- 1Department of Planning, Aalborg University, Aalborg, Denmark
- 2School of Business, Worcester Polytechnic Institute, Worcester, MA, United States
- 3Hanken School of Economics, Humlog Institute, Helsinki, Finland
Blockchain technology has been promised as a solution to social and environmental issues in supply chains. The potential includes reduction of vulnerable party exploitation and avoiding environmentally harmful practices. Yet, it remains unclear how these potential improvements are created and whether blockchain can truly contribute. Therefore, this field study explores and identifies the mechanisms for blockchain technology to facilitate positive social and environmental impacts in supply chains. We applied an explorative qualitative research approach and interviewed blockchain technology implementers and practitioners that allowed a detailed analysis of this problem despite the scarcity of practice data. The results include the development of a middle-range theory that shows barriers and drivers of blockchain-based technologies in supply chains, introduces the concept of blockchain-enabled system, and outlines expected outcomes and impacts. We further identify four impact pathways that describe how blockchain-enabled system create positive impact: (voluntary) market mechanisms, plausibility checks, smart contracts and tokenisation, and peer-to-peer trust. The study contributes by providing insights into “how” blockchain-based technologies in supply chains can lead to social and environmental impacts. The study also furthers the discussion on blockchain technology’s role in supply chain implementation and addresses the yet unresolved problem of measuring the impact of such blockchain-enabled systems.
1 Introduction
Globalisation has made modern supply chains increasingly complex as technology, culture, and value chain activities become entwined and supply chains reach deep into various regions of the world. Companies often do not know their tier three or four suppliers; with limited visibility beyond the first tier. Similarly, producers do not always know who consumes or manages the materials they supply. Information flow and visibility between these and other actors in supply chains is low. Thus, identifying product or material sourcing and process activities that include questionable and illegal practices including human rights abuses, environmental damage, or fraud is extremely difficult (Clarke and Boersma, 2017). Blockchain technology proclamations include the ability to solve the problem of lack of trusted information from supply chains and consequently increase visibility (Feng et al., 2020; Wang et al., 2019). In short, blockchain technology is a decentralised add-only ledger that makes it virtually impossible to change data that has been entered. In supply chains, blockchain technology is implemented together with other components—such as tracking technologies—to address issues of traceability (Köhler and Pizzol, 2020).
These blockchain-based technologies in supply chains can bring advantages in terms of transparency and efficiency (Köhler and Pizzol, 2020). Based on their recent literature review, Lim et al. (2021) state that there are three major aspects that blockchain technology improve current supply chains: shareability, security, and smart capabilities. Some of the most prominent potentials in that respect include preventing data fraud, eliminating intermediaries, reducing time and cost of transactions, enabling secure data sharing, and automating processes (Lim et al., 2021). For example, IBM and Walmart implemented a blockchain-based traceability system for mangoes that reduced the time for tracking product origins from 7 days to 2.2 s (Kamath, 2018). In another project, Maersk and IBM collaborated to increase efficiencies of international supply chains by using blockchain technology to securely share data among trading partners and replace hardcopies with digital records (Kouhizadeh et al., 2020). Blockchain-based technologies together with other technologies are expected to become the standard for tracing and sharing product-related information (Kopyto et al., 2020).
One interesting statement in the latest review of blockchain applications in the supply chain by Lim et al. (2021) is that “the discussion on sustainability seems to be limited compared with other subthemes”. They further note that there is no research that systematically proposes a sustainable supply chain performance model using blockchain technology (Lim et al., 2021). This suggests that the research on sustainability of blockchain-based technologies in supply chains is currently underdeveloped. While there are numerous studies exploring the potential of blockchain-based technologies in supply chains (Azzi et al., 2019; Rejeb et al., 2020), only very few focus explicitly on sustainability. Kamilaris et al. (2019) identify among other points the potential of blockchain technology to support small farmers, create a platform for emission reduction efforts, reduce fraud, and generate consumer awareness. Kouhizadeh and Sarkis (2018) highlight that trusted information and visibility provided by blockchains can support purchasing decisions and selecting sustainable products. They further point out that verified data on blockchain can provide better input and output data for eco-design and life cycle assessment facilitating more sustainable production (Kouhizadeh and Sarkis, 2018).
A close examination of this literature indicates that while existing studies explore and identify what potential benefits blockchain technology in supply chains could bring, they do not address in-depth how such positive impact will be reached. This becomes apparent when looking at how the studies describe the potential. Kamilaris et al. (2019), for example, discuss a “hypothetical scenario” in which a cooperative uses a smart contract to conduct sales of their cereal production and uses auxiliary verbs such as could or might to describe the potentials. Similarly, Kouhizadeh and Sarkis (2018) use verbs such as may throughout their study to illustrate blockchain potentials in supply chains. They further highlight that they have only scratched the surface of the role of blockchain in sustainable supply chain management. There is limited empirical evidence of the fulfilment of these potentials.
Thus, while other fields of research such as big data and developmental studies have consolidated an understanding of the pathway from decisions and actions made to impact on specific endpoints (Dubey et al., 2019; França et al., 2020; Jørgensen et al., 2010; Olney et al., 2009), this has not been investigated for blockchain-based technologies in supply chains. A social science approach can be valuable to address the research question of how to achieve positive impact from blockchain technology—especially in early blockchain technology adoption stages (França et al., 2020).
Therefore, the purpose of our study is to discover the mechanisms by which blockchain-based technologies in supply chains create positive social and environmental impact. Given early and only emergent understanding in this field of study and with limited explanation—theoretically or practically—of how blockchain-based technologies in the supply chain create positive impact, an explorative qualitative research approach is taken. Blockchain-based technology experts, the majority of whom have first-hand experiences implementing the technology in the supply chain, are interviewed. This information provides first-hand insights from some of the few cases that have seen actual blockchain implementation. Since blockchain-based implementations in supply chains are still in early development stages, it would be unrealistic to provide a conclusive explanation of this phenomenon. Thus, we intend to propose a “middle-range theory” that is context specific and should be further tested and adjusted over time (Craighead et al., 2016). This explorative study aims to address the following research questions:
• How and by what mechanisms do blockchain-based technologies in the supply chain lead to positive social and environmental impact?
• What is the role of the blockchain component in the supply chain that generates this impact and can this component’s contribution be separated from the impact generated by other components?
The study contributes to and advances the existing body of knowledge in several ways. Where previous studies mainly focussed on blockchain-based technology impact (Lim et al., 2021), we develop a middle-range theory on how blockchain technology implemented in supply chains creates impact. Additionally, we describe four specific impact pathways. Second, we further discussion blockchain’s role in supply chain operations. Köhler and Pizzol (2020) highlight the importance of taking a systemic perspective—we add to this perspective by evaluating whether blockchain technology can be investigated separately and by highlighting the importance of the system architecture design. Finally, we emphasise early impact measurement while determining the potential of implementing blockchain technology. The actual implementation effects need to be tracked and measured in order to verify the potentials and understand the extent these potentials are met.
2 Materials and Methods
For this study an explorative qualitative research approach was used based on conducting and analysing in-depth interviews. Since the implementation of blockchain-enabled systems is still in the early stages, their impact cannot yet be fully known and measured. A conclusive explanation of the blockchain-impact nexus is thus currently beyond reach. Instead, we propose here a middle-range theory defined as a conceptualisation to a specific context (Craighead et al., 2016). In our case, we derive a conceptual scheme that serves as an explanation to how blockchain-enabled systems in supply chains currently create impact and how experts believe they will create impact in the future. As blockchain-enabled systems advance and are implemented for longer periods of time, the conceptual scheme—our middle-range theory—needs to be tested and may need to be refined. This explorative approach was chosen since there are no previous studies of how blockchains implemented in supply chains generate sustainability impacts. Figure 1 provides an overview of the research procedure.
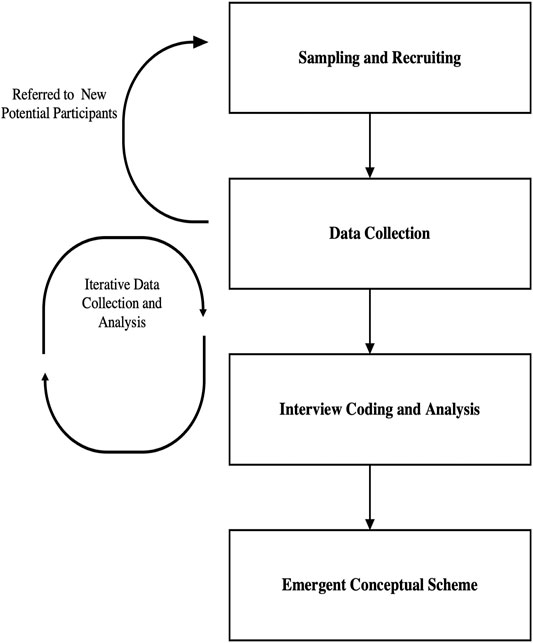
FIGURE 1. Research procedure. The research procedure started with sampling and recruiting study participants before conducting interviews. The interviews were then individually coded before all quotes belonging to one theme were grouped. From some interviews new study participants were identified. The interview and analysis process proceeded iteratively until theoretical saturation was reached. Finally, a conceptual scheme was formulated from the themes that emerged from the interview data.
2.1 Participant Sampling Strategy
We used both theoretical sampling and snowball sampling in this study. In theoretical sampling, participants are selected based on leads in the data (Reilly et al., 2012). For this study, we sought individuals who had first-hand experiences in developing and implementing blockchain-based technologies in supply chains. We specifically did not focus on a single sector and were interested in speaking with different actors including technology developers, brands, consultants or other actors in the supply chain. Theoretical sampling is an iterative process. Based on the results from already conducted interviews, new participants can be identified. In theoretical sampling researchers move back and forth between sampling, data collection, and analysis until data saturation is reached or no new information is collected with new interviews (Tie et al., 2019). We also used snowball sampling. At the end of each interview we asked study participants for additional participant suggestions (Naderifar et al., 2017). Since the study participants typically have contacts in their field, they were able to bring other experts to our attention that we otherwise may have missed.
In total, 16 interviews were conducted. Of the study participants 63% were technology providers, 19% were actors in the direct supply chain, and 19% were other actors such as consultants. 44% of the participants worked on projects in the agricultural sector, 31% in the mining sector, and 25% in the fishing sector. 50% of the interviews were CEOs of which all but one were also the (co-)founders. 22% of the study participants were more technical including chief technology officers and one developer. The remaining participants were from marketing, procurement, product management, and consulting. The majority of the CEOs that participated belonged to small companies (<50 employees) and were considered to be sufficiently involved in the implementation process to be able to discuss it in detail in an interview. Their explanations of how their implementation works confirmed that they were intimately involved in any developments and implementations. The study participants come from more than 10 different countries with the majority of them (69%) being located in Europe.
The projects were built on different blockchains including Ethereum (36%) and Hyperledger Fabric (29%). One study participant stated that they have implemented solutions for clients using different kinds of blockchains, while another said that their solution is built so that they could move to another blockchain if required. Projects involving large companies also favoured Hyperledger solutions. Most of the projects with large companies were within mining supply chains, while projects within agricultural supply chains tended to include smaller companies.
2.2 Interview Procedure
We conducted semi-structured interviews (Kallio et al., 2016). They allowed us to gain detailed information on each implementation, and at the same time dive deep into factors shaping them. It further allowed us to adapt the interview to learn more about participant specific experiences and knowledge. For example, if one study participant mentioned that they will add a specific feature for the next version of their implementation, it was asked if they could talk more about this feature.
We sent interview invitations via e-mail accompanied by a signed invitation letter. In some cases, one reminder was sent. If the contacted person agreed to be interviewed, a call was scheduled, and the interview guide was sent in advance. The interview typically started with an introduction of the interviewer and the project before asking for consent to audio record the conversation. The interview questions were not necessarily asked in the order presented in the interview guide and not necessarily all questions were asked depending on how relevant they were for the specific interview. Additional questions may have been included such as “You mentioned efficiencies. In what ways does blockchain help?” in order to get more detailed answers.
The study participants had the chance to ask questions about the project and the purpose of the study. The interviews were typically conducted via video call and lasted between 30 and 45 min. The interviews were audio recorded with the consent of the participants and then transcribed. Prior to analysis, all identifying information was removed from the transcripts. All interviews were carried out between April 2020 and September 2020. The interview guide (SI.1) can be found in the Supplementary Material. While the interview data are the main information source, other sources were used to support the findings. For example, two study participants demonstrated the software dashboard during the interview. Another study participant sent additional material to understand their case.
2.3 Interview Analysis
The interview analysis was completed using a “quote by theme” matrix and using anonymised transcripts. A qualitative content analysis was completed in order to identify common themes. The analysis was an iterative process and was comprised of different coding rounds until a satisfactory combination of themes was found (Gioia et al., 2012). It was considered satisfactory when the themes did not overlap and allowed for an explanation of the story across all the interviews.
In the first round of coding, three interviews from participants of different industries—agriculture, fishing, and mining—were used to establish a first collection of 54 codes. These codes were then—based on similarities and common meaning—grouped into 17 themes. For example, the codes “network effects” and “automating payements” were both grouped into the theme “identification of efficiencies”. Subsequent interviews were then analysed using the themes only. Relevant quotes from each interview were selected and for each quote the theme was identified. One quote could address several themes. All quotes belonging to one theme were put in a table and analysed to extract a summary for each theme. With each interview analysis it was tested if the previously identified themes were fitting or how they could be adapted. The interview and analysis processes proceeded therefore together and iteratively until theoretical saturation was reached. Theoretical saturation was considered reached when the themes suitably described all interviews and no substantially new content emerged during the interviews that could not be described by the existing themes. The final set of themes could then be grouped into four different dimensions describing the entire topic under analysis, i.e., how blockchain leads to impact. The now organized dimensions and themes were then displayed in a scheme summarising the results. Examples of coding are provided as Supplementary Material (SI.2).
3 Results
The interview data shows that the supply chain implementation of blockchain-based technologies remains in its early stages. While three quarters of the study participants have implemented their blockchain-based technologies, all of the study participants are still improving their implementations.
3.1 How Blockchain-Enabled Systems Create Positive Impact
Figure 2 summarises a conceptual structure that we developed from the analysis of the interview data. The coded interview data was condensed into themes through an iterative process, until reaching saturation. The themes were further grouped into dimensions shown as white boxes in Figure 2. Each dimension and theme is described in the following discussion.
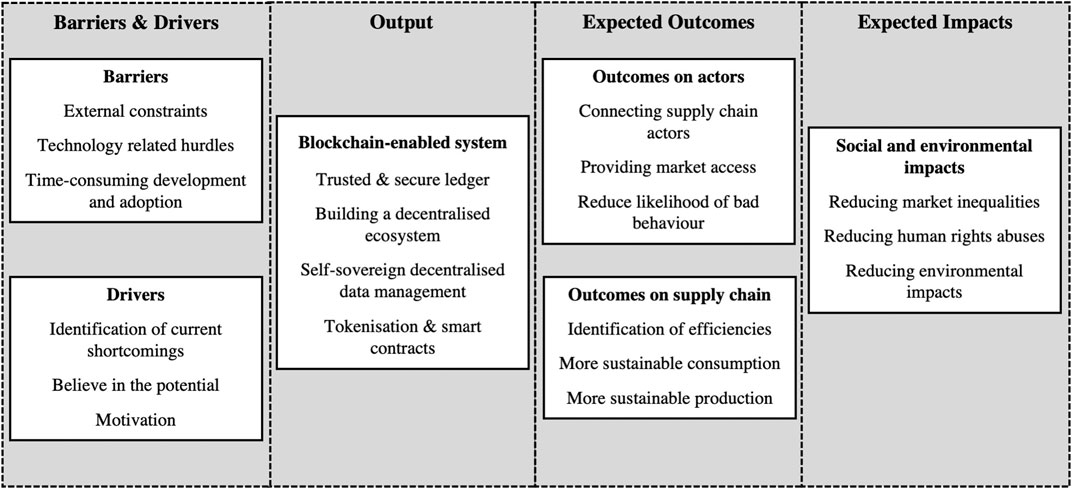
FIGURE 2. Outputs, outcomes, impacts, and factors influencing blockchain-enabled systems. The grey columns resemble a pressures, practices, and performance framework (Choudhary and Sangwan, 2018) where barriers and drivers represent pressures, the output is the practice, and outcomes and impacts are performance. The columns for practice and performance also illustrate an output, outcomes, and impacts approach (Koontz et al., 2020). The output is the decision taken to implement a blockchain-enabled system. The outcomes are the changes directly resulting from the output. The social and environmental impacts are changes on human wellbeing and ecosystem wellbeing—including reductions of existing issues. The white boxes represent dimensions. The bullet points summarise the themes.
We firstly introduce and define the concept of a blockchain-enabled system that emerged from the interview data analysis. A blockchain-enabled system is a system of technologies that uses a blockchain platform to securely manage supply chain data and connect supply chain actors. It is the underlying system of technology enabling positive impact. We then describe how the concept of blockchain-enabled systems is central to and relates with the other elements of our conceptual structure.
3.1.1 Barriers of Blockchain-Enabled System Adoption
This dimension comprises factors that influence the adoption—or lack thereof—of blockchain in supply chains. External constraints to blockchain-enabled systems include a lack of digitisation, social and political instability, and a lack of standards and policies for implementing blockchain-enabled systems.
Technology related barriers refer to blockchain technology immaturity. Some examples of this immaturity include limitations in transaction speed and transaction costs. Governance was also identified as a barrier as “people find a decentralised reality very uncomfortable” and finding agreement across supply chain actors can be time-consuming with no guaranteed consensus causing further delays in decisions and operations.
Time-consuming development and adoption refers to implementation processes that can take a long time requiring substantial resources. For example, digitisation of paper-based processes and the development of trust among actors.
3.1.2 Drivers of Blockchain-Enabled System Adoption
This dimension includes factors that influence and drive implementation of blockchain-enabled system. Identification of current shortcomings refers to the process of isolating existing problems such as diffused and opaque supply chains where little is known about the product journeys or suspecting that the supply chain actors may behave unethically or illegally—for example, human rights violations. Study participants identified the need to move beyond existing centralised solutions resulting from past negative experiences or the need to develop a traceability solution given that they had no existing ones. Other driving factors include the lack of trust and communication in the supply chain and sometimes within their own organisations. Another driver factor is the desire of improving operational inefficiencies that caused delays of payments and shipments. Counterfeit products in the supply chain were also considered a major issue by some study participants. Study participants felt that current systems provided limited data, even for sustainability measures such as product-specific carbon footprints. Knowing true product sustainability characteristics such as product carbon footprints or human rights compliance are needed by companies to truly differentiate products beyond price and quality.
We observed a strong belief among study participants in the potential of blockchain-enabled systems that championed many characteristics. “It’s the future. Embrace it now or embrace it later.”; “For me, it’s the next step of human evolution after the internet.”; “I think it could be revolutionary in the way that supply chains work”; and “The future world is decentralised. It is inevitable.”
The third major driver theme we identified is motivation. Study participants had differing reasons for implementing a blockchain-enabled system in their supply chain: to gain competitive advantages by helping to improve their reputation through building this capability; to protect their brand against supply chain risk; to ensure compliance with regulation; to build a system that is not owned by one entity and creating a platform that competitors want to join and where they feel safe to share data.
3.1.3 Blockchain-Enabled System
Blockchain technology provides a trusted and secure ledger which creates trust in no-trust environments through its immutability.
Blockchain-enabled systems support building a decentralised ecosystem where not one person or entity owns the platform, with many actors involved in its data sharing and management. Blockchain-enabled systems provide a platform for collaboration such that everyone benefits from the data visibility. However, this is also the biggest challenge—finding a governance model that works for everyone involved.
Blockchain technology allows self-sovereign decentralised data management. Participants maintain the ownership of their data and then they can decide who gets access and the type of data to share. This is one of the key advantages of such a system mentioned by the study participants.
Tokenisation and smart contracts allow for automated and faster transactions and payments, which in turn creates liquidity and removes time delays for payments. Tokens—a representation of money, digital items, or real-life objects—further facilitate the connection of consumers and producers through tip-your-farmer schemes, micro-lending, or crowdfunding. It represents an easy and efficient way for payments and incentives to be traded globally.
3.1.4 Outcomes on Actors
This dimension comprises the supply chain actor outcomes from implementing blockchain-enabled systems. Blockchain-enabled systems connect supply chain actors. Every actor’s voice has the possibility of being heard and a peer-to-peer network can supplement or even replace some official systems.
Blockchain-enabled systems can provide market access. “Nobody can stop you from getting in” as one of the study participants framed it.
A decentralised trusted ledger reduces the likelihood of bad behaviour. Mistakes, fraud, and other bad behaviour can more easily be detected on the blockchain and will disincentivise such behaviour. Some bad behaviour could become significantly more difficult to complete—such as using a sustainability certificate for a larger amount that it was granted for.
3.1.5 Outcomes on the Supply Chain
This dimension encompasses the outcomes on the supply chain from implementing blockchain-enabled systems. Efficiencies arise from implementing blockchain-enabled systems through automating payments and contracts, while simplifying processes such as entering a country through a port. These efficiencies save time and money.
Blockchain-enabled systems incentivise more sustainable production. This is based on the assumption that companies either care enough about sustainable supply chains or are held accountable for their supply chains. Companies can use trusted and accessible data on environmental and social hotspots in the supply chain provided by blockchain-enabled systems. Companies can specifically support supply chain actors, ask suppliers to reduce impact, and criteria such as carbon footprints can become procurement conditions—incentivising greener practices.
Blockchain-enabled systems provide incentives for more sustainable consumption. The assumption is that having more specific and trusted information allows consumers to make more informed decisions. Blockchain-enabled systems allow for closer interactions between consumers and producers—even down to individual farmers in agriculture supply chains. Micro-loan schemes, tip-your-farmer schemes, or buying coffee from a specific lot drives loyalty among consumers.
3.1.6 Social and Environmental Impacts
We categorised the social and environmental impacts from implementing blockchain-enabled systems into three themes. These themes reflect participant expectations only, as the impacts have yet to be measured.
Reducing market inequalities include lowering inequities through increased access to markets and financial services for vulnerable supply chain actors. For example, blockchain-enabled systems can support circumventing traditional systems including banking or property registration, for instance by providing access to banking for those who are currently outside formal financial systems.
Blockchain-enabled systems can provide trusted and accessible information about product production including labour conditions, which can support the reduction of human rights abuses. Having visibility of this information allows companies to improve working conditions within their supply chain. With this information consumers can more easily identify products certified to not contain human rights abuses; allowing consumers to make more informed buying decisions. This activity puts pressure on actors who do not provide this information or do so inaccurately. Fairer prices for good actors incentivise good behaviour such as paying a living wage or investing in health insurance for workers.
Reduction of environmental impacts can also be supported by blockchain-enabled systems. Knowing where impacts occur allows supply chain actors to address these concerns. Buyers can directly support supply chain actors in reducing impacts, can incentivise good behaviour through including environmental impacts as a purchasing criterion, and consumers have better information to make buying decisions.
3.2 Impact Pathways and Mechanisms
Based on the analysis of the interview data we derived several possible impact pathways for a blockchain-enabled system to create positive impact. We then identified four mechanisms underlying the impact pathways: (voluntary) market mechanisms, plausibility checks, smart contracts and tokenisation, and peer-to-peer trust. Key examples of these impact pathways and mechanisms are illustrated in Figure 3.
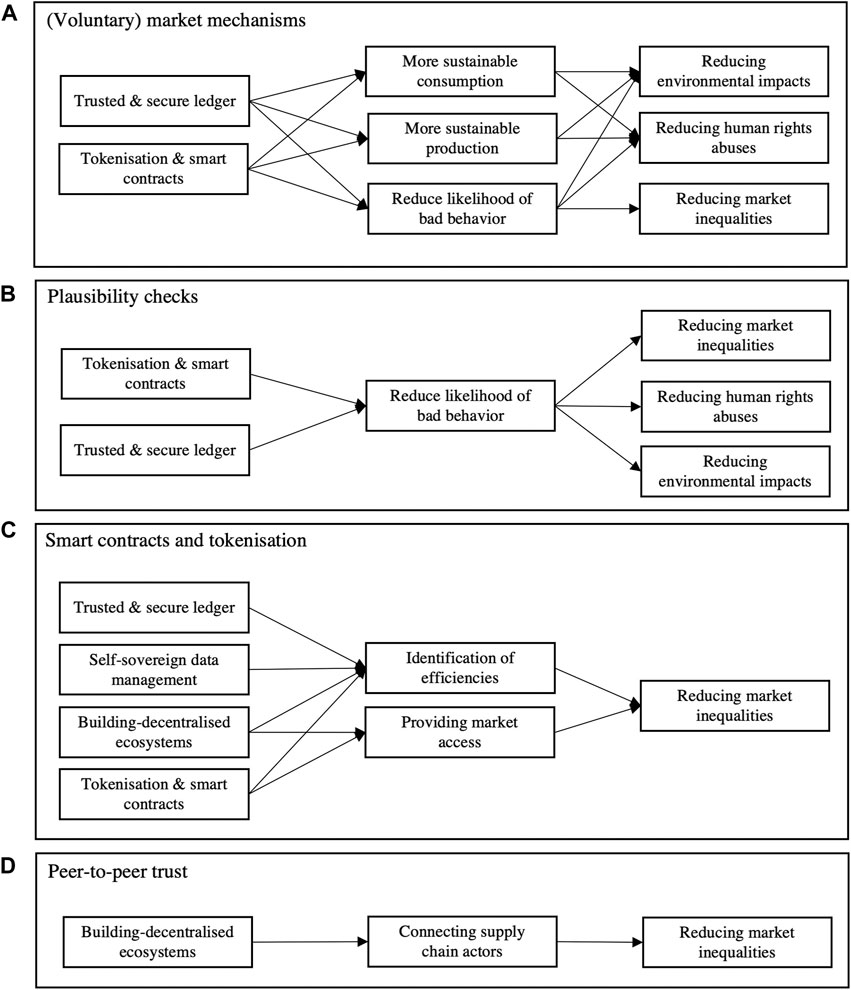
FIGURE 3. Impact Pathways and Mechanisms. Four mechanisms creating positive environmental and social impact have been identified. They are depicted in boxes (A–D). Within each box it is shown how the outputs (first column) influence the outcomes (middle column) and how the outcomes influence the resulting impacts (last column). The arrows link these boxes portraying impact pathways.
3.2.1 (Voluntary) Market Mechanisms
These mechanisms are based on obtaining trusted information about products that can be verified by third parties. This mechanism is facilitated by blockchain-enabled systems and can allow consumers to access specific product information such as product source and processing characteristics. Accessible traceability creates transparency and can be a market differentiator for sustainable product markets, increase consumer engagement, and build loyalty. Study participants believed that consumers could make better and more sustainable decisions based on this accessible and trusted product information.
Similarly, companies may gain additional access to supply chain and product information that was previously opaque to them; in this way the blockchain-enabled system is able to reduce information asymmetry. In these systems companies can make buying decisions beyond standard price and quality business dimensions. Companies can more easily expand decision and management criteria to include human rights abuses records or carbon footprints of products and supplier processes. Companies can further take actions to increase sustainability by knowing the environmental and social hotspots in their supply chain. For example, an action would be to directly support supply chain actor improvement or by paying premiums for reducing sustainability impact.
In general, study participants argued for a positive transparency spiral leading to “squeezing out bad actors” over time, where initial supply chain actors collect and share supply chain data to make some information transparently available resulting in pressures for other supply chain actors to follow suit. In this situation, as more information becomes visible bad behaviour such as fraud or human rights abuse will see the “light of day”. Actors displaying such bad behaviour are either removed from the supply chain or have severely limited market access. It is important however to highlight that this is still a conjecture by the respondents, as these results remain to be seen in practice. Policies supporting transparent and responsible supply chains will likely facilitate this development.
3.2.2 Plausibility Checks
Another mechanism leading to positive environmental and social impact relates to employing validation algorithms for complex data. These validations can ensure that production volumes remain accurate or that certificates are not double counted or over the dedicated amount. Blockchain-enabled systems ensure that the data provided by supply chain actors such as farmers or processors cannot be manipulated or reverse engineered, especially proprietary information. After an asset is registered on the blockchain only the specific item registered can be used within the supply chain. The amount cannot be multiplied or unreasonably changed at a later stage. This makes both fraudulent behaviour and honest mistakes less likely to occur.
Machine learning algorithms were mentioned by one study participant as a tool to identify anomalies that humans would potentially not catch and that could lead to identifying other bad behaviour. These machine learning algorithms can be ingrained in blockchain smart contract-like systems.
3.2.3 Smart Contracts and Tokenisation
Interviews supported the ability of blockchain-enabled systems to achieve positive impact through automation and the use of tokens. One respondent provided an example:
“The other aspect of blockchain that we looked into […] is the potential of smart contracts to play a role. Where transactional agreements can be executed without an exchange of paperwork and signatures. [Actors in the supply chain] were able to conduct in a matter of two and a half hours a transaction that normally would take 10 days to complete.”
Automating processes—such as payments upon execution of contracts—can speed the collection of data and create incentives for transparency by saving time and money. This kind of automation of payments further creates liquidity for supply chain actors as delays in payments are significantly reduced. This mechanism especially supports poorer actors such as farmers where a lack of funds can prevent them from participating in the market.
Tokenisation can be a valuable mechanism for connecting consumers with producers. Example schemes include tip-the-farmer and micro-lending activities. These mechanisms may also increase consumer engagement, in turn leading to more sustainable consumption.
3.2.4 Peer-to-Peer Trust
Building decentralised networks can support actors—especially in the Global South—that don’t have an official identity, access to bank accounts, or other official documentation (World Bank, 2019). Providing decentralised identities that securely keep a record of individual activities and are not part of existing formal systems, such as bank transactions, can facilitate individual participating in the business ecosystem.
Blockchain-enabled systems could capture transactions, for example those that are conducted after receiving money through micro-loans from consumers. Using a blockchain-enabled system allows individuals that are disadvantaged to circumvent some existing systems such as banks; this approach can reduce barriers for market entry for these vulnerable actors.
4 Discussion
Blockchain-enabled systems in supply chains can potentially result in a variety of positive social and environmental impacts. These can happen through (voluntary) market mechanisms, plausibility checks, smart contracts and tokenisation, and peer-to-peer trust.
Market-based certification schemes such as FairTrade or Forest Stewardship Council (FSC) use (voluntary) market mechanisms in similar ways. These instruments work on the assumption that consumers are willing to choose more sustainably-produced products, which encourages competitors to follow this practice (Swartz et al., 2017; Taylor, 2005). This virtuous cycle can eventually push less sustainable products out of the market (Grankvist et al., 2004).
An increase in market shares of sustainably certified products over the past few years (Bullock and van der Ven, 2020) indicates that these market mechanisms work as consumers shift to certified products increasing the money invested in sustainable production. However, literature shows mixed results regarding the impact of certification schemes on reducing social and environmental impacts (Santika et al., 2020).
4.1 The Role of Blockchain in Creating Positive Impacts
We asked if the impacts are due to the blockchain component in a system of technologies or due to other factors. The interviews provided only a partial answer to this question. We were able to determine that there are several reasons why study participants chose a blockchain-enabled system over a centralised one. Among these reasons we find negative past experiences, previous unsuccessful implementation of a centralised traceability solution for the company’s business, and previous centralised technology providers that exploited the company’s dependency on the providers’ services to put pressure on the company.
According to the study participants another concern with a centralised solution is the difficulty with getting competing actors to participate in such a system. As one of the participants stated, they would not join a centralised system where they are not the host and not in control. Maintaining ownership of their data is another reason why blockchain-enabled systems are preferred by some actors.
It was further stated that using blockchain-enabled systems may mean that some companies are willing to allocate a larger budget for traceability solutions. This budgetary increase may occur since in some cases the funding for a blockchain-enabled traceability system comes from the innovation budget and not from the sustainability or a back-office budget. Thus, using a blockchain-enabled system can be a driver for implementing traceability systems and digitising supply chains through innovation channels.
However, based on the overall information retrieved from the interviews it was not possible to clarify to what extent the outcomes and impacts identified in this study are due specifically to the blockchain component in the systems analysed. It is also conceivable that determining if and to what extent outcomes and impacts are due to the blockchain component is in fact infeasible. Many technologies are interlinked in a system and the outcomes and impacts are generated from the emergent behaviour of such a system making it difficult to parse contribution by a specific technology. This observation means the overall system design and integration, not just the blockchain component, is of tremendous importance for achieving positive outcomes and impacts.
4.2 Measuring Impact
Most study participants were not able to provide an answer to how they measure impacts of implementing a blockchain-enabled system, their justification being that it is too early to measure impacts.
What became clear is that different participants have different key interests—key performance indicators—in what needs to be measured. While some are interested in looking at increasing incomes of farmers, others are concerned with reducing human rights abuses and environmental impacts. Others were interested in consumer engagement and cost reductions. Thus, choosing relevant indicators for measuring impact is important as is the choice of an appropriate method to assess the relevant indicators. It may be advisable to consider indicator development early in blockchain-enabled system design so that pertinent information could be collected automatically.
We find it concerning that only a few study participants had thought concretely about measuring impacts as it makes it difficult to evaluate the success of their own projects and its potential continuation, further development, or dissolution. The lack of performance measurement might hinder the transfer of knowledge to other projects within a company or outside of it. This information is likely needed if positive impacts should be replicated and scaled.
4.3 Comparison With Previous Studies
In this study, we focus on how blockchain-enabled systems can bring positive social and environmental impacts, whereas other studies emphasise what kind of benefits blockchain technology in supply chains can have (Kamilaris et al., 2019; Kouhizadeh and Sarkis, 2018). While we propose a middle-range theory that should further be refined and tested, our findings are based on insights from cases that have seen actual implementation. This permits not only a detailed analysis, but also a realistic assessment of the current status-quo of blockchain-enabled systems and how they lead to positive impact.
This study confirms previous findings that blockchain technology can increase trust and transparency (Galvez et al., 2018; Köhler and Pizzol, 2020) and use them to facilitate positive social and environmental impact. Many of the outcomes described in this study are also mentioned in previous literature. For example, Kamilaris et al. (2019) mention both increased consumer awareness which relates to the theme of more sustainable consumption as well as reducing fraud which describes part of the theme reducing the likelihood of bad behaviour. Similarly, Kouhizadeh and Sarkis (2018) describe several components of the theme more sustainable production. This present study provides in contrast to previous literature a comprehensive overview of how positive social and environmental impact can be created with blockchain-enabled systems in supply chains including descriptions of four specific mechanisms based on insights from practice. The study further supports previous findings that the design of blockchain-enabled systems is important when implementing blockchain technology to achieve specific sustainability objectives (Kewell et al., 2017; Saurabh and Dey, 2021). Finally, the study confirms previous findings that the majority of blockchain technology is related to changing business processes and only a smaller part about implementing the technology (Drljevic et al., 2019).
4.4 Risks, Barriers, and Uncertainties of Blockchain-Enabled Systems in Supply Chains
While the study focuses on how blockchain-enabled systems can create positive social and environmental impacts, we also want to highlight some risks, barriers, and uncertainties related to their implementations in supply chains.
Blockchain-enabled systems in supply chains are typically built on either Ethereum or on non-proof-of-work blockchains such as Hyperledger Fabric. The proof-of-work consensus mechanisms is energy intensive by design (Köhler and Pizzol, 2019; Truby, 2018) and its use bears the risk of counteracting the positive sustainability impacts of employing blockchain-enabled systems. Ethereum is still using the proof-of-work consensus mechanism and consumes over 50 TWh per year with an estimated carbon footprint of over 25 MtCO2 per year (Digiconomist, 2021). However, Ethereum is in the process of transitioning to proof-of-stake, which will reduce its energy consumption by over 99.95% (Ethereum Foundation, 2021). Nevertheless, as long as blockchain-enabled systems are built on proof-of-work blockchains their negative environmental impacts should be considered when planning an implementation. In the long-term, intensive energy usage of blockchains within supply chains is not expected to be an issue, but research on the negative environmental impacts of blockchain technologies should be closely followed.
The different kinds of blockchain also have diverging advantages and drawbacks. For example, performance issues such as limited throughput (i.e., the number of transactions processed per second) are considered a barrier to blockchain adoption (Monrat et al., 2019). Some blockchains, particularly private ones such as Hyperledger Fabric, have a higher throughput than public-permissionless blockchains such as Ethereum. However, this comes at the cost of degree of decentralisation, which is considered to be a security risk: private blockchains have higher risks of collusion due to few dominant authorities (Kannengießer et al., 2020). Related to a higher throughput, private blockchains tend to have lower transaction costs which is a benefit for users.
While blockchains are often considered immutable, risks of attack do exist (Kannengießer et al., 2020). Most research focuses on attacks on public blockchains, particularly proof-of-work blockchains such as Ethereum (Kannengießer et al., 2020). One of the most recognised risks is the 51%-attack. This is the case when a malicious entities gain majority control of a blockchain (typically referred to proof-of-work blockchains). This can be used to reverse transactions and prevent other peers to confirm new blocks (Monrat et al., 2019). So it should be taken into consideration that while (public) blockchains are considered virtually immutable, it is possible to manipulate them. Additionally, the use of smart contracts, which are an integral part of most applications in supply chains, can pose a risk. For example, inappropriate handling may cause the smart contracts to be locked away preventing them from executing (Kannengießer et al., 2020).
Lack of interoperability has been identified as both a risk and an opportunity of blockchain technology (Drljevic et al., 2019). There are over 6,500 active blockchain projects using different platforms, vary in programming languages, consensus mechanisms used, protocol, and privacy features (Monrat et al., 2019). Standardisation between these different blockchain platforms is lacking. Particularly private blockchains risk being a silo-solution (Kannengießer et al., 2020) while public blockchains may be part of an ecosystem of applications. The present study shows that while Ethereum and Hyperledger Fabric solutions are leading blockchain platforms for supply chain applications, there is substantial heterogeneity in the type of blockchain platforms used by the study participants. One way technology developers are addressing this issue is by building blockchain-enabled systems that are “blockchain agnostic” as one study participant puts it. This means that the blockchain-enabled system can be moved to a different blockchain—if needed.
Another source of uncertainty in the implementation of blockchain-enabled systems in supply chains is regarding potential government regulations (Monrat et al., 2019). According to the respondents, the main legislative issue that is a source of uncertainty is the regulation of financial transactions across borders and multiple jurisdictions. The context was that regulation is lagging blockchain developments causing great uncertainty in the technology and its application. This is relevant for these projects that include financial services in their implementations and may pose risks in case regulations interfere. However, there is also the chance that regulation regarding due diligence (Sarkis et al., 2021), sustainability reporting, and carbon pricing is passed that could incentivise the adoption of traceability and transparency solutions such as blockchain-enabled systems.
This study also shows that different blockchain-enabled systems face varying challenges and uncertainties depending on their design. A blockchain-enabled system using Ethereum may benefit from experiences from other applications using Ethereum, while a blockchain-enabled system built on a lesser known blockchain platform may be the first of its kind and require more extensive testing. Additionally, the combination of different components in the system design may be unique and with limited previous experiences. This means that unexpected challenges can occur and would need to be overcome. Another challenge arises from making early design choices—such as choosing a private blockchain platform - that may need to be revised later based on experiences or technology developments. For example, one study participant explained how the initial implementation commenced on one blockchain platform, but due to price and functionality constraints the company decided to move to a different blockchain platform. Designing and implementing such blockchain-enabled system therefore may require continuous iterations, which can be time-consuming, particularly for early movers.
Summing up, this section highlights that while blockchain technologies may have advantages over existing solutions (e.g., virtual immutability), blockchains are different in terms of decentralisation and performance and security risk still exist for this technology. It is uncertain which security challenges will result in increased uptake of the technology. The section further highlights that system design matters and different trade-offs are relevant depending on system architecture choices.
4.5 Limitations and Validity of the Qualitative Methodological Approach
We discuss here potential sources of bias due to the choice of respondents and more broadly the use of an explorative qualitative research approach.
The findings of this study are based on the information provided by 16 interviews with experts on blockchain-enabled systems in supply chains including technology developers, actors in the supply chain, and consultants in the agricultural, fishing, and mining sectors covering a broad range of actors and sectors. While the study participants were predominantly technology providers, other perspectives from within the supply chain were also represented. However, the study would have likely benefitted from a more balanced representation of different perspectives. Nevertheless, considering the still nascent and thus small industry of blockchain-enabled systems in supply chain 16 interviews with experts holding key positions within their organisations was considered satisfactory.
Additionally, interviews were conducted until theoretical saturation was reached, meaning that new interviews were carried out until no substantially new information was generated. Thus, the validity of the results based on the interviews can be considered high. Different questions in the interviews, different methods used for coding and analysis of the interview data, and having different researchers conducting the analysis may have lead to slightly but not substantially different results—because both the selection of respondents, the interview procedure, and the coding procedure were performed iteratively thus leaving the opportunity to cross-check the validity and completeness of the identified themes across the interviews.
The interview protocol was designed to answer the research questions and discussed by all authors. The interviewer was also given the freedom to ask questions outside the interview guide when the study participant seemed to be especially knowledgeable about a topic or to elaborate on previous answers. An analysis of the first interviews was used to assess if the questions asked during the interviews were able to address the research questions. The results were deemed satisfactory.
Given the significant championing and hype of new innovations we had to be careful that the results of this study might be biased towards unrealistic benefits of blockchain technology. The study participants are in fact implementing the technology and may have a more optimistic perspective of the capabilities and outcomes. However, not all study participants provided and used only blockchain technologies. For example, two technology providers stated that they are primarily supporting their clients with the process of digitisation, which is generally a requirement for introducing blockchain technology (Köhler and Pizzol, 2020). These companies then offer blockchain technology as a supplemental product. The interviews further showed that suppliers do not use blockchain for the sake of blockchain, but because blockchain is the right tool to solve specific problems or to address specific needs. One study participant explained that “We don’t do blockchain because it says blockchain […]. For us it doesn’t matter. […] We only have technical requirements. With this project we coincidentally ended up using blockchain.” While the results should be considered with the knowledge that the study participants decided to implement blockchain-enabled systems, critical viewpoints are also represented within this study. However, we do recognise that there is a possible limitation regarding the lack of interviews with supply chain experts that did not implement blockchain technology in supply chains as they might have more critical perspectives on how blockchain technology does or does not create impact within supply chains.
The research was further limited by the early stages of development. While information saturation was reached (Tie et al., 2019), some impacts could just be forecasted at this point. These limitations due to early stages of technology development and implementation as well as the limitations regarding qualitative research highlights the importance of taking a middle-range theory approach, which is considered suitable to provide a first assessment of how blockchain-enabled systems in supply chains can create positive impact. Clearly, more research is needed to further develop this theory.
4.6 Scientific Contribution
The specific contribution to the scientific body of this research is manyfold. While previous research mainly focussed on what impact blockchain-based technologies in supply chains can have, we developed a middle-range theory on how such implementations can create positive impact and detail four specific impact pathways. For example, plausibility checks can be used to catch honest mistakes as well as fraud and thus disincentivise said fraudulent behaviour. The study further advances the discussion on the role of the blockchain component in supply chain implementations and emphasises the issue of measuring impact early on. We find it concerning that only few study participants measure impact of their projects and recommend using project-specific indicators early on in order to automatically collect pertinent information that help to evaluate the success of the project.
4.7 Future Research Proposals
Additional research should further address the role of blockchain technology in a blockchain-enabled system and evaluate to what degree generated impacts are due to the blockchain component. In this study, we were not able with the data available to clarify to what extent the blockchain component contributes to positive social and environmental impact generated by the blockchain-enabled system as a whole. However, we believe it is important to determine the role of specific components in a system of technologies in creating impact (Köhler and Pizzol, 2020), and in particular of the blockchain component that is often used to brand, promote, and justify such system—in order to understand how to achieve the full potential of this component and to maximise its impact, or to understand what factors prevent the component to achieve its full potential. It may be impossible to isolate the effect of a single component as only the “emergent behaviour” of the system of technologies can be deemed responsible for generating impact and this systemic property does not allow disentangling the contributions of the individual components. In this case, we suggest a different approach for future research on blockchain-enabled systems, which should embrace the complexity and put more emphasis on overall system design and integration by investigating what is the importance and role of different design choices in generating positive social and environmental impact.
An important insight that we gained from this study is that there is currently a gap in measuring the impact of blockchain-enabled systems in supply chains. This gap is concerning because not measuring impact makes it difficult to evaluate the success of the implementation of blockchain. Measuring impact is not only a tool to assess the accomplishments of an implementation, but also a tool that can be used to make strategic decisions about project continuation or adaptation and can allow knowledge transfer to other projects ultimately achieving impact at larger scale. Future research could address this gap by, for example, developing a methodology to measure the impact of implementing blockchain-enabled systems and to test such methodology on real-world cases. These new measurement approaches need to be adaptable to specific use cases and allow to determine the extent to which perceived and projected impacts match actual measurable results. Since previous research on blockchain-enabled systems has mostly been conceptual, taking an experimental approach where the proposed methodology is tested in an actual project will allow to base findings on practical experiences and take into account real-world constraints that are not foreseen in conceptual studies.
Finally, future research should test and adjust the middle-range theory proposed as needed. Limitations regarding the early stages of technology development and implementation as well as the use of an explorative qualitative research approach were identified. In order to address these limitations, future research could conduct long-term—longitudinal—studies of specific cases. A long-term approach is needed in order to see impacts of implementations of blockchain-enabled systems and analyse if they are aligned with the ones forecasted by the current study participants. More in-depth case studies can be used to test the middle-range theory, adjust it, and provide additional detail, given that multiple functions and organisations will be impacted. Focusing on specific case studies can further help to provide a more balanced view of different perspectives within the supply chain addressing potential biases towards technology providers in this study.
Data Availability Statement
The original contributions presented in the study are included in the article/Supplementary Material, further inquiries can be directed to the corresponding author.
Author Contributions
SK: Conceptualization, conduction of interviews, interview coding, data analysis, writing – original draft preparation; MP: Conceptualization, feedback on analysis, writing – review and editing; JS: Conceptualization, feedback on analysis, writing – review and editing.
Funding
SK’s and MP’s contribution has been funded by the grant 7015-00006 of the Independent Research Fund Denmark – Social Sciences.
Conflict of Interest
The authors declare that the research was conducted in the absence of any commercial or financial relationships that could be construed as a potential conflict of interest.
Publisher’s Note
All claims expressed in this article are solely those of the authors and do not necessarily represent those of their affiliated organizations, or those of the publisher, the editors and the reviewers. Any product that may be evaluated in this article, or claim that may be made by its manufacturer, is not guaranteed or endorsed by the publisher.
Supplementary Material
The Supplementary Material for this article can be found online at: https://www.frontiersin.org/articles/10.3389/fbloc.2021.720347/full#supplementary-material
References
Azzi, R., Chamoun, R. K., and Sokhn, M. (2019). The Power of a Blockchain-Based Supply Chain. Comput. Ind. Eng. 135, 582–592. doi:10.1016/j.cie.2019.06.042
Bullock, G., and van der Ven, H. (2020). The Shadow of the Consumer: Analyzing the Importance of Consumers to the Uptake and Sophistication of Ratings, Certifications, and Eco-Labels. Organ. Environ. 33, 75–95. doi:10.1177/1086026618803748
Choudhary, K., and Sangwan, K. S. (2018). Benchmarking Indian Ceramic Enterprises Based on green Supply Chain Management Pressures, Practices and Performance. Bij 25, 3628–3653. doi:10.1108/BIJ-12-2017-0330
Clarke, T., and Boersma, M. (2017). The Governance of Global Value Chains: Unresolved Human Rights, Environmental and Ethical Dilemmas in the Apple Supply Chain. J. Bus. Ethics 143, 111–131. doi:10.1007/s10551-015-2781-3
Craighead, C. W., Ketchen, D. J., and Cheng, L. (2016). "Goldilocks" Theorizing in Supply Chain Research: Balancing Scientific and Practical Utility via Middle-Range Theory. Transportation J. 55, 241–257. doi:10.5325/transportationj.55.3.0241
Chun Tie, Y., Birks, M., and Francis, K. (2019). Grounded Theory Research: A Design Framework for Novice Researchers. SAGE Open Med. 7, 205031211882292–205031211882298. doi:10.1177/2050312118822927
Digiconomist (2021). Ethereum Energy Consumption Index. [WWW Document]. URL https://digiconomist.net/ethereum-energy-consumption.
Drljevic, N., Aranda, D. A., and Stantchev, V., 2020. Perspectives on Risks and Standards that Affect the Requirements Engineering of Blockchain Technology. Computer Stand. Inter. 69, 103409. doi:10.1016/j.csi.2019.103409
Dubey, R., Gunasekaran, A., Childe, S. J., Papadopoulos, T., Luo, Z., Wamba, S. F., et al. (2019). Can Big Data and Predictive Analytics Improve Social and Environmental Sustainability? Technol. Forecast. Soc. Change 144, 534–545. doi:10.1016/j.techfore.2017.06.020
Ethereum Foundation (2021). A Country’s worth of Power. no more! [WWW Document]. URL https://blog.ethereum.org/2021/05/18/country-power-no-more/ (accessed 7 29, 21).
Feng, H., Wang, X., Duan, Y., Zhang, J., and Zhang, X. (2020). Applying Blockchain Technology to Improve Agri-Food Traceability: A Review of Development Methods, Benefits and Challenges. J. Clean. Prod. 260, 121031. doi:10.1016/j.jclepro.2020.121031
França, A. S. L., Amato Neto, J., Gonçalves, R. F., and Almeida, C. M. V. B. (2020). Proposing the Use of Blockchain to Improve the Solid Waste Management in Small Municipalities. J. Clean. Prod. 244, 118529. doi:10.1016/j.jclepro.2019.118529
Galvez, J. F., Mejuto, J. C., and Simal-Gandara, J. (2018). Future Challenges on the Use of Blockchain for Food Traceability Analysis. Trac Trends Anal. Chem. 107, 222–232. doi:10.1016/j.trac.2018.08.011
Gioia, D. A., Corley, K. G., and Hamilton, A. L. (2012). Seeking Qualitative Rigor in Inductive Research. Organizational Res. Methods 16, 15–31. doi:10.1177/1094428112452151
Grankvist, G., Dahlstrand, U., and Biel, A. (2004). The Impact of Environmental Labelling on Consumer Preference: Negative vs. Positive Labels. J. Consumer Pol. 27, 213–230. doi:10.1023/b:copo.0000028167.54739.94
Jørgensen, A., Lai, L. C. H., and Hauschild, M. Z. (2010). Assessing the Validity of Impact Pathways for Child Labour and Well-Being in Social Life Cycle Assessment. Int. J. Life Cycle Assess. 15, 5–16. doi:10.1007/s11367-009-0131-3
Kallio, H., Pietilä, A.-M., Johnson, M., and Kangasniemi, M. (2016). Systematic Methodological Review: Developing a Framework for a Qualitative Semi-structured Interview Guide. J. Adv. Nurs. 72, 2954–2965. doi:10.1111/jan.13031
Kamath, R. (2018). Food Traceability on Blockchain: Walmart's Pork and Mango Pilots with IBM. The JBBA 1, 1–12. doi:10.31585/jbba-1-1-(10)2018
Kamilaris, A., Fonts, A., and Prenafeta-Boldύ, F. X. (2019). The Rise of Blockchain Technology in Agriculture and Food Supply Chains. Trends Food Sci. Technology 91, 640–652. doi:10.1016/j.tifs.2019.07.034
Kannengießer, N., Lins, S., Dehling, T., and Sunyaev, A. (2020). Trade-offs between Distributed Ledger Technology Characteristics. ACM Comput. Surv. 53, 1–37. doi:10.1145/3379463
Kewell, B., Adams, R., and Parry, G. (2017). Blockchain for Good? Strateg. Change 26, 429–437. doi:10.1002/jsc.2143
Köhler, S., and Pizzol, M. (2019). Life Cycle Assessment of Bitcoin Mining. Environ. Sci. Technol. 53, 13598–13606. doi:10.1021/acs.est.9b05687
Köhler, S., and Pizzol, M. (2020). Technology Assessment of Blockchain-Based Technologies in the Food Supply Chain. J. Clean. Prod. 269, 122193. doi:10.1016/j.jclepro.2020.122193
Koontz, T. M., Jager, N. W., and Newig, J. (2020). Assessing Collaborative Conservation: A Case Survey of Output, Outcome, and Impact Measures Used in the Empirical Literature. Soc. Nat. Resour. 33, 442–461. doi:10.1080/08941920.2019.1583397
Kopyto, M., Lechler, S., von der Gracht, H. A., and Hartmann, E. (2020). Potentials of Blockchain Technology in Supply Chain Management: Long-Term Judgments of an International Expert Panel. Technol. Forecast. Soc. Change 161, 120330. doi:10.1016/j.techfore.2020.120330
Kouhizadeh, M., and Sarkis, J. (2018). Blockchain Practices, Potentials, and Perspectives in Greening Supply Chains. Sustainability 10, 3652. doi:10.3390/su10103652
Kouhizadeh, M., Zhu, Q., and Sarkis, J. (2020). Blockchain and the Circular Economy: Potential Tensions and Critical Reflections from Practice. Prod. Plann. Control. 31, 950–966. doi:10.1080/09537287.2019.1695925
Lim, M. K., Li, Y., Wang, C., and Tseng, M.-L. (2021). A Literature Review of Blockchain Technology Applications in Supply Chains: A Comprehensive Analysis of Themes, Methodologies and Industries. Comput. Ind. Eng. 154, 107133. doi:10.1016/j.cie.2021.107133
Monrat, A. A., Schelén, O., and Andersson, K. (2019). A Survey of Blockchain from the Perspectives of Applications, Challenges, and Opportunities. IEEE Access 7, 117134–117151. doi:10.1109/ACCESS.2019.2936094
Naderifar, M., Goli, H., and Ghaljaie, F. (2017). Snowball Sampling: A Purposeful Method of Sampling in Qualitative Research. Strides Dev. Med. Educ. 14 (3). doi:10.5812/sdme.67670
Olney, D. K., Talukder, A., Iannotti, L. L., Ruel, M. T., and Quinn, V. (2009). Assessing Impact and Impact Pathways of a homestead Food Production Program on Household and Child Nutrition in Cambodia. Food Nutr. Bull. 30, 355–369. doi:10.1177/156482650903000407
O’Reilly, K., Paper, D., and Marx, S. (2012). Demystifying Grounded Theory for Business Research. Organizational Res. Methods 15, 247–262. doi:10.1177/1094428111434559
Rejeb, A., Keogh, J. G., Zailani, S., Treiblmaier, H., and Rejeb, K. (2020). Blockchain Technology in the Food Industry: A Review of Potentials, Challenges and Future Research Directions. Logistics 4, 27. doi:10.3390/logistics4040027
Santika, T., Wilson, K. A., Law, E. A., St John, F. A. V., Carlson, K. M., Gibbs, H., et al. (2020). Impact of palm Oil Sustainability Certification on Village Well-Being and Poverty in Indonesia. Nat. Sustain. 4, 109–119. doi:10.1038/s41893-020-00630-1
Sarkis, J., Dewick, P., Hofstetter, J. S., and Schröder, P. (2021). Changing of the Guard: A Paradigm Shift for More Sustainable Supply Chains. Resour. Conserv. Recycl. 170, 105587. doi:10.1016/j.resconrec.2021.105587
Saurabh, S., and Dey, K. (2021). Blockchain Technology Adoption, Architecture, and Sustainable Agri-Food Supply Chains. J. Clean. Prod. 284, 124731. doi:10.1016/j.jclepro.2020.124731
Swartz, W., Schiller, L., Rashid Sumaila, U., and Ota, Y. (2017). Searching for Market-Based Sustainability Pathways: Challenges and Opportunities for Seafood Certification Programs in Japan. Mar. Pol. 76, 185–191. doi:10.1016/j.marpol.2016.11.009
Taylor, P. L. (2005). In the Market but Not of it: Fair Trade Coffee and Forest Stewardship Council Certification as Market-Based Social Change. World Development 33, 129–147. doi:10.1016/j.worlddev.2004.07.007
Truby, J. (2018). Decarbonizing Bitcoin: Law and Policy Choices for Reducing the Energy Consumption of Blockchain Technologies and Digital Currencies. Energ. Res. Soc. Sci. 44, 399–410. doi:10.1016/j.erss.2018.06.009
Wang, Y., Singgih, M., Wang, J., and Rit, M. (2019). Making Sense of Blockchain Technology: How Will it Transform Supply Chains? Int. J. Prod. Econ. 211, 221–236. doi:10.1016/j.ijpe.2019.02.002
Keywords: traceability, impact, responsible production, responsible consumption, digitization
Citation: Köhler S, Pizzol M and Sarkis J (2021) Unfinished Paths—From Blockchain to Sustainability in Supply Chains. Front. Blockchain 4:720347. doi: 10.3389/fbloc.2021.720347
Received: 04 June 2021; Accepted: 27 October 2021;
Published: 16 November 2021.
Edited by:
Leanne Ussher, Copenhagen Business School, DenmarkReviewed by:
Raul Zambrano, Independent Researcher, New York, NY, United StatesMeghana Kshirsagar, University of Limerick, Ireland
Melanie Birks, James Cook University, Australia
Copyright © 2021 Köhler, Pizzol and Sarkis. This is an open-access article distributed under the terms of the Creative Commons Attribution License (CC BY). The use, distribution or reproduction in other forums is permitted, provided the original author(s) and the copyright owner(s) are credited and that the original publication in this journal is cited, in accordance with accepted academic practice. No use, distribution or reproduction is permitted which does not comply with these terms.
*Correspondence: Massimo Pizzol, bWFzc2ltb0BwbGFuLmFhdS5kaw==