Movement of Rehabilitated African Elephant Calves Following Soft Release Into a Wildlife Sanctuary
- 1San Diego Zoo Wildlife Alliance, Escondido, CA, United States
- 2Conservation Ecology Center, Smithsonian Conservation Biology Institute, Front Royal, VA, United States
- 3Save the Elephants, Nairobi, Kenya
- 4Department of Fish, Wildlife, and Conservation Biology, Colorado State University, Fort Collins, CO, United States
- 5Northern Rangelands Trust, Isiolo, Kenya
- 6Department of Zoology, Oxford University, Oxford, United Kingdom
- 7Sera Wildlife Conservancy, Samburu County, Kenya
- 8Kenya Wildlife Service, Nairobi, Kenya
The ability to locate essential resources is a critical step for wildlife translocated into novel environments. Understanding this process of exploration is highly desirable for management that seeks to resettle wildlife, particularly as translocation projects tend to be expensive and have a high potential for failure. African savannah elephants (Loxodonta africana) are very mobile and rely on large areas especially in arid environments, and are translocated for differing management and conservation objectives. Thus, research into how translocated elephants use the landscape when released may both guide elephant managers and be useful for translocations of other species that adjust their movement to social and ecological conditions. In this study, we investigated the movement of eight GPS tracked calves (translocated in three cohorts) following their soft release into a 107 km2 fenced wildlife sanctuary in northern Kenya and compared their movement with that of five tracked wild elephants in the sanctuary. We describe their exploration of the sanctuary, discovery of water points, and activity budgets during the first seven, 14, and 20 months after release. We explored how patterns are affected by time since release, ecological conditions, and social factors. We found that calves visited new areas of the sanctuary and water points during greener periods and earlier post-release. Social context was associated with exploration, with later release and association with wild elephants predictive of visits to new areas. Wild elephants tended to use a greater number of sites per 14-day period than the released calves. Activity budgets determined from hidden Markov models (including the states directed walk, encamped, and meandering) suggested that released calves differed from wild elephants. The first two cohorts of calves spent a significantly greater proportion of time in the directed walk state and a significantly lower proportion of time in the encamped state relative to the wild elephants. Our results represent a step forward in describing the movements of elephant orphan calves released to the wild following a period of profound social disruption when they lost their natal family and were rehabilitated with other orphan calves under human care. We discuss the implications of the elephant behavior we observed for improving release procedures and for defining success benchmarks for translocation projects.
Introduction
Translocated wild animals face challenges once they are released: they must explore their new environment; learn where to find food, water, and refuge; how to find or avoid conspecifics; how to avoid or evade threats like predators (including humans); and how resources and threats may change seasonally. As they gain more knowledge about resources in the landscape and become more comfortable with their surroundings, they may adjust their movement patterns (Berger-Tal and Avgar, 2012; Berger-Tal and Saltz, 2014). Research in translocated populations has provided support for this idea. In reintroduced scimitar-horned oryx (Oryx dammah) in Chad, dispersal and home range establishment were correlated with time at the release site, though they were strongly influenced by past experience and seasonality (Mertes, 2019). Similarly, reintroduced ‘Alalā crows (Corvus hawaiiensis) in Hawaii dispersed more from supplemental feeders as the post-release period progressed (Smetzer et al., 2021). Tracking the movement of released animals as they explore can provide insights into the time it takes to discover and use vital resources and the factors that influence this resource use. This in turn may be used to guide decisions like where, when, and in what groupings to release cohorts, as well as whether and for how long to provide supplemental resources to released populations.
Exploration of release environments and how to use resources may be facilitated by social interactions, as released individuals learn from conspecifics which areas and resources to use or avoid (Owen et al., 2017). This pattern has been found across a range of taxa. For example, pine martens (Martes martes; a solitary mustelid) released into habitat without conspecifics took longer to settle and dispersed shorter distances than a subsequent cohort released into the same area (McNicol et al., 2020); familiarity within translocation cohorts decreased dispersal distances in the solitary and territorial Stephens' kangaroo rat (Dipodomys stephensi) (Shier and Swaisgood, 2012); higher pup ratios in the local population increased post-release dispersal distances in territorial and aggregating southern sea otters (Enhydra lutris nereis) (Becker et al., 2020); and after translocation, the Alpine ibex (Capra ibex) preferentially selected sites already used by residents (Scillitani et al., 2013). Thus, regardless of the social structure of a target species, investigation into an animal's exploration of a release site should account for the social context that may structure their decisions. Managers may have control over release cohort composition or release site, and an understanding of how social context influences release site exploration may be leveraged to hasten the process of exploration that may ultimately be tied to survival.
The African savannah elephant (Loxodonta africana) is an endangered species that is translocated for different management aims across its range, including reinforcing populations in protected areas (Slotow et al., 2005), conflict mitigation (Pinter-Wollman, 2009), and release of rescued and rehabilitated individuals (McKnight, 1995; Evans et al., 2013). Where post-release monitoring has occurred, success of translocations has been mixed, with some individuals failing to settle at release sites (Pinter-Wollman, 2009; Tiller et al., in preparation1). A better understanding of the exploratory behavior of translocated elephants is relevant to managers planning and implementing translocations, as it could be leveraged in decisions aimed at facilitating settlement at release sites and minimizing vulnerability that may be associated with lack of familiarity with the landscape (Pinter-Wollman et al., 2009). Exploration had a high coefficient of variation (62%) among translocated savannah elephants to the Tsavo ecosystem in Kenya (Pinter-Wollman, 2009), suggesting strong differences among individuals in exploration tendencies. Identifying predictors of exploratory behaviors that are consistent across individuals may be useful, as may identifying individuals with disproportionate impacts on group behavior. Savannah elephants are highly mobile, dependent on widespread resources, and maintain complex movement strategies that are seasonally variable and individualistic (Wall et al., 2013; Bastille-Rousseau et al., 2020). In the semi-arid regions that many savannah elephant populations inhabit, space use is strongly influenced by the use of water points (Loarie et al., 2009; Polansky et al., 2015; Bastille-Rousseau et al., 2020); knowledge of permanent water sites is particularly important for translocated elephants during the dry season (Tiller et al., in preparation)1. Understanding the influence of ecological conditions on translocated elephant exploration may serve to hone protocols, like timing releases with ecological conditions to maximize early exploration, ensuring that release cohorts include individuals likely to be exploratory, or setting rainfall thresholds below which translocated elephants would be supplemented with water or food if elephants have not adequately explored the resources available to them.
In addition to ecological predictors of exploration, social context is known to influence elephant space use and likely influences exploratory behavior. How elephants move around the landscape is influenced by dominance-based spatial partitioning (Wittemyer et al., 2007) and by leveraging the ecological knowledge of family members and other social associates (Foley et al., 2008). The Tsavo study of translocated elephants (Pinter-Wollman et al., 2009) found strong associations among translocated elephants and decreased segregation between translocated and resident elephants over time, which may ultimately facilitate landscape exploration as naive individuals may benefit from knowledgeable individuals. Elephants are also known to make exploratory forays with changing ecological or social conditions (Thouless, 1995; Goldenberg et al., 2018). Therefore, in order to understand exploration and establishment of translocated elephants, it is critical to understand how changing ecological and social conditions at release sites influence this process, particularly related to a translocated elephant's familiarity with other individuals at the site.
Parsing the influence of social context and seasonality on the exploratory behavior of translocated elephants may provide further insight into the range and predictability of the movement patterns that elephants exhibit post-release.
In the present study, we explore the movement tracks of eight elephant calves that were rehabilitated at an elephant orphanage and subsequently released into a fenced site in northern Kenya prior to their planned hard release onto the larger, unfenced landscape. We describe their range expansion within the fenced soft release site, first visits to water points, and activity budgets, while accounting for the influence of social, ecological, and individual factors. We relate these patterns to those of tracked wild elephants at the same site to determine whether orphan space use converged with that of wild elephants. Because elephants in semi-arid environments are known to expand their range during the wet season when they are not as constrained by limited resources (Wittemyer et al., 2007; Loarie et al., 2009), we expected visits to new sites and exploratory movements to be associated with improving ecological conditions. We expected social familiarity to facilitate spatial exploration such that calves released later and those with greater interaction with wild elephants would use more areas. Finally, we expected the activity budgets of released calves to become more similar to those of the wild elephants as time progressed, reflecting less time spent in exploratory behavior over time. We discuss our results in the context of establishing management benchmarks to guide translocation projects that progress through the stages of rehabilitation, soft release, and hard release.
Methods
Study Animals and Site
The elephant calves in this study were rescued in northern Kenya following reports of distress, orphaning or abandonment. They were rehabilitated at the Reteti Elephant Sanctuary (1.11°N, 37.46°E), an elephant orphanage in the Namunyak Conservancy of the Northern Rangelands Trust. Following rescue, calves were given veterinary care, quarantined, and introduced to the other orphans. At Reteti they were fed milk from a bottle every three h, allowed to forage freely during the day, and penned at night. Between the ages of 3 and 4 years old, calves were weaned from being bottle fed and separated from the larger orphan herd in preparation for release. Ten calves were released in three cohorts (NC1 = 3, NC2 = 3, NC3 = 4) into the neighboring Sera Rhino Sanctuary within the Sera Wildlife Conservancy (1.04–1.66°N, 37.75–37.92°E) in May 2019, November 2019, and May 2020. Eight of these calves (NC1 = 3, NC2 = 2, NC3 = 3) were fitted with GPS collars prior to release and are the focus of this study.
The Sera Rhino Sanctuary is a fenced 107 km2 wildlife sanctuary within the Sera Wildlife Conservancy which was established in 2015 to support a reintroduced population of black rhinoceros (Diceros bicornis), during which time a diversity of fauna, including elephants, were fenced in in the process of creating a secure habitat for rhino. The region is semi-arid savannah and is drought prone, receiving rain during two wet seasons: April–May and November–December. The vegetation is dominated by Commiphora and Acacia species. The terrain within Sera is relatively flat with granite outcrops. There are seven permanent, mostly artificial, and several naturally occurring and seasonally ephemeral water sources distributed throughout the sanctuary, which supports a population of wild elephants which ranged between ~25–40 individuals over the course of the study as well as a range of native fauna. A complete ground count and photo-identification file was made of the wild elephants at the release site prior to the first release, from which 5 adult elephants from distinct social units (Nfemales = 4, Nmales = 1) were selected and collared in May 2019 (Table 1). This was to compare their movements with the released calves to better understand any social integration that might happen with the wild elephants. Although collared released elephants were young calves and collared wild elephants were adults, we considered this a reasonable comparison because calves of this age in the wild are typically strongly cohesive with their natal groups and would therefore be expected to demonstrate similar movement patterns to those of adults. Two of these wild elephants, Serteta and Kaingus, were later driven out of the sanctuary with their families in September 2019 by the management of the Sera Rhino Sanctuary in order to reduce the local elephant density and prevent overbrowsing.
Data Collection
Once slated for release, calves were fitted with Savannah Tracking satellite collars (http://www.savannahtracking.com/) at Reteti Elephant Sanctuary (collar deployments ranged from 2–10 weeks prior to translocation to the release site) (Table 1). Fittings were done while calves were bottle fed to minimize disturbance. Five wild elephants were selected for collaring. They were immobilized by a Kenya Wildlife Service veterinarian following standard procedures (Kenya Wildlife Service, 2018). Collars were set to collect GPS points every 30 min, with wild elephant collars switched to an hourly schedule midway through the study to extend battery life. Collars died and failed to collect subsequent GPS coordinates on different dates over the course of the study period (Table 1).
We collected vehicle-based observations of elephant aggregations as a part of a larger post-release monitoring program. When elephants were encountered when driving along the road network, observers recorded as many individuals as could be identified using individual characteristics like body and tusk shape and ear tears; an estimated group size; and whether the group was an orphan group, a bull group, a cow/calf group, or a group comprised of orphans and any resident elephants, whether females, calves, or bulls (“mixed”). Overflights were also used to locate the orphans and adults and their proximity to each other on occasion. Vehicle-based and aerial observations spanned May 2020–April 2021.
Data Analysis
We filtered tracking datasets for points outside of the fenced sanctuary, points taken prior to each cohort's release date, and biologically implausible speeds >7 km/h. We divided the study into 14-day periods, assigned GPS locations to these periods, and assessed movement metrics within each period. In order to investigate calf discovery of critical resources, range expansion, and convergence with wild elephant space use, movement metrics calculated per time period included visits to permanent water points, visits to 1 km2 grid cells, and time spent in discrete activity budget states. Periods were calculated relative to the first cohort's release (May 2, 2019) and ran through the end of the study period for a total of 48 periods.
We used ArcPro 2.7.3 (ESRI, Redlands, CA, USA) to create a grid consisting of 1 km2 cells, which we then clipped to the extent of the fenced sanctuary. Each grid square was then assigned a number and overlaid with the points from the GPS collars.
We mapped permanent water sources using ArcPro, which were verified by field observers. We focused on permanent water sources because knowledge and use of permanent water points is critical to translocated elephants during dry periods (Tiller et al., in preparation)1. We then digitized the boundary of each mapped water source based on aerial imagery and drew a 150-m buffer around each polygon. The points from GPS collars that fell within the buffer were extracted and considered a visit to that water point. For water points and grid squares, we extracted both the total number used and the number of unique sites used per 14-day time period. Total numbers of water points and grid squares used per time period were compared between released calves and wild elephants using Wilcoxon rank sum tests, treating all released calves pooled in one grouping compared to the five wild elephants pooled.
To test our expectations that improving ecological conditions and social familiarity would influence released calf exploration of the sanctuary, we conducted two generalized linear mixed effects models with the number of new grid squares visited in a 14-day period and the number of new water points visited in a 14-day period as the response variables, respectively. Response variables were modeled with a negative binomial distribution. We included a random effect for calf identity. Covariates in each of these models reflected our expectations that exploration would be influenced by time, season, and social context: the number of 14-day time periods since the calf was released (“time since release”); which of the three cohorts the calf belonged to (“cohort”); the mean normalized difference vegetation index (NDVI) over the 14-day period derived from MOD13Q1 satellite imagery at 250 m resolution (https://lpdaac.usgs.gov/products/mod13q1v006/) and averaged across the fenced sanctuary as a proxy for ecological conditions, which were extracted using the MODISTools (Tuck et al., 2014) and raster (Hijmans and van Etten, 2012) packages in R v.4.0.4 (R Core Team, 2018); the proportion of vehicle-based observations during which the calf was observed with resident elephants (Range Nobs/calf = 41–86), similar to that used in another African elephant translocation study (Pinter-Wollman et al., 2009) (“co-occurrence”); and calf sex (“sex”). Models were conducted in the glmmTMB package (Magnusson et al., 2017) after standardizing non-binary predictor variables. We checked model residual diagnostics using 1,000 simulated residuals in the DHARMa package to ensure models were properly specified (Hartig, 2021).
To assess whether activity budgets changed over time and converged with wild elephants, we segmented movement tracks by behavioral classifications. For each individual movement path k, we used hidden Markov models (Morales et al., 2004) to estimate the latent behavioral state mode bi,t,k for each observation i at time t using log speed and turning angle parameters. Polansky et al. (2015) identified three distinct states in elephant movement based on these two metrics, which we adapted for our dataset: S1—“Encamped” characterized by slow speeds and high tortuosity associated with localized foraging and resting, S2—“meandering” characterized by moderate speeds and meandering directions associated with active foraging, and S3—“directed walk” characterized by high speeds and directional travel associated with dispersal behaviors. Speed and turning angle are calculated from successive GPS fixes, so that accurate estimates of the latent states requires temporally regular GPS fixes (McClintock et al., 2012). To ensure a regular fix rate, we sampled fixes at a standard hourly fix rate across all collars and set a threshold to exclude individuals with >5% missing fixes, though none of the tracking datasets met that threshold necessitating exclusion. Model fitting and evaluation was implemented using the momentuHMM package for R, which uses maximum likelihood estimation of the transition matrix (McClintock and Michelot, 2018). Proportion of time in each of the three states summed to one, as all GPS points were assigned to one of the three states.
Following model fitting, we used the Viterbi algorithm to estimate a behavioral state for each GPS location. Behavioral state estimates were used to construct state-level activity time budgets, defined as the percentage of fixtures in a behavioral state over time, for the full dataset and for each 14-day period. To compare activity between each release cohort and the wild elephants, we conducted generalized linear models with the activity budgets for each 14-day period as the response variable. A separate model was fitted for each state, using the cohort ID (including wild elephants as a distinct cohort) and 14-day period as covariates. We set the wild elephants as the cohort reference level for each model to assess differences relative to them.
Results
All release cohorts continued to discover new permanent water points and access new grid squares throughout the study period, though there were distinct differences among cohorts (Figure 1). By the end of the study period, only the first cohort had visited all seven water points, and the third cohort had visited the fewest. The first cohort took longer to visit more than one water site than the subsequent two cohorts, a pattern that was also apparent in the grid analysis (Figure 2). In contrast, the second cohort, and to a lesser extent the third cohort, visited more water sites and areas earlier after being released.
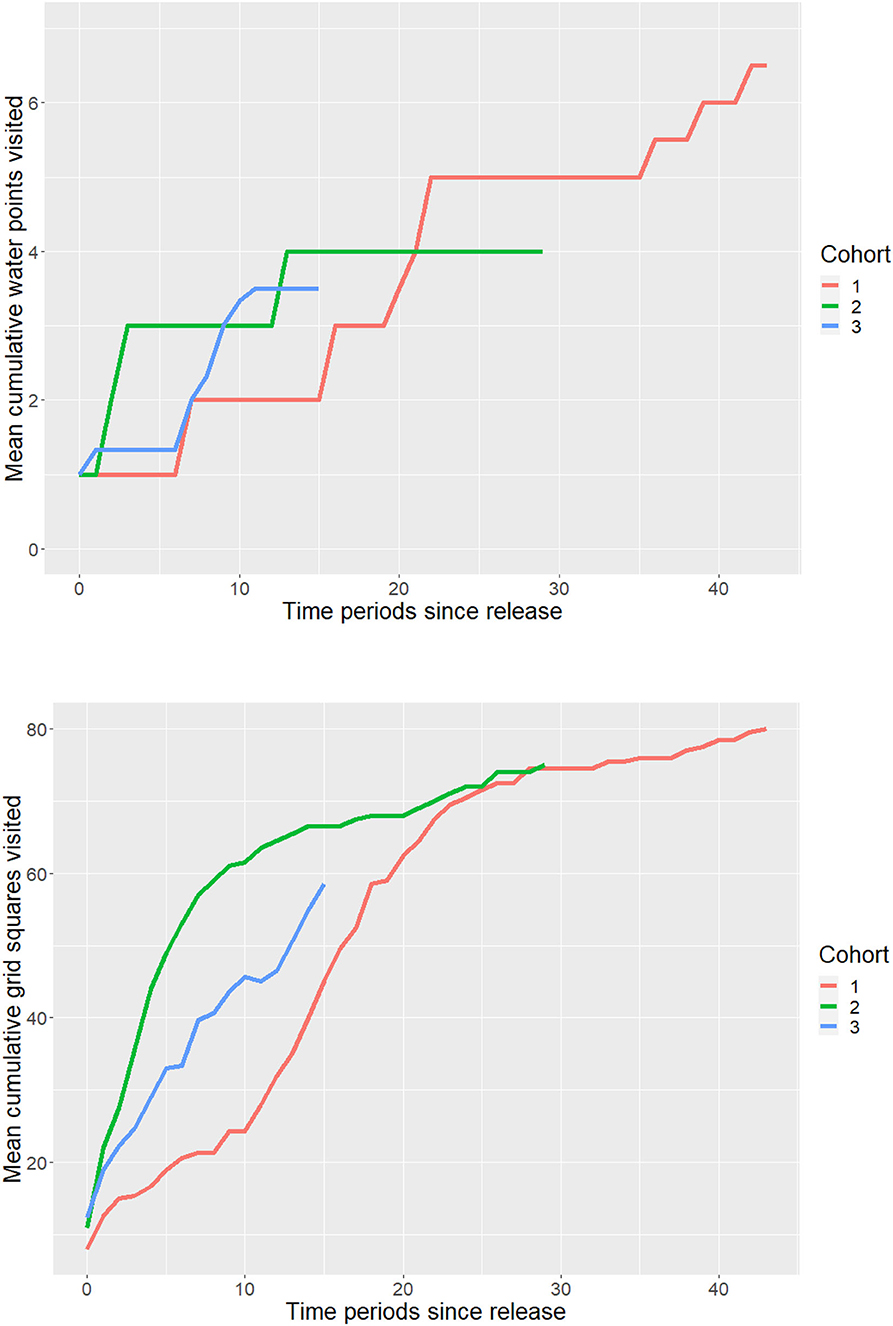
Figure 1. Within-cohort mean number of cumulative water points (top) and grid squares (bottom) accessed by released calves. Means were calculated for each 14-day time period post-release.
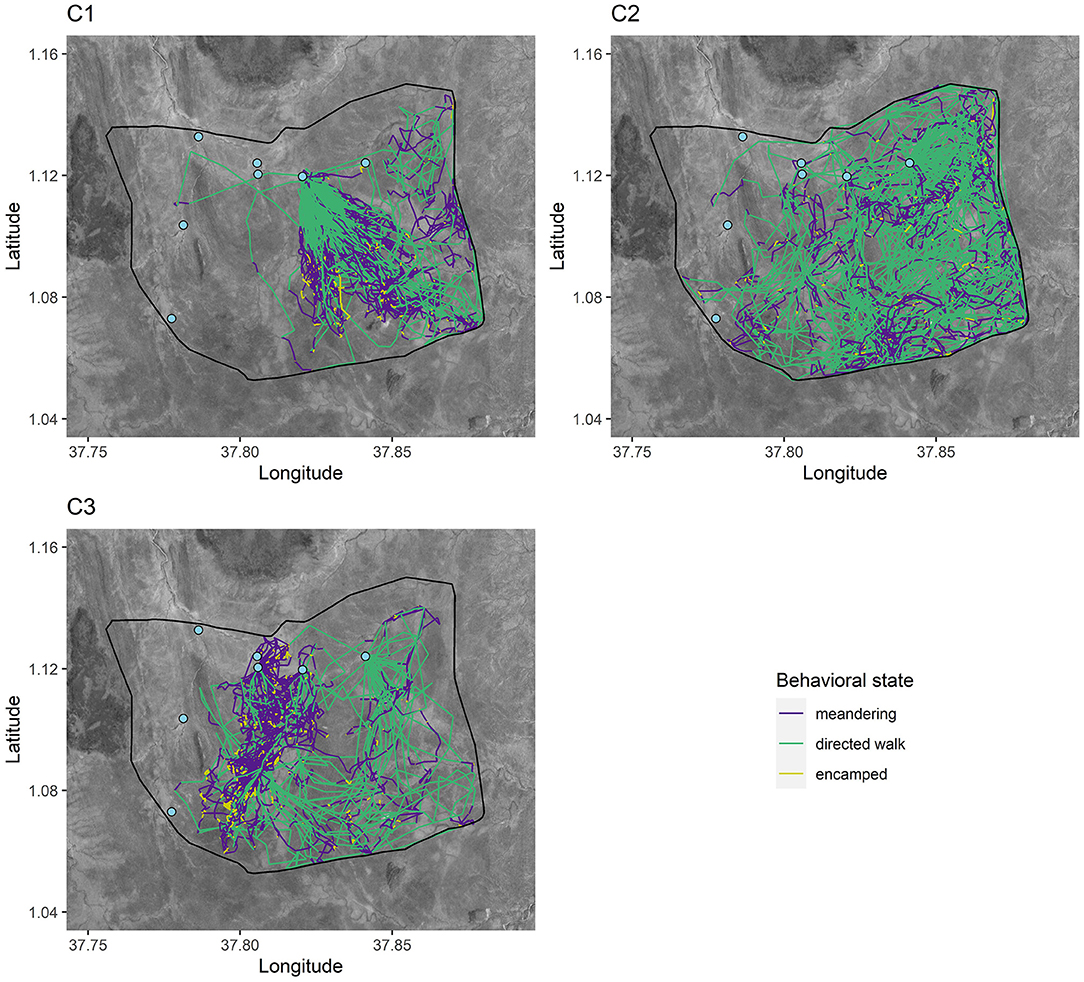
Figure 2. GPS tracks for the first 6 months post-release for a representative member of each release cohort. Tracks are categorized by behavioral state. The fenced soft release site is outlined in black and permanent water points are represented as light blue points.
The median (IQR) number of grid squares used per 14-day period differed by cohort: C1 = 9 (8–12); C2 = 10 (9–11); C3 = 8 (6–10); wild = 11 (10–12), with release cohorts tending to use a smaller number of grid squares per time period than wild elephants (Wilcoxon rank-sum test: W = 7859, p < 0.05), though interquartile ranges overlapped (Figure 3). Similarly, release cohorts used fewer of the seven permanent water points per 14-day period relative to wild, with some overlap in interquartile ranges: C1 = 1 (1–2); C2 = 1 (1–1); C3 = 1 (1–2); wild = 2 (1–4) (Wilcoxon rank-sum test: W = 7205.5, p < 0.05).
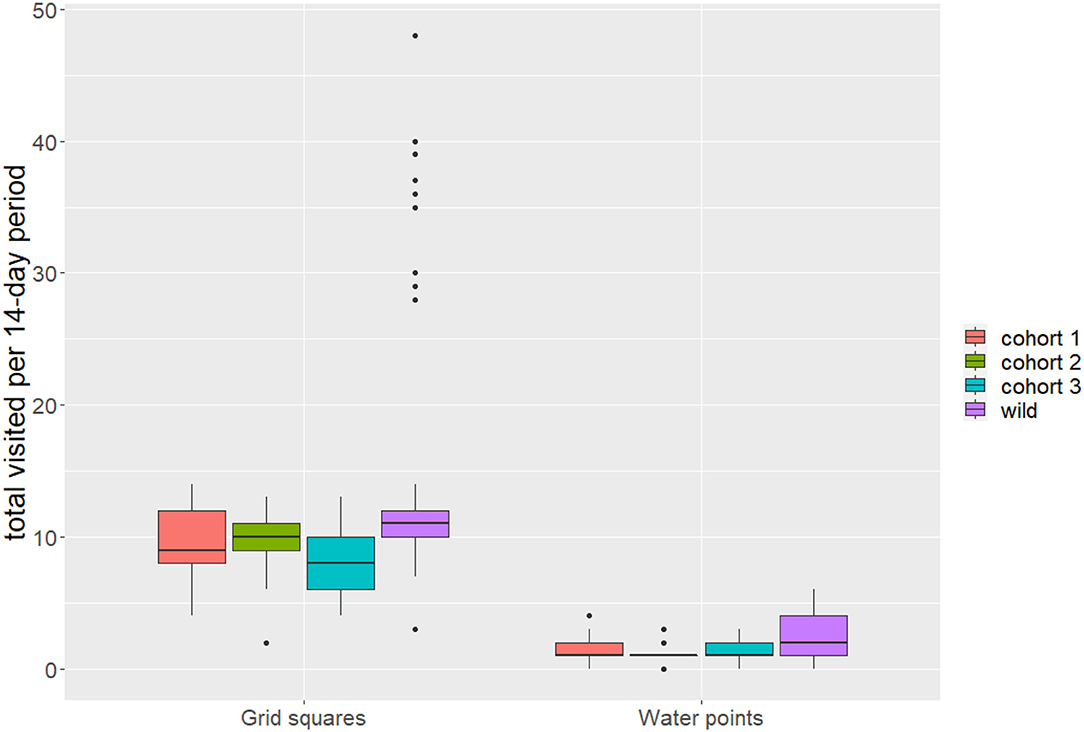
Figure 3. Distribution of total 1 km2 grid squares and water points used per 14-day period. Bold horizontal lines represent median values, boxes represent interquartile ranges, vertical lines extend to the highest and lowest points within 1.5 times the interquartile range, and points represent outliers.
Predictor variables in models showed wide variation, with mean NDVI per time period ranging between 0.197–0.618 and the proportion of observations during which released calves were recorded with wild elephants ranging across calves from 0.063–0.978. Examination of residual diagnostics for generalized linear models investigating the number of new grid squares or new water sites visited by calves per 14-day period indicated that models were correctly specified (grid: pKS_test = 0.27; pdispersion_test = 0.82; poutlier_test = 1; water: pKS_test = 0.42; pdispersion_test = 0.21; poutlier_test = 1). Models revealed NDVI and time since release to be strong predictors of both response variables, with calves visiting more new squares and water sites during greener periods (βNDVI_grid = 0.41, 95% CI[0.31, 0.50]; βNDVI_water = 0.39, 95% CI[0.06, 0.71]) and earlier post-release (βtime_grid = −0.85, 95% CI[−1.00, −0.69]; βtime_water = −0.54, 95% CI[−1.01, −0.07]) (Figure 4). Additionally, more first visits to grid squares were associated with social facilitation, with later cohorts (βcohort_grid = 0.51, 95% CI[0.21, 0.81]) and higher proportions of observations co-occurring with wild elephants (βco−occurrence_grid = 0.40, 95% CI[0.14, 0.66]) significantly predictive of more new grid squares visited.
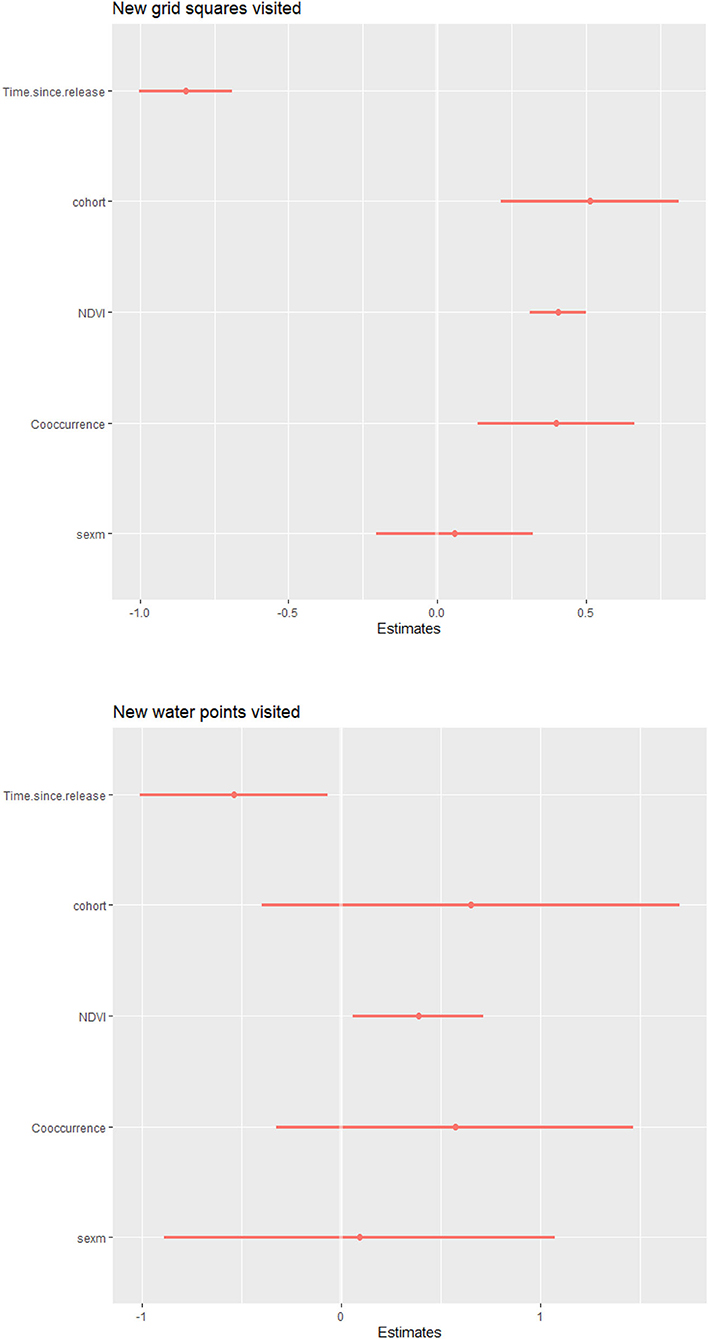
Figure 4. Results for negative binomial models predicting new grid squares visited by 14-day time period (top) and new water points visited by 14-day period (bottom). Earlier time periods post-release, being in a later cohort, higher mean NDVI, and higher proportions of observations sighted with wild elephants were the covariates associated with a greater number of grid squares visited. Earlier time periods post-release and higher mean NDVI were associated with a greater number of water points visited.
From the hidden Markov model, we classified behavioral states and constructed activity budgets from 123,054 GPS locations. C1 and C2 spent more overall time in directed walk movements compared to C3 and less time in encamped movements, while C3 had the most similar activity to the wild group (Figure 5). Directed walk movements appeared to be used for accessing water points and exploratory movements around the sanctuary, which helps explain why C1 and C2 had similar activity budgets but explored the sanctuary at different rates (Figure 2; Supplementary Material Video 1). Over time, models investigating the relationship between released and wild elephant activity across the 14-day periods indicated small trends in overlap of state-level activity (Figures 5, 6). C1 and C2 spent less time in encamped movements than the wild elephants (βC1 = −0.05, 95% CI[−0.08, −0.03]; βC2 = −0.05, 95% CI[−0.08, −0.02]), and more time in directed walk (βC1 = 0.07, 95% CI[0.02, 0.11]; βC2 = 0.07, 95% CI[0.02, 0.12]). In contrast, C3 spent significantly less time in directed walk movements than the wild elephants (βC3 =-0.08, 95% CI[−0.14, −0.02]), which was similarly reflected in their slower exploration of new grid squares.
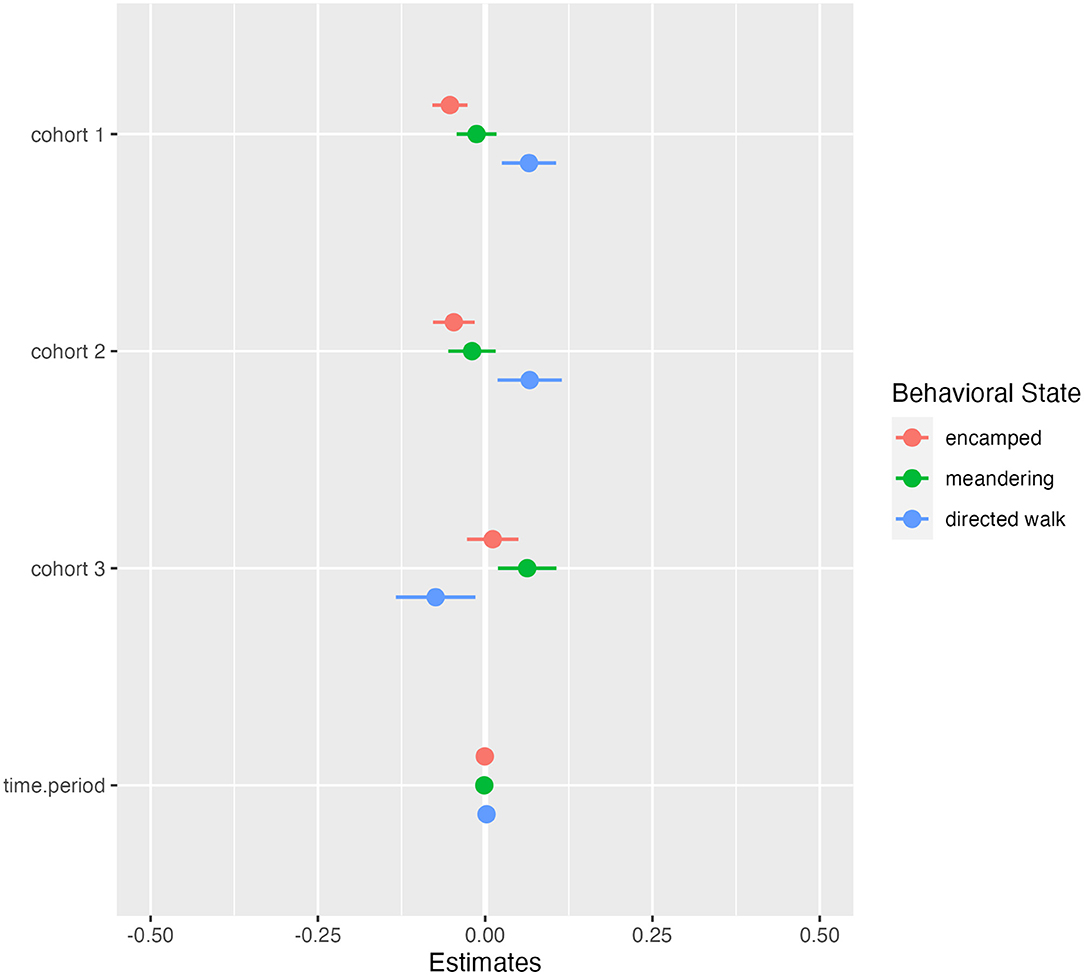
Figure 5. Results for linear regression models predicting difference in state-level activity budgets for each cohort compared to the wild elephants over time. Color corresponds to the behavioral state (pink = encamped, green = meandering, blue = directed walk). Negative estimates indicate less time spent in a state compared to the wild elephants, and positive estimates indicate more time spent compared to the wild elephants. Bars indicate 95% confidence intervals and are significant when they do not overlap zero.
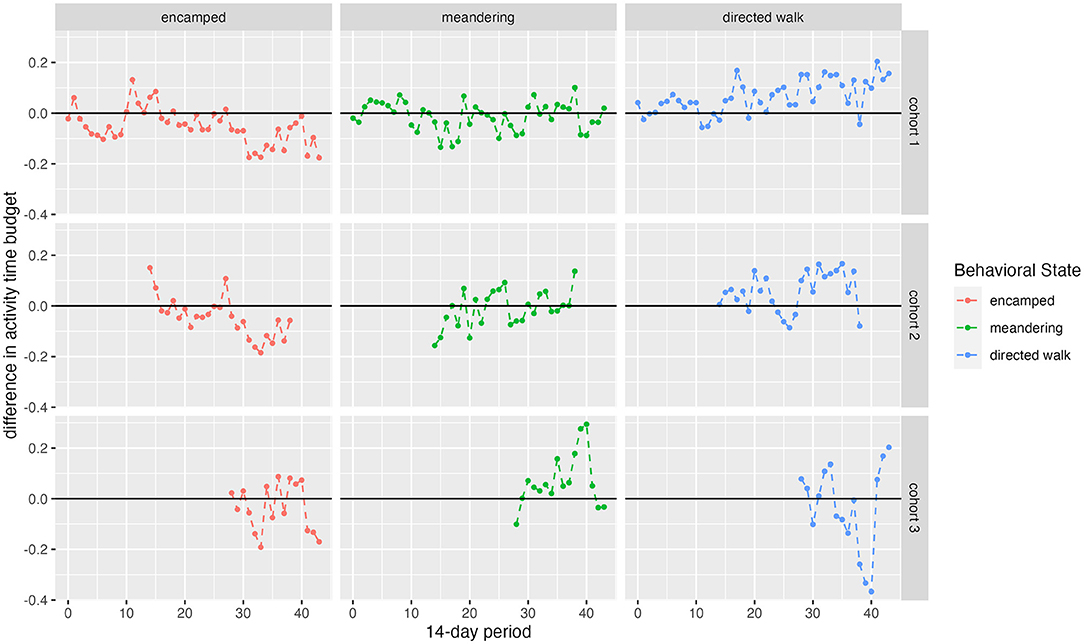
Figure 6. Difference in activity budget plots by cohort/wild. Points closer to the bold line indicate more overlap with wild elephants.
Discussion
Understanding the exploration patterns of translocated wildlife following release into a novel environment may provide managers with information to define project milestones, cohort release composition and timing, and resource supplementation, among other management options. Elephants have been a particularly challenging taxon to translocate, and thus investigation into their exploration patterns in novel environments post-release may provide insight that improves conservation resource allocation (Pinter-Wollman, 2009; Fernando et al., 2012; Tiller et al., in preparation1). Our analysis of the movement patterns of eight elephant calves that were soft released into a large fenced wildlife sanctuary paired with the movement of five wild elephants in the site provides new insight into elephant space use following translocation. We found ecological and social conditions to be strongly predictive of released calf exploration of a novel landscape, and did not find support for convergence in activity budgets between translocated and resident elephants over the time period examined (up to 20 months post-release). Additionally, we found substantial differences among release cohorts in movement behavior.
Ecological conditions were strongly predictive of exploration of the soft release site, both for initial visits to permanent water points and area (1 km2 grid squares). This is consistent with studies of wild savannah elephants indicating that home ranges expand during the wet season when proximity to permanent water is no longer limiting (Redfern et al., 2003; Young et al., 2009; Wall et al., 2021). To date, elephant translocations into Sera Wildlife Conservancy have been timed to coincide with rains and favorable ecological conditions. Our results highlight the importance of those favorable periods in facilitating landscape exploration while primary productivity is high and water is not limiting. If there is a lag in cohort exploration, as was the case for the first cohort released, discovery of new areas and water sources may not occur until subsequent wet seasons when elephants are more likely to make exploratory forays or associate in larger aggregations that may facilitate exploration of new areas. This may translate to greater concentrations of translocated elephants around the release site through at least the first dry season, with implications for the forage available to elephants and other fauna. Additionally, acquiring knowledge of permanent water sources early on post-release is particularly important for translocated elephants in arid environments. In a study comparing the tracks of translocated and resident elephant bulls in the Tsavo ecosystem in southern Kenya, Tiller et al. (in preparation)1 documented the challenges for naive individuals during the dry season when familiar seasonal water points dried up. Thus, management that facilitates elephant discovery of permanent water points in semiarid systems earlier post-release should be prioritized in these systems (e.g., releasing elephants near concentrations of permanent water). In turn, as data are collected post-release they may be used to gauge the degree of success that individuals have shown in accessing key resources. For example, evidence of updated behavior (Berger-Tal and Saltz, 2014; Smetzer et al., 2021) like exploration pulses following initial adjustment periods may reflect learning and integration of new spatial information (e.g., steeper slopes in cumulative area plots; Figure 1), and changes in movement that track changes in ecological conditions may indicate greater exploitation of dynamic landscapes (e.g., the significance of variables related to rainfall or vegetation predicting exploration behavior; Figure 4). This may be particularly informative for determining when to transition animals from soft to hard release as soft release sites may function as “training grounds” that allow released animals to gain local knowledge and to develop the necessary skills to search for resources effectively.
As expected, social familiarity was correlated with more new grid squares visited per 14-day period. Calves that associated more with wild elephants and those released later ranged into more new areas. This pattern suggests an important role for social context in facilitating and hastening landscape exploration. Movement in elephants is known to be related to established social relationships (Wittemyer et al., 2007; Foley et al., 2008) and it should therefore be expected that the social relationships of translocated elephants or lack thereof may influence the extent to which they explore their new surroundings (Goldenberg et al., 2019). There were clear distinctions in social context among the cohorts released: the first cohort was completely unfamiliar with any elephants at the soft release site, whereas the second and third cohorts were familiar with all individuals previously resident to the Reteti Elephant Sanctuary during their rehabilitation period. Thus, learning about the novel landscape from existing relationships with knowledgeable individuals was not an option for the first cohort until they established relationships with residents, a process that may take time in elephants (Goldenberg and Wittemyer, 2017). This distinction among cohorts may explain the relatively slow speed at which the first cohort visited new sites when compared to subsequent cohorts. Subsequent cohorts had the advantage of familiarity with knowledgeable individuals (Goldenberg et al., in revision)2, which may have facilitated their earlier exploration of the sanctuary.
There is also evidence that not only familiarity, but close bonding between individuals in distinct cohorts, may be leveraged when determining cohort composition to achieve management aims, which we investigate in depth elsewhere (Goldenberg et al., in revision)2. Many translocation studies, including those of elephants (Pinter-Wollman, 2009; Fernando et al., 2012) and other taxa (Flanagan et al., 2016; Berger-Tal et al., 2020), have documented long range linear movements and cases where animals reject the release sites, by leaving and sometimes attempting to return to their original location. While the fencing at the soft release site in this study precludes homing, fencing may not be possible or practical in other locations. Our results suggest that familiarity and bonding with knowledgeable individuals at release sites is one way of encouraging site exploration, rather than site rejection. While the significance of these results for elephant translocation projects is clear, we note that established social relationships across a diverse range of taxa may improve conservation translocation outcomes (Shier, 2006; Shier and Swaisgood, 2012).
In our analysis, sex was not predictive of exploration behavior in released cohorts. In their study of bull elephants translocated into the Tsavo ecosystem, Tiller et al. (in preparation)1 suggested that younger bulls may be more successful in adjusting to release sites because younger bulls naturally undergo periods of exploration as they disperse from their families and learn their place within bull society. Studies of wild orphaned elephants suggest variability among individuals in their ability to integrate with new groups, with some females demonstrating dispersal behavior more typically associated with males (Goldenberg et al., 2016; Goldenberg and Wittemyer, 2017; Parker et al., 2021). The sample size represented in the present study is small; it will be worthwhile to revisit whether males are more exploratory post-release than females in future work and how released male calf behavior compares with that of young, dispersing wild bulls, of which only one was collared in this study. Additionally, the released population in this study is unique among the literature on elephant translocations in that calves are young (~four years old) when they are translocated to the soft release site. Whether or not being released at a young age affects integration into wild herds and subsequent landscape exploration and convergence with wild elephant movement behavior warrants further study. Other individual characteristics in addition to age and sex that may be measured pre-release and potentially tied to exploratory movement post-release, like leadership and curiosity, may be worth investigating in the future.
Comparison of wild and translocated elephant movement revealed distinct differences, suggesting that released calves had not yet converged with wild elephant movement patterns over the time period analyzed. Wild elephants on average used more grid squares and more permanent water points per 14-day period than calves in release cohorts (Figure 3), and activity budgets suggested differences between the translocated and wild groups in the proportion of time allocated to each movement state (Figure 6). These results contrast with Pinter-Wollman et al. (2009), who found activity budgets between translocated and resident elephants based on observational data to converge over <one year post-release. This difference across studies may be attributable to the different pre-release experiences of the populations under study. Whereas Pinter-Wollman et al. (2009) studied wild elephant families and bulls that were translocated from one region to another, this study involves calves that were rescued and rehabilitated under human care and thus had limited experience in wild landscapes or moving at night. This highlights the additional challenges faced by orphaned wildlife raised partly under human care prior to release, and underscores the importance of extended learning periods prior to hard release. Regardless of the specific circumstances of translocated individuals, convergence in activity budgets with wild elephants (e.g., differences between activity proportions in released and wild animals trending toward zero; Figure 6) may serve as benchmarks to gauge individual competency post-release.
Despite the general mismatch between resident and released calf activity budgets, there were notable differences across cohorts, indicating different degrees of exploration. The first and second cohorts spent less time encamped and meandering (corresponding approximately to resting and foraging) and more time in directed walk than the third cohort and the wild residents (Figure 5). The directed walk state is often associated with targeted movement toward water points or prospecting behavior (Figure 2). This distinction among cohorts therefore suggests that the third cohort has not yet exhibited the same extent of exploration as the first and second cohorts. Translocations of other species have documented delays in exploration behavior. For example, reintroduced ‘Alalā crows made longer exploratory movements as time post-release progressed (Smetzer et al., 2021), and reintroduced European bison (Bison bonasus) took approximately 10 days to exhibit exploratory behavior following release (Schmitz et al., 2015). Drivers of such behavior, and particularly differences between cohorts, remain unknown.
Conservation translocations are high risk endeavors with the potential for significant conservation benefits. Understanding how individuals navigate post-release environments to obtain the resources necessary to survive and contribute to wild populations is critical to support such endeavors. The exploratory behavior of released savannah elephant calves investigated here provides insight into the strong influence of seasonality and social familiarity on resource use post-release, and the extended period over which managers should expect released calves to demonstrate activity budgets similar to wild elephants. Further, analyses like these may be useful as guides to gauge benchmarks of individual competency, like periods characterized by pulses in exploration, movement that tracks changing ecological conditions, and convergence of activity budgets with wild elephants, which may be a particularly important focus for animals that have spent time under human care. Whether or not such benchmarks correlate with the long-term survival and success of calves is yet to be determined. As noted throughout the literature on conservation translocations (IUCN/SSC, 2013; Berger-Tal et al., 2020), sustained monitoring over several years in an adaptive framework is needed to best guide the challenging decisions translocation managers are tasked with making to maximize conservation benefit and welfare. Our study contributes to this important literature to elucidate the post-release behavior of rehabilitated young orphans in a highly mobile and social species.
Data Availability Statement
The datasets presented in this article are not readily available because elephant tracking data are sensitive due to high rates of illegal killing of elephants in the region, and the data are therefore not archived. Requests to access the datasets should be directed to sgoldenberg@sdzwa.org.
Ethics Statement
The animal study was reviewed and approved by San Diego Zoo Wildlife Alliance IACUC committee.
Author Contributions
SG wrote the first draft of the manuscript. SG, NH, and JS-D performed the statistical analyses. NH and JS-D wrote sections of the manuscript. SC, DD, ID-H, RL, ML, LL, FO, and FP contributed to acquisition/maintenance of the data. ID-H, FP, CT, GW, and MO revised the manuscript. All authors contributed to conception, design of the study, contributed to the article, and approved the submitted version.
Funding
Donors to Save the Elephants and San Diego Zoo Wildlife Alliance funded this work.
Conflict of Interest
The authors declare that the research was conducted in the absence of any commercial or financial relationships that could be construed as a potential conflict of interest.
Publisher's Note
All claims expressed in this article are solely those of the authors and do not necessarily represent those of their affiliated organizations, or those of the publisher, the editors and the reviewers. Any product that may be evaluated in this article, or claim that may be made by its manufacturer, is not guaranteed or endorsed by the publisher.
Acknowledgments
We thank the Office of the President of Kenya for permission to conduct this work, and to the Sera Wildlife Conservancy for facilitating this research. We thank the staff at the Reteti Elephant Sanctuary, Katie Rowe, and Jeremy Bastard for elephant care and support of pre-release collaring. We are indebted to Save the Elephants for the ground identification and air surveys, and to KWS for veterinary oversight on the immobilization operations. We are grateful to Jenna Parker and Nelson Mwangi for field support and data management, and Toby Dunn for piloting the helicopter for immobilization.
Supplementary Material
The Supplementary Material for this article can be found online at: https://www.frontiersin.org/articles/10.3389/fcosc.2021.720202/full#supplementary-material
Footnotes
1. ^Tiller, L. N., King, L. E., Ouma-Okita, B., Lala, F., Pope, F., Douglas-Hamilton, I., et al. (in preparation). The behaviour and fate of translocated bull African elephants (Loxodonta africana) into a novel environment.
2. ^Goldenberg, S. Z., Chege, S. M., Mwangi, N., Craig, I., Daballen, D., Douglas-Hamilton, I., et al. (in revision). Social integration of translocated wildlife: a case study of rehabilitated and released elephant calves in northern Kenya. Mamm. Biol.
References
Bastille-Rousseau, G., Wall, J., Douglas-Hamilton, I., Lesowapir, B., Loloju, B., Mwangi, N., et al. (2020). Landscape-scale habitat response of African elephants shows strong selection for foraging opportunities in a human dominated ecosystem. Ecography 43, 149–160. doi: 10.1111/ecog.04240
Becker, S. L., Nicholson, T. E., Mayer, K. A., Murray, M. J., and Van Houtan, K. S. (2020). Environmental factors may drive the post-release movements of Surrogate-Reared Sea Otters. Front. Mar. Sci. 7, 539904. doi: 10.3389/fmars.2020.539904
Berger-Tal, O., and Avgar, T. (2012). The glass is half-full: overestimating the quality of a novel environment is advantageous. PLoS ONE 7:10. doi: 10.1371/journal.pone.0034578
Berger-Tal, O., Blumstein, D. T., and Swaisgood, R. R. (2020). Conservation translocations: a review of common difficulties and promising directions. Anim Conserv 23, 121–131. doi: 10.1111/acv.12534
Berger-Tal, O., and Saltz, D. (2014). Using the movement patterns of reintroduced animals to improve reintroduction success. Curr. Zool. 60, 515–526. doi: 10.1093/czoolo/60.4.515
Evans, K., Moore, R., and Harris, S. (2013). The social and ecological integration of captive-raised adolescent male african elephants (Loxodonta africana) into a wild population. PLoS ONE 8, 18–21. doi: 10.1371/journal.pone.0055933
Fernando, P., Leimgruber, P., Prasad, T., and Pastorini, J. (2012). Problem-elephant translocation: translocating the problem and the elephant? PLoS ONE 7:e50917. doi: 10.1371/journal.pone.0050917
Flanagan, S. E., Brown, M. B., Fennessy, J., and Bolger, D. T. (2016). Use of home range behaviour to assess establishment in translocated giraffes. Afr. J. Ecol. 54, 365–374. doi: 10.1111/aje.12299
Foley, C., Pettorelli, N., and Foley, L. (2008). Severe drought and calf survival in elephants. Biol. Lett. 4, 541–544. doi: 10.1098/rsbl.2008.0370
Goldenberg, S. Z., Douglas-Hamilton, I., and Wittemyer, G. (2016). Vertical transmission of social roles drives resilience to poaching in elephant networks. Curr. Biol. 26, 75–79. doi: 10.1016/j.cub.2015.11.005
Goldenberg, S. Z., Douglas-Hamilton, I., and Wittemyer, G. (2018). Inter-generational change in African elephant range use is associated with poaching risk, primary productivity and adult mortality. Proc. R. Soc. B. Biol. Sci. 285:20180286. doi: 10.1098/rspb.2018.0286
Goldenberg, S. Z., Owen, M. A., Brown, J. L., Wittemyer, G., Oo, Z. M., and Leimgruber, P. (2019). Increasing conservation translocation success by building social functionality in released populations. Global Ecol. Conserv. 18:e00604. doi: 10.1016/j.gecco.2019.e00604
Goldenberg, S. Z., and Wittemyer, G. (2017). Orphaned female elephant social bonds reflect lack of access to mature adults. Sci. Rep. 7:14408. doi: 10.1038/s41598-017-14712-2
Hartig, F. (2021). DHARMa: Residual Diagnostics for Hierarchical (Multi-Level/Mixed) Regression Models. Available online at: http://florianhartig.github.io/DHARMa/ (accessed April 6, 2021).
Hijmans, R. J., and van Etten, J. (2012). raster: Geographic analysis and modeling with raster data. Available at: http://CRAN.R-project.org/package=raster (accessed April 6, 2021).
IUCN/SSC (2013). Guidelines for Reintroductions and Other Conservation Translocations. Gland: IUCN Species Survival Commission.
Kenya Wildlife Service (2018). Immobilization and Translocation Protocol for the African elephant (Loxodonta africana) in Kenya (2018). Available online at: http://kws.go.ke/Downloads (accessed May 26, 2021).
Loarie, S. R., Aarde, R. J. V., and Pimm, S. L. (2009). Fences and artificial water affect African savannah elephant movement patterns. Biol. Conserv. 142, 3086–3098. doi: 10.1016/j.biocon.2009.08.008
Magnusson, A., Skaug, H. J., Nielsen, A., Berg, C. W., Kristensen, K., Maechler, M., et al (2017). glmmTMB: Generalized Linear Mixed Models Using Template Model Builder. Available online at: https://github.com/glmmTMB (accessed April 6, 2021).
McClintock, B. T., King, R., Thomas, L., Matthiopoulos, J., McConnell, B. J., and Morales, J. M. (2012). A general discrete-time modeling framework for animal movement using multistate random walks. Ecol. Monogr. 82, 335–349. doi: 10.1890/11-0326.1
McClintock, B. T., and Michelot, T. (2018). momentuHMM: R package for generalized hidden Markov models of animal movement. Methods Ecol. Evol. 9, 1518–1530. doi: 10.1111/2041-210X.12995
McKnight, B. L. (1995). Behavioural ecology of “hand-reared” African elephants (Loxodonta africana (Blumenbach)) in Tsavo East National Park, Kenya. Afr. J. Ecol. 33, 242–256. doi: 10.1111/j.1365-2028.1995.tb00802.x
McNicol, C. M., Bavin, D., Bearhop, S., Bridges, J., Croose, E., Gill, R., et al. (2020). Postrelease movement and habitat selection of translocated pine martens Martes martes. Ecol. Evol. 10, 5106–5118. doi: 10.1002/ece3.6265
Mertes, K. (2019). Management background and release conditions structure post-release movements in reintroduced ungulates. Front. Ecol. Evol. 7:14. doi: 10.3389/fevo.2019.00470
Morales, J. M., Haydon, D. T., Frair, J., Holsinger, K. E., and Fryxell, J. M. (2004). Extracting more out of relocation data: building movement models as mixtures of random walks. Ecology 85, 2436–2445. doi: 10.1890/03-0269
Owen, M. A., Swaisgood, R. R., and Blumstein, D. T. (2017). Contextual influences on animal decision-making: significance for behavior-based wildlife conservation and management. Integr. Zool. 12, 32–48. doi: 10.1111/1749-4877.12235
Parker, J. M., Webb, C. T., Daballen, D., Goldenberg, S. Z., Lepirei, J., Letitiya, D., et al. (2021). Poaching of African elephants indirectly decreases population growth through lowered orphan survival. Curr. Biol. doi: 10.1016/j.cub.2021.06.091. [Epub ahead of print].
Pinter-Wollman, N. (2009). Spatial behaviour of translocated African elephants (Loxodonta africana) in a novel environment: using behaviour to inform conservation actions. Behaviour 146, 1171–1192. doi: 10.1163/156853909X413105
Pinter-Wollman, N., Isbell, L. A., and Hart, L. A. (2009). The relationship between social behaviour and habitat familiarity in African elephants (Loxodonta africana). Proc. R. Soc. B. 276, 1009–1014. doi: 10.1098/rspb.2008.1538
Polansky, L., Kilian, W., and Wittemyer, G. (2015). Elucidating the significance of spatial memory on movement decisions by African savannah elephants using state-space models. Proc. R. Soc. B. Biol. Sci. 282, 20143042–20143042. doi: 10.1098/rspb.2014.3042
R Core Team (2018). R: A language and environment for statistical computing. Vienna: R Foundation for Statistical Computing Available onlin at: https://www.R-project.org/ (accessed April 6, 2021).
Redfern, J. V., Grant, R., Biggs, H., and Getz, W. M. (2003). Surface-water constraints on herbivore foraging in the Kruger National Park, South Africa. Ecology 84, 2092–2107. doi: 10.1890/01-0625
Schmitz, P., Caspers, S., Warren, P., and Witte, K. (2015). First steps into the wild – exploration behavior of European Bison after the first reintroduction in Western Europe. PLoS ONE 10:e0143046. doi: 10.1371/journal.pone.0143046
Scillitani, L., Darmon, G., Monaco, A., Cocca, G., Sturaro, E., Rossi, L., et al. (2013). Habitat selection in translocated gregarious ungulate species: an interplay between sociality and ecological requirements. J. Wildlife Manage. 77, 761–769. doi: 10.1002/jwmg.517
Shier, D. M. (2006). Effect of family support on the success of translocated black-tailed prairie dogs. Conserv. Biol. 20, 1780–1790. doi: 10.1111/j.1523-1739.2006.00512.x
Shier, D. M., and Swaisgood, R. R. (2012). Fitness costs of neighborhood disruption in translocations of a solitary mammal. Conserv. Biol. 26, 116–123. doi: 10.1111/j.1523-1739.2011.01748.x
Slotow, R., Garaï, M. E., Reilly, B., Page, B., and Carr, R. D. (2005). Population dynamics of elephants re-introduced to small fenced reserves in South Africa. 35:10.
Smetzer, J. R., Greggor, A. L., Paxton, K. L., Masuda, B., and Paxton, E. H. (2021). Automated telemetry reveals post-reintroduction exploratory behavior and movement patterns of an endangered corvid, ‘Alalā (Corvus hawaiiensis) in Hawai‘i, USA. Global Ecol. Conserv. 26:e01522. doi: 10.1016/j.gecco.2021.e01522
Thouless, C. R. (1995). Long distance movements of elephants in northern Kenya. Afr. J. Ecol. 33, 321–334. doi: 10.1111/j.1365-2028.1995.tb01042.x
Tuck, S. L., Phillips, H. R. P., Hintzen, R. E., Scharlemann, J. P. W., Purvis, A., and Hudson, L. N. (2014). MODISTools - downloading and processing MODIS remotely sensed data in R. Ecol. Evol. 4, 4658–4668. doi: 10.1002/ece3.1273
Wall, J., Wittemyer, G., Klinkenberg, B., LeMay, V., Blake, S., Strindberg, S., et al. (2021). Human footprint and protected areas shape elephant range across Africa. Curr. Biol. 31, 2437–2445.e4. doi: 10.1016/j.cub.2021.03.042
Wall, J., Wittemyer, G., Klinkenberg, B., LeMay, V., and Douglas-Hamilton, I. (2013). Characterizing properties and drivers of long distance movements by elephants (Loxodonta africana) in the Gourma, Mali. Biol. Conserv. 157, 60–68. doi: 10.1016/j.biocon.2012.07.019
Wittemyer, G., Getz, W. M., Vollrath, F., and Douglas-Hamilton, I. (2007). Social dominance, seasonal movements, and spatial segregation in African elephants: a contribution to conservation behavior. Behav. Ecol. Sociobiol. 61, 1919–1931. doi: 10.1007/s00265-007-0432-0
Keywords: activity budgets, conservation behavior, conservation translocation, Loxodonta africana, rehabilitation and release, rewilding, social network
Citation: Goldenberg SZ, Hahn N, Stacy-Dawes J, Chege SM, Daballen D, Douglas-Hamilton I, Lendira RR, Lengees MJ, Loidialo LS, Omengo F, Pope F, Thouless C, Wittemyer G and Owen MA (2021) Movement of Rehabilitated African Elephant Calves Following Soft Release Into a Wildlife Sanctuary. Front. Conserv. Sci. 2:720202. doi: 10.3389/fcosc.2021.720202
Received: 03 June 2021; Accepted: 23 August 2021;
Published: 28 September 2021.
Edited by:
Katherine Moseby, University of New South Wales, AustraliaReviewed by:
David Saltz, Ben-Gurion University of the Negev, IsraelColleen Cassady St. Clair, University of Alberta, Canada
Michelle Henley, Elephants Alive (NGO), South Africa
Copyright © 2021 Goldenberg, Hahn, Stacy-Dawes, Chege, Daballen, Douglas-Hamilton, Lendira, Lengees, Loidialo, Omengo, Pope, Thouless, Wittemyer and Owen. This is an open-access article distributed under the terms of the Creative Commons Attribution License (CC BY). The use, distribution or reproduction in other forums is permitted, provided the original author(s) and the copyright owner(s) are credited and that the original publication in this journal is cited, in accordance with accepted academic practice. No use, distribution or reproduction is permitted which does not comply with these terms.
*Correspondence: Shifra Z. Goldenberg, sgoldenberg@sdzwa.org