- 1Pacific Rim Conservation, Honolulu, HI, United States
- 2Kauai`i Endangered Seabird Recovery Project, Hanapepe, HI, United States
Newell’s Shearwater (Puffinus auricularis newelli; NESH) and Hawaiian Petrel (Pterodroma sandwichensis; HAPE) are listed under the U.S. Endangered Species Act and have declined by 94% and 78%, respectively, since 1993 due to habitat degradation, predation by introduced predators, collisions with powerlines and light attraction. Given the challenges in protecting nesting birds in their rugged montane habitats, it has long been desirable to create populations of both species in more accessible locations that offer a higher level of protection. We translocated 110 HAPE and 86 NESH chicks over a six-year period from 2015-2020 to a 2.5-ha predator-free enclosure on Kaua`i, Hawai`i. In addition to invasive plant removal and native plant out-planting, we installed 76 artificial burrows to provide nesting sites. Chicks were tube fed 1-20% of their body weight daily in the form of a slurry comprised of squid, fish, salmon oil, and Pedialyte. All NESH and 96% (N=106) of HAPE survived to fledging. Eight HAPE, including three breeding pairs, and one NESH have returned as adults to the translocation site and HAPE have bred at the site, resulting in the first predator-free breeding colony of this species.
Introduction
Global biodiversity loss has galvanized the restoration of threatened and endangered species across the globe in an effort to stem those losses. Two techniques commonly used, translocation and social attraction, actively move or lure a target species to a site to restore or supplement populations and enhance ecosystem resilience (Deguchi et al., 2012; VanderWerf et al., 2022). Seabirds are one of the most threatened groups of vertebrates, with almost half of all species listed as globally threatened or near threatened with extinction by the International Union for Conservation of Nature (IUCN) (Dias et al., 2019). Based on IUCN Red List categorizations, a higher proportion of albatrosses, petrels, and storm petrels (Procellariiformes) and penguins (Sphenisciformes) are at risk of extinction than in other avian orders and thus have been the targets of many conservation interventions.
Birds in the Order Procellariiformes exhibit strong natal philopatry and high nest-site fidelity (Antaky et al., 2021). These behavioral traits, along with a protracted nesting period and ground nesting habit, result in high vulnerability to predation by introduced mammals and exploitation by humans at the breeding colonies (Jones et al., 2008; Croxall et al., 2012; Dias et al., 2019). This vulnerability has led to the extirpation of many island populations of Procellariiformes around the world and made the consequences of stochastic events such as hurricanes, volcanic eruptions, epizootics, or fires at the remaining safe breeding sites much more significant (Croxall et al., 2012; Spatz et al., 2017) since birds are unlikely to colonize new sites without intervention.
In the case of seabirds, translocation typically involves moving eggs or chicks from their natal site prior to imprinting, and hand rearing them at a new release site. Burrowing petrels and shearwaters imprint on their natal colony when they first emerge above ground, which often occurs at night, and likely involves several environmental cues, including the constellation of stars and magnetic inclination (Warham, 1990; Wynn et al., 2020). Although some studies suggest imprinting may begin while chicks are still in the burrow (Serventy et al., 1989), most burrowing seabirds can be moved later in development, just before they emerge for the first time (Miskelly et al., 2009). It is thought that removing birds earlier could result in higher rates of return when the precise imprinting date is unknown. The propensity of most Procellariiformes to congregate with other individuals of the same species is another important life history trait that facilitates colony creation through social attraction. Social attraction involves a range of tools, from acoustic playbacks to artificial decoys, scent, and mirrors, all aimed at exploiting the colonial nesting characteristics of seabirds by luring them to a new location by mimicking the existence of a colony.
Translocations involving hand-rearing of burrow-nesting Procellariids have been undertaken around the world, but particularly in New Zealand since the early 1990s (Miskelly and Taylor, 2004; Bell et al., 2005; Madeiros et al., 2012). Active seabird restoration projects (which include both social attraction and translocation) have shown a rapid increase in both the number of projects and the species targeted. A rigorous systematic review by Spatz et al. (2023) of active restoration efforts identified over 851 projects using social attraction and translocation for over 138 seabird species, which is now available as the global “Seabird Restoration Database” (2021). Translocations have been undertaken successfully for highly endangered Procellariformes including Bermuda Cahow (Pterodroma cahow) and Chatham Island Taiko (Pterodroma magentae), in which the World population numbered fewer than 100 breeding pairs (Carlile et al., 2012). Techniques have been established for these species to a level where health issues are minimal and all transferred chicks fledge at measured condition parameters similar to, or exceeding those, of parent-raised chicks (Gummer, 2020). Guidelines for the appropriateness, planning, implementation, and monitoring of such actions have been written for the Agreement on the Conservation of Albatrosses and Petrels (ACAP; Gummer, 2020) and similar guidelines were adopted by the IUCN Species Survival Commission in 2012 and updated again in 2021 (https://acap.aq/en/resources/acap-conservation-guidelines/2640-translocation-guidelines/file Downloaded 5 Aug 2021.). Transferring Procellariiformes chicks to a new colony site is just the beginning of a long process of colony establishment that depends on survival of the translocated birds, their recruitment to the new colony site, and the social attraction of other pre-breeding individuals that will accelerate the growth of the colony.
In Hawai’i, two of the three seabirds listed under the Endangered Species Act, the threatened Newell’s Shearwater (Puffinus auricularis newelli; NESH) and the endangered Hawaiian Petrel (Pterodroma sandwichensis; HAPE), are also the only endemic seabirds in Hawai’i. Both species are rapidly declining due to habitat degradation by feral ungulates (pigs (Sus scrofa), goats (Capra hircus)) and invasive exotic plants, predation by invasive feral domestic cats (Felis catus), pigs, rats (Rattus sp), and Barn Owls (Tyto alba), collisions with power lines and light attraction (Raine et al., 2020; Travers et al., 2021). Recent ornithological radar surveys, combined with grounded birds turned in to the Save Our Shearwaters (SOS) program (a seabird rehabilitation facility), show apparent declines from 1993 to 2013 of 94% for NESH and 78% for HAPE on Kaua`i (Raine et al., 2017). Protection of NESH and HAPE on their nesting grounds and reduction of collision and lighting hazards are high priority recovery actions for the species. Given the challenges in protecting nesting birds in their rugged montane habitats, however, it has long been desirable to create populations of both species in more accessible locations that offer a higher level of protection (Holmes et al., 2011).
In 2012, funding became available through several programs to create such a population at Kīlauea Point National Wildlife Refuge (KPNWR), which is home to one of the largest seabird colonies in the main Hawaiian Islands (Young et al., 2018; Felis et al., 2020). The project was named the “Nihoku Ecosystem Restoration Project” after the area on the refuge where the placement of the future colony was planned. There were four stages to this multi-faceted project: permitting and biological monitoring, fence construction, habitat restoration and predator eradication, and translocation of birds to the newly secured habitat. A predator exclusion fence was constructed at Nihoku in September 2014 and all mammalian predators were eradicated by January 2015. Habitat restoration (invasive plant weeding and native plant out-planting) was done in phases (10-15% of the project area in each year) until most of the area was restored. In addition to habitat restoration, 76 artificial burrows were installed to facilitate translocation activities (Young et al., 2018).
This paper evaluates the success rates of established benchmarks for the translocation of NESH and HAPE and reports on the techniques and outcomes used to translocate and hand-raise NESH and HAPE, and on the project outcomes after five and six years of successful translocations for each species, respectively.
Methods
Source colony searches and characteristics
Source colony searching and selection was described in Young et al. (2018). In summary, from 2012-2020, auditory surveys were conducted at suspected NESH and HAPE breeding sites to locate potential source colonies. Surveys at these sites were conducted using a standardized auditory survey protocol (Raine et al., 2017) in which trained observers listened for calls for two hours beginning at sunset and then again two hours prior to sunrise for 1.5 hours. Surveys were conducted during the peak breeding season from June-September when birds are most vocal. Surveys were accompanied by burrow searches in areas where the highest levels of ground calling activity were identified. These surveys were initially undertaken at low elevation, unmanaged colonies that were considered to have the highest threat of extirpation due to fallout, powerline collision, predation, and habitat loss. When it became clear that a suitable number of source burrows would not be located in these colonies due to rapid population declines, surveys were expanded to include high elevation and predator- managed sites.
Restoration site characteristics
Kīlauea Point National Wildlife Refuge (KPNWR), owned by the US Department of the Interior and managed by the US Fish and Wildlife Service (USFWS), is one of the few places in the main Hawaiian Islands (MHI) with high seabird species diversity and abundance, and it provides a high-island refugium for seabird populations potentially displaced by sea level rise as a result of climate change in the Northwestern Hawaiian Islands (Reynolds et al., 2015). The area on the refuge that was chosen to construct the fence, called Nihoku, was selected for its bowl-shaped topography that faces Northeast into the prevailing winds which are important to facilitate take off flights for the birds. The site is fully shielded from anthropogenic light sources and was suitable for a fully enclosed predator exclusion fence (vs. a peninsula-style fence). Known breeding habitat for both species varies and is thought to be an artifact of range contraction rather than true preference. The breeding habitat of extant NESH is characterized by steep slopes within areas dominated by native vegetation such as ōhi’a and uluhe fern or steep cliff walls (Ainley et al., 2020). At KPNWR, where several pairs of NESH currently breed, they are found in a combination of artificial nest boxes placed under vegetation (typically naupaka) and in naturally excavated tunnels or depressions under Hala debris which is similar to the restoration site they were translocated into. HAPE breeding sites range from open, rocky subalpine habitat at high-elevation to wet montane forest with dense uluhe fern, similar to NESH (VanZandt et al., 2014), and were historically found in coastal sand dunes (Pyle and Pyle, 2017).Full details on how the fence was constructed, predator eradication and subsequent vegetation restoration can be found in Young et al. (2018).
While HAPE were not previously known from KPNWR, NESH do occur in the area and there have been multiple attempts to attract and translocate NESH to the area. In response to declines in the montane colonies, in 1978 and 1980, 65 and 25 NESH eggs were translocated to Kīlauea Point and Moku’ae’ae Island (just offshore of KPNWR), respectively, and cross-fostered by Wedge-tailed Shearwater (Ardenna pacifica; WTSH) pairs in an attempt to establish a NESH colony at a protected site (Byrd et al., 1984). Seventy-nine percent of these eggs hatched and 94% of the chicks fledged (Byrd et al., 1984) and several pairs of NESH now breed at KPNWR today (USFWS unpubl. data). In addition to these egg cross fostering experiments, chick cross fostering experiments were conducted in the 1980s at KPNWR by removing NESH from their montane colonies and placing them with WTSH parents (A. Silva pers comm). Today, the population contains up to 11 active burrows.
Social attraction and artificial burrows
In addition to the habitat restoration, we installed 76 artificial nest boxes to provide suitable nesting locations. The artificial burrows also facilitated better access to reduce disturbance and handling time to the translocated chicks. Artificial burrows were dug into the ground so that just the lid was exposed. The lids were painted white with thermally reflective paint to reduce the internal temperature, and sandbags were placed on burrow lids to block direct sun and reduce thermal fluctuations. We monitored interior burrow temperatures for several weeks prior to the first translocation (see Young et al., 2018). By painting and covering the lids, we reduced the average temperature by 2°C, and most importantly, reduced the upper end of the range from 30°C to 25°C. We continued to monitor temperature during the translocations to assess the potential for heat stress on the birds. Burrows were open to the ground and the floor was covered with a layer of tumbled 0.5-1cm pea gravel topped with wood shavings to prevent flooding and mud accumulation.
We installed a solar-powered social attraction system with two waterproof speakers at the site from March-September of each year starting in 2016. The goals of social attraction were to attract wild adults to the site, simulate natural colony sounds for the growing chicks, and serve as a cue to returning birds in future years. The calls of both HAPE and NESH were broadcast in continuous sequential loops of each species from dusk until dawn each night. During the chick translocation season (September-December), the social attraction unit was turned off to avoid attracting Barn Owls, a known seabird predator (Raine et al., 2019), to the area when chicks were exercising outside their burrows. Barn Owl control also was conducted during the fledgling period to prevent any predation on chicks.
Selecting and translocating chicks
After candidate source burrows had been identified, they were monitored monthly during the breeding season using Reconyx Hyperfire PC900 and HP2X, and Bushnell Aggressor infrared motion sensor trail cameras. Cameras were used to evaluate the frequency of parental visits, and most importantly, whether the chick had emerged from the burrow. By only selecting chicks that had not emerged, we maximized the chances of the chicks imprinting on and returning to the translocation site rather than their natal burrow.
The week prior to collection, trail cameras that had been pointed towards on candidate burrows were checked to ensure chicks had not emerged; chicks that had emerged were removed as translocation candidates. On the translocation day, 2-3 teams of three people were flown by helicopter to the source colonies. Teams were equipped with a list of candidate burrows and a collection kit consisting of pet carriers, towels, scales, stomach tubes and fluids for the birds (in the event that birds needed fluids if held overnight). If the chick had not emerged, the date of the last parental feeding was noted, and the chick was removed by hand from its burrow and placed into the pet carrier. Once the chick was in the pet carrier, it was weighed and all data recorded. Birds were then hiked up to the landing zone and picked up by the helicopter. Care was taken to reduce the amount of time birds spent in the pet carriers and to ensure they were always in the shade and protected from predators. The birds were flown by helicopter for 10-15 minutes to an airstrip and then driven approximately 20 minutes to the restoration site at KPNWR.
Upon arrival at the restoration site, we banded the chicks on the right leg with a federally issued metal leg band and took a small blood sample for sex identification purposes. We also assessed their overall health and plumage to determine if any birds needed immediate veterinary care and/or arrived with any pre-existing health conditions before placing the chicks in their burrows.
Diet and husbandry
We fed chicks a room- temperature blended diet of squid, fish, unflavored Pedialyte, canned sardines in water, vitamins, and salmon oil, which was modified from diets used previously in New Zealand (Miskelly et al., 2009). Food was pureed until smooth and fed via a sterile 18-gauge French red rubber stomach tube attached to a 100-ml syringe. We chose not to feed birds on the translocation day to prevent regurgitation and further stress, and because many of the chicks had been fed recently by their parents prior to collection.
We fed chicks 1-20% (5-60g) of their body weight daily depending on several factors, including age, current weight relative to expected weight and behavior. Expected weight was based off growth curves for each species following Simons (1985) and Simons and Bailey (2020) for HAPE and USFWS unpubl. data for NESH. If the birds showed signs of regurgitation during feeding, the feeding was stopped and the tube was pinched and pulled out quickly to prevent the aspiration of food. After the birds’ weights had stabilized post-translocation, feeding days were skipped to better mimic their wild feeding schedule. The closer the birds were to fledging, the less readily they accepted food and thus the less they were fed. Many Procellariformes chicks achieve maximum chick weights of 50-100% above their adult mass and thus need to lose weight prior to fledging (Ainley et al., 2020, Simons and Bailey, 2020). Both the diet composition as well as delivery methods were tailored to mimic the wild growth curves of the chicks, and often chicks arriving at their peak mass had feedings gradually reduced until their weights stabilized close to average fledging weights for their species.
We made every effort to maintain sterile conditions to prevent the spread of bacteria and viruses between individual chicks and species. While most NESH had fledged by the time HAPE had arrived, there was typically one week of overlap when both species were on the site. During that week, we fed all NESH first, disinfected the feeding area, and then fed HAPE afterwards. Food preparation occurred off site in a dedicated indoor kitchen, but feeding of the birds occurred at the translocation site under a covered gazebo located 20m from the chicks artificial burrows. Chicks were removed from their burrows and placed in a pet carrier and walked to the covered gazebo for each feeding. Individual stomach tubes and syringes were used for each bird to prevent cross-contamination. We hand- washed all food preparation and feeding equipment in a dishwasher, soaked in a 10% bleach solution for 10 minutes, and sterilized each item by soaking it in a 2% Chlorhexidine gluconate solution (brand name Hibiclens) overnight. After soaking in the antiseptic bath, we rinsed feeding equipment with cool boiled water. We handled birds with cotton gloves to prevent oils from human skin compromising feather waterproofing. Hands were sterilized between each bird with hand sanitizer to prevent the spread of bacteria between birds in the field.
Growth and monitoring
We weighed chicks of both species daily to assess growth and measured their flattened wing chords every 3-4 days. We compared wing chord lengths to wild growth curves in Simons (1985) and Simons and Bailey (2020) for HAPE and USFWS unpubl. data for NESH. We blocked the chicks in their burrows until they reached an average wing chord length of 250 mm for HAPE and 208mm for NESH, which are considered the minimum wind chord lengths at which each species could successfully fledge. We blocked birds in their burrows for three reasons. First, we did not want chicks to leave due to stress before they were physically ready. Second, to ensure they had adequate time to imprint on the site and acclimate to the artificial feeding regime. Third, we wanted to minimize the potential exposure to Barn Owl predation by minimizing the time the chicks spent outside the burrow.
We developed several new indicators to assess whether birds were ready to fledge. We used a combination of mass, wing chord, smell, and primary feather length to assess fledging readiness. We used the crossing of the longest primary feathers on each wing to assess readiness and the development of seabird ‘smell’. Many Procellariformes have a distinctive musty odor, and the captive reared chicks did not acquire this odor until they were fully feathered. When we checked burrows each morning and a chick was absent, we conducted a thorough search of the fenced area, nearby burrows, and immediately outside the fence to ensure the chicks safely made it out to sea and did not crash into vegetation or get predated by Barn Owls. If no evidence of fledging mortality was observed, we assumed that all chicks that fledged made it safely out to sea.
Monitoring for returning chicks
Starting in 2018 when the first returning chicks would be three years old, we began monitoring at the restoration site to check for returning adults. We placed ten Bushnell and Reconyx Hyperfire infrared motion sensor trail cameras on the sound system speakers (where most returning birds are typically first attracted to) and throughout the artificial burrow area to document whether birds visited at night. Since all translocated chicks were banded, we used the presence of a band to determine if the adults on camera were returning chicks or new recruits attracted to the sound system. In most cases the band number was not readable in photos, but was determined eventually when they began spending time in the nest boxe; only birds whose band number could be verified were counted towards returning bird totals. In addition, from March-October of each year, we conducted evening auditory surveys monthly, and checked burrows manually every two weeks to search for any returning birds. Surveys for returning chicks were not conducted at the source sites.
Metrics of success and statistical analysis
With any conservation project, defining, measuring and evaluating project success is critical. For this project, metrics of success were broken down into stages to correspond to transport, captive rearing, social attraction, and future reproductive success. Targets were for project parameters to meet or exceed those measured in wild colonies (i.e. mass, wing chord, and fledging rates), and to attract at least one new individual of each species to the site during the project period.
We determined age of each chick based on wing chord length since both species follow growth curves and wing chord length is strongly correlated with age (Simons, 1985; Simons and Bailey, 2020). Using Minitab, we used ANOVAs to examine variation among years in several variables, including age, body weight, and wing chord at collection and at fledging, with sex as an additional factor to account for differences between the sexes. In judging significance, we adjusted the alpha levels using Tukey’s correction for multiple comparisons (n = 7). Tukey’s adjustments: 0.05/7 = 0.0071; 0.01/7 = 0.0014.
We examined factors that affected fledging size using multiple regression analyses, with either fledging mass or fledging wing chord as the dependent variable, and either body mass or wing chord at collection, and body condition index at collection and days in care as predictors. We used a Chi-squared test to compare the proportion of HAPE that returned as adults from the 2015 + 2016 cohorts vs. the 2017 cohort. Birds translocated in years after 2017 were not included because it is too early for all birds from that cohort to have returned yet.
Results
Source colony and chicks removed
From 2012-2020, a total of 269 surveys were conducted at 12 colonies for both species revealing 85 possible candidate NESH burrows and 238 possible HAPE burrows in seven colonies from which to select chicks. In each year, a maximum of 20 chicks of each species were taken from potential candidate burrows. Colonies where chicks were removed from were: Hanakoa, Hanakapii, Kilauea Point National Wildlife Refuge, North Bog, Pohakea, Pihea, and Upper Limahuli Preserve.
Translocation day outcomes
For the first year of HAPE translocations, we collected 10 chicks on 2 November 2015. Because several candidate chicks had already emerged, and the need to increase the cohort size to 20 in year two, in 2016 we collected chicks a week earlier, on 24 October 2016, to reduce the chance that chicks has emerged from their burrow. In 2017 and subsequent years we collected chicks another week earlier to ensure that an adequate number of birds could be removed. We translocated a total of 110 HAPE over a six-year period from 2015-2020.
The first NESH translocation occurred on 19 September 2016, when we collected seven chicks. While our goal had been to collect 10 birds, on translocation day some birds had emerged early and others proved to be too deep in the burrow to collect. An eighth bird, which had been in care at the Save our Shearwaters (SOS) program for the previous month after being found outside its burrow in August, was brought to Nihoku on 21 September to join the translocation cohort. However, because this bird had already emerged from its burrow at the time it was rescued and had seen the night sky in the wild, we are not assuming this bird will return to Nihoku and did not consider it part of the translocation cohort. In 2017, we moved the translocation date up by six days to 13 September to minimize the number of chicks that had emerged. Even with this schedule change, we were only able to collect 18 birds. The following three years (2018-2020), we conducted translocations during the same week and successfully collected 20 chicks each year, for a total of 86 NESH translocated over five years.
Chick growth and fledging
Both species showed consistent growth curves (Figures 1, 2). HAPE arrived at a mass of 533 ± 7 g, which was 100g (23%) above their average fledging mass of 433 ± 3g (Table 1). Mass plotted against age showed an initial increase above adult mass and fledging mass then a gradual decrease as birds lost weight prior to fledging (Figure 1). HAPE arrived with highly variable wing lengths depending on the age of the bird, but fledged with an average wing chord length of 287 ± 0.8 mm. Maximum wing chord length was reached about nine days prior to fledging for most chicks. Chicks fledged at 110.7 ± 0.4 days old.
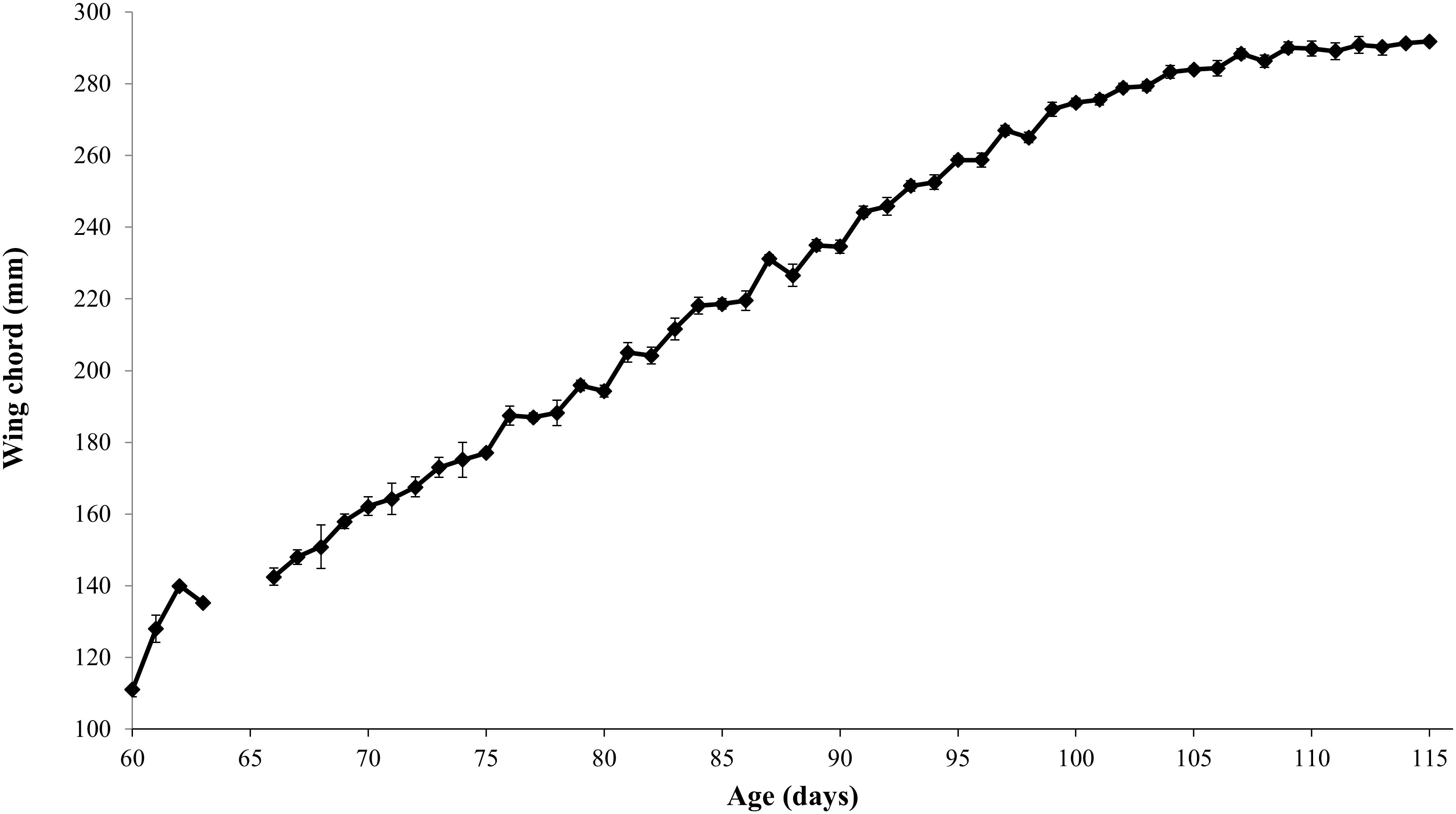
Figure 1 Hawaiian Petrel mass plotted against age for translocated chicks on Kaua`i, Hawai`i, 2015-2020. Average collection age was 76.8 ± 0.8 days.
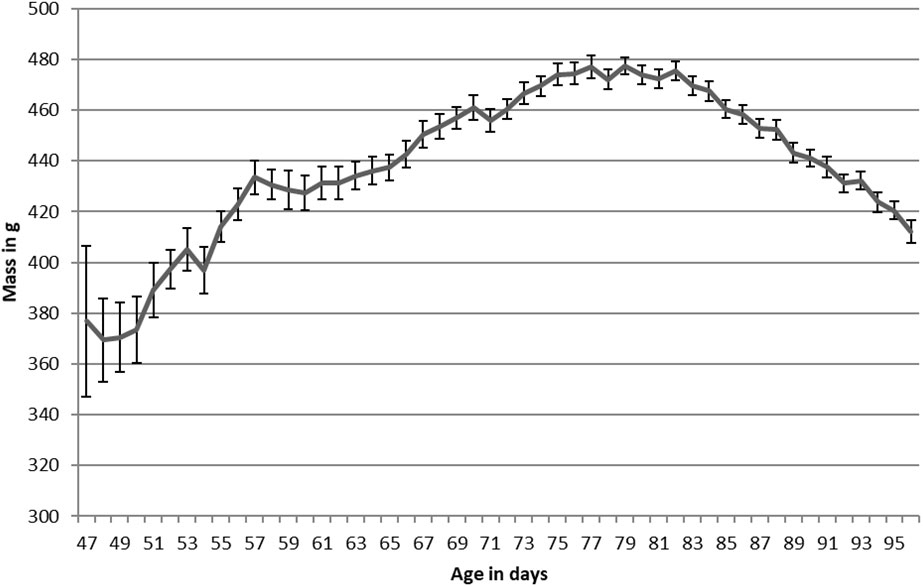
Figure 2 Newell’s Shearwater mass plotted against age for translocated chicks on Kauai, Hawaii, 2016-2020. Average collection age was 61.2 ± 0.8 days.
In contrast to HAPE, NESH arrived at an average mass of 430 ± 13 g, only 3% above their 418 ± 6 g fledging mass (Table 2; Figure 2). NESH chicks were in care for longer than HAPE chicks, but similar to HAPE, they gained weight after intake to reach their maximum mass (451g; 8% above fledging mass) before slimming down at fledging time. Chicks fledged at 96.5 ± 0.4 days old, and 16 ± 0.5 days (range 2-25 days) after the burrows were unblocked.
Differences in fledging size and age by sex
There were sex-based differences in average fledging mass and wing chord in both species, but with opposite patterns. Male HAPE had a higher body weight at fledging than females (440 ± 4.0 g vs. 425 ± 4 g, respectively; t = 2.65, p = 0.009), but there was no difference between male and female HAPE in fledging wing chord (287.6 ± 1.2 mm vs. 287.1 ± 1.2 mm, respectively; t = 0.28, p = 0.78). In NESH the pattern was opposite; there was no difference in fledging mass of males and females (419 ± 4.0 g vs. 418 ± 3 g, respectively; t = 0.21, p = 0.84), but males had a longer fledging wing chord (244 ± 1 vs. 241 ± 1 mm, respectively; t = 2.97, p = 0.004). Because the sexes of both species differed in either body weight or wing chord, we included sex as a factor in other analyses of factors affecting fledging size. There was no difference in fledging age between males and females in HAPE (110.5 ± 0.8 d vs. 110.5 ± 0.5 d, respectively; t = 0.43, p = 0.67) or in NESH (96.8 ± 0.5 d vs. 96.2 ± 0.5 d, respectively; t = 0.87, p = 0.39).
Variation in age and size at collection and fledging
There was significant variation among years in several metrics at collection and fledging of the translocated HAPE and NESH. In HAPE, age at collection varied among years; chicks were collected at older ages during the first two years of the project in 2015 and 2016 (Table 1). Newell’s Shearwaters also were collected at an older age in the first year of the project in 2016, but the ages were similar among subsequent years and there was less variation overall (Table 2). Wing chord length at collection of HAPE varied among years, reflecting the older collection ages in 2015 and 2016, but body weight at collection did not vary among years. In both species, fledging wing chord was higher in the later years of the project when chicks were collected at a younger age.
Factors affecting size at fledging
Body weight at collection was not a significant predictor of fledging weight or fledging wing chord in either species (Table 3). Wing chord at collection was a significant predictor of wing chord at fledging but was not a significant predictor of body weight at fledging. Days in care had a strong negative effect on fledging body weight, but a strong positive effect on fledging wing chord.
Chick mortality
There was no NESH mortality and every single bird survived to fledge in all six years. A total of four HAPE died during the project. In 2015, a single HAPE chick died six days after collection. The chick was collected from a burrow where it had been sitting in the remains of its dead parent. At the time of collection it weighed 125g less than the average mass of the cohort despite having a wing chord of 197mm compared to the average wing chord of 195mm for the cohort. It accepted food readily for the first five days and was behaving normally until the sixth morning. When it was removed from its burrow for its daily feeding, it had regurgitated in its burrow prior to being handled (which is unusual) and had lost more than 10% of its body weight overnight. When the aviculturalist returned that evening, the chick was dead.
A veterinarian completed a full necropsy and determined the bird died from a disseminated bacterial infection of unknown origin. We suspect that this bird came in compromised in some way given that none of the other chicks presented bacterial infections. From 2018-2020, three more HAPE chicks died during the first week post translocation as a result of disseminated bacterial infections that likely were obtained prior to translocation. All mortality experienced by HAPE during the translocation process happened during the first six days and appeared to be a result of pre-existing bacterial infections.
Returning birds and metrics of success
Several, but not all, of the metrics of success that can be measured at this stage of the project have been achieved (Table 4), with chick fledging parameters exceeding that of their wild counterparts, but return and recruitment rates lower than expected based on known values from wild birds.
A total of eight translocated HAPE (four females and four males) and one NESH have returned to the restoration site as adults Table 5. In 2021, a pair of translocated HAPE nested at the site and successfully hatched a chick, which unfortunately appeared to have died during the hatching process. In 2022, the same pair, plus two new pairs, laid eggs. Two of the eggs hatched, and one chick fledged (from the parents who bred at the site in 2021). The proportion of petrels that have returned from the 2017 cohort (N= 5/20; 25%) was somewhat higher than the proportion that have returned from the 2015 and 2016 cohorts (N= 2/29; 7%), but the difference was not significant because of small samples sizes (Chi-square = 2.32, df = 1, P = 0.13; see Tables 1, 2). A single HAPE has returned from the 2018 cohort, but was not included in the analysis because it is still too soon to determine ultimate return rates for that year. A single banded NESH had returned as of 2021, but the band number could not be read from the trail camera photo to ascertain which cohort it came from, nor could the possibility be eliminated that it was a banded bird from a nearby colony or a bird released from the Save Our Shearwaters rehabilitation program. A male NESH from the 2018 cohort returned in 2022 and was the first verified return of a translocated NESH chick to the site.
The returning HAPE first returned at an average age 3.6 years (N=4 at age three, N=3 at age four, N=1 at age five). Birds returning from the 2016 cohort (both females) returned at ages 4 and 5 respectively, whereas 4/5 birds that returned from the 2017 cohort returned at age three.
Of the eight returnees, there was an equal number of each sex, and the birds formed four male-female pairs; three of which bred in 2022.
Discussion
Overall, the translocation of Newell’s Shearwaters and Hawaiian Petrels went well, with the project meeting or exceeding, many of the established metrics of success. Growth curves for translocated birds mimicked natural curves, but with decreased variability because of more regular feeding regimes in captivity (see Simons, 1985 and Ainley et al., 2020). Interestingly, while fledging age or mass did not change by extracting the birds earlier each year, the average fledging wing chord of both species increased the longer birds were in care.
Significant patterns were noted in mass and wing chord at intake, and in fledging, for both species. While body mass at collection was not a significant predictor of fledging mass or fledging wing chord in either species, wing chord at collection was a significant predictor of wing chord at fledging for both species. Days in care had a strong negative effect on fledging body weight, but a strong positive effect on fledging wing chord. If a bird was in care longer it was possible to make up for previous deficiencies in wing growth, but extra time in care was counterproductive to fledging body weight. We expected there to be a stronger positive relationship between size at fledging and days in care, but some birds that arrived in poor condition lingered much longer at the release site and eventually fledged at a relatively low weight and these individuals had a strong effect on the regression because of their extreme fledging age values.
For both species, while fledging age or mass did not change by collecting the birds earlier each year, the average fledging wing chord increased each year the longer birds were in care. The increase in wing chord at intake in later years of this project has several possible explanations. This could be due to the age at which birds were collected- birds collected at older ages in 2015 and 2016 may weigh less than younger chicks collected at their peak mass and have naturally longer wing chords simple because they are older. It is also possible that there were environmental factors at play in 2015 and 2016 which were part of the strong El Nino event of 2015. As a result, this could have in turn influenced parental provisioning prior to collection for translocation resulting in decreased body condition. Finally, it could be that collecting younger chicks (as was done from 2017 onwards) reduced the influence of any parental and/or environmental variation and gave chicks a longer period in which they were being fed on a consistent, high nutrition schedule to optimize their growth rates. While there isn’t enough data to be able to tease apart these factors, it is potentially the start of a trend that warrants further investigation. While the decision to translocate chicks at an earlier age was a management decision meant to maximize the chances of translocating a larger cohort by reducing the chances a chick had emerged from the burrow, it provided a natural experiment and lens from which to analyze the data.
As of November 2022, 8/69 HAPE translocated between 2015 and 2018 (the earliest possible cohorts that we could expect to have fully returned as adults) had returned as adults, which we considered to be a success, but albeit at a lower rate than we had expected based on the return rates of wild birds. A single bird has returned from the 2018 cohort, but it is too early to determine whether the final return rates has been achieved for that cohort. The trends in characteristics of returning birds were surprising to us. As stated above, birds were translocated at older ages in 2015 and 2016, and fewer birds from these two cohorts returned as adults (2/29) and those that returned did so at a later age (four or five years old compared to three). While the possible reasons for this are varied and described above, it has caused us to re-evaluate our translocation strategy to be more conservative and take birds at an earlier age to ensure imprinting on the new site is maximized and nutritional stress is minimized. Other projects, particularly with surface nesting albatross, have had better success in birds returning to the translocation site when they are moved earlier (Deguchi et al., 2017) and indeed, late translocation has been listed as a reason for failure for some projects, such as the Chatham Albatross (E. Bell pers comm).
All three HAPE pairs that bred at the restoration site began prospecting the site at least one or two years before they ultimately laid an egg. The first breeding pair in 2021 were observed copulating late in the 2020 season and returned to lay an egg in 2021 breeding season nine months later.
While this project provided a wealth of data and information not previously known for either of these species, there were limitations to the conclusions we can draw from it. The largest obstacle to examining return rates to inform future management is the fact that it is difficult to search for returning chicks in their natal montane colonies due to the terrain and geographical extent of the colonies. All the natal montane colonies are monitored on an annual basis through direct burrow checks and searches for new burrows, but locating a returning chick in these areas is challenging. As a result, it is difficult to know whether chicks that didn’t return died or returned to their natal colony instead. For example, we have assumed that larger body size at fledging is generally advantageous and will lead to higher survival and return rates, but we will not know whether this is valid until more birds have returned. It is possible that the smaller size of some chicks was natural variation and was not caused by better nutrition during development. Our preliminary data on the returning chicks is limited, but doesn’t appear to support this previous assumption of size and return rates. Future studies would benefit from a robust post-translocation monitoring program in the natal source colony to examine these questions and contribute to the growing body of knowledge on the success of translocation and social attraction as conservation interventions.
Conclusions
The results from this intervention highlight the need for rigorous post-translocation monitoring and evaluation of the metrics of success. Based on the results obtained, better outcomes for future projects translocating burrow nesting Procellariformes could be obtained by translocating birds at an earlier age rather than waiting until the period just prior to burrow emergence. Collecting birds earlier resulted in better body condition, large cohort possibilities (because fewer chicks had emerged), and higher adult return rates. While this results in birds being in captive care for longer, it resulted in a higher proportion of the cohort returning to the release site as adults and returned at an earlier age for HAPE. Given the increase in the number of translocations occurring for seabirds word wide, these results can be applied to a large number of future management projects and significantly improve their outcomes.
Data availability statement
The raw data supporting the conclusions of this article will be made available by the authors, without undue reservation.
Ethics statement
Ethical review and approval was not required for the animal study because there were no University affiliations with this project that required an animal ethics committee. However, ethical guidelines are found within the permitting process associated with the Endangered Species Act Recovery permit and the State of Hawaii Scientific Research Permits, and this project met and exceeded all state and federal guidelines found within those regulatory bodies. This study adhered to all applicable state and federal permits and animal safety guidelines. This project was done under the US Fish and Wildlife Service recovery permit # TE-67121B, state of Hawai`i Protected Wildlife permit #WL19-45, US Geological Survey Bird Banding permit 23462 and after the finding of No significant impact from two federal environmental assessments (one for each species) that can be found in the federal register.
Author contributions
LY, CK, AR and EV contributed to the study design, implementation of the field work and writing of the manuscript. LF, DC, ED, MD, MK, AP and MV contributed to the field work.
Funding
Funding for this project was provided from the National Fish and Wildlife Foundation and Earthjustice and administered through the American Bird Conservancy.
Acknowledgments
We thank Kīlauea Point National Wildlife Refuge and the U.S. Fish and Wildlife Service for their support during this project, and for being willing to provide a long-term commitment to the conservation of these species. We thank Hallux Ecosystem Restoration and the National Tropical Botanical Gardens for conducting predator control in the montane colonies to ensure birds were available for translocation, and both the state of Hawai`i Natural Area Reserves System and the National Tropical Botanical Garden for granting this project permission to remove birds from their land. We thank Airborne Aviation for providing helicopter transport, Dr. J. Woltman and the Save our Shearwaters program for veterinary care, and the Bishop Museum for genetically sexing all of our translocation chicks. We thank the National Fish and Wildlife Foundation, the American Bird Conservancy and Earthjustice for funding and technical expertise on this project. Finally, we thank H. Gummer and the New Zealand Department of Conservation for their assistance in providing training opportunities for this project.
Conflict of interest
The authors declare that the research was conducted in the absence of any commercial or financial relationships that could be construed as a potential conflict of interest.
Publisher’s note
All claims expressed in this article are solely those of the authors and do not necessarily represent those of their affiliated organizations, or those of the publisher, the editors and the reviewers. Any product that may be evaluated in this article, or claim that may be made by its manufacturer, is not guaranteed or endorsed by the publisher.
Supplementary material
The Supplementary Material for this article can be found online at: https://www.frontiersin.org/articles/10.3389/fcosc.2023.1177789/full#supplementary-material
References
Ainley T. R., Tefer T. C., Reynolds M. H., Raine A. F. (2020). “Newell’s shearwater (Puffinus newelli),” in The birds of north America (Ithaca, NY, USA: Cornell Lab of Ornithology). Available at: https://birdsoftheworld.org/bow/species/towshe2/cur/introduction.
Antaky C. C., Young L., Ringma J., Price M. R. (2021). Dispersal under the seabird paradox: probability, life history, or spatial attributes? Mar. Ornithology 49, 1–8.
Bell M., Bell B. D., Bell E. A. (2005). Translocation of fluttering shearwater (Puffinus gavia) chicks to create a new colony. Notornis 52, 11–15.
Byrd G. V., Sincock J. L., Telfer T. C., Moriarty D. I., Brady B. G. (1984). A cross-fostering experiment with newell’s race of Manx shearwater. J. Wildlife Manage. 48, 163. doi: 10.2307/3808464
Carlile N., Priffel D., Madeiros J. (2012). Establishment of a new, secure colony of endangered Bermuda Petrel pterodroma cahow by translocation of near-fledged nestlings. Bird Conserv. Int. 22, 46–58. doi: 10.1017/S0959270911000372
Croxall J. P., Butchart S. H. M., Lascelles B. E. N., Staainttersfield A. J., Sullivan B. E. N., Symes A., et al. (2012). Seabird conservation status, threats and priority actions: a global assessment. Bird Conserv. Int. 22 (1), 1–34. doi: 10.1017/S0959270912000020
Deguchi T., Jacobs J., Harada T., Perriman L., Watanabe Y., Sato F., et al. (2012). Translocation and hand-rearing techniques for establishing a colony of threatened albatross. Bird Conserv. Int. 22, 66–81. doi: 10.1017/S0959270911000438
Deguchi T., Sato F., Eda M., Izumi H., Suzuki H., Suryan R. M., et al. (2017). Translocation and hand-rearing result in short-tailed albatrosses returning to breed in the ogasawara islands 80 years after extirpation. Anim. Conserv. 20, 341–349. doi: 10.1111/acv.12322
Dias M. P., Martin R., Pearmain E. J., Burfield I. J., Small C., Phillips R. A., et al. (2019). Threats to seabirds: a global assessment. Biol. Conserv 237, 525–537. doi: 10.1016/j.biocon.2019.06.033
Felis J. J., Kelsey E. C., Adams J., Stenske J. G., White L. M. (2020). “Population estimates for selected breeding seabirds at kīlauea point national wildlife refuge, kaua“i, in 2019,” in US Geological survey data series 1130 (Reston, USA: US Geological Survey). doi: 10.3133/ds1130
Jones H. P., Tershy B. R., Zavaleta E. S., Croll D. A., Keitt B. S., Finkelstein M. E., et al. (2008). Severity of the effects of invasive rats on seabirds: a global review. Conserv. Biol 22, 16–26. doi: 10.1111/j.1523-1739.2007.00859.x
Gummer H. (2020). Best practice techniques for translocations of burrow-nestingpetrels and shearwaters (New Zealand: Wellington).
Jones H. P., Tershy B. R., Zavaleta E. S., Croll D. A., Keitt B. S., Finkelstein M. E., et al. (2008). Severity of the effects of invasive rats on seabirds: a global review. Conserv. Biol 22, 16–26. doi: 10.1111/j.1523-1739.2007.00859.x
Madeiros J., Carlile N., Priddel D. (2012). Breeding biology and population increase of the endangered Bermuda petrel Pterodroma cahow. Bird Conserv. Int. 22, 35–45. doi: 10.1017/S0959270911000396
Miskelly C. M., Taylor G. A. (2004). Establishment of a colony of common diving petrels (Pelecanoides urinatrix) by chick transfers and acoustic attraction. Emu 104, 205–211. doi: 10.1071/MU03062
Miskelly C. M., Taylor G. A., Gummer H., Williams R. (2009). Translocations of eight species of burrow-nesting seabirds (genera pterodroma, pelecanoides, pachyptila and puffinus: family procellariidae). Biol. Conserv. 142, 1965–1980. doi: 10.1016/j.biocon.2009.03.027
Pyle R. L., Pyle P. (2017). The birds of the Hawaiian islands: occurrence, history, distribution, and status (Honolulu, HI, U.S.A: Bishop Museum). Available at: http://hbs.bishopmuseum.org/birds/rlp-monograph/. Version. Volume 2. B.P.
Raine A. F., Driskill S., Vynne M., Harvey D., Pias K. (2020). Managing the effects of introduced predators on Hawaiian endangered seabirds. J. Wildlife Manage. 84, 425–435. doi: 10.1002/jwmg.21824
Raine A. F., Holmes N. D., Travers M., Cooper B. A., Day R. H. (2017). Declining population trends of Hawaiian petrel and newell’s shearwater on the island of kaua’i (Hawaii, USA: The Condor) 119 (3), 405–415.
Raine A. F., Vynne M., Driskill S. (2019). The impact of an introduced avian predator, the barn owl tyto alba, on Hawaiian seabirds. Mar. Ornithology 47, 33–38.
Reynolds M. H., Courtot K. N., Berkowitz P., Storlazzi C. D., Moore J., Flint E. (2015). Will the effects of sea-level rise create ecological traps for pacific island seabirds? PloS One 10 (9), e0136773. doi: 10.1371/journal.pone.0136773
Spatz D, Young L. C., Holmes N. D., Jones H. P., Lyons D., VanderWerf E. A., et al (2023). Tracking the global effort to reverse seabird declines with active restoration techniques. Proceedings of the National Academy of Sciences 120, e2214574120.
Serventy D. L., Gunn B. M., Skira I. J., Bradley J. S., Wooller R. D. (1989). Fledgling translocation and philopatry in a seabird. Oecologia 81, 428–429. doi: 10.1007/BF00377094
Simons T. R. (1985). Biology and behavior of the endangered dark-rumped petrel. Condor 87, 229–245. doi: 10.2307/1366887
Simons T. R., Bailey C. N. (2020). Hawaiian Petrel (Pterodroma sandwichensis), version 1.0. In Birds of the World Poole A.F., Gill F.B. Editors. (Ithaca, NY, USA: Cornell Lab of Ornithology). doi: 10.2173/bow.hawpet1.01.
Spatz D. R., Holmes N. D., Reguero B. G., Butchart S. H. M., Tershy B. R., Croll D. A. (2017). Managing invasive mammals to conserve globally threatened seabirds in a changing climate. Conserv. Letters. 10, 736–747. doi: 10.1111/conl.12373
Tinker M. T., Zilliacus K. M., Ruiz D., Tershy B. R., Croll D. A. (2022). Seabird meta-population viability model (mPVA) methods. MethodsX 9, 101599. doi: 10.1016/j.mex.2021.101599
Travers M., Driskill S., Stemen A., Geelhoed T., Golden D., Koike S., et al. (2021). Post-collision impacts, crippling bias, and environmental bias in a study of newell's shearwater and Hawaiian petrel powerline collisions. Avian Conserv. Ecol. 16, 15. doi: 10.5751/ACE-01841-160115
VanderWerf E. A., Kress S., Guzmán Y. B., Spatz D., Taylor G., Gummer H. (2022). “Restoration: social attraction and translocation,” in Conservation of marine birds. first edition. Eds. Young L. C., VanderWerf E. A. (Cambridge: Elsevier).
VanZandt M., Delparte D., Hart P., Duvall F., Penniman J. (2014). Nesting characteristics and habitat use of the endangered Hawaiian petrel (Pterodroma sandwichensis) on the island of lāna´i. Waterbirds 37, 43–52. doi: 10.1675/063.037.0107
Warham J. (1990). The petrels: Their ecology and breeding systems. (London: Academic Press), pp. 440.
Wynn J., Padget O., Mouritsen H., Perrins C., Guilford T. (2020). Natal imprinting to the earth’s magnetic field in a pelagic seabird. Curr. Biol. 30, 2869–2873. doi: 10.1016/j.cub.2020.05.039
Young L. C., Behnke J. H., Vanderwerf E. A., Raine A. F., Mitchell C., Kohley C. R., et al. (2018). The nihoku ecosystem restoration project: a case study in predator exclusion fencing, ecosystem restoration, and seabird translocation. pacific cooperative studies unit technical report 198 (Honolulu, HI: University of Hawai’i at Mānoa, Department of Botany), 83.
Keywords: seabird translocations, Newell’s Shearwater, Hawaiian Petrel, translocation, Hawaii, rewilding
Citation: Young LC, Kohley CR, VanderWerf EA, Fowlke L, Casillas D, Dalton M, Knight M, Pesque A, Dittmar EM, Raine AF and Vynne M (2023) Successful translocation of Newell’s Shearwaters and Hawaiian Petrels to create a new, predator free breeding colony. Front. Conserv. Sci. 4:1177789. doi: 10.3389/fcosc.2023.1177789
Received: 02 March 2023; Accepted: 12 May 2023;
Published: 29 May 2023.
Edited by:
David R. Breininger, University of Central Florida, United StatesReviewed by:
Alison L. Greggor, San Diego Zoo Institute for Conservation Research, United StatesJohannes Fischer, Department of Conservation, New Zealand
Copyright © 2023 Young, Kohley, VanderWerf, Fowlke, Casillas, Dalton, Knight, Pesque, Dittmar, Raine and Vynne. This is an open-access article distributed under the terms of the Creative Commons Attribution License (CC BY). The use, distribution or reproduction in other forums is permitted, provided the original author(s) and the copyright owner(s) are credited and that the original publication in this journal is cited, in accordance with accepted academic practice. No use, distribution or reproduction is permitted which does not comply with these terms.
*Correspondence: Lindsay C. Young, bGluZHNheUBwYWNpZmljcmltY29uc2VydmF0aW9uLm9yZw==
†Present address: Andre F. Raine, Archipelago Research and Conservation, Hanapepe, HI, United States
Megan Vynne, Little Tree Environmental, Kapa’a, HI, United States