- 1Department of Cardiology, Wenzhou People’s Hospital, Wenzhou, China
- 2Independent Researcher, Hangzhou, China
Objective: To examine whether the influence of hypertension (HTN) status on longitudinal changes in brain glucose metabolism was modified by the apolipoprotein 4 (APOE4) status among older people with normal cognition.
Methods: In this study, we included 217 older individuals with normal cognition from the Alzheimer’s Disease Neuroimaging Initiative (ADNI) study. Participants were divided into the HTN and no HTN groups based on self-reported medical history. Brain glucose metabolism was assessed by 18F-fluorodeoxyglucose-positron emission tomography (FDG-PET). Linear mixed model was fitted to examine the association between the HTN × APOE4 interaction and longitudinal changes in brain glucose metabolism after controlling for several covariates.
Results: In the present study, we found that the association between HTN status and longitudinal changes in brain glucose metabolism varied as a function of the APOE4 status, such that the HTN/APOE4+ group showed a steeper decline in FDG SUVR than all other groups (No HTN/APOE4-, HTN/APOE4-, and No HTN/APOE4+). Nevertheless, there was no significant difference in the rate of decline in FDG SUVR among other groups (No HTN/APOE4-, HTN/APOE4-, and No HTN/APOE4+).
Conclusion: The APOE4 genotype interacted with hypertension status to affect longitudinal changes in brain glucose metabolism among older individual with normal cognition, such that the HTN/APOE4+ group showed a steeper decline in FDG SUVR than other groups.
Introduction
Deteriorating brain glucose metabolism is a key feature of Alzheimer’s disease (AD) and precedes the clinical onset of AD (Small et al., 1995; Reiman et al., 1996; Li et al., 2008; Chen and Zhong, 2013). Cerebral glucose metabolic rates, assessed by 18F-fluorodeoxyglucose-positron emission tomography (FDG-PET), provide an crucial measure of the dysfunction of neurons and synapses in living human (De Leon et al., 2001; Dubois et al., 2007).
The apolipoprotein E (APOE) gene is the most important genetic risk factor for late-onset sporadic AD (Safieh et al., 2019). This gene has three polymorphic forms, ε2, ε3, ε4; and the APOE4 allele increases the risk of cognitive decline and AD dementia (Safieh et al., 2019). However, most, but not all FDG studies have suggested that the APOE4 allele is associated with reduced levels of brain glucose metabolism (Reiman et al., 1996, 2004, 2005; Corder et al., 1997; Samuraki et al., 2012). There is a possibility that the APOE4 allele may interact with other cardiovascular diseases [e.g., hypertension (HTN)] to affect the levels of brain glucose metabolism. In line with this notion, previous observational studies indicated that APOE4 and HTN act synergistically to influence cognitive performance, subcortical white matter integrity, and cortical amyloid accumulation (Peila et al., 2001; De Leeuw et al., 2004; De Frias et al., 2014; Oberlin et al., 2015; Jeon et al., 2019). However, no prior studies have attempted to assess the contributions of the APOE4 and HTN status to longitudinal changes in brain glucose metabolism among older individuals with normal cognition.
In this study, among older individuals with normal cognition, we hypothesized that the interaction between APOE4 and HTN is associated with longitudinal changes in brain glucose metabolism, such that APOE4 carriers with a history of hypertension (APOE4 + /HTN) show a steeper rate of decline in brain glucose metabolism than other groups (APOE4-/HTN, APOE4-/No HTN, and APOE4/No HTN).
Materials and Methods
Alzheimer’s Disease Neuroimaging Initiative (ADNI)
Longitudinal data used in the preparation of this work were extracted from the Alzheimer’s Disease Neuroimaging Initiative (ADNI) database1. The ADNI study was conducted with the primary aim of discovering potential biomarkers of cognitive decline for clinical trials. At ADNI centers, local institutional review boards approved the study, and each participant provided written informed consent.
Participants
At baseline, we included a total of 217 older individuals with normal cognition. In the present analysis, we included subjects who had baseline and follow-up measurement of brain glucose metabolism. The sample size at baseline and each follow-up visit were displayed in Table 1. Participants with normal cognition had a Clinical Dementia Rating (CDR) (Morris, 1993) of 0 and a mini-mental state examination (MMSE) (Folstein et al., 1975) of 24 or higher.
Hypertension (HTN) Status
Participants were further categorized into the No HTN (n = 121) and HTN (n = 96) groups according to the self-reported history of HTN. Several search terms (hypertension, high blood pressure, and HTN) were used to screen medical history of ADNI’ subjects.
APOE Genotyping
APOE4 genotypes of ADNI’s participants were extracted from the ADNI website2. Participants were divided into the APOE4- (absence of the APOE4 allele) and APOE4 + (presence of at least one APOE4 allele) groups.
Measurement of Brain Glucose Metabolism
Cerebral metabolic rates for glucose were examined using FDG-PET by Susan Landau and William Jagust’s group, Helen Wills Neuroscience Institute, UC Berkeley and Lawrence Berkeley National Laboratory. The neuroimaging techniques have been described previously (Landau et al., 2011). Five pre-defined regions of interest (MetaROIs) were identified according to coordinates reported frequently in previously FDG investigations comparing healthy controls, MCI, and AD patients. These five crucial hypometabolic ROIs included left angular gyrus, right angular gyrus, left inferior temporal gyrus, right inferior temporal gyrus, and bilateral posterior cingulate gyrus. In the present analysis, FDG standardized uptake value ratios (SUVR) were defined by averaging FDG uptake of these five regions and then dividing by FDG uptake of pons and cerebellum (a reference region) (Jagust et al., 2010; Landau et al., 2011). The reference region (the cerebellum and pons) was used in order to reduce between-subject nuisance variability in trace uptake.
Statistical Analysis
Group differences were examined with ANOVA tests for continuous parameters and Chi-squared tests for categorical parameters. In an effort to assess the association of the HTN∗APOE4 interaction with longitudinal changes in FDG SUVR among cognitively normal older individuals, we performed the linear mixed model including the three-way HTN × APOE4 × Time interaction term. This model also included main effects of age, educational years, gender, serum glucose, triglyceride, total cholesterol and their interactions with time, along with a random intercept for each subject. Finally, to examine interactions between HTN and APOE4 genotype, longitudinal changes in FDG SUVR across all pairwise group contrasts (No HTN/APOE4-, HTN/APOE4-, No HTN/APOE4+, and HTN/APOE4+) were conducted. The Tukey method was used for multiple comparisons correction. All statistical work was conducted using R version 3.6.0.
Results
Demographic and Clinical Variables by HTN Status
At baseline, a total of 217 older individuals with normal cognition was included. As shown in Table 1, no significant differences in demographics (age, education, gender and APOE4 genotype) and clinical variables (MMSE scores, FDG SUVR, serum glucose, total cholesterol, and triglyceride) were found between two groups (No HTN vs. HTN).
Demographics by HTN and APOE4 Status
Our participants were divided into four groups according to HTN and APOE4 status (Table 2). Demographics were compared between these four groups. However, there were no significant differences in age, educational years or the percentage of female gender across the four groups (all p > 0.05).
Longitudinal Change Models
To assess the contributions of HTN and APOE4 status to longitudinal changes in FDG SUVR, the linear mixed model was fitted. As shown in Table 3, we found that the 3-way interaction between HTN, APOE4, and time was significant for FDG SUVR (estimate = −0.0181, SE = 0.0054, p = 0.0008). To better understand this interaction, longitudinal changes in FDG SUVR across all pairwise group contrasts (No HTN/APOE4-, HTN/APOE4-, No HTN/APOE4+, and HTN/APOE4+) were conducted (Figure 1 and Table 4). Compared with other groups, the HTN/APOE4+ group demonstrated significantly or marginally significant steeper decline in FDG SUVR (Figure 1 and Table 4). However, there was no significant difference in the rate of decline in FDG SUVR among other groups (No HTN/APOE4-, HTN/APOE4-, and No HTN/APOE4+; Figure 1 and Table 4).
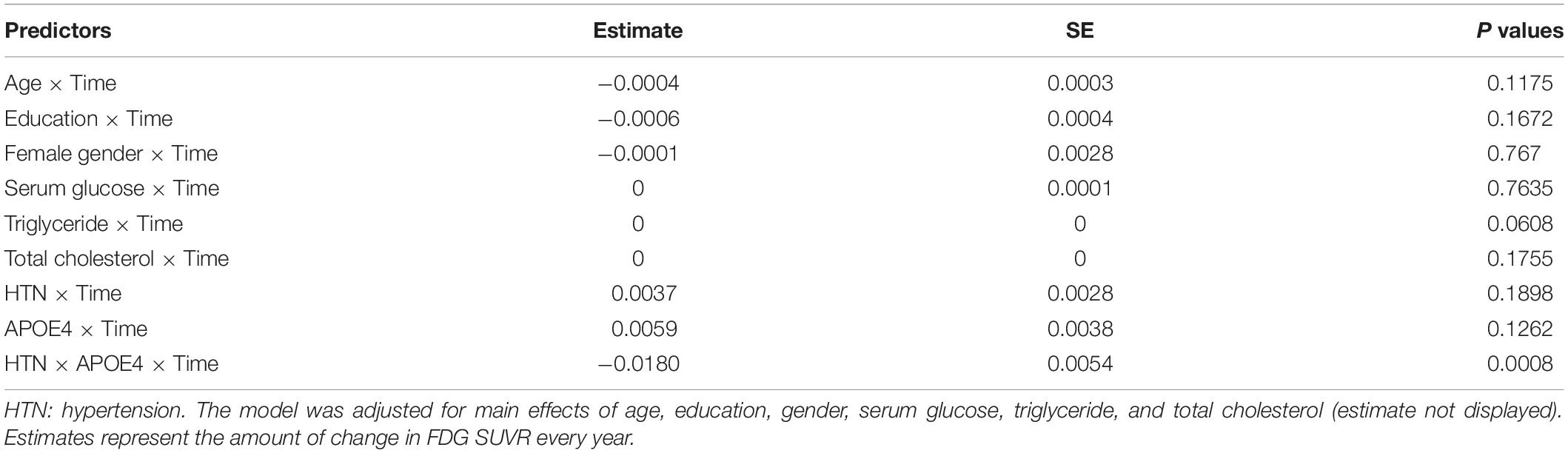
Table 3. Linear mixed models examining the influence of the HTN∗APOE4 interaction on longitudinal changes in FDG SUVR.
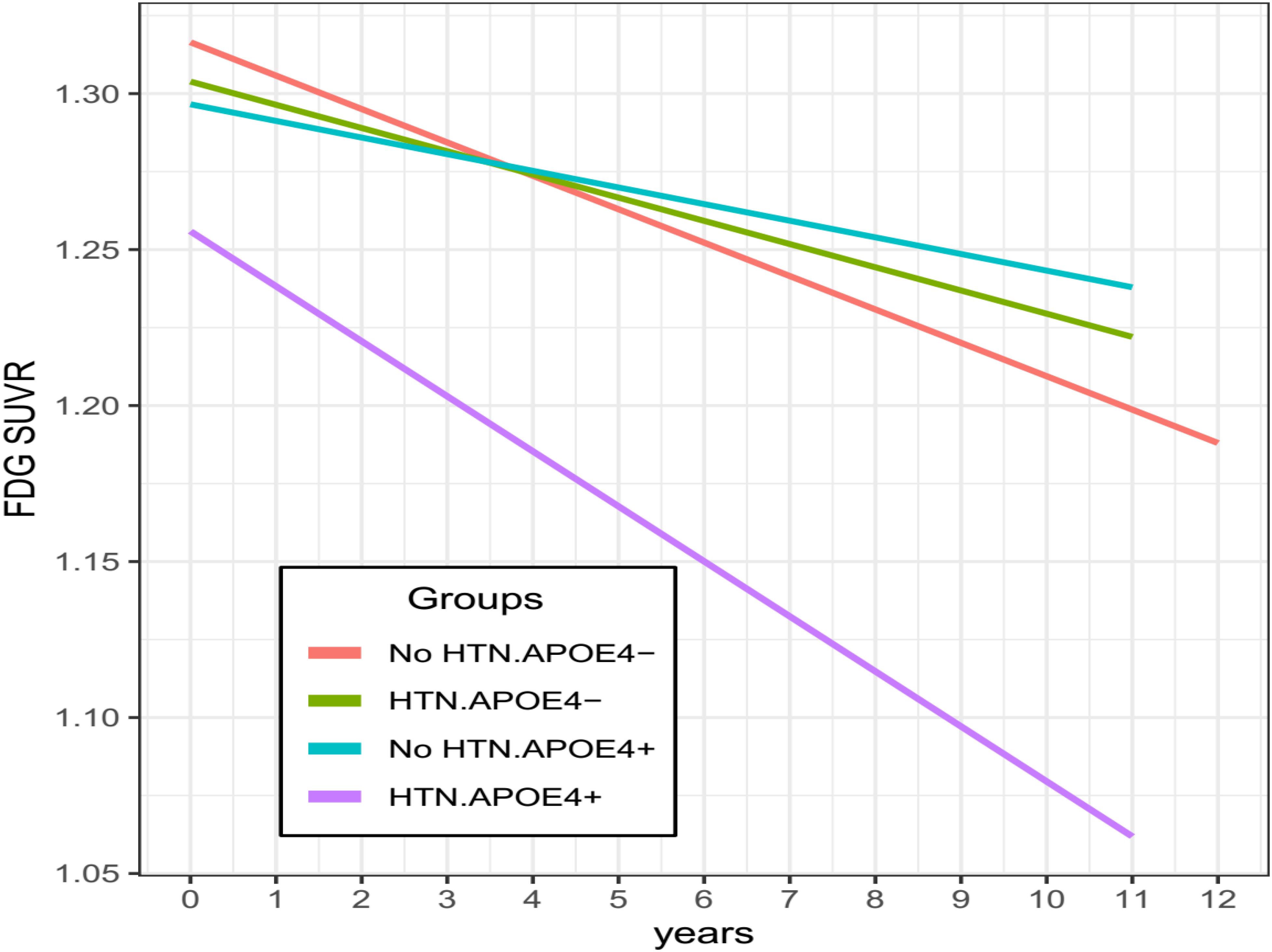
Figure 1. Longitudinal changes in FDG SUVR stratified by HTN and APOE4 status. Compared with other groups, the HTN/APOE4 + group demonstrated significantly steeper decline in FDG SUVR. However, there was no significant difference in the rate of decline in FDG SUVR among other groups. HTN, hypertension.
Discussion
In this study, we hypothesized that the APOE4 allele would moderate the influence of HTN status on longitudinal changes in brain glucose metabolism among cognitively normal older people. In line with this hypothesis, we observed that the relationship between HTN status and longitudinal changes in brain glucose metabolism varied as a function of the APOE4 status, such that the HTN/APOE4+ group showed a steeper decline in FDG SUVR than all other groups (No HTN/APOE4-, HTN/APOE4-, and No HTN/APOE4+). Nevertheless, there was no significant difference in the rate of decline in FDG SUVR among other groups (No HTN/APOE4-, HTN/APOE4-, and No HTN/APOE4+).
The finding that the HTN/APOE4+ group had a steeper decline in FDG SUVR than all other groups among cognitively normal older people is novel. Consistent with our finding, previous observational studies showed that the influence of hypertension on cognitive deficits, medial temporal atrophy, subcortical white matter lesions, cortical amyloid deposition, and tau phosphorylation was greater in APOE4 carriers than in APOE4 non-carriers (Peila et al., 2001; De Leeuw et al., 2004; Korf et al., 2004; Den Heijer et al., 2005; Kester et al., 2010; De Frias et al., 2014; Andrews et al., 2015; Oberlin et al., 2015; Jeon et al., 2019). Collectively these data and ours indicate that the impact of hypertension on neuronal damage and synapse loss appears to be exacerbated by the APOE4 allele.
The mechanisms by which the APOE4 allele could modify the association of hypertension on longitudinal changes in FDG SUVR are not very clear. However, there are several potential possibilities for the APOE4 × Hypertension interaction in relation to longitudinal changes in FDG SUVR. First, APOE is thought to play an important role in the response to neuronal damage by redistributing lipids to facilitate the regeneration of neuronal axons and maintaining the structure and function of the microtubules (Handelmann et al., 1992). Compared with wild-type mice, APOE-deficient mice have demonstrated much greater ischemic neuronal injury after ischemic episodes (Horsburgh et al., 1999). However, this beneficial effect is dependent on polymorphic forms: the APOE3 allele appears to facilitate the repair process, while the APOE4 allele tends to retard the process (Nathan et al., 1994; Bellosta et al., 1995; Teter et al., 1999). Therefore, it is likely that the influence of hypertension on neuronal structure would be greater in APOE4 carriers because of their limited ability to promote the repair process.
Second, it has been reported that a history of hypertension is associated with higher levels of neuritic plaques and neurofibrillary tangles (Sparks et al., 1995; Petrovitch et al., 2000). Similarly, compared to APOE4 non-carriers, APOE4 carriers shows a greater amount of amyloid and tau pathologies (Leoni, 2011). More importantly, a recent study using [11C]-Pittsburgh-compound-B-positron emission tomography showed that in APOE4 carriers, hypertension was associated with increased cortical Aβ accumulation (Jeon et al., 2019), which could contribute to brain glucose hypometabolism (Lowe et al., 2014). Therefore, the effect of hypertension on neuronal injury, measured by FDG SUVR, would be expected to be larger for APOE4 carriers.
Several limitations should be noted. First, participants in the ADNI study were highly educated and had fewer comorbidities. For instance, participants who had the Hachinski Ischemic Scale (HIS) score of 5 or higher were excluded from the ADNI study. Thus, this may limit the generalizability of our findings. Further studies, especially population-based studies, were needed to replicate our results. Second, the present study primarily focused on the association of the APOE4∗hypertension interaction with changes in FDG SUVR. It would be interesting to examine the association of this interaction with clinical progression and other AD-related markers, including cognitive function and CSF AD pathologies.
Conclusion
In conclusion, the APOE4 interacted with hypertension status to affect longitudinal changes in brain glucose metabolism among older individual with normal cognition, such that the HTN/APOE4+ group showed a steeper decline in FDG SUVR than other groups (Nos HTN/APOE4-, HTN/APOE4-, and No HTN/APOE4+).
Data Availability Statement
The datasets generated for this study will not be made publicly available. Datasets can be found at the ANDI website (http://adni.loni.usc.edu).
Ethics Statement
The studies involving human participants were reviewed and approved by the ADNI centers approved the ADNI study. The patients/participants provided their written informed consent to participate in this study.
Author Contributions
JZ and SH conceived and designed the study. RZ, HC, and FY performed the research and analyzed the data. RZ wrote the manuscript. All authors approved the final version of the manuscript.
Conflict of Interest
The authors declare that the research was conducted in the absence of any commercial or financial relationships that could be construed as a potential conflict of interest.
Acknowledgments
Data collection and sharing for this project was funded by the Alzheimer’s Disease Neuroimaging Initiative (ADNI) (National Institutes of Health Grant U01 AG024904) and DOD ADNI (Department of Defense award number W81XWH-12-2-0012). ADNI is funded by the National Institute on Aging, the National Institute of Biomedical Imaging and Bioengineering, and through generous contributions from the following: AbbVie, Alzheimer’s Association; Alzheimer’s Drug Discovery Foundation; Araclon Biotech; Bioclinica Inc.; Biogen; Bristol-Myers Squibb Company; CereSpir Inc.; Cogstate; Eisai Inc.; Elan Pharmaceuticals Inc.; Eli Lilly and Company; EuroImmun; F. Hoffmann-La Roche Ltd and its affiliated company Genentech, Inc.; Fujirebio; GE Healthcare; IXICO Ltd.; Janssen Alzheimer Immunotherapy Research & Development, LLC.; Johnson & Johnson Pharmaceutical Research & Development LLC.; Lumosity; Lundbeck; Merck & Co., Inc.; Meso Scale Diagnostics, LLC.; NeuroRx Research; Neurotrack Technologies; Novartis Pharmaceuticals Corporation; Pfizer Inc.; Piramal Imaging; Servier; Takeda Pharmaceutical Company; and Transition Therapeutics. The Canadian Institutes of Health Research is providing funds to support ADNI clinical sites in Canada. Private sector contributions are facilitated by the Foundation for the National Institutes of Health (www.fnih.org). The grantee organization is the Northern California Institute for Research and Education, and the study is coordinated by the Alzheimer’s Therapeutic Research Institute at the University of Southern California. ADNI data are disseminated by the Laboratory for Neuro Imaging at the University of Southern California.
Footnotes
References
Andrews, S., Das, D., Anstey, K. J., and Easteal, S. (2015). Interactive effect of APOE genotype and blood pressure on cognitive decline: the PATH through life study. J. Alzheimers Dis. 44, 1087–1098. doi: 10.3233/JAD-140630
Bellosta, S., Nathan, B. P., Orth, M., Dong, L. M., Mahley, R. W., and Pitas, R. E. (1995). Stable expression and secretion of apolipoproteins E3 and E4 in mouse neuroblastoma cells produces differential effects on neurite outgrowth. J. Biol. Chem. 270, 27063–27071.
Chen, Z., and Zhong, C. (2013). Decoding Alzheimer’s disease from perturbed cerebral glucose metabolism: implications for diagnostic and therapeutic strategies. Prog. Neurobiol. 108, 21–43. doi: 10.1016/j.pneurobio.2013.06.004
Corder, E. H., Jelic, V., Basun, H., Lannfelt, L., Valind, S., Winblad, B., et al. (1997). No difference in cerebral glucose metabolism in patients with Alzheimer disease and differing apolipoprotein E genotypes. Arch. Neurol. 54, 273–277.
De Frias, C. M., Schaie, K. W., and Willis, S. L. (2014). Hypertension moderates the effect of APOE on 21-year cognitive trajectories. Psychol. Aging 29, 431–439. doi: 10.1037/a0036828
De Leeuw, F. E., Richard, F., De Groot, J. C., Van Duijn, C. M., Hofman, A., Van Gijn, J., et al. (2004). Interaction between hypertension, apoE, and cerebral white matter lesions. Stroke 35, 1057–1060.
De Leon, M. J., Convit, A., Wolf, O. T., Tarshish, C. Y., Desanti, S., Rusinek, H., et al. (2001). Prediction of cognitive decline in normal elderly subjects with 2-[(18)F]fluoro-2-deoxy-D-glucose/poitron-emission tomography (FDG/PET). Proc. Natl. Acad. Sci. U.S.A. 98, 10966–10971.
Den Heijer, T., Launer, L. J., Prins, N. D., Van Dijk, E. J., Vermeer, S. E., Hofman, A., et al. (2005). Association between blood pressure, white matter lesions, and atrophy of the medial temporal lobe. Neurology 64, 263–267.
Dubois, B., Feldman, H. H., Jacova, C., Dekosky, S. T., Barberger-Gateau, P., Cummings, J., et al. (2007). Research criteria for the diagnosis of Alzheimer’s disease: revising the NINCDS-ADRDA criteria. Lancet Neurol. 6, 734–746.
Folstein, M. F., Folstein, S. E., and Mchugh, P. R. (1975). Mini-mental state. A practical method for grading the cognitive state of patients for the clinician. J. Psychiatr. Res. 12, 189–198.
Handelmann, G. E., Boyles, J. K., Weisgraber, K. H., Mahley, R. W., and Pitas, R. E. (1992). Effects of apolipoprotein E, beta-very low density lipoproteins, and cholesterol on the extension of neurites by rabbit dorsal root ganglion neurons in vitro. J. Lipid Res. 33, 1677–1688.
Horsburgh, K., Kelly, S., Mcculloch, J., Higgins, G. A., Roses, A. D., and Nicoll, J. A. (1999). Increased neuronal damage in apolipoprotein E-deficient mice following global ischaemia. Neuroreport 10, 837–841.
Jagust, W. J., Bandy, D., Chen, K., Foster, N. L., Landau, S. M., Mathis, C. A., et al. (2010). The Alzheimer’s disease neuroimaging initiative positron emission tomography core. Alzheimers Dement. 6, 221–229. doi: 10.1016/j.jalz.2010.03.003
Jeon, S. Y., Byun, M. S., Yi, D., Lee, J. H., Choe, Y. M., Ko, K., et al. (2019). Influence of hypertension on brain amyloid deposition and Alzheimer’s disease signature neurodegeneration. Neurobiol. Aging 75, 62–70. doi: 10.1016/j.neurobiolaging.2018.11.001
Kester, M. I., Van Der Flier, W. M., Mandic, G., Blankenstein, M. A., Scheltens, P., and Muller, M. (2010). Joint effect of hypertension and APOE genotype on CSF biomarkers for Alzheimer’s disease. J. Alzheimers Dis. 20, 1083–1090. doi: 10.3233/JAD-2010-091198
Korf, E. S., White, L. R., Scheltens, P., and Launer, L. J. (2004). Midlife blood pressure and the risk of hippocampal atrophy: the Honolulu Asia aging study. Hypertension 44, 29–34.
Landau, S. M., Harvey, D., Madison, C. M., Koeppe, R. A., Reiman, E. M., Foster, N. L., et al. (2011). Associations between cognitive, functional, and FDG-PET measures of decline in AD and MCI. Neurobiol. Aging 32, 1207–1218. doi: 10.1016/j.neurobiolaging.2009.07.002
Leoni, V. (2011). The effect of apolipoprotein E (ApoE) genotype on biomarkers of amyloidogenesis, tau pathology and neurodegeneration in Alzheimer’s disease. Clin. Chem. Lab. Med. 49, 375–383. doi: 10.1515/CCLM.2011.088
Li, Y., Rinne, J. O., Mosconi, L., Pirraglia, E., Rusinek, H., Desanti, S., et al. (2008). Regional analysis of FDG and PIB-PET images in normal aging, mild cognitive impairment, and Alzheimer’s disease. Eur. J. Nucl. Med. Mol. Imaging 35, 2169–2181. doi: 10.1007/s00259-008-0833-y
Lowe, V. J., Weigand, S. D., Senjem, M. L., Vemuri, P., Jordan, L., Kantarci, K., et al. (2014). Association of hypometabolism and amyloid levels in aging, normal subjects. Neurology 82, 1959–1967. doi: 10.1212/WNL.0000000000000467
Morris, J. C. (1993). The clinical dementia rating (CDR): current version and scoring rules. Neurology 43, 2412–2414.
Nathan, B. P., Bellosta, S., Sanan, D. A., Weisgraber, K. H., Mahley, R. W., and Pitas, R. E. (1994). Differential effects of apolipoproteins E3 and E4 on neuronal growth in vitro. Science 264, 850–852.
Oberlin, L. E., Manuck, S. B., Gianaros, P. J., Ferrell, R. E., Muldoon, M. F., Jennings, J. R., et al. (2015). Blood pressure interacts with APOE epsilon4 to predict memory performance in a midlife sample. Neuropsychology 29, 693–702. doi: 10.1037/neu0000177
Peila, R., White, L. R., Petrovich, H., Masaki, K., Ross, G. W., Havlik, R. J., et al. (2001). Joint effect of the APOE gene and midlife systolic blood pressure on late-life cognitive impairment: the Honolulu-Asia aging study. Stroke 32, 2882–2889.
Petrovitch, H., White, L. R., Izmirilian, G., Ross, G. W., Havlik, R. J., Markesbery, W., et al. (2000). Midlife blood pressure and neuritic plaques, neurofibrillary tangles, and brain weight at death: the HAAS. Honolulu-Asia aging study. Neurobiol. Aging 21, 57–62.
Reiman, E. M., Caselli, R. J., Yun, L. S., Chen, K., Bandy, D., Minoshima, S., et al. (1996). Preclinical evidence of Alzheimer’s disease in persons homozygous for the epsilon 4 allele for apolipoprotein E. N. Engl. J. Med. 334, 752–758.
Reiman, E. M., Chen, K., Alexander, G. E., Caselli, R. J., Bandy, D., Osborne, D., et al. (2004). Functional brain abnormalities in young adults at genetic risk for late-onset Alzheimer’s dementia. Proc. Natl. Acad. Sci. U.S.A. 101, 284–289.
Reiman, E. M., Chen, K., Alexander, G. E., Caselli, R. J., Bandy, D., Osborne, D., et al. (2005). Correlations between apolipoprotein E epsilon4 gene dose and brain-imaging measurements of regional hypometabolism. Proc. Natl. Acad. Sci. U.S.A. 102, 8299–8302.
Safieh, M., Korczyn, A. D., and Michaelson, D. M. (2019). ApoE4: an emerging therapeutic target for Alzheimer’s disease. BMC Med. 17:64. doi: 10.1186/s12916-019-1299-4
Samuraki, M., Matsunari, I., Chen, W. P., Shima, K., Yanase, D., Takeda, N., et al. (2012). Glucose metabolism and gray-matter concentration in apolipoprotein E epsilon4 positive normal subjects. Neurobiol. Aging 33, 2321–2323. doi: 10.1016/j.neurobiolaging.2011.11.020
Small, G. W., Mazziotta, J. C., Collins, M. T., Baxter, L. R., Phelps, M. E., Mandelkern, M. A., et al. (1995). Apolipoprotein E type 4 allele and cerebral glucose metabolism in relatives at risk for familial Alzheimer disease. JAMA 273, 942–947.
Sparks, D. L., Scheff, S. W., Liu, H., Landers, T. M., Coyne, C. M., and Hunsaker, J. C. III (1995). Increased incidence of neurofibrillary tangles (NFT) in non-demented individuals with hypertension. J. Neurol. Sci. 131, 162–169.
Keywords: brain glucose metabolism, FDG-PET, hypertension, APOE4, longitudinal study
Citation: Zhou R, Chen H, Ye F, Huang S and Zhang J (2020) Influence of Hypertension on Longitudinal Changes in Brain Glucose Metabolism Was Modified by the APOE4 Allele Among Cognitively Normal Older Individuals. Front. Aging Neurosci. 12:85. doi: 10.3389/fnagi.2020.00085
Received: 22 August 2019; Accepted: 12 March 2020;
Published: 02 April 2020.
Edited by:
Nibaldo C. Inestrosa, Pontifical Catholic University of Chile, ChileCopyright © 2020 Zhou, Chen, Ye, Huang and Zhang. This is an open-access article distributed under the terms of the Creative Commons Attribution License (CC BY). The use, distribution or reproduction in other forums is permitted, provided the original author(s) and the copyright owner(s) are credited and that the original publication in this journal is cited, in accordance with accepted academic practice. No use, distribution or reproduction is permitted which does not comply with these terms.
*Correspondence: Shiwei Huang, MTM3MzYzNjAyOTFAMTYzLmNvbQ==; Jie Zhang, amF5emhhbmcxMDE0QGdtYWlsLmNvbQ==
†Data used in preparation of this article were obtained from the Alzheimer’s Disease Neuroimaging Initiative (ADNI) database (adni.loni.usc.edu). As such, the investigators within the ADNI contributed to the design and implementation of ADNI and/or provided data but did not participate in analysis or writing of this report. A complete listing of ADNI investigators can be found at: http://adni.loni.usc.edu/wp-content/uploads/how_to_apply/ADNI_Acknowledgement_List.pdf