- 1Lumir Oy, Vantaa, Finland
- 2Aalto Acoustics Lab, Department of Signal Processing and Acoustics, School of Electrical Engineering, Aalto University, Espoo, Finland
- 3Department of Computer Science, School of Science, Aalto University, Espoo, Finland
Acoustic comfort is directly related to enhanced well-being and performance of people. A typical challenge faced by architects and acousticians is to achieve adequate acoustics while maintaining the aesthetics of the space and reducing the visual aspects of acoustic materials and elements. In this study, we present a biofiber-based acoustic coating as a feasible solution to improve acoustic environments while preserving the aesthetics of spaces. An acoustic coating is a thin layer of absorption material, but the coating can be sprayed on other sound absorbing structures to make it more effective on a wide frequency range. In addition, this biofiber-based coating acts as a carbon sink during its operating life, thus reducing the carbon footprint of the building. Therefore, the coating is sustainable and is an environmental friendly solution. The absorption properties of the biofiber-based coating are demonstrated in the present study with three case studies, which all had demanding requirements to conceal the acoustic structures.
1. Introduction
The acoustic design is included in the plans in many cases at the expense of architectural vision. Traditionally, acoustic structures are suspended ceiling systems or glued sound absorbing panels which might not be visually the most preferred solution. Using panels or suspended structures are often impossible to use in historically significant or otherwise architecturally protected buildings. Acoustic coatings offer a solution to improve acoustic comfort, while maintaining the aesthetics of surfaces, and even be difficult to be visually perceived as acoustical material. The main constraint of acoustic coatings is the limitation of their acoustic properties due to their relatively small thickness. Despite this fact, acoustic coatings can benefit from underlying surfaces so that they can be applied on top of other acoustic materials to improve the acoustic properties of the whole structure and, at the same time, enhance their visual aspects.
Over the past years, the study and development of acoustic eco-materials has received much attention (Arenas and Asdrubali, 2018). Cucharero et al. (2021) studied the influence of the ultrastructure of wood-based pulp fibers on sound absorption. They reported that wood-based pulp fiber foams exhibit similar sound absorption properties as commercial glass wool panels and that pulp fibers of smaller dimensions and processed fiber foams are more effective to produce fibrous sound absorbers. Similar findings regarding fiber dimensions have been reported by other authors (Koizumi et al., 2003; Oldham et al., 2011). Other researchers have reported efficient sound absorption properties of several biomaterials, such as cork (Iannace et al., 2020), bamboo (Koizumi et al., 2003), jute (Oldham et al., 2011), cotton (Oldham et al., 2011), hemp (Oldham et al., 2011; Berardi and Iannace, 2015), kenaf (Lim et al., 2018), coir (Fouladi et al., 2010), fique (Navacerrada et al., 2014; Berardi and Iannace, 2015), ramie (Yang and Li, 2012). Furthermore, models to predict the sound absorption properties of some natural fibers have also been developed (Berardi and Iannace, 2017). Some researchers have reported cases in which acoustic corrections of a variety of spaces have been successfully accomplished with the help of green acoustic materials (Iannace et al., 2013, 2020).
Acoustical measurements are necessary for the prediction of the most appropriate acoustic treatment. Predictions of room acoustics can be conducted using techniques ranging from analytical expressions to computer simulations. Several analytical expressions, namely, Sabine (Sabine, 1900), Eyring (Eyring, 1930), Millington (Millington, 1932), Cremer (Cremer and Müller, 1982), Kuttruff (Kuttruff, 1994), Fritzroy (Fitzroy, 1959), Neubauer, and Arau-Puchades (Arau-Puchades, 1988), have been developed to predict reverberation time. The accuracy of different analytical expressions has also been studied by many authors. Bistafa and Bradley (2000) reported that the expression developed by Arau-Puchades was the formulae that more accurately predicts reverberation time for different configurations of sound absorbing materials in an unoccupied classroom. The authors also indicated that the most accurate analytical expression depends on the amount and distribution of sound-absorbing material in the room. Astolfi et al. (2008) concluded that the use of Sabine and Eyring expressions is sufficient to predict reverberation time in small classrooms since neither more complex models nor numerical models lead to higher accuracy. Similar findings were reported by Passero and Zannin (2010). In the study conducted by Prawda et al. (2020), the model of Fitzroy provided the most accurate prediction of reverberation time, whereas Sabine formula was reported to predict reverberation time more accurately than other more complex analytical expressions.
In this study, we present three cases where bio-based acoustic coatings have been used to improve the acoustic environment. Cases consist of a traditional small office space, a staircase functioning as an art installation, and a historically significant space at the Supreme Court of Finland. High visual requirements were set in all of the cases for the sound absorbing structures; in practice, the acoustic treatment had to be unnoticeable. Appearance of the spaces were protected for historical reasons in cases I and III, which sets higher requirements for the surface structure of the materials and also structure dimensions. In the staircase, the aim was to produce nearly anechoic acoustic environment to the multistore narrow space made out of concrete with visually unnoticable structures.
The visual evaluation of the spaces was done by the users of the spaces and clients. To evaluate the acoustics of the three premises, reverberation time, T30, and speech clarity, C50, were determined according to ISO 3382-1:2009 before and after installation of the acoustic treatment. Additionally, the effect of the acoustic treatment to reverberation time was predicted using Sabine equation.
In the case studies presented in this study, we demonstrate how bio-based acoustic coating can be used to sustainably increase the comfort of spaces and, in some cases, even decrease the carbon footprint of the building. For example, the most commonly used acoustic material, mineral wool, is very energy intensive, which leads to high carbon footprint (Ruuska, 2013). In contrast, plants are an abundant and sustainable source of fibrous raw material for acoustic products. Cellulose, the main structural component of natural fibers, consist about 49 weight percent (wt%) of carbon. Plants acquire this percent of carbon mainly as carbon dioxide from the air, which is processed into cellulose and other components via biosynthesis, with oxygen resulting as a side product. As a rule of thumb, carbon bound in 1 kg of cellulose corresponds to roughly 1.5 kg of atmospheric carbon dioxide. Although the cellulose represents only a fraction of all building materials, their ability to bind atmospheric carbon influences the total carbon footprint of the building. In this study, we speculated the effects of the biofiber-based acoustic coating on the carbon footprint of the space and buildings, as acoustic materials seldom have a positive impact on the total carbon footprint of the buildings. Nevertheless, in this study, we demonstrate that bio-based products could vastly reduce the carbon footprint of acoustic materials.
2. Materials and Methods
2.1. Materials
A biofiber-based acoustic coating sprayed on different underlying surfaces was used as the acoustic solution for all the case studies presented in this study. The biofiber-based acoustic coating is manufactured by Lumir Ltd., a Finnish company specializing in bio-based and circular economy acoustic materials. Acoustic coating is sprayed on different kinds of surfaces as 6–8 mm layer. Sound absorption properties of the acoustic coating strongly depends on the underlying surface on top of which the coating is sprayed. Figure 1 shows the sound absorption coefficients measured for the acoustic coating sprayed on the following underlying surfaces:
• Solution 1: Biofiber-based acoustic coating sprayed on the solid surface without any air cavity left behind.
• Solution 2: Biofiber-based acoustic coating sprayed on a 20 mm glass wool.
• Solution 3: Biofiber-based acoustic coating sprayed on a perforated gypsum board (8 mm side square hole and 20% open area) with an air cavity of 200 mm filled with 50 mm of rock wool.
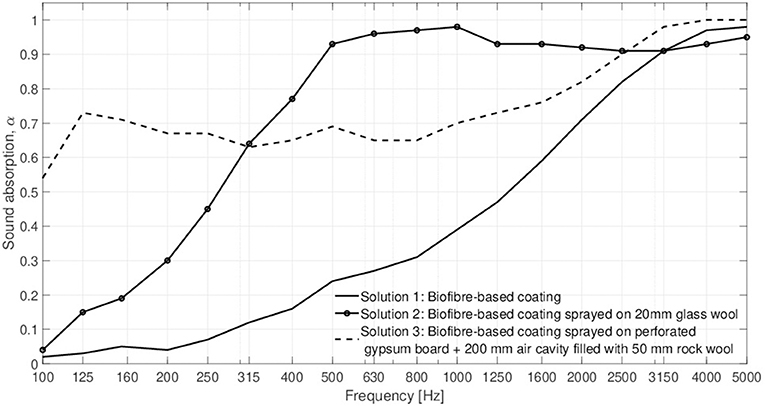
Figure 1. Sound absorption coefficients of biofiber-based acoustic coating sprayed on different base surfaces.
Sound absorption coefficients were measured according to the ISO 354 standard (ISO 354, 2003) regarding sound absorption measurements in a reverberation room operated by a Finnish Accreditation Service (FINAS) company.
In addition to the acoustic properties, biofiber-based acoustic coating acts as a carbon sink during its operating life, which, under normal conditions, extends to several decades. The coating used in this study contains about 80 wt% cellulosic fibers as the raw material. We can calculate from the density of the coating (1,250 kg/m3) that the biofibers used in the coating bind ~1.2 kg of CO2/m2 in its fibers. In this way, the installation of this acoustic coating reduces the carbon footprint of buildings, thus contributing to alleviate climate change. Table 1 presents carbon footprint and carbon uptake data of the acoustic materials used in this study (Ruuska, 2013). The carbon footprint is expressed in terms of carbon dioxide equivalent (CO2e), which is a sum of fossil emissions calculated with the help of the Intergovernmental Panel on Climate Change (IPCC) weighting factors for 100 years (IPCC, 2007). The units are expressed in grams of CO2e per kilogram of product. Carbon uptake values of glass wool and gypsum plasterboard are 0 since they consist of minerals, which inherently do not contain any carbon. The carbon footprint value of the pulp obtained from literature (Sun et al., 2018) is used in calculations for the biofiber-based acoustic coating. Coatings commonly contain binding agents, pigments, and other additives, which can be biobased or inorganic. However, their contribution to the carbon footprint of the acoustic coating is disregarded in this study.
2.2. Methodology
The acoustics of three different premises, including an artwork in a staircase space, a multifunctional room, and an office, have been designed, implemented, and measured. The models and dimensions of the spaces are shown in Figure 2. Effect of the designed acoustic treatment on reverberation time was predicted using the reverberation time measurements before the installation of acoustic materials as the starting point for the predictions. Sound absorption coefficients used in the predictions are shown in Figure 1 and Table 2. The acoustic structures in spaces were combinations of biofiber-based acoustic coatings and different base materials. Selection of the base material to the acoustic coating depended on the constrictions set by the owners of the building. Such restrictions were always mainly determined by minimization of the visual impact of acoustic materials and elements. In addition to changes in room acoustics, the environmental impact of the acoustic treatment to the building has been estimated by considering the carbon footprint and uptake of the installed acoustic materials.
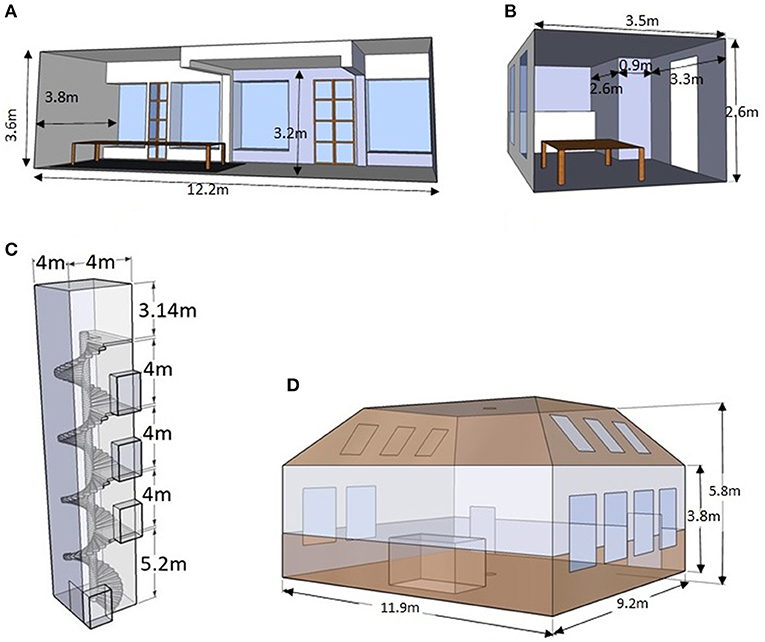
Figure 2. Models and dimensions of the acoustically treated premises: (A) The office of Co-founders, (B) Co-founder meeting room, (C) staircase space, (D) a multifunctional room in the Supreme Court.

Table 2. Sound absorption coefficients of building structures used to predict reverberation time through Sabine's theory (Cox and d'Antonio, 2009).
Among all the alternative reverberation formulae, the equations of Sabine (Sabine, 1900) and Arau-Puchades (Arau-Puchades, 1988) are used here. The Sabine equation requires uniform distribution of sound absorbing materials in the room, as well as diffused sound field, i.e., equal energy density in all positions of the room, as well as equal probability of sound propagating in all directions (Hodgson, 1996). Obviously, these conditions are not fulfilled in the rooms considered in this study. However, in the acoustic designs reported in the present study, rather than high prediction accuracy, we needed a fast tool to predict reverberation time, and within these terms, Sabine's equation was considered adequate. Additionally, it has been shown by many authors that, in regular shaped rooms, the accuracy of Sabine's equation is not much poorer than that of other more complex analytical expressions or room acoustic software (Bistafa and Bradley, 2000; Astolfi et al., 2008; Passero and Zannin, 2010; Prawda et al., 2020). The predictions obtained by using Sabine's equation are compared with those given by Arau-Puchades' theory. The expression of Arau-Puchades, as opposed to Sabine's theory, takes into account the non-uniform distribution of sound absorbing materials in the room. Furthermore, this expression has been reported to provide greater accuracy than Sabine's equation in spaces with high value of average sound absorption coefficient of room boundaries (Bistafa and Bradley, 2000).
Predictions of reverberation time presented in this study are realized by using measurements of reverberation time before the installation of acoustic materials as the starting point of predictions, which ensures greater accuracy than applying the formulas of Sabine or Arau-Puchades from scratch. Additionally, the simplicity of Sabine's equation may be a benefit for this prediction procedure, as it requires sound absorption coefficients of only the materials being replaced with the acoustic treatment, whereas Araus-Puchades' theory requires the sound absorption coefficients of all the materials located in the same dimension. In the Supplementary Material the procedure followed to predict reverberation time using Sabine and Arau-Puchades formulae has been described.
Evaluation of the acoustics parameters, reverberation time, T30, and speech clarity, C50, were done according to ISO 3382-1 (2009), before and after the installation of acoustic treatment. The software ARTA (Artalabs, 2019) was used to obtain impulse responses using the inverse swept-sine technique (Farina, 2000). The sound source was a Genelec 8030B, and the microphones were omnidirectional 1/4-inch measurement microphones of the model Superlux ECM-999. The Genelec 8030B is not fulfilling the directivity requirements of the ISO 3382 standard; thus, in these measurements, the sound source was always located in a sound reflecting corner to simulate the omnidirectional sound source. In addition, the results of all presented measurement are averages of individual measurements with at least two sound sources and three receiver positions, except for the meeting room shown in Figure 2B, where, due to the small dimensions of the room, only one sound source and two receiver positions were considered. Furthermore, all reported before and after measurements were done with the same equipment and in the same source and receiver positions to enable a fair comparison.
2.2.1. Case Study I: The Office of Co-founders
The office of Co-founders Ltd. consisted of two rooms, a meeting room and a common office space, with six workstation around a big table were considered. The meeting room was lightly furnished, and all the surfaces were concrete except one wall, which was covered with acoustic perforated gypsum boards. The common office space was over 3 m high and lightly furnished; all surfaces were concrete, and there were large windows on one side. The users of the space noticed that the most unpleasant acoustic problems were poor speech intelligibility, as well as bad sound insulation between rooms for speech.
The conditions for the acoustic treatment set by the clients were as few visual impacts as possible, brevity in the acoustic design, and installation of acoustic materials. Moreover, the thickness of the acoustic structure to be installed in the ceiling was restricted to 4 cm due to lighting and electrical devices and structural elements found in the ceiling. The proposed acoustic treatment involves:
• Ceiling of the common office space: biofiber-based acoustic coating sprayed on 20 mm glass wool panels glued to the ceiling (LW20 Comf).
• Ceiling of the meeting room: biofiber-based acoustic coating sprayed on 20 mm glass wool panels glued to the ceiling (LW20 Comf).
Acoustical measurements were conducted in the common office space using a total of four sound source–receiver combinations (three source and four receiver positions), and in the meeting room using two sound source–receiver combinations (one source and two receivers). The same source–receiver positions were used for the measurements before and after the installation of acoustic treatment.
2.2.2. Case Study II: Artwork by the Artist Group IC-98
The artist group IC-98 produced an artwork, named Mare Tranquillitatis, aimed to create a zone of complete silence that serves as a place of tranquillity and confrontational encounter, as described by the authors. The artwork was created in a five-floor spiral staircase, and the acoustic design of this space was part of the work.
The spiral staircase is located in the School of Business building constructed in 2018 at Aalto University. The dimensions of the staircase space are 4 × 4 × 20.3 m, with a volume of 325 m3, as shown in Figure 2C. All the walls, the ground floor, and the ceiling are painted concrete. The stairs and landings were mosaic concrete and the underneath of the stair-landing was plywood with an air cavity behind it. A concrete pile of 0.5 m of diameter was stranded in the middle of the spiral staircase from the ground floor up to a height of 18.5 m. There were neither sound absorbing materials nor acoustic elements in the space.
There were strict requirements for the acoustic treatment. Changes in the appearance of the staircase space were to be avoided, taking into account the color and the structure of the surfaces. Very thin acoustic structures had to be used as there was very little space between the stairs and walls. Only the walls, ceiling, and underneath of stair-landings were available for acoustic materials. To remain within the set requirements, the following acoustic design was proposed:
• Walls and ceiling: biofiber-based acoustic coating directly sprayed on concrete surfaces.
• Underneath of stair-landings: biofiber-based acoustic sprayed on perforated gypsum board with an air gap of ~20 cm.
Acoustical measurements were conducted in the five floors of the building, in a total of five sound source–receiver combinations (five source and five receiver positions). The same source–receiver positions were used for the measurements before and after the installation of acoustic treatment.
There are two architecturally exactly similar staircases in the building of the School of Business but acoustic treatment was applied only to one of them. Building users can therefore experience extremely different sonic environments in two architecturally equal spaces.
2.2.3. Case Study III: Multifunctional Space in the Supreme Court of Finland
The Supreme Court of Finland is located in Helsinki in a building that dates back to 1816. The building has gone through several renovations, additions, and modernizations in the past years to provide functional premises for different uses. All renovations were always realized by preserving the character of the building and under the supervision of the National Board of Antiquities.
In case study III, a multifunctional premises of the Supreme Court, equipped with the AV system and lightly furnished, is acoustically treated. The room is used for meetings, educational events, and other event purposes. The room has parquet flooring and vaulted ceiling at the borders and is flat in the middle. The height of the hall reaches a maximum of 5.8 m in the middle of the room. The ceiling is covered with wood paneling, and it has six windows in the vaulted area. The side and front walls are painted plastered brick masonry. One half of the back wall is painted plastered brick masonry and the other half is painted plain gypsum board attached to a frame, thus leaving an air activity of 10 cm behind the gypsum board. The inferior area of all the walls is covered with wood paneling. There are two wooden doors in the back wall. The volume of the room is about 530 m3.
The users of the hall claimed that the main acoustic problem was poor speech intelligibility, even with the AV system due to excessive reverberation. The surfaces available for acoustic treatment were all the areas of the walls without wood paneling. Requirements for the acoustic treatment included keeping the exact same colors of the surfaces and the greatest grade of smootheness to minimize visual impact. Under these requirements, the following acoustic treatment was proposed:
• Front and side walls: biofiber-based acoustic coating directly sprayed on painted plastered brick masonry.
• Painted plastered brick masonry in the back wall: biofiber-based acoustic coating directly sprayed on hard surface.
• Plain gypsum paneling in the back wall: replacement of plain gypsum boards with perforated gypsum boards and biofiber-based acoustic coating sprayed on the perforated gypsum board (~90 cm air-gap behind the perforated boards).
Acoustical measurements before the installation of acoustic treatment were conducted using a total of three sound source–receiver combinations. After installation of acoustic materials, measurements were taken using the same sound source–receiver positions with an additional sound source position; thus, a total of six sound source–receiver combinations were used.
3. Results and Discussion
Table 3 presents a summary of the geometrical dimensions of the room, acoustically treated surface area between the parallel surfaces, and total surface area treated in the room, as well as average measured reverberation times and reverberation time predictions across the third-octave-bands from 125 to 5,000 Hz.
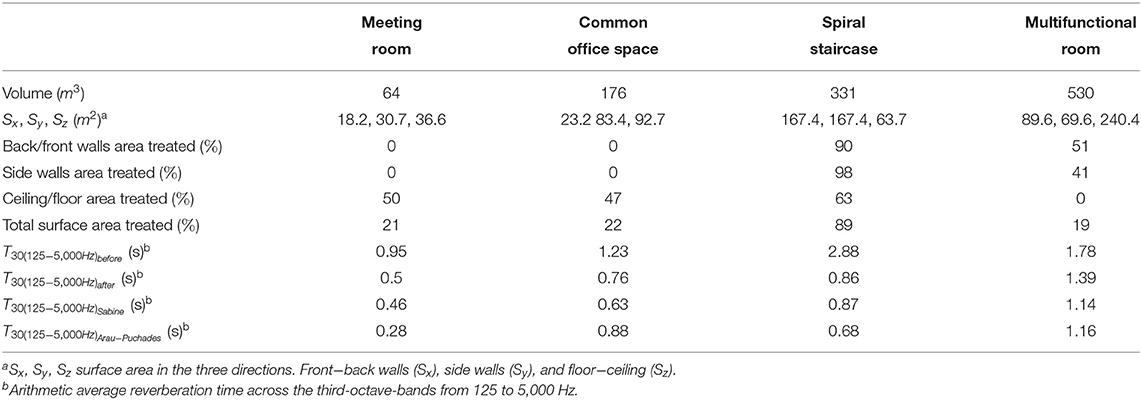
Table 3. Summary of room dimensions, acoustically treated surfaces, and average reverberation time before and after the installation of acoustic materials.
3.1. The Office of Co-founders
Employees of Co-founders Ltd. felt working in their office space unsuitable for efficient working due to poor acoustics. Space was not suitable for having more than one ongoing conversation between people or in phone simultaneously. Office is located along the one of the first “modern” shopping streets in Helsinki, and the owner of the estate wanted to preserve the original appearance of the space. Figure 3 presents photographs of the Co-founder office before (Figures 3A,C) and after (Figures 3B,D) the installation of the acoustic treatment. The seamlessly applied acoustic coating on glass wool panels glued to the ceiling resulted in a smooth and seamless structure that has the same color as that of the underlying painted concrete. Thus, the aesthetics of the room have been successfully preserved.
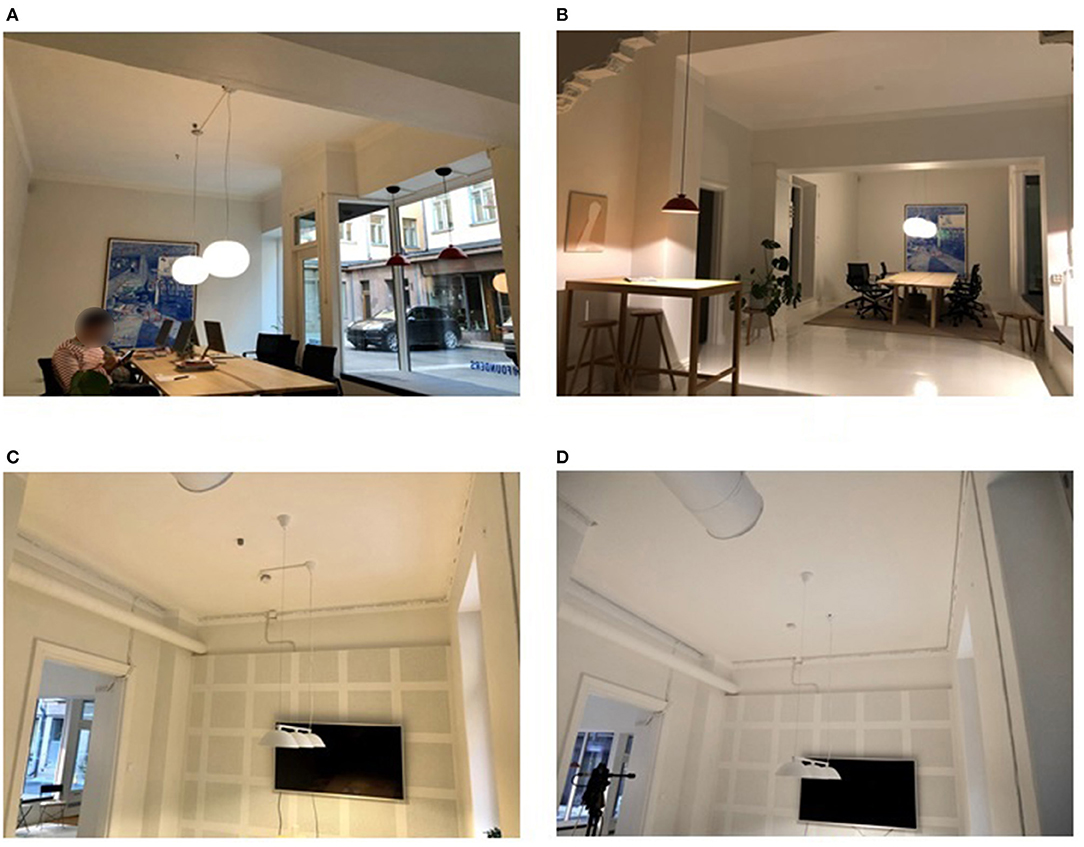
Figure 3. The common office space of Co-founders before (A) and after (B) the installation of acoustic materials; and the meeting of Co-founders before (C) and after (D) the installation of acoustic materials.
Acoustic measurements of T30 and C50 before and after the installation of acoustic treatment reflects remarkable improvement of acoustic conditions in both spaces, as shown in Figure 4. In the common office space, reverberation time decreased significantly, reaching values of 0.6 s at frequencies above 250 Hz, 0.8 s at 250 Hz, and 1.35 s at 125 Hz. The mild change in reverberation time at 125 Hz octave band results from the restriction of 4 cm of the thickness of the acoustic structure in the ceiling due to structural elements and electrical and lighting devices. In the case of the meeting room, the acoustic treatment reduced reverberation time to 0.4–0.6 s at frequencies above 125 Hz and 0.8 s at 125 Hz. Speech clarity is also enhanced in both rooms between 2 and 6 dB at frequencies above 125 Hz. Deviations between the individual measurements from the common office space may be caused by the fact that there were three different sound source positions among the four measurements, which together with the varying surface materials as well as furniture in the room may influence the ratio between early and late reflections. The acoustics of the meeting room belongs now to class A acoustic comfort according to the Finnish standard SFS-5907 (SFS 5907, 2002). Employees of Co-founders Ltd. expressed that their office felt cozy after the acoustic improvements and that no visible changes in the appearance of the rooms could be observed.
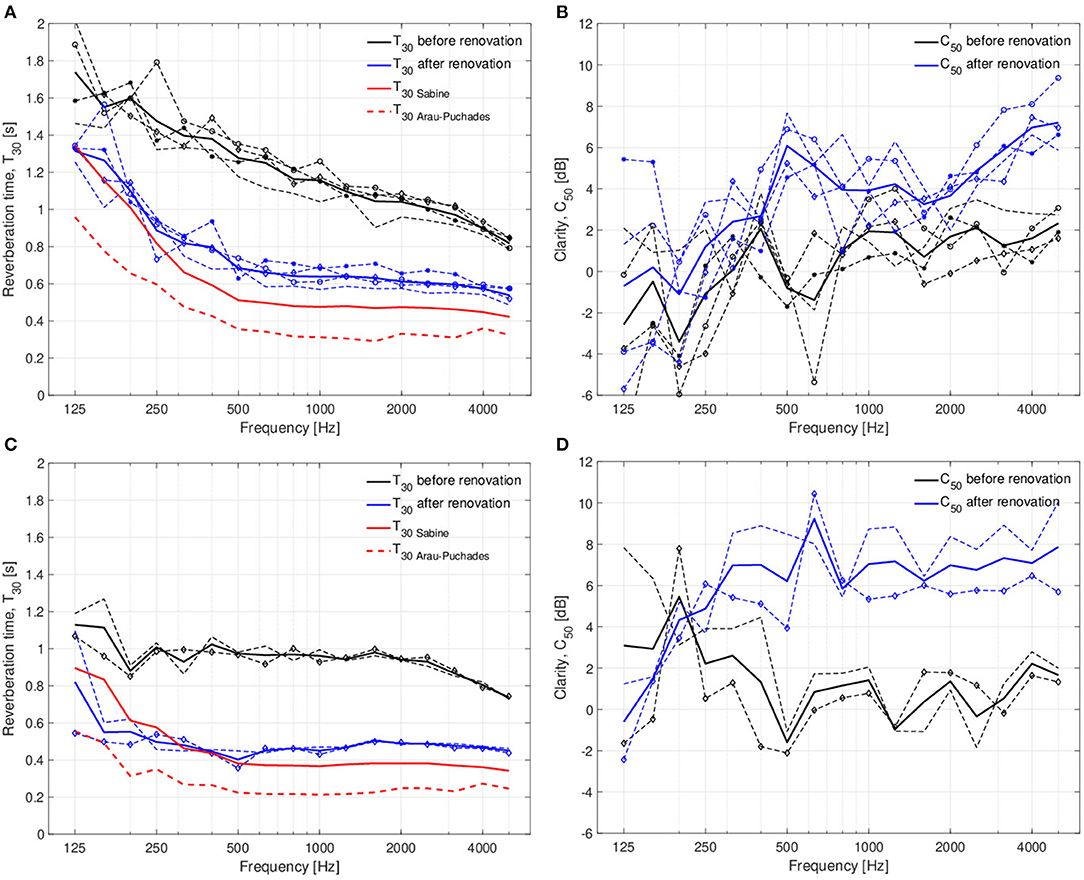
Figure 4. Measurement results of T30 and C50 before and after the installation of acoustic treatments in the Co-founder spaces. Common office space (A,B), and meeting room (C,D). Reverberation time predictions according to Sabine's and Arau-Puchades theories are included in (A).
Predicted reverberation time according to Sabine's equation shows good agreement with the reverberation time measured after the installation of acoustic treatment. The slight differences between the predicted and the measured reverberation time, [0–0.2] s, is probably related to the unfulfilled diffuse field conditions required by Sabine's theory, which is mainly attributed to the non-uniform distribution of sound absorbing materials in the room, as well as to the high average absorption coefficient of the room boundaries. Surprisingly, the prediction given by Arau-Puchades is considerably more inaccurate than Sabine's prediction in both rooms, although according to theory, Arau-Puchades equation should be more adequate for the sound field conditions given in these rooms. This result could be partially attributed to the sound absorption coefficients given to the concrete floor, which, if treated with some coating or painting, could indeed be a more reflective surface than that of concrete. The sound absorption coefficients of the floor surface are only used in the reverberation time predictions by Arau-Puchades theory. They are not needed in the predictions by Sabine's theory. In addition, as shown in Table 3, the total surface area of the acoustically treated rooms is 21% for the meeting room and 22% for the common office space. This value may be still too low to get the full benefit from Arau-Puchades equation.
3.2. Artwork by the Artist Group IC-98
Figure 5 presents photographs of the spiral staircases before and after the installation of acoustic materials. The acoustic coating sprayed on all the concrete walls, ceilings, and underneath of stair-landings has minimal visual impact but huge effects in the sonic environment of the space. Acoustic measurements of T30 and C50 were conducted in the five floors of the building, averaging the results in each floor. The results are shown in Figure 6. T30 after installation of acoustic treatment is significantly reduced, especially at mid-frequencies and high frequencies, where the biofiber-based acoustic coating sprayed on hard surface provides greater sound absorption. T30 is also reduced to some extent at frequencies below 500 Hz. This is attributed to the perforated gypsum panels sprayed with biofiber-based acoustic coating that are installed on the stair-landings. This significant difference in T30 at frequencies below and above 500 Hz causes an interesting effect for speech, as one could clearly hear immediate attenuation of consonant sounds and emphasis of vowel sounds. This is also reflected in Figure 6B, where it can be seen that speech clarity increases with frequency, with an improvement of ~15–20 dB at frequencies above 2,000 Hz.
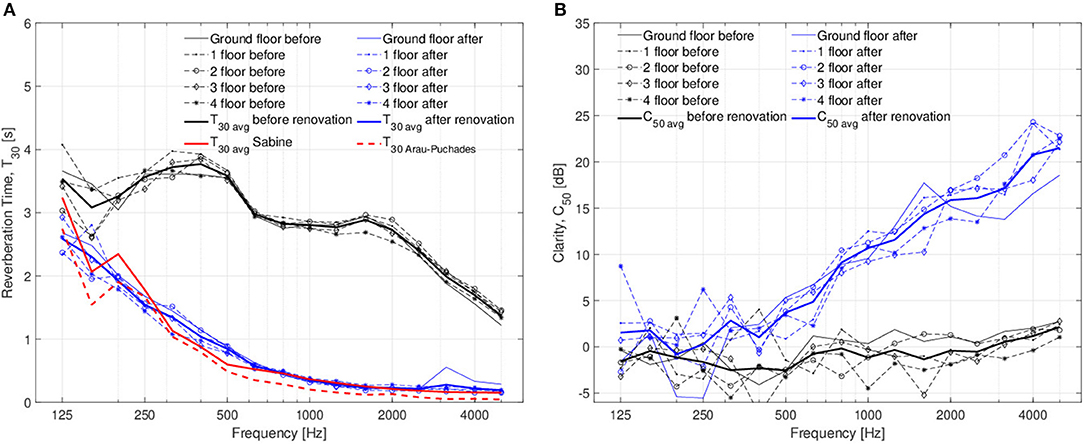
Figure 6. Measurement results of T30 (A) and C50 (B) before and after the installation of acoustic treatment in the spiral staircase. Reverberation time predictions according to Sabine's and Arau-Puchades theories are included in (A).
Both Sabine and Arau-Puchades reverberation time predictions showed high accuracy, with Sabine's theory presenting slightly more accurate predictions. The high accuracy of Sabine's theory in this space could be expected as the sound absorbing materials were uniformly distributed in all the surfaces of the space. However, such high average absorption coefficient of boundaries is not suitable for Sabine's theory (Sabine, 1900). On the contrary, high average absorption coefficients of room boundaries are beneficial for Arau-Puchades theory (Bistafa and Bradley, 2000).
Such an extreme use of acoustic materials in a space where long and loud reverberation is expected resulted in a baffling effect, according to designers of the art installation. Tinted acoustic coating conceals the sound absorbing surface in plain sight, which was one of the objectives.
3.3. Multifunctional Space in the Supreme Court of Finland
Figure 7 presents photographs of the multifunctional space before and after the installation of acoustic treatment. In this case, the acoustic coating was finished with care to make the surface as smooth as possible. As shown in the figures, only the upper part of the wall could be treated, as all other surfaces and the whole ceiling are made of wood.
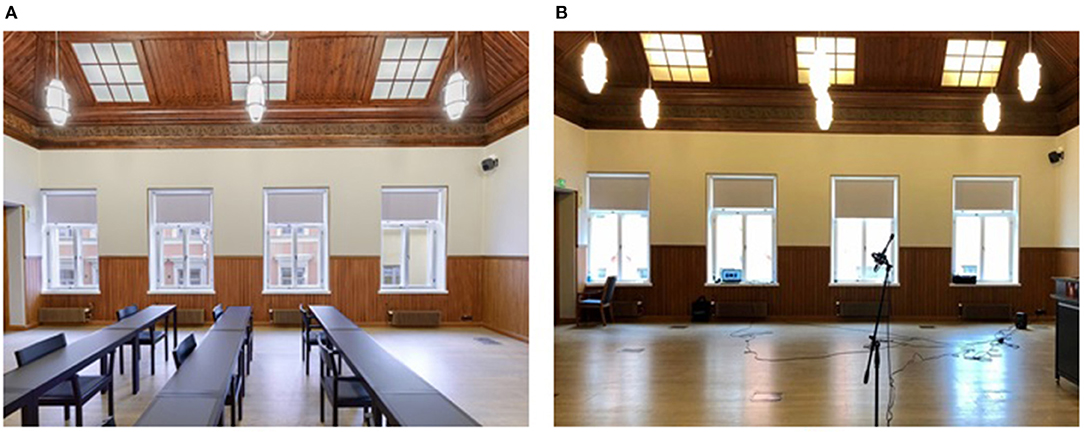
Figure 7. The historical room in the Supreme Court, before (A) and after (B) the acoustic treatment.
Results from acoustical measurements, T30 and C50, are shown in Figure 8. The average results from speech clarity measurements indicate an improvement of C50 of around 2–4 dB at frequencies above 250 Hz. However, individual clarity measurements show remarkable fluctuations at different frequencies, as well as significant deviations between measurements. On the contrary, such fluctuations are not reflected in reverberation time measurements. Such fluctuations could be attributed to the fact that only 19 % of the total surface area of the room was acoustically treated. The reverberation time could not be lowered as much as it would be required due to the small area that was possible to cover with acoustic coating. However, a deftly audible result was obtained. The client commented that they could not see the acoustical treatment at all; thus, visually, the renovation was successful. Moreover, the users of the room have reported that now the sound system of the room is improving the speech intelligibility as it did not help at all before the renovation.
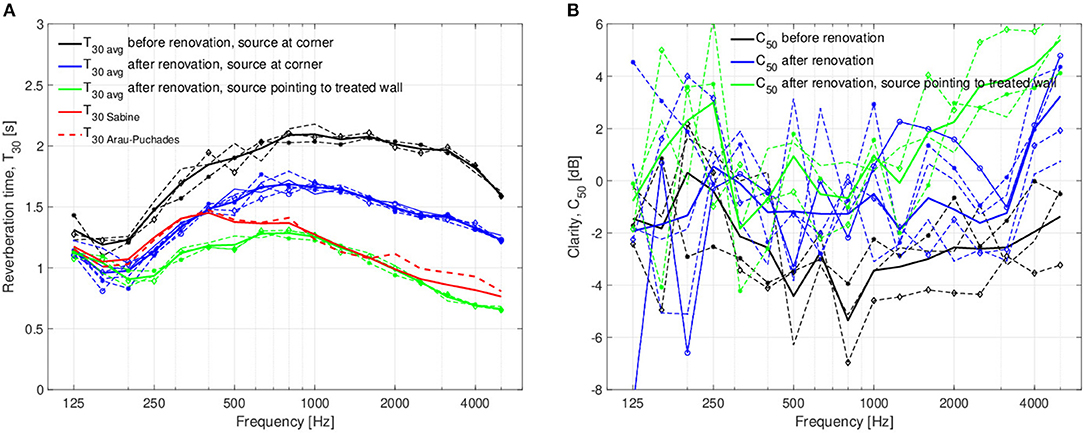
Figure 8. Measurement results of T30 (A) and C50 (B) before and after the installation of acoustic treatment in the historical room in the Supreme Court. Reverberation time predictions according to Sabine's and Arau-Puchades theories are included in (A).
The sound sources of the sound system are located on the acoustically treated walls, and they are pointing to the opposite treated walls. In an effort to understand the reasons for the remarkable enhancement on sound quality of the sound system in the hall, we measured T30 and C50 with the sound source pointing to the acoustically treated wall at a height of 1.7 m. The results are shown in Figure 8 in green color. The results show a reduction of over 0.5 s of T30 with respect to the reverberation time measurements taken with the sound source located in the corner. In this measurement, the directivity of the sound source is playing a crucial role, as most of the sound energy at mid-frequencies and high frequencies is directed toward the treated wall, resulting in greater energy dissipation of first reflections and more significant reduction of reverberation time. This second set of acoustical measurements with the source pointing to the treated wall is an excellent example on why, when using directive sound sources for acoustical measurements, the source has to be pointing to a corner with reflective surface, preferably a 3D corner, to distribute sound energy all around the room and approximate, to the extent possible, the response of omnidirectional sound sources.
In this room, Sabine's and Arau-Puchades theories provide similar predictions of reverberation time. Interestingly, at frequencies above 500 Hz, they are in good agreement with the reverberation time measured for the sound source pointing to the treated wall, whereas below 500 Hz, the predictions agree with the reverberation time measured for the source located in the corner. However, considering the adequate position of the sound source being the reflective corner, both prediction methods considerably overestimate reverberation time at mid and high frequencies. The failure of both prediction methods may be attributed to the irregular shape of the ceiling, as well as the large dimensions of the space.
3.4. Environmental Viewpoint
The biofiber-based acoustic coating used in all of the cases increased the bound atmospheric carbon of the buildings. The most efficient way to use such carbon negative material would be applying it directly to the existing structures, for example, the staircase walls in case II (biofiber-based acoustic coating). The carbon footprint of this structure would be about −980 CO2e g/m2. The use of thick and tinted acoustic coating decreases the need of other building materials, such as filler and paints, which also decreases the carbon footprint; however, this has not been taken into consideration in the calculations.
In many cases, however, the sound absorption properties of the coating are not enough and other acoustic materials required to be used as a base for coating. The carbon negative footprint of the coating decreases the carbon footprint of the whole acoustic solutions. By coating the 20 mm 95 kg/m3 glass wool, the carbon footprint of the structure is decreased by 16 % (5,000 CO2e g/m2). For coated 12.5 mm 760 kg/m3 gypsum, the same effect is merely 5 % (17,706 CO2e g/m2).
In individual spaces, the decrease of the carbon footprint by biofiber-based acoustic coating might seem insignificant, especially in presented case studies, as the rooms are quite small. However, if we consider a small office building with 15,000 m2 of acoustic surface, the reduction of the carbon footprint of such a building by the coating would be 14.7 tons CO2e, which equals to emissions of flying around the earth for about 2.5 times (Ciers et al., 2019).
4. Conclusions
This article presents three case studies in which the acoustical conditions were significantly improved with sustainable biofiber-based acoustic coatings. The acoustic structures consisted of acoustic coatings applied directly on an existing non-acoustic surface and combined structures with glass wool or perforated gypsum. Moreover, the acoustic treatment was implemented so that it is visually unnoticeable, which is often an important aspect, e.g., in historical buildings or in some special purpose rooms. The results of the case studies surprised the clients as they noticed the large change in acoustics but no visual changes.
The clients frequently demanded the solutions that have the minimal carbon footprint. In fact, European Union is aiming to be climate neutral by 2050 with net zero greenhouse gas emissions (GGE). This goal is unachievable merely by reducing the GGE, and industries need to find ways to produce carbon negative solutions for current products. Although acoustic products are responsible for merely a fraction of the total carbon footprint of the building, they play an important role in achieving zero carbon building. We foresee that bio-based acoustic absorption materials are one of the most important sustainable solutions for sound absorption. The presented case studies proved that acoustical treatment can be done while respecting the visual aesthetics with environment friendly implementation.
Data Availability Statement
The raw data supporting the conclusions of this article will be made available by the authors, without undue reservation.
Author Contributions
JC, TH, and MM: design of the renovations. JC: acoustical measurements. JC, TH, and TL: writing and editing the text. All authors contributed to the article and approved the submitted version.
Conflict of Interest
JC, TH, and MM were employed by company Lumir Oy.
The remaining author declares that the research was conducted in the absence of any commercial or financial relationships that could be construed as a potential conflict of interest.
Acknowledgments
The authors gratefully acknowledge support from Lumir Oy (Finland) and the cooperation of Lumir personnel Kari Kammiovirta and Hannes Hynninen. The members from artist group IC-98, Patrik Söderlund and Visa Suonpää, are also acknowledged for their excellent work and contribution to this study. The authors would like to express their gratitude to Co-Founder Ltd., the Supreme Court of Finland, and Aalto University for their permission to publish the results presented in this study.
Supplementary Material
The Supplementary Material for this article can be found online at: https://www.frontiersin.org/articles/10.3389/fbuil.2021.665332/full#supplementary-material
References
Arenas, J. P., and Asdrubali, F. (2018). “Eco-materials with noise reduction properties,” in Handbook of Ecomaterials, eds L. M. T. Martínez, O. V. Kharissova, and B. I. Kharisov (Cham: Springer), 3031–3056. doi: 10.1007/978-3-319-48281-1_137-1
Artalabs (2019). Arta Software. Available online at: www.artalabs.hr/a (accessed February 5, 2021).
Astolfi, A., Corrado, V., and Griginis, A. (2008). Comparison between measured and calculated parameters for the acoustical characterization of small classrooms. Appl. Acoust. 69, 966–976. doi: 10.1016/j.apacoust.2007.08.001
Berardi, U., and Iannace, G. (2015). Acoustic characterization of natural fibers for sound absorption applications. Build. Environ. 94, 840–852. doi: 10.1016/j.buildenv.2015.05.029
Berardi, U., and Iannace, G. (2017). Predicting the sound absorption of natural materials: best-fit inverse laws for the acoustic impedance and the propagation constant. Appl. Acoust. 115, 131–138. doi: 10.1016/j.apacoust.2016.08.012
Bistafa, S. R., and Bradley, J. S. (2000). Predicting reverberation times in a simulated classroom. J. Acoust. Soc. Am. 108, 1721–1731. doi: 10.1121/1.1310191
Ciers, J., Mandic, A., Toth, L. D., and Op't Veld, G. (2019). Carbon footprint of academic air travel: a case study in Switzerland. Sustainability 11:80. doi: 10.3390/su11010080
Cox, T., and d'Antonio, P. (2009). Acoustic Absorbers and Diffusers: Theory, Design and Application. London: Taylor and Francis group.
Cremer, L., and Müller, H. A. (1982). Principles and Applications of Room Acoustics, Vol. 1. Essex: Applied Science publishers.
Cucharero, J., Ceccherini, S., Maloney, T., Lokki, T., and Hänninen, T. (2021). Sound absorption properties of wood-based pulp fibre foams. Cellulose 28, 4267–4279. doi: 10.1007/s10570-021-03774-1
Eyring, C. F. (1930). Reverberation time in “dead” rooms. J. Acoust. Soc. Am. 1, 217–241. doi: 10.1121/1.1915175
Farina, A. (2000). “Simultaneous measurement of impulse response and distortion with a swept-sine technique,” in The 108th Audio Engineering Society Convention (Paris: Audio Engineering Society). paper 5093, 23 p.
Fitzroy, D. (1959). Reverberation formula which seems to be more accurate with nonuniform distribution of absorption. J. Acoust. Soc. Am. 31, 893–897. doi: 10.1121/1.1907814
Fouladi, M. H., Nor, M. J. M., Ayub, M., and Leman, Z. A. (2010). Utilization of coir fiber in multilayer acoustic absorption panel. Appl. Acoust. 71, 241–249. doi: 10.1016/j.apacoust.2009.09.003
Hodgson, M. (1996). When is diffuse-field theory applicable? Appl. Acoust. 49, 197–207. doi: 10.1016/S0003-682X(96)00010-2
Iannace, G., Ciaburro, G., Guerriero, L., and Trematerra, A. (2020). Use of cork sheets for room acoustic correction. J. Green Build. 15, 45–55. doi: 10.3992/1943-4618.15.2.45
Iannace, G., Trematerra, A., and Trematerra, P. (2013). Acoustic correction using green material in classrooms located in historical buildings. Acoust. Aust. 41, 213–218.
IPCC (2007). Climate Change 2007: The Physical Science Basis: Summary for Policymakers. Assessment Report of the Intergovernmental Panel on Climate Change. Geneva: IPCC.
ISO 3382-1 (2009). Measurement of Room Acoustic Parameters–Part 1: Performance of Spaces. Geneva: International Organization for Standardization.
ISO 354 (2003). Acoustics–Measurements of Sound Absorption in a Reverberation Room. Geneva: International Organization for Standardization.
Koizumi, T., Tsuijuchi, N., and Adachi, A. (2003). The development of sound absorbing materials using natural bamboo fibers and their acoustic properties. J. INCE Jpn. 2, 210–216. doi: 10.11372/souonseigyo1977.27.210
Kuttruff, H. (1994). “Sound decay in enclosures with non-diffuse sound field,” in Proceedings of the Wallace Clement Sabine Centennial Symposium (Cambridge MA), 5–7.
Lim, Z., Putra, A., Nor, M., and Yaakob, M. (2018). Sound absorption performance of natural Kenaf fibres. Appl. Acoust. 130, 107–114. doi: 10.1016/j.apacoust.2017.09.012
Millington, G. (1932). A modified formula for reverberation. J. Acoust. Soc. Am. 4, 69–82. doi: 10.1121/1.1915588
Navacerrada, M. A., Díaz, C., and Fernández, P. (2014). Characterization of a material based on short natural fique fibers. BioResources 9, 3480–3496. doi: 10.15376/biores.9.2.3480-3496
Oldham, D. J., Egan, C. A., and Cookson, R. D. (2011). Sustainable acoustic absorbers from the biomass. Appl. Acoust. 72, 350–363. doi: 10.1016/j.apacoust.2010.12.009
Passero, C. R. M., and Zannin, P. H. T. (2010). Statistical comparison of reverberation times measured by the integrated impulse response and interrupted noise methods, computationally simulated with Odeon software, and calculated by Sabine, Eyring and Arau-Puchades' formulas. Appl. Acoust. 71, 1204–1210. doi: 10.1016/j.apacoust.2010.07.003
Prawda, K., Schlecht, S. J., and Välimäki, V. (2020). “Evaluation of reverberation time models with variable acoustics,” in Proceedings of the 17th Sound and Music Computing Conference (Torino), 145–152.
Ruuska, A. (2013). Carbon footprint for building products. ECO2 data for materials and products with the focus on wooden building products. VTT Technol. 115 p. Available online at: https://cris.vtt.fi/en/publications/carbon-footprint-for-building-products-eco2-data-for-materials-an
Sabine, W. (1900). “Reverberation: Introduction”, Am. Arch. [Reprinted in W. C. Sabine, Collected Papers on Acoustics (London: Harvard University Press, 1922), pp. 3–68.]
SFS 5907 (2002). SFS 5907. Acoustic Classification of Spaces in Buildings. Helsinki: Finnish Standards Association SFS.
Sun, M., Wang, Y., Shi, L., and Klemeš, J. J. (2018). Uncovering energy use, carbon emissions and environmental burdens of pulp and paper industry: a systematic review and meta-analysis. Renew. Sustain. Energy Rev. 92, 823–833. doi: 10.1016/j.rser.2018.04.036
Keywords: acoustic coating, architectural acoustics, carbon sink, bio-based acoustic materials, acoustic measurements, acoustic design
Citation: Cucharero J, Hänninen T, Makkonen M and Lokki T (2021) Acoustic Coatings—A Discreet Way to Control Acoustic Environment. Front. Built Environ. 7:665332. doi: 10.3389/fbuil.2021.665332
Received: 07 February 2021; Accepted: 29 March 2021;
Published: 11 May 2021.
Edited by:
Arianna Astolfi, Politecnico di Torino, ItalyReviewed by:
Gino Iannace, University of Campania Luigi Vanvitelli, ItalyRosario Romano, University of Naples Federico II, Italy
Copyright © 2021 Cucharero, Hänninen, Makkonen and Lokki. This is an open-access article distributed under the terms of the Creative Commons Attribution License (CC BY). The use, distribution or reproduction in other forums is permitted, provided the original author(s) and the copyright owner(s) are credited and that the original publication in this journal is cited, in accordance with accepted academic practice. No use, distribution or reproduction is permitted which does not comply with these terms.
*Correspondence: Tuomas Hänninen, tuomas.hanninen@lumir.fi