- 1Sanquin Research and Landsteiner Laboratory, Department of Hematopoiesis, Amsterdam UMC, University of Amsterdam, Amsterdam, Netherlands
- 2Department of Cell Biology and Genetics, Erasmus University Medical Center, Rotterdam, Netherlands
- 3Integrative Biology of Marine Models, Station Biologique de Roscoff, CNRS, UMR 8227, Sorbonne Université, Roscoff Cedex, France
- 4Laboratoire d’Excellence GR-Ex, Paris, France
- 5Pathophysiology of Anemia Unit, Hematology Unit, Fondazione IRCCS Ca’ Granda Ospedale Maggiore Policlinico of Milan, Milan, Italy
- 6Theoretical Medicine and Biosciences, Medical Faculty, Saarland University, Homburg, Germany
- 7Dynamics of Fluids, Experimental Physics, Saarland University, Saarbrücken, Germany
Erythrocytes represent at least 60% of all cells in the human body. During circulation, they experience a huge variety of physical and chemical stimulations, such as pressure, shear stress, hormones or osmolarity changes. These signals are translated into cellular responses through ion channels that modulate erythrocyte function. Ion channels in erythrocytes are only recently recognized as utmost important players in physiology and pathophysiology. Despite this awareness, their signaling, interactions and concerted regulation, such as the generation and effects of “pseudo action potentials”, remain elusive. We propose a systematic, conjoined approach using molecular biology, in vitro erythropoiesis, state-of-the-art electrophysiological techniques, and channelopathy patient samples to decipher the role of ion channel functions in health and disease. We need to overcome challenges such as the heterogeneity of the cell population (120 days lifespan without protein renewal) or the access to large cohorts of patients. Thereto we will use genetic manipulation of progenitors, cell differentiation into erythrocytes, and statistically efficient electrophysiological recordings of ion channel activity.
Introduction
Ion channels are crucial to feel, to see, to hear, to move, and to think. In other words, they are essential to live. They are important membrane transporters that have the singular capacity to generate transmembrane currents, and to convey signals that rapidly modify the properties of a cell on a time scale of the order of μ-seconds. Their function is evident in sensory organs, including the skin, and excitable cells such as neurons, where they are the core of signal transduction. Remarkably, evolution has conserved ion channels in all cell types, also in red blood cells (RBCs). This may not be surprising because ion channels are important to sense shear stress, so called mechano-sensors. RBCs have to “feel” shear stress when they pass the capillary bed in the tissues, and during quality control when they pass the spleen and have to slide through slits between endothelial cells. The 2021 Nobel prize for medicine or physiology placed the mechano-sensor Piezo1, a nonspecific cation channel, in the spotlights.
Although RBCs were among the first cell types used to study ion channels with the patch-clamp technique (Hamill, 1981, 1983), researcher quickly lost interest in the topic, i.e., after a number of initial publications in the first half of the 1980s, for 15 years almost no work about RBC ion channels was published. Indeed, especially human RBC presented a number of bottlenecks such as the limited copy number of channels per cell (Grygorczyk et al., 1984; Egée et al., 2002; Bennekou et al., 2003; Kaestner, 2015) and the impossibility to experimentally manipulate them genetically which made their study in this aspect complex and difficult. Therefore, most of the information available today is scattered, contradictory and does not permit the reconstruction of the whole jigsaw puzzle that would reveal the role of ion channels in the function of the RBCs. This holds true, especially in the context that ion channels in RBCs are still being discovered, like the transient receptor potential channel TRPV2 (Belkacemi et al., 2021; Egee and Kaestner, 2021) suggesting that further ion channels can be discovered, or new electrophysiological effects like “pseudo action potentials,” which are found in RBCs (Jansen et al., 2021) and awaiting their full physiological explanation.
Functional Evidence for Ion Channels in RBCs
There is a growing body of evidence that ion channels play an essential role in RBCs from their terminal differentiation in the bone marrow (Moura et al., 2019; Caulier et al., 2020), during their enucleation, and during the subsequent 120 days that they circulate in the bloodstream (Moras et al., 2017) and pass through capillaries sometimes much smaller than their own diameters (Faucherre et al., 2013; Cahalan et al., 2015; Danielczok et al., 2017). Mature RBCs cannot renew their proteins. Therefore, ion channels have to function or gradually lose their function within the 120 days (in humans) until the cells are removed from the circulation in the spleen (Deplaine et al., 2011). This generates a tremendous functional heterogeneity (Wang et al., 2013; Bogdanova et al., 2020).
In RBCs, activation of Piezo1 results in Ca2+ influx, which activates the Gárdos channel to release K+ from the cells and subsequently results in export of H2O. This rapid, transient process facilitates the cells to move through narrow passages. Congenital mutations in Piezo1 and the Gárdos channel were found in hemolytic anemia and unexpected iron overload, underscoring the importance of this ion channel interaction in RBCs. The role of most other ion channels remains unexplored.
Technological Progress and Advancements of the Recent Years
Limitations and technological bottlenecks that have hitherto hindered the study of ion channels in RBCs are just about to be overcome: RBCs can be analyzed by automated high throughput patch-clamp robots (Rotordam et al., 2019), in vitro erythropoiesis is mastered up to mature erythrocytes (Heshusius et al., 2019) and available for systematic genetic screens (Verhagen et al., 2021), and significant cohorts of genotyped/phenotyped patients with channelopathies are available.
Transcriptomic (An et al., 2014) and proteomic (Gautier et al., 2016; Karayel et al., 2020) studies, clearly show that ion channels are not only differentially expressed upon differentiation but also selectively sorted during the enucleation step before the release of reticulocytes within the bloodstream. RBC production is qualitatively impaired in hereditary hemolytic anemia, linked directly or indirectly to ion channel defects (Moura et al., 2019; Caulier et al., 2020). This suggests that ion channels are critical regulators during the 2-week period required for complete maturation of a precursor culture to enucleated, hemoglobinized RBCs and for their function in the circulation. An accurate knowledge of the role of these channels is paramount for our understanding of erythropoiesis, especially as no less than 80 genes that are directly linked to membrane transport are expressed during erythropoiesis, including at least a dozen ion channels (Karayel et al., 2020). Tackling this knowledge gap will result in a major advance in the understanding and personalized management of rare inherited diseases but also in the role played by transport defects in much more common diseases such as sickle cell disease.
RBC Ion Channels in Molecular Signaling
Enucleated RBCs have evolved to optimize the transport of O2 and CO2. These cells are unique as they have a membrane potential dictated by anions (in contrast to almost all other eukaryotic cells) to ensure optimal CO2 transport (VM = −12 mV; Thomas et al., 2011). Consequently, RBCs must maintain their cationic permeability (Na+, K+, and Ca2+) at the lowest possible level to survive. Having a permeability tightly under control can be seen as a major advantage in terms of signaling because the simple flux of a few cations generates a variation in membrane potential equivalent to the variations recorded during a neuronal action potential (Dyrda et al., 2010; Jansen et al., 2021). The role of these “pseudo action potentials” occurring in RBCs is completely unknown. Importantly, ion channel activity becomes a threat if the fine-tuned equilibrium is disrupted by a genetic defect (Lew et al., 2002; Bogdanova et al., 2013; Rapetti-Mauss et al., 2017) or any other external signal (Li et al., 1996; Kaestner et al., 2004; Föller et al., 2008; Bogdanova et al., 2013; Wang et al., 2021). The dissipative power of the ion channels will immediately compromise the survival of the RBCs by altering their volume, shape, and deformability, which are the hallmarks of the longevity of RBCs within the circulation. The last decade has been marked by the discovery of several causative genes for hereditary RBC diseases (PIEZO1, Zarychanski et al., 2012, KCNN4, Glogowska et al., 2015; Rapetti-Mauss et al., 2015, and RhAG, Stewart et al., 2011). Their mutation alters the ionic balance directly (Rapetti-Mauss et al., 2015; Fermo et al., 2017, 2020; Hertz et al., 2017; Filser et al., 2020; Pérès et al., 2021) or indirectly as the result of deregulated ion channel activity (Barneaud-Rocca et al., 2011; Flatt et al., 2011). It has been hypothesized that incidence of this group of disorders may be underestimated and could affect one in 8,000 adults (Kaufman et al., 2018).
Required Technological and Organizational Innovations
To obtain a better and more complete picture of the role of ion channels in RBCs, further efforts are required. Here, we propose two complementary concepts that need to be combined: (I) high throughput measurement of ion channel activity and membrane potential, and (II) the in vitro culture and maturation of genetically manipulated RBCs.
I: Automated patch-clamp technology became available to scientific community within the past 10–15 years. Due to the intrinsic properties of RBCs to pass small constrictions, e.g., capillaries or slits in the spleen, make them difficult to patch, i.e., seal them tightly on a small artificial opening (giga-seal formation). What is well described and required a lot of initial research in manual patch-clamp (Kaestner, 2011), holds also true for automated approaches, although, the aperture geometry changed from micropipettes to mainly planar chips with a hole. Therefore protocols to measure ion conductance and membrane potential of RBCs in automated high throughput patch-clamp robots need the active collaboration of the manufacturers to optimize chip geometries for RBCs (Rotordam et al., 2019). However, such a patch-clamp robot is to our best knowledge not available in any hematological laboratory – worldwide, i.e., the technology is in principle available but cannot be used for regular RBC research. Part of the problem is the high costs of initial required investment, the still unique qualification required to run the machines and in this context the putative operating grade, which might not be high enough to justify such an investment. Furthermore, hard core electrophysiology and hard core cell biology laboratories are often spatially separated (different Universities, even different countries). Shipment of RBCs is problematic and likely to result in compromised measurements (Makhro et al., 2016; Hertz et al., 2017; Rotordam et al., 2019). Therefore, we propose a mobile concept of such a patch-clamp robot. Such an implementation is not just placing a machine in a van, it is more like a small version of a space-lab module with a high integration level between the vehicle and the delicate instrument as well as the associated equipment to work autonomously from an independent power supply to sample preparation equipment. Nevertheless, Nan]i[on Technologies regards it a feasible concept (Nils Fertig and Michael George, personal communication). In the following, we refer to this mobile patch-clamp robot concept as the “PatchC]l[amper.”
II: Furthermore, a systematic approach of RBC investigations combining functional electrophysiology with a genetic approach based on an extended scale patient screening as well as accompanying in vitro erythropoiesis is necessary. This would allow to implement various concepts of genetic manipulations to functionalize RBCs and to manipulate their properties for medical needs (knock-out or downregulation of ion channels, their mutations – including the results from patients screening – the overexpression of ion channels to address experimental difficulties due to their low copy number in the membrane or even the expression of new and heterologous channels). The in vitro erythropoiesis also allows RBC age synchronization overcoming the RBC age variation occurring in human samples.
Proposed Concrete Research Activities
The research innovations outlined above would require to be embedded into research activities to address the most urgent needs in RBC ion channel research. We propose three lines of activity as outlined below and summarized in Figure 1.
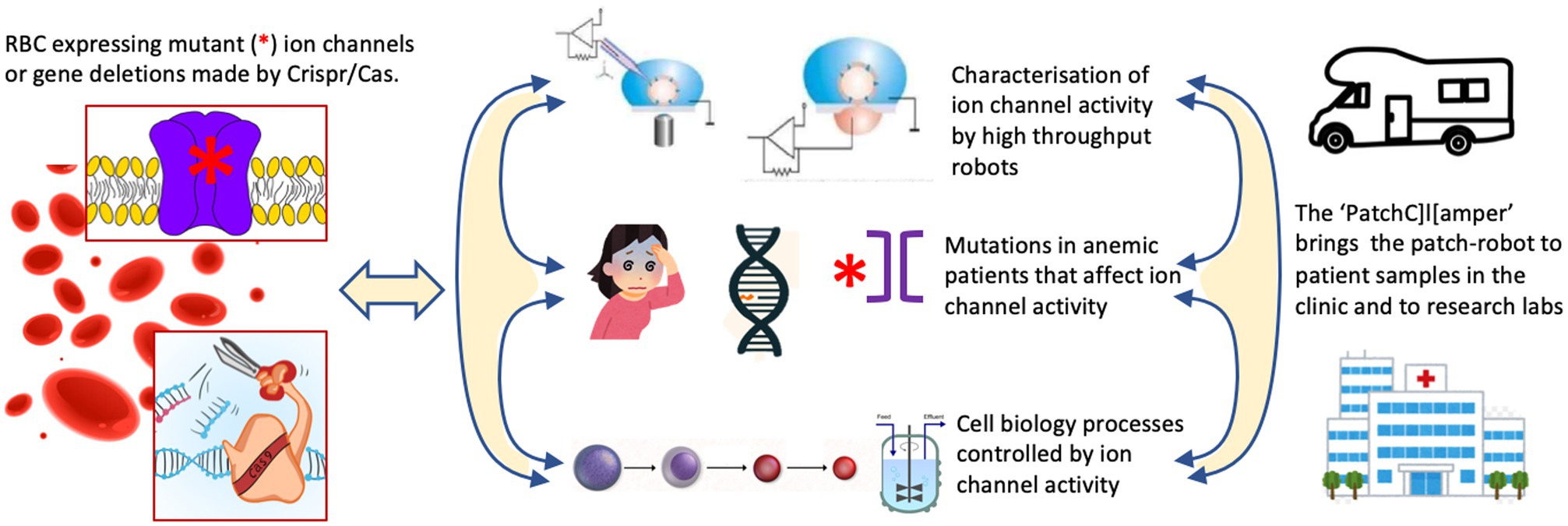
Figure 1. The proposed research activities to address the most urgent needs in red blood cell (RBC) ion channel research. One should make use of freshly isolated or cultured RBCs from healthy donors or channelopathy patients. Cultured precursors can be genetically modified to delete ion channels or to (over)express (mutated) ion channels in mature RBCs. This is the common basis for three conceptual research line activities. (i) The systematic investigation of RBC ion channels, their interactions, modulation by external factors and the induced signaling in the RBCs using classical patch-clamp and high throughput planer chips. (ii) Anemic patients suspected of channelopathies can be diagnosed by next generation sequencing (NGS) technology. This will allow to elucidate the molecular regulation of RBC channelopathies and RBC diseases with secondary involvement of ion channels. It should aid to develop more precise diagnosis and assessment of disease state, and future (personalized) therapeutic approaches. (iii) Although erythropoiesis is well documented for gene expression the functional activity of ion channels during the differentiation process needs to be investigated. Additionally, various gene-manipulation concepts should be applied and investigated in terms of cell function and efficiency of in vitro RBC production including the use of bioreactors.
To Understand the Regulation and Concerted Activity of Ion Channels in RBCs and to Unravel the Generation and Role of “Pseudo Action Potentials”
Based on the low K+ conductance of the RBC membrane, activations of the Gárdos channel (KCa3.1) result in a hyperpolarization of the membrane potential (Monedero Alonso et al., 2021). Because the Gárdos channel number per RBC is low (in average approximately 10 copies per cell) and due to the stochastic nature of the channel openings, membrane potential jumps (cycles of hyperpolarization and depolarizations) occur, an effect that we call “pseudo action potential”. These “pseudo action potentials” appear to induce the opening of voltage activated Ca2+ channels in RBCs (Kaestner et al., 2018; Jansen et al., 2021). This process could partly explain the increased intracellular Ca2+ in patients with gain of function mutations of the Gárdos channel (Fermo et al., 2017, 2020; Jansen et al., 2021). This “pseudo action potential” is a new and unique finding among non-excitable cells and requires further investigations concerning its contribution to the ion homeostasis, the elucidation of the associated Ca2+ signaling and the involvement of other ion channels as associated processes. Aside the potential use of the PatchC]l[amper, we propose the use of RBCs from patients and genetically modified RBCs and to combine it with other biophysical methods like confocal live cell imaging, microfluidic approaches, and mathematical modeling. This research activity would require a level of patch-clamp mobility involving, e.g., the laboratories of all authors of this Speciality Grand Challenge. As a result we expect to understand the role of transient transmembrane cation transport in the dynamical behavior of RBCs in the circulation, and how this sustains the lifespan of in average 120 days.
To Elucidate the Molecular and Functional Basis of Channelopathies in RBCs, as Well as the Secondary Involvement of Ion Channels in Congenital Anemia
We are only at the beginning to understand the role of ion channels as molecular regulators or modulators of RBC channelopathies and other RBC diseases, respectively. The availability of large patient cohorts allows to identify and characterize new genes involved in aberrant activation of ion channels, to clarify the pathogenetic mechanism of new variants identified in known genes and to verify the functional effects of the large number of “variants with unknown significance” (VUS) identified by next generation sequencing (NGS) approaches. A common treatment of hemolytic anemia is splenectomy. In hereditary xerocytosis (caused by a gain of function mutation in Piezo1), splenectomy results in a high incidence of thrombotic complications (Picard et al., 2019). One should make use of the available mouse model with a Piezo1 gain of function mutation (Ma et al., 2018) to investigate the effect of splenectomy on the flow behavior of RBCs in vivo, and the thrombus formation in an experimental setup. The knowledge gained in the animal model should be used to further investigate blood samples of splenectomized patients to endorse a better patient treatment and disease management. This research activity would require a broad involvement of patients and the maximal regional operation of the PatchC]l[amper, i.e., whenever the patient/hospital can be reached on road within a reasonable effort. For individual patients this is within Europe, where the RBC community is traditionally well connected, e.g., through the EuroBloodNet initiative, the European Red Cell Society (ERCS) and numerous projects funded by the European Commission. For the involvement of patient groups, e.g., malaria, sickle cell disease, or thalassemia, the PatchC]l[amper could also move to other continents like Africa or Asia. For transoceanic investigations, a second PatchC]l[amper would probably being a preferred solution. Overall, we expect novel causative genes to be found involved in deregulated ion channel activity, and to have better insight in the role of ion channels in disease modulation including thrombus formation.
To Assess the Role of Ion Channels During RBC Production (in Bioreactors)
We anticipate to bring automated high throughput patch-clamp recordings to the clinic and to the cell biology laboratories combined with the opportunity of bioreactor based in vitro erythropoiesis and the expertise in molecular biology to perform genetic manipulations. Recently, bioreactor based erythropoiesis progressed considerably (Hansen et al., 2019; Heshusius et al., 2019; Connor et al., 2021; Pellegrin et al., 2021), which showed the importance to understand channel activity (Piezo1) in this process (Aglialoro et al., 2021), especially since the cells in bioreactors are due to the nature of these reactors under constant flow or shear stress. Erythropoiesis under physiological conditions in the bone marrow occurs rather in the absence of flow, but crawling over macrophages may cause shear stress. Several groups work on the further improvement of bioreactor technology. The goal is to decrease the costs with the clear aim to allow the use of in vitro (designed) RBCs in transfusion medicine.
All these developments enable a unique systematic approach to identify and understand the role of ion channels during maturation and function of RBCs in health and disease. To this end, it is advisable to compare patient RBCs with known mutations with their counterparts generated in vitro to understand the ion channel contribution during cellular development and to create treatment concepts based on that knowledge. The inclusion of multi-omics offers the possibility to identify cellular processes controlled by ion fluxes and membrane potential. One can in principle test the physical and biological effect of novel mutations and one can explore how to control ion channel activity during the large scale production of cultured RBCs for transfusion purposes in a stirred bioreactor. There is the option to characterize ion channel activity during terminal differentiation of RBC precursors to mature, functional cells. Furthermore, we have the opportunity to confirm or negate the role of VUS in ion channels detected by NGS in anemia patients.
Summary
The research concept described above enables a unique systematic approach to identify and understand the role of ion channels during maturation and function of RBCs in health and disease and we expect to understand how ion channel activity has to be regulated during the production of cultured blood to obtain functional RBCs for transfusion purposes.
Author Contributions
ML, SE, PB, and LK jointly wrote the manuscript and agreed to be accountable for the content of the work. All authors contributed to the article and approved the submitted version.
Conflict of Interest
The authors declare that the research was conducted in the absence of any commercial or financial relationships that could be construed as a potential conflict of interest.
Publisher’s Note
All claims expressed in this article are solely those of the authors and do not necessarily represent those of their affiliated organizations, or those of the publisher, the editors and the reviewers. Any product that may be evaluated in this article, or claim that may be made by its manufacturer, is not guaranteed or endorsed by the publisher.
Acknowledgments
The authors would like to thank Dr. Sonja Bergner for superb technical support in compiling the text of the manuscript.
References
Aglialoro, F., Abay, A., Yagci, N., Rab, M. A. E., Kaestner, L., van Wijk, R., et al. (2021). Mechanical stress induces Ca2+ dependent signal transduction in erythroblasts and modulates erythropoiesis. Int. J. Mol. Sci. 22:955. doi: 10.3390/ijms22020955
Monedero Alonso, D., Pérès, L., Hatem, A., Bouyer, G., and Egée, S. (2021). The chloride conductance inhibitor NS3623 enhances the activity of a non-selective cation channel in hyperpolarizing conditions. Front. Physiol. 12:743094. doi: 10.3389/fphys.2021.743094
An, X., Schulz, V. P., Li, J., Wu, K., Liu, J., Xue, F., et al. (2014). Global transcriptome analyses of human and murine terminal erythroid differentiation. Blood 123, 3466–3477. doi: 10.1182/blood-2014-01-548305
Barneaud-Rocca, D., Pellissier, B., Borgese, F., and Guizouarn, H. (2011). Band 3 missense mutations and stomatocytosis: insight into the molecular mechanism responsible for monovalent cation leak. Int. J. Cell Biol. 2011:136802. doi: 10.1155/2011/136802
Belkacemi, A., Trost, C. F., Tinschert, R., Flormann, D., Malihpour, M., Wagner, C., et al. (2021). The TRPV2 channel mediates Ca2+ influx and the D9-THC-dependent decrease in osmotic fragility in red blood cells. Haematologica 106, 2246–2250. doi: 10.3324/haematol.2020.274951
Bennekou, P., Kristensen, B. I., and Christophersen, P. (2003). The human red cell voltage-regulated cation channel. The interplay with the chloride conductance, the Ca(2+)-activated K(+) channel and the Ca(2+) pump. J. Membr. Biol. 195, 1–8. doi: 10.1007/s00232-003-2036-6
Bogdanova, A., Kaestner, L., Simionato, G., Wickrema, A., and Makhro, A. (2020). Heterogeneity of red blood cells: causes and consequences. Front. Physiol. 11:392. doi: 10.3389/fphys.2020.00392
Bogdanova, A., Makhro, A., Wang, J., Lipp, P., and Kaestner, L. (2013). Calcium in red blood cells-a perilous balance. Int. J. Mol. Sci. 14, 9848–9872. doi: 10.3390/ijms14059848
Cahalan, S. M., Lukacs, V., Ranade, S. S., Chien, S., Bandell, M., and Patapoutian, A. (2015). Piezo1 links mechanical forces to red blood cell volume. Elife 4:e07370. doi: 10.7554/eLife.07370
Caulier, A., Jankovsky, N., Demont, Y., Ouled-Haddou, H., Demagny, J., Guitton, C., et al. (2020). PIEZO1 activation delays erythroid differentiation of normal and hereditary xerocytosis-derived human progenitor cells. Haematologica 105, 610–622. doi: 10.3324/haematol.2019.218503
Connor, K., Fannin, M., Kent, J., and Toye, A. (2021). Blood culture: reimag(in)ing life at a cellular scale. Interdiscip. Sci. Rev. 46, 655–676. doi: 10.1080/03080188.2020.1855377
Danielczok, J. G., Terriac, E., Hertz, L., Petkova-Kirova, P., Lautenschläger, F., Laschke, M. W., et al. (2017). Red blood cell passage of small capillaries is associated with transient Ca2+-mediated adaptations. Front. Physiol. 8:979. doi: 10.3389/fphys.2017.00979
Deplaine, G., Safeukui, I., Jeddi, F., Lacoste, F., Brousse, V., Perrot, S., et al. (2011). The sensing of poorly deformable red blood cells by the human spleen can be mimicked in vitro. Blood 117, e88–e95. doi: 10.1182/blood-2010-10-312801
Dyrda, A., Cytlak, U., Ciuraszkiewicz, A., Lipinska, A., Cueff, A., Bouyer, G., et al. (2010). Local membrane deformations activate Ca2+-dependent K+ and anionic currents in intact human red blood cells. PLoS One 5:e9447. doi: 10.1371/journal.pone.0009447
Egee, S., and Kaestner, L. (2021). The transient receptor potential vanilloid type 2 (TRPV2) channel—a new druggable Ca2+ pathway in red cells, implications for red cell ion homeostasis. Front. Physiol. 12:677573. doi: 10.3389/fphys.2021.677573
Egée, S., Lapaix, F., Decherf, G., Staines, H. M., Ellory, J. C., Doerig, C., et al. (2002). A stretch-activated anion channel is up-regulated by the malaria parasite Plasmodium falciparum. J. Physiol. 542, 795–801. doi: 10.1113/jphysiol.2002.022970
Faucherre, A., Kissa, K., Nargeot, J., Mangoni, M., and Jopling, C. (2013). Piezo1 plays a role in erythrocyte volume homeostasis. Haematologica 99, 70–75. doi: 10.3324/haematol.2013.086090
Fermo, E., Bogdanova, A., Petkova-Kirova, P., Zaninoni, A., Marcello, A. P., Makhro, A., et al. (2017). “Gardos Channelopathy”: a variant of hereditary stomatocytosis with complex molecular regulation. Sci. Rep. 7:1744. doi: 10.1038/s41598-017-01591-w
Fermo, E., Monedero-Alonso, D., Petkova-Kirova, P., Makhro, A., Pérès, L., Bouyer, G., et al. (2020). Gardos channelopathy: functional analysis of a novel KCNN4 variant. Blood Adv. 4, 6336–6341. doi: 10.1182/bloodadvances.2020003285
Filser, M., Giansily-Blaizot, M., Grenier, M., Monedero Alonso, D., Bouyer, G., Peres, L., et al. (2020). Increased incidence of germline PIEZO1 mutations in individuals with idiopathic erythrocytosis. Blood 137, 1828–1832. doi: 10.1182/blood.2020008424
Flatt, J. F., Guizouarn, H., Burton, N. M., Borgese, F., Tomlinson, R. J., Forsyth, R. J., et al. (2011). Stomatin-deficient cryohydrocytosis results from mutations in SLC2A1: a novel form of GLUT1 deficiency syndrome. Blood 118, 5267–5277. doi: 10.1182/blood-2010-12-326645
Föller, M., Huber, S. M., and Lang, F. (2008). Erythrocyte programmed cell death. IUBMB Life 60, 661–668. doi: 10.1002/iub.106
Gautier, E.-F., Ducamp, S., Leduc, M., Salnot, V., Guillonneau, F., Dussiot, M., et al. (2016). Comprehensive proteomic analysis of human erythropoiesis. Cell Rep. 16, 1470–1484. doi: 10.1016/j.celrep.2016.06.085
Glogowska, E., Lezon-Geyda, K., Maksimova, Y., Schulz, V. P., and Gallagher, P. G. (2015). Mutations in the Gardos channel (KCNN4) are associated with hereditary xerocytosis. Blood 126, 1281–1284. doi: 10.1182/blood-2015-07-657957
Grygorczyk, R., Schwarz, W., and Passow, H. (1984). Ca2+-activated K+ channels in human red cells. Comparison of single-channel currents with ion fluxes. Biophys. J. 45, 693–698. doi: 10.1016/S0006-3495(84)84211-3
Hamill, O. P. (1981). Potassium channel currents in human red blood cells. J. Physiol. 319, 97P–98P.
Hamill, O. P. (1983). “Potassium and chloride channels in red blood cells,” in Single Channel Recording. eds. B. Sakmann and E. Neher (New York: Plenum Press), 451–471.
Hansen, M., von Lindern, M., van den Akker, E., and Varga, E. (2019). Human-induced pluripotent stem cell-derived blood products: state of the art and future directions. FEBS Lett. 593, 3288–3303. doi: 10.1002/1873-3468.13599
Hertz, L., Huisjes, R., Llaudet-Planas, E., Petkova-Kirova, P., Makhro, A., Danielczok, J. G., et al. (2017). Is increased intracellular calcium in red blood cells a common component in the molecular mechanism causing anemia? Front. Physiol. 8:673. doi: 10.3389/fphys.2017.00673
Heshusius, S., Heideveld, E., Burger, P., Thiel-Valkhof, M., Sellink, E., Varga, E., et al. (2019). Large-scale in vitro production of red blood cells from human peripheral blood mononuclear cells. Blood Adv. 3, 3337–3350. doi: 10.1182/bloodadvances.2019000689
Jansen, J., Qiao, M., Hertz, L., Wang, X., Fermo, E., Zaninoni, A., et al. (2021). Mechanistic ion channel interactions in red cells of patients with Gárdos channelopathy. Blood Adv. 5, 3303–3308. doi: 10.1182/bloodadvances.2020003823
Kaestner, L. (2011). Cation channels in erythrocytes—historical and future perspective. Open Biol. J. 4, 27–34. doi: 10.2174/1874196701104010027
Kaestner, L. (2015). Channelizing the red blood cell: molecular biology competes with patch-clamp. Front. Mol. Biosci. 2:46. doi: 10.3389/fmolb.2015.00046
Kaestner, L., Tabellion, W., Lipp, P., and Bernhardt, I. (2004). Prostaglandin E2 activates channel-mediated calcium entry in human erythrocytes: an indication for a blood clot formation supporting process. Thromb. Haemost. 92, 1269–1272. doi: 10.1160/TH04-06-0338
Kaestner, L., Wang, X., Hertz, L., and Bernhardt, I. (2018). Voltage-activated ion channels in non-excitable cells-a viewpoint regarding their physiological justification. Front. Physiol. 9:450. doi: 10.3389/fphys.2018.00450
Karayel, Ö., Xu, P., Bludau, I., Bhoopalan, S. V., Yao, Y., Rita, F. C. A., et al. (2020). Integrative proteomics reveals principles of dynamic phosphosignaling networks in human erythropoiesis. Mol. Syst. Biol. 16:e9813. doi: 10.15252/msb.20209813
Kaufman, H. W., Niles, J. K., Gallagher, D. R., Rivera, A., Alper, S. L., Brugnara, C., et al. (2018). Revised prevalence estimate of possible hereditary xerocytosis as derived from a large U.S. laboratory database. Am. J. Hematol. 93, E9–E12. doi: 10.1002/ajh.24923
Lew, V. L., Etzion, Z., and Bookchin, R. M. (2002). Dehydration response of sickle cells to sickling-induced Ca(++) permeabilization. Blood 99, 2578–2585. doi: 10.1182/blood.V99.7.2578
Li, Q., Jungmann, V., Kiyatkin, A., and Low, P. S. (1996). Prostaglandin E2 stimulates a Ca2+-dependent K+ channel in human erythrocytes and alters cell volume and filterability. J. Biol. Chem. 271, 18651–18656. doi: 10.1074/jbc.271.31.18651
Ma, S., Cahalan, S., LaMonte, G., Grubaugh, N. D., Zeng, W., Murthy, S. E., et al. (2018). Common PIEZO1 allele in African populations causes RBC dehydration and attenuates plasmodium infection. Cell 173, 443.e12–455.e12. doi: 10.1016/j.cell.2018.02.047
Makhro, A., Huisjes, R., Verhagen, L. P., Mañú-Pereira, M. M., Llaudet-Planas, E., Petkova-Kirova, P., et al. (2016). Red cell properties after different modes of blood transportation. Front. Physiol. 7:288. doi: 10.3389/fphys.2016.00288
Moras, M., Lefevre, S. D., and Ostuni, M. A. (2017). From erythroblasts to mature red blood cells: organelle clearance in mammals. Front. Physiol. 8:1076. doi: 10.3389/fphys.2017.01076
Moura, P. L., Hawley, B. R., Dobbe, J. G. G., Streekstra, G. J., Rab, M. A. E., Bianchi, P., et al. (2019). PIEZO1 gain-of-function mutations delay reticulocyte maturation in hereditary xerocytosis. Haematologica 105, e268–e271. doi: 10.3324/haematol.2019.231159
Pellegrin, S., Severn, C. E., and Toye, A. M. (2021). Towards manufactured red blood cells for the treatment of inherited anemia. Haematologica 106, 2304–2311. doi: 10.3324/haematol.2020.268847
Pérès, L., Monedero Alonso, D., Nudel, M., Figeac, M., Bruge, J., Sebda, S., et al. (2021). Characterisation of Asp669Tyr Piezo1 cation channel activity in red blood cells: an unexpected phenotype. Br. J. Haematol. 194, e51–e55. doi: 10.1111/bjh.17467
Picard, V., Guitton, C., Thuret, I., Rose, C., Bendelac, L., Ghazal, K., et al. (2019). Clinical and biological features in PIEZO1-hereditary xerocytosis and Gardos channelopathy: a retrospective series of 126 patients. Haematologica 104, 1554–1564. doi: 10.3324/haematol.2018.205328
Rapetti-Mauss, R., Lacoste, C., Picard, V., Guitton, C., Lombard, E., Loosveld, M., et al. (2015). A mutation in the Gardos channel is associated with hereditary xerocytosis. Blood 126, 1273–1280. doi: 10.1182/blood-2015-04-642496
Rapetti-Mauss, R., Picard, V., Guitton, C., Ghazal, K., Proulle, V., Badens, C., et al. (2017). Red blood cell Gardos channel (KCNN4): the essential determinant of erythrocyte dehydration in hereditary xerocytosis. Haematologica 102, e415–e418. doi: 10.3324/haematol.2017.171389
Rotordam, G. M., Fermo, E., Becker, N., Barcellini, W., Brüggemann, A., Fertig, N., et al. (2019). A novel gain-of-function mutation of Piezo1 is functionally affirmed in red blood cells by high-throughput patch clamp. Haematologica 104, e179–e183. doi: 10.3324/haematol.2018.201160
Stewart, A. K., Shmukler, B. E., Vandorpe, D. H., Rivera, A., Heneghan, J. F., Li, X., et al. (2011). Loss-of-function and gain-of-function phenotypes of stomatocytosis mutant RhAG F65S. Am. J. Phys. Cell Physiol. 301, C1325–C1343. doi: 10.1152/ajpcell.00054.2011
Thomas, S. L. Y., Bouyer, G., Cueff, A., Egée, S., Glogowska, E., and Ollivaux, C. (2011). Ion channels in human red blood cell membrane: actors or relics? Blood Cells Mol. Dis. 46, 261–265. doi: 10.1016/j.bcmd.2011.02.007
Verhagen, H. J. M. P., Kuijk, C., Kokke, A. M., Rutgers, L., Meulen, S. A., Mierlo, G., et al. (2021). Optimized guide RNA selection recommendations for using spCas9 gene editing in human hematopoietic stem and progenitor cells. Biorxiv [Preprint]. doi: 10.1101/2020.12.18.423329
Wang, J., Hertz, L., Ruppenthal, S., Nemer, W. E., Connes, P., Goede, J. S., et al. (2021). Lysophosphatidic acid-activated calcium signaling is elevated in red cells from sickle cell disease patients. Cells 10:456. doi: 10.3390/cells10020456
Wang, J., Wagner-Britz, L., Bogdanova, A., Ruppenthal, S., Wiesen, K., Kaiser, E., et al. (2013). Morphologically homogeneous red blood cells present a heterogeneous response to hormonal stimulation. PLoS One 8:e67697. doi: 10.1371/journal.pone.0067697
Keywords: erythrocyte, erythropoiesis, patch-clamp, ion channel, electrophysiology, channelopathy, genotype-phenotype correlation, pseudo action potential
Citation: von Lindern M, Egée S, Bianchi P and Kaestner L (2022) The Function of Ion Channels and Membrane Potential in Red Blood Cells: Toward a Systematic Analysis of the Erythroid Channelome. Front. Physiol. 13:824478. doi: 10.3389/fphys.2022.824478
Edited and reviewed by:
Constance Tom Noguchi, National Institutes of Health (NIH), United StatesCopyright © 2022 von Lindern, Egée, Bianchi and Kaestner. This is an open-access article distributed under the terms of the Creative Commons Attribution License (CC BY). The use, distribution or reproduction in other forums is permitted, provided the original author(s) and the copyright owner(s) are credited and that the original publication in this journal is cited, in accordance with accepted academic practice. No use, distribution or reproduction is permitted which does not comply with these terms.
*Correspondence: Lars Kaestner, bGFyc19rYWVzdG5lckBtZS5jb20=