- 1Department of Surgical Oncology, The First Affiliated Hospital of Xi’an Jiaotong University, Xi’an, China
- 2Department of Surgery, University of Virginia, Charlottesville, VA, United States
Gamma delta (γδ) T cells can effectively recognize and kill colorectal cancer (CRC) cells, thereby suppressing tumor progression via multiple mechanisms. They also have abilities to exert a protumor effect via secreting interleukin-17 (IL-17). γδ T cells have been selected as potential immunocytes for antitumor treatment because of their significant cytotoxic activity. Immunotherapy is another potential anti-CRC strategy after an operation, chemotherapy, and radiotherapy. γδ T cell-based immunotherapy for CRC shows fewer side effects and better toleration. This review will outline the immune functions and the mechanisms of γδ T cells in the growth and progression of CRC in recent years, and summarize the immunotherapies based on γδ T cells, thus providing a direction for future γδ T cells in CRC research.
Introduction
Colorectal cancer (CRC) is one of the most common gastrointestinal malignant tumors in the world, with a high therapeutic need. Globally, CRC is the third most frequently diagnosed cancer and the second leading cause of death from tumor diseases (1). The incidence and mortality of CRC are rising rapidly in many low- and middle-income countries (2). In some high-income countries, although the overall incidence and mortality of CRC has declined or stabilized (the decline of CRC incidence and mortality in the older age group is partly due to the implementation of CRC screening), the CRC incidence and mortality in individuals under 50 has increased significantly (3, 4). Presently, surgery remains the primary strategy to treat advanced CRC. Even though advances in screening, diagnosis, and treatment have improved the prognosis of CRC in some countries, there are still many people (about 25%) that are diagnosed with advanced CRC, among which almost 50% develop metastases (5, 6). Only a few patients diagnosed with advanced CRC can receive radical surgeries (7), and resection of resectable liver and lung metastases offers 20–45% 5-year survival rates in carefully selected individuals (6). The overall efficacy of surgery and adjuvant therapy in these individuals is not satisfactory. It has been shown that most patients (71.2%) with advanced CRC develop recurrence within the first 2 years after surgery and the 5-year overall survival rate of these patients was 34.7% (8). It is becoming more evident that the components of the tumor microenvironment (TME) play critical roles in the occurrence, development, and prognosis of CRC (9, 10). For example, NK cells (one of the immunocyte subsets in TME) that can combine HLA class I molecules are able to recognize and eliminate CRC cells with aberrant HLA class I expression (11). Cytokines, like IL-17, have abilities to perpetuate CRC progression via promoting angiogenesis and the production of myeloid-derived suppressor cells (MDSCs) (12). Moreover, a meta-analysis showed a statistically significant inverse relationship between intratumoral vessel density (a surrogate marker of tumoral angiogenesis) or vascular endothelial growth factor (one of the proangiogenic factors in TME) expression and overall survival in CRC (13). Therefore, it is necessary to discover a novel reasonable treatment strategy based on the understanding of the TME.
Gamma delta (γδ) T cells are one small population of the TME and a small subset of peripheral blood T lymphocytes, which express heterodimeric receptor composed of γ and δ chains on the cell surface. Most γδ T cells are CD4– or CD8– T cells, while αβ T cells express CD4+ or CD8+ molecules. γδ T cells are mainly distributed in subcutaneous tissue, the mucosa of the intestinal tract, respiratory tract, and urogenital tract. They are involved in the composition of intestinal intraepithelial lymphocytes. Since γδ T cells recognize infected and transformed cells rapidly, they are recognized as the first line of defense against infections and malignant tumors (14). γδ T cells have pleiotropic biological effects, including exerting cytotoxicity to kill tumor cells, involved in immune regulation, presenting antigen, inducing dendritic cells (DCs) maturation, and so on (15, 16). Moreover, γδ T cells are capable of infiltrating into various tumor tissues, like rectal cancer, breast cancer, or pancreatic cancer (17–19). In addition, they have abilities to recognize different tumor cells in a major histocompatibility complex (MHC)-unrestricted manner and lyse cancer cells by producing chemokines and cytokines, or by direct contact with cancer cells through the death receptor signal (19, 20), which suggests that γδ T cells may have a potential role in antitumor immunotherapy. However, accumulating evidence suggests that γδ T cells also play a protumor role mainly by expressing interleukin-17 (IL-17) in several cancers (17, 21–26).
Reviews published in the past few years mainly discuss the functions of γδ T cells and their immunotherapeutic potential against cancer in general. However, only a few foci were placed on the immune effects and immune treatment strategies of γδ T cells against CRC. In this review, we will summarize recent advances in the role of γδ T cells in CRC immunity, as well as γδ T cells-based immunotherapies against CRC.
γδ T Cells
Human γδ T cells are classified into three subtypes based on the expression of δ chains: (1) Vδ1 T cells are enriched in the thymus and mucosal epithelial tissues. They produce a variety of cytokines like TNF-α and IFN-γ, and lyse infected or transformed target cells by cytotoxicity (20, 27). (2) Vδ2 T cells, which exert cytotoxicity in tumor immunoregulation and viral infection, are mainly distributed in peripheral blood (28). T cell receptors (TCRs) expressed on the surface of Vδ2 T cells are able to recognize phosphoantigens produced by malignant cells, thereby activating Vδ2 T cells to release perforins, granzymes, and IFN-γ (20, 29). MDSCs may inhibit the production of IFN-γ and degranulation in phosphoantigens-activated Vδ2T cells (30). (3) Vδ3 T cells are mainly distributed in the liver tissue and rarely in the peripheral blood, which can directly kill the target cells and secrete cytokines. In mice, γδ T cells are categorized into two subtypes based on what cytokines they release. Murine IFN-γγδ T cells express the CD27 molecule (which is a member of the TNF receptor family and can bind to CD70), whereas IL-17 γδ T cells cannot display CD27 (31). In addition to mice, IL-17 γδ T cells are also found in humans (32–34). Although the production of IL-17 by human γδ T cells is rare, it is involved in the initiation and progression of CRC by producing growth factors (12, 32). Pin Wu et al. previously reported that tumor-infiltrating IL-17 γδ T cells might be a key player in human CRC progression and metastasis (32). It is also reported that IL-17 perpetuate CRC progression via promoting angiogenesis and the IL-17/IL-23 pathway plays a critical role in pathogenesis of CRC (IL-23 is a key modulator of IL-17 γδ T cells responses) (12) (Figure 1).
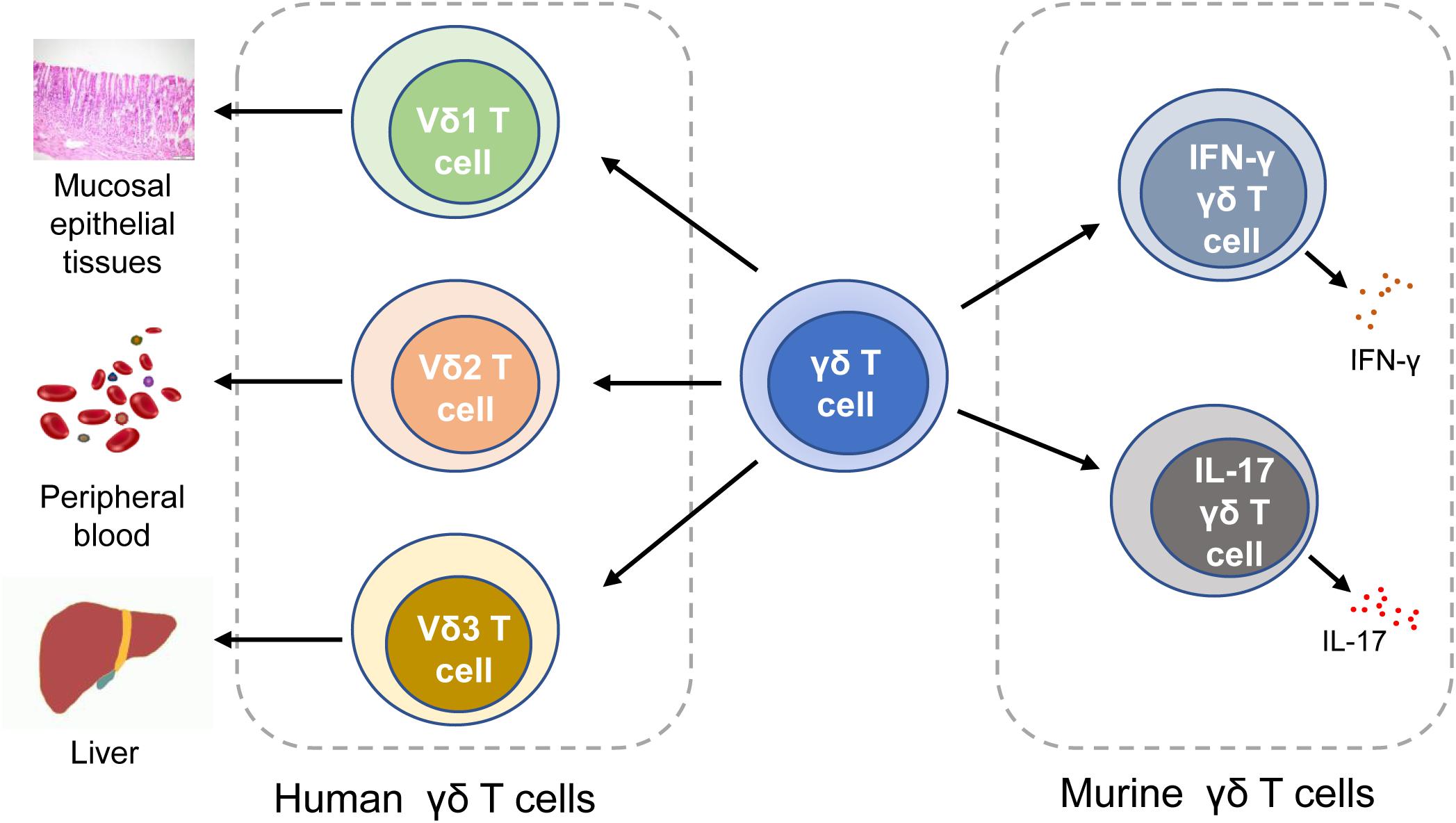
Figure 1. Classification of γδ T cells. In human, γδ T cells can be classified into Vδ1 T cells, Vδ1 T cells, and Vδ3 T cells. In mice, γδ T cells can be categorized into IFN-γγδ T cells and IL-17 γδ T cells. IFN-γ, interferon-γ.
Vδ2 T Cells
Vδ2 T cells are the most abundant subset of γδ T cells, accounting for 50 to 90% of the total number of γδ T cells. Among Vδ2 T cells, Vγ9Vδ2 T cells that co-express Vδ2 and Vγ9 chains are the most abundant subtype. Vγ9Vδ2 T cells elicit robust inhibitory effects on the tumorigenesis and growth of different tumors (35–37). Murielle Corvaisier et al. (38) found that colon tumor is frequently infiltrated by Vγ9Vδ2 T lymphocytes. Moreover, a recent analysis of expression signatures from 39 different malignancies, including CRC, revealed that tumor-infiltrating γδ T cell is one of the most significantly favorable cancer-wide prognostic immunocytes (39). Later, Liang Rong et al. (17) used the Application of Fluorescent-activated Cell Sorting (FASC) to analyze the percentage of γδ T cells (including Vδ1 T cells and Vδ2 T cells) in tumor tissues, para-carcinoma tissues and peripheral blood from 20 rectal cancer patients. The results showed that although the percentage of Vδ2 T cells in tumor tissues was negatively correlated with the T staging, the tumor-infiltrating Vδ2 T cells showed robust cytolytic activity. Recently, an assessment of tumor-infiltrating Vγ9Vδ2 T cell frequency by deconvolution of human cancers microarrays showed that CRC patients with high infiltration of Vγ9Vδ2 T cells had higher overall survival rates (40). These findings indicate that Vδ2 T cells are closely related to the progression and prognosis of tumor patients.
Circulating Vδ2 T cells that are stimulated by microbial phosphoantigens and IL-2 would express gut-homing integrin α4β7 (molecules that mediate Vδ2 T cells trafficking to the human intestinal mucosa) and widely populate the mucosa of the human intestinal tract (41). Moreover, Vδ2 T cells can respond and proliferate rapidly, following stimulation with microbial phosphoantigens, and produce proinflammatory cytokines such as IFN-γ and TNF-α, thus contributing to mucosal immune responses (41). Aminobisphosphonates (42) (zoledronate and pamidronate) induce isopentenyl pyrophosphate (IPP) accumulation in tumor cells, thus activating and amplifying Vγ9Vδ2 T cells (43). Vγ9Vδ2 T cells that were stimulated by zoledronate and exposed to colon cancer stem cells are induced to proliferate and secrete cytokines (TNF-α and IFN-γ), cytotoxic and apoptotic molecules [TNF-related apoptosis-inducing ligand (TRAIL) and BLT esterase], resulting in the capability of killing and lysing colon tumor cells (20). And the cytotoxicity of Vγ9Vδ2 T cells in colon cancer is mainly mediated by the granule exocytosis pathway of effector substances (cytokines and granzymes). Moreover, Maria Raffaella Zocchi et al. (44) found that the local TME of CRC stimulated by zoledronate expresses BTN3A1/CD277 (BTN3A1 can bind to phosphoantigens and drive the activation of Vγ9Vδ2 T cells through conformational changes of the extracellular domains) to stimulate and expand effector Vγ9Vδ2 T cells that carry antitumor activity. Therefore, Vδ2 T cells, especially Vγ9Vδ2 T cells, have the potential to be used in immunotherapy against CRC, and zoledronate serves a stimulator in antitumor immunotherapy to activate and expand γδ T cells.
Vδ1 T Cells
Compared with Vδ2 T cells, Vδ1 T cells account for a small proportion of γδ T cells. They are mainly distributed on the surface of the mucosa. Although a large number of studies about the antitumor effects of γδ T cells mainly focus on Vγ9Vδ2 T cell subset, it is becoming more evident that Vδ1 T cells also play a critical role in progression of hematological malignancies and some epithelial-derived solid tumors (including CRC) (45–47).
A study showed that cytomegalovirus-induced Vδ1 T cells could inhibit not only the primary colon tumor growth but also the emergence of metastases (48). After that, Wu, Dang et al. (47) found that the freshly isolated human peripheral blood Vδ1 T cells, especially the Vδ1 T cells expanded ex vivo, had better cytotoxicity to adherent and sphere-forming colon tumor cells than Vδ2 T cells. The antitumor effect for colon cancer mediated by Vδ1 T cells could be achieved not only by the secretion of cytokines (CD107a, perforin, granzyme B), but also by the direct contact between cells and the cytotoxicity-related receptors and ligands (Fas, death receptor 4/5, MICA/B, and ICAM-1) (47). The findings also showed that adoptive transferred Vδ1 T cells that were expanded by PHA and IL-7 could significantly inhibit tumor growth and prolong survival of colon tumor-bearing mice (47). Also, by comparing the characteristics of γδ T cells in CRC, normal colon tissue, and normal peripheral blood, Meraviglia, S. et al. (49) found that there were 4% of γδ T cells in tumor-infiltrating lymphocytes, and Vδ1 T cells were the dominant γδ T cell subtype in CRC. Comparing to the adjacent normal colon tissues in CRC patients, Vδ1 T cells from tumor tissues produced significantly less IFN-γ, which was likely due to the presence of some identified inhibitory molecules by colon tumor stem cells. In addition, another recent study showed that the human gut-resident intraepithelial Vδ1 T cell subset, that constitutively expressed NKp46, exhibited high antitumor activity against CRC, and the expression of NKp46 on intestinal intraepithelial lymphocytes was associated with high cytolytic potential. The data of this study also showed that lower frequencies of NKp46pos/Vδ1 IELs in tumor-free tissues from CRC patients was associated with a higher risk of metastasis (50). In conclusion, the findings of these studies demonstrate that Vδ1 T cells are involved in the progression of CRC and pave the way for utilizing Vδ1 T cells in anti-CRC immunotherapy.
Immune Functions of γδ T Cells
General Functions of γδ T Cells in Cancer
Tumor immunosurveillance is a critical part of immunosurveillance. As a key player in tumor immune surveillance, γδ T cells are able to perceive the changes of the antigens on the malignant cells. The mevalonate biosynthetic pathway is a metabolic pathway of synthetic IPP and dimethylallylpyrophosphate (DMAPP) in general cells. When this pathway in tumor cells was inhibited, it could lead to the accumulation of IPP, thereby activating γδ T cells to play an antitumor role. A recent study focused on CRC has shown that zoledronate, which can suppress the mevalonate biosynthetic pathway, plays a key role in the activation of γδ T cells (44). It is reported that phosphoantigens activate γδ T cells in a TCRs-dependent manner (51), but the concrete mechanism of recognition and activation is still not clear. Furthermore, studies have shown that γδ T cells not only have antitumor effects but also protumor effects, which are mainly mediated by IL-17 (17, 23) (Figure 2).
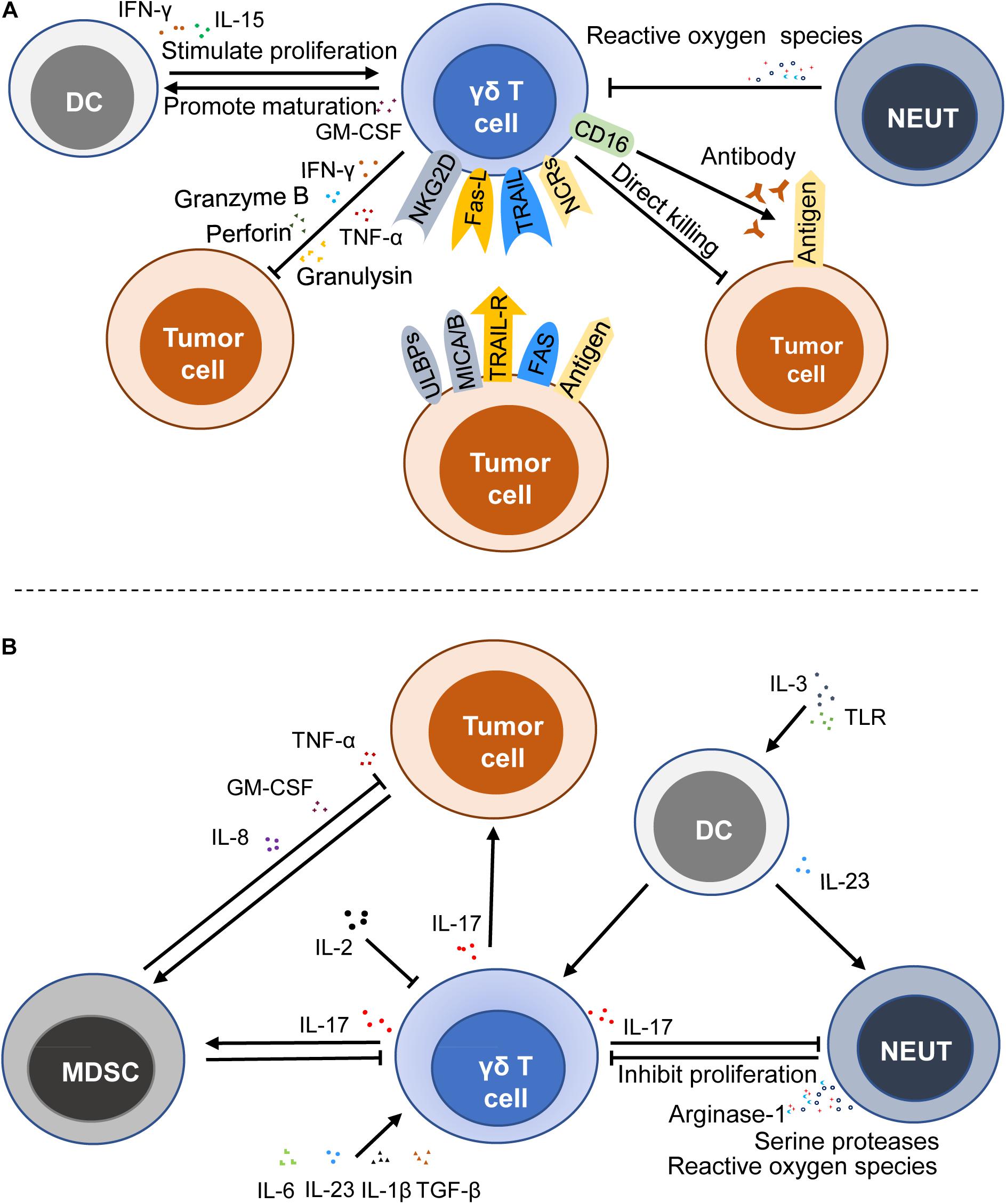
Figure 2. Immune functions of γδ T cells in cancers. γδ T cells can exert an antitumor effect by a direct killing effect, death receptor signal pathway, ADCC, and secreting cytokines and chemokines (A), whereas γδ T cells can stimulate and promote the growth and progression of cancer cells by producing IL-17 (B). MDSC, myeloid-derived suppressor cell; DC, dendritic cell; NEUT, neutrophil; NCRs, NK cell receptors; ULBPs, UL16-binding proteins; NKG2D, natural killer group 2, member D; MICA/B, MHC Class I-related sequence A and B; TRAIL, TNF-related apoptosis-inducing ligand; TRAIL-R, TNF-related apoptosis-inducing ligand-receptor; Fas-L, fas ligand; ADCC, antibody-dependent cytotoxicity; GM-CSF, granulocyte-macrophage colony stimulating factor; TLR, toll-like receptor; TNF-α, tumor necrosis factor-α.
Antitumor Effects and the Mechanism
Previous studies have shown that accumulation of IPP and DMAPP in cancer cells induces abnormal expression of MHC Class I polypeptide-related sequence A and/or B (MICA/MICB) and UL16-binding proteins (ULBPs), thus activating γδ T cells by binding MICA/MICB and ULBPs to NKG2D (a lectin-type activating receptor, which is expressed on the surface of the most NK and NKT cells) (52–54). It is reported that the activation of the cytolytic response of human γδ T cells is associated with the secretion of perforin and granzyme B, which is mediated by NKG2D (55, 56). Besides, Simoes André E. et al. reviewed that γδ T cells also express NK cell receptors (NCRs) like NKp30, NKp44, and NKp46, showing NK cell-like effects to recognize tumor antigens (50, 57). A recent study also demonstrated that the NKp46 expression on γδ intestinal epithelial lymphocytes was associated with high antitumor activity against CRC (50). Since γδ T cells express NCRs and have both T cell characteristics and NK cell characteristics, they are regarded as a bridge between innate immunity and adaptive immunity.
In addition to the recognition and activation mediated by TCRs and NCRs, γδ T cells can be activated by TRAIL. TRAIL receptors often overexpressed and usually located in the endochylema and cell nucleus of tumor cells. Doaa Tawfik et al. (19) found that the knockout of TRAIL-R4 could reduce the sensitivity of cancer cells to cytotoxicity through up-regulation of COX-1 and COX-2 as well as the production of PGE2 that could attenuate the toxic activity and proliferation of γδ T cells. Another death receptor signal pathway is mediated by Fas ligand (FasL) produced by γδ T cells, which induce the killing of target cells via the binding with the Fas receptor (22, 58). Antibody-dependent cytotoxicity (ADCC) is also a significant death-inducing mechanism. Vδ2 T cells that express FcγRIIIA (CD16) could kill colon carcinoma through ADCC (51). When tumor antigens are bound to the corresponding antibody, the Fc segment of the antibody will combine with the receptor on the surface of γδ T cells, thereby killing target tumor cells. Moreover, it is reported that IL-15, programmed death receptor-1 (PD-1), and CD19-specific triple body SPM-1 can enhance the ADCC of γδ T cells (59–61). Apart from these, γδ T cells show a direct killing effect on transformed cells by producing perforin, granzymes, and granulysin.
In addition to the above-mentioned cytokines and the corresponding receptors that are able to affect the antitumor activity of γδ T cells, some immunocytes also affect their antitumor immune activity. A recent study has shown that the levels of cytotoxicity-related markers (CD16) and co-stimulatory molecules (CD80 and CD86) are higher when γδ T cells are exposed to IL-15 DCs (16). And IL-15 DCs could stimulate the proliferation of the γδ T cells by inducing the production of soluble IL-15 and IFN-γ and the contact-independent mechanism in the leukemia environment, thus promoting the antitumor activity (16). Activated γδ T cells also promote the maturation of DCs (62). Moreover, the killing capabilities of freshly isolated resting human γδ T cells on ductal pancreatic adenocarcinoma cells was reduced when neutrophils were present, and it was more obvious when neutrophils were activated by zoledronate (63). Although the interaction between these immunocytes and γδ T cells has not been demonstrated in the current CRC studies, many studies have clearly shown that these cells can interact with each other. Therefore, further investigation into the interaction between these cells in CRC is warranted (Figure 2A).
Protumor Effects and the Mechanism
IL-17 is an inflammatory cytokine that is mainly produced by activated T cells and can mediate inflammation. In humans, IL-17 is believed to promote angiogenesis and tumor growth by recruiting MDSCs (26, 32, 64) (MDSCs can further promote tumor growth after being attracted and activated by tumor cells). IL-17 producing γδ T cells are recognized as a critical source of IL-17 and play an important role in the tumorigenesis of many cancer types (32–34, 49). IL-17 produced by γδ T cells drives the occurrence and progression of the tumor through several downstream effects on tumor cells, endothelia, and other immunocytes.
Some cytokines, like IL-1β and IL-23, could induce the production of IL-17 by γδ T cells (65). Microbial products are likely to trigger IL-23, which is mainly produced by tumor-associated myeloid cells, to facilitate the tumoral IL-17 response, thereby promoting tumor growth and progression (66). IL-6 and TGF-β promote the production of IL-17 mediated by C5a (26, 67). In addition, the absence of IL-2 can result in higher IL-17 production in IL-2-deficient mice, and IL-2 is able to down-regulate IL-7R to negatively impact the survival of γδ T17 cells (68).
Indeed, the tumor promotion by IL-17 γδ T cells is also regulated by neutrophils, DCs, and other cells. Neutrophils interact with IL-17 γδ T cells and play a critical role in regulating TME. Pioneering work has demonstrated that tumor-infiltrating neutrophils inhibit the proliferation of γδ17 T cells through inducing oxidative stress and restraining the production of IL-17 in TME (69). Hans-Heinrich Oberg et al. (70) noted that neutrophils activated by zoledronate inhibited the γδ T cell proliferation due to not only the production of reactive oxygen species but also the expression of arginase-1 and serine proteases. However, their subsequent study showed that neutrophils co-cultured with γδ T cells could enhance the killing ability of activated IL-17 γδ T cells in the presence of zoledronate, which might be attributed to the fact that direct TCRs-dependent activation enhanced the cytotoxic activity and cytokine/granzyme B production of γδ T cells (63). The direct TCRs-dependent activation is mediated by γδ T cell-specific pyrophosphate antigens or bispecific antibodies. In turn, the activity of neutrophils is influenced by the production of IL-17 and other substances. These studies indicate that the interaction of neutrophils and T cells perhaps depend on the local microenvironment of the tumor. Therefore, further studies are needed to explore the specific factors that can affect the interaction between neutrophils and γδ T cells. In other diseases, it has been proved that IL-23 induced γδ T cells to produce IL-17 and DCs secreting IL-23 could promote the infiltration of neutrophils in stress sites (71). Later, Lo Presti, E. et al. (72) reported that plasmacytoid DCs stimulated by the Toll-like receptor (TLR) and activated by IL-3 could induce the proliferation of Vγ9Vδ2 T cells and selectively induce the production of IL-17 when they were co-cultured with Vγ9Vδ2 T cells. However, there are few reports about the interaction between IL-17 γδ T cells and DCs in CRC, which need to be further investigated in the future (Figure 2B).
Functions of γδ T Cells in CRC
Antitumor Effects
γδ T cells are suggested to inhibit the formation and progression of colorectal adenocarcinoma (73). Tumor-derived γδ T cells that were stimulated by CD3 monoclonal antibodies could produce a lot of IFN-γ and showed strong cytotoxic activities to autologous and allogenic gastrointestinal tumor cells (74). A recent study showed that Phloretin could enhance the cytolytic effects of γδ T cells on colon cancer cells by facilitating the proliferation of IFN-γ producing γδ T cells, and the underlying mechanism might be associated with the increased expression of PFP, GraB, and CD107a as well as the activation of the Wnt signaling pathway (75). Moreover, Vδ2 T cells that expressed FcγRIIIA (CD16) could kill colon carcinoma cells via ADCC (51).
A series of ex vivo expansion experiments have shown that γδ T cells stimulated by phosphoantigens have robust killing capabilities on CRC (20, 44, 45, 50, 51). Human γδ T cells stimulated by zoledronate produced higher IFN-γ, TNF-α, granzymes, and TRAIL, thus enhancing the killing effect on the colon cancer stem cells, which was mediated by the granule exocytosis pathway and was related to the expression of isoprenoid by tumor cells (20). TCRs expressed on γδ T cells are able to mediate colon cancer stem cell recognition and killing, whereas NKG2D play a role only when tumor targets express several NKG2D ligands (20). Moreover, a recent study suggested that the high cytolytic potential and the production of IFN-γ were relevant to the NKp46 expression on γδ intestinal epithelial lymphocytes, which was associated with the high antitumor activity against CRC (50). Tumor-infiltrating γδ T cells (Vδ 1 and Vδ 2 T cells) expanded ex vivo exert strong inhibitory effects and killing activity to rectal cancer cells (17). Furthermore, zoledronate could induce the CRC microenvironment expressing BTN31 to produce effector γδ T cells with anti-CRC activity (44). Altogether, γδ T cells are characterized as effector cells with tumor-killing abilities.
Protumor Effects
A recent study has shown that the upregulation of IL-17 is correlated with the colorectal tumorigenesis (66), suggesting that if the expression of IL-17 decreases, the occurrence and development of CRC will be inhibited. Indeed, several studies have confirmed that inhibition of IL-17 suppresses the occurrence of colitis, colonic dysplasia, and colon cancer (64, 76). For example, research by Mahesh Kathania et al. (24) has shown that the ubiquitin ligase Itch can restrain the expression of IL-17 by inhibiting or deactivating ROR-γt ubiquitination, therefore protecting against colitis-associated colon cancer.
In 2014, Pin Wu et al. (32) were the first to report the role of IL-17 γδ T cells in promoting human CRC and demonstrated that γδ T cells are the main source of IL-17 in human CRC. They found that disruption of CRC epithelial barrier resulted in the accumulation and activation of inflammatory DCs, and triggered γδ T17 cells polarization as well as production of IL-17, IL-8, TNF-α, and GM-CSF in γδ T cells, which promoted the recruitment and proliferation of MDSCs, and finally inhibited inflammation triggered by CRC and promoted tumorigenesis (32) (Figure 2). Another research team assessed the secretion of IL-17, IFN-γ, and TNF-α by CRC-infiltrating γδ T cells stimulated by ionomycin and PMA in vitro, then used three different FACS gating strategies. Surprisingly, they confirmed that the majority of CD45+ IL-17+ cells both in CRC and in adjacent non-tumor colon tissues were CD3+ cells but not γδ T cells that preferentially produced IFN-γ in CRC and adjacent normal tissues (49). Moreover, the production of IFN-γ by γδ T cells (Vδ 1 and Vδ 2 T cells) was significantly reduced in CRC tissues compared with adjacent normal tissues. The results of this study showed that compared with normal colon tissue and blood, tumor-infiltrating γδ T cells had reduced the capacities to produce IFN-γ but did not produce IL-17 (49). The discrepancy that the results of this study are not consistent with the former study is likely due to some unknown inhibitory components in local TME.
Functions of γδ T Cells in IBD and CAC
Inflammatory bowel diseases (IBD) including Crohn’s disease (CD) and ulcerative colitis (UC) are complex chronic inflammatory disorders of unknown origin that could affect the intestinal tract (77). Colitis-associated cancer (CAC) may develop in patients with IBD, which is primarily due to chronic intestinal inflammation (78, 79). It is reported that the cumulative incidence of CRC by colitis duration was 2.5% at 20 years, 7.6% at 30 years, and 10.8% at 40 years (78). As the population of patients with IBD grows older, there is an increasing risk of CAC development (80). However, the underlying mechanisms of initiation and development of IBD and how chronic inflammation in IBD leads to CAC development remain unclear. It is well recognized that multiple components of the immune system are involved in the pathogenesis of IBD and CAC (81). γδ T cells, as a critical component of the immune system, are mainly distributed in the mucosa of the intestinal epithelium and regarded as the first line of defense against pathogens, and may play a significant role in the pathogenesis of IBD and CAC (82).
Both human Vδ 1 T cells and Vδ 2 T cells are able to exert an immunoregulatory function and contribute to the pathophysiology of IBD (83–85). Initial studies demonstrated that the number of Vδ 1 T cells were increased in the inflammatory tissue of IBD patients, and Vδ 1 T cells were a major source of IFN-γ (86), suggesting that Vδ 1 T cells played a role in controlling IBD. Moreover, IL-15 produced by epithelial cells plays a key role in γδ T cells, regulating mucosal inflammation in the mouse colon (83). Later, a study showed that the frequency of Vδ 1 T cells in tissue from IBD patients was decreased while Vδ2 T cells were increased in the gut of IBD patients and contributed to TNF-α production (84). Two similar studies also showed that Vδ 2 T cells were the dominant tissue-infiltrating γδ T cells in chronic inflamed IBD, whereas Vδ 1 T cells presented more in healthy colon tissue (85, 87). The tissue-infiltrating Vδ 2 T cells in IBD produced amounts of IFN-γ and TNF-α. Moreover, Lo Presti, E. et al. found that low percentages of Vδ1 and Vδ2 T cells were infiltrated in CAC tissue, but the levels of IFN-γ, TNF-α and IL-17 produced by Vδ2 T cells were increased (85, 87). Transcriptomes also showed a clusterization of gene expression found in IBD patients, which was related to the induction and the maintenance of the inflammatory status (85). The gene expression profile revealed that patients with sustained IBD had an overexpression of the pro-inflammatory cytokine genes (87). These findings demonstrated that Vδ 2 T cells were related to the IBD and CAC pathogenesis and played a protective role in both diseases. The different findings of early studies and later studies, is probably due to the limitation of sample size and the difference of experimental materials as well as methods. Further studies are needed to determine the functions of different γδ T cell subpopulations in the pathophysiology of human IBD. In addition to Vδ 1 T cells and Vδ 2 T cells, Kadivar et al. have defined and characterized a novel subtype of human CD8αβ+ γδ T cells that were enriched in the intestinal tract of patients with active IBD and produced cytotoxic mediators, such as IFN-γ and TNF-α, suggesting a role in IBD (88).
CRC Immunotherapy Based on γδ T Cell
Immunotherapy is another important strategy of antitumor therapy after surgery and adjuvant therapies, which has shown unprecedented success in clinical practice. Application of programmed cell death protein-1/programmed cell death-ligand 1 (PD-1/PD-L1) and chimeric antigen receptor-T (CAR-T) cells, have completely changed the treatment landscape of many different malignancies like leukemia, melanoma and so on (59, 89, 90). However, in solid tumors like CRC, the role of immunotherapy based on PD-1/PD-L1 and CAR-T cells is limited. By contrast, immunotherapy based on innate immunocytes like γδ T cells shows antitumor activity with fewer observed side effects (91, 92). The reaction of γδ T cells to recognize tumor antigens in the MHC-unrestricted manner is rapid, which makes them a key player in immune surveillance. Moreover, γδ T cells could be amplified easily either in vivo or ex vivo. Studies also reported that the prognosis of cancer patients is related to the percentage of γδ T cells (39, 49). Therefore, it is a feasible antitumor strategy to use γδ T cells against CRC.
Treatment Approaches
Because tumor phosphoantigens are recognized and activated by TCRs of γδ T cells in an MHC-unrestricted manner (29), phosphoantigens are usually used to stimulate γδ T cells ex vivo and in vivo. Chemosynthetic phosphoantigens (like bromohydrin pyrophosphate, BrHPP) and aminobisphosphonates (like pamidronate and zoledronate) are able to activate and enhance the cytotoxicity of Vγ9Vδ2 T cells by blocking the mevalonate pathway and promoting the intracellular accumulation of IPP (43). In addition to the stimulation of phosphoantigens, the proliferation of γδ T cells depends on the existence of IL-2. IL-2 is a type of cell growth factor in the immune system and plays an important role in anti-infection and antitumor by: (1) regulating the activity of lymphocytes in peripheral blood; (2) promoting the proliferation of activated T cells; (3) inducing the cytotoxicity of NK cells and cytotoxic T lymphocytes; and (4) enhancing the ADCC against malignant cells via stimulating the expression of CD56, IL-2Rα and TNF receptors. Adoptive immunotherapy based on IL-2 has been used in different tumors, such as melanoma, kidney cancer, hepatic carcinoma, and CRC. In addition, early studies have shown that the use of low-dose IL-2 can reduce the recurrence of patients with hematological malignancies treated by bone marrow transplantation (93). Although a high dose of IL-2 may produce a lot of side effects, the adverse reactions caused by a low dose of IL-2 cannot be ignored. For instance, when a low dose of IL-2 stimulates γδ T cells, it will increase the number of circulating regulatory T cells, thus resulting in strong immunologic suppression. Therefore, an accurate dosage and regimen of IL-2 are required to expand γδ T cells when treating patients with tumors.
There are two strategies to activate and amplify γδ T cells. (1) In vitro expansion: γδ T cells are isolated from the tissues or peripheral blood of the patients with tumors, stimulated with phosphoantigen, anti-TCRγδ antibody (17) or IL-2, expanded to a certain number, and finally reinfused back to patients to play an antitumor role. This immunotherapy strategy is also known as adoptive transfer, which can expand γδ T cells ex vivo to obtain the required number of cells. But this method also has limitations like a high treatment cost and the need for accurate control of the amplification process. (2) In vivo expansion: a process by which the circulating γδ T cells are expanded by intravenous administration of phosphoantigens or aminobisphosphonates in the presence of IL-2, is relatively less expensive. However, clinical trials aimed at the in vivo expansion of γδ T cells in CRC have not been carried out so far.
Studies Based on γδ T Cell Immunotherapy
During the past few years, most of the studies about γδ T cells-based immunotherapies mainly focused on Vγ9Vδ2 T cell subtypes, especially on how to activate them in vivo or ex vivo and how to improve their concentration in peripheral blood. A series of ex vivo studies and clinical trials based on γδ T cells against CRC have also been reported in recent years.
Bouet-Toussaint, F. et al. (94) collected peripheral blood samples from 11 tumor patients (six with hepatocellular carcinoma, four with CRC, one with sarcoma) and 16 healthy people. They then amplified Vγ9Vδ2 T cells ex vivo with a single dose of phosphoantigen (BrHPP or zoledronate) in the presence of exogenous IL-2. Two weeks later, the expanded Vγ9Vδ2 T cells moderately expressed CD16 (an NK marker related to cytotoxic function) and strongly expressed C-type lectin receptor CD161 (NKRP-1A), NKG2D, and CD94 co-receptor. Moreover, autologous Vγ9Vδ2 T cells stimulated by BrHPP or zoledronate showed specific cytolytic activity against HCC and CRC primary cells but not against autologous primary normal cells, which might be related to the NKG2D expression on Vγ9Vδ2 T cells (94). Moreover, the proliferated Vγ9Vδ2 T cells that were stimulated by BrHPP could selectively recognize and lyse HCC and CRC by releasing IFN-γ and TNF-α. Altogether, it is feasible in adoptive immunotherapy for HCC and CRC, that Vγ9Vδ2 T cells can be expanded from peripheral blood mononuclear cells (PBMCs) of patients with advanced cancer and stimulated by phosphoantigens.
Although Vγ9Vδ2 T cells stimulated by phosphoantigens have lytic activity to a variety of human malignant tumors, Cabillic, F. et al. (43) observed that nearly half of tumor patients showed a low proliferative response of Vγ9Vδ2 T cells after the conventional stimulation of phosphoantigens. Subsequently, they co-cultured PBMCs from HCC patients and CRC patients with hepatic metastases (mCRC) with immature DCs stimulated by aminobisphosphonate zoledronate to amplify γδ T cells ex vivo. They found that PBMCs co-cultured with zoledronate-pretreated DCs could induce strong γδ T cell expansion, significantly increased the expression of IPP and IFN-γ, thus enhanced the cytotoxicity of Vγ9Vδ2 T cells. Interestingly, the stimulation of zoledronate-pretreated DCs on Vγ9Vδ2 T cells resulted in higher IFN-γ production than that of BrHPP or zoledronate. The tumor cells pretreated with zoledronate could significantly increase the cytotoxicity of Vγ9Vδ2 T cells against isolated tumor cells from patients. Therefore, Vγ9Vδ2 T cell expansion can be effectively improved through the co-culture of PBMCs with DCs pretreated by zoledronate, indicating that this strategy could be used to improve the efficacy of immunotherapy for HCC and mCRC patients.
The above researches indicated that both phosphoantigens and IL-2 were necessary for the expansion of γδ T cells ex vivo. Some clinical studies about Vγ9Vδ2 T cell-based adoptive transfer therapies also showed that IL-2 was necessary for the proliferation of γδ T cells in vivo (92). However, a recent study showed that adoptively transferred Vγ9Vδ2 T cells could also be amplified well in vivo without the infusion of extrinsic IL-2. The research group of Izumi, T. et al. (42, 95) used zoledronate + IL-2 to prepare a large scale of proliferated Vγ9Vδ2 T cells ex vivo. They applied the expanded Vγ9Vδ2 T cells to phase I study of adoptive immunotherapy for patients with recurrent non-small-cell lung cancer, and the patients showed great tolerance to the cultured Vγ9Vδ2 T cells (95). After that, they conducted a clinical trial to evaluate the efficacy of adoptive immunotherapy based on autologous γδ T cells for patients who received pulmonary metastasectomy of CRC. They identified the characteristics of Vγ9Vδ2 T cells in patients who received the adoptive transfer. The number of Vγ9Vδ2 T cells increased gradually in six patients who received autogenous Vγ9Vδ2 T cells injection, and the percentage of IFN-γ+ or CD107a+ cells in Vγ9Vδ2 T cells was higher than that of other Vγ9-CD3+ T cells, suggesting that Vγ9Vδ2 T cells in vivo had toxicity against cancer cells. Moreover, in the absence of exogenous IL-2, the ex vivo-expanded γδ T cells infused back into the patients showed a CD45RA–CD27– effector phenotype and were IL-2Rα-IL-7Rα-IL-15Rα-IL-2Rβ+ γc+ that might bind to the IL-15Rα/IL-15 complex, which suggested that endogenous IL-15 might sustain the proliferation of γδ T cells in patients treated with adoptive transfer (42) (Table 1).
Other several clinical trials based on γδ T cells are also conducted in CRC patients (Table 1). A total of 28 patients with advanced solid tumors, including three colon tumor patients, were enrolled in a two-center, open-label, phase I study, and the results showed that the application of the combination of bromohydrin pyrophosphate (IPH1101) and a low-dose of IL-2 was safe and well-tolerated in antitumor immunotherapy, which could effectively induce the expansion of γδ T cells in tumor patients, and the degree of expansion depended on the concentration of IPH1101 (92). In this trial, no patient achieved an evaluable objective response; stable disease was observed in 12 patients, and progressive disease was observed in 16 cases. No grade 4 toxicity was observed at any dose level among 28 patients. Similarly, another clinical trial involving 25 patients with advanced solid tumors (including 1 patient with colon cancer) also showed that no severe adverse events were observed after the reinfusion of γδ T cells stimulated and amplified with zoledronate and IL-2 (91). A total of 14 patients were evaluable for objective tumor response. Among these 14 patients, eight cases of progressive disease, three cases of stable disease, and three cases of partial response were observed. In addition, A. J. Nicol et al. (96) enrolled 18 patients with advanced solid tumors (three cases of colon cancer) into a phase I clinical study to evaluate the feasibility and safety of immunotherapy based on ex vivo expanded, activated Vγ9Vδ2 T cells. No dose-limiting toxicity was observed, but three cases who received Vγ9Vδ2 T cell reinfusion, while continuing previously ineffective therapy, had disease responses. The clinical outcome of this trial is shown in Table 1.
Altogether, we can see that γδ T cell-based immunotherapy is safe and well-tolerated in tumor patients including CRC patients. γδ T cells-based immunotherapy has so far, however, mainly focused on solid tumors such as melanoma, lung cancer, breast cancer, prostate cancer, and so on, and the results of these trials show great tolerance and safety. The clinical research on solid tumors of hollow organs such as CRC and gastric cancer is still insufficient. Therefore, the application of γδ T cells-based immunotherapies in cavity organ tumors requires substantial research in the future.
Conclusion Remarks
As a bridge between innate immunity and adaptive immunity, γδ T cells have attracted great interest from the research community because of their rapid recognition of stressed cells in an MHC-unrestricted manner, coupled with their robust killing activity in different tumor types. The killing effects are mainly mediated by direct cytotoxicity, secretion of cytokines, expression of death receptors, and so on. γδ T cells also play a role in perpetuating CRC progression, which is closely related to the survival of CRC patients. Studies have shown that they could significantly inhibit the occurrence and development of CRC. On the other hand, recent studies showed that γδ T cells could promote the occurrence and growth of CRC by secreting IL-17. Their antitumor and protumor effects on CRC perhaps mainly depend on the components of local TME. Compared with αβ T cells, γδ T cells recognize tumor cells via an MHC-unrestricted manner and can be expanded ex vivo easily, thus being used in tumor immunotherapy. Immunotherapies based on γδ T cells are safe and well-tolerated in patients with solid tumors. However, some patients do not respond to γδ T cells-based immunotherapies. It is therefore necessary to explore the interaction between γδ T cells and tumor cells in vivo, and to explore what components affect and regulate this process, as well as exploring approaches to the reasons why some patients did not respond to immunotherapy or showed a low proliferative response of Vγ9Vδ2 T cells after the conventional stimulation of phosphoantigens. Further studies are needed to enhance the immunotherapy strategies on CRC, including better amplification of γδ T cells and a better clinical response. Clinical trials with large sample sizes on in vivo expansion and adoptive transfer of γδ T cells in CRC should be carried out to optimize current strategies. Also, the combination of traditional chemotherapy and immunotherapy that may improve patient’s response and overall survival is necessary to be explored to investigate the efficacy and safety. Of note, the interaction and mechanism between γδ T cells and other immunocytes remain unclear, so it will be valuable to elucidate it.
Author Contributions
RM wrote the manuscript. DY, YG, and RY revised the manuscript. KL directed the project, reviewed, and revised the manuscript. All authors contributed to the article and approved the submitted version.
Conflict of Interest
The authors declare that the research was conducted in the absence of any commercial or financial relationships that could be construed as a potential conflict of interest.
References
1. Keum N, Giovannucci, E. Global burden of colorectal cancer: emerging trends, risk factors and prevention strategies. Nat Rev Gastroenterol Hepatol. (2019) 16:713–32. doi: 10.1038/s41575-019-0189-8
2. Arnold M, Sierra MS, Laversanne M, Soerjomataram I, Jemal A, Bray, F. Global patterns and trends in colorectal cancer incidence and mortality. Gut. (2017) 66:683–91. doi: 10.1136/gutjnl-2015-310912
3. The Lancet Gastroenterology Hepatology The shifting epidemiology of colorectal cancer. Lancet Gastroenterol Hepatol. (2019) 4:489. doi: 10.1016/s2468-1253(19)30150-5
4. Siegel RL, Miller KD, Goding Sauer A, Fedewa SA, Butterly LF, Anderson JC, et al. Colorectal cancer statistics, 2020. CA Cancer J Clin. (2020) 70:145–64. doi: 10.3322/caac.21601
5. van der Geest LG, Lam-Boer J, Koopman M, Verhoef C, Elferink MA, de Wilt, JH. Nationwide trends in incidence, treatment and survival of colorectal cancer patients with synchronous metastases. Clin Exp Metastasis. (2015) 32:457–65. doi: 10.1007/s10585-015-9719-0
6. Van Cutsem E, Cervantes A, Nordlinger B, Arnold D, Esmo Guidelines Working Group. Metastatic colorectal cancer: ESMO clinical practice guidelines for diagnosis, treatment and follow-up. Ann Oncol. (2014) 25(Suppl. 3):iii1–9. doi: 10.1093/annonc/mdu260
7. Galizia G, Lieto E, Orditura M, Castellano P, Imperatore V, Pinto M, et al. First-line chemotherapy vs bowel tumor resection plus chemotherapy for patients with unresectable synchronous colorectal hepatic metastases. Arch Surg. (2008) 143:352–8; discussion 358. doi: 10.1001/archsurg.143.4.352
8. Ryuk JP, Choi GS, Park JS, Kim HJ, Park SY, Yoon GS, et al. Predictive factors and the prognosis of recurrence of colorectal cancer within 2 years after curative resection. Ann Surg Treat Res. (2014) 86:143–51. doi: 10.4174/astr.2014.86.3.143
9. Junttila MR, de Sauvage, FJ. Influence of tumour micro-environment heterogeneity on therapeutic response. Nature. (2013) 501:346–54. doi: 10.1038/nature12626
10. Wu T, Dai, Y. Tumor microenvironment and therapeutic response. Cancer Lett. (2017) 387:61–8. doi: 10.1016/j.canlet.2016.01.043
11. Krijgsman D, de Vries NL, Skovbo A, Andersen MN, Swets M, Bastiaannet E, et al. Characterization of circulating T-, NK-, NKT cell subsets in patients with colorectal cancer: the peripheral blood immune cell profile. Cancer Immunol Immunother. (2019) 68:1011–24. doi: 10.1007/s00262-019-02343-7
12. Razi S, Baradaran Noveiry B, Keshavarz-Fathi M, Rezaei, N. IL-17 and colorectal cancer: from carcinogenesis to treatment. Cytokine. (2019) 116:7–12. doi: 10.1016/j.cyto.2018.12.021
13. Des Guetz G, Uzzan B, Nicolas P, Cucherat M, Morere JF, Benamouzig R, et al. Microvessel density and VEGF expression are prognostic factors in colorectal cancer. Meta-analysis of the literature. Br J Cancer. (2006) 94:1823–32. doi: 10.1038/sj.bjc.6603176
14. Poggi A, Zocchi, MR. gammadelta T Lymphocytes as a first line of immune defense: old and new ways of antigen recognition and implications for cancer immunotherapy. Front Immunol. (2014) 5:575. doi: 10.3389/fimmu.2014.00575
15. Liang D, Shao H, Born WK, O’Brien RL, Kaplan HJ, Sun, D. Connection between gammadelta T-cell- and Adenosine- mediated Immune regulation in the pathogenesis of experimental autoimmune uveitis. Crit Rev Immunol. (2018) 38:233–43. doi: 10.1615/CritRevImmunol.2018026150
16. Van Acker HH, Anguille S, De Reu H, Berneman ZN, Smits EL, Van Tendeloo, VF. Interleukin-15-cultured dendritic cells enhance anti-tumor gamma delta T cell functions through IL-15 secretion. Front Immunol. (2018) 9:658. doi: 10.3389/fimmu.2018.00658
17. Rong L, Li K, Li R, Liu HM, Sun R, Liu, XY. Analysis of tumor-infiltrating gamma delta T cells in rectal cancer. World J Gastroenterol. (2016) 22:3573–80. doi: 10.3748/wjg.v22.i13.3573
18. Patin EC, Soulard D, Fleury S, Hassane M, Dombrowicz D, Faveeuw C, et al. Type I IFN receptor signaling controls IL7-dependent accumulation and activity of protumoral IL17A-producing gammadelta T cells in breast cancer. Cancer Res. (2018) 78:195–204. doi: 10.1158/0008-5472.CAN-17-1416
19. Tawfik D, Groth C, Gundlach JP, Peipp M, Kabelitz D, Becker T, et al. TRAIL-receptor 4 Modulates gammadelta T cell-cytotoxicity toward cancer cells. Front Immunol. (2019) 10:2044. doi: 10.3389/fimmu.2019.02044
20. Todaro M, D’Asaro M, Caccamo N, Iovino F, Francipane MG, Meraviglia S, et al. Efficient killing of human colon cancer stem cells by gammadelta T lymphocytes. J Immunol. (2009) 182:7287–96. doi: 10.4049/jimmunol.0804288
21. Aotsuka A, Matsumoto Y, Arimoto T, Kawata A, Ogishima J, Taguchi A, et al. Interleukin-17 is associated with expression of programmed cell death 1 ligand 1 in ovarian carcinoma. Cancer Sci. (2019) 110:3068–78. doi: 10.1111/cas.14174
22. Caccamo N, La Mendola C, Orlando V, Meraviglia S, Todaro M, Stassi G, et al. Differentiation phenotype, and function of interleukin-17-producing human Vgamma9Vdelta2 T cells. Blood. (2011) 118:129–38. doi: 10.1182/blood-2011-01-331298
23. Fabre J, Giustiniani J, Garbar C, Antonicelli F, Merrouche Y, Bensussan A, et al. Targeting the Tumor microenvironment: the protumor effects of IL-17 related to cancer type. Int J Mol Sci. (2016) 17:1433. doi: 10.3390/ijms17091433
24. Kathania M, Khare P, Zeng M, Cantarel B, Zhang H, Ueno H, et al. Itch inhibits IL-17-mediated colon inflammation and tumorigenesis by ROR-gammat ubiquitination. Nat Immunol. (2016) 17:997–1004. doi: 10.1038/ni.3488
25. Raverdeau M, Cunningham SP, Harmon C, Lynch, L. γδ T cells in cancer: a small population of lymphocytes with big implications. Clin Transl Immunol. (2019) 8:e01080. doi: 10.1002/cti2.1080
26. Wakita D, Sumida K, Iwakura Y, Nishikawa H, Ohkuri T, Chamoto K, et al. Tumor-infiltrating IL-17-producing gammadelta T cells support the progression of tumor by promoting angiogenesis. Eur J Immunol. (2010) 40:1927–37. doi: 10.1002/eji.200940157
27. Di Lorenzo B, Ravens S, Silva-Santos, B. High-throughput analysis of the human thymic Vdelta1(+) T cell receptor repertoire. Sci Data. (2019) 6:115. doi: 10.1038/s41597-019-0118-2
28. Li X, Lu H, Gu Y, Zhang X, Zhang G, Shi T, et al. Tim-3 suppresses the killing effect of Vgamma9Vdelta2T cells on colon cancer cells by reducing perforin and granzyme B expression. Exp Cell Res. (2020) 386:111719. doi: 10.1016/j.yexcr.2019.111719
29. Gober HJ, Kistowska M, Angman L, Jeno P, Mori L, De Libero, G. Human T cell receptor gammadelta cells recognize endogenous mevalonate metabolites in tumor cells. J Exp Med. (2003) 197:163–8. doi: 10.1084/jem.20021500
30. Sacchi A, Tumino N, Sabatini A, Cimini E, Casetti R, Bordoni V, et al. Myeloid-derived suppressor cells specifically suppress IFN-gamma production and antitumor cytotoxic activity of vdelta2 T cells. Front Immunol. (2018) 9:1271. doi: 10.3389/fimmu.2018.01271
31. Ribot JC, deBarros A, Pang DJ, Neves JF, Peperzak V, Roberts SJ, et al. CD27 is a thymic determinant of the balance between interferon-gamma- and interleukin 17-producing gammadelta T cell subsets. Nat Immunol. (2009) 10:427–36. doi: 10.1038/ni.1717
32. Wu P, Wu D, Ni C, Ye J, Chen W, Hu G, et al. gammadeltaT17 cells promote the accumulation and expansion of myeloid-derived suppressor cells in human colorectal cancer. Immunity. (2014) 40:785–800. doi: 10.1016/j.immuni.2014.03.013
33. Patil RS, Shah SU, Shrikhande SV, Goel M, Dikshit RP, Chiplunkar, SV. IL17 producing γδT cells induce angiogenesis and are associated with poor survival in gallbladder cancer patients. Int J Cancer. (2016) 139:869–81. doi: 10.1002/ijc.30134
34. Coffelt SB, Kersten K, Doornebal CW, Weiden J, Vrijland K, Hau CS, et al. IL-17-producing γδ T cells and neutrophils conspire to promote breast cancer metastasis. Nature. (2015) 522:345–8. doi: 10.1038/nature14282
35. Di Carlo E, Bocca P, Emionite L, Cilli M, Cipollone G, Morandi F, et al. Mechanisms of the antitumor activity of human Vγ9Vδ2 T cells in combination with zoledronic acid in a preclinical model of neuroblastoma. Mol Ther. (2013) 21:1034–43. doi: 10.1038/mt.2013.38
36. Sun L, Li Y, Jiang Z, Zhang J, Li H, Li B, et al. Vγ9Vδ2 T cells and zoledronate mediate antitumor activity in an orthotopic mouse model of human chondrosarcoma. Tumour Biol. (2016) 37:7333–44. doi: 10.1007/s13277-015-4615-4
37. Zysk A, DeNichilo MO, Panagopoulos V, Zinonos I, Liapis V, Hay S, et al. Adoptive transfer of ex vivo expanded Vγ9Vδ2 T cells in combination with zoledronic acid inhibits cancer growth and limits osteolysis in a murine model of osteolytic breast cancer. Cancer Lett. (2017) 386:141–50. doi: 10.1016/j.canlet.2016.11.013
38. Corvaisier M, Moreau-Aubry A, Diez E, Bennouna J, Mosnier JF, Scotet E, et al. V gamma 9V delta 2 T cell response to colon carcinoma cells. J Immunol. (2005) 175:5481–8. doi: 10.4049/jimmunol.175.8.5481
39. Gentles AJ, Newman AM, Liu CL, Bratman SV, Feng W, Kim D, et al. The prognostic landscape of genes and infiltrating immune cells across human cancers. Nat Med. (2015) 21:938–45. doi: 10.1038/nm.3909
40. Tosolini M, Pont F, Poupot M, Vergez F, Nicolau-Travers ML, Vermijlen D, et al. Assessment of tumor-infiltrating TCRVgamma9Vdelta2 gammadelta lymphocyte abundance by deconvolution of human cancers microarrays. Oncoimmunology. (2017) 6:e1284723. doi: 10.1080/2162402x.2017.1284723
41. McCarthy NE, Bashir Z, Vossenkamper A, Hedin CR, Giles EM, Bhattacharjee S, et al. Proinflammatory Vdelta2+ T cells populate the human intestinal mucosa and enhance IFN-gamma production by colonic alphabeta T cells. J Immunol. (2013) 191:2752–63. doi: 10.4049/jimmunol.1202959
42. Izumi T, Kondo M, Takahashi T, Fujieda N, Kondo A, Tamura N, et al. Ex vivo characterization of gammadelta T-cell repertoire in patients after adoptive transfer of Vgamma9Vdelta2 T cells expressing the interleukin-2 receptor beta-chain and the common gamma-chain. Cytotherapy. (2013) 15:481–91. doi: 10.1016/j.jcyt.2012.12.004
43. Cabillic F, Toutirais O, Lavoue V, de La Pintiere CT, Daniel P, Rioux-Leclerc N, et al. Aminobisphosphonate-pretreated dendritic cells trigger successful Vgamma9Vdelta2 T cell amplification for immunotherapy in advanced cancer patients. Cancer Immunol Immunother. (2010) 59:1611–9. doi: 10.1007/s00262-010-0887-0
44. Zocchi MR, Costa D, Vene R, Tosetti F, Ferrari N, Minghelli S, et al. Zoledronate can induce colorectal cancer microenvironment expressing BTN3A1 to stimulate effector gammadelta T cells with antitumor activity. Oncoimmunology. (2017) 6:e1278099. doi: 10.1080/2162402x.2016.1278099
45. Maeurer MJ, Martin D, Walter W, Liu K, Zitvogel L, Halusczcak K, et al. Human intestinal Vdelta1+ lymphocytes recognize tumor cells of epithelial origin. J Exp Med. (1996) 183:1681–96. doi: 10.1084/jem.183.4.1681
46. Almeida AR, Correia DV, Fernandes-Platzgummer A, da Silva CL, da Silva MG, Anjos DR, et al. Delta one T cells for immunotherapy of chronic lymphocytic leukemia: clinical-grade expansion/differentiation and preclinical proof of concept. Clin Cancer Res. (2016) 22:5795–804. doi: 10.1158/1078-0432.Ccr-16-0597
47. Wu D, Wu P, Wu X, Ye J, Wang Z, Zhao S, et al. Ex vivoexpanded human circulating Vδ1 γδT cells exhibit favorable therapeutic potential for colon cancer. OncoImmunology. (2015) 4:e992749. doi: 10.4161/2162402x.2014.992749
48. Devaud C, Rousseau B, Netzer S, Pitard V, Paroissin C, Khairallah C, et al. Anti-metastatic potential of human Vdelta1(+) gammadelta T cells in an orthotopic mouse xenograft model of colon carcinoma. Cancer Immunol Immunother. (2013) 62:1199–210. doi: 10.1007/s00262-013-1402-1
49. Meraviglia S, Lo Presti E, Tosolini M, La Mendola C, Orlando V, Todaro M, et al. Distinctive features of tumor-infiltrating gammadelta T lymphocytes in human colorectal cancer. Oncoimmunology. (2017) 6:e1347742. doi: 10.1080/2162402x.2017.1347742
50. Mikulak J, Oriolo F, Bruni E, Roberto A, Colombo FS, Villa A, et al. NKp46-expressing human gut-resident intraepithelial Vdelta1 T cell subpopulation exhibits high antitumor activity against colorectal cancer. JCI Insight. (2019) 4:e125884. doi: 10.1172/jci.insight.125884
51. Varesano S, Zocchi MR, Poggi, A. Zoledronate triggers vdelta2 T cells to destroy and kill spheroids of colon carcinoma: quantitative image analysis of three-dimensional cultures. Front Immunol. (2018) 9:998. doi: 10.3389/fimmu.2018.00998
52. Lanier, LL. NKG2D receptor and its ligands in host defense. Cancer Immunol Res. (2015) 3:575–82. doi: 10.1158/2326-6066.CIR-15-0098
53. Wrobel P, Shojaei H, Schittek B, Gieseler F, Wollenberg B, Kalthoff H, et al. Lysis of a broad range of epithelial tumour cells by human gamma delta T cells: involvement of NKG2D ligands and T-cell receptor- versus NKG2D-dependent recognition. Scand J Immunol. (2007) 66:320–8. doi: 10.1111/j.1365-3083.2007.01963.x
54. Maccalli C, Pende D, Castelli C, Mingari MC, Robbins PF, Parmiani, G. NKG2D engagement of colorectal cancer-specific T cells strengthens TCR-mediated antigen stimulation and elicits TCR independent anti-tumor activity. Eur J Immunol. (2003) 33:2033–43. doi: 10.1002/eji.200323909
55. Bauer S, Groh V, Wu J, Steinle A, Phillips JH, Lanier LL, et al. Activation of NK cells and T cells by NKG2D, a receptor for stress-inducible MICA. Science. (2009) 285:727–9.
56. Lin L, He J, Li J, Xu Y, Li J, Wu, Y. Chitosan nanoparticles strengthen vgamma9vdelta2 T-cell cytotoxicity through upregulation of killing molecules and cytoskeleton polarization. Int J Nanomedicine. (2019) 14:9325–36. doi: 10.2147/IJN.S212898
57. Simoes AE, Di Lorenzo B, Silva-Santos, B. Molecular determinants of target cell recognition by human gammadelta T cells. Front Immunol. (2018) 9:929. doi: 10.3389/fimmu.2018.00929
58. Kondo M, Sakuta K, Noguchi A, Ariyoshi N, Sato K, Sato S, et al. Zoledronate facilitates large-scale ex vivo expansion of functional gammadelta T cells from cancer patients for use in adoptive immunotherapy. Cytotherapy. (2008) 10:842–56. doi: 10.1080/14653240802419328
59. Rossi C, Gravelle P, Decaup E, Bordenave J, Poupot M, Tosolini M, et al. Boosting gammadelta T cell-mediated antibody-dependent cellular cytotoxicity by PD-1 blockade in follicular lymphoma. Oncoimmunology. (2019) 8:1554175. doi: 10.1080/2162402X.2018.1554175
60. Waldmann TA, Miljkovic MD, Conlon, KC. Interleukin-15 (dys)regulation of lymphoid homeostasis: implications for therapy of autoimmunity and cancer. J Exp Med. (2020) 217:e20191062. doi: 10.1084/jem.20191062
61. Schiller CB, Braciak TA, Fenn NC, Seidel UJ, Roskopf CC, Wildenhain S, et al. CD19-specific triplebody SPM-1 engages NK and gammadelta T cells for rapid and efficient lysis of malignant B-lymphoid cells. Oncotarget. (2016) 7:83392–408. doi: 10.18632/oncotarget.13110
62. Wang B, Tian Q, Guo D, Lin W, Xie X, Bi, H. Activated gammadelta Tcells promote dendritic cell maturation and exacerbate thedevelopment of experimental autoimmune uveitis (EAU) in mice. Immunol Investig. (2020) 3:1–20. doi: 10.1080/08820139.2020.1716786
63. Oberg HH, Wesch D, Kalyan S, Kabelitz, D. Regulatory interactions between neutrophils, tumor cells and T cells. Front Immunol. (2019) 10:1690. doi: 10.3389/fimmu.2019.01690
64. Wu S, Rhee KJ, Albesiano E, Rabizadeh S, Wu X, Yen HR, et al. A human colonic commensal promotes colon tumorigenesis via activation of T helper type 17 T cell responses. Nat Med. (2009) 15:1016–22. doi: 10.1038/nm.2015
65. Sutton CE, Lalor SJ, Sweeney CM, Brereton CF, Lavelle EC, Mills, KH. Interleukin-1 and IL-23 induce innate IL-17 production from gammadelta T cells, amplifying Th17 responses and autoimmunity. Immunity. (2009) 31:331–41. doi: 10.1016/j.immuni.2009.08.001
66. Grivennikov SI, Wang K, Mucida D, Stewart CA, Schnabl B, Jauch D, et al. Adenoma-linked barrier defects and microbial products drive IL-23/IL-17-mediated tumour growth. Nature. (2012) 491:254–8. doi: 10.1038/nature11465
67. Xu R, Wang R, Han G, Wang J, Chen G, Wang L, et al. Complement C5a regulates IL-17 by affecting the crosstalk between DC and gammadelta T cells in CLP-induced sepsis. Eur J Immunol. (2010) 40:1079–88. doi: 10.1002/eji.200940015
68. Corpuz TM, Vazquez-Lombardi R, Luong JK, Warren J, Stolp J, Christ D, et al. IL-2 shapes the survival and plasticity of IL-17-producing gammadelta T cells. J Immunol. (2017) 199:2366–76. doi: 10.4049/jimmunol.1700335
69. Mensurado S, Rei M, Lanca T, Ioannou M, Goncalves-Sousa N, Kubo H, et al. Tumor-associated neutrophils suppress pro-tumoral IL-17+ gammadelta T cells through induction of oxidative stress. PLoS Biol. (2018) 16:e2004990. doi: 10.1371/journal.pbio.2004990
70. Kalyan S, Chandrasekaran V, Quabius ES, Lindhorst TK, Kabelitz, D. Neutrophil uptake of nitrogen-bisphosphonates leads to the suppression of human peripheral blood gammadelta T cells. Cell Mol Life Sci. (2014) 71:2335–46. doi: 10.1007/s00018-013-1495-x
71. Gelderblom M, Gallizioli M, Ludewig P, Thom V, Arunachalam P, Rissiek B, et al. IL-23 (Interleukin-23)-Producing conventional dendritic cells control the detrimental IL-17 (Interleukin-17) response in stroke. Stroke. (2018) 49:155–64. doi: 10.1161/strokeaha.117.019101
72. Lo Presti E, Caccamo N, Orlando V, Dieli F, Meraviglia, S. Activation and selective IL-17 response of human Vgamma9Vdelta2 T lymphocytes by TLR-activated plasmacytoid dendritic cells. Oncotarget. (2016) 7:60896–905. doi: 10.18632/oncotarget.11755
73. Matsuda S, Kudoh S, Katayama, S. Enhanced formation of azoxymethane-induced colorectal adenocarcinoma in gammadelta T lymphocyte-deficient mice. Jpn J Cancer Res. (2001) 92:880–5. doi: 10.1111/j.1349-7006.2001.tb01176.x
74. Murayama M, Tanaka Y, Yagi J, Uchiyama T, Ogawa, K. Antitumor activity and some immunological properties of gammadelta T-cells from patients with gastrointestinal carcinomas. Anticancer Res. (2008) 28:2921–31.
75. Zhu SP, Liu G, Wu XT, Chen FX, Liu JQ, Zhou ZH, et al. The effect of phloretin on human gammadelta T cells killing colon cancer SW-1116 cells. Int Immunopharmacol. (2013) 15:6–14. doi: 10.1016/j.intimp.2012.11.001
76. Housseau F, Wu S, Wick EC, Fan H, Wu X, Llosa NJ, et al. Redundant innate and adaptive sources of IL17 production drive colon tumorigenesis. Cancer Res. (2016) 76:2115–24. doi: 10.1158/0008-5472.CAN-15-0749
77. Shapiro JM, Subedi S, LeLeiko, NS. Inflammatory bowel disease. Pediatr Rev. (2016) 37:337–47. doi: 10.1542/pir.2015-0110
78. Rutter MD, Saunders BP, Wilkinson KH, Rumbles S, Schofield G, Kamm MA, et al. Thirty-year analysis of a colonoscopic surveillance program for neoplasia in ulcerative colitis. Gastroenterology. (2006) 130:1030–8. doi: 10.1053/j.gastro.2005.12.035
79. Zhou Q, Shen ZF, Wu BS, Xu CB, He ZQ, Chen T, et al. Risk of colorectal cancer in ulcerative colitis patients: a systematic review and meta-analysis. Gastroenterol Res Pract. (2019) 2019:5363261. doi: 10.1155/2019/5363261
80. Axelrad JE, Lichtiger S, and Yajnik V. Inflammatory bowel disease and cancer: the role of inflammation, immunosuppression, and cancer treatment. World J Gastroenterol. (2016) 22:4794–801. doi: 10.3748/wjg.v22.i20.4794
81. Goethel A, Croitoru K, Philpott, DJ. The interplay between microbes and the immune response in inflammatory bowel disease. J Physiol. (2018) 596:3869–82. doi: 10.1113/jp275396
82. Park SG, Mathur R, Long M, Hosh N, Hao L, Hayden MS, et al. T regulatory cells maintain intestinal homeostasis by suppressing γδ T cells. Immunity. (2010) 33:791–803. doi: 10.1016/j.immuni.2010.10.014
83. Inagaki-Ohara K, Chinen T, Matsuzaki G, Sasaki A, Sakamoto Y, Hiromatsu K, et al. Mucosal T cells bearing TCRgammadelta play a protective role in intestinal inflammation. J Immunol. (2004) 173:1390–8. doi: 10.4049/jimmunol.173.2.1390
84. Lo Presti E, Di Mitri R, Mocciaro F, Di Stefano AB, Scibetta N, Unti E, et al. Characterization of γδ T cells in intestinal mucosa from patients with early-onset or long-standing inflammatory bowel disease and their correlation with clinical status. J Crohns Colitis. (2019) 13:873–83. doi: 10.1093/ecco-jcc/jjz015
85. Lo Presti E, Mocciaro F, Mitri RD, Corsale AM, Di Simone M, Vieni S, et al. Analysis of colon-infiltrating γδ T cells in chronic inflammatory bowel disease and in colitis-associated cancer. J Leukoc Biol. (2020) 108:749–60. doi: 10.1002/jlb.5ma0320-201rr
86. McVay LD, Li B, Biancaniello R, Creighton MA, Bachwich D, Lichtenstein G, et al. Changes in human mucosal gamma delta T cell repertoire and function associated with the disease process in inflammatory bowel disease. Mol Med. (1997) 3:183–203.
87. Lo Presti E, Meraviglia S, Corsale AM, Mocciaro F, De Simone M, Gambino D, et al. Role of γδ T cells in inflammatory bowel disease and colitis-associated cancer. Eur J Immunol. (2019) 49:168–9. doi: 10.1002/eji.201970300
88. Kadivar M, Petersson J, Svensson L, Marsal, J. CD8αβ+ γδ T cells: a novel T cell subset with a potential role in inflammatory bowel disease. J Immunol. (2016) 197:4584–92. doi: 10.4049/jimmunol.1601146
89. Hoeres T, Holzmann E, Smetak M, Birkmann J, Wilhelm, M. PD-1 signaling modulates interferon-gamma production by Gamma Delta (gammadelta) T-Cells in response to leukemia. Oncoimmunology. (2019) 8:1550618. doi: 10.1080/2162402x.2018.1550618
90. Ugurel S, Schadendorf D, Horny K, Sucker A, Schramm S, Utikal J, et al. Elevated baseline serum PD-1 or PD-L1 predicts poor outcome of PD-1 inhibition therapy in metastatic melanoma. Ann Oncol. (2020) 31:144–52. doi: 10.1016/j.annonc.2019.09.005
91. Noguchi A, Kaneko T, Kamigaki T, Fujimoto K, Ozawa M, Saito M, et al. Zoledronate-activated Vgamma9gammadelta T cell-based immunotherapy is feasible and restores the impairment of gammadelta T cells in patients with solid tumors. Cytotherapy. (2011) 13:92–7. doi: 10.3109/14653249.2010.515581
92. Bennouna J, Levy V, Sicard H, Senellart H, Audrain M, Hiret S, et al. Phase I study of bromohydrin pyrophosphate (BrHPP, IPH 1101), a Vgamma9Vdelta2 T lymphocyte agonist in patients with solid tumors. Cancer Immunol Immunother. (2010) 59:1521–30. doi: 10.1007/s00262-010-0879-0
93. Soiffer RJ, Murray C, Gonin R, Ritz, J. Effect of low-dose interleukin-2 on disease relapse after T-cell-depleted allogeneic bone marrow transplantation. Blood. (1994) 84:964–71.
94. Bouet-Toussaint F, Cabillic F, Toutirais O, Le Gallo M, Thomas de la Pintiere C, Daniel P, et al. Vgamma9Vdelta2 T cell-mediated recognition of human solid tumors. Potential for immunotherapy of hepatocellular and colorectal carcinomas. Cancer Immunol Immunother. (2008) 57:531–9. doi: 10.1007/s00262-007-0391-3
95. Nakajima J, Murakawa T, Fukami T, Goto S, Kaneko T, Yoshida Y, et al. A phase I study of adoptive immunotherapy for recurrent non-small-cell lung cancer patients with autologous gammadelta T cells. Eur J Cardiothorac Surg. (2010) 37:1191–7. doi: 10.1016/j.ejcts.2009.11.051
Keywords: γδ T cells, colorectal cancer, antitumor effects, protumor effects, immunotherapy
Citation: Ma R, Yuan D, Guo Y, Yan R and Li K (2020) Immune Effects of γδ T Cells in Colorectal Cancer: A Review. Front. Immunol. 11:1600. doi: 10.3389/fimmu.2020.01600
Received: 15 April 2020; Accepted: 16 June 2020;
Published: 09 September 2020.
Edited by:
John – Maher, King’s College London, United KingdomReviewed by:
Bruno Silva-Santos, Universidade de Lisboa, PortugalSerena Meraviglia, University of Palermo, Italy
Copyright © 2020 Ma, Yuan, Guo, Yan and Li. This is an open-access article distributed under the terms of the Creative Commons Attribution License (CC BY). The use, distribution or reproduction in other forums is permitted, provided the original author(s) and the copyright owner(s) are credited and that the original publication in this journal is cited, in accordance with accepted academic practice. No use, distribution or reproduction is permitted which does not comply with these terms.
*Correspondence: Kang Li, healthlee@xjtu.edu.cn