Smart Contracts for Sustainable Supply Chain Management: Conceptual Frameworks for Supply Chain Maturity Evaluation and Smart Contract Sustainability Assessment
- 1Department for Global Business and Trade, Institute for Transport and Logistics Management, Vienna University of Economics and Business, Vienna, Austria
- 2OMV Refining & Marketing GmbH, Schwechat, Austria
Current research on smart contracts focuses on technical, conceptual, and legal aspects but neglects organizational requirements and sustainability impacts. We consider this a significant research gap and explore the relationship between smart contracts and sustainability in supply chains. First, we define the concept of smart contracts in terms of supply chain management. Then, we conduct a content analysis of the literature to explore the overlapping research fields of smart contracts and sustainability in supply chains. Next, we develop a semi-structured assessment framework to model the potential environmental and social impacts induced by smart contracts on supply chains. We propose a conceptual framework for supply chain maturity by mapping the relationships between organizational development, sustainability, and technology. We identify smart contracts as a foundational technology that enables efficient and transparent governance and collaborative self-coordination of human and non-human actors. Thus, we argue that smart contracts can contribute to the economic and social development of networked value chains and Society 5.0. To stimulate interdisciplinary research on smart contracts, we conclude the article by formulating research propositions and trade-offs for smart contracts in the context of technology development, business process and supply chain management, and sustainability.
Introduction
The idea of sustainability dates back to forestry in the eighteenth century when increasing industrialization fueled the demand for wood. Forest owner and lumberjack Hans Carl von Carlowitz considered sustainability in the context of trees' natural reproduction rate to ensure the long-term survival of his enterprise (Carlowitz, 1713). While this first approach to sustainability focused on economic and ecological dimensions, later developments also considered social aspects (WCED, 1987). In 1994, Elkington coined the concept of the triple bottom line (TBL, Elkington, 2004). The integrative view on people, planet, and profit creates a complex interdependency system, leading to different literature interpretations. For instance, Seuring and Müller (2008, p. 1700) perceive the TBL as “a minimum performance […] to be achieved in the environmental, economic and social dimensions,” while Kirchherr et al. (2017, p. 224) formulate: the “three aims […] must be accomplished simultaneously.” Elkington (2018) himself recalls that the TBL's stated goal from the outset was the transformation of capitalism by introducing breakthrough change and asymmetric growth, rather than just introducing a novel accounting system.
Despite TBL's introduction over 25 years ago, significant environmental and social challenges remain unsolved. In context with the overuse of natural resources, Hardin (1968) describes the underlying problem as the “Tragedy of the Commons.” Commons-based resources are free of ownership (e.g., world climate, high seas); property rights are indistinct or cannot be enforced (Tornell and Velasco, 1992). Due to existing externalities, individual costs for exploiting the commons are lower than overall costs imposed on society. Therefore, individuals often behave against group interests (Loebbecke and Picot, 2015) and hinder the business case for sustainable products and services (Burrit and Christ, 2016). In addition to opportunistic behavior, lacking knowledge and skills lead to gradual sustainability adoption. For consumers, the effects of their individual choices are often nebulous due to complex sustainability implications and poor availability of information (Sörqvist and Langeborg, 2019). Companies are starting to recognize the benefits and competitive advantage by incorporating sustainability to a growing extent (Bonilla et al., 2018). Challenges in the development of CSR strategies, sustainable business models, and products comprise a necessary shift in conventional thought, additional competencies, technologies, and tools (Burrit and Christ, 2016).
Slow transformation toward sustainability leads to poor social performance in the current state of the climate crisis. The United Nations (2015) defines 17 Social Development Goals (SDGs) to address critical areas for humanity and our planet.1 To meet these SDGs, companies need to rethink and redesign their business strategy and operations to eliminate waste, use resources more efficiently, and contribute to communities (Tate et al., 2010). Furthermore, the evolving nature of Society 5.0 drives socially inclusive Industry 5.0. The envisioned “super-smart society” strives to create a “sustainable, inclusive socio-economic system, powered by digital technologies” (Sato, 2019, online). Convergent innovation concepts lead to integrative technological-social-institutional innovations and post-humanization through digitalization in social society, industrialized economy, and open institutions (Herneth, 2020). The adequate use of technological and social advancements becomes essential to ensure welfare, economic, and social value capture for future generations (Porter and Kramer, 2011).
The challenging objectives for a sustainable future comprise the fundamental revision of business models and business processes along the supply chain. Supply chains consist of all parties directly or indirectly involved in the design, production, storage, delivery, sales, finance, and use of a single product. This holistic approach to joint value creation and delivery leads to complex global economic and social networks (Mentzer et al., 2001). For example, Toyota's upstream tier 1–3 supply chain consists of 13 sub-networks with 2,191 suppliers located all around the globe (Kito et al., 2014). Supply chain management (SCM) pursues the goal of managing all flows of goods, materials and services, funds, and information across these complex networks of independent stakeholders (Kummer and Groschopf, 2019). The increasing number of parties and transactions along the supply chain fuels the search for “new ways of coordinating activities such as task allocation, coordination, and supervision of a group of people who share common economic interests” (Voshmgir, 2017, p. 501). Formal contracts, information-sharing for forecasting and monitoring purposes, and trust are the main approaches to achieve alignment. Information transparency and aligned incentives reduce opportunistic behavior and transaction costs between supply chain partners (Narayanan and Raman, 2004). Besides, a recent study conducted by Connelly et al. (2018) reveals the importance of integrity-based trust over competence-based trust in inter-organizational relationships to lower transaction costs.
To improve supply chain sustainability, SCM needs to reduce the negative environmental and social effects of focal companies, suppliers, and customers without compromising their economic performance (Seuring and Müller, 2008). In this context, sustainable SCM (SSCM) refers to corporate plans and activities integrating environmental and social goals into SCM. Fields of activity include internal processes like production, logistics, and initiatives to lower impacts of supplier and customer-related processes (Gimenez et al., 2012). Raising institutional and stakeholder pressure influences corporate sustainability strategies (Herold et al., 2018). Emerging technologies and tools increase data availability and foster transparency in supply chain sustainability (e.g., carbon accounting tools for logistics operations, Groschopf et al., 2019).
For centuries, transactions have required trust between parties involved or a trustworthy intermediary as a centralized ledger, resulting in hierarchical hub-and-spoke transaction systems (Wheeler, 2017). Emerging digital technologies provide alternatives to present centralized approaches and solve critical problems, such as trust issues (Giusti et al., 2019). For instance, distributed ledger technology (DLT) and blockchain as a concept of DLT enable permanent, immutable, and transparent record-keeping of transactions in decentralized systems (Burkhardt et al., 2019). The joint use of innovative technologies along the supply chain shows great potential for addressing information needs and reducing sustainability-related uncertainty (Busse et al., 2017). The combined application of innovative technologies (e.g., blockchain and the internet of things) allows for new approaches to trace the origin and track the current status of products, supplier identity, and reputation, and to reduce fraud and costs along the supply chain (Treiblmaier, 2018). Adding an application layer on top of the technology stack expands the scope of opportunities toward supply chain governance. In this context, a promising concept is smart contracts, initially developed by Nick Szabo in 1997 to build trust in distributed environments without a central authority (Reyna et al., 2018). Smart contracts enable self-enforcement of transactions in supply chains (Prause, 2019), automated relationship management, and a flexible definition of governance rules for individual transactions (Voshmgir, 2017). Due to their complex nature, different approaches to defining smart contracts exist (see section Smart Contracts). Forthcoming smart contract development may lead to automated inclusion of sustainability in supply chains. Technological and conceptual innovation toward autonomous business process and supply chain management will “eventually eliminate the need for management altogether” (Mendling et al., 2018, p. 11).
While numerous publications discuss the potential uses and benefits of blockchain and smart contracts (Kosba et al., 2016; Nofer et al., 2017; Surujnath, 2017), we identify several research gaps to be addressed by the following questions: (1) Which elements are necessary for a comprehensive definition of smart contracts in the context of supply chain management? (2) What knowledge is provided in the literature at the intersection of smart contracts and supply chain sustainability? (3) How can possible relationships between smart contracts and supply chain sustainability be classified? (4) Which levels of digital supply chain maturity support blockchain and smart contracts to improve sustainability? (5) Which main propositions need to be addressed in a research agenda for sustainable smart contracts in supply chains?
The contribution of this article is threefold: First, we contribute to theory development within the theoretical framework for blockchain-based SCM research introduced by Treiblmaier (2018) at the intersection of blockchain, smart contracts, and sustainable supply chain management by systematically matching supply chain and organization maturity with relevant technology and sustainability challenges. Second, we present a new approach to assess technology investments' impacts by categorizing possible relationships between smart contracts and supply chain sustainability. Third, we propose future research directions for academia and industry to develop and implement sustainable smart contract solutions and increase supply chain sustainability through smart contract deployment.
To achieve these ambitious goals, we use various research methods and structure our work as follows: section Contracts and Smart Contracts analyzes different approaches to defining smart contracts and presents a comprehensive definition. Section State of the Field of Smart Contracts and Sustainability in Supply Chains provides a qualitative synthesis of relevant publications on smart contracts and supply chain sustainability. In both sections, we use a content analysis-based literature review (Seuring and Gold, 2012). In section Semi-Structured Assessment Framework for the Sustainability of Smart Contracts, we explicate possible relationships between smart contracts in supply chains and sustainability by constructing a bi-dimensional, semi-structured assessment framework. Section A Conceptual Framework for Supply Chain Maturity Linking Organization, Digitalization, and Sustainability contains a conceptual framework to map relationships between supply chain maturity from an organizational, sustainability, and technology perspective. We address the individual maturity aspects through the lens of multi-dimensionally aligned maturity models with a focus on blockchain and smart contracts. Section Research Propositions for Smart Contracts in Sustainable Supply Chains provides central propositions for future research on smart contracts and supply chain sustainability. Section Conclusion concludes the article.
Contracts and Smart Contracts
This section discusses the nature and types of contracts to introduce the basic concept of smart contracts. First, we describe the main elements of contracts and contracts' different purposes in the relationship lifecycle from a general legal and economic perspective. Then we analyze different approaches to defining smart contracts and propose a general definition. We conclude this section by critically reflecting on the role of distributed ledger technologies regarding the concept of smart contracts.
Contracts
A contract is a legally binding oral or written agreement between two or more legal entities to govern voluntary exchange relationships (Suchman, 2003). The main elements of a contract need to be present to create legal ties between the parties involved (LawDepot, 2019). In a business context, contracts between firms and other legal entities usually exist in the form of structured documents that are subject to contract law (Schwartz and Scott, 2003). The main elements of a contract are the parties involved, the agreement's subject, and a series of norm clauses regulating the expected activities of parties involved (Aires et al., 2019). To a large extent, contracts contain penalties in case of partial or total non-performance.
Through a historical lens, legal systems and contract types applied evolved in conjunction with human development. Predominant societal and economic systems, types of goods and services traded, and transactions' complexity interact with the development of legal systems. Harayama (2017) distinguishes five types of societies: hunter, agrarian, industrialized, information, and human-centered smart high-tech society (Society 5.0). Savelyev (2017) discusses the predominant contract types aligned to the different phases of societal evolution. For example, the dominant contract type in agrarian economies was individual agreements with all terms negotiated directly between the parties involved “at arm's length.” Concerning industrialized societies, modern economic literature analyzes firms mainly from a competence-related or contractual perspective (Foss, 1993). The latter emphasizes costs incurring during the creation and monitoring of transactions. The main challenges in this context comprise information gathering and handling, formulation, monitoring, and policing contracts (Hodgson, 1998). Industrial societies with developed mass markets address these issues by using simplified forms of contracts. The latter rely on standardized terms such as Incoterms to minimize individual negotiation processes, human involvement, and transaction costs (Savelyev, 2017).
While agreements within organizations are often informal, formal contracts between legally independent entities facilitate different functions along the relationship life cycle (Mesquita and Brush, 2008). Central phases of the relationship life cycle are exploratory, expansion, and maturity phases (Dwyer et al., 1987; Jap and Ganesan, 2000). Contracts facilitate safeguarding and coordination throughout these phases to a different extent: The exploratory phase is characterized by low mutual trust between the parties involved and high insecurity about the relationship's future directions. Safeguarding against opportunistic behavior is essential in environments of low mutual trust. Formal agreements in this phase ensure equity and proportional benefits to investments. During the expansion phase, mutual trust and understanding rise. The main purpose of contracts then shifts toward improving efficiency. In mature relationships, the safeguarding and coordination functions of contracts become significant (Shen et al., 2020). For example, recent incidents in automotive supply chains show the need for clear and enforceable contracts to ensure safeguarding even in mature relationships as customers tend to refuse contract performance.
In information societies, contractual arrangements aim to further reduce human involvement during the contract agreement phase and execution over the entire contract period (Savelyev, 2017). To balance economic development with the resolution of social issues in emerging human-centered, knowledge-intensive Society 5.0 (Deguchi et al., 2020), contractual arrangements will need to incorporate and codify respective rules and norms. To anticipate the emerging contractual relations in Society 5.0, we understand its post-humanization driver “as the disappearance of the modern metanarratives of the enlightenment and human emancipation” (Herbrechter, 2013, p. 78). A post-humanized society and its organizations expand membership to intelligent social actors beyond just human beings (Gladden, 2019) “that contribute to the structure, activities, or meaning of that society” (Gladden, 2018, p. 98). Increasing human-robot collaboration and autonomous systems require rules, regulations, knowledge, awareness, and behavior to organize new collaborative self-coordination in intelligent machine-service teams. Normative legal contracts, as well as arrangements for motivational, social behavior, need to represent the expansion of human actors' and software agents' societal membership to “living” intelligent human-machine ecosystems of systems. In essence, ongoing technological and societal development will lead to new types of agreements, which could fundamentally change intercompany transactions and transform social as well as legal institutions (Sklaroff, 2017). One compelling approach to incorporate these developments and impact the “notion of the social contract within society” (Swan, 2015, p. 17) is smart contracts, referred to in the next section.
Smart Contracts
Given the relatively novel nature of the concept and its complex technological basis, up to this point, no universal definition of smart contracts exists (ISDA, 2017; Lauslahti et al., 2018). Considering the lack of consensus regarding the terminology, it seems to be of utmost importance to provide an overview of existing approaches and refine a suitable definition. Hence, after briefly explicating and reflecting on existing definitions for smart contracts, we propose an abstract conceptualization.
Scholars and practitioners have different approaches to defining smart contracts. The main approaches to defining smart contracts are “smart legal contracts,” “smart contract code,” and narrow definitions in connection with application-specific implementation. In his analysis of commonly used ways to characterize smart contracts, Stark (2016) concludes by differentiating basic forms – with the first variant being operational and involving software agents fulfilling certain obligations and exercising defined rights. These agents can be referred to as “smart contract code.” An alternative perspective emphasizes how legal contracts can be expressed and implemented in software. Stark (2016) uses the term “smart legal contract” to subsume these specific characteristics. This approach includes operational aspects as well as issues relating to how to write and interpret legal contracts.
The term smart contract was originally coined by Szabo (1997, online), who argues that smart contracts are a combination of technology and promises communicated via interfaces “to formalize and secure relationships over public networks,” thereby providing a basis for their implementation. In this respect, Szabo (1997) differentiates between mental and computational transaction costs. Computational transaction costs form a small proportion of total costs, in cases where all parameters of the contract are computable. Transaction costs concerning fraud, theft, or unforeseeable incidents form essential cost components to be addressed by smart contracts. Pãnescu and Manta (2018, p. 236) also understand smart legal contracts as protocols that allow “to digitally facilitate, verify, or enforce the negotiation and performance of a contract.” From a technological perspective, Macrinici et al. (2018, p. 2338) recognize smart contracts as “containers of code that encode and mirror the real-world contractual agreements in the cyber realm.” They correspondingly note that “the agreement has to be enforceable by law and can be used to replace intermediaries between contract members leveraging automatic code execution […].” In recent literature, smart contracts are often subject to joint-use cases with blockchain technology (Wright and De Filippi, 2015; Garrod, 2016; Kosba et al., 2016; Nofer et al., 2017; Surujnath, 2017). Consequently, Lauslahti et al. (2017, p. 21) define smart contracts in the context of blockchain as “code-based programs that can be used to generate contractual effects between parties.” As a concept of distributed ledger technologies, blockchain consensually keeps, updates, and validates all transactions fulfilled by parties involved in the decentralized network (Risius and Spohrer, 2017). Besides, Treiblmaier (2018, p. 547) regards blockchain as a digital, “decentralized and distributed ledger in which transactions are logged and added in chronological order to create permanent and tamper-proof records.” Table 1 lists different smart contract definitions.
Another group of scholars strives to define smart contracts by systematically assessing their underlying characteristics. For example, Sillaber and Waltl (2017) recognize (1) the contractual arrangements between the parties, (2) the governance of pre-conditions necessary for the contractual obligations to take place, as well as (3) the actual execution of the contract as relevant factors. Savelyev (2017) provides a more sophisticated approach by identifying the following features: (1) solely electronic nature; (2) software implementation; (3) increased certainty; (4) conditional nature; (5) self-performance; (6) self-sufficiency. Bottoni et al. (2020) summarize the main features of smart contracts as (1) automation; (2) determinism; (3) distribution; (4) immutability; (5) transparency; (6) trust. All definitions based on key characteristics encompass contract execution as a constituting element. We conclude that a comprehensive definition of the term “smart contract” should cover both of the above-mentioned approaches. Hence, with reference to Clack et al. (2016), this paper adopts a higher-level definition.
A smart contract is a mutual agreement between two or more parties that is automatable, although some parts may require human input and control, and where enforceability is achieved either by legal enforcement of rights and obligations or by tamper-proof execution of computer code (adapted from Clack et al., 2016).
The definition above relies on two core features used as central and constituting elements regarding the idea of smart contracts: automation and enforceability. On the one hand, the contract must be enforceable, without precisely pre-specifying which aspects are being enforced. Regarding smart legal contracts, complex rights and obligations must be addressed, while smart contract code may be enforced by applying programmed code. Describing smart contracts as “automatable” rather than using the term “automated” considers that there may be parts of legal agreements whose performance still requires human input and control in practice. Nevertheless, to categorize contracts as “smart,” it is necessary that at least some part of the agreement is capable of automated execution by electronic means (Clack et al., 2016). It seems essential to note that automation of transactions per se does not transform “the automaton into a smart contract or makes the transaction itself smart” (Mik, 2017, p. 274).
Although smart contracts have always been intriguing from a theoretical perspective, at the time of their conceptualization, economic limitations and insufficient communications infrastructure often imposed obstacles to adopting smart protocols in real-world applications (Omohundro, 2014). However, recent advancements in cryptoeconomics have given rise to numerous blockchain-based applications, including smart contracts (Cong and He, 2019). In this respect, it is worth noting that Szabo (1997) already developed the concept of the smart contract long before the pseudonymous Nakamoto (2008) proposed the fundamental idea of blockchain. Nevertheless, we acknowledge that Szabo's original idea gained increasing attention due to current developments and dynamics caused by blockchain (Pãnescu and Manta, 2018).
Savelyev (2017) assumes blockchain-based smart contracts to become a “paradigm-shifter” in the sphere of contracting by enabling decentralized, tamper-proof storage of transactions as well as automation along the contract lifecycle. The author defines smart contracts in context with blockchain as “agreements existing in the form of software code implemented on the blockchain platform, which ensures the autonomy and self-executive nature of smart contract terms based on a pre-determined set of factors” (Savelyev, 2017, p. 116). Although theoretically recognizing and postulating that, in its simplest form, “a smart contract is an agreement whose performance is automated,” the author later justifies his rather narrow interpretation of the term. He points out that otherwise, one could “hardly identify the difference of actual smart contracts from related contractual constructs implementing automated performance, such as vending machines” (Savelyev, 2017, p. 120). Other authors also emphasize the role of blockchain as a foundational technology (Iansiti and Lakhani, 2017). For example, Greenspan (2016, online) portrays a smart contract as “a piece of code which is stored on a blockchain, triggered by blockchain transactions, and which reads and writes data in that blockchain's database.” Lauslahti et al. (2017) focus on blockchain consensus architecture-based smart contracts. Clack et al. (2016) also explicitly address that smart contracts may be based on different types of distributed ledger technologies and thus avoid defining a certain technological form of implementation as a constituting factor. We share this compromise-oriented view and thus reflectively opt for a more general definition, encompassing all forms of smart contracts based on centralized and decentralized ledger technology without rigorously excluding variants not employing blockchain technology.
State of the Field of Smart Contracts and Sustainability in Supply Chains
DLT provides a platform for hosting and executing smart contracts (ISDA, 2017). Joint use of smart contracts and DLT like blockchain removes typical barriers and facilitates the deployment of inter-organizational processes (Mendling et al., 2018). A common definition, the legal status, and implications of smart contracts for contract law are still discussed (Lauslahti et al., 2018). The concepts of supply chains, SSCM (Beske and Seuring, 2014), and sustainability (Carter and Rogers, 2008) are relatively new and complex domains. The effects of blockchain adoption in the supply chain (Dobrovnik et al., 2018; Treiblmaier, 2018) and effects on supply chain sustainability are still in their infancy (Kouhizadeh and Sarkis, 2018). The same applies to smart contracts. Adoption of digital technologies like smart contracts and blockchain could potentially “create new foundations for our economic and social systems” (Iansiti and Lakhani, 2017, p. 2703). Research on sustainability effects of technology adoption in the context of DLT and smart contracts in general, but especially regarding SSCM, is at a very early stage. This section provides a content analysis-based literature review in the form of a qualitative synthesis of recent scientific and business-related publications to assess the knowledge base at the intersection of smart contracts and sustainable supply chains.
Sustainability and Sustainable Supply Chain Management
Linton et al. (2007) describe sustainability as an interdisciplinary topic, combining natural and social sciences. Economic activity along the supply chain has multiple effects on the natural environment and influences life quality. Communities and governments influence supply chain strategies and practices through the external pressure, legal frameworks, and policy development. Salmerón-Manzano and Manzano-Agugliaro (2019) conducted a bibliometric study on the role of smart contracts in sustainability and highlighted worldwide research trends. Their review covers more than 1,700 publications, showing exponential growth in smart contract-related publications since 2014. More than half of the publications are conference papers and reviews, a typical development in emerging topics. Computer science, engineering, and mathematics are the main thematic fields of publications. The authors mention different use-cases as regards traceability, supporting SSCM information needs (Busse et al., 2017). Interestingly, neither sustainability nor supply chain or SSCM form part of the identified research clusters or related keywords, leading to the authors' conclusion that smart contracts could contribute to sustainability in the near future (Salmerón-Manzano and Manzano-Agugliaro, 2019).
The value of timely, accurate, and relevant information in corporate decision-making (Feltham, 1968), as well as the value of sharing specific information in the form of inventory levels, order status, forecasts, and production scheduling across firms along the supply chain (Lee and Whang, 2000), is manifold. The literature on SSCM addresses the value of information and information-sharing in different ways: Beske and Seuring (2014) refer to enhanced communication and technology integration as enablers for long-term collaboration along the supply chain, requiring joint structures and processes to contribute to SSCM. Dubey et al. (2017) describe enabling information technology as a linkage variable in their strategic framework formulation of SSCM. Busse et al. (2017) describe the task, source, and supply chain uncertainty as obstacles for SSCM. The authors identify sustainability-related information processing as a pre-requisite and instrument for successful SSCM, requiring further research in order to achieve the goal of transparency along the supply chain formulated by Carter and Rogers (2008).
Saberi et al. (2019) examine the relationships between blockchain technology and SSCM, describing smart contracts as a central element in blockchain-based supply chains. Smart contracts enable credible transactions without involving third parties and lead to improvements by governing supply chain processes and defining process rules. The authors show sustainability opportunities of different blockchain-use cases, mainly in the field of environmental sustainability, and discuss barriers to blockchain adoption. Their concluding propositions and research agenda imply efficiency gains and reduced supply chain risks by using smart contracts, contributing mainly to the economic and environmental dimensions of SSCM. So far, the relationship between smart contracts and sustainability both in general and in SSCM is underrepresented in the specific literature.
Economic, Environmental and Social Aspects of Smart Contract Implementation Along the Supply Chain
Essentially, smart contracts form a central element in supply chain transformation by fulfilling verification processes and the generation of new transactions on a ledger (Kouhizadeh and Sarkis, 2018; Saberi et al., 2019). Implementation of technology in the context of Industry 4.0 along the supply chain enables self-organizing and self-optimizing production and logistics systems. Technological advancements applied include IoT, cyber-physical systems, and data science (Prause, 2019). The combination of IoT and blockchain provides an interesting example in manufacturing, logistics, and SCM (Treiblmaier, 2018). Smart contracts add value by coordinating self-execution and self-enforcement of transactions between internal and external supply chain partners (Lauslahti et al., 2018; Prause, 2019). Besides, smart contracts facilitate the management and control of IoT-systems by maintaining access control between IoT devices, managing data-sharing among devices, and user authentication (Sultana et al., 2020). Huh et al. (2017) provide a proof of concept using Ethereum blockchain and smart contracts to manage configuration and build a key management system for IoT-devices.
From an economic perspective, reliable, standardized, and automatic execution of transactions and resulting disintermediation in distributed systems support low transaction costs (Kouhizadeh and Sarkis, 2018; Prause, 2019). The main cost components of blockchain-based systems comprise computation, data storage and transmission, and the creation of smart contracts. The latter represents a relatively high-cost fraction, leading to cost allocation questions between supply chain partners (Weber et al., 2016). In different Industry 4.0 scenarios, timing is essential; for example, in certain IoT-equipped environments. These use-cases require smart contracts to fulfill real-time requirements to satisfy timing constraints and prevent inconsistencies or conflicts (Fournier and Skarbovsky, 2019). This need for speed results in the necessity for performant equipment, networks and the willingness to pay higher fees for fast smart contract execution, e.g., in the form of performance-related “gas prices” on Ethereum (Rosic, 2017).
The conclusion of a contract triggers activities along the supply chain. Contractual disputes resulting from incidents like misunderstandings, fraud, or lack of performance can harm supply chain partnerships and disrupt necessary supply chain activities (Min, 2019). Moreover, disputes can have negative economic and possibly social and environmental consequences due to unused production and logistics capacities, prolonged throughput times, and longer cash-to-cash cycles. Sound self-enforcing smart contracts contribute to economic sustainability by creating trust between supply chain partners. In contrast, bugs and technical vulnerabilities can lead to opposite effects, like the Parity Wallet library contract's vulnerability, resulting in high losses for individuals using multi-sig wallets (Alois, 2017). In this context, Nikolić et al. (2018) define three categories of trace vulnerabilities of smart contracts: Greedy contracts locking funds indefinitely, prodigal contracts leaking funds to random users, and suicidal contracts, which can be killed by anybody. Further development is necessary to overcome these vulnerabilities and strengthen trust in decentralized systems and the positive relationship between smart contracts and economic sustainability.
Besides economic aspects, sustainability considerations with smart contracts need to address environmental and social aspects, resulting interdependencies, and economic impacts on supply chain partners. On the one hand, smart contracts help to green the supply chain in different ways. Pre-defined quality standards or environmental conditions can be checked for transaction approval, for example, supplier-, material-, or product-related certifications (Saberi et al., 2019). Besides, smart contracts enable environmentally friendly business models and supply chain designs. Recent literature discusses different energy sector applications (Park et al., 2018; Wu and Tran, 2018; Zhao et al., 2018; Andoni et al., 2019). The use of smart contracts enables decentralized energy trading, including wholesale trading, platforms providing end consumers access to wholesale markets, and microgrid solutions using smart meters as external oracles. Other applications focus on trading services for environmental assets and carbon certificates using blockchain and smart contracts (Andoni et al., 2019). Besides forward-oriented processes, smart contracts provide opportunities for managing reverse flows along the supply chain. In reverse logistics and waste management, supplier performance indicators for waste reduction, an obligation for certified disposal of hazardous waste, or fulfillment of regulatory take-back policies can be defined in smart contracts (Kouhizadeh and Sarkis, 2018).
On the other hand, negative environmental and related social impacts of digitalization, blockchain, and smart contract implementation along the supply chain need to be considered (Bonilla et al., 2018). Challenges include the life cycle assessment of physical assets and energy, enabling the use of smart contracts. Important aspects comprise activities along the entire supply chain and respective categories of environmental and social effects. The main categories comprise land and resource use for data centers, network infrastructure and hardware for smart contract computation, electric energy consumption during the operational phase, as well as end-of-life and recovery processes for data centers and technical equipment (see Figure 1). For example, specific IT equipment used for bitcoin mining outdates on average every 1.5 years due to technological advancements. The annual amount of electronic waste from bitcoin mining corresponds to Luxembourg's electronic waste amount (Digiconomist, 2019a). In contrast, for Ethereum mining, regular graphics processing units are used (Digiconomist, 2019c), processing at least twice the bitcoin network's transactions at less than one-third of bitcoin's electricity consumption (Kamiya, 2019).
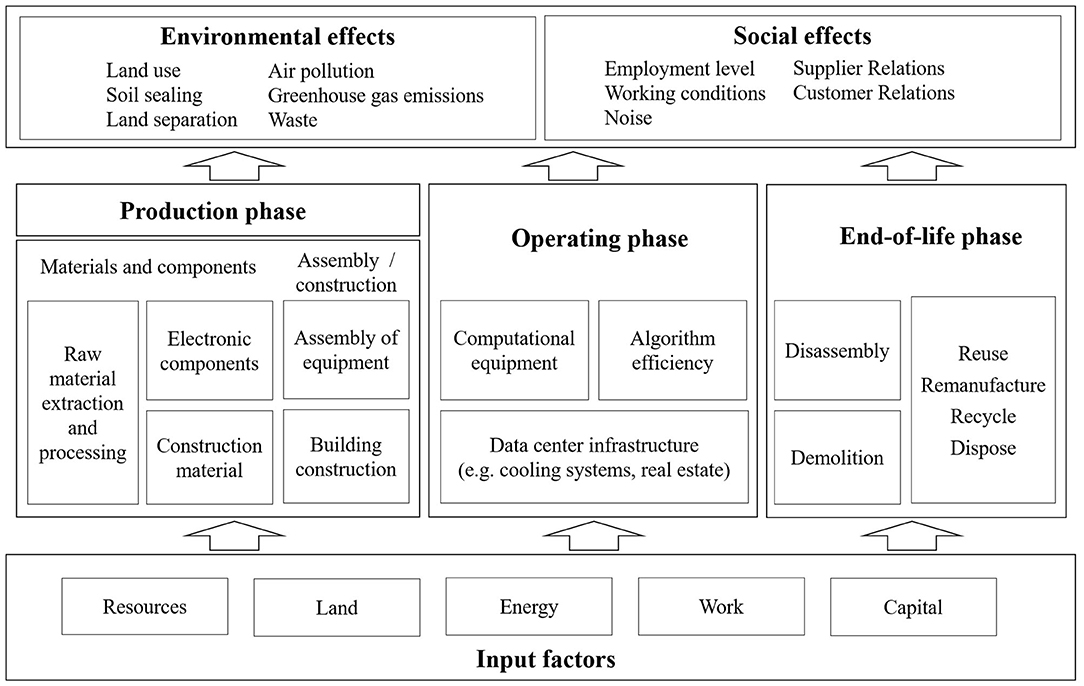
Figure 1. Main input factors, environmental and social effects along the smart contract supply chain.
As regards energy demand and greenhouse gas emissions from data center operations, network design decisions define data center locations, cooling requirements, available energy sources, and respective greenhouse gas emissions. Large miners often choose locations offering cheap electricity sources such as geothermal or hydropower (Vranken, 2017). Transaction-related energy consumption varies across different system architectures, specific hardware equipment, and consensus algorithms used. While transactions in centralized systems like credit card transactions consume 0.01 kWh per transaction (Brosens, 2017), top-down estimates for the energy consumption of decentralized blockchain systems vary considerably between 22 and 37 kWh for Ethereum (Brosens, 2017; Hurwitz, 2019) and from 200 to 613 kWh for Bitcoin transactions (Brosens, 2017; Digiconomist, 2019b). The estimates for total network energy consumption provided by scientific literature vary substantially (Vranken, 2017) and are still subject to discussion (Truby, 2018).
In essence, smart contracts' energy consumption relates to different operations performed and the amount of computational power required for each operation. For example, in Ethereum, smart contracts are usually coded in Solidity, requiring a certain amount of computational power for different operations measured in Ethereum Gas (Rosic, 2017). Wood (2017) defines a Gas fee schedule in his Ethereum whitepaper for different operations affecting transactions. Hurwitz (2019) offers an approximation accounting for the energy consumption of mining validators of 0.0004 kWh per Ethereum Gas as a lower boundary to determine the energy consumption of different specific smart contract operations, as shown in Table 2.
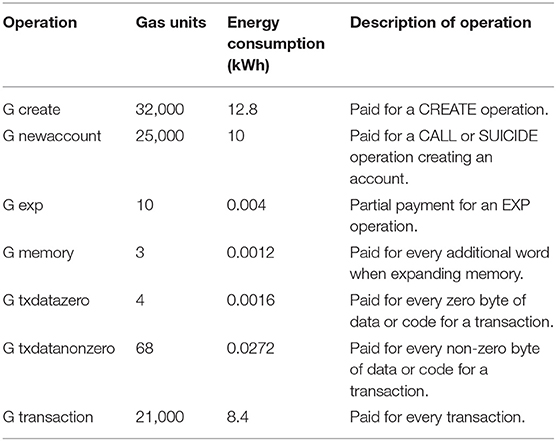
Table 2. Energy consumption of different specific smart contract operations [based on Wood (2017) and Hurwitz (2019)].
The estimates in Table 2 provide insights into smart contract-induced energy consumption. Current publications show a contradictory picture of the relationship between smart contracts and environmental sustainability. Bonilla et al. (2018) suppose smart contracts to have a negative impact due to increased energy flows. Regulatory measures for miners (Truby, 2018) toward using renewable energy and operation of dedicated power plants for data centers, certified end-of-life processes for IT equipment, and technological advancements using alternative consensus algorithms could support a positive relation between smart contracts and environmental sustainability. For example, Ethereum plans to introduce a new proof-of-stake consensus algorithm called Caspar, leading to massive energy consumption reductions (Digiconomist, 2019c).
Further research is necessary to reveal the total environmental footprint and social effects induced by smart contract implementation along the supply chain. Accurately designed and comparable case studies can lead to valuable insights for academia and industry. A pre-requisite to achieve cross-case study comparability is to follow clear guidelines, such as those proposed by Treiblmaier (2019) for blockchain case studies.
Semi-Structured Assessment Framework for the Sustainability of Smart Contracts
In this section, we explicate possible relationships between smart contracts in supply chains and sustainability by constructing a bi-dimensional, semi-structured assessment framework. Frameworks provide normative guidance and explicit explanations (Manuj and Mentzer, 2008) by structuring influencing factors and providing decision support (Tummala and Schoenherr, 2011). Applying a consistent set of principles and employing a transparent procedure is essential to achieve sustainability assessment progress. Sala et al. (2015) propose an adequate scope, core indicators, and potential data sources as fundamental principles. First, we provide a bi-dimensional framework to assess the main risks and introduce transparency (Carter and Rogers, 2008) on smart contracts' overall sustainability performance. With reference to the last section of this article, we consider the life cycle-oriented sustainability performance of the smart contract supply chain on one dimension. The second dimension consists of the sustainability effects of smart contract use along the implementing supply chain. First, we elaborate on both aspects and then present the integrating framework in Figure 2.
Smart contracts and blockchains represent digital products, often provided as services by specialized technology companies. Main service components consist of code developed and implemented and physical assets for computation and transmission - the latter consisting of hardware, data centers, and networks. The sustainability performance of smart contracts depends on the underlying supply chain. In this sector, Gartner (2017, online) expects “consolidation of existing solutions into broader, multidomain suites” as well as fragmentation “of new point solutions that will support innovation, address specific needs and offer new value” to happen simultaneously. According to Columbus (2019), the worldwide public cloud service market will grow from $182.4bn in 2018 to $331.2bn in 2022. Main areas of application for Software as a Service (SaaS) include supply chain management and enterprise resource management systems. To assess smart contracts' overall sustainability performance, related social and environmental effects of smart contract implementation and execution need to be considered (e.g., eco-efficiency of transactions). Figure 1 shows the main stages along the smart contract supply chain life cycle, considering the production of raw materials, components, and finished products in the form of IT equipment and data centers. Subsequent life cycle phases consist of the operating phase and end-of-life of physical assets. In addition, we map the main input factor categories, environmental and social effects (Kummer and Groschopf, 2019), along the smart contract supply chain.
Each supply chain stage may face various sustainability challenges simultaneously. Resource consumption and modern slavery-related issues play a major role during the production phase considering raw materials extraction, building construction, logistics operations, and end-of-life (ASCI, 2019). According to a recent study by Kim and Davis (2016), almost 90% of companies in the electronics and transport equipment industry cannot determine if the minerals used for material and component production are conflict-free, due to the global nature and complexity of upstream supply chains. During the operating phase, computation, data storage, and bandwidth capacities induce high electricity consumption levels, partially wasted due to consensus algorithms (Mendling et al., 2018). Main electricity consumers consist of computing power for smart contracts, related blockchain computation, and data center infrastructure. To develop eco-efficient, green smart contracts (Hurwitz, 2019), energy sources and algorithm efficiency need to be assessed and improved.
The second dimension of the assessment framework proposed in Figure 2 addresses smart contract use sustainability effects along the implementing supply chain. Extreme values indicate the range of potential positive (enable) to negative effects (backfire). Enable refers to positive sustainability impacts by enabling new business processes that increase process sustainability and lower environmental effects. For example, the implementation of smart purchasing contracts could tackle the current challenges of supplier sustainability assessment in dis-integrated, global supply chains (Kim and Davis, 2016). On the opposite end of the scale, backfire indicates the occurrence of the rebound effect. Rebound effects refer to a rise in efficiency which does not lead to the same level of decline in resource consumption (York and McGee, 2016). Backfire occurs when efficiency gains result in higher total demand. For example, a rise in energy efficiency can induce different effects, totally outweighing energy efficiency gains – a phenomenon frequently discussed in connection with energy efficiency gains and rising total energy demand in the transport sector (Matos and Silva, 2011; Yu et al., 2013; York and McGee, 2016). A wide interpretation of the term backfire refers to overall negative sustainability effects along the implementing supply chain.
The proposed framework supports decision-making and evaluation of smart contract design and application regarding sustainability impacts in a supply chain context. The color code used in Figure 2 indicates the capability of the solution to meet sustainability goals. Red denotes an overall unsustainable setup, resulting in an imperative for a redesign. Yellow indicates the need to optimize smart contract conception and/or implementation, while green characterizes a sustainable solution along both dimensions.
To achieve desirable sustainability levels when implementing smart contracts along the supply chain, all partners involved need to fulfill a set of pre-requisites. To address this issue, we develop a conceptual framework mapping supply chain maturity levels from different perspectives. We characterize and assess current and future sustainability effects of smart contracts and corresponding development issues by systematically integrating the parameters organization, sustainability, and digitization.
A Conceptual Framework for Supply Chain Maturity Linking Organization, Digitalization, and Sustainability
Based on the literature analyzed and the sustainability assessment framework presented in Figure 2, we develop a conceptual framework for classifying supply chain maturity. We systematically link supply chain organization, sustainability, and digitalization in context with smart contracts and blockchain technologies.
The underlying concept of “maturity” refers to a “state of being complete, perfect, or ready” (Simpson and Weiner, 1989, online) and thereby implies a certain level of progress in the development of a system. The term conveys the idea of development from an initial state to an advanced state (Lahti et al., 2009). Accordingly, maturing systems (e.g., biological, organizational, or technological) increase their capabilities over time regarding the achievement of a desirable future state (Schumacher et al., 2016). In this context, maturity also refers to the degree of preparedness for setting new business challenges and the ability to develop capabilities (Rudnicka, 2017). Quantitative and qualitative metrics using discrete or continuous scales facilitate assessing a system's individual maturity level (Kohlegger et al., 2009). Maturity models are commonly employed as vehicles to conceptualize and measure organizational or process maturity regarding specific target states. Schumacher et al. (2016) interpret maturity models as frameworks to define starting points for development processes and initialize corresponding transformation activities. Numerous authors have developed maturity models regarding business process and supply chain management (Lockamy and McCormack, 2004; Lockamy et al., 2008; Cronemyr and Danielsson, 2013; Tarhan et al., 2016), interpreting maturity as the ability of an organization and its processes to deliver better business results systematically (Kalinowski, 2016). Seminal work includes the Capability Maturity Model (CMM) for software developed by the Software Engineering Institute at Carnegie Mellon University (Paulk et al., 1991; Paulk, 2002) and the SCM process maturity model developed by Lockamy and McCormack (2004).
In the context of supply chain management (SCM), different researchers use process-oriented supply chain maturity models (SCMM) to examine activities that supply chains go through in an attempt to improve their effectiveness and efficiency (cf. Jording and Sucky, 2016 for a systematic analysis). Although these various models differ concerning the number of phases or their underlying terminology, they all have the same primary intention. They aim to break down the process of reaching supply chain maturity into distinct phases and identify each phase's characteristics. Thereby, they provide a valuable tool for studying supply chain processes (Varoutsaa and Scapens, 2015).
For instance, Lockamy and McCormack (2004) integrate the concepts of process maturity, business process orientation, and CMM using SCOR framework process types to differentiate five SCM maturity levels. Paché and Spalanzani (2007) have developed a five-level maturity framework to capture organizational and SCM maturity, addressing sustainable development in level five. While Lockamy and McCormack (2004) define target states on the progression of activities toward process maturity and effective SCM practices, the maturity levels modeled by Paché and Spalanzani (2007) represent integration objectives for each maturity level, depicted as successive and interlocking levels of progress in SCM. Both maturity models emphasize the importance of multi-firm supply chains but rank this development on different maturity levels. In addition to organizational development perspectives covered in both maturity models, Paché and Spalanzani (2007) address environmental and societal supply chain sustainability as the highest maturity level (see Table 3).
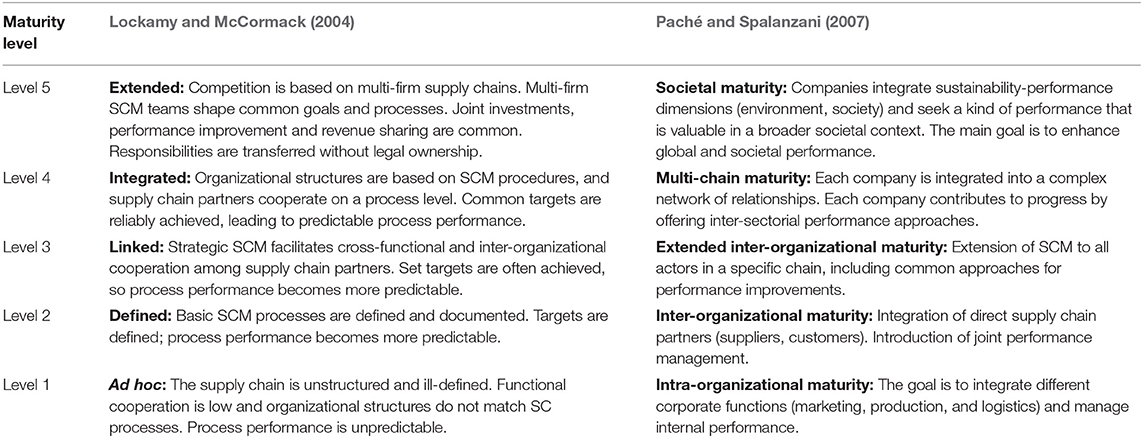
Table 3. SCM maturity models [based on Lockamy and McCormack (2004) and Paché and Spalanzani (2007)].
Recent approaches to defining supply chain maturity emphasize specific aspects focusing on digital transformation or sustainability. For example, Kurnia et al. (2014) propose a SSCM capability maturity framework to help organizations identify potential areas of improvement. Correia et al. (2017) stress the contribution of developing such maturity models with a focus on sustainability to research in SSCM and provide a systematic literature analysis categorizing existing work in this area. The authors employ a SSCM maturity model based on Reefke et al. (2010, 2014) to describe typical development phases. Most recently, a growing body of literature also addresses digital readiness and proposes progression models for organizations and supply chains to master digital transformation (e.g., Schumacher et al., 2016; Klötzer and Pflaum, 2017; Herneth, 2018).
Although the before-mentioned maturity models already address aspects of supply chain organization, sustainability, and digitization individually, they fail to provide an integrated perspective. Clohessy et al. (2019) address these three dimensions in context with blockchain adoption. In this study, we use a synthesizing approach to investigate interconnected development phases and progression paths. We conceptually adapt and link existing domain-specific maturity models using the approach proposed by Paché and Spalanzani (2007) to develop a conceptual framework for supply chain maturity linking organization, sustainability, and digitalization. Furthermore, we use blockchain (Swan, 2015; Yang et al., 2018; Herneth, 2019; Xu et al., 2019) and smart contract development stages (Szabo, 1997; Clack et al., 2016; Savelyev, 2017) as contextualizing and influencing factors. Figure 3 depicts the underlying relationships between the three domain-specific concepts of maturity and contextual factors.
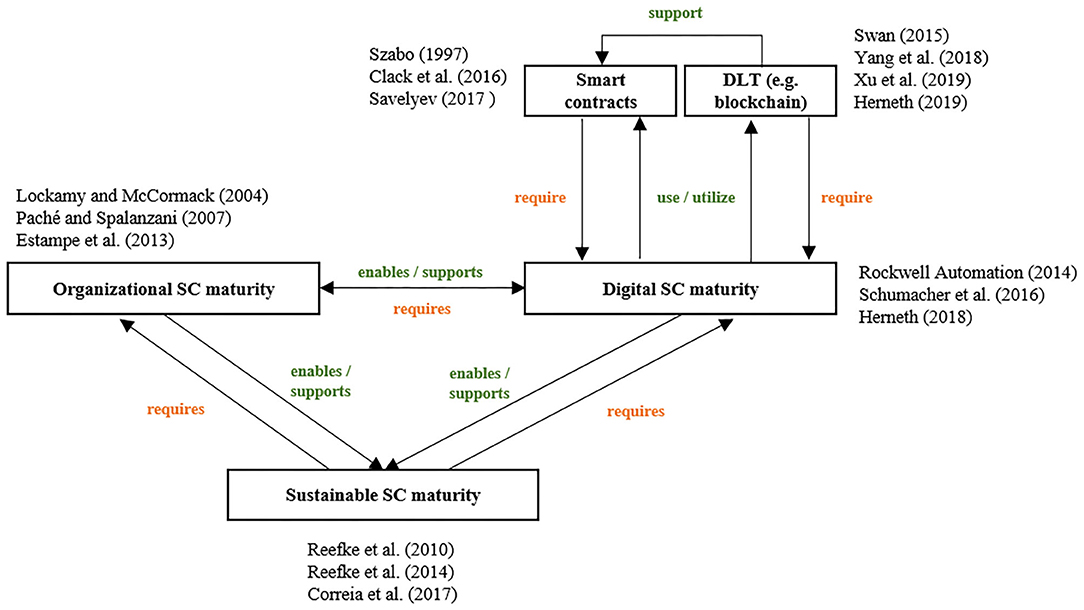
Figure 3. A conceptual framework linking organizational, sustainable, and digital supply chain maturity considering smart contract and blockchain development.
Links in the framework are classified as reciprocal (two-way) and directed (one-way). Furthermore, the framework differentiates enabling/supporting and requiring relationships. While the former type describes that higher levels of maturity in one domain positively influence the feasibility of advancing in the dependent domain, the latter expresses that development in a certain area depends on progress in a different domain. The reciprocal linkage between organizational and digital maturity is identified for both enabling/supporting and requiring relationships. We assume a positive impact of organizational and digital maturity on supply chain maturity and that supply chain maturity depends on progress in these two areas. Although neither DLT in the broader sense nor blockchain in the narrower sense are constituting elements for smart contracts, recent advancements in the field of DLT inspire new developments regarding smart contracts (cf. section Smart Contracts and Pãnescu and Manta, 2018). However, both smart contracts and distributed ledger technologies are applicable to supply chains and potentially enable advanced states of supply chain digitalization. At the same time, supply chains require a certain digital readiness level to employ these technologies successfully.
In the next step, we use the relationships presented in Figure 3 to explicate an integrated perspective on supply chain maturity by incorporating and adapting existing maturity models, as shown in Figure 4. The subsequent paragraphs explain and discuss the individual development phases by employing organizational supply chain maturity as a guiding classifier and structuring dimension. In the context of digital supply chain maturity, “digitization” refers to the migration from analog to digital. At the same time, “digitalization” encompasses business process modernization (Rejeb et al., 2020) using “digital technologies to change a business model and provide new revenue and value-producing opportunities” (Gartner, 2020, online).
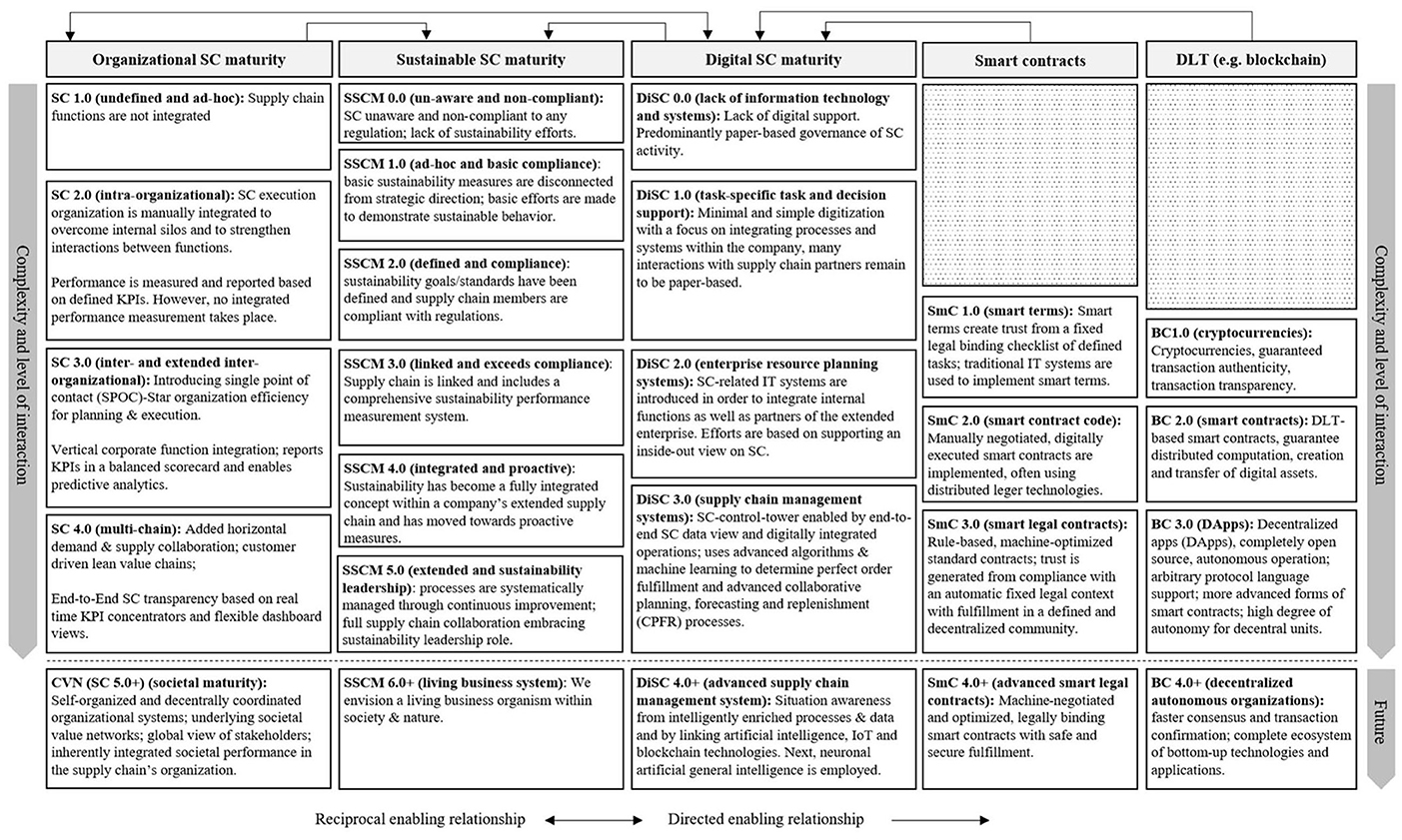
Figure 4. Integrated view of organizational, sustainable, and digital supply chain maturity considering smart contract and blockchain development.
Supply chain 1.0 is characterized by undefined processes executed in an ad-hoc manner (Lockamy and McCormack, 2004). Tasks and activities are typically “paper-based,” while data collection and storage occur fragmented for single supply chain activities. Employees fulfill single orders, mainly adopting local communication in separate business functions. Lacking digitization of paper-based data implies low digital organizational maturity, which neither supports blockchain technology nor smart contract deployment. The scalability of supply chain organizations is very limited, as changes require physical reorganization to improve functional effectiveness. Corporate performance is described based on historical information using descriptive analytics (Cruijssen, 2020). The lack of transparency and information often reflects the absence of sustainability efforts. Consequently, even basic and ad-hoc compliance applies to a limited number of supply chain stakeholders at this maturity level.
Supply chain 2.0 is still “predominantly paper-based,” exhibiting low levels of digitization. Supply chain partner integration focuses on intra-organizational aspects (Paché and Spalanzani, 2007; Estampe et al., 2013) to overcome internal functional silos. Basic business process orientation evolves (e.g., by defining and documenting processes; Lockamy and McCormack, 2004), while process execution and data entry into IT systems are still performed manually. Digital organizational capabilities are limited; available data is often fragmented and inconsistent, thereby providing limited opportunities to improve business decisions (Herneth, 2018). Simple smart contracts in the form of smart terms allow for the specification of clauses within a traditional, paper-based contract to reduce complexity and facilitate efficient agreements. Pre-defined tasks, expressed in the form of such terms, improve transparency, and enhance trust. Task execution can be confirmed either manually or in respective applications. The application of basic smart terms improves supply chain visibility on an activity level by capturing production, transportation, or warehousing records (Herneth, 2018). Effective internal supply chain execution across functional silos predominantly depends on personal skills and relationships. Corporate performance is aggregated and reported as KPIs for diagnostic purposes (Cruijssen, 2020 and Herneth, 2018).
Supply chain 3.0 describes the subsequent stage of supply chain maturity. Main intra- and inter-organizational processes are well-defined, and corresponding IT systems are in place to support digitalization, while extended inter-organizational integration is limited to key customers and suppliers. This maturity stage allows for a comprehensive approach to SCM and performance measurement (Paché and Spalanzani, 2007; Estampe et al., 2013). While material flow control is visualized mainly within single organizations, data flows between IT systems are integrated and validated. Digital capabilities for collaborative supply chain management evolve, introducing basic algorithms for planning, forecasting, and decision support to introduce predictive analytics (Cruijssen, 2020) and strengthen alignment across organizational boundaries (Herneth, 2018). Smart terms (SmC 1.0) emerge by leveraging e.g., blockchain-technologies toward distributed ledger-based smart contracts (Swan, 2015; Yang et al., 2018; Xu et al., 2019). Consequently, smart contract code (SmC 2.0) refers to coded components of traditional legal contracts negotiated by human contractual parties. Trust or the history of “immutable lies” results from a distributed ledger of automatic fixed legal consent with binding security. Improved supply chain visibility and traceability derive from trapping production and logistics records. Single point of contact (SPOC)-star organization efficiency is introduced for resource planning and execution. Vertical integration of corporate functional hierarchies and systems using standardized business processes facilitates consistent data flows. Corporate and SC process performance is reported in detailed KPIs and analytics using dashboards (Herneth, 2018, 2019). Supply chain 3.0 enables the evolution of comprehensive sustainability-performance measurement systems toward fully integrated concepts employing proactive measures within a company's extended supply chain (Reefke et al., 2010).
Supply chain 4.0 introduces a new paradigm by enabling a holistic supply network view on operational business processes. The concept of supply chain control towers facilitates integrated, end-to-end (e2e) supply chain data visibility (Strategy & PWC, 2017). Fast and granular prescriptive analytics (Cruijssen, 2020) support decision-making across all partners to ensure cost-efficient, perfect fulfillment on the order execution level (e.g., on perfect order delivery date, PODD) (Herneth, 2018). Advanced algorithms and machine learning enable collaborative demand and supply planning to align the supply chain organization toward a digitally optimized supply chain-service orchestration, which rapidly responds to demand and supply changes at all tiers.
Blockchain 3.0 is implemented on mainnets (Robinson, 2020) such as EOS (Cai et al., 2019), enabling large-scale decentralized applications (DApps) (Wang et al., 2019). Technological advantages comprise increasingly resource-efficient consensus algorithms, raising cross-chain scalability (Robinson, 2020), the ability to conduct large-scale business governance, and high protocol performance, resulting in transaction rates exceeding one million transactions per second (TPS, Ventura, 2020). Smart contracts 3.0 are rule-based and machine-optimized standard contracts creating trust from the automatically fixed legal context with binding fulfillment security within a defined decentralized community. These smart legal contracts improve supply network knowledge on traceability and authentication (who did what and when?). Using data from external oracles (e.g., IoT sensors), automated workflows validate production and transportation records against the smart legal contract. Organizations' horizontal collaboration with corporate supply chain partners on a functional level enables alignment toward a customer-driven lean value chain (Duarte and Cruz-Machado, 2017). Main organizational maturity aspects include joint goals, standardized supply chain data-models, and system interfaces facilitating operational pairing of common workflows. Resulting e2e supply chain transparency on a business process level allows for aggregation and analysis of interdependent demand and supply data in real-time KPI concentrators and performance dashboards (Herneth, 2019).
While elaborated supply chains already meet the above-mentioned maturity level 4.0, supply chain 5.0+ denotes future states exceeding today's business practices and technological foundations. Connected value networks (CVN) (Stabell and Fjeldstad, 1998; Allee, 2008; Smith and Allee, 2009; Allee et al., 2015) achieve seamless actionable SC visibility and value exchange from peer to peer and autonomous actor communication in real-time. Customer order, transport fulfillment, and experience data are enriched with process and context information from different digital sources, constituting the next level toward digital transformation. For example, using a responsibility assignment matrix (RAM) determines which upskilled actors need to align on deviations across dependent business processes, while AI applications improve the process by introducing contextualization (Cabanillas et al., 2018).
Integrated supply chain planning with execution feedback schedules semi-autonomous fulfillment-oriented human-robot collaboration (HRC) (Bauer et al., 2008) and intelligent machine service (iMS)-teams (Hoffman and Breazeal, 2004; Herneth, 2018; Iqbal and Riek, 2019). Resulting adaptive supply networks (ASN) are digital and secure networks for autonomous collaboration and intelligent decision-making (Rodewald et al., 2015; Herneth, 2019). Supply chain services are risk-aware and proactively respond to exceptions and deviations with distributed decision management (DMN) (Object Management Group (OMG), 2019) and automated decision-making (ADM) (Newell, 1979; Fink, 2006) as distributed automated decision-making (DADM) processes (Herneth, 2018). For example, systems decide on re-planning vs. accepting alternative process actions and anticipate threats to solve them before their actual materialization.
The concept of Blockchain 4.0 (Seele, 2018) considers general conditions of emerging Society 5.0, while previous blockchain generations predominantly focused on technical performance improvement. In a supply chain context, BC 4.0 adds value by uniting HRC as customer and supplier iMS-teams, e.g., through governing adaptable contracts in decentralized autonomous organizations (DAO) (Norta et al., 2015; Goertzel et al., 2017; Herneth, 2019). Smart contracts 4.0+ introduce autonomous actors and intelligent agent-based (iAgent) (Allee, 2008; Allee et al., 2015; Herneth, 2019) negotiation of motivational and socially-inspired contracts. Automatic, machine-negotiated, legally binding contracts with adaptive, safe, and secure fulfillment establish trust in a distributed community.
In supply chain 5.0+, a shared value network provides a secure ecosystem for knowledge-sharing as intellectual property (e.g., designs, bills of material, production, and shipping schedules) among suppliers, manufacturers, and shippers. Multiple, hyper-adaptive 5A (automated, autonomous, agile, aligned, adaptive) (Lee, 2004; Herneth, 2019) component SCs are governed in near real-time and allow for self-organization and -optimization within decentralized systems of systems (Ssystems) (DeLaurentis, 2005) of complimentary component capabilities. The networked value chain (NVC) organization incubates fair fulfillment-actor-behavior (Gloeckler and Herneth, 2000). For example, the smart contract's SC-fulfillment auto-capture function traps activities on an atomic level. It facilitates real-time traceability while contextual machine learning (ML) and AI, as a form of intelligent process mining, extract the value-creating network intelligence (Bechetoille, 2010) as intelligent network operating cultures (INOCs) (Herneth, 2019). These INOCs address the issue of adding vitality of the human mind and behavioral interactions to the knowledge and value creation challenge, governing the evolution of “open living systems of systems” toward an innovative, healthy, socially responsible, and environmentally sound ecosystem.
Research Propositions for Smart Contracts in Sustainable Supply Chains
Recent literature formulates a set of future research directions in the form of research questions or propositions regarding the relevant topics blockchain, smart contracts, sustainability, and supply chain management. Treiblmaier (2018) provides a theoretical framework for blockchain-related theory development and formulates exemplary research questions to create middle-range theories. Mendling et al. (2018) develop research propositions for future business process-related blockchain research. Adams et al. (2017) provide a blockchain research agenda, raising questions on sustainability issues. Other authors formulate research propositions for blockchain adoption along the supply chain (Francisco and Swanson, 2018; Queiroz et al., 2019; Wang et al., 2019) and in context with sustainable supply chain management (Kouhizadeh and Sarkis, 2018; Saberi et al., 2019). Unterweger et al. (2018) formulate future privacy-preserving smart contract development challenges considering cost and capacity issues. Sklaroff (2017) addresses challenges for future smart contract deployment, considering legal and cost aspects. Hu et al. (2019) systematically frame eleven open research challenges for blockchain-based smart contracts along different dimensions. Clack et al. (2016) propose long-term research challenges for smart contract language development and standardization, including legal aspects.
Technological advancements, streamlined business processes, and supply chain stakeholders need to embrace and contribute to the development of a sustainable and socially inclusive society. Corporate and governmental organizations and academia have to enhance digital capabilities, as well as understand the challenges and opportunities of technology and impacts on society to support societal goals. So far, authors addressing smart contracts, blockchain, and sustainability follow different paths. In this context, literature separately focuses on future research in relevant subdomains, while joint research on smart contracts, supply chains, and sustainability is scarce. The complex concept of smart contracts demands a multifaceted approach to research covering legal, functional, technological, sustainability-related, and other perspectives. Following results from Salmerón-Manzano and Manzano-Agugliaro (2019), we assess smart contracts and sustainability research at a very early stage. We formulate corresponding research propositions considering technology, business process and supply chain management, and sustainability to address this gap. We structure our research propositions from short- to long-term perspectives and address the main trade-offs to be considered to facilitate and guide prospective research.
Technology
Technological innovation enables new approaches to value creation in the form of business models and the redesign of business processes. Blurring industry boundaries and combined use of different technologies lead to technology convergence. Technological readiness and shrinking costs support technology use along the supply chain and enable the integration of information technology and operational technologies. From a sustainability perspective, trade-offs between reduced resource consumption due to improved operational efficiency and resource consumption enabled by convergent innovation in technology need to be adopted and considered.
Research Proposition 1
Convergent innovation in different key technologies, including telecommunication standards (e.g., 5G), blockchain, IoT, and AI, supports adoption and diffusion of smart contracts in supply chains to meet business needs.
Technology enables new approaches to value creation as well as cost savings. From a cost perspective, the total cost of ownership is essential. Smart contracts can reduce costs during individual contract conclusion and enforcement (e.g., labor costs) but imply additional costs for smart contract deployment and operations. From a sustainability perspective, trade-offs between environmental effects along the smart contract supply chain and possible changes in employment levels in administration, especially legal departments, procurement, and logistics, need further investigation.
Research Proposition 2
Society 5.0 requires socially inclusive Industry & Logistics 4.0 and massive parallel-enhanced operations; technological advancements and smart contract maturity decrease transaction costs and enhance transparency and security along the supply chain.
Technological development, as well as industry-specific applications of smart contracts, are at a very early stage. Today, different types of smart contract platforms compete on developing a dominant design in terms of functions, business logic, and underlying consensus algorithms. From a sustainability perspective, creating a dominant design could imply lower energy use and risks for corporate technology investments but raise questions on dominant market players' economic and social effects.
Research Proposition 3
Proprietary solutions and open alternatives will compete for being accepted as (de-facto) standards/dominant designs in markets and their global supply chains.
Research Proposition 4
The emergence of dominant designs will facilitate smart contract diffusion in supply chains due to a reduced risk of technological obsolescence and business acceptance.
Business Process and Supply Chain Management
Supply chains aim at fulfilling customer needs by joint value creation across numerous legal entities. The number of interfaces and the need for coordination determine the complexity of the supply chain. Main influencing factors include the nature of the underlying goods or services, the number of actors, and local dispersion. The lack of alignment of single business processes is a particular challenge in highly fragmented environments. The introduction of automated collaboration using AI and distributed automated decision-making increases alignment between business processes along the supply chain and opens the research field for digitized “situational awareness.” From a sustainability perspective, trade-offs between process performance and resulting environmental and social effects need consideration.
Research Proposition 5
The high degree of fragmentation in specific supply chains and the resulting need for coordination bear a high potential for adopting smart contract standards as common IT systems and SC organization-integration bridges.
Recent technology adoption circles around specific business processes and industries to enable new business models or address actual pain points. Growing numbers of use cases and developing a vision, strategy, and roadmap for the creation of digital end-to-end business processes within and across companies support technology adoption along the supply chain. Evolution toward connected value networks could help supply chain partners develop common goals and agree upon shared value creation approaches in adaptive supply networks (e.g., perfect order delivery date) and related digitization standards. From a sustainability perspective, trade-offs between process efficiency, environmental goals, the value of social relationships, and new approaches to supplier and customer relationship management in digital ecosystems need further consideration.
Research Proposition 6
The field of supply chain management will adopt smart contracts step-by-step from single business processes to holistic, industry-specific frameworks.
Understanding the dimensions of human vitality and applying such cybernetic and behavioral interaction cycles to knowledge and value creation in complex systems may open a pathway to evolve the current business and AI development toward an “Open Living System” design and corresponding corporate and supply chain culture. From a sustainability perspective, trade-offs with the current short and medium-range individual economic objectives and social developments need to be addressed.
Research Proposition 7
Industry-specific smart contract frameworks will convert toward industry-agnostic blueprints, enabling decentralized, autonomous business networks.
Sustainability
Emerging technologies need to demonstrate economic benefits to achieve user acceptance and large-scale adoption in different sectors. The development of hardware and algorithms mainly focuses on economic advances in speed, capacity, and costs. Energy-consuming consensus algorithms, electronic waste, and the environmental effects of data centers impose actual sustainability challenges.
Research Proposition 8
Short and mid-term smart contract adoption will lead to ecological rebound effects due to disproportional resource consumption.
Reducing the environmental effects of technology creates value for society and companies. From a corporate perspective, the development of green smart contracts leads to a competitive advantage in different ways. Savings on energy costs and risk mitigation in context with upcoming environmental taxes support cost leadership. A shift in customer requirements toward sustainable service provision and raising interest in supply chain discovery and life cycle-oriented supply chain assessment create new business opportunities and support differentiation strategies.
Research Proposition 9
On a long-term basis, eco-efficient smart contracts will gradually replace dominant proof-of-work-based solutions.
The state of climate crisis creates public awareness and stakeholder pressure toward the environmental sustainability of goods and services. Sustainability research provides a growing number of tools to assess environmental effects from economic activity along the supply chain. The urgency of environmental topics and low attention to social challenges along the supply chain will continue to steer research and regulatory attention toward topics associated with environmental rather than social sustainability.
Research Proposition 10
Existing environmental challenges of smart contracts will be addressed prior to social challenges.
So far, knowledge about the overall social effects of digitization is limited. In the context of employment levels, industrial process automation mainly affects standardized tasks and the respective low to mid-skilled workforce. Automated contract execution and autonomous contract conclusion substitute human work by technology and are likely to influence employment levels in various business functions along the supply chain. Impacts of automated contract execution or even autonomous contract conclusion on employment in existing occupational areas need to be contextualized with jobs created in raising areas and resulting implications for social welfare systems. Future research is necessary to provide deeper insights into the intersection between smart contract adoption's overall economic and social effects.
Research Proposition 11
Mass adoption of smart contracts in supply chains and the resulting autonomous self-organization will lead to social backfire on employment levels in different business functions.
Algorithms draw rule-based decisions in order to optimize output. Today, underlying smart contract rules are negotiated and coded by human beings. Future developments point toward growing capabilities of AI and convergence of operational and information systems along the supply chain. The resulting integrated networks gradually develop into autonomous, self-learning systems of systems. Public discussion and interdisciplinary research on ethics, social values, and legal frameworks are necessary to introduce new governance paradigms and create a desired future state of society and economy in a digitally transforming world.
Research Proposition 12
As AI and the concept of autonomy become inherent elements of smart contracts, ethical aspects of computing, governance, and societal values will gain importance.
The concept of smart contracts represents a major opportunity for corporate sustainability management and SSCM. Sustainability strategies and goals are defined on a corporate level but need alignment with supply chain partners. In global supply chains, safeguarding compliance of operations throughout all business processes along the supply chain is rare today. The main challenges are network complexity and anonymity of supply chain partners. Smart contracts introduce the basis for operationalizing sustainability goals as coded elements. Besides developing methods for assessing supply chain sustainability, research on trusted sources of data (e.g., external oracles) is necessary, especially in raw material extraction and processing, logistics, and the end-of-life phase of products and assets.
Research Proposition 13
Implementation of smart contracts along the supply chain introduces a new paradigm for corporate and governmental sustainability strategies following societal development goals.
Developing societal concepts formulate the basis for future human coexistence. Governing economic and social transactions with smart contracts enables implementing sustainability goals in governmental and corporate decision-making and operational procedures. Civil society, governmental institutions, and academia need to collaborate with industry to create a common sense of predominant societal goals, general norms, and implementation-related rules to achieve overall society objectives.
Conclusion
Smart contracts' implementation will become a foundational capability of supply chains, potentially having vast impacts on today's manual monitoring and control business models, thus reducing throughput time, human error, and cost. Szabo (1997) introduced the basic idea of smart contracts long before the emergence of blockchain and the corresponding bitcoin hype. Ongoing technological development (e.g., IoT devices as external oracles) will facilitate the diffusion of smart contracts in specific business processes along the supply chain. While recent publications address technological advancements and possible applications, transdisciplinary research areas like sustainability are still underrepresented. Given the relatively young nature of the underlying academic field, many important questions at the intersection of supply chain management, sustainability, and smart contracts remain to be addressed.
This paper contributes to the state of the field in various ways and frames possible directions for future research but has limitations due to the novelty of the field and the underlying research approach. The article provides a comprehensive definition of smart contracts in the context of supply chain management and shows the state of the field of smart contracts and sustainability in supply chains, using a content analysis-based literature review. Furthermore, it identifies the possible effects of smart contracts on supply chain sustainability in the form of a semi-structured assessment framework. It proposes a conceptual framework to map relationships between supply chain maturity from an organizational, sustainability, and technology perspective, focusing on blockchain as a use case of DLT and smart contracts. It identifies different supply chain maturity levels and gives an outlook on possible future development perspectives. Finally, it offers research propositions and trade-offs for smart contracts regarding technology development, business processes along the supply chain, and sustainability. Limitations result from the literature-based and conceptual research approach and our individual blind spots. Nevertheless, the work at hand supports introducing the new paradigm of a paired physical and digital world for self-organizing and -optimizing supply chains in living Industry 5.0 and Society 5.0 ecosystems. In a nutshell, the authors strive to stimulate future research and practical applications through this article.
Data Availability Statement
The original contributions presented in the study are included in the article/supplementary material, further inquiries can be directed to the corresponding author/s.
Author Contributions
WG proposed the initial idea for this article. All authors contributed to the development, composition, and review of the article. All authors approved the article for publication.
Conflict of Interest
CH is employed by OMV Refining & Marketing GmbH.
The remaining authors declare that the research was conducted in the absence of any commercial or financial relationships that could be construed as a potential conflict of interest.
Acknowledgments
The authors would like to thank their families for their ongoing support and sympathy for long working hours and late-night sessions in order to create this article, especially our children Jonathan, Charlotte, and Pia, as well as Christopher and Matthias. The authors are grateful for the valuable feedback of two reviewers. Furthermore, the authors are thankful for the linguistic advice by Dr. Martin Herles and the support provided by frontiers in Blockchain, especially Sarah Yardley.
Footnotes
1. ^For detailed information on the 17 SDGs, please refer to: https://sdgs.un.org/goals.
References
Adams, R, Parry, G, Godsiff, P, and Ward, P. (2017). The future of money and further applications of the blockchain. Strat. Change 26, 417–422. doi: 10.1002/jsc.2141
Aires, J. P., Granada, R., and Meneguzzi, F. (2019). “ConCon: a contract conflict identifier,” in Proc. of the 18th International Conference on Autonomous Agents and Multiagent Systems (AAMAS 2019), eds N. Agmon, M. E. Taylor, E. Elkind, M. Veloso (Montreal, Canada).
Allee, V. (2008). Value network analysis and value conversion of tangible and intangible assets. J. Intellect. Cap. 9, 5–24. doi: 10.1108/14691930810845777
Allee, V., Schwabe, O., and Krause Babb, M. (2015). Value Networks and the True Nature of Collaboration. Tampa, FL: Meghan-Kiffer Press.
Alois, J. D. (2017). Ethereum Parity Hack May Impact ETH 500,000 or $146 Million. Available online at: https://www.crowdfundinsider.com/2017/11/124200-ethereum-parity-hack-may-impact-eth-500000-146-million/ (accessed September 19, 2019).
Andoni, M., Robua, V., Flynna, D., Abramb, S., Geach, D., Jenkins, D., et al. (2019). Blockchain technology in the energy sector: a systematic review of challenges and opportunities. Renewable Sustain. Energy Rev. 100, 143–174. doi: 10.1016/j.rser.2018.10.014
ASCI (2019). Modern Slavery – Rights, Risks and Responsibilities. A guide for companies and investors. Available online at: https://assets.kpmg/content/dam/kpmg/au/pdf/2019/modern-slavery-guide-for-companies-investors-feb-2019.pdf (accessed October 3, 2019).
Bauer, A., Wollherr, D., and Buss, M. (2008). Human–robot collaboration: a survey. Int. J. Hum. Rob. 5, 47–66. doi: 10.1142/S0219843608001303
Bechetoille, T. (2010). The Everyday Relationship Between You and Your Information on the Internet. Available online at: http://technews.tmcnet.com/ipcommunications/topics/ip-communications/articles/52992-everyday-relationship-between-and-information-whats-out-there.htm (accessed August 6, 2019).
Beske, P., and Seuring, S. (2014). Putting sustainability into supply chain management. Supply Chain Manage. 19, 322–331. doi: 10.1108/SCM-12-2013-0432
Bonilla, S. H., Silva, H. R. O., da Silva, M. T., Gonçalves, R. F., and Sacomano, J. B. (2018). Industry 4.0 and sustainability implications: a scenario-based analysis of the impacts and challenges. Sustainability 10:3740. doi: 10.3390/su10103740
Bottoni, P., Gessa, N., Massa, G., Pareschi, R., Selim, H., and Arcuri, E. (2020). Intelligent smart contracts for innovative supply chain management. Front. Blockchain 3:535787. doi: 10.3389/fbloc.2020.535787
Brosens, T. (2017). Why Bitcoin Transactions Are More Expensive Than You Think. Economic and Financial Analysis, ING. Available online at: https://think.ing.com/opinions/why-bitcoin-transactions-are-more-expensive-than-you-think/ (accessed September 23, 2019).
Burkhardt, D., Frey, P., Hiller, S., Neff, A., and Lasi, H. (2019). “Distributed ledger enabled internet of things platforms: symbiosis evaluation,” in Business Transformation through Blockchain Volume II, eds H. Treiblmaier, and R. Beck (Basingstoke: Palgrave Macmillan), 77–118. doi: 10.1007/978-3-319-99058-3_4
Burrit, R., and Christ, K. (2016). Industry 4.0 and environmental accounting: a new revolution? Asian J. Sustain. Soc. Responsib. 1, 23–38. doi: 10.1186/s41180-016-0007-y
Busse, C., Meinlschmidt, J., and Foerstl, K. (2017). Managing information processing needs in global supply chains: a prerequisite to sustainable supply chain management. J. Supply Chain Manage. 53, 87–113. doi: 10.1111/jscm.12129
Cabanillas, C., Resinas, M., and Ruiz-Cortés, A. (2018). A template-based approach for responsibility management in executable business processes. Enterprise Inf. Syst. 12, 550–586. doi: 10.1080/17517575.2017.1390166
Cai, T., Cai, H. J., Wang, H., Cheng, X., and Wang, L. (2019). Analysis of blockchain system with token-based bookkeeping method. IEEE Access 7, 50823–50832. doi: 10.1109/ACCESS.2019.2911124
Carlowitz, H. C. v. (1713). Sylvicultura oeconomica oder Haußwirthliche Nachricht und Naturmäßige Anweisung zur Wilden Baum-Zucht nebst Darstellung, Wie … vieler hand Bäume zu prospiciren. Leipzig, Braun.
Carter, C., and Rogers, D. (2008). A framework of sustainable supply chain management: moving toward new theory. Int. J. Phys. Distribution Logistics Manage. 38, 360–387. doi: 10.1108/09600030810882816
Clack, C. D., Bakshi, V. A., and Braine, L. (2016). Smart contract templates: foundations, design landscape and research directions. arxiv:1608.00771. 2016. Available online at: http://arxiv.org/abs/1608.00771 (accessed October 16, 2019).
Clohessy, T., Acton, T., and Rogers, N. (2019). “Blockchain adoption: technological, organisational and environmental considerations,” in Business Transformation Through Blockchain, eds H. Treiblmaier, and R. Beck (Cham: Palgrave Macmillan), 47–76. doi: 10.1007/978-3-319-98911-2_2
Columbus, L. (2019). Public Cloud Soaring To $331B By 2022 According To Gartner. Available online at: https://www.forbes.com/sites/louiscolumbus/2019/04/07/public-cloud-soaring-to-331b-by-2022-according-to-gartner/#408001d35739 (accessed October 17, 2019).
Cong, L. W., and He, Z. (2019). Blockchain disruption and smart contracts. Rev. Financ. Stud. 32, 1754–1797. doi: 10.1093/rfs/hhz007
Connelly, B. L., Russell, T., Combs, J. G., Ketchen, D. J. Jr., and Aguinis, H. (2018). Competence- and Integrity-Based Trust in Interorganizational Relationships: which matters more? J. Manage. 44, 919–945. doi: 10.1177/0149206315596813
Correia, E., Carvalho, H., Azevedo, S. G., and Govindan, K. (2017). Maturity models in supply chain sustainability: a systematic literature review. Sustainability 9:64. doi: 10.3390/su9010064
Cronemyr, P., and Danielsson, M. (2013). Process management 1-2-3–a maturity model and diagnostics tool. Total Qual. Manage. Bus. Excell. 24–28, 933–944. doi: 10.1080/14783363.2013.791114
Cruijssen, F. (2020). “Cross-chain collaboration in logistics,” in International Series in Operations Research & Management Science (ISOR), Vol. 297 (Cham: Springer Nature). doi: 10.1007/978-3-030-57093-4
Deguchi, A., Hirai, C., Matsuoka, H., Nakano, T., Oshima, K., Tai, M., et al. (2020). “What is society 5.0?,” in Society 5.0, ed Hitachi-UTokyo Laboratory (Singapore: Springer), 1–23.
DeLaurentis, D. (2005). “Understanding transportation as a system-of-systems design problem,” in 43rd AIAA Aerospace Sciences Meeting and Exhibit, January 10-13, 2005 (Reno, Nevada). doi: 10.2514/6.2005-123
Digiconomist (2019a). Bitcoin Electronic Waste Monitor. Available online at: https://digiconomist.net/bitcoin-electronic-waste-monitor/ (accessed September 20, 2019).
Digiconomist (2019b). Bitcoin Energy Consumption Index. Available online at: https://digiconomist.net/bitcoin-energy-consumption (accessed October 4, 2019).
Digiconomist (2019c). Ethereum Energy Consumption Index (beta). Available online at: https://digiconomist.net/ethereum-energy-consumption (accessed October 5, 2019).
Dobrovnik, M., Herold, D. M., Fürst, E., and Kummer, S. (2018). Blockchain for and in logistics: what to adopt and where to start. Logistics 2:18. doi: 10.3390/logistics2030018
Duarte, S., and Cruz-Machado, V. (2017). “Exploring linkages between lean and green supply chain and the industry 4.0,” in Proceedings of the Eleventh International Conference on Management Science and Engineering Management. ICMSEM 2017. Lecture Notes on Multidisciplinary Industrial Engineering, eds J. Xu, M. Gen, A. Hajiyev, and F. Cooke (Cham: Springer). doi: 10.1007/978-3-319-59280-0_103
Dubey, R., Gunasekaran, A., Papadopoulos, T., Childe, S. J., Shibin, K. T., and Wamba, S. F. (2017). Sustainable supply chain management: framework and further research directions. J. Clean. Prod. 142, 1119–1130. doi: 10.1016/j.jclepro.2016.03.117
Dwyer, F. R., Schurr, P. H., and Oh, S. (1987). Developing buyer-seller relationships. J. Mark. 51, 11–27. doi: 10.1177/002224298705100202
Elkington, J. (2004). Enter the Triple Bottom Line. Available online at: http://www.johnelkington.com/archive/TBL-elkington-chapter.pdf (accessed August 4, 2019).
Elkington, J. (2018). 25 Years Ago I Coined the Phrase “Triple Bottom Line.” Here's Why It's Time to Rethink It. Harvard Business Review. Available online at: https://hbr.org/2018/06/25-years-ago-i-coined-the-phrase-triple-bottom-line-heres-why-im-giving-up-on-it (accessed January 16, 2021).
Estampe, D., Lamouri, S., Paris, J.-L., and Brahim-Djelloul, S. (2013). A framework for analysing supply chain performance evaluation models. Int. J. Prod. Econ. 142, 247–258. doi: 10.1016/j.ijpe.2010.11.024
Feltham, G. A. (1968). The value of information. Account. Rev. 43, 684–696. Available online at: https://www.jstor.org/stable/243630 (accessed October 10, 2019).
Fink, A. (2006). “Supply chain coordination by means of automated negotiations between autonomous agents,” in Multiagent based Supply Chain Management. Studies in Computational Intelligence, eds B. Chaib-draa, and J. P. Müller (Berlin, Heidelberg: Springer), 351–372. doi: 10.1007/978-3-540-33876-5_13
Foss, N. J. (1993). Theories of the firm: contractual and competence perspectives. J. Evol. Econ. 3,127–144. doi: 10.1007/BF01213830
Fournier, F., and Skarbovsky, I. (2019). “Enriching smart contracts with temporal aspects,” in Blockchain – ICBC 2019. ICBC 2019. Lecture Notes in Computer Science, Vol. 11521, eds J. Joshi, S. Nepal, Q. Zhang, and L. J. Zhang (Cham: Springer), 126–141. doi: 10.1007/978-3-030-23404-1_9
Francisco, K., and Swanson, D. (2018). The supply chain has no clothes: technology adoption of blockchain for supply chain transparency. Logistics 2:2. doi: 10.3390/logistics2010002
Garrod, J. Z. (2016). The real world of the decentralized autonomous society. Triplec-Commun. Cap. Crit. 14, 62–77. doi: 10.31269/triplec.v14i1.692
Gartner (2017). Gartner Says Supply Chain Management Market Will Exceed $13 Billion in 2017, Up 11 Percent from 2016. Available online at: https://www.gartner.com/en/newsroom/press-releases/2017-06-22-gartner-says-supply-chain-management-market-will-exceed-13-billion-in-2017-up-11-percent-from-2016 (accessed October 17, 2019).
Gartner (2020). Gartner Information Technology Glossary – Defining Digitalization. Available online at: https://www.gartner.com/en/information-technology/glossary/digitalization (accessed December 15, 2020).
Gimenez, C., Sierra, V., and Rodon, J. (2012). Sustainable operations: their impact on the triple bottom line. Int. J. Prod. Econ. 140, 149–159. doi: 10.1016/j.ijpe.2012.01.035
Giusti, R., Iorfida, C., Li, Y., Manerba, D., Musso, S., Perboli, G., et al. (2019). Sustainable and de-stressed international supply-chains through the SYNCHRO-NET approach. Sustainability 11:1083. doi: 10.3390/su11041083
Gladden, M. E. (2018). “Dynamics of technological posthumanization: distinguishing the anticipated paths of developed and emerging economies,” in Proceedings of the International Conference on ICT Management for Global Competitiveness and Economic Growth in Emerging Economies (Wroclaw, Poland), 98–109. Available online at: https://ictm2018.edukacja.wroc.pl/docs/ICTM2018_Proceedings.pdf (accessed November 12, 2020).
Gladden, M. E. (2019). Who will be the members of society 5.0? Towards an anthropology of technologically posthumanized future societies. Soc. Sci. 8:148. doi: 10.3390/socsci8050148
Gloeckler, J., and Herneth, C. (2000). Networked Value Chains NVC – eFulfillment. Ernst and Young Global Office of Supply Chain Technology. Available online at: https://www.researchgate.net/publication/336686143_Networked_Value_Chain_NVC_Technologies_eF_v041_short_HEC (accessed September 12, 2019).
Goertzel, B., Giacomelli, S., Hanson, D., Pennachin, C., and Argentieri, M. (2017). SingularityNET: A Decentralized, Open Market and Inter-Network for AIs. Available online at: https://public.singularitynet.io/whitepaper.pdf (accessed October 10, 2019).
Greenspan, G. (2016). Beware the Impossible Smart Contract. The Three Most Common Smart Contract Misconceptions. Available online at: https://www.multichain.com/blog/2016/04/beware-impossible-smart-contract/ (accessed August 4, 2019).
Groschopf, W., Fürst, E. W., Kummer, S., and Herold, D. M. (2019). Identifying carbon reduction potentials in road transportation: creating a carbon-tracking tool for small and medium enterprises (SMEs). Environ. Clim. Technol. 23, 98–110. doi: 10.2478/rtuect-2019-0007
Harayama, Y. (2017): Society 5.0: aiming for a new human-centered society Japan's science and technology policies for addressing global social challenges. Hitachi Rev. 66, 558–559. Available online at: https://www.hitachi.com/rev/archive/2017/r2017_06/pdf/p08-13_TRENDS.pdf (accessed January 5, 2021).
Hardin, G. (1968). The tragedy of the commons. Science 162, 1243–1248. doi: 10.1126/science.162.3859.1243
Herbrechter, S. (2013). Posthumanism – A Critical Analysis. London; New York, NY: Bloomsbury Academic.
Herold, D. M., Farr-Wharton, B., Lee, K.-H., and Groschopf, W. (2018). The interaction between institutional and stakeholder pressures: advancing a framework for categorising carbon disclosure strategies. Bus Strat Dev. 2, 77–90. doi: 10.1002/bsd2.44
Hodgson, G. M. (1998). Competence and contract in the theory of the firm. J. Econ. Behav. Organ. 35, 179–201. doi: 10.1016/S0167-2681(98)00053-5
Hoffman, G., and Breazeal, C. (2004). “Collaboration in human-robot teams,” in AIAA 1st Intelligent Systems Technical Conference, September 20-22, 2004 (Chicago, IL). doi: 10.2514/6.2004-6434
Hu, Y., Liyanage, M., Manzoor, M., Thilakarathna, K., Jourjon, G., and Seneviratne, A. (2019). Blockchain-based smart contracts - applications and challenges. arXiv arXiv:1810.04699
Huh, S., Cho, H., and Kim, S. (2017). “Managing IoT devices using blockchain platform,” in 19th International Conference on Advanced Communication Technology (ICACT) (PyeongChang). doi: 10.23919/ICACT.2017.7890132
Hurwitz, S. (2019). Green Smart Contracts: There's More to Blockchain Energy Consumption than Consensus. Available online at: https://hackernoon.com/green-smart-contracts-theres-more-to-blockchain-energy-consumption-than-consensus-898fb23eea75 (accessed September 15, 2019).
Iqbal, T., and Riek, L. D. (2019). “Human-robot teaming: approaches from joint action and dynamical systems,” in Humanoid Robotics: A Reference, eds A. Goswami, and P. Vadakkepat (Dordrecht: Springer), 2293–2312. doi: 10.1007/978-94-007-6046-2_137
ISDA (2017). Smart Contracts and Distributed Ledger - A Legal Perspective. Available online at: https://www.isda.org/a/6EKDE/smart-contracts-and-distributed-ledger-a-legal-perspective.pdf (accessed October 2, 2019).
Jap, S. D., and Ganesan, S. (2000). Control mechanisms and the relationship life cycle: implications for safeguarding specific investments and developing commitment. J. Mark. Res. XXXVII, 227–245. doi: 10.1509/jmkr.37.2.227.18735
Jording, T., and Sucky, E. (2016). “Improving the development of supply chain management maturity models by analyzing design characteristics,” in Supply Management Research. Advanced Studies in Supply Management, eds R. Bogaschewsky, M. Eßig, R. Lasch, and W. Stölzle (Wiesbaden: Springer Gabler), 96–119. doi: 10.1007/978-3-658-08809-5_5
Kalinowski, T. B. (2016). Analysis of business process maturity and organizational performance relations. Management 20, 87–101. doi: 10.1515/manment-2015-0052
Kamiya, G. (2019). Commentary: Bitcoin Energy Use - Mined the Gap. Available online at: https://www.iea.org/newsroom/news/2019/july/bitcoin-energy-use-mined-the-gap.html (accessed September 20, 2019).
Kim, Y. K., and Davis, G. F. (2016). Challenges for global supply chain sustainability: evidence from conflict minerals reports. Acad. Manage. J. 59, 1896–1916. doi: 10.5465/amj.2015.0770
Kirchherr, J., Reike, D., and Hekkert, M. (2017). Conceptualizing the circular economy: an analysis of 114 definitions. Resour. Conserv. Recycl. 127, 221–232. doi: 10.1016/j.resconrec.2017.09.005
Kito, T., Brintrup, A., New, S., and Reed-Tsochas, F. (2014). The structure of the toyota supply network: an empirical analysis. SSRN Electron. J. doi: 10.2139/ssrn.2412512
Klötzer, C., and Pflaum, A. (2017). “Toward the development of a maturity model for digitalization within the manufacturing industry's supply chain,” in Proceedings of the 50th Hawaii International Conference on System Sciences (Waikoloa, Hawaii), 4210–4219. doi: 10.24251/HICSS.2017.509
Kohlegger, M., Maier, R., and Thalmann, S. (2009). “Understanding maturity models. Results of a structured content analysis,” in Proceedings of I-KNOW'09 and I-SEMANTICS'09, September 2-4, 2009 (Graz), 51–61.
Kosba, A., Miller, A., Shi, E., Wen, Z., and Papamanthou, C. (2016). “Hawk: The Blockchain Model of Cryptography and Privacy-Preserving Smart Contracts,” in 2016 IEEE Symposium on Security and Privacy (SP) (San Jose, CA), 839–858. doi: 10.1109/SP.2016.55
Kouhizadeh, M., and Sarkis, J. (2018). Blockchain practices, potentials, and perspectives in greening supply chains. Sustainability 10:3652. doi: 10.3390/su10103652
Kummer, S., and Groschopf, W. (2019). “Grundlagen der betrieblichen leistungserstellung,” in Grundzüge der Beschaffung, Produktion und Logistik, eds S. Kummer, (Hrsg.), O. Grün, and W. Jammernegg (Hallbergmoos: Pearson Education), 23–131.
Kurnia, S., Rahim, M. M., Samson, D., and Singh, P. (2014). “Sustainable supply chain management capability maturity: framework development and initial evaluation,” in Proceedings of the 22nd European Conference on Information Systems (ECIS) (Tel Aviv), 1–10.
Lahti, M., Shamsuzzoha, A. H. M., and Helo, P. (2009). Developing a maturity model for Supply Chain Management. Int. J. Logistics Syst. Manag. Vol. 5, 654–678. doi: 10.1504/IJLSM.2009.024796
Lauslahti, K., Mattila, J., Hukkinen, T., and Seppälä, T. (2018). “Expanding the Platform: Smart Contracts as Boundary Resources,” in Collaborative Value Co-creation in the Platform Economy. Translational Systems Sciences, Vol. 11, eds A. Smedlund, A. Lindblom, and L. Mitronen (Singapore: Springer), 65–90. doi: 10.1007/978-981-10-8956-5_4
Lauslahti, K., Mattila, J., and Seppälä, T. (2017). Smart contracts – how will blockchain technology affect contractual practices? ETLA Rep. doi: 10.2139/ssrn.3154043
LawDepot (2019). Are Verbal Agreements Legally Binding? Available online at: https://www.lawdepot.com/blog/are-verbal-agreements-legally-binding/ (accessed December 5, 2020).
Lee, H. L. (2004). The triple-A supply chain. Harv. Bus. Rev. 82, 102–112. Available online at: https://hbr.org/2004/10/the-triple-a-supply-chain (accessed September 2, 2019).
Lee, H. L., and Whang, S. (2000). Information sharing in a supply chain. Int. J. Manufact. Technol. Manage. 1, 79–93. doi: 10.1504/IJMTM.2000.001329
Linton, J. D., Klassen, R., and Jayaraman, V. (2007). Sustainable supply chains: an introduction. J. Oper. Manage. 25, 1075–1082. doi: 10.1016/j.jom.2007.01.012
Lockamy, I. I. I. A, Childerhouse, P., Disney, S. M., Towill, D. R., and McCormack, K. (2008). The impact of process maturity and uncertainty on supply chain performance: an empirical study. Int. J. Manufact. Technol. Manage. 15, 12–27. doi: 10.1504/IJMTM.2008.018237
Lockamy, I. I. I. A., and McCormack, K. (2004). The development of a supply chain management process maturity model using the concepts of business process orientation. Supply Chain Manage. 9, 272–278. doi: 10.1108/13598540410550019
Loebbecke, C., and Picot, A. (2015). Reflections on societal and business model transformation arising from digitization and big data analytics: a research agenda. J. Strateg. Inf. Syst. 24, 149–157. doi: 10.1016/j.jsis.2015.08.002
Macrinici, D., Cartofeanu, C., and Gao, S. (2018). Smart contract applications within blockchain technology: a systematic mapping study. Telematics Informatics 35, 2337–2354. doi: 10.1016/j.tele.2018.10.004
Manuj, I., and Mentzer, J. T. (2008). Global supply chain risk management. J. Bus. Logistics 29, 133–155. doi: 10.1002/j.2158-1592.2008.tb00072.x
Matos, F. J. F., and Silva, F. J. F. (2011). The rebound effect on road freight transport: empirical evidence from Portugal. Energy Policy 39, 2833–2841. doi: 10.1016/j.enpol.2011.02.056
Mendling, J., Weber, I., Van Der Aalst, W., Vom Brocke, J., Cabanillas, C., Daniel, F., et al. (2018). Blockchains for business process management - challenges and opportunities. ACM Trans. Manage. Inf. Syst. 9:1. doi: 10.1145/3183367
Mentzer, J. T., DeWitt, W., Keebler, J. S., Min, S., Nix, N. W., Smith, C. D., et al. (2001). Defining supply chain management. J. Bus. Logistics 22, 1–25. doi: 10.1002/j.2158-1592.2001.tb00001.x
Mesquita, L. F., and Brush, T. H. (2008). Untangling safeguard and production coordination effects in long-term buyer-supplier relationships. Acad. Manage. J. 51, 785–807. doi: 10.5465/amr.2008.33665612
Mik, E. (2017). Smart contracts: terminology, technical limitations and real world complexity. Law Innov. Technol. 9, 269–300. doi: 10.1080/17579961.2017.1378468
Min, H. (2019). Blockchain technology for enhancing supply chain resilience. Bus. Horiz. 62, 35–45. doi: 10.1016/j.bushor.2018.08.012
Nakamoto, S. (2008). Bitcoin: A Peer-to-Peer Electronic Cash System. Available online at: https://cryptoexpert.net/wp-content/uploads/2018/11/bitcoinwhitepaper.pdf (accessed September 9, 2019).
Narayanan, V. G., and Raman, A. (2004). Aligning incentives in supply chains. Harvard Bus. Rev. 82, 94–102. Available online at: https://portal.regenesys.net/course/discussions/editors/kcfinder/upload/files/HBR%20Aligning%20Incentives%20in%20Supply%20Chains%2C%202004.pdf (accessed December 5, 2020).
Newell, A. (1979). “Reasoning, Problem Solving And Decision Processes: The Problem Space as a Fundamental Category,” Carnegie Mellon University Research Showcase CMU. Available online at: http://repository.cmu.edu/cgi/viewcontent.cgi?article=2574andcontext=compsci (accessed October 10, 2019).
Nikolić, I., Kolluri, A., Sergey, I., Saxena, P., and Hobor, A. (2018). “Finding the greedy, prodigal, and suicidal contracts at scale,” in Proceedings of the 34th Annual Computer Security Applications Conference (ACSAC '18) (New York, NY: ACM), 653–663. doi: 10.1145/3274694.3274743
Nofer, M., Gomber, P., Hinz, O., and Schiereck, D. (2017). Blockchain. Bus. Inf. Syst. Eng. 59, 183–187. doi: 10.1007/s12599-017-0467-3
Norta, A., Othman, A. B., and Taveter, K. (2015). “Conflict-resolution lifecycles for governed decentralized autonomous organization collaboration,” in Proceedings of the 2015 2nd International Conference on Electronic Governance and Open Society: Challenges in Eurasia (EGOSE'15) (St. Petersburg, Russian Federation). doi: 10.1145/2846012.2846052
Object Management Group (OMG) (2019). Decision Model and Notation Standard (DMN), v1.2 from January 2019. Available online at: https://www.omg.org/spec/DMN/1.2/ (accessed October 12, 2019)
Omohundro, S. (2014). Cryptocurrencies, smart contracts, and artificial intelligence. AI Matters 1, 19–21. doi: 10.1145/2685328.2685334
Paché, G., and Spalanzani, A. (2007). La gestion des Chaines Logistiques Multi-Acteurs: Perspectives Stratégiques, Ed. PUG.
Pãnescu, A.-T., and Manta, V. (2018). Smart contracts for research data rights management over the ethereum blockchain network. Sci. Technol. Libr. 37, 235–245. doi: 10.1080/0194262X.2018.1474838
Park, L. W., Lee, S., and Chang, H. (2018). A sustainable home energy prosumer-chain methodology with energy tags over the blockchain. Sustainability 10:658. doi: 10.3390/su10030658
Paulk, M. C. (2002). “Capability maturity model for software,” in Encyclopedia of Software Engineering, ed J. J. Marciniak. (New York, NY: John Wiley & Sons), 97–112. doi: 10.1002/0471028959.sof589
Paulk, M. C., Curtis, B., Chrissis, M. B., Averill, E. L., Bamberger, J., Kasse, T. C., et al. (1991). Capability Maturity Model for Software. CMU/SEI-91-TR-24. Pittsburgh: Carnegie Mellon University, Software Engineering Institute.
Porter, M. E., and Kramer, M. R. (2011). How to Reinvent Capitalism-and Unleash a Wave of Innovation and Growth. Harvard Business Review. p. 63–70.
Prause, G. (2019). “Smart contracts for smart supply chains,” in 9th IFAC/IFIP/IFORS/IISE/INFORMS Conference Manufacturing Modelling, Management and Control (MIM 2019) (Berlin). Available online at: https://www.researchgate.net/profile/Gunnar_Prause/publication/334262502_Smart_Contracts_for_Smart_Supply_Chains/links/5d1f844692851cf44068de37/Smart-Contracts-for-Smart-Supply-Chains.pdf (accessed September 5, 2019).
Queiroz, M. M., Telles, R., and Bonilla, S. H. (2019). Blockchain and supply chain management integration: a systematic review of the literature. Supply Chain Manag. 25, 241–254. doi: 10.1108/SCM-03-2018-0143
Reefke, H., Ahmed, M. D., and Sundaram, D. (2014). Sustainable supply chain management - Decision making and support: the SSCM maturity model and system. Glob. Bus. Rev. 15, 1S−12S. doi: 10.1177/0972150914550138
Reefke, H., Sundaram, D., and Ahmed, M. D. (2010). “Maturity progression model for sustainable supply chains,” in Advanced Manufacturing and Sustainable Logistics. IHNS 2010. Lecture Notes in Business Information Processing, 46, eds W. Dangelmaier, A. Blecken, R. Delius, and S. Klöpfer (Springer, Berlin, Heidelberg), 308–319. doi: 10.1007/978-3-642-12494-5_28
Rejeb, A., Keogh, J. G., Zailani, S., Treiblmaier, H., and Rejeb, K. (2020). Blockchain technology in the food industry: a review of potentials, challenges and future research directions. Logistics 4:27. doi: 10.3390/logistics4040027
Reyna, A., Martín, C., Chen, J., Soler, E., and Díaz, M. (2018). On blockchain and its integration with IoT. Challenges and opportunities. Fut. Gen. Comput. Syst. 88, 173–190. doi: 10.1016/j.future.2018.05.046
Risius, M., and Spohrer, K. (2017). A blockchain research framework - what we (don't) know, where we go from here, and how we will get there. Bus. Inf. Syst. Eng. 59, 385–409. doi: 10.1007/s12599-017-0506-0
Robinson, P. (2020). The merits of using ethereum mainnet as a coordination blockchain for ethereum private sidechains. Knowl. Eng. Rev. 35:E30. doi: 10.1017/S0269888920000296
Rockwell Automation. (2014). The Connected Enterprise Maturity Model. Available online at: http://literature.rockwellautomation.com/idc/groups/literature/documents/wp/cie-wp002_-en-p.pdf (accessed October 4, 2019).
Rodewald, J., Colombi, J., Oyama, K., and Johnson, A. (2015). Using information-theoretic principles to analyze and evaluate complex adaptive supply network architectures. Procedia Comput. Sci. 61, 147–152. doi: 10.1016/j.procs.2015.09.176
Rosic, A. (2017). What is Ethereum Gas? Available online at: https://blockgeeks.com/guides/ethereum-gas/ (accessed September 20, 2019).
Rudnicka, A. (2017). Sustainable supply chain maturity model. Res. Logistics Prod. 7, 201–209. doi: 10.21008/j.2083-4950.2017.7.3.2
Saberi, S., Kouhizadeh, M., Sarkis, M., and Shen, L. (2019). Blockchain technology and its relationships to sustainable supply chain management. Int. J. Prod. Res. 57, 2117–2135. doi: 10.1080/00207543.2018.1533261
Sala, S., Ciuffo, B., and Nijkamp, P. (2015). A systemic framework for sustainability assessment. Ecol. Econ. 119, 314–325. doi: 10.1016/j.ecolecon.2015.09.015
Salmerón-Manzano, E., and Manzano-Agugliaro, F. (2019). The role of smart contracts in sustainability: worldwide research trends. Sustainability 11:3049. doi: 10.3390/su11113049
Sato, Y. (2019). Japan Pushing Ahead With Society 5.0 to Overcome Chronic Social Challenges. Available online at: https://en.unesco.org/news/japan-pushing-ahead-society-50-overcome-chronic-social-challenges (accessed November 4, 2020).
Savelyev, A. (2017). Contract law 2.0: “Smart” contracts as the beginning of the end of classic contract law. Inf. Commun. Technol. Law 26, 116–134. doi: 10.1080/13600834.2017.1301036
Schumacher, A., Erol, S., and Sihn, W. (2016). A maturity model for assessing Industry 4.0 readiness and maturity of manufacturing enterprises. Procedia CIRP 52, 161–166. doi: 10.1016/j.procir.2016.07.040
Schwartz, A., and Scott, R. E. (2003). Contract theory and the limits of contract law. Yale Law J. 113, 541–620. doi: 10.2307/3657531
Seele (2018). Building the Internet of Value for the future. Seele Whitepaper. Available online at: https://whitepaper.io/document/369/seele-whitepaper (accessed October 12, 2019).
Seuring, S., and Gold, S. (2012). Conducting content-analysis based literature reviews in supply chain management. Supply Chain Manage. 17, 544–555. doi: 10.1108/13598541211258609
Seuring, S., and Müller, M. (2008). From a literature review to a conceptual framework for sustainable supply chain management. J. Clean. Prod. 16, 1699–1710. doi: 10.1016/j.jclepro.2008.04.020
Shen, L., Sub, C., Zhengb, Y., and Zhuangc, G. (2020). Between contracts and trust: disentangling the safeguarding and coordinating effects over the relationship life cycle. Indus. Mark. Manage. 84, 183–193. doi: 10.1016/j.indmarman.2019.06.006
Sillaber, C., and Waltl, B. (2017). Life cycle of smart contracts in blockchain ecosystems. Datenschutz Datensicherheit – DuD 41, 497–500. doi: 10.1007/s11623-017-0819-7
Simpson, J. A., and Weiner, E. S. C. (1989). The Oxford English Dictionary. Oxford: Oxford University Press.
Sklaroff, J. M. (2017). Smart contracts and the cost of inflexibility. Univ. Pennsylvania Law Rev. 166:263. Available online at: https://heinonline.org/HOL/P?h=hein.journals/pnlr166andi=267 (accessed September 8, 2019).
Smith, P., and Allee, V. (2009). Value creating networks: organizational issues and challenges. Learn. Organ. 16, 427–442. doi: 10.1108/09696470910993918
Sörqvist, P, and Langeborg, L. (2019). Why people harm the environment although they try to treat it well: an evolutionary-cognitive perspective on climate compensation. Front. Psychol. 10:348. doi: 10.3389/fpsyg.2019.00348
Stabell, C., and Fjeldstad, Ø. D. (1998). Configuring value for competitive advantage: on chains, shops, and networks. Strat. Manage. J. 19, 413–437. doi: 10.1002/(SICI)1097-0266(199805)19:5andlt;413::AID-SMJ946andgt;3.0.CO;2-C
Stark, J. (2016). Making Sense of Blockchain Smart Contracts. Available online at: https://www.coindesk.com/making-sense-smart-contracts (accessed September 8, 2019).
Strategy & PWC. (2017). Industry 4.0. How Digitization Makes the Supply Chain More Efficient, Agile, and Customer-Focused. Available online at: https://www.strategyand.pwc.com/gx/en/reports/industry-4-0.pdf (accessed August 11, 2019).
Suchman, M. C. (2003). The contract as social artifact. Law Soc. Rev. 37, 91–142. doi: 10.1111/1540-5893.3701003
Sultana, T., Almogren, A., Akbar, M., Zuair, M., Ullah, I., and Javaid, N. (2020). Data sharing system integrating access control mechanism using blockchain-based smart contracts for IoT devices. Appl. Sci. 10:488. doi: 10.3390/app10020488
Surujnath, R. (2017). Off the chain: a guide to blockchain derivatives markets and the implications on systemic risk. Fordham J. Corp. Finan. Law 22, 257–304. Available online at: https://ir.lawnet.fordham.edu/jcfl/vol22/iss2/3 (accessed September 14, 2019).
Swan, M. (2015). Blockchain – Blueprint for a New Economy. Available online at: https://isidore.co/calibre/legacy/get/PDF/5503/CalibreLibrary/Blockchain_%20Blueprint%20for%20a%20Ne%20-%20Swan%2C%20Melanie_5503.pdf (accessed November 5, 2020).
Szabo, N. (1997). Formalizing and securing relationships on public networks. First Monday 2:9. doi: 10.5210/fm.v2i9.548
Tarhan, A., Turetken, O., and Reijers, H. A. (2016). Business process maturity models: a systematic literature review. Inf. Software Technol. 75, 122–134. doi: 10.1016/j.infsof.2016.01.010
Tate, W. L., Ellram, L. M., and Kirchoff, J. F. (2010). Corporate social responsibility reports: a thematic analysis related to supply chain management. J. Supply Chain Manage. 46, 19–44. doi: 10.1111/j.1745-493X.2009.03184.x
Tornell, A., and Velasco, A. (1992). The tragedy of the commons and economic growth: why does capital flow from poor to rich countries? J. Political Econ. 100, 1208–1231. doi: 10.1086/261858
Treiblmaier, H. (2018). The impact of the blockchain on the supply chain: a theory-based research framework and a call for action. Supply Chain Manage. 23/6, 545–559. doi: 10.1108/SCM-01-2018-0029
Treiblmaier, H. (2019). Toward more rigorous blockchain research: recommendations for writing blockchain case studies. Front. Blockchain 2:3. doi: 10.3389/fbloc.2019.00003
Truby, J. (2018). Decarbonizing bitcoin: law and policy choices for reducing the energy consumption of blockchain technologies and digital currencies. Energy Res. Soc. Sci. 44, 399–410. doi: 10.1016/j.erss.2018.06.009
Tummala, R., and Schoenherr, T. (2011). Assessing and managing risks using the supply chain risk management process (SCRMP). Supply Chain Manage. 16/6, 474–483. doi: 10.1108/13598541111171165
United Nations (2015). Transforming Our World – the 2030 Agenda for Sustainable Development. Available online at: https://sustainabledevelopment.un.org/content/documents/21252030%20Agenda%20for%20Sustainable%20Development%20web.pdf (accessed August 19, 2019).
Unterweger, A., Knirsch, F., Leixnering, C., and Engel, D. (2018). “Lessons learned from implementing a privacy-preserving smart contract in ethereum,” in 2018 9th IFIP International Conference on New Technologies, Mobility and Security (NTMS) (Paris), 1–5. doi: 10.1109/NTMS.2018.8328739
Varoutsaa, E., and Scapens, R. W. (2015). The governance of inter-organisational relationships during different supply chain maturity phases. Indus. Mark. Manage. 46, 68–82. doi: 10.1016/j.indmarman.2015.01.006
Ventura, T. (2020). The World's Fastest Blockchain Exceeds 1 Million Transactions Per Second. Available online at: https://medium.com/predict/the-worlds-fastest-blockchain-exceeds-1-million-transactions-per-second-8931df09320d (accessed January 14, 2021).
Voshmgir, S. (2017). Disrupting governance with blockchains and smart contracts. Strat. Change. 26, 499–509. doi: 10.1002/jsc.2150
Vranken, H. (2017). Sustainability of bitcoin and blockchains. Curr. Opin. Environ. Sustain. 28, 1–9. doi: 10.1016/j.cosust.2017.04.011
Wang, Y., Han, J. H., and Beynon-Davies, P. (2019). Understanding blockchain technology for future supply chains: a systematic literature review and research agenda. Supply Chain Manag. 24, 62–84. doi: 10.1108/SCM-03-2018-0148
WCED (1987). Report of the World Commission on Environment and Development: Our Common Future. United Nations General Assembly document A/42/427.
Weber, I., Xu, X., Riveret, R., Governatori, G., Ponomarev, A., and Mendling, J. (2016). “Untrusted business process monitoring and execution using blockchain,” in Business Process Management. BPM 2016. Lecture Notes in Computer Science 9850, eds M. La Rosa, P. Loos, and O. Pastor (Cham: Springer). doi: 10.1007/978-3-319-45348-4_19
Wheeler, S. (2017). Modelling the contracts of the future. Griffith Law Rev. 26, 593–614. doi: 10.1080/10383441.2017.1552547
Wood, G. (2017). Ethereum: A Secure Decentralized Generalized Transaction Ledger - EIP-150 REVISION. Available online at: https://gavwood.com/paper.pdf (accessed September 11, 2019).
Wright, A., and De Filippi, P. (2015). Decentralized blockchain technology and the rise of lex cryptographia. SSRN. doi: 10.2139/ssrn.2580664
Wu, J., and Tran, N. K. (2018). Application of blockchain technology in sustainable energy systems: an overview. Sustainability 10:3067. doi: 10.3390/su10093067
Xu, M., Chen, X., and Kou, G. (2019). A systematic review of blockchain. Finan. Innov. 5. doi: 10.1186/s40854-019-0147-z
Yang, W., Garg, S., Raza, A., Herbert, D., and Kang, B. (2018). “Blockchain: trends and future,” in Knowledge Management and Acquisition for Intelligent Systems. PKAW 2018. Lecture Notes in Computer Science, 11016, eds K. Yoshida, and M. Lee (Cham: Springer), 201–210. doi: 10.1007/978-3-319-97289-3_15
York, R., and McGee, J. A. (2016). Understanding the jevons paradox. Environ. Sociol. 2, 77–87. doi: 10.1080/23251042.2015.1106060
Yu, B., Zhang, J., and Fujiwara, A. (2013). Rebound effects caused by the improvement of vehicle energy efficiency: an analysis based on a SP-off-RP survey. Transportation Res. D 24, 62–68. doi: 10.1016/j.trd.2013.06.001
Keywords: supply chain management, smart contracts, blockchain, sustainability, supply chain maturity model, living system, networked value chain, research propositions
Citation: Groschopf W, Dobrovnik M and Herneth C (2021) Smart Contracts for Sustainable Supply Chain Management: Conceptual Frameworks for Supply Chain Maturity Evaluation and Smart Contract Sustainability Assessment. Front. Blockchain 4:506436. doi: 10.3389/fbloc.2021.506436
Received: 30 October 2019; Accepted: 08 March 2021;
Published: 09 April 2021.
Edited by:
Xiaonan Wang, National University of Singapore, SingaporeReviewed by:
John G. Keogh, University of Reading, United KingdomAndreas Freund, Independent Researcher, San Diego, United States
Copyright © 2021 Groschopf, Dobrovnik and Herneth. This is an open-access article distributed under the terms of the Creative Commons Attribution License (CC BY). The use, distribution or reproduction in other forums is permitted, provided the original author(s) and the copyright owner(s) are credited and that the original publication in this journal is cited, in accordance with accepted academic practice. No use, distribution or reproduction is permitted which does not comply with these terms.
*Correspondence: Wolfram Groschopf, wolfram.groschopf@wu.ac.at