- Structural Xploration Lab, Ecole Polytechnique Fédérale de Lausanne (EPFL), Fribourg, Switzerland
Amid escalating concerns over CO2 emissions, resource depletion, and waste generation in construction, reusing building elements from obsolete structures presents a sustainable solution. This paper critically reviews 21 procedures used in Europe and the United States (2001–2021) for identifying and evaluating reusable elements prior to transformation projects. Developed by various stakeholders with differing goals, these procedures propose diverse approaches. The study provides a comprehensive overview of their purposes, evaluation criteria, data requirements, and timeframes. Comparative analysis reveals subjectivity in data inputs and a lack of consensus on the comprehensiveness required for effective evaluation. To move from recycling to reuse, the criteria must expand to include projective values, deconstruction processes, and second-use planning. These aspects are essential for assessing the availability, deconstruction, and reuse potential of building elements. The findings offer key insights for developing standardized, adaptable, and automatable assessment procedures that can facilitate efficient and effective reuse practices in future projects.
1 Introduction
1.1 Context: from recycling towards reuse
The systematic reuse of components originating from obsolete buildings reduces pressure on landfill sites and the need to produce new components, which is generally expected to decrease greenhouse gas emissions and pressure on finite resources (Baker-Brown, 2019; McDonough and Braungart, 2010; Ghyoot et al., 2018; Nakamura and Halada, 2015). Nevertheless, reuse rates are still low today - Pristerà et al. (2024) estimates European rates below 15% when reuse potential can be up to 80% - and most current policies focus on limiting the impact of building operations, overlooking the significant savings that a more circular construction sector could bring (Mata et al., 2020).
When aiming at increasing material circularity, most regulatory frameworks – with the notable exception of the European Commission’s Waste Framework Directive 2008/98/EC that prioritizes preparing for re-use as the second-best option after waste prevention (European Parliament, 2008) – still favor recycling processes and better management of demolition waste over the reuse of whole components (Defra, 2019). While beneficial in diverting waste from landfills, recycling is energy-intensive and imposes significant environmental burdens regarding greenhouse gas emissions and other impacts (Addis, 2006). Reusing materials, in contrast, is generally associated with minimal material reprocessing, lower energy demands, and greenhouse gas emissions (Gorgolewski et al., 2008). For instance, the environmental impact of using new steel sections with 60% recycled content is still 25 times higher than reusing equivalent reclaimed steel sections (WRAP, 2008). Reusing reclaimed structural steel or timber sections can reduce environmental impacts by 96% and 83%, respectively (Lazarus, 2003; Brütting et al., 2019).
When considering a conventional life-cycle of a building (Figure 1), the generation of elements for reuse is not natural. For this to be achieved, an additional operation must be implemented following the building’s obsolescence, as this condition does not inherently signify the end-of-life of its components. Consequently, enabling reuse necessitates deconstruction rather than conventional demolition. When it is not feasible to adapt and reuse an entire building, the focus should thus shift to the reuse of individual elements rather than recycling. However, in practice, the traditional demolition methods currently lead to the creation of waste, which, in the best cases, is subsequently recycled. As shown in Figure 1, introducing deconstruction into this scenario alters the sequence. The introduction of the deconstruction process allows Elements and Reuse consideration. With Demolition, the actual system passes from building to waste and matter. The only way to follow policies’ incentives towards reuse is by introducing deconstruction.
Paradoxically, despite the proliferation of overarching circular policies (Ellen MacArthur Foundation, 2013; European Commission, 2015), a notable dearth of specific assessments and frameworks designed to guide practitioners in the practical implementation of deconstruction and reuse initiatives exists. This underscores a critical gap in the current understanding and application of sustainable construction methodologies. It calls for a more in-depth exploration of the existing and various methods developed by practitioners to bridge this gap between policy formulation and implementation in the field.
1.2 Background: context and goals of reuse potential assessments
Once a building or a part of a building is deemed obsolete, rather than considering its end-of-use solely, the owner generally organizes its end-of-life by contracting a demolition company that aims to dispose of the considered waste as profitably as possible. Due to economic reasons and an inertia toward a change of habits, current demolition practices generally favor the quick crushing, melting, shredding, or burning of the building pieces. In this context, reuse potential assessments are meant to be performed before demolition happens in order to replace it with a more careful deconstruction and storage of the building components that are worth reselling and reusing. Currently, there is no specific profession dedicated to this task. Unlike the well-established assessments of thermal and acoustic performance and or related to the compliance of buildings and their systems, expertise in deconstruction and reuse is generally gained from within the practice and not provided by educational programs. Existing tools, methodologies, and training programs support performance assessments, but similar resources for deconstruction and reuse are lacking.
Current regulations touching post-obsolescence assessments generally focus on how to sort the waste generated when buildings are demolished, on the basis of the composition of materials alone, with the aim of efficiently supplying the various recycling channels. Criteria for evaluating a recycling potential, however, differ fundamentally from those for reuse potential. As depicted in the previous section, political measures fail to provide guidelines or methods for implementing their ambitions. The scientific literature is therefore explored in search of theoretical contributions that will enable proper, generalizable assessments of deconstruction and reuse potential.
1.3 State-of-the-art: theoretical groundwork for a comprehensive procedure
Research has been conducted to understand the reuse of building elements at the end-of-life of existing buildings and to evaluate their potentiality in new constructions (Rakhshan et al., 2020). Various approaches have been explored. Previous studies have focused on identifying the mechanical and dimensional properties of the components, using non-destructive testing techniques (Devènes et al., 2023), and some even develop automated recognition algorithms (Cavalli et al., 2016; Fujita and Kuki, 2016; Yeung et al., 2015). Other research addressed the reuse potential of elements and sought to identify barriers and drivers in processes, networking environments, stakeholder chains, or regulatory context (Gorgolewski, 2008; Rakhshan et al., 2020; Condotta and Zatta, 2021; Hobbs and Adams, 2017; Jabeen, 2020). For instance, TG39 et al. (2000), a comprehensive collection of global scientific literature summarizes the deconstruction and reuse policies implemented in various countries worldwide. Some studies explore ways to attribute values, such as environmental or recycling aspects (Saghafi and Teshnizi, 2011; De Wolf et al., 2020). Digital material banks are also developed to stock data on existing components and material properties promoting future reuse (Cai and Waldmann, 2019; Durmisevic et al., 2017; Arora et al., 2020; Akanbi et al., 2019; Laefer and Manke, 2008; Heisel et al., 2022).
To summarize, the existing scientific literature concerning reuse practice has mainly focused on two areas. The first area concerns the broader aspects of reuse, such as the economic implications of deconstruction, identification of barriers and drivers, et cetera (Gorgolewski, 2008; Rakhshan et al., 2020; Condotta and Zatta, 2021). The second area explores specific technical facets, such as generating information, tools for data collection, or establishing values being technical, dimensional, environmental, circular … (Durmisevic et al., 2017; Arora et al., 2020; Heisel et al., 2022; Fujita and Kuki, 2016; Yeung et al., 2015). However, a critical research gap remains in the examination of the current implementation of deconstruction and reuse practices. How, at a practical level, are deconstruction and reuse achieved? Surprisingly, the field lacks insights into the procedures or methodologies currently applied.
Aside from the scientific literature, Guy, (2006) describes, analyzes, and develops methodologies for deconstructing American buildings safely. Interreg, (2019) provides a synthesis of elements-reuse-assessment used by cities and architects in Europe. No study about deconstruction methodology or assessment procedure is found outside these two documents. A comprehensive investigation into existing operational practices, methods, tools, subsequent analysis, and the identification of areas for enhancement, essential for advancing the methodologies of deconstruction and reuse, are notably absent, especially in the academic discourse.
1.4 Objectives
The objective of this article is to understand better the needs and requirements to efficiently plan the deconstruction of an obsolete building in view of the constitution of a stock and further reuse of its components, which is revealed as a missing sequence in Section 1.1 and illustrated in Figure 1. To do so, the study generates an informed critique of the existing audits, from maintenance to waste assessments, as illustrated in Figure 2, highlighting their strengths and weaknesses and suggesting areas for improvement with a particular focus on deconstruction. This is done by analyzing and comparing the objectives of the collected procedures concerning elements’ reclamation, the nature of data typically collected, and the stakeholders involved in initiating, conducting, and exploiting such procedures. The study investigates specific facets such as conditions favoring deconstruction over demolition, the criteria employed to evaluate the potential for reuse, and whether they align with broader procedures or are unique to the domain of deconstruction and reuse.
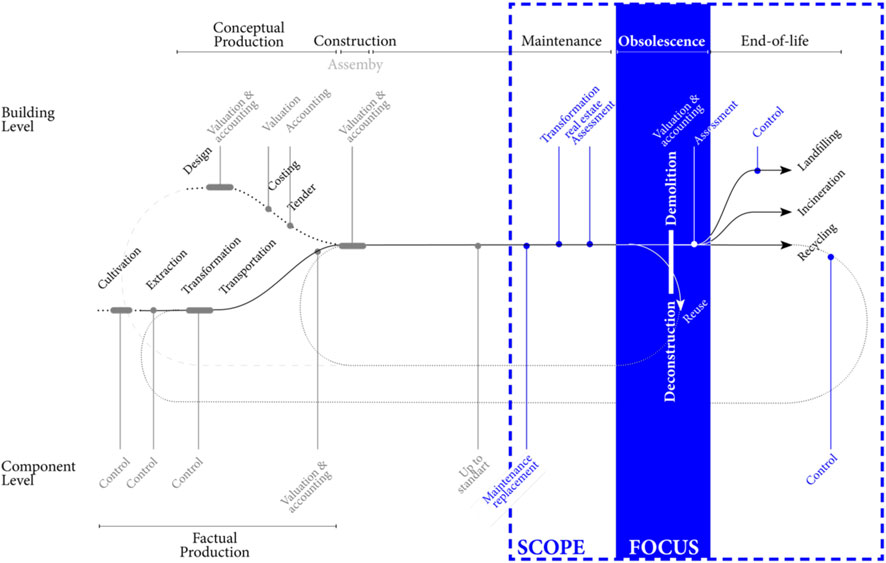
Figure 2. Obsolescence contextualized within the overall building construction industry framework, from production to the end-of-life.
1.5 Scope
As shown in Figure 2, each building element is assessed and controlled several times during its lifespan, either individually or as part of the building. This study focuses on improving or introducing deconstruction goals within the obsolescence phase, represented in blue. The scope of the study goes beyond the obsolescence phase to encompass maintenance and end-of-life procedures while including considerations of various scales, from the building system to the element. Abandoned or damaged buildings following fire, flooding, or explosions are not included. Toxicity and thermal performance assessments are specific evaluations assumed to be completed beforehand. Criteria concerning suspicion of toxicity of an element are considered, but toxicity audits with criteria requiring specific technical equipment are excluded. The study disregards the assessment of elements designed for disassembly, focusing on current empirical assessments, nor delves into Life Cycle Assessment (LCA), considering they help to convince or compare, not to act. While the study includes recycling or waste sorting assessments, the analysis focuses solely on data relevant to deconstruction or reuse potential. Also, all existing assessment procedures reviewed in this study assume the non-existence of a pre-existing Building Information Modeling (BIM) representation.
1.6 Limitations
One of the limitations of this study is that the corpus of reclamation audits is predominantly based on developed-country models, which may limit the generalizability of the findings to regions with different socio-economic contexts or building practices. Future research should aim to include a more diverse range of countries to ensure a broader representation of global practices. Additionally, the study is limited to English, French, and German texts. This language restriction may have excluded relevant information published in other languages, leading to potential language bias in the analysis. Including texts in a wider range of languages would enhance the cross-cultural applicability of the research.
Another limitation is the corpus’ reliance on text-based sources. By focusing solely on written materials, important unwritten practices or tacit knowledge that could influence reuse potential assessments may be missed. Incorporating non-textual sources, such as interviews, case studies, or field observations, would provide a more comprehensive understanding of the subject. Furthermore, the corpus is collected solely from Internet sources. Relying on freely available online content may have introduced sampling biases, as not all relevant materials may be easily accessible online.
1.7 Paper organization
Section 1.1 highlights current efforts toward circular practices in the construction sector, primarily focusing on recycling. This study posits the necessity of incorporating deconstruction processes to alter obsolescence phases, thereby transitioning from recycling and landfilling towards reuse, illustrated in Figure 1. However, there is a critical lack of guidelines in policy formulations and gaps in scientific considerations – contextual or technical specific, as seen in Section 1.2. This leads to the study (Section 4) of in-practice workflows (Section 3) to improve/introduce deconstruction consideration (Section 5.1) within the obsolescence phase (Section 1).
Figure 3 summarizes the paper’s organization. First, Section 3 offers a detailed description of the corpus. It lays the foundation for understanding the various procedures examined in this study. Section 2 outlines the overall method. To process a multidimensional comparison between all procedures composing the corpus, a graph embodying defined parameters synthesizes each procedure. Parameters – defining inputs, outputs, and processes – are created based on constituents from the assessments of the corpus, illustrated in Figure 4. Section 4.1: Settings defines the constituents of the parameters. These constituents are sorted, compared, and analyzed to identify similarities or contradictions within parameters. Section 4.2 conducts a detailed comparison of parameters across all procedures. Section 5.1 evaluates the identified gaps and strengths, suggesting potential enhancements. Finally, Section 5.2 discusses overarching considerations regarding reuse assessments. It provides a broader context for the study’s findings and recommendations.
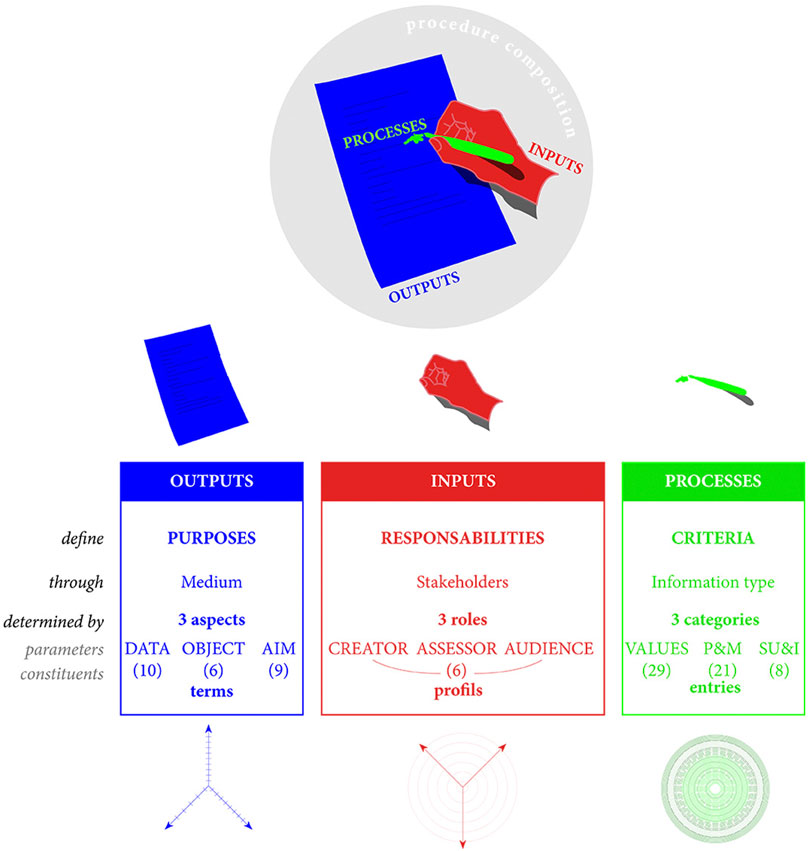
Figure 4. Methodology. Procedures decomposition leads to graphical representation and vocabulary used in this paper.
Given the extensive use of specialized and overlapping terminology throughout the paper, a glossary of key terms is provided in the 9. Supplementary Material to support clarity and consistency.
2 Methodology
2.1 Overview
First, an internet tree search is conducted to compile the corpus, as summarized in Figure 5 Since search engine queries rarely yield direct references, initial searches focus on mentions in guides, reports, or text collections. The corpus is considered complete once all newly found evaluations match a pre-selected equivalent.
Then, a comprehensive multidimensional comparative study is developed to compare inputs, outputs, and processes of audit procedures. This study posits that the outputs, inputs, and processes define purposes, responsibilities, and decision criteria. As shown in Figure 4, each of those components is embedded by the chosen medium, the Stakeholders involved, and the information types used. The outputs are determined by three aspects: DATA, OBJECT, and AIM. The inputs correspond to three roles: CREATOR, ASSESSOR, and AUDIENCE. Processes contain three categories of criteria: VALUES, Processes & Management (P&M), and Second Use & Implementation (SU&I). These are parameters used to create synthesis graphs. This decomposition and graphic expression allow for a concurrent comparative analysis to identify commonalities, differences, and gaps in current practices. Comparisons are elaborated at diverse levels: first, constituents and then parameters within each component corresponding to Section 4.1; second, constituents and parameters of different components to finish with overall procedure comparisons in Section 4.2.
To finish, the first author of this paper, a heritage specialist and condominium architect with over 6 years of experience, has inspected numerous existing buildings in Paris, conducted audits, and supervised maintenance and transformation projects for around 100 buildings. Based on her extensive practical experience and thorough examination of the procedures included in the corpus, she identified the procedures as incomplete. Her in-depth understanding of existing buildings and her onsite expertise, combined with the descriptions and recommendations in the deconstruction guides, allowed her to critically analyze the study’s data and highlight its deficiencies. The second author, an engineer with significant site experience, concurred with the first author’s observations, affirming the validity of the points raised.
2.1.1 Corpus constitution
The constitution of the corpus was executed in 2021. As drawn in Figure 5, three steps have been necessary to achieve it. First, a search within the scientific literature, namely Google Scholar, Scopus, and Web of Science, proved fruitless. The search uses the keywords “deconstruct*,” “polic*” and “build*.” Second, an extended search on mainstream engines, namely, Google and Ecosia, procures four references. To refine the results, the search query has been expanded to include the terms “assessment” or “diagnos*” and later “ordinance.” Third, in-depth research within the references leads to targeted querying of legislative texts or tools’ names, reading other texts from quoted authors, or exploring governmental websites and regulations of mentioned cities/countries.
2.1.2 Comparative analysis framework
The study involves observing the corpus’ procedures medium, stakeholders, and information types that determine inputs, outputs, and processes. Procedure constituents are grouped into parameters. To allow concurrent comparisons, graphs are created for purposes (outputs), responsibilities (inputs), and criteria (processes), constituents of procedures. Taxonomies are established to sort the words used in titles as well as the stakeholders’ roles. Each constituents’s graph is represented in Figure 4. The following paragraphs give detailed descriptions of each constituent.
Assessment results are supposed to be determined by the purpose for which a procedure is designed and implemented. The wording of the procedure titles is used to define their purposes according to three aspects: DATA, AIM, and OBJECT. DATA informs the quantity of required information and the complexity of the procedure. AIM defines the goal of the assessment, whether for waste management or reuse planning. Finally, OBJECT is the focus of the procedure, which can be waste, material, or elements. As seen in Figure 4, each of these aspects is represented by a blue axis in the spider graph. Using the corpus, a list of possible wording is established and hierarchized for every aspect. DATA is composed of Worksheet, Information, Form, Summary, Plan, Inventory, Characterization, Audit, Diagnostic, and Assessment; AIM of Reduct*, Divers*, Recycl*, Renovat*/rehabilitat*, Deconstruct*, Availab*, Salvag*, Resourc*, Reus* (the * include all declination in -ing or -tion or -e et cetera.); OBJECT of Waste, Debris, Construction and Demolition (C&D), Deposit, Material, and Element. The words most related to reuse are placed further from the center of the graph. Section 4.1.1 gives a detailed definition of each wording.
The responsibilities are defined by the stakeholders responsible for different phases of the procedure. Three key roles are defined: AUDIENCE, CREATOR, and ASSESSOR. These roles play a crucial part in shaping the procedure and exert influence on its development and implementation. The AUDIENCE determines the specific needs and objectives for which the procedure is designed and later uses the information gathered through the procedure. Its aims and interests influence the aspects that are prioritized or denied in the procedure, which consequently shapes the choice of input data. The CREATOR, based on the needs defined by the AUDIENCE, develops the procedure. The creator is responsible for designing the framework, including data capture, categorization, and output values type. The more precise and quantifiable the definition of needs, the more obvious and specific the input data will be. Finally, the ASSESSOR implements the procedure in actual projects to collect and generate the necessary data that satisfies the AUDIENCE’s needs. In Figure 4, each of these parameters is represented by a red axis in the spider graph. Using the corpus, a list of possible stakeholders - Owners, Contractors, Architects/Engineers, Scientists, Local authorities, and National authorities - is created and hierarchized along the axis according to their level of involvement and impact, ranging from individual to collective influence.
The criteria are defined by the information type required in the assessment procedure. After compiling entries from the procedures of the corpus, they are grouped into common themes. These entries are further classified as either descriptive - focusing on the existing building and its components - or projective - related to the deconstruction project and the future use of reclaimed elements. For each illustration of procedures, entries are visually represented by green circles in the spider graph. A more detailed analysis of the entries then distinguishes three categories of entries: Values, Processes and Management (P&M), and Second Use and Implementation (SU&I), represented with different type lines in the graphs. The Values category is composed of data valuing, rating, and measuring elements. The P&M category examines the practical aspects of deconstruction and reuse, including the logistics, planning, and management strategies required to effectively salvage elements. Finally, the SU&I evaluates the feasibility and practical application of reusing salvaged materials in new construction projects.
These representations of purpose, responsibility, and criteria are finally overlaid, providing a comprehensive view of all parameters and constituents. Additional details such as localization (United States/EU) and effective year are also included, enabling a simultaneous understanding across multiple dimensions.
2.2 Corpus creation
First, a detailed research description is proposed in the next chapter and summarized in Figure 5. Connections between the various readings that led to more in-depth research in a targeted country are described and represented. Then, the selected corpus is described, highlighting the breadth of considerations included to qualify the obsolescence phases. This search yielded a concise selection of four documents that directly align with the topic of this research, as represented in Figure 5. Two texts, Crowther (2000) and Schultmann and Rentz (2000), are encompassed within the collection of TG39 et al. (2000), already mentioned in Section 1.3. The fourth text, Chini & Bruening, (2003), primarily focuses on policies related to the United States. Through the extended search, the Environmental Protection Agency (EPA) website emerged as a highly recommended resource for this study, providing a multitude of guides and pertinent information. The US Green Building Council report (USGBC, 2019) sourced from the EPA website offered insightful details pertaining to the cities of Portland and San Antonio, while also referring to the document published by the National Association of Home Builders (NAHB, 2001), which sheds light on the Milwaukee ordinance. The Milwaukee ordinance is one of the numerous legislations referenced in the Delta collection (DELTA, 2018). The examination of the Delta collection led to the meticulous selection of more references. Additionally, the comprehensive guide authored by Brad Guy (Guy, 2006) emerged as an essential inclusion in this study. Moreover, the comprehensive synthesis provided by Interreg, (2019) encompassed a range of cities and methodologies.
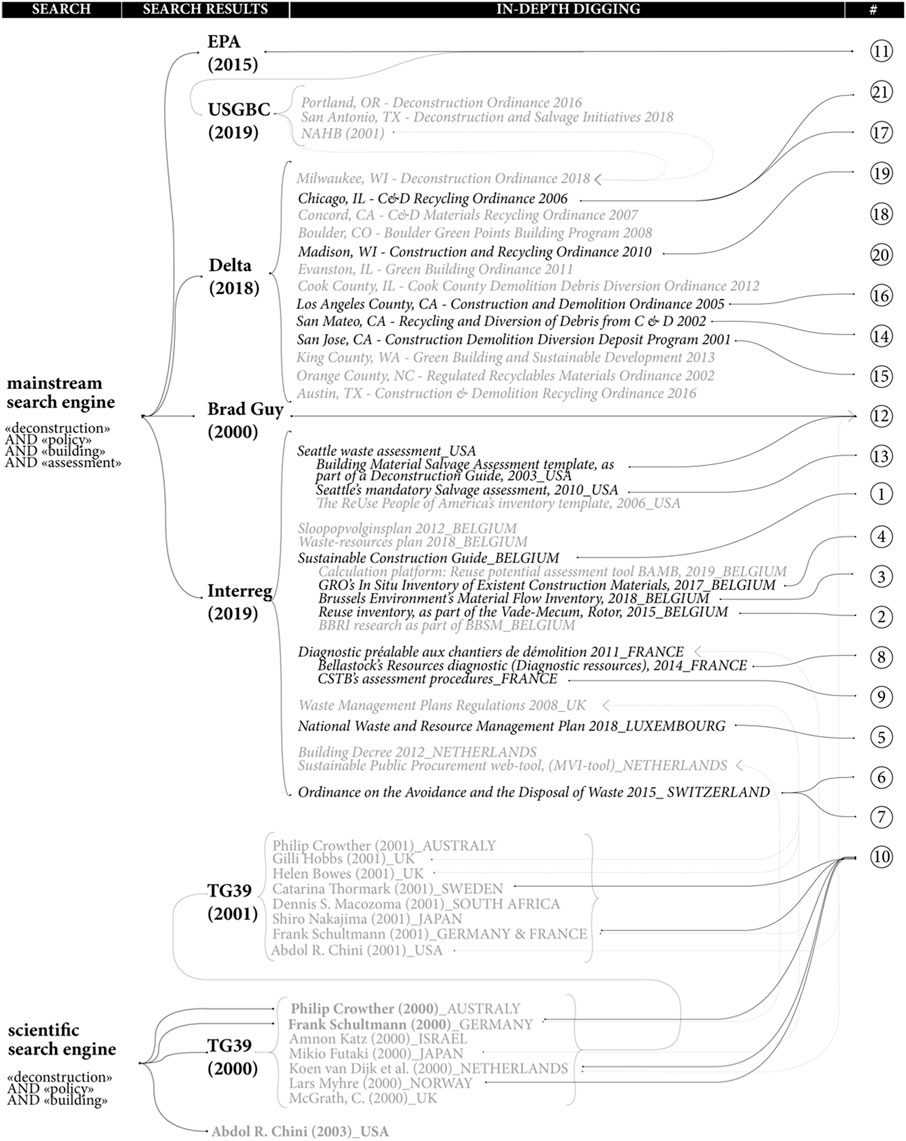
Figure 5. Corpus constitution. Detailed tree-search. Numbers on the rights are used to name the procedures of the Corpus. A detailed description is available in Supplementary Table SA1.
The corpus’s procedures are deliberately chosen to cover a broad spectrum of purposes as shown in Figure 6. The corpus, therefore, takes into account the assessment of components integrated into the building, the feasibility of deconstruction, the potential for reuse of elements and waste, and waste facilities certification requirements.
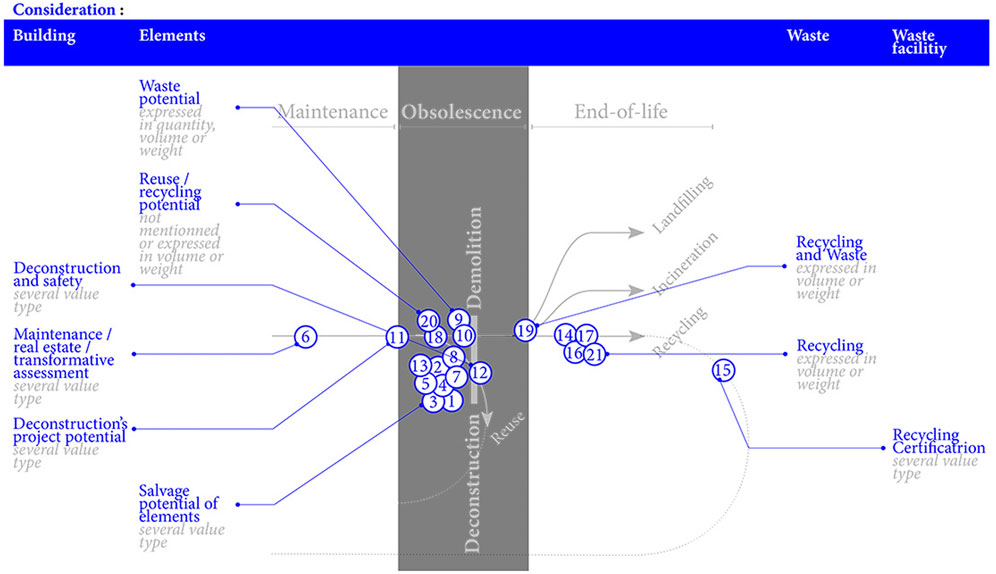
Figure 6. Placement of the selected procedures constituting the Corpus within the scope presented in Figure 2. The selection encompasses various considerations (building, elements, waste, waste facility) with diverse objectives to maximize the collection of information.
Some procedures (in grey in Figure 5) mentioned in the important references (USGBC, 2019; NAHB, 2001; DELTA, 2018; Interreg, 2019) were not available to the authors by the time of this study and, therefore, discarded, such as The Reuse People of America’s inventory template, which was made available on 2nd May 2024, within the Palo Alto Municipal Code Section 5.2.4 (City of Palo Alto, 2024). Other procedures were discarded when considered very similar to the ones already included in the corpus. For example, Boulder is proposing a procedure judged similar to the P17 (City of Chicago, 2007a) only to acquire a Green Building certificate. The reuse potential assessment tool from BAMB (Rose and Stegemann, 2018) evaluates the reversibility of assemblage designs. This assessment tool has been considered out of scope as this study focuses on existing building deconstruction and the assessment of a stock of reusable elements. The BBSM tool (Gobbo et al., 2019) assesses renovation strategies based on only three types of buildings, and this is considered out of the scope of this study.
3 Corpus constitution
3.1 Corpus description
Supplementary Table SA1, describes and numbers each procedure. This study pays particular attention to the wording of the titles (see Section 4.1.1), so a specific column is composed in Supplementary Table SA1 to make this feature obvious. Additionally, a detailed description of each procedure is made, including the number of themes and criteria (see Section 4.1.3 for terminologies). 21 procedures compose the corpus, encompassing a selection of 326 criteria. Procedures composing the corpus, referenced as Homegrade (2019), is P1, ROTOR (2015) P2, Interreg (2019) encompasses procedure P3 made by Brussels Environment, procedure P4 made by GRO and procedure P8 from Bellastock, LIST (2018) is P5, Flourentzou et al. (2001) has developed EPIQR+ named P6, Devènes et al. (2024) P7, the procedure developed by ADEME (2021) is P9, the one of the European Commission (2018) is P10, EPA (2015) P11, Guy et al. (2003) P12, the procedure of the Seattle Department of Construction and Inspections (2014) is P13, City of San Mateo, (2017) P14, City of San José (2001) P15, Los Angeles County (2005) P16, City of Chicago (2007) P17, Fitchburg public works, (2015) P18, City of Madison (2010) P19, City of Shoreline (2018) P20 and the procedure of the City of Chicago (2007b) is P21.
Existing procedures from France, Belgium, Switzerland, Luxembourg, as well as Washington, California, Wisconsin, and Illinois in the United States compose the corpus. Additionally, the corpus includes procedures that concern the European Union and the United States. Ten are of European origin (P1 to P10), while eleven are American (P11 to P21). These procedures were developed between 2001 and 2021, with the majority occurring between 2015 and 2021. The date is the year when the procedure was initially made available and not the year of updates or paper diffusion. Two of them are privately used by architects or scientists (P6,P8), and the nineteen others are freely accessible to anyone.
As explained in Section 1.4 and Section 1.5 and represented in Figure 2, efforts were made to collect procedures within the obsolescence phases with an extended scope to maintenance and end-of-life. Figure 6 is based on Figure 2, complete with the placement of the corpus procedures within the scope.
As shown in Figure 6, Procedure P6 concerns renovations and maintenance of existing buildings in the Maintenance phase with consideration to the entire Building. Procedures P11 and P12 consider the entire Building as well, P11 at an early stage, to determine the deconstruction’s project potential, while P12 has concerns for operational safety during deconstruction. Procedures P18 and P20 determine if elements must rather be reused, recycled, or wasted, while procedures P1-5, P7-8, and P13 assess the salvage potential of elements, and procedures P9 and P10 already consider elements as waste prior to demolition. Procedure P19 determines the best end-of-life path for waste. Procedures P14, P16-17, and P21 concern waste “reuse,” leading to the recycling processes. Finally, procedure P15 concerns the waste facilities certification requirements, informing with overall waste consideration.
Within the constituted corpus, some assessments encompass diverse objectives. Only criteria concerning second use (the assessment of reuse potential or usefulness for the deconstruction processes) have been selected for this study. All criteria concerning the end-of-life of material (recycling, landfilling, waste management …, etc.) or fine attribution have been ignored.
4 Comparative analysis
4.1 Settings
Figure 7 establishes three graphical frameworks defining inputs, outputs, and processes of procedures named purposes, responsibilities, and criteria, respectively. The purposes axis is determined by procedure title terminologies. The responsibilities axis is based on stakeholder roles and implications. Parameters concerning the criteria are represented by circles. Other reading levels are offered, distinguishing description and projection-based criteria with color code, and themes, in which entries are grouped, are written on top of the circles.
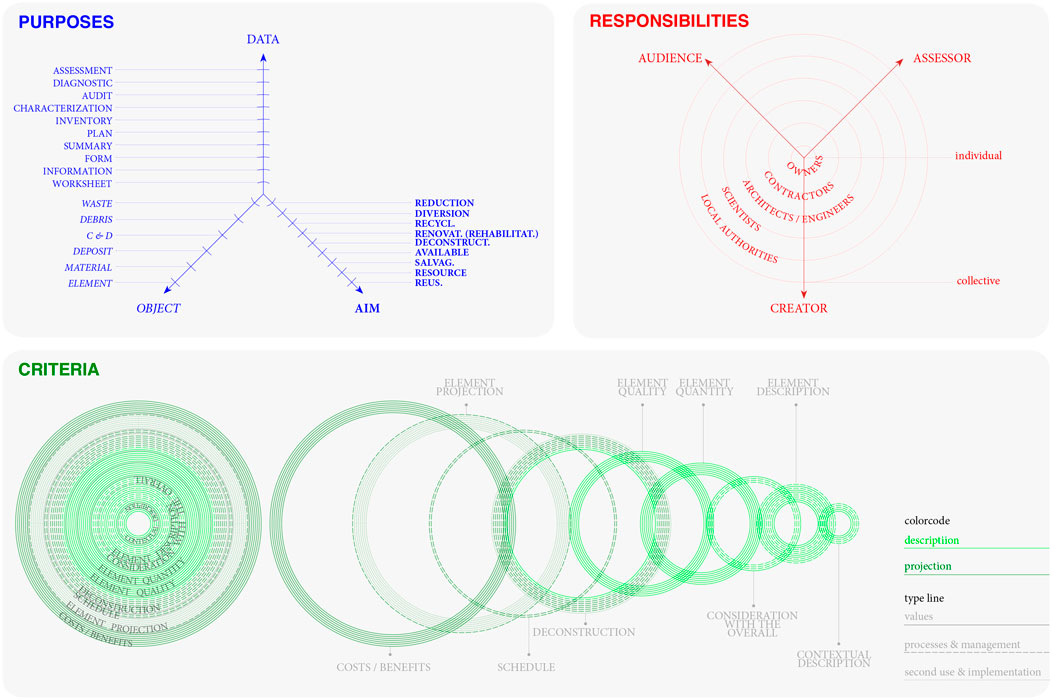
Figure 7. Settings: 3 procedure characteristics: purposes, responsibilities, and criteria defining inputs, outputs, and processes, respectively.
4.1.1 Purposes
Terms forming the analytic framework are extracted from the corpus procedures titles. This chapter presents the terminologies and corresponding procedures, focusing exclusively on purposes.
As introduced in Section 2.1.2, the terminologies used in the procedure titles are supposed to provide information on the purpose and expected results. Purpose-defining terms are classified along 3 axes: DATA, AIM, and OBJECT, identified in blue in Figures 7, 10.
The terms considered to qualify DATA in the procedures making up the corpus are: Worksheet P21, Information P14, Form P8, P12, P15, P17-19, Summary P16, Plan P5, P14, P18, P20, Inventory P1, P3-4, P8, Characterization P2, P8, Audit P9, Diagnostic P6, P10 and Assessment P7, P11, P13, P20. They are classified here according to the degree of precision and expertise expected. Thus, Worksheet, Information, and Form refer to a simple method; Summary and Plan indicate that the information is simplified and potentially incomplete; Inventory is a complete enumeration; Characterization gives, in addition to the enumeration of the Inventory, additional information allowing a perfect understanding of the object studied; Audit, Diagnosis, and Assessment bring, in addition to a description, expertise, recommendations, and hypotheses.
The terms used to qualify the AIM of the procedures making up the corpus are: Reduct* P14, Divers* P20, Recycl* P12, P14-18, P21, Renovat*/rehabilitat* P10, Deconstruct* P11, P20, Availab* P8, Salvag* P12, P20, Resourc* P7, Reus* P1, P3, P18. They are classified here according to the 3Rs policy (Reduce, Reuse, Recycle) (G8 summit, 2004), considering Reduct*and Divers* as the lowest levels – since the terms are associated with Waste – while Deconstruct*, Availab*, Salvag*, Resourc*, Reus* constitute the higher levels.
The terms used to qualify the OBJECT of the procedures making up the corpus are: Waste P12, P14, P20, Debris P16-17, Construction and Demolition (C&D) P14-19, P21, Deposit P18, Material P1, P4-5, P8, P12, Element P7. Waste and Debris are at the lowest levels since both terms consider the studied object as having no use. C&D provides no specific qualification and is considered neutral. Deposit implies potential future use, either as is or in a transformed form. The Material and Element retain the capacity and use inherent in their qualification.
Once constituents are defined for a given procedure, connecting the corresponding dots along the axis creates a blue form in the visual representation of purposes, as seen in Figure 10. The blue form highlights procedures P1, P5, P7-8, and P18, which have high purposes in all three axes, leading to big blue triangle shapes. Procedures P3-4, P6, P11, and P13 also have high purposes but do not address one or two of the axes. Procedures P9-10, P12, and P20 deal with Waste, the lowest constituent on the OBJECT axis. Nevertheless, their purposes on the other axes are high. Procedures P14-17, P19, and P21 can also be grouped, as their purposes are lower overall.
4.1.2 Responsibilities
As outlined in Section 2.1.2, three key roles were identified in the procedure framework: CREATOR, ASSESSOR, and AUDIENCE, represented along red axes in Figures 7, 10.
Profiles were established based on the corpus for each role. These categories include Owners, Contractors, Architects/Engineers, Scientists, Local authorities, and National authorities. These categories are ranked on the graph according to their level of involvement and impact, ranging from individual to collective influence. Each profile category is then assigned a role for each procedure.
Figure 8 shows that the creators of five procedures were states, ten were cities or counties, three were scientists, and three were architects or engineers. In the case of six procedures, the assessment responsibility (ASSESSOR) is shared between architects and engineers, while contractors assume it for ten procedures and owners for eleven. The assessment responsibility lies solely with the scientists when the procedure has been developed by them. The information collected through these procedures serves the following audience: the states (10), cities or counties (10), architects, engineers, and contractors (2), and owners (9). Regarding the stakeholders involved, architects and engineers are the only ones capable of both developing (CREATOR) and conducting assessments (ASSESSOR) for their own purposes (AUDIENCE). In contrast, authorities do not typically conduct assessments, and scientists are responsible for developing and conducting assessments on behalf of others. Contractors and owners conduct assessments mostly for their own needs or authorities’. These observations are also visible through the red form emerging from the linkage of the dots along the red axis in Figure 10.
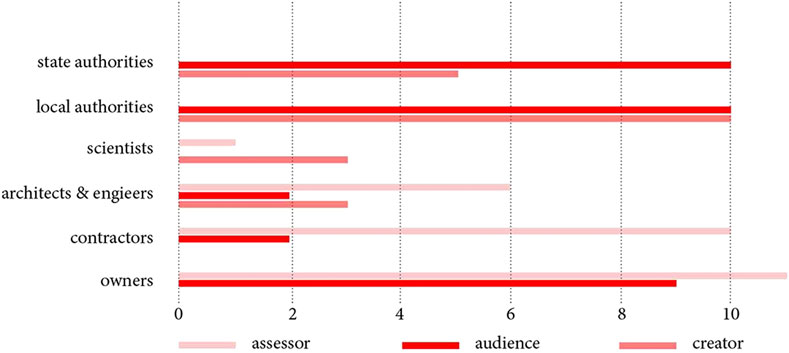
Figure 8. Distribution of the responsibilities. Profiles compose the y-axis, the x-axis represents the number of procedures concerned, and the charts’ colors define the roles.
4.1.3 Criteria
Not all the criteria’s constituents included in the procedures of the corpus have been extracted. The ones concerning hazardous elements or waste sorting are not taken into consideration as precise in Section 1.4. 58 data entries of criteria are selected from the corpus. Through the analysis process, they have been categorized into 9 themes, as represented in Figure 9, corresponding either to a description or projection information types. Figure 9 first offers a listing of the 58 data entries selected and their affiliation to a theme. Their names have been designed to be self-explanatory and require no further description. Different type lines inform the Values, P&M, and SU&I categories of data entries. Secondly, it gives the number of times a data entry is used by procedures as well as the number of data entries composing each procedure. Procedures are sorted by the number of data entries.
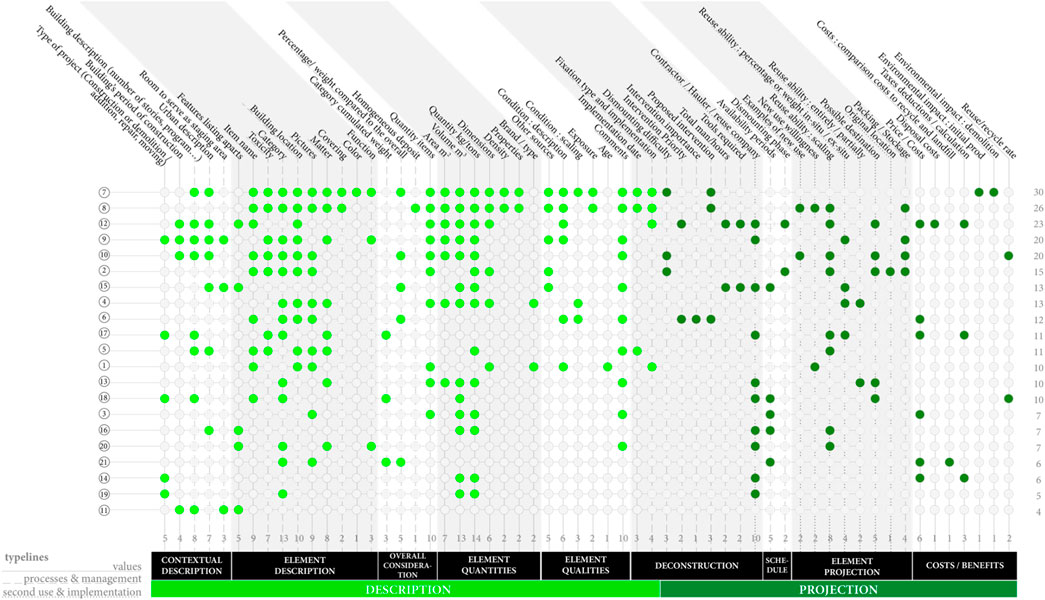
Figure 9. Criteria analysis. Data entries composing criteria are listed at the top. Themes are linked at the bottom of the illustration. Procedures with the most criteria entries are at the top.
As illustrated in Figure 9, descriptive criteria prevail throughout the corpus - 253 descriptive criteria are used versus 73 projective. Interestingly, certain data entries are only used once within the corpus - homogeneous deposit, element age, intervention importance, original location, disposal costs, cost comparison between recycle and landfill, Environmental impact: initial production, and Environmental impact: demolition. Conversely, the most frequently used criteria are Category (13 uses), Volume (13), and Quantity (14).
4.1.3.1 Descriptive criteria categories
This section describes the five themes, composed of 34 descriptive criteria. Data entries are named in italics, and their categories are in parentheses.
Contextual description: General criteria for the building or its environment that help in understanding the site, the possible building archetype, and the feasibility of the deconstruction project. This theme has 5 criteria, used 27 times in the corpus - Type of project (Construction or demolition/addition, repair, moving) (V), Building’s period of construction (V), Building description (number of stories, program …) (P&M), Urban description (P&M), Room to serve as staging area(P&M).
Element description: Criteria focusing on building components, which help to understand the observed element. This theme is made of 10 criteria, used 67 times in the corpus - Features listing aparts (V), Item name (V), Toxicity (P&M), Category (P&M), Building location (P&M), Pictures (V), Matter (V), Covering (P&M), Color (V), Function (P&M).
Consideration with the overall: Criteria to determine the presence of a source based on scalability and repeatability. This theme is made of 4 criteria, used 19 times in the corpus – Category cumulated weight (P&M), Percentage/weight compared to the overall (P&M), Homogeneous deposit (P&M), Quantity of items (V).
Element quantity: These are the criteria used to quantify the elements studied. Different types of values are considered. Properties and capabilities are part of this theme, which is why the brand or type of element is also considered here, as it also provides technical information. This theme is made of 7 criteria, used 46 times in the corpus – Area in m2(V), Volume in m3 (V), Quantity kg/tons (V), Dimensions (V), Density (V), Properties (V), Brand/type (V).
Element quality: These criteria are qualitative and provide information on the conditions of the elements observed. They are subjective and depend on the assessor’s expertise. This theme consists of 6 criteria, used 27 times in the corpus – Other sources (V), Condition: description (V), Condition: scaling (V), Exposure (V), Age (V), Comments (V).
Deconstruction: These criteria concern the initial implementation of the elements in the building. This category is made of 2 criteria, used 7 times in the corpus – Implementation date (V), Fixation type and implementation (P&M).
4.1.3.2 Projective criteria categories
Projective criteria are grouped into four themes, composed of 24 descriptive criteria.
Deconstruction: This theme is also projective. Hypotheses and management suggestions are developed prior to deconstruction or demolition. This category is made of 7 criteria, used 23 times in the corpus -Dismounting difficulty (P&M), Intervention priority (P&M), Intervention importance (P&M), Proposed intervention (P&M), Total man/hour (P&M), Tools required (P&M) Contractor/Hauler/reuse company (SU&I).
Schedule: These criteria provide information on deconstruction project planning. This theme consists of 2 criteria, used 7 times in the corpus – Availability periods (P&M), Dismounting phase (P&M).
Element projection: These criteria provide information on possible future use. This theme is made of 8 criteria, used 28 times in the corpus – Examples of new use (SU&I), New use willingness (SU&I), Reuse ability: scaling (SU&I), Reuse ability: percentage or weight in-situ/ex-situ (SU&I), Reuse ability: entirely/partially (SU&I), Possible destination (SU&I), Original location (SU&I), Packing/stockage (P&M).
Costs/benefits: These criteria provide value guidance. This theme consists of 7 criteria, used 15 times in the corpus – Price/costs (V), Disposal costs (V), Costs: comparison with costs to recycle and landfill (V), Taxes deductions/calculations (V), Environmental impact: initial production (V), Environmental impact: demolition (V), Reuse/recycle rate (V).
All this information is embedded within the framing of criteria, as represented in Figure 7 and characterized for each procedure in Figure 10.
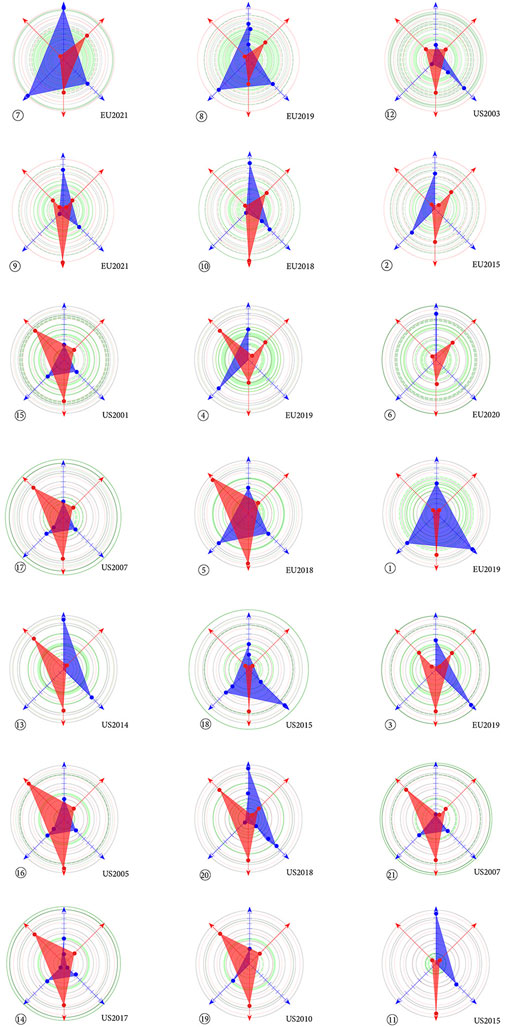
Figure 10. Procedure representations: sorted by number of criteria (green circles) Light green is the description criteria while the darkest is the projection ones. Grey circles mark each theme. Blue represents the purposes: top being DATA, right AIM, and left OBJECT. Red represents responsibilities: the bottom being CREATOR, right ASSESSOR, and left AUDIENCE.
4.2 Comparisons
Now that the graph components have been established and each procedure component—purposes, responsibilities, and criteria—has its own representation in Figure 10, comparisons between the different components can be drawn. This section describes and analyses these observed comparisons.
4.2.1 Criteria, date and origin
Upon analyzing the number of procedure criteria entries (green circles in Figure 10), it is observed that American procedures tend to have fewer criteria entries compared to European ones. Also, the most recent procedures reviewed in this study predominantly originate from Europe, spanning from 2015 to 2021. Nevertheless, three American procedures are part of the ten with the most criteria entries P12, P15, P17 and date from 2003, 2001, and 2007, respectively. Therefore, the number of criteria does not correspond to a more favorable region of the world, culture, or time context.
4.2.2 Criteria and purposes
This section focuses on criteria (green circles) and purposes (blue axis). While the five procedures with the most criteria P7-10, P12 prioritize the desire for a second life with the terms Reuse, Resources, Salvage, Available, or Deconstruction (AIM axis), the subsequent five procedures P2, P4, P6, P15, P17 do not. Some fail to specify their intention (AIM axis), and others emphasize Recycling. The DATA axis significantly influences the number of criteria entries as it tends to correspond to the position of its constituents. The higher the dot is on the axis, the more criteria entries are. The AIM is either precise and for a second life or does not need to be mentioned when the OBJECT is considered as an Element or a Material. Interestingly, procedures specifically targeting Reuse or Resources do not necessarily rank among the top ten procedures with the most criteria. The procedures with the most criteria use the terms Recovery, Availability, and Deconstruction. However, two notable exceptions in regard to the ten procedures with the most criteria are procedures developed by and for authorities P15 and P17. The terminologies of their titles are not precise in any purposes axis, with an AIM axis focused on Recycling, yet they are the seventh and tenth with the most criteria entries. It reflects the advanced waste management practices in the cities of San José and Chicago. The consideration of projective criteria has not been linked with any purpose axis (DATA, AIM, OBJECT).
4.2.3 Criteria and responsibilities
The procedures with the most criteria entries are those for which the CREATOR and ASSESSOR are the same stakeholders. In addition, the six procedures not created by authorities but by specialists (scientists, architects, or engineers) P2, P4, P6-8, and P12 are among the nine with the most criteria. Procedures drawn up by-and-for national or local authorities P5, P13-14, P16, and P19-20 are in most cases low in the number-of-criteria-entries ranking, exception made for P15 and P17 as described and explained in 4.2.2. The ASSESSOR is always a specialist (1 time is a scientist, 6 architects/engineers, 10 contractors) or owner (11 times), as shown already in Figure 8, rather than state or local bodies. When the ASSESSOR is solely an owner P11, P13, P18, the procedures tend to have a lower number of criteria entries. Owners are mainly the AUDIENCE (10 procedures). Among the 10 procedures with the least amount of criteria, 6 are targeting national or local authorities as AUDIENCE (P13-14, P16, and P19-21). It is noteworthy that the six procedures developed by-and-for American authorities considering Construction and Demolition (C&D) P14-17, P19, P21 share considerable visual similarities with elevated CREATOR and AUDIENCE dots and low ASSESSOR dots, with medium to low purposes of relatively equivalent distance of dots on the different axes but with a radical difference between the number of criteria entries composing P15 and P17 as already explained, and P14, P16, P19, P21 being parts of the 6 with the least criteria entries.
4.2.4 All components
There is a discrepancy between the precision of the terminology used to describe the procedures – purposes blue graph - and the number of criteria – green circles - within the nine procedures developed by-and-for authorities (CREATOR and AUDIENCE) – responsibilities red axis. Eight of these nine are developed in the United States. The European one is titled Inventory (DATA axis) of Material (OBJECT axis) during Deconstruction (AIM axis). Nevertheless, it only focuses on the possible presence of pollutants and encompasses 11 criteria entries. The American ones are also highly formulated but with only between 5 and 13 criteria, while the five procedures with the most criteria have between 30 and 20. It is also important to mention that the procedure P11 stands out, having the least number of criteria, yet it serves a unique purpose. This procedure P11 focuses on conducting an upstream assessment to determine the feasibility of a deconstruction project based on potential damage and time constraints without specifically considering the elements themselves (see Figure 6).
All procedures contain more descriptive criteria than projective ones. No specific trend has been revealed. Every procedure possesses singular features. Therefore, comparisons have been extended to the themes within the criteria in the following chapter.
4.2.5 Criteria themes
This section analyzes the comparison of procedures based on purposes, responsibilities, and criteria, focusing on the criteria themes (as presented in Figures 9, 10).
Twelve procedures consider contextual description P5, P7, P9-12, P14, P16-19, ten of which are developed by authorities (CREATOR). This reflects the authorities’ emphasis on considering the contextual aspects, particularly when they are also the AUDIENCE of the assessment. Most procedures drawn up by-and-for national or local authorities do not have consideration with the overall P5, P11, P14, P16, and P19-20. A global reflection on the site is therefore not envisaged in these procedures. In contrast, procedures targeting owners P1-3, P6-10, and P18 tend to incorporate a holistic vision in their assessment. Most of the criteria entries composing element quantities are addressed in five out of six procedures developed by specialists P2, P4, P7, P8, P12, with four of them being the ones with the most criteria. The sixth procedure P6 deviates from this trend, as it focuses on building diagnosis without specific aims or purposes, which explains the absence of quantification. Other procedures have little consideration for the OBJECT assessed as they are designating quantities as tons or m3. Procedures developed by-and-for authorities generally do not emphasize element qualities, as their objectives are not strongly linked to future use or projection. Only five of the procedures P3-5, P11, and P21 do not include any criteria related to element deconstruction, notably the ones developed by authorities. This observation aligns with the previous conclusion, indicating the authorities’ lack of interest in second-use possibilities for the elements. Instead, the focus seems to be on Deconstruction, suggesting the building up of a probably undervalued stock rather than prioritizing high-potential reuse with optimized capacity. Out of all the procedures, only seven consider solely the time factor P2-3, P12, P15-16, P18, and P21 (schedule). Planning is rarely considered in procedures. Regarding the element projection, only seven procedures do not include any criteria in this aspect P3, P6-7, P11, P14, P19, and P21. Due to advanced and strict waste management regulations in Europe and the US, procedures dealing with waste must provide information on the end-of-life location of the OBJECTS involved. Notably, procedure P7, with the most criteria, does not contain any criteria for the element projection. This procedure was designed to create a viable and well-informed stock without specific consideration for reuse or receiver projects, as it was developed within a mission that did not encompass these aspects. The ones with high AIM P1, P3, P12-13, P18, and P20 dealing specifically with Reuse, Resource, or Salvage do consider this theme. Nine procedures offer evaluations of costs/benefits P3, P6-7, P10, P12, P14, P17-18, and P21. Three of the five procedures with the most criteria address this aspect (P7, P10, and P12). Except for procedure P7, those 8 assessments focus on construction and demolition (C&D) or waste or do not specify the assessed OBJECT (blue axis). This suggests that only unconsidered objects (useless) are being estimated, indicating potential challenges in evaluating the costs and benefits associated with stock creation or even reuse.
4.2.6 Conclusion
No clear pattern emerged from the various comparisons. Similarities have been observed, as described in Section 4.2.3, but the presence of recurring exceptions has prevented patterns from being determined. Key takeaways include:
Purposes
• Few procedures prioritize reuse: Only a small subset (e.g., P1, P3, P7–8, and P18) explicitly target reuse or resource recovery. Most procedures focus on broader waste management or compliance objectives.
• Terminology ≠ depth: Procedures with reuse-related wording in their titles do not consistently contain more reuse-relevant data.
Responsibilities
• Specialist-developed procedures (e.g., by architects, engineers, or scientists) often include more detailed, reuse-oriented criteria.
• Authority-driven procedures tend to emphasize compliance or inventory goals over deconstruction logistics or second-use planning.
• When owners act as assessors, procedures tend to be simpler, with fewer criteria and limited foresight for reuse.
Criteria
• Quantity vs. quality of data: Some procedures contain many criteria but still miss key reuse aspects like second-use potential or disassembly logistics. Only a handful (e.g., P2, P8, P12–13, P17) include substantial second use and implementation (SU&I) content.
• Descriptive over projective: Descriptive criteria dominate (253 vs 73 projective). Projective criteria (e.g., second-use planning, timing, or cost projections) are rare but crucial for actual reuse implementation.
In the corpus, only a small number of procedures specifically aim at reusing or recovering Elements or Materials P1, P4-5, P7-8. They carry out an Inventory prior to deconstruction determining a stock potential, but few go beyond this phase. None provides an overall figure for elements with high reuse potential, with the phasing and cost required to develop the practice.
• Context is often under-considered: Few procedures (P7, P9-10, P12, P15, and P17-18) account for site-level logistics (e.g., access, storage areas, work phasing), yet these are essential for planning deconstruction or reuse workflows.
• Costs and timelines are neglected: Despite their importance in real-world reuse decisions, these aspects are rarely addressed explicitly. In general, the notions of time and cost are not considered, even though they dominate practice (Rakhshan et al., 2021; Lambec et al., 2024), lacking in procedures P1, P4-5, P7-11, P13, P19-20.
• Recycle-focused: The procedures with the most criteria seek to assess matters leaving sites (waste or C&D), with concerns on the end of the matters cycle, and are therefore developed and effective for landfill or recycling.
Continuum gaps
Most procedures lack frameworks assessing an object’s position along a lifecycle continuum—from Reuse as-is, to Repurposing, Recycling, or Waste. Attention to the object is often narrow, missing a progressive, scalable evaluation. This limits the effective positioning of elements within a circular economy.
5 Discussion
5.1 Critical evaluation of procedures to achieve reuse potential assessment
After comparing the purpose and different criteria used in the corpus procedures, it is interesting to understand how these are defined and how they could be improved or completed to assess the reuse potential of elements within buildings. The evaluation of a potential stock is mainly descriptive, while the reuse potential is projective and, therefore, partly subjective. When designing the procedures, choices are made in line with the defined needs and goals. Consequently, for a given object, some information is collected while others are ignored, which will ultimately influence the outputs. In the following, the phasing, the input methods, the categories of outputs, and the chosen criteria are reviewed, and possible developments are proposed.
First, the many ways of collecting, organizing, and recording information are reviewed, and the criteria used in the procedures are examined. The expected outputs are also detailed. Finally, the optimal criteria for increasing the reuse potential of elements are detailed. Then, the time factor, an implacable determinant of any implementation and concretization of a project, is considered. Depending on the specific purpose and objectives, the assessment of reuse potential can vary in scope and intensity, ranging from minimal information to an extensive research process. Based on the studied corpus of procedures, different phases are proposed, each building upon the previous one with refined objectives and means.
5.1.1 Input determinism
5.1.1.1 Collection
Various methods are available to collect information on building elements: visual inspections, destructive and non-destructive investigations, background and historical information review, etc. Most of the corpus is the result of visual inspection. In some cases, a document may be attached to complete the information on an element, like P1 and P4, using a Brand/type criterion, or P2, P7-9, P15 using Other sources as shown in Figure 9 and represented in Figure 10.
5.1.1.2 Organization
Given the large number of components in a building, procedures are mostly based on classification and categorization. Sometimes, procedures base their categories on construction standards, such as P4-5, P10. In other cases, they are based on building information modeling (BIM), such as the BAMB Circular Building Assessment (part of the discarded procedures of Section 2.1.1). Most of the time, categories are designer-specific.
5.1.1.3 Recording
The recording concerns the way the information is transcribed. Often, the ASSESSOR inputs a specific value expressed in a certain unit, such as weight in tons or volume in cubic meters, as seen in P9, P14, P16-19, and P21. Sometimes, expected values are not specified. The information collected will, therefore, depend on the capabilities and meticulousness of the ASSESSOR, as in P1-8, P10-13, P15. The possibility of comparing elements and projects will depend on the specificity of each description. To hinder this, some procedures offer a nomenclature like P10, checkboxes like P12, or scrolling choices like P20. This ensures that similar terms are used, making comparing and analyzing elements and projects easier.
5.1.2 Assumed outputs
The procedure outputs depend on the suggestions and expertise of those who create (CREATOR) and apply it (ASSESSOR). Assessing reuse potential means being able to construct plausible hypotheses about an element. Based on the 58 criteria from the corpus, three categories of outputs have been identified: Value (V), Processes and Management (P&M), and Second Use and Implementation (SU&I)- represented with different type lines in Figures 7, 9, 10. As a reminder, the V entries describe, quantify, and assess the profitability of the element, and the P&M entries allow for the plan of deconstruction while the SU&I entries envision a future use for the stock. These three categories are complementary and should be inseparable when assessing the reuse potential of an element within a building. However, it is not uncommon for not all of them to be addressed in the procedures. This leads to an incomplete evaluation of the reuse potential through all phases.
For instance, procedure P7, which has the most criteria, is composed of 26 descriptive criteria and 4 projective criteria: none of them includes SU&I entries. This procedure is very complete in describing elements (26 out of 33 descriptive criteria in total) and giving V category of information type concerning a potential stock. Some information is also given concerning P&M entries (11 descriptive P&M criteria out of 21 overall), but the reuse potential is not treated by this procedure. This is probably linked to high expectancies in every purpose’s axes and the use of the term Resource that might evoke potential mining without necessary a foreseen use.
Procedures P2, P8, P12-13, and P17 contain the most criteria for the SU&I category of entries, each informing 3 SU&I entries out of a possible total of 8. P2, P8, P12-13 have the same consideration concerning the salvage of element back in Figure 6, P2, P8, and P13 have high DATA expectancies. P2, P8, and P12 are developed by scientists and architects/engineers with strong deconstruction and reuse experiences, P13, P17 are developed by cities with advanced regulations concerning recycling and salvaging.
Procedure P17 concerns C&D, Debris (OBJECT) recycling (AIM), Form (DATA), low purposes, and is composed of only 11 entries in total. This procedure, referring to debris, describes only necessary V information with four entries, informs P&M with 4, and foresees transportation and reuse ability with 3 SU&I entries. In that sense, this procedure is very effective in achieving its purposes. A complete assessment contains V, P&M, and SU&I entries that serve the established purpose. Defining the purposes of an assessment influences the outputs. A willingness to achieve reuse requires the assumptions of P&M and SU&I information.
5.1.2.1 Values (V)
In the corpus, elements are quantified, mainly in terms of volume and weight, to produce results concerning the material. Indeed, these outputs are necessary to determine the costs of landfilling or recycling. Changing these values to consider reuse implies moving from consideration of the material to that of the element. Procedures P1-2, P4, P7-8, and P12 include dimensional criteria of the elements (Elements or Materials).
While the matter will remain the same during deconstruction or demolition, the properties of an element are not guaranteed. Extraction and restoration involve operations that need to be anticipated and planned to enable any costs/benefits quantification. Seeking to reuse an element implicitly means making assumptions about what it will become once it is put back on the market and implemented again, and therefore producing values based on developed scenarios. To inform with projection-based values concerning costs and benefits, criteria concerning P&M as well as SU&I need to be anticipated.
Procedures P12 and P15 estimate the necessary tools and time for the site work to be done as their AIMS are on Deconstruction and Recycling facility certification. Procedures P9 and P15 inform the rooms to serve as staging areas. No procedure foresees the volume of storage needed for the potential salvaged elements. No corpus procedure currently proposes a comparison between standard demolition or reuse options when procedures P18 and P21 compare recycling weights or costs to landfilling ones. No procedure estimates the costs and benefits of deconstruction or reuse of elements. This knowledge is reserved for waste management.
5.1.2.2 Processes and management (P&M)
Knowing how to disassemble an element is knowing how it was assembled. However, this information is often difficult to obtain. Indeed, certain elements, such as structures, are made invisible during the evaluation due to other applied layers. Also, construction plans are not always easy to acquire. Often, the plans obtained are from the project phase, not as-build ones, and do not exactly correspond to reality. However, the industrial production of some element types leads to the standardization of assemblies and compositions, thereby making it possible to find the corresponding technical sheet retroactively or to carry out a proofing test valid for a series of elements.
The deconstruction process relies heavily on historical and localized knowledge of the techniques employed in constructing a built archetype. Expertise is essential to determining the assembly hypothesis and, therefore, to formulating the disassembly hypothesis. In addition, it is necessary to imagine the type of employable machines, their number, the number of workers, and their qualifications to estimate the feasibility of a project. Knowing the time available or the minimum time necessary for the work is also decisive for the feasibility of a deconstruction project and provides a greater guarantee of high achievements.
Only procedures P12 and P15 provide information on how to extract the elements and the necessary tools and qualifications. Procedure P9 only has a package/storage criterion concerning stock management, while procedures P3-4, P11, and P14 have no P&M criteria. Again, without this P&M information, it is difficult to plan any deconstruction project.
5.1.2.3 Second use and implementation (SU&I)
Procedures P8 and P10 provide existing examples of new uses to be inspirational, while the procedure P1 requires a projection of a new use per item. Therefore, a high reuse potential is measured not only by the conservation of properties of the element but also by its versatility to respond to another project. In other words, most of the outputs can only be assumed - as any other project based on an existing building (renovation, transformation). Because reuse is not a typical production operation but a multitude of specific products, only estimations can be assumed. This leads to a greater risk of inadequacy between assumptions and reality or a greater demand for project adaptation. It appears, therefore, necessary to quantify an acceptable margin of inadequacy for a construction project involving reused elements.
5.1.3 Incomplete processes
The following paragraphs discuss criteria not covered by the corpus of procedures, but that nevertheless seem essential to the authors based on other references (Guy, 2006; Interreg et al., 2020; DELTA, 2018; NAHB, 2001) and practical experiences.
5.1.3.1 Descriptive criteria
The criteria composing the procedure must allow versatility, considering multiple scenarios. It is crucial to present values comprehensively, considering various data to fulfill any future interest. Evaluating reuse potential also depends on the versatility of elements to adapt to different projects. A multifaceted approach thus ensures a comprehensive evaluation of the reuse potential of elements. The procedure should allow the collection of all required or available information concerning an element or a set of elements. To ensure successful deconstruction, remarketing, and traceability of building elements, data sheets should be provided in the assessment, like procedures P7 and P8. These sheets synthesize information about the considered elements’ composition, capacity, implementation, maintenance, and other essential details. By issuing data sheets that meet the requirements of new elements ex-factory, it becomes possible to compare and maintain competitiveness in the market.
5.1.3.2 Projective criteria
The lack of accessibility to an element constitutes a potential limit to the deconstruction project. Accessibility is a crucial factor that can impact the difficulty and duration of the work, making it necessary to anticipate and plan accordingly. Determining an inventory of elements with reuse potential and locating the elements allows a comprehensive understanding of the project and assesses the accessibility of each element. No matter how viable the reuse of an element is, if it is too difficult to reach, extract, or move, then it is likely that its potential for reuse is low. The assessment must include information on the accessibility of elements:
1. At the regional level (distance and access to landfills or storage).
2. At the site level (place for dumpsters, machinery, on-site workshop, and storage area)
3. At the building level (circulation, staircases, elevators).
The assessment should then provide information concerning the assemblies and installations of elements to anticipate extraction operations and ensure elements are delivered in good condition after removal. This includes information about coatings and stains to anticipate stripping operations necessary for remarketing.
Finally, in the context of deconstruction, it is interesting and helpful to produce a work schedule and inform the availability of an element in a just-in-time circuit (direct delivery from the source site to the receiving project site without storage). Compared to a newly manufactured product where the production time, and therefore its availability, is known, the ability to define the availability of a reclaimed element is crucial for efficient project planning.
Procedure outputs, including values (V), processes and management (P&M), and second use and implementation (SU&I), are crucial for assessing reuse potential. However, it is not uncommon for procedures to address only a subset of these categories, resulting in an incomplete evaluation of reuse potential across all phases. This highlights the need for a unified set of criteria to capture diverse aspects of reuse potential, including projective hypotheses. Implementing scenarios with projective hypotheses is crucial to promoting reuse over landfilling and recycling. This necessitates considerations such as site schedules, element availability, and the assessment of prices and various values.
5.1.4 Phasing
5.1.4.1 Feasibility assessment
When considering the deconstruction of a building, it is crucial to weigh the potential benefits against the associated drawbacks carefully. Factors such as the presence of hazardous materials, the time and resources required, and the risks to workers need to be considered. Conducting a preliminary study like the procedure P11 or what is recommended within P12, including a short site visit and gathering basic information about the building’s construction and maintenance, is essential to determining the feasibility of deconstruction, whether reuse is feasible, and to what extent.
Certain building elements are well suited for reuse, particularly those easily accessible or dismountable. These features are often found in the superficial layers, referred to as Stuff and Skin by Brand (1995). Additionally, elements with a consistent market demand and those that require minimal guarantee or assurance for their second use hold high potential for reuse. This potential can be assessed during the first visit and included in the feasibility assessment, helping to determine whether further research is necessary.
5.1.4.2 Targeted assessment
In a more targeted evaluation, it is crucial to establish relevant criteria and values for assessing a potential stock. The aim can first be to identify potential reuse applications for the different building elements. This can be achieved by identifying batches of elements repeated throughout the site, or by recognizing elements with high market value, such as those designed by renowned designers, rare and luxurious materials, or items with heritage value. Procedures P2, P7-9, and P15 are the only ones using the criterion. Other sources, as shown in Figure 9, demonstrate that additional research is carried out to qualify an element with high market value and is ranked among the seven procedures with the most criteria. The evaluation can also be based on pre-established projects, focusing on elements that can be integrated into a receiving building, as proposed by P1 and P8 with a New use willingness. The assessment then centers on the compatibility between the elements and this receiving building. Finally, another objective may be to prioritize elements with a significant environmental impact, such as reusing the whole building structure or its foundations, using criteria such as Environmental impact: initial production and Environmental impact: demolition as P7.
5.1.4.3 Comprehensive approach
Another approach is to strive for the reuse of everything, or at least to bring all elements back to the market, regardless of the time, resources, and profitability involved in the project. This approach aims to minimize waste generation and preserve the intrinsic qualities of the elements. It entails considering every element as having potential value, dismantling everything with care, and avoiding establishing a hierarchy of values.
5.2 Overarching insights and future directions
The multidimensional analysis of the 21 assessment procedures based on their decomposition into inputs, outputs, and processes, synthesized with graphical representation, revealed a lack of clear patterns drawn. While some procedures are comprehensive, with numerous criteria, they tend to focus on assessing matters that come out of working sites, such as waste or construction and demolition debris. However, few procedures specifically target reusing (P1, P3, and P18) elements (P7), or materials (P1, P4-5, P8, and P12), as described in Section 4.1.1. Most procedures conduct an inventory prior to deconstruction (Figure 6), but they generally lack comprehensive values for elements with high reuse potential. Additionally, considerations of time, cost, and the holistic vision of the site and its context are often overlooked. Such criteria should go beyond the mere conservation of material properties and consider the versatility of elements to respond to different project requirements. Nearly 20 years later, based on a corpus of applied procedures, this study reaffirms Addis (2006) “key issues need{ed} to be addressed when seeking the goods and comparing the various alternatives that are found: performance, quality, and durability; installation procedures;(…) cost and value-for-money;(…) procedure for purchasing, including guarantees on price and delivery;(…) assessing and comparing the environmental benefits of using reclaimed materials and goods.”
Furthermore, a single scalable assessment procedure with unified criteria could facilitate the refinement of information and expertise as projects progress. Processes and management in the assessment require considering site characteristics, workers’ skills, and the tools required for successful deconstruction. A scalable assessment with unified criteria would allow additional layers of specialized expertise. As mentioned by Charef et al. (2021), the ISO framework standard (ISO/DIS 59004) will be challenged by the need for a framework to be comprehensive and holistic by covering a range of geographical and temporal scales while also being practically useful.
5.2.1 Subjectivity is ubiquitous
The potential for reuse of building elements is influenced by a range of factors beyond their physical and intrinsic capacity. Social aspects, environmental benefits, financial considerations, and market dynamics also shape the potential for reuse. Understanding the market for each item is, therefore, crucial for accurate assessments. Time constraints on the construction site also challenge deconstruction projects (as evoked in Section 4.1.3.2). Land profitability and knowledge of local partners affect decision-making processes. Additionally, expertise and knowledge of future component use contribute to a more accurate assessment of reuse potential (see Sections 5.1.2.3, 5.1.3.2). These factors, which are diverse and challenging to quantify, go beyond the physical and material aspects of deconstruction and reuse.
Moreover, the CREATORS and ASSESSORS bring their own perspectives, biases, and expertise to the assessment process, thus influencing the assessment results. The information gathered during the assessment is inherently subjective and dependent on several factors. First, it is influenced by the expectations of the CREATOR, who may prioritize certain aspects or outcomes based on their specific purposes and interests (as seen in Section 4). Additionally, how information is captured introduces limitations and biases and can shape the assessment outcome (see Section 5.1.1). Second, the capabilities and knowledge of the ASSESSOR play a vital role in determining the depth and accuracy of the assessment (referring to Section 4.2.3).
Also, depending on the context, assessments may be pre-oriented towards specific criteria or tailored to match a predetermined receiving project. This targeted approach limits the assessment’s scope and focuses on the compatibility of individual elements with specific projects rather than considering the overall reuse potential (see Section 5.1.4.2). This highlights the need to carefully consider purposes and methodologies in assessing reuse potential and recognizing the inherent limitations and biases that may arise in the process.
Overall, it is essential to acknowledge and be aware of the inherent subjectivity in the assessment process. To mitigate the influence of individual biases and promote objectivity, the assessment design should incorporate measures to minimize personal decision-making (as studied in Section 5.1.1) and allow for a diverse range of potential outcomes (in Section 5.1.4). This can be achieved through standardized methodologies, clear criteria, expected values, and transparent evaluation processes that enable multiple perspectives to be considered, mitigate individual subjectivity’s influence, and comprehensibly facilitate comparisons and additions. By doing so, assessments can aim to provide more objective and comprehensive evaluations of reuse potential.
5.2.2 Procedure completeness is not consensual yet
Completeness should ensure that all relevant information is captured with sufficient details and that the assessment results are relevant to the market realities (evoked in Sections 5.1.2.1, 5.1.4). The findings highlight the importance of considering both general building information and specific element details in evaluating reuse potential.
The assessment criteria vary depending on the stakeholders’ interests, underscoring the need for a comprehensive and inclusive approach. Moreover, current assessments tend to overlook the deconstruction phase (see Section 5.1.2.2), which is crucial to ensure a comprehensive assessment of reuse potential. Several aspects related to completeness thus remain unresolved, including the treatment of flows such as supply and demand dynamics, the consideration of temporal phases, and geometric properties beyond simply volumes and weights. Efforts are needed to further develop and refine assessment methodologies, addressing these unresolved issues to achieve a more comprehensive and standardized approach to assessing reuse potential in building elements.
Furthermore, it is essential to recognize that the potential for reuse can evolve and change throughout different phases and purposes. Therefore, assessments should be designed to accommodate and incorporate all newly-seen-as-relevant criteria. This ensures that all stakeholders contribute valuable information to the assessment process and that the assessment itself can be refined as needed to align with projected needs and potential. To enhance the effectiveness and accuracy of reuse potential assessments, additional criteria that capture the value of elements in relation to their accessibility and consider all relevant phases and objectives of a project should exist (from Sections 5.1.3, 5.1.4). By adopting a comprehensive approach, assessments can provide a more holistic understanding of reuse potential and support informed decision-making in the field of deconstruction and salvage.
5.2.3 Norms are challenged
Measurement and quantification of building elements before dismantling pose significant challenges. Assessing reuse potential relies on assumptions regarding the existing building, the dismantling process, and the transformation of materials. These assumptions, along with considerations for sales and reuse, contribute to the estimation of reuse potential. These assessments involve inherent uncertainties.
Efforts to establish common frameworks, guidelines, and best practices as (Interreg et al., 2020, Guy et al., 2003 or Addis, 2006) can help to minimize uncertainties and enhance the consistency and reliability of reuse potential assessments. It would provide stakeholders with a standardized approach and ensure consistency in evaluating reuse potential across projects. Such a framework would not only open new market opportunities but also pave the way for the emergence of new professions dedicated to reuse assessment.
5.2.4 Future research
Reuse is gaining momentum. Studies made before 2021 have already been overcome by new studies or have evolved considerably since then. New graphs illustrating these procedures could complete this comparative study, confirm the proposed observations, or highlight other trends.
Efforts should be made to enhance the objectivity and completeness of reuse potential assessments. This can be achieved through the development of standardized assessment procedures. The following points should be addressed.
- Identify and quantify the diverse range of values, including economic, environmental, social, and cultural aspects, and integrate them into the assessment framework;
- Address the full range of building elements and their potential for reuse. This includes identifying and incorporating all relevant factors and criteria contributing to the assessment process to strive for completeness.
- Explore strategies for sequencing assessments to align with project timelines. This involves determining the optimal timing and frequency of assessments throughout different project stages to ensure the timely identification of reuse opportunities.
This could be achieved by automating reuse potential assessment and developing a universal reuse assessment framework that is adjustable to diverse geographic regions.
6 Conclusion
The main objective of this study was to identify and compare existing procedures for assessing the reuse potential of building elements. Through an extensive collection of procedures and their in-depth comparative analyses, including a critical comparison of reuse-potential assessment inputs, outputs, and processes, this study provides valuable insights into the assessment methodologies currently in use or proposed.
This study has illuminated the complex landscape of stakeholders involved in these procedures. Notably, architects and engineers are uniquely positioned to both create (CREATOR) procedures and evaluate (ASSESSOR) for their own use (AUDIENCE), whereas authorities typically do not engage in assessments. Scientists, conversely, are tasked with developing and conducting assessments on behalf of other stakeholders. Contractors and owners primarily conduct assessments to meet their own needs or those of authorities. Assessments tend to be conducted by specialists or owners rather than by state or local bodies. Procedures assessed solely by owners often have fewer criteria entries.
The analysis revealed a marked predominance of descriptive criteria over projective criteria, with 253 descriptive criteria compared to 73 projective criteria. The consideration of projective criteria has not been linked with any purpose axis (DATA, AIM, OBJECT). Procedures developed by specialists—such as scientists, architects, and engineers—rank highly in terms of criteria count, demonstrating their detailed and specialized nature. In contrast, procedures developed by national or local authorities generally feature fewer criteria, with notable exceptions being those from cities with advanced waste management practices, such as San José and Chicago. All procedures analyzed contained more descriptive criteria than projective ones, indicating a general trend toward current-state evaluations rather than future projections.
Interestingly, procedures that specifically target Reuse or Resource management do not always rank among the top in terms of criteria count. Instead, the most criteria-rich procedures emphasize terms like Recovery, Availability, and Deconstruction. Furthermore, procedures developed by-and-for authorities often do not focus on element qualities or element deconstruction, reflecting a lack of interest in the reuse potential for elements. The procedures with the most criteria are primarily focused on assessing materials exiting sites, such as Waste or C&D Debris, making them effective for landfill or recycling purposes. Within the corpus, only a limited number of procedures specifically target the Reuse or Recovery of Elements or Materials. These procedures typically include an Inventory prior to Deconstruction to determine stock potential but rarely advance beyond this initial phase. None of the procedures provide comprehensive data on elements with high reuse potential or address the phasing and costs necessary for implementing reuse practices. A holistic vision of the site and its context, crucial for effective deconstruction, is rare. The focus tends to be very narrow, concentrating on specific objects without a progressive approach from element to matter to waste. This limited scope suggests that while current procedures may effectively address immediate waste management concerns, they fall short of facilitating comprehensive reuse and recovery planning.
Although some similarities were observed with the graphical representation of procedures (Figure 10, noted in Section 4.2.3), recurring exceptions have hindered the identification of consistent visual patterns.
Overall, the study highlights several gaps in existing procedures. Their analysis leads to the following needs for development.
1. Criteria expansion: Transitioning from waste recycling to element reuse necessitates revising purposes and expanding criteria to include projective Values, deconstruction Processes and Management, and foresee Second-Use and Implementation. These criteria are essential for assessing the inventory of building elements, their deconstruction, and potential reuse. Current practices lack completeness.
2. Versatility through refinement: Versatility allows for the inclusion of additional expertise, enhancing the robustness of evaluations. Implementing a versatile approach requires a scalable, compartmentalisable and progressive refinement of the assessment process.
3. Need for standardization: Many aspects of deconstruction and reuse are currently only implicitly addressed. A standardized assessment would pull the deconstruction and reuse practice upwards in all markets, included where it is not well-established.
4. Reducing subjectivity: Subjectivity remains prevalent, influenced by the profiles and roles of those conducting assessments. A standardized procedure with automated criteria would help mitigate biases and ensure more objective evaluations.
By shedding light on the strengths and weaknesses of assessment procedures, this research offers a foundation for enhancing their effectiveness. Building on the study findings, the authors propose the development of a generic, adaptable, and automatable reusability assessment procedure that would provide a standardized framework easily tailored to specific projects and contexts. Such framework is believed to streamline the evaluation process with more consistent, practical, and efficient records on reusability potentials, which should boost exchanges of information and thereof exchanges of reused materials.
Data availability statement
The original contributions presented in the study are included in the article/Supplementary Material, further inquiries can be directed to the corresponding author.
Author contributions
BL: Conceptualization, Data curation, Formal Analysis, Investigation, Methodology, Visualization, Writing – original draft, Writing – review and editing. MB-M: Conceptualization, Supervision, Writing – review and editing. CF: Conceptualization, Funding acquisition, Project administration, Supervision, Writing – review and editing.
Funding
The author(s) declare that financial support was received for the research and/or publication of this article. The Swiss National Science Foundation funds this work through grant FNS 105216_192693.
Conflict of interest
The authors declare that the research was conducted in the absence of any commercial or financial relationships that could be construed as a potential conflict of interest.
Generative AI statement
The authors declare that no Generative AI was used in the creation of this manuscript.
Publisher’s note
All claims expressed in this article are solely those of the authors and do not necessarily represent those of their affiliated organizations, or those of the publisher, the editors and the reviewers. Any product that may be evaluated in this article, or claim that may be made by its manufacturer, is not guaranteed or endorsed by the publisher.
Supplementary material
The Supplementary Material for this article can be found online at: https://www.frontiersin.org/articles/10.3389/fbuil.2025.1511109/full#supplementary-material
References
Addis, B. (2006). Building with reclaimed components and materials: a design handbook for reuse and recycling. Routledge.
ADEME (2021). “Guide à la rédaction d’un cahier des charges,” in Diagnostic déchets préalable à une opération de déconstruction ou réhabilitation de bâtiment.
Akanbi, L. A., Oyedele, L. O., Omoteso, K., Bilal, M., Akinade, O. O., Ajayi, A. O., et al. (2019). Disassembly and deconstruction analytics system (D-DAS) for construction in a circular economy. J. Clean. Prod. 223, 386–396. doi:10.1016/j.jclepro.2019.03.172
Arora, M., Raspall, F., Cheah, L., and Silva, A. (2020). Buildings and the circular economy: estimating urban mining, recovery and reuse potential of building components. Resour. Conserv. Recycl. 154, 104581. doi:10.1016/j.resconrec.2019.104581
Baker-Brown, D. (2019). The re-use atlas: a designer’s guide towards the circular economy. Routledge.
Brütting, J., Desruelle, J., Senatore, G., and Fivet, C. (2019). “Design of truss structures through reuse,” in Presented at the structures. Elsevier, 128–137.
Cai, G., and Waldmann, D. (2019). A material and component bank to facilitate material recycling and component reuse for a sustainable construction: concept and preliminary study. Clean. Technol. Environ. Policy 21, 2015–2032. doi:10.1007/s10098-019-01758-1
Cavalli, A., Cibecchini, D., Togni, M., and Sousa, H. S. (2016). A review on the mechanical properties of aged wood and salvaged timber. Constr. Build. Mater. 114, 681–687. doi:10.1016/j.conbuildmat.2016.04.001
Charef, R., Ganjian, E., and Emmitt, S. (2021). Socio-economic and environmental barriers for a holistic asset lifecycle approach to achieve circular economy: a pattern-matching method. Technol. Forecast. Soc. Change 170, 120798. doi:10.1016/j.techfore.2021.120798
Chini, A. R., and Bruening, S. (2003). Deconstruction and materials reuse in the United States. Future sustain. Constr. 14, 1–22.
City of Chicago (2007b). Best management practices. Chicago’s Guide to construction and demolition cleanliness and recycling.
City of San Mateo, P. W. (2017). Construction and demolition recycling and waste reduction plan and information.
Condotta, M., and Zatta, E. (2021). Reuse of building elements in the architectural practice and the European regulatory context: inconsistencies and possible improvements. J. Clean. Prod. 318, 128413. doi:10.1016/j.jclepro.2021.128413
Crowther, P. (2000). Building deconstruction in Australia. Overv. Deconstruction Sel. Ctries., 14–44.
De Wolf, C., Hoxha, E., and Fivet, C. (2020). Comparison of environmental assessment methods when reusing building components: a case study. Sustain. Cities Soc. 61, 102322. doi:10.1016/j.scs.2020.102322
DELTA. (2018). Deconstruction and building material reuse: a tool for local governments and economic development practitioners.
Devènes, J., Bastien-Masse, M., and Fivet, C. (2024). Reusability assessment of reinforced concrete components prior to deconstruction from obsolete buildings. J. Build. Eng. 84, 108584. doi:10.1016/j.jobe.2024.108584
Devènes, J., Bastien-Masse, M., Küpfer, C., and Fivet, C. (2023). “Reusability assessment of obsolete reinforced concrete structural components,” in Presented at the international symposium of the international federation for structural concrete. Springer, 440–449.
Durmisevic, E., Beurskens, P. R., Adrosevic, R., and Westerdijk, R. (2017). “Systemic view on reuse potential of building elements, components and systems-comprehensive framework for assessing reuse potential of building elements,” in International HISER conference on advances in recycling and management of construction and demolition waste (Delft, The: Delft University of Technology Delft University of Technology), 275–280.
European Commission. (2018). Guidelines for the waste audits before demolition and renovation works of buildings.
European Parliament (2008). Directive 2008/98/EC of the European parliament and of the Council of 19 november 2008 on waste and repealing certain directives (text with EEA relevance). OJ L.
Fitchburg public works. (2015). Preliminary CDRR (construction and demolition reuse/recycling), plan and deposit form.
Flourentzou, F., Genre, J.-L., and Roulet, C.-A. (2001). “EPIQR-TOBUS: a new generation of refurbishment decision aid methods,” in Towards sustainable building (Springer), 161–169.
Fujita, M., and Kuki, K. (2016). An evaluation of mechanical properties with the hardness of building steel structural members for reuse by NDT. Metals 6, 247. doi:10.3390/met6100247
Ghyoot, M., Devlieger, L., Billiet, L., and Warnier, A. (2018). Déconstruction et réemploi: comment faire circuler les éléments de construction. Lausanne: Presses polytechniques et universitaires romandes.
Gobbo, E., Trachte, S., and Massart, C. (2019). “Energy retrofit scenarios: material flows and circularity,” in Presented at the IOP conference series: earth and environmental science. IOP Publishing.012029
Gorgolewski, M. (2008). Designing with reused building components: some challenges. Build. Res. Inf. 36, 175–188. doi:10.1080/09613210701559499
Gorgolewski, M., Straka, V., Edmonds, J., and Sergio-Dzoutzidis, C. (2008). Designing buildings using reclaimed steel components. J. Green Build. 3, 97–107. doi:10.3992/jgb.3.3.97
Guy, B. (2006). The optimization of building deconstruction for department of defense facilities: FT. Mcclellan Deconstruction Project. J. Green Build. 1, 102–122. doi:10.3992/jgb.1.1.102
Heisel, F., McGranahan, J., and Boghossian, A. (2022). “ScanR: a composite building scanning and survey method for the evaluation of materials and reuse potentials prior to demolition and deconstruction,” in Presented at the IOP conference series: earth and environmental science. IOP Publishing.012012.
Hobbs, G., and Adams, K. (2017). Reuse of building products and materials – barriers and opportunities.
Interreg, F. (2019). “Facilitating the circulation of reclaimed building elements in northwestern Europe, review of existing pre-demolition tools, policies, resources for identifying,” in Quantifying and organizing the reclamation of reusable elements.
Interreg, F., Deweerdt, M., and Mertens, M. (2020). A guide for identifying the reuse potential of construction products.
Jabeen, I. (2020). “Economic feasibility of reusing structural components,” in How to quantitatively assess the economic feasibility of reusing structural components from existing buildings into new construction?
Laefer, D. F., and Manke, J. P. (2008). Building reuse assessment for sustainable urban reconstruction. J. Constr. Eng. Manag. 134, 217–227. doi:10.1061/(asce)0733-9364(2008)134:3(217)
Lambec, B., Bastien-Masse, M., and Fivet, C. (2024). How gained experience influences perceived levers and barriers of reuse practices: learning from north Americans. Sustainability 16, 10999. doi:10.3390/su162410999
Lazarus, N. (2003). Beddington zero (fossil) energy development construction materials report toolkit for carbon neutral developments-part 1.
Los Angeles County, P. W. (2005). Application Form, construction and demolition debris recycling summary.
Mata, E., Harris, S., Novikova, A., Fp Lucena, A., and Bertoldi, P. (2020). Climate mitigation from circular and sharing economy in the buildings sector. Resour. Conserv. Recycl. 158.
McDonough, W., and Braungart, M. (2010). Cradle to cradle: remaking the way we make things. North point press.
NAHB. (2001). A guide to deconstruction: an overview of destruction with a focus on community development opportunities.
Nakamura, T., and Halada, K. (2015). Urban mining systems, SpringerBriefs in applied sciences and technology. Japan, Tokyo: Springer. doi:10.1007/978-4-431-55075-4
Pristerà, G., Tonini, D., Tornaghi, M. L., Caro, D., and Sala, S. (2024). Taxonomy of design for deconstruction options to enable circular economy in buildings. Resour. Environ. Sustain. 15, 100153. doi:10.1016/j.resenv.2024.100153
Rakhshan, K., Morel, J.-C., Alaka, H., and Charef, R. (2020). Components reuse in the building sector – a systematic review. Waste Manag. Res. J. sustain. Circ. Econ. 38, 347–370. doi:10.1177/0734242X20910463
Rakhshan, K., Morel, J.-C., and Daneshkhah, A. (2021). A probabilistic predictive model for assessing the economic reusability of load-bearing building components: developing a Circular Economy framework. Sustain. Prod. Consum. 27, 630–642. doi:10.1016/j.spc.2021.01.031
Rose, C. M., and Stegemann, J. A. (2018). “Characterising existing buildings as material banks (E-BAMB) to enable component reuse,” in Presented at the proceedings of the institution of civil engineers-engineering sustainability. Thomas Telford Ltd, 129–140.
ROTOR. (2015). Vade-mecum pour le réemploi hors-site, Comment extraire les matériaux réutilisables de bâtiments publics ?
Saghafi, M. D., and Teshnizi, Z. S. H. (2011). Recycling value of building materials in building assessment systems. Energy Build. 43, 3181–3188. doi:10.1016/j.enbuild.2011.08.016
Schultmann, F., and Rentz, O. (2000). The state of deconstruction in Germany. Kibert CJ Chini AR Eds 45–74.
Keywords: reuse, deconstruction, reverse logistics, assessments, procedures, United States, europe
Citation: Lambec B, Bastien-Masse M and Fivet C (2025) Analysis and synthesis of existing procedures used to determine the reuse potential of building elements. Front. Built Environ. 11:1511109. doi: 10.3389/fbuil.2025.1511109
Received: 14 October 2024; Accepted: 01 April 2025;
Published: 01 May 2025.
Edited by:
Timothy O. Olawumi, Edinburgh Napier University, United KingdomReviewed by:
Hasim Altan, Prince Mohammad bin Fahd University, Saudi ArabiaAmir Ali Shahmansouri, Washington State University, United States
Copyright © 2025 Lambec, Bastien-Masse and Fivet. This is an open-access article distributed under the terms of the Creative Commons Attribution License (CC BY). The use, distribution or reproduction in other forums is permitted, provided the original author(s) and the copyright owner(s) are credited and that the original publication in this journal is cited, in accordance with accepted academic practice. No use, distribution or reproduction is permitted which does not comply with these terms.
*Correspondence: Barbara Lambec, YmFyYmFyYS5sYW1iZWNAZXBmbC5jaA==