MiR-200b expression in breast cancer: a prognostic marker and act on cell proliferation and apoptosis by targeting Sp1. J. Cell. Mol. Med. 19 (4), 760–769. doi: 10.1111/jcmm.12432
Ye, F., Tang, H., Liu, Q., Xie, X., Wu, M., Liu, X., et al. (2014). miR-200b as a prognostic factor in breast cancer targets multiple members of RAB family. J. Transl. Med. 12 (1), 17. doi: 10.1186/1479-5876-12-17
Zhan, Y., Li, X., Liang, X., Li, L., Cao, B., Wang, B., et al. (2017). MicroRNA-182 drives colonization and macroscopic metastasis via targeting its suppressor SNAI1 in breast cancer. Oncotarget 8 (3), 4629. doi: 10.18632/oncotarget.13542
Zhang, H., Fan, Q. (2015). MicroRNA-205 inhibits the proliferation and invasion of breast cancer by regulating AMOT expression. Oncol. Rep. 34 (4), 2163–2170. doi: 10.3892/or.2015.4148
Zhang, M. H., Man, H. T., Zhao, X. D., Dong, N., Ma, S. L. (2014a). Estrogen receptor-positive breast cancer molecular signatures and therapeutic potentials (Review). Biomed. Rep. 2 (1), 41–52. doi: 10.3892/br.2013.187
Zhang, N., Wang, X., Huo, Q., Sun, M., Cai, C., Liu, Z., et al. (2014b). MicroRNA-30a suppresses breast tumor growth and metastasis by targeting metadherin. Oncogene 33 (24), 3119–3128. doi: 10.1038/onc.2013.286
Keywords: Breast cancer, luminal subtype, endocrine therapy, endocrine resistance, biomarkers, microRNAs
Citation: Amorim M, Lobo J, Fontes-Sousa M, Estevão-Pereira H, Salta S, Lopes P, Coimbra N, Antunes L, Palma de Sousa S, Henrique R and Jerónimo C (2019) Predictive and Prognostic Value of Selected MicroRNAs in Luminal Breast Cancer. Front. Genet. 10:815. doi: 10.3389/fgene.2019.00815
Received: 16 January 2019; Accepted: 07 August 2019;
Published: 11 September 2019.
Edited by:
Michael E. Symonds, University of Nottingham, United KingdomReviewed by:
Nejat Dalay, Istanbul University, TurkeyMustafa Ozen, Baylor College of Medicine, United States
Copyright © 2019 Amorim, Lobo, Fontes-Sousa, Estevão-Pereira, Salta, Lopes, Coimbra, Antunes, Palma de Sousa, Henrique and Jerónimo. This is an open-access article distributed under the terms of the Creative Commons Attribution License (CC BY). The use, distribution or reproduction in other forums is permitted, provided the original author(s) and the copyright owner(s) are credited and that the original publication in this journal is cited, in accordance with accepted academic practice. No use, distribution or reproduction is permitted which does not comply with these terms.
*Correspondence: Carmen Jerónimo, carmenjeronimo@ipoporto.min-saude.pt; , cljeronimo@icbas.up.pt
†These authors share second authorship
‡These authors share senior authorship