- 1Department of Civil Engineering, Alex Ekwueme Federal University Ndufu-Alike Ikwo, Abakaliki, Nigeria
- 2Department of Civil Engineering, Niger Delta University, Amassoma, Nigeria
- 3Department of Civil Engineering, University of Nigeria, Nsukka, Nigeria
Nanotechnology has been widely discussed in extant literature as a technological solution to several problems associated with the use of construction materials for sustainable infrastructure delivery. This paper discusses findings from research that investigated applications of Nanotechnology on active soils. It highlights research findings on various areas Nanotechnology can provide innovative solutions for sustainable infrastructure delivery. The paper uses results from analysis of laboratory tests to discuss improvements in soil engineering properties that result from re-engineering construction materials and processes. The research results demonstrate improvements in soil engineering properties that could result from re-engineering construction materials and/or processes. The engineering properties of active soils investigated include: Atteberg Limits (liquid limit-LL, plastic limit-PL etc.), Shrinkage, Dry density Bearing capacity, California Bearing Ratio (CBR), and hydrophobic behavior. Other engineering properties of the active soils investigated include: shrink-swell, water absorption, and activity ratings /classification. Improvements were observed on the engineering properties of soils treated with the nanomaterial. The LL, PL, shrinkage, activity, and CBR showed the following levels of improvement: −24.21, 54.02, −50.24, −38, −12,59, and 14% respectively, while the shrink-swell, and water absorption improved by over 60 and 70% respectively. The soils also showed improved hydrophobicity when treated with nanomaterial. The activity ratings transitioned between low, high, and very high depending on the mineralogical and chemical compositions of the soils in their natural states. Statistical tests confirmed that the improvements were attributable to the nanomaterial agent in the active soil samples. However, the statistical tests show that addition of nanomaterial did not have effect on unconfined compressive strength and maximum dry density of the active soil. The paper concludes that given some potential risks in Nanotechnology applications in construction, there is an overarching need to formulate National policies and roadmaps for broader Nanotechnology applications in construction industries at country-specific levels.
Introduction
Active or expansive soils are a worldwide problem that have posed and continue to pose several challenges to civil engineers in infrastructure delivery. For example in Nigeria, the flood zone of the Niger Delta region is underlain by active soils that are distributed laterally and vertically. These soils are prone to shrink characteristics coupled with the alternating dry and wet seasons having annual rainfall in excess of 2,000 mm. Such soils are considered potential natural hazard. The risks they pose to infrastructures, include extensive damage to structures if not adequately treated (Al–Rawas et al., 2002), and Nigeria has experienced its own share of such risk. As an illustration, their adverse effect in the fresh water zone of the Niger Delta in Nigeria, was exacerbated and demonstrated by the soil-moisture variations that resulted from the 2012 flood in Nigeria. Several roads were cut and broken resulting in total collapse of transportation infrastructure. Electrical grids and, telecom-communications fell and many buildings were washed away with infrastructure loss amounting to billions of Nigeria Naira (Bariwen et al., 2012). In Nigeria's Niger Delta, these active soils exist to irreplaceable extent (both laterally and vertically) and are highly undesirable in their natural forms for civil engineering works. This is because they swell extensively in the rainy season and shrink proportionately in the dry months together with any structure including road pavements constructed with them (Omotosho and Ogboin, 2009). Given their natural abundance in several parts of the world including Nigeria's Niger Delta region, it is necessary to investigate innovative solutions that could improve the active soils and also characterize their properties when treated with such additive agents that impart on their engineering properties. Seed et al. (1962) developed a classification system for active soils based on type, proportion, size and activity as defined by Skempton (1953). It has been shown that activity represents the plasticity index for the clay fraction alone (Barnes, 2000). On the other hand. Chen (1988) classified active soils based on the relative proportion of expansive and non-expansive.
Classification system for expansive soils is based on the problem they create in the construction of foundations (potential swell). Several investigators have developed correlation to predict swelling characteristics based on pavement conditions. They are dry density, initial moisture content and Atterberg limits (Mowafy and Bauer, 1986; Erzin and Erol, 2004). The most satisfactory and convenient method of determining the swelling properties of an expansive clay is by direct measurement. Direct measurement of expansive soil can be achieved by the use of the conventional one dimensional consolidometers (Chen, 1988).
It is imperative to improve the engineering properties by stabilization using stabilizing agent(s) that re-engineer the active soil for better engineering application. Nanomaterials which are by-products of Nanotechnology could provide innovative solution to this congenital problem. Such innovative solution would improve the stability, durability and the soil water proofing requirements essential for active soils environment. This will result in sustainable infrastructure delivery (Ugwu, 2013; Ugwu et al., 2013, 2014).
This paper reports on research that investigated the impact(s) of innovative state-of-the-art re-engineering solutions using Nanomaterial as a stabilizing agent, on improving the hydrophobic (water repelling) characteristics and other geotechnical properties of active soils such as shrink-swell potential. The research reported in this paper contributes to a more resourceful exploitation of the abundant active soils for sustainable construction and civil engineering practice. This will enhance better understanding of active soils and their engineering application. The rest of the paper is organized as follows.
The paper reviews related work on the application Nanotechnology in civil engineering and construction sector. It then describes the experimental design and laboratory works before discussing the results. The paper then discusses the statistical tests (ANOVA) draws conclusions and gives recommendations for further work.
Review of Related Research
Extensive literature is available on soil improvement by the application of additives, notably cement and lime. Lately, many researchers have reported on additives that could substitute lime as a soil modifier. Such material include fly ash (Ali and Korrane, 2011), rice husk (Muntohar, 1999; Muntohar and Hantoro, 2000), marble dust (Okagbue and Onyeobi, 1999), limestone ash (Okagbue and Yakubu, 2000 cited in Okagbue, 2007) granite dust and Nanomaterial (Taha, 2009; Ugwu, 2013; Ugwu et al., 2013, 2014). Nanomaterial is an organosilane compound with nonfunctional organic R(alkyl) group and trial koxy groups (Taha, 2009). The stabilization characteristics of Nanomaterials and the reaction chemistry are based Feynman's phenomenal work on: “manipulating and controlling things on a small scale” (Feynman, 1960). Some of the application and/or potential application areas discussed in extant literature include; design and construction, processes (Sobolev and Gutiérrez, 2005a,b; Rana et al., 2009), transportation engineering (Steyn, 2008), general construction applications (Bartos, 2006), concrete technology, (Sobolev and Gutiérrez, 2005a,b; Zydex Industries, 2010; Agrawal and Agrawal, 2011), general civil engineering applications (Boyd, 2010; Lee et al., 2010), geotechnical engineering (Taha, 2009; Ugwu et al., 2013), civil engineering materials (Kennep, 2006), and highways infrastructure: pavements (Liu et al., 2007; Ugwu et al., 2013). Ugwu et al. (2013) discuss the reaction chemistry of Nanomaterial with the aim of facilitating a better understanding of the fundamental science that underpins the observed changes in soil geotechnical properties (i.e., hydrophobicity, plasticity, and swell potential). Choi et al. (2016) investigated the potential of chemically treated water-repellent kaolin clay as a landfill cover material is explored by examining its characteristics including hydraulic and mechanical properties. Athulya et al. (2017) Investigated effective utilization of steel slag, a by-product from the steel industry, as construction material in highway pavement layers so that the industrial wastes can be used and disposed properly with reduction in environmental impact as well as the demand for natural construction materials can be minimized. Prasanna and Jnanendra (2017) investigated the adequacy of fly ash as an additive in improving the geotechnical properties of medium expansive silty soil in conjunction with nano material. Abu et al. (2018) evaluated the strength characteristics of tropical soil treated with nano calcium silicate (NCS) and in combination with two different materials such as lime and fly ash added. Pan et al. (2018) syudied the long-term mechanical behavior of nano silica sol grouting. The nano silica sol was activated with different proportions of a NaCl catalyst and cured under fluctuating temperature and humidity conditions.
The preceding section shows that although several articles in extant literature have articulated potential applications of nanotechnology in civil engineering and construction sectors, there is dearth of literature discussing empirical work to investigate the applications on active soils. In few cases where work has been reported, gaps exist in the characterization of active soils studied. This research contributes to fill the existing gap by conducting detailed investigation and characterization of active soil properties and stabilization using nanomaterial. It includes empirical laboratory work, and the paper highlights observed limitations of the technology in the domain application area (i.e., active soils).
Study Area and Experimental Design
The study area is located in Nigeria's Niger Delta. In the Niger Delta of Nigeria active soil cover the often seasonally flooded fresh water area called the Niger Flood Zone as shown in the map in Figure 1.
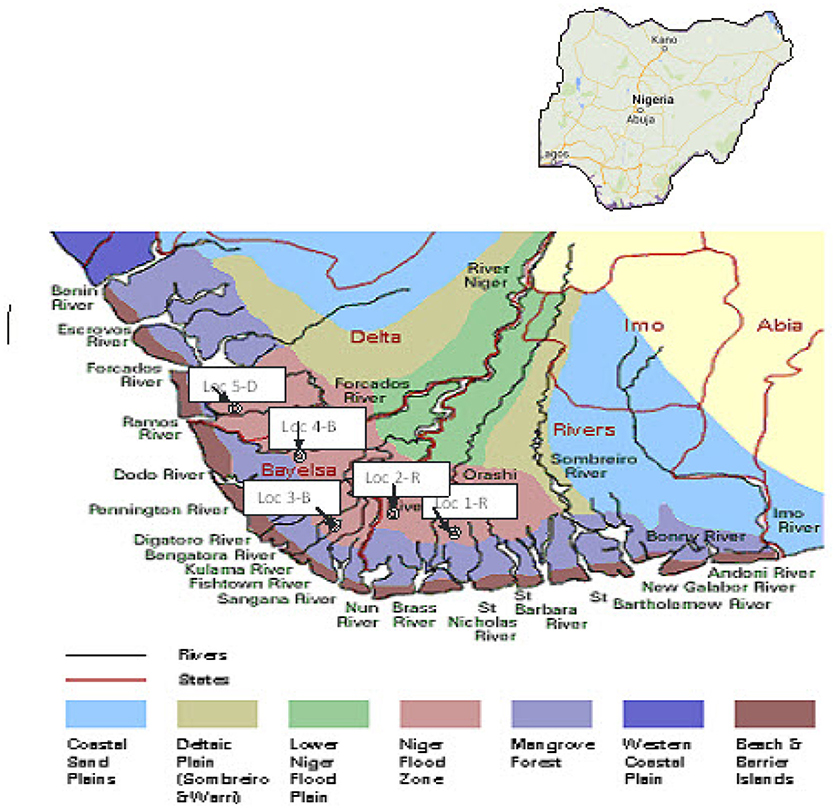
Figure 1. Active soil zone and layout of sampling i. The zone as delineated by Akpokodje and Arumala (1987) is characterized superficially by n study area.
These areas often has high annual rainfall in excess of 2,000 mm. However, the terrain is near flat resulting in impeded drainage and consequently poor laterization very active (montmonillinitic) silty clay. Their characteristics when in contact with water result in swelling and shrinkage when dry, thereby causing severe surface deformations, pavement cracking and failures to infrastructures founded with them or on them. These pose serious challenges to sustainable engineering and construction in infrastructure delivery in the area. Several attempts have been made to control the swell-shrink behavior of these soils. Examples include stabilizing with cement lime, calcium chloride and other admixture. This is often integrated with good drainage system to ensure good performance base course, paved shoulders, sub grades and sub bases.
These have shown to be highly undesirable in their natural forms for many construction purposes. Solutions such as partial/wholesale replacement of active soils from better lateritic spoils in borrow pits has several sustainability footprints. These include expensive haulage of better base material from long distances in addition to serious environmental and ecological footprints that result from huge hips and borrow pits that occupy vast areas. The research reported in this paper investigated the impact(s) of innovative state-of–the–art re-engineering solution such as application of Nanomaterial, on improving the engineering properties (consistency limits and free swell) of active soil.
Methodology: Material Extraction Methods and Empirical Works
The active soils used in this study were obtained from five locations distributed across the Niger Flood zone of the Niger Delta in Southern Nigeria. They include two locations in Rivers State two locations in Bayelsa State and a location in Delta state as shown in Table 1 (see also Figure 1).
Test Procedures
24-H Free Swell Test for Classification
In order to study the magnitude of possible swell in the clay, the test suggested by Sivapullaiah et al. (1996) was followed (Bailey, 1980; Sivapullaiah, 1996; ASTM Standard Test Methods for One–Dimensional Swell or Settlement Potential of Cohesive Soils, 1999; Das, 1999; Venkatramaiah, 2006; Maghsoudi and Dahooei, 2009; Okagbue, 2014; Choi et al., 2016; Syed et al., 2018). This appears to give a better indication for the swelling potential of clayey soil with a mass of about 10 g. The soil mass was well pulverized and transferred into a 100 ml graduated jar containing distilled water. After 24 h, the swollen sediment is measured. The modified free swell index is calculated;
Where,
Classification of soils are based on the modified free swell index, the swelling potential of soil may be qualitatively classified as listed in Table 2. Table 3 shows the various dilution ratios.
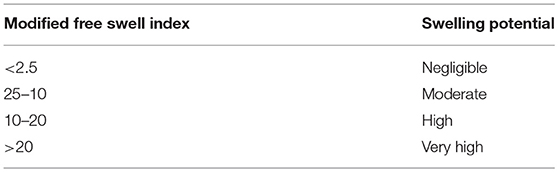
Table 2. Classification of soils based on modified free swell index (after Sivapullaiah et al., 1996).
Unrestrained Swell Test
This is a simple laboratory oedometer test. The specimen was placed in an oedometer under a small surcharge of about 6.9 kN/m2. Water was then added to the specimen, and the expansion of the volume of the specimen (i.e., height; the area of cross section was constant) was measured until equilibrium was reached. The percent of free swell can be expressed as a ratio.
Where Sw (free) = Free swell, as a percent
ΔH = Change in initial height (H) of the specimen
H = Initial height of the specimen
Using the correlation chart of O'Neill and Poormoayed (1980) the relationship for calculating the free surface swell has been established as a standard.
Compaction and CBR Test
Standard proctor compaction tests were carried out in accordance to BS 1377(5). About 3,000 g of air dried sample thoroughly mixed, was divided into three parts. The proctor mold was filled in three layers and compacted by 25 blows of a rammer 2.5 kg falling over a height of 300 mm. The collar of the mold was then removed and the compacted sample weighed while specimen was taken around the middle to determine the corresponding moisture contents until the weight of compacted sample started falling.
The optimum moisture content (OMC) obtained from the Standard Proctor Test above was then used to compact California Bearing Ratio (CBR) samples for all the soils. Test was conducted after air dry for 4 days and moisture curing for 6 days. The procedure involved allowing a standard plunger penetrates the compacted sample at controlled rate, while the load required for various levels of penetration was recorded.
Water Absorption Capacity
Using the Oedometer test equipment, absorption experiments were performed. The samples were placed in the oedometer cell in accordance with BS 13 and were allowed to absorb water through capillarity. During this period a pressure to prevent swelling was applied so as to keep the vertical deformation indicator at zero.
In order to determine the water absorption capacity, samples were allowed to absorb water through capillarity in the oedometer test cell. In the process, the weight was measured with an electronic balance having a sensitivity of 1/100. After 3 or 4 days, a constant weight was obtained. The ultimate water content of the soil which was left to absorb water through capillarity in unrestricted conditions is the water absorption capacity.
Unconfined Compressive Strengths (UCS)
Cylindrical specimens were prepared for the determination of the UCS. The mold used was of the split type consisting of two semi-circular sections of pipe circumference of diameter 100 mm and area of 78.53 mm2. Specimens were tested for their dry unconfined compressive strength in accordance with BS 1924(5).
Durability Test
The test method covers procedure for determining the soil losses, moisture changes, volume changes (swell and shrinkage). This wetting and drying test was performed according to ASTM D 599(2), 1999. Specimens were prepared and after 7-day curing period, they were submerged in tap water at room temperature for a period of 5 h, after which they removed and placed in an oven at 60°C for 42 h. The entire surface area was wire brushed to remove all material loosened during wetting and drying, with two firm strokes used on each portion of the surface. The application of these strokes were of full height and the process consisted of 5 h of water immersion, 42 h of drying, and 1 h of handling. A complete operation was conducted for a total of 12 cycles. After 12 cycles, the test specimens are dried to a constant temperature of 110°C, and weighed to determine the oven-dry weights.
Laboratory Analysis
The laboratory study was conducted in seven phases. These included:
(i) Analyses of the active soils both in untreated and treated states. These were performed by two recognized national laboratories.
(ii) Hydrometer tests, Atterberg Limit tests, and specific gravity tests were applied to the samples,
(iii) Absorption and unrestrained swell test,
(iv) 24 h free swell test,
(v) Compaction and CBR Test,
(vi) Unconfined Compressive Strength Test, and
(vii) Durability Test.
Tests were conducted on both untreated and treated samples, and after curing for 4 days. The ratio of nanomaterial to water mixture in the study were: 1:50, 1:100, 1:150, 1:200, and 1; 250. The nanomaterial: water ratio is a measure of the concentration of the nanomaterial, the lower the water ratio, the higher the concentration and vice-versa.
Analysis of Results and Discussion
Active Soil (Untreated)
The soils collected from the five locations were all clayey with clay content ranging from 25 to 32%. Table 4 summarizes the investigation and soil classification of the soils in their natural state. Figure 2 which was extracted from laboratory data analyses, shows the proportion of fines.
Key to symbols- NMC, Natural Moisture Content; C, Clay Content.
It was observed that the percentage passing through or finer than sieve 75 mm opening increased toward Rivers State Loc 1–R from Delta State. Loc 5-D. It was further observed to decrease progressively from 95 to 74% respectively. There was a steady decrease noticed in plasticity moving from Rivers, toward Delta State (i.e., Loc1 –R to Loc 5- D). However, all the location samples are grouped as CH, which signify inorganic clay of high plasticity. Also all the samples are rated active since results of activity are above 1.25, signifying the sample was calcium mortimorillonite and organic alluvial clay (Table 4).
Figure 2 and Table 4 shows that clay content decreased from the Loc 1- R down to Loc 5-D.
Chemical Analysis of Active Soil, Untreated and Treated
Chemical test was conducted on the active soil in both treated and untreated states, to enable analysis of the composition and the effect the stabilizer contributes on the chemical elements of the mineral matter in the soils.
The test results as presented in Tables 4, 5 show elements that result from chemical reactions such as cation exchange, flocculation agglomerations and pozzolanic reactions. These reactions occur when soil is improved by means of chemical stabilization. The results also show concentrations and potentials of calcium or magnesium, which may be high enough to preserve active soil minerals. In these environments, such minerals may be a transitory weathering product (Borchardt and Smectites, 1989). Basic cation calcium Ca2+ is normally the predominant exchangeable cation in soils, even in acid, weathered soils. In highly weathered soils, such as oxisoils, aluminum (Al3+) may become the dominant exchangeable cation. These minerals commonly occur in fresh water sediments and this explains their presence in the freshwater zone of the Niger Delta.
Table 6 shows general decreasing trend in the values of the parameters measured as the samples transitioned from untreated to treated state. These decreases in values could be due to changes that result from the reaction of nanomaterial with the active soils. However, a detailed discussion of such possible changes is outside the scope of this paper.
Effect of Stabilizer on Free Swell Index
Table 7 indicates increase in the organic matter of the soil which can be traced to the organic loading of the treatment reagent. The chemical oxygen demand (COD) of the nanomaterial ranges between 53,200 mg/l and above while Biochemical Oxygen Demand range between 9.5 mg/l and 22.9 mg/l. Lower results in BOD oxygen demand to COD shows that the organic content is very high for bacterial decomposition. Likewise, comparing the results of the soil dissolved organic matter show that the nanomaterial contributes to the high yield compound with the untreated samples. This impacted organic matter composed chiefly of carbon, hydrogen, oxygen, nitrogen and smaller quantities of sulfur and other element, has a great influence on the soil chemical properties.
The organic fraction serves as a reservoir for the plant essential nutrients; nitrogen, phosphorus, and sulfur, increases soil water holding and cation exchange capacities and this observed in the various test results.
The most chemically active fraction of soils consists of colloidal clays and organic matter Colloidal particles that are so small (<0.002 mm) and in the soil samples investigated, they range between 21 and 26%. They remain suspended in water and exhibit a very large surface area per unit weight. These materials also generally exhibit net negative charge and high adsorptive capacity.
The chemical process of mixing with nanomaterial altered the active soil properties geotechnical properties such as strength, swell potential, volume changes and hydrophobicity.
According to Modified Free Swell Index suggested by Sivapullaih et al. (1987), the modified free swell index for all locations is above 10. The classification of soils based on Modified Free Swell Index for all the locations samples could be considered as high swelling potential.
Table 7 shows result of free swell test for two sample locations. Analysis of results of the 24-h free swell test showed that the swell potential decreased with increasing stabilizer content. This could be due to chemical reaction. The nanomaterial was able to effectively absorb the penetrating water causing swelling at the plate-plate interface and possibly seal up the interface such that water ingress is prevented, also the stabilizer in attracting and absorbing the penetrating water through an irreversible reaction, form a more stable precipitation to seal up the interface. That is;
Also from mineralogical consideration, the divalent Ca2+ ion readily replaces and substitutes the monovalent Na and K+ ions. This explains the improved mechanical and physical characteristics of the soils (Lambe and Whitman, 1969; Zydex Industries, 2010). At a maximum allowable 24 h free swell of about 2.5% as specified by Sivapullaiah et al. (1987). Table 9 summarize the stabilizer (Nanomaterial) requirements for all the samples to achieve moderate swell.
Nanostructure and Free Swell
This section summarizes the effect of fineness of the soil particles on the impact of nanomaterial as stabilizing additive on the soil. The degree of fineness of the soil material is indicative of the microstructure of the soil at the Nano level. Table 8 shows the nanomaterial concentration (measured in water dilution ratio) for a 24 h 2.5% free swell.
It is observed from Table 8 that samples from Loc 1-R, Loc 2 –R and Loc 3-B achieved maximum allowable 2.5% free swell (24 h) at nanomaterial nanomaterial / water ratio of 1:50 and 1:100 while Loc 4-B and Loc 5 –D needed 1:150 nanomaterial ratio to reach moderate level. The divergent results show that the lower the percentage fines of the active soil, the more effective the stabilizer is in achieving the desire free swell and minimize if not eliminate the swelling or expansive potential of the active soils. This result was broadly expectations (see also Figure 3).
Effect of Stabilizer or Grain Size Distribution of Active Soil
Addition of stabilizers (and with their increasing concentration), shifted the grain size distribution curves of all the sample for all locations to a lesser percent finer than D. The trend was observed as shown in the grain size distribution curves of Figure 4. The same patter was observed in all cases.
The curve in Figure 4 shows the effect of the nanomaterial. It results in pozzolanic reactions that cause the flocculation of the clay particles and consequent agglomeration. As stated previously, the more fines in the sample, the higher the concentration of Nanomaterial stabilizer required.
Effect of Stabilizer on the Atterberg Limits of Active Soil
The results on Atterberg Limits show changes in plasticity characteristics of the original untreated active soil and the treated samples. Table 10 highlights an increase in the plastic limit, a decrease in liquid limit, a decrease in plasticity index, decrease in shrinking limits and decrease in activity as observed with increasing concentration of the nanomaterial stabilizer.
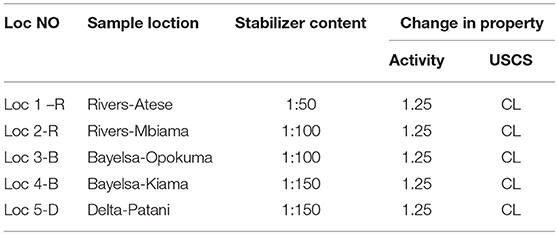
Table 10. Effect of nanomaterial on classification by activity levels (source: analysis of laboratory results).
The reduction in plasticity can be explained by the fact that Cation exchange reactions result in the flocculation and agglomeration of soil particles. Consequently, there is reduction in the amount of clay-size material and hence the soil surface area (Terzaghi et al., 1996).
In all the locations, the samples investigated showed remarkable improvements when treated with nanomaterial. LL, PL, SL, plasticity index (PI) for Loc 1–R improved by 9.49, 7.28, 5.21, and 18.08% respectively for a nanomaterial dilution ratio of 1:250. These pattern/trend of improvement was observed in all the location samples as shown in Table 10.
Effect of Stabilizer on Classification Based on Soil Activity and USCS
Based on activity as defined by Skempton (1953), the activity of studied samples from the five locations ranged between 1.25 and 1.35. This indicated that the soils were all active in their untreated state, by description. Also they have typical soil/ mineral values that describe them as calcium montmorillonite and organic alluvial clay (Barnes, 2000).
Table 9 shows the transitions in the activity of soil samples from high to medium after treatment at 1.50 dilution ratio (nanomaterial concentration). Thus the activity level changes from high to medium for location samples in Loc 1 and Loc 2. Also the same change in site activity level was observed from Loc 2 at dilution ratio of 1:100.
Table 10 summarizes the stabilization dilution level that achieved the minimum acceptable change in activity for each location studied.
It is noted that the nanomaterial had effect in dilution rations as low as 1:150 (lower nanomaterial concentrations), for samples LOC 4-B and LOC 5-D. Table 5 also shows that these two samples have higher Silicon concentrations of 28 and 29 parts per million respectively.
Effect of Stabilizer on the Activity and Swelling Potential of Active Soil
Activity of the location samples decreased with increasing concentration of the stabilizer as stated in preceding section. It was also observed that such increase caused the swelling potential to change from high to moderate (see Table 10 in preceding section). The classification and consequent observation is in accordance to Seed et al. (1962) (see Figures 5, 6).
Effect of Stabilizer on the Specific Gravity of Active Soil
The specific gravity decreased with the increase of stabilizer (see Figure 7). This decrease could result from likely reduction in the density of solids in the active soil. Also, it is observed that the decrease of the specific gravity resulted in decreasing the Modified Free Swell Index.
The specific gravity value was 2.56–2.68 and this falls within the expected values for un-stabilized expansive soils.
Effect of Stabilizer on the Percent Swell and Water Absorption
Percent swell and water absorption decreased with the addition of the stabilizer (Table 10). It is envisaged that with increasing amount of stabilizer, the organosilane compound which is the nanomaterial released a reactive silanol (Si- oH) group which had condensed with other group of silanol group. This results in the surface of siliceous fillers forming siloxan linkage (Zydex Industries, 2010) and consequently affect decrease in water absorption with a resultant improved hydrophobicity. The maximum reduction in percent swell achieved was less than the maximum allowable swell, which is 2% Ola (1983a,b). It is observed that the nanomaterial have progressive improvement in the engineering properties in all the location soils and that it can be used to alter the active soil properties seeing its effects on the swell and water absorption as shown in Table 11. Table A-1 in Appendix (Supplementary Material) contains an enlarged data set for all the locations. It shows that, samples of Loc 1-R, Loc 2 –R and Loc 3 –B improved in percent Swell and absorption were over 60% and 70% respectively as compared to Loc 4 –B and Loc 5 –D for which results were less than 40% and 30% for percent swell and absorption respectively. It was generally observed that free swell decreases continually with increasing percent fines. Thus, the higher the percentage fines of the active soil, the more effective the Nanomaterial stabilizer. From Figure 2 the fines in Loc 4-B samples are over 90% in average while the fines in Loc5-D are less than 80%. This indicates that clay particles of the former samples will exhibit larger surface area and electrical forces than the latter samples. The active soil identified to contain montmorillonite mineral is subject to isomorphous substitution, which results in giving the mineral a residual negative charge on the surface and positive charge on the edges. This further results in interparticle contact between the positively charged edges and the negatively charged surface, developing flocculated structure. Thus, with the presence of the surrounding fresh water and the stabilizer, cation exchange reaction further results in the flocculation and agglomeration of the soil particles. The cumulative results are a reduction in the amount of the clay–size materials and hence the soil surface area, which inevitably amount to reduction in plasticity. Due to the change in texture, a significant reduction in the swelling of the soil occurs and also, the solubility of silica is increased in a higher pH environment and silica becomes available as a cementing agent. The results shows that hydrophobicity increased for all samples.
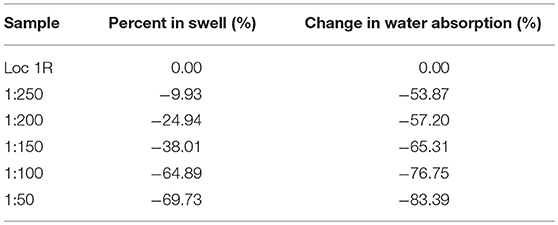
Table 11. Percentage changes in percent swell (%) and water absorption (source: analysis of laboratory results).
Influence of Mechanical Stabilization
Generally, mechanical stabilization is the compaction process whereby soil particles or grains are constrained to pack more closely together by mechanical means through expulsion of gas or air from the soil mass, and hence reduction of voids.
Maximum Dry Density (MDD)
Figure 8 shows a plot of Maximum Dry Density (MDD) versus stabilizer content extracted from laboratory results. It is observed that the MDD increases continually with increasing stabilizer content. This is a variance with what obtains in other soils where density reduces or minimized before increasing continually (Ola, 1974).
However, the improved densities could be explained by the existence of repulsive force between the stabilizer molecules and the clay intermolecular structure. This causes increases in the inter-molecular distance and increase in the void ratio Santamarina (2001). In addition, from the chemistry of reaction of nanomaterial reported in literature (Ugwu et al., 2013), some of the nanomaterial ions may have attracted some of the clay particles, which lead to forming coagulation-a phenomena that affect the fragility of clay compaction test.
Optimum Moisture Content (OMC)
Figure 9 shows the effect of the stabilizer on the moisture content of active soils.
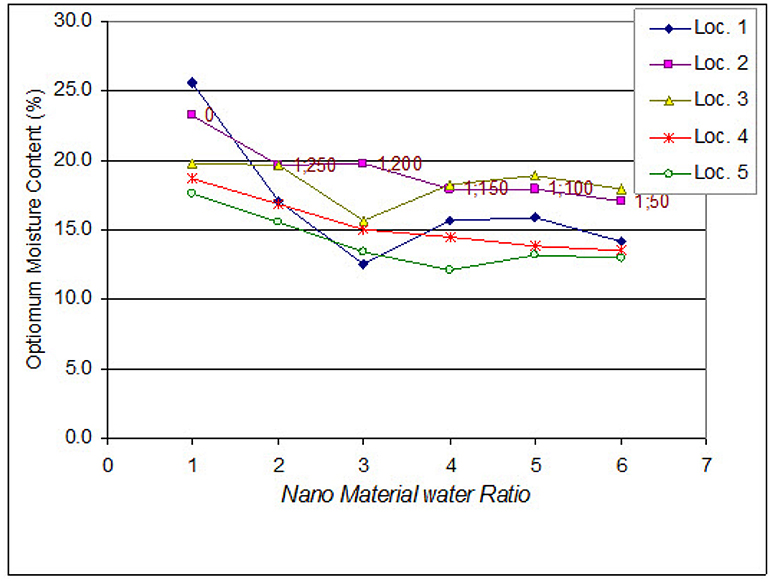
Figure 9. Variation of optimum moisture content with nanomaterial (source: analysis of laboratory results).
In all cases, the OMC of the samples decreased from the start and the decrease continued with increasing concentration of the nanomaterial stabilizer. The soil mixed with the stabilizer causes cation exchange, flocculation and agglomeration of the clay particles which decrease the plasticity index of the soil and decrease the optimum moisture content, because of the decrease in surface area.
California Bearing Ratio (CBR)
Figure 10 shows the result of CBR test. It is observed that untreated samples had CBR values as low as 1%, but after treatment the CBR improved to 16% with increasing nanomaterial concentration. This is because the efficacy of the nanomaterial stabilization is a function of portion and mineral type (active or inactive) as observed in previous research reported in literature.
Thus as the stabilizer content increased, the CBR also increased for all the sample locations. However it is observed that the trend of increase moved progressively from Loc 1-R toward Loc 5-D, and can be explained due to the content of fines.
Effect of Stabilizer on Unconfined Compressive Strength (UCS)
Untreated and treated samples were cured for 1 day and 7 days and subsequently subjected to unconfined compressive strength Test. Results are as shown in Table A-1 and Figure 11. This test was conducted to evaluate the performance of soil stabilization and is one of the main parameters applied in the design of earth work projects (Yarbasi et al., 2007).
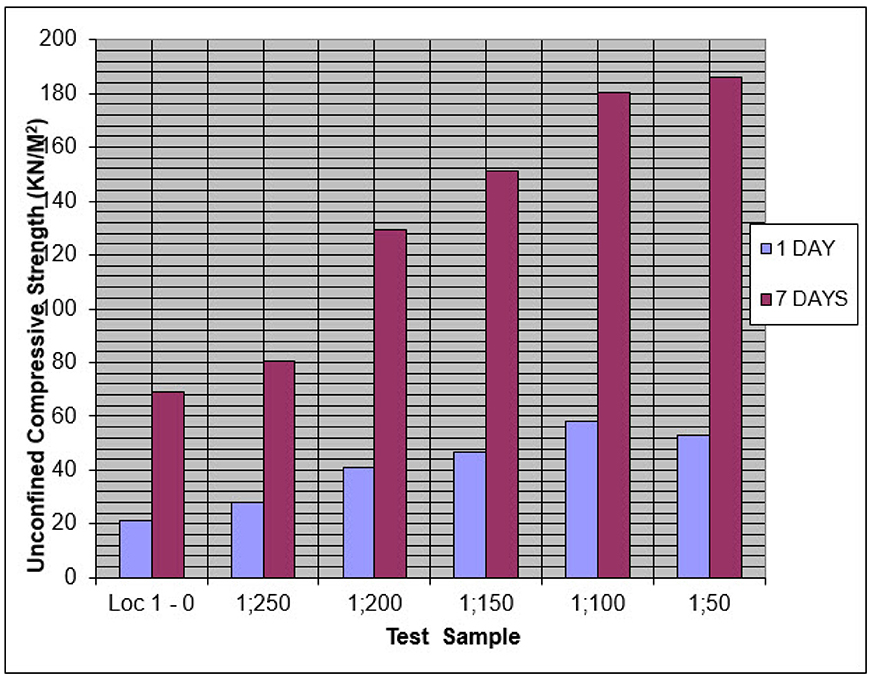
Figure 11. Effect of addition of nanomaterial on the unconfined compressive strength (source: analysis of laboratory results).
Figure 11 shows that at 7 days cure, samples had higher compressive strength Values than at 1 day cure. It was also observed that the UCS values increased with increase of stabilizer content. The increase in the UCS strength could be attributed to Pozzolanic Reaction.
Effect of Stabilizer on Durability
Table A-2 and Figure 12 show results of durability test conducted to evaluate the durability of stabilized active soil subjected to weathering conditions (Zhang and Tao, 2008; Ghazavi and Roustaie, 2010). The results indicate decrease in probable amount of deterioration with increase in stabilization content. The durability index were estimate after two drying and wetting cycles with absorption in accordance with ASTM D 4644 −08, 1999. It was observed that Locations 1-R and 5- D show a durability index improvement, reducing from 65 to 20% and 55 to 13% respectively.
Also durability index reduced progressively from Loc 1-R in Bayelsa State toward Loc 5 –D in Delta State. This indicates that the nanomaterial stabilizer performs better with samples with lesser fines as indicated in the gradation curves in Figure 2.
Statistical Tests on Effects on Nanomaterial on Engineering Properties of Active Soil
This section discusses the results of statistical tests performed on the results discussed in preceding sections. The objective was to use standard statistical tests and validate that the observed changes in the engineering properties actually resulted from the addition of the nanomaterial as stabilizing agent. The statistical tests involve comparing the values of the soil engineering properties before and after treatment and thus establishing that the nanomaterial additive is the main causation agent. Thus analysis of variance (ANOVA) tests were done at 95% confidence limit. Details of the tests are available in mainstream statistics literature. The statistical tool used was Statistical Package for Social Sciences (SPSS, 2015). The ensuing section discusses the results of the tests with observation and/or comments.
The ANOVA results summarized in Table A-3 show different levels of effect of the nanomaterial on the expansive soil studied. These observations are summarized graphically in Figure 13.
In Table A-3 and Figure 13, the higher the value of F, the more significant the effect of addition of nanomaterial on soil properties. Hence addition of nanomaterial has the most effect on plasticity index and the least effect on 1 day unconfined compressive stress. The other engineering properties where the nanomaterial has high effect are: clay content, free swell, CBR, shrinkage limit, soil activity, liquid limit, swell, water absorption, and silt a & fines. The results validate the effect of nanomaterial on re-engineering the properties of active soils.
Conclusion and Recommendations
This paper discussed research that investigated reengineering the properties of active soils using nanomaterial. Active soils cause serious economic damage to sustainable infrastructure development wherever there are found. Detailed characterization of the active soil and the suitability of nanomaterial as stabilizer in tackling the swelling potential of active soil were discussed. From the series of tests conducted on the active soil treated with nanomaterial as stabilizer, the following conclusions are drawn.
Addition of nanomaterial decreases liquid limit, plasticity index and shrinkage limit but increased plastic limit with the increase in the nanomaterial percentage. The liquid limit values decreased from 63.2 to 47.9%. The plasticity index values decreased from 42.6 to 23.6%, while the shrinkage limit values decreased from 9.6 to 6.8%. However, the plastic limit increased from 20.6 to 25.4%. Modified free swell also decreased with the addition of nanomaterial stabilizer and subsequently altered the swell potential from high to moderate.
The application of nonmaterial to the active soil reduced the water absorption from 27.1 to 4.5%. This shows that the nonmaterial imparts molecular level hydrophobicity on the treated surface resulting to water repellant zone as observed in the result.
With the addition of the stabilizer, CBR and UCS values increased from 5 to 15% and 6.90 to 185.9 KN/m2 respectively. The addition of the nanomaterial stabilizer was found to improve the bearing and the strength of the active soil and as such and is recommended for road works.
Specifically, durability of the nanomaterial-stabilized active soil improved considerably from the very low values of 37.1% at low content to very high values in excess of 80% at higher nanomaterial content.
The addition of nanomaterial as stabilizer to active soils decreases its swelling behavior, and also the hydrophobicity to a great extent. The outcome of the Active soil–Nano material interactions and also with the enhanced knowledge of Active soils implies that evolving Nano science and Nanotechnology can solve the global engineering problems and challenges posed by active soils in sustainable infrastructure delivery.
Analysis of variance (ANOVA) tests was carried out at 95% confidence limit validate the effect of nanomaterial on re-engineering the properties of active soils.
The research shows that the presence of a siliceous film enhances the active soil-nanomaterial interaction, and consequently improves the engineering properties. It is therefore recommended that future work investigate the injection of silica into active soil as an “admixture” to accelerate the nanomaterial-driven reengineering process. In addition there is need to investigate the health and safety as well as life cycle cost (LCC) implication of nanomaterial as additives to stabilize soils in civil engineering. In view of the existence of potential risks in Nanotechnology applications in construction, there is an overarching need to formulate National policies and roadmaps for broader Nanotechnology applications in construction industries at country-specific levels.
Author Contributions
OU the first and corresponding author initiated the research reported in the paper as part of his over 10-year research portfolio on Nanotechnology in Civil Engineering. He supervised and approved the experimental design from data collection to laboratory tests and analysis of results to ensure that the work followed international best practices in conducting such research from the perspectives of research ethics and integrity etc. He was the main supervisor of the Ph.D. work associated with the research. AO the second author collected the field data and conducted the laboratory experiments as part of the requirements for his Ph.D. work at the University of Nigeria. CN the third author assisted the first author in the Ph.D. supervision, and contributed in ensuring quality of the laboratory work and data analysis.
Conflict of Interest Statement
The authors declare that the research was conducted in the absence of any commercial or financial relationships that could be construed as a potential conflict of interest.
Supplementary Material
The Supplementary Material for this article can be found online at: https://www.frontiersin.org/articles/10.3389/fbuil.2018.00065/full#supplementary-material
References
Abu, S., Mohammed, S., and Minghal, A. A. B. (2018). “Strength characteristics of nano calcium silicate, fly ash and lime blended tropical soils,” in Conference: IFCEE 2018. March 2018, Vol. 296.
Agrawal, T., and Agrawal, M. (2011). Performance of concrete with its new atomic strength by use of Nanotechnology. Int. Trans. Alied Sci. Technol. 1, 17–20.
Akpokodje, E. G., and Arumala, J. O (1987). Soil properties and pavement performance in niger delta. Q. J. Eng. Geol. Hydrogeol. 20, 287–296. doi: 10.1144/GSL.QJEG.1987.020.04.05
Ali, S. M., and Korrane, S. S. (2011). Performance Analysis of Expansive Soil Treated With Stone Dust and Fly Ash. 16, 913–982.
Al–Rawas, A. A., Taha, R., Nelson, J. D., Al–Shab, T. B., and Al–Siyabi, H. (2002). A comparative evaluation of various used in the stabilization of expansive soils. Geotech. Test. J. 25, 199–209. doi: 10.1520/GTJ11363J
ASTM Standard Test Methods for One–Dimensional Swell or Settlement Potential of Cohesive Soils (1999). Annual Book of ASTM Standards (West Conshohocken, PA), D4546–03, 4–8, 1–7.
Athulya, G. K., Sushovan, D., and Mandal, J. N (2017). Performance evaluation of stabilised soil–slag mixes as highway construction material. Int. J. Geotechn. Eng. 11, 51–61. doi: 10.1080/19386362.2016.1184874
Bailey, S. W. (1980). Summary of recommendation of AIPEA nomenclature committee. Clays Clay Min. 28, 73–78. doi: 10.1180/claymin.1980.015.1.07
Bariwen, B. A., Tawari, C. C., and Abawei, J. F. (2012). Some environmental effects of flooding in the niger delta region of Nigeria. Int. J. Fish. Aquat. Sci. 1, 35–46.
Bartos, P. J. M. (2006). “Nanotechnology in construction: a roadmap for development” in Proceedings of ACI Session on ‘Nanotechnology of Concrete: Recent Developments and Future Perspectives (Denver, CO).
Borchardt, G., and Smectites, P. (1989). Minerals in Soil Environments, 2nd Edn (Madison, WI: Soil Science Society of America), 675–727.
Boyd, J. (2010). Nanomaterials poised for big impact in construction. Availablle online at: www.sciencedaily.com/releases/2010/07/100728121337.htm (Accessed, July 2010).
Choi, Y., Hyunwook, C., and Tae, S. Y. (2016). Engineering characteristics of chemically treated water–repellent kaolin. Materials 9:E978. doi: 10.3390/ma9120978
Das, B. M. (1999). Principles of Foundation Engineering, 2 Forth Edn. New York, NY: Brooks/Cole, 739.
Erzin, Y., and Erol, O. (2004). Correlations for quick prediction of swelling pressure. Elect. J. Geotech. Eng. 9, 476.
Feynman, R. (1960). There's plenty of room at the bottom (reprint from speech given at annual meeting of the West of the American Physical Society). Eng. Sci. 23, 22–36.
Ghazavi, M., and Roustaie, M. (2010). The influence of freeze–thaw cycles on the unconfined compressive strength of fiber-reinforced clay. Cold Reg. Sci. Technol. 61, 125–131. doi: 10.1016/j.coldregions.2009.12.005
Kennep, G. (2006). “Nanotechnology for transportation engineers.” in CPATT Symposium (Waterloo, ON: University of Waterloo), October 13, 2006.
Lee, J., Mahendra, S., and Alvarez, P. J. I. (2010). Nanomaterials in the construction industry: a review of their alications and environmental health and safety considerations. ACS Nano 4, 3580–3590. doi: 10.1021/nn100866w
Liu, R., Zhang, R., Zhong, X., and Chen, J. L (2007). Nanotechnology Synthesis Study: Research Report. Department of Electrical and Computer Engineering, University of Houston, Texas, USA.
Maghsoudi, A. A., and Dahooei, F. A. (2009). Application of nanotechnology in self–compacting concrete design. IJE Trans. B Alicat. 22, 229–244.
Mowafy, Y. M., and Bauer, G. E. (1986). Prediction of swelling pressure and factors affecting the swell behavior of an expansive soil. Transport. Res. Rec. 1032, 23–27.
Muntohar, A. S. (1999). Behaviour of Engineering Properties of the Clay Blended with LRHA (Lime Rice Husk Ash). Research Rep. from Grant, Muhammadiyah University of Yogya – Karta, Yogya – Karta, Indonesia.
Muntohar, A. S., and Hantoro, G. (2000). Influence of rice husk ash and lime on engineering properties of clayey subgrade. Electron. J. Geotech. Eng. 5.
Okagbue, C. O. (2007). Stabilization of clay using wood ash. J. Mater. Sci. Civil Eng. ASCE 19, 14–18. doi: 10.1061/(ASCE)0899-1561(2007)19:1(14)
Okagbue, C. O. (2014). Geotechnical and environmental problems of the niger delta. Bull. Int. Assoc. Eng. Geol. 40, 119–126. doi: 10.1007/BF02590349
Okagbue, C. O., and Onyeobi, T. U. S. (1999). Potentials of marble dust to stabilize red tropical soils for mad construction. Eng. Geol. 53, 371–380.
Okagbue, C. O., and Yakubu, J. A. (2000). Limestone lime ash waste as a substitute for lime in soil improvement for engineering construction. Bull. Eng. Geol. Environ. 58, 107–113. doi: 10.1007/s100640050004
Ola, S. A. (1974). Need for estimated cement requirements for stabilizing lateritic soils. J. Transp. Engrg. ASCE. 100, 379–388.
Ola, S. A. (1983a). “The geotechnical properties of the black cotton soils of Northeastern Nigeria,” in Tropical soils inNigeria in Engineering Practice,ed S. A. Ola (Rotterdam: A. A. Balkema), 85–101.
Ola, S. A. (1983b). “The geotechnical properties of the Sokoto Soft Clay Shales of Northwestern Nigeria,” in Tropical soils in Nigeria, in Engineering Practice, ed S. A. Ola (Rotterdam: A. A. Balkema), 131–144.
Omotosho, O., and Ogboin, S. A. (2009). Active soils of the nigerdelta in road pavement design and construction. Geotech. Geol. Eng. 27, 81–88. doi: 10.1007/s10706-008-9213-4
O'Neill, M. W., and Poormoayed, N. (1980). Methodology for foundation on expansive clays. J. Geotech. Eng. Div. Am. Soc. Civil Eng. 106, 1345–1367.
Pan, D., Nong, Z., Chenghao, Z., Deyu, Q., Changliang, H., and Sen, Y. (2018). Long–term mechanical behavior of nano silica sol grouting. Nanomaterials 8, E46. doi: 10.3390/nano8010046
Prasanna, P. K., and Jnanendra, N. M. (2017). Performance assessment of stabilized soil with fly ash– nano material mixes. J. Geotech. Transport. Eng. 3, 35–47.
Rana, K., Rana, S. B., Kumarai, A., and Kran, V. (2009). Significance of nanotechnology in construction engineering. Int. J. Recent Trends Eng. 1, 44–48.
Santamarina, J. C. (2001). “Soil behavior at the microscale : particle forces,” in Soil Behavior and Soft Ground Construction, Proceedings of the Symposium October 5-6, 2001, eds J. T. Germaine, T. C. Sheahan, and R. V. Whitman (Cambridge, MA; Reston, VA: American Society of Civil Engineers), 25–56.
Seed, H. B., Woodward, R. J., and Lungren, R. (1962). Prediction of swelling potential for compacted clays. J. Soil Mech. Found. Div. 88, 53–87.
Sivapullaiah, P., Sitharam, T., and Subba Rao, K. (1987). Modified free swell index for clays. Geotechn. Test. J. 10, 80–85. doi: 10.1520/GTJ10936J
Sivapullaiah, P. V. (1996). “Pozzolanic stabilization of expansive soils,” Expansive Soils: Recent Advances in Characterization and Treatment, editors A. A. Ali–Rawas and M. F. A. Goosen (London: Taylor and Francis Group), 419–434.
Sivapullaiah, P. V., Shidharan, A., and Stalin, V. K. (1996). Swelling behaviour of soil–bentonite mixtures. Can. Geotech. J. 33, 808–814. doi: 10.1139/t96-106-326
Skempton, A. W. (1953). “The colloidal activity of clays.” in Proceedings of the Third International Conference on Soil Mechanics and Foundation Engineering (Zurich), 57–61.
Sobolev, K., and Gutiérrez, M. F. (2005a). How nanotechnology can change the concrete world, part, I. Am. Ceram. Soc. Bull. 84, 14–18.
Sobolev, K., and Gutiérrez, M. F. (2005b). How nanotechnology can change the concrete world, part, I. Am. Ceram. Soc. Bull. 84, 16–20.
Steyn, W. J. (2008). “Research and alication of nanotechnology in transportation.” in 27th Southern African Transport Conference (SATC 2008) (Pretoria), 345–353.
Syed, A., Sayeed, M., Arif, A. B. M., and Mohammed, A. L. (2018). “Strength characteristics of nano calcium silicate, fly ash and lime blended tropical soils” in IFCEE 2018 (Orlando, FL). doi: 10.1061/9780784481592.011
Taha, M. R. (2009). “Geotechnical properties of soil–ball milled soil mixtures,” in 3rd Symposium on Nanotechnology in Construction (Berlin; Heidelberg: Springer–Verlag), 377–382. doi: 10.1007/978-3-642-00980-8_51
Terzaghi, K., Peak, R. B., and Mesri, G. (1996). Soil Mechanics in Engineering Practice, 3rd Edn. New York, NY: John Wiley and Sons Inc.
Ugwu, O. O. (2013). Nanotechnology for sustainable infrastructure in 21st century civil engineering. J. Innov. Eng. 1:E2.
Ugwu, O. O., Arop, J., Nwoji, C. U., and Osadebe, N. N. (2013). Nanotechnology as preventive engineering solution to highway infrastructure failures. ASCE J. Construct. Eng. Manag. 139, 987–993. doi: 10.1061/(ASCE)CO.1943-7862.0000670
Ugwu, O. O., Olowogbayi, T., and Nwoji, C. U. (2014). Nanomaterials for sustainable highway pavement. Trans. Niger. Soc. Eng. 48:56.
Yarbasi, E., and Kalkan, S., Akabulut (2007). Modification of the geotechnical properties as influenced by freeze– thaw, of granular soils with waste additives. J. Cold Reg. Sci. Technol. 48, 44–54. doi: 10.1016/j.coldregions.2006.09.009
Keywords: nanotechnology, construction process innovation, infrastructure sustainability, sustainable built environment, expansive soil stabilization, sustainable engineering practice, characterization
Citation: Ugwu OO, Ogboin AS and Nwoji CU (2018) Characterization of Engineering Properties of Active Soils Stabilized With Nanomaterial for Sustainable Infrastructure Delivery. Front. Built Environ. 4:65. doi: 10.3389/fbuil.2018.00065
Received: 25 April 2018; Accepted: 12 October 2018;
Published: 13 December 2018.
Edited by:
Jean-Claude Morel, Coventry University, United KingdomReviewed by:
Eric Vincens, Ecole Centrale of Lyon, FranceYaolin Lin, Wuhan University of Technology, China
Copyright © 2018 Ugwu, Ogboin and Nwoji. This is an open-access article distributed under the terms of the Creative Commons Attribution License (CC BY). The use, distribution or reproduction in other forums is permitted, provided the original author(s) and the copyright owner(s) are credited and that the original publication in this journal is cited, in accordance with accepted academic practice. No use, distribution or reproduction is permitted which does not comply with these terms.
*Correspondence: Onuegbu O. Ugwu, b251ZWdidS51Z3d1QGZ1bmFpLmVkdS5uZw==; b251ZWdidXVnd3VAZ21haWwuY29t orcid.org/0000-0002-6746-1464