- 1Division of Diabetes and Endocrinology, Kobe University Hospital, Kobe, Japan
- 2School of Medicine, Kobe University, Kobe, Japan
- 3Division of Diabetes and Endocrinology, Kobe University Graduate School of Medicine, Kobe, Japan
Cushing’s disease is a syndromic pathological condition caused by adrenocorticotropic hormone (ACTH)-secreting pituitary adenomas (ACTHomas) mediated by hypercortisolemia. It may have a severe clinical course, including infection, psychiatric disorders, hypercoagulability, and metabolic abnormalities, despite the generally small, nonaggressive nature of the tumors. Up to 20% of ACTHomas show aggressive behavior, which is related to poor surgical outcomes, postsurgical recurrence, serious clinical course, and high mortality. Although several gene variants have been identified in both germline and somatic changes in Cushing’s disease, the pathophysiology of aggressive ACTHomas is poorly understood. In this review, we focused on the aggressiveness of ACTHomas, its pathology, the current status of medical therapy, and future prospects. Crooke’s cell adenoma (CCA), Nelson syndrome, and corticotroph pituitary carcinoma are representative refractory pituitary tumors that secrete superphysiological ACTH. Although clinically asymptomatic, silent corticotroph adenoma is an aggressive ACTH-producing pituitary adenoma. In this review, we summarize the current understanding of the pathophysiology of aggressive ACTHomas, including these tumors, from a molecular point of view based on genetic, pathological, and experimental evidence. The treatment of aggressive ACTHomas is clinically challenging and usually resistant to standard treatment, including surgery, radiotherapy, and established medical therapy (e.g., pasireotide and cabergoline). Temozolomide is the most prescribed pharmaceutical treatment for these tumors. Reports have shown that several treatments for patients with refractory ACTHomas include chemotherapy, such as cyclohexyl-chloroethyl-nitrosourea combined with 5-fluorouracil, or targeted therapies against several molecules including vascular endothelial growth factor receptor, cytotoxic T lymphocyte antigen 4, programmed cell death protein 1 (PD-1), and ligand for PD-1. Genetic and experimental evidence indicates that some possible therapeutic candidates are expected, such as epidermal growth factor receptor tyrosine kinase inhibitor, cyclin-dependent kinase inhibitor, and BRAF inhibitor. The development of novel treatment options for aggressive ACTHomas is an emerging task.
Introduction
Cushing’s disease is a hypercortisolemic state caused by adrenocorticotropic hormone (ACTH)-secreting pituitary adenomas (ACTHomas). Although most ACTHomas can be successfully resected using the transsphenoidal approach, up to 20% of ACTHomas exhibit aggressive behavior, which is defined on the basis of clinical behavior, with a generally invasive, high rate of recurrence, lack of response to optimal standard therapies, or atypical pathological findings including carcinoma like features (1). These result in poor surgical and hormonal outcomes. Crooke’s cell adenomas (CCAs) are one of the well-known aggressive ACTHomas that exhibit characteristic pathological features. ACTHomas can be transformed into an aggressive nature after bilateral adrenalectomy, and such tumors are called Nelson’s syndrome. In pituitary carcinomas, the most aggressive tumoral nature, corticotroph carcinomas followed by or along with PRL-secreting pituitary carcinomas are the most common features. Patients frequently have a clinically serious course due to corticotroph carcinomas, and their management is challenging. In contrast, silent corticotroph adenomas (SCAs) exhibit aggressive tumor behavior, whereas hypercortisolemia is not present. In this review, we summarize our current knowledge of the definition, pathophysiology, and treatment of refractory ACTHomas and provide directions for future research.
Aggressive ACTHomas
Crooke’s Cell Adenomas
Crooke’s changes are characterized by large perinuclear cytokeratin filament accumulation in normal corticotrophs due to long-term exposure to endogenous or exogenous glucocorticoid excess, including Cushing’s syndrome. The pathological finding represents an eosinophilic perinuclear hyaline appearance on hematoxylin and eosin staining (2, 3). Crooke’s changes were also discovered within corticotroph adenomas by Kovacs et al. in 1981, called Crooke’s cell adenomas (CCAs) (4). The frequency of Crooke’s changes in ACTHomas varies from 36% to 100% among several reports, and this change is significantly increased in cases with severe hypercortisolism, at least fourfold greater than the upper limit of the normal range of UFC (1, 3, 5). CCA is diagnosed when Crooke’s cells account for more than 50% of the tumor cells (1). These tumors exhibit a high frequency of macroadenoma (77.2%) and more aggressive behavior with invasion to the cavernous sinuses (79.2%) than macro-ACTHomas without Crooke’s change. They can also transform into metastatic pituitary carcinoma, which was previously shown in 7.5% of cases. Some CCAs (24.4%) are clinically silent (6, 7). The recurrence rate of CCAs after surgery is 66% due to the higher frequency of cavernous sinus invasion (8).
Nelson’s Syndrome
The first case of Nelson’s syndrome was reported in 1958, in a 33-year-old woman who underwent bilateral adrenalectomy due to refractory Cushing’s disease. Three years later, skin hyperpigmentation and visual defects with elevated ACTH levels appeared. These symptoms improved after surgical removal of pituitary tumors, which pathologically exhibited ACTH production (9). Nelson’s syndrome is observed in 8–38% of cases after bilateral adrenalectomy for Cushing’s disease (10, 11).
Accumulating data suggest several risk factors for this syndrome, including a rapid elevation of plasma ACTH after bilateral adrenalectomy (12–14), insufficient steroid replacement therapy (10, 15), residual corticotroph tumor after transsphenoidal surgery (TSS) (16), younger age (17), and histopathological characteristics of corticotroph tumor specimens. Elevation of plasma ACTH levels of more than 100 pg/mL in the first year after bilateral adrenalectomy is associated with the development of Nelson’s syndrome (18).
From a histological point of view, there is no difference between the tumors of Nelson’s syndrome and those of Cushing’s disease. Despite the low expression of Ki-67 (usually less than 3%), tumor behavior is aggressive and invasive. The most common clinical manifestation of Nelson’s syndrome is dark skin hyperpigmentation, with markedly elevated plasma ACTH levels. Bitemporal hemianopia and progressive visual loss caused by aggressive tumors are clinically important issues to be addressed. Therefore, regular follow-up should be monitored using MRI.
Corticotroph Carcinoma
Pituitary carcinomas are currently defined as pituitary tumors with craniospinal dissemination or metastasis to other types of tissues (19). Pituitary carcinomas occupy only 0.1–0.2% of pituitary neoplasms derived from the anterior pituitary (19). Epidemiologically, there is no gender difference in the prevalence of pituitary carcinomas (20). Metastasis is commonly identified in the central nervous system, followed by the liver, bones, and lungs (20). The specific symptoms of pituitary carcinoma are absent and depend on the region of metastasis, such as hearing loss, ataxia, or motor impairment (21). While CT and MRI are most often utilized to identify the metastatic region, 18F-FDG, 111In-labeled octreotide, and 68Ga-DOTANOC in scintigraphy are also useful according to recent case reports (21–23).
There are no pathological criteria to distinguish aggressive adenomas from carcinomas. The following morphological features are not useful in predicting malignant transformation of pituitary adenomas: hypercellularity, nuclear and cellular pleomorphism, increased mitotic activity, necrosis, and dural and/or bony invasion, which are generally associated with malignancy (24, 25).
In endocrinological manifestation, 85–90% of carcinomas express pituitary hormone, and 15–20% of them are clinically nonfunctioning. Prolactin or ACTH-secreting carcinomas are the most frequent, followed by growth hormones and other rare hormones (23, 26–28). In a literature review, corticotroph carcinomas were shown to be the most common (34.7%) among pituitary carcinomas, followed by prolactin-secreting (23.6%) and null cells (15.3%) (20). Corticotroph carcinoma is usually developed from a group that exhibits aggressive phenotypes, such as invasive, rapid growth, and prone to recurrence. One case series of 31 patients with CCAs exhibited more than 80% with single or multiple recurrences, and two patients developed corticotroph carcinoma (6). Nelson’s syndrome occurring after bilateral adrenalectomy has also potentially progressed to carcinomas (21, 29). Furthermore, malignant transformation of SCAs has been reported in rare case reports (30).
Regarding prognosis, one case series of 15 patients with pituitary carcinoma reported that 66% of the patients died within 1 year and 20% were alive at the last follow-up 9–18 months after diagnosis (28). A recent review showed that 34 of 62 patients (55%) died within approximately 10 months after the diagnosis of pituitary carcinoma (31–33).
Silent Corticotroph Adenomas (SCAs)
SCAs are defined as ACTH-expressing pituitary tumors that lack both the clinical symptoms of Cushing’s syndrome and evidence of autonomous cortisol secretion, which is diagnosed with nonfunctioning pituitary adenomas (34–36). The clinical importance of differentiating SCAs from other nonfunctioning pituitary adenomas is due to their aggressive nature (37). The prevalence of SCAs ranges from 3% to 6% in all pituitary adenomas and less than 40% in corticotroph adenomas (36–39).
Patients with SCAs are younger and predominantly females and have a higher prevalence of giant adenomas and an association with cavernous sinus invasion than other nonfunctioning pituitary adenomas, such as silent gonadotroph adenomas (SGA) and null cell adenomas (40, 41). In imaging studies of nonfunctioning pituitary adenomas, cystic or hemorrhagic changes on MRI T2WI are observed in SCAs (42). Regardless of tumor size, multiple microcystic changes are more frequently observed within SCAs and are highly specific to SCAs. These multiple microcysts have been correlated with pseudopapillary features of SCA pathological findings (42). According to the WHO classification, SCAs are classified into two subtypes: type 1 (densely granulated) and type 2 (sparsely granulated) (24, 34, 41). Type 1 SCAs show strong ACTH immunoreactivity similar to typical ACTHomas, while type 2 SCAs exhibit weak and focal expression (43). The lack of galectin-3 expression in corticotroph adenomas can be pathologically diagnostic of SCAs rather than functioning corticotroph adenomas (44). In type 2 SCAs, the expression levels of fibroblast growth factor receptor-4, matrix metalloproteinase-1, and β1-integrin which associates with tumor aggressiveness, are higher than that in type 1 SCAs (45), suggesting different tumor pathologies, however it remains unclear whether these two subtypes indeed influence tumor behavior.
The underlying mechanism of the discrepancy between elevated ACTH levels and normocortisolemia remains unclear in these patients. Various hypotheses have been reported to date. First, SCAs are driven from an intermediate lobe, which, in turn, demonstrates a low ACTH secretory capacity (30, 46). However, this concept was not established in a subsequent study (47). As a second hypothesis, SCAs secrete predominantly unprocessed high-molecular-weight ACTH (also known as big ACTH), which causes competition with mature ACTH at the receptor binding level (48). Another mechanism was suggested to be an increased intracellular degradation of ACTH, which resulted in insufficient ACTH exocytosis from the cell membrane (49). As the most widely accepted concept, the expression levels of prohormone convertase (PC), which is a critical enzyme in POMC posttranslational processes, determine the characteristic difference between SCAs and Cushing’s disease. POMC is cleaved by PC1/3 and PC2 into biologically active ACTH and alpha-MSH, respectively (50). Several reports have demonstrated that SCAs exhibit decreased protein expression levels of PC1/3 concomitant with PC1/3 mRNA downregulation compared to typical corticotroph adenomas (35, 51).
In a certain portion of corticotroph adenomas, bidirectional transformation of the tumor phenotype between SCA and Cushing’s disease has been reported (52) in 3.9% of cases, with a transformation period ranging from 1 to 7 years (52). Interestingly, an altered expression level of PC1/3 has been observed with the lapse of time in identical pituitary adenomas. The clinical phenotype correlates with PC1/3 mRNA or protein levels in corticotroph adenomas (43), suggesting that PC1/3 expression levels play an important role in determining the characteristics of these tumor phenotypes.
Molecular Pathology
Typical ACTHomas and Their Aggressiveness
The most common genetic cause of ACTHomas is the somatic ubiquitin-specific protease 8 (USP8) variant within the 14-3-3 binding motif, which accounts for approximately 20–60% of these tumors (53, 54). The underlying mechanisms of USP8 variants are thought to be mediated by increasing the deubiquitylation activity of this enzyme, leading to epidermal growth factor receptor (EGFR) overexpression (53). EGFR overexpression in corticotrophs has been proven to be a pathogenesis of ACTHomas due to its enhanced proliferation and ACTH hypersecretion (55, 56). In USP8 wild-type ACTHomas, the p.Met415 variant within the catalytic domain of USP48 has been identified (57). However, these genetic variants are found in small tumors and are not associated with tumor aggressiveness in ACTHomas. The BRAF p.V600E variant, which is also found in other cancers, has been identified in ACTH-secreting macroadenomas (57). However, tumor behavior remains unclear due to its low frequency (58).
Next-generation sequencing of USP8 wild-type ACTHomas has revealed TP53 pathogenic variants that are associated with larger and invasive tumors including tumors from patients with Nelson’s syndrome or pituitary carcinomas (58, 59). TP53 variants with wild-type USP8 are associated with chromosome instability, aneuploidy, and tumor aggressiveness (60). Another possible gene associated with tumor aggressiveness is CABLES1, a major glucocorticoid-dependent cell cycle regulator in corticotrophs (61). Recently, mutations in the ATRX gene, which is one of the driver mutations in neuroendocrine tumors and regulates chromatin remodeling and telomere maintenance, have been shown to be associated with aggressive pituitary adenomas, especially in ACTHomas (62). ATRX gene mutations are associated with a lack of ATRX expression in tumor specimens. Loss of function somatic mutations have been found in the CABLES1 gene in children or young adult patients with aggressive corticotroph adenomas (63).
As germline mutations, MEN1, PRKAR1A, CDKN1B, and AIP genes should be considered as a young age-onset genetic syndrome phenotype (64). In aggressive pediatric Cushing’s syndrome, the DICER1 gene has been reportedly identified to have a causal role in ACTH-producing pituitary blastoma caused by DICER1 syndrome (65–67) (Table 1). Since DICER1 is an enzyme required for the cleavage of a precursor into mature microRNA, noncoding RNA, including microRNA, are associated with the pathogenesis of pituitary ACTH-producing tumors and their aggressiveness.
Crooke’s Cell Adenomas (CCAs)
The genetic causes of CCAs have not been elucidated. In histopathologic findings, the Ki-67 score has limitations in predicting tumor proliferation and aggressiveness (6, 19). Rather than the Ki-67 labeling index, miR-106b–25 and its host gene MCM7, a member of the minichromosome maintenance complex (MCM) family of proteins, have been shown to be novel markers that correlate with tumor recurrence and progression in invasive ACTH-producing pituitary adenomas, including CCAs (68, 69).
Nelson’s Syndrome
The underlying mechanisms of pituitary tumorigenesis and autonomous ACTH secretion in Nelson’s syndrome are not fully understood. Corticotropin-releasing hormone (CRH) hyperactivity induced by rapid cortisol reduction is thought to be one of the pathogenesis of its marked ACTH elevation and aggressive tumor enlargement. These tumors were originally derived from monoclonal cells (70, 71). Essential transcription factors (e.g., Ptx1, Tpit, NeuroD, Nur77) in corticotroph cells and POMC gene posttranscriptional processes are properly conserved, which results in a mature POMC product (72–74). Regarding the molecular function of corticotroph tumors, CRHR1 and AVPR1b receptors on the tumor exhibit good responsiveness to their ligands (72–75). Intriguingly, loss of heterozygosity of the glucocorticoid receptor (GR) gene has been reported in patients with Nelson’s syndrome, while GR expression of the corticotroph tumor in Nelson’s syndrome is conserved, similar to that in Cushing’s disease (76, 77). In some tumors in Nelson’s syndrome, TP53 loss of function has been identified after radiation therapy (78). The primary management mode (pituitary surgery and radiotherapy (RT) followed by adrenalectomy) of Cushing’s disease before the diagnosis of Nelson’s syndrome has been reported as the highest risk and a predictor of tumor progression (79).
Pituitary Carcinomas
The pathogenesis of pituitary carcinomas is not fully understood due to its low frequency. However, TP53 variants or ATRX variants have been shown in some corticotroph pituitary carcinomas (59, 62). Lynch syndrome, which is caused by MSH2 gene mutation complicated with pituitary carcinoma, has been reported as a case report, showing an association between this tumor-prone syndrome and pituitary tumors (80). However, further investigations need to clarify the tumor transformation’s underlying mechanism to the malignant behavior in pituitary adenomas.
Silent Corticotroph Adenomas
The transformation from functioning ACTHomas into SCAs is very rare (3.9%) (52). From an autopsy pathological study of the human pituitary gland, it has been suggested that SCA originates from pars intermedia POMC-positive cells, while ACTHomas originate from the anterior lobe (46). This hypothesis was confirmed by an animal study using tamoxifen-inducible Pax7CreERp/WT Rbflox/flox mice, which revealed that Rb loss in the Pax7-expressing pituitary intermediate lobe results in cell proliferation leading to tumorigenesis expressing POMC without circulating ACTH elevation (81). Regarding the genetic cause of SCAs, candidate genes have not yet been clarified, and the USP8 mutation commonly found in functioning and silent corticotroph adenomas (30, 82). Immunohistochemical analysis of SCA has demonstrated that lower expression of some proteins implicated in tumor progression and metastasis, such as galectin-3, a beta-galactoside-binding protein, and KLK10, belonging to the kallikrein family, in SCA than functioning ACTHomas may be one mechanism of its aggressiveness (30). Lower expression of CDKN2A with upregulated cyclin D1 in SCA than functioning ACTHomas has been shown to be another reason for its aggressive behavior (83). Recently, gene and protein expression comparison analysis between SCAs and functioning ACTHomas has been performed using both RNA-seq and mass spectrometry-based proteomics technology, revealing the downregulation of the gene related to protein processing in the endoplasmic reticulum (ER) pathway and upregulation of PCSK1N, an inhibitor of PC1/3 coding PCSK1 gene. These results suggest a reason for the lack of active ACTH secretion from these tumors. Moreover, the extracellular matrix (ECM) protein cluster is downregulated in SCAs compared to functioning ACTHomas, suggesting that this is associated with their invasive behavior (84).
Treatment
Surgery is the first-line treatment to control tumor volume for refractory corticotroph tumors, even though the postoperative recurrence rate remains high (8, 85). Radiosurgery is an important option for treating postoperative residual tumor or recurrence and progression of tumors. Stereotactic radiosurgery is especially superior to radiosurgery in terms of a lower incidence of adverse events and earlier remission (86, 87). In aggressive ACTHomas, medical therapy is required, followed by surgery and radiosurgery in most cases. Although hypercortisolemia can be controlled by adrenal or GR-targeted drugs, the effect of targeted therapy on ACTHoma remains a challenge. Medical treatment can be initiated immediately after the diagnosis until hypercortisolemia is collected, including the perioperative period.
Surgery
Surgical treatment remains the first-line treatment choice even for aggressive ACTHomas by a skilled neurosurgeon with extensive experience in pituitary surgery (88). Endoscopic or microscopic TSS can be performed according to the neurosurgeon’s preference (89). Preoperative medical treatment to improve hypercortisolemia is recommended, mainly using steroidogenesis inhibitors, including metyrapone, ketoconazole, and osilodrostat with or without hydrocortisone replacement (90–92). Since a higher rate of morbidity, including poorly controlled diabetes mellitus, hypokalemia, venous thromboembolism, gastrointestinal hemorrhage, and osteoporosis, has been complicated in patients with refractory Cushing’s disease, several pharmaceutical treatments such as insulin, mineral corticoid antagonists, anticoagulants, proton pump inhibitors, and anti-osteoporotic agents are required during the perioperative period (91, 93). In a recent systematic review, the complete surgical remission and recurrence rates of macro-tumors in primary surgery were 68% (95% confidence interval [CI]; 60–76) and 30% (95% CI; 18–43), and those in revision surgery are 49% (95% CI; 23–75) and 45% (95% CI; 0–98), respectively (94). If the tumor extends into the suprasellar region, a transcranial approach may be needed (88). In a literature review of initial surgery for ACTHomas, a tumor with Knosp grades 3–4 have been identified in 12–20% of all tumors, and 12 of 36 patients (33.3%) have achieved the remission criteria (95), indicating that further treatment option is emergently required for these tumors. In Nelson’s syndrome, pituitary surgery is the first-line treatment; however, the complete remission rate depends on whether the pituitary tumor extends to the extrasellar region, similar to an ordinal pituitary tumor (96).
Radiotherapy (RT)
Stereotactic RT (SRT), including the Gamma Knife™ (GK), Cyberknife™, and proton-beam RT, has become the mainstream rather than the conventional fractionated RT (CRT) and could be a second treatment option for aggressive Cushing’s disease if residual or recurrent tumors are visible on MRI despite TSS (97). Because of the recent development of drug therapy, the choice of second-line therapy needs to be individualized according to tumor progression speed by MRI, pathological findings, and patient background. In a systematic review from 2000 to 2017, the tumor was controlled in 95% of cases (83.3–100%) with a median follow-up of 56 months (2–17 years). Hormonal control has achieved 54–68% in SRT with a follow-up of 5–10 years, while the definition of biochemical remission is not unified. However, the recurrence rate of RT is 20–32% with a median time of 25.5–37 months (range 6–60) after an initial remission. Adverse radiation effects for patients with Cushing’s disease including hypopituitarism [12.3–52% (median 22.6%)], visual toxicity (0–39%), and cranial nerve neuropathy (0–5.5%) have reported, while secondary brain tumors have not occurred yet. The median time to hormonal normalization is 12–25 months (98–102). In aggressive Cushing tumors, the mean time of hormonal control may have taken a longer period than that in those with nonaggressive ones (33.0 ± 5.0 vs. 23.5 ± 6.3 months) (101). In patients with CCAs, SRT has shown to be as effective as ACTHomas without Crooke’s hyaline changes (87).
Medical Therapy
There are three therapeutic targets for drug therapy in patients with Cushing’s disease: pituitary directed therapies, adrenal directed therapies, and cortisol-target tissues. In aggressive Cushing’s disease, which is usually accompanied by remarkable hypercortisolemia, adrenal gland-targeted steroidogenesis inhibitors, including ketoconazole, metyrapone, and etomidate, can be the first choice for acute phase intervention or preoperative treatment. Further medical treatment might be required in the chronic phase, or if there are residual tumors that oversecrete ACTH after the operation of the tumors. Pituitary gland-targeted drugs, such as second-generation somatostatin receptor ligands (SRLs), pasireotide, cabergoline is a dopamine receptor agonist, could be the next treatment choice with or without steroidogenesis inhibitors.
Our manuscript mainly focused on pituitary gland-targeted therapies including currently approved and further developing drugs (Figure 1), besides described adrenal gland-targeted drugs and peripheral GR blockers.
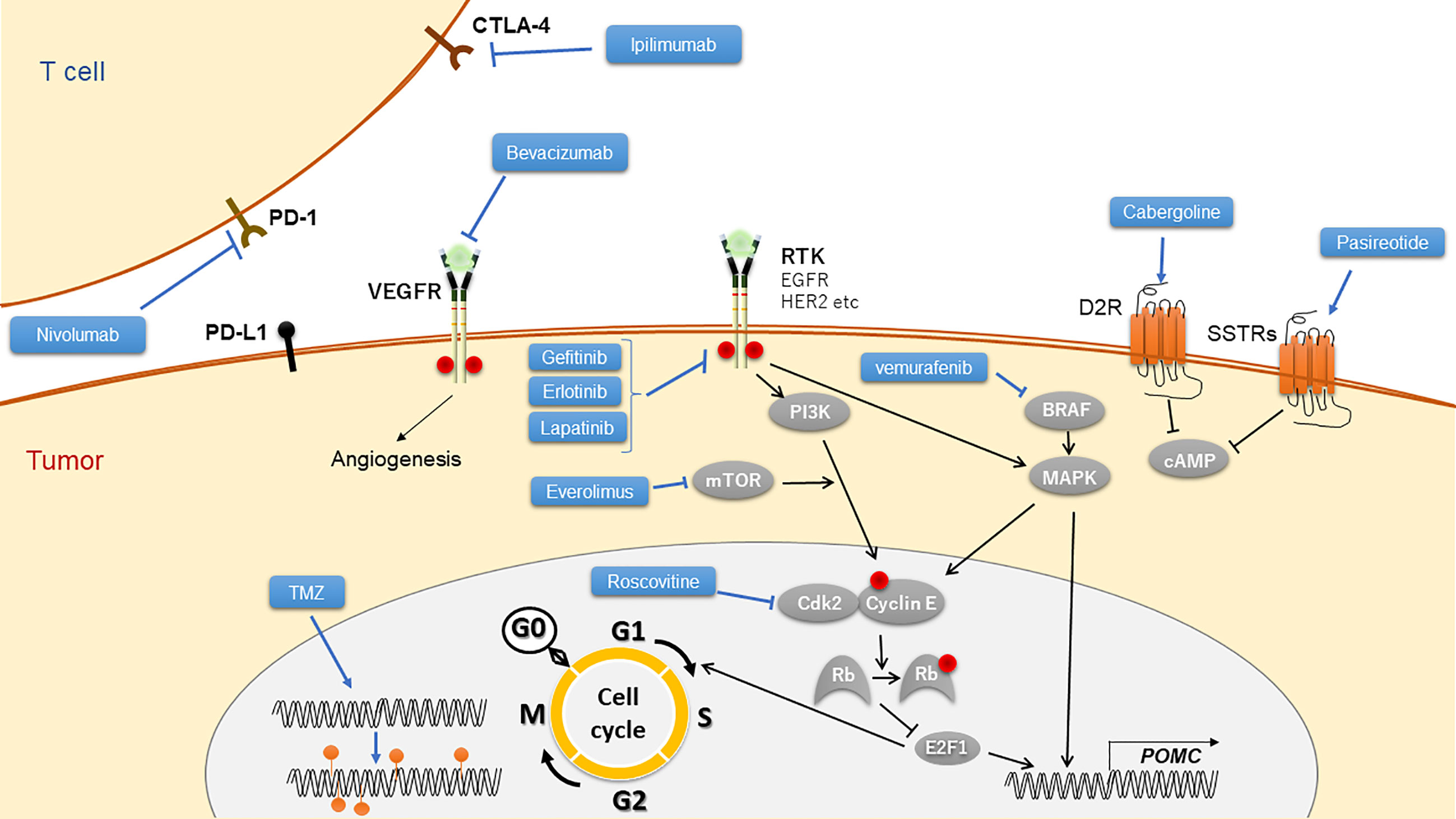
Figure 1 Targeted drugs to Cushing’s disease and their mechanistic scheme. TMZ, temozolomide; RTK, receptor of tyrosine kinase.
Somatostatin Receptor Ligands
First-generation SRLs, octreotide and/or lanreotide, which mainly target SSTR2, are not effective in most ACTHomas because of their low expression of membrane SSTR2. Pasireotide, a second-generation SRL that targets SSTR1, 2, 3, and 5 with the highest affinity for SSTR5, has been approved as a promising drug for the treatment of ACTHomas (103, 104). In a recent meta-analysis, pasireotide was shown to be effective in normalizing cortisol in 41.1% (95% CI, 32.7–49.8) of patients (105).
In ACTH-secreting macroadenomas, ACTH reduction and tumor shrinkage have been reported by initial treatment of pasireotide (106). Furthermore, the effectiveness of rapid ACTH and cortisol suppression as preoperative treatment has been shown in several ACTH-secreting macroadenoma cases (107). Conversely, escape from ACTH reduction, or even a paradoxical rise of ACTH has been reported (108), indicating further investigation to clarify the effect of this drug on aggressive ACTHomas.
The effect of pasireotide on CCAs is still under debate and requires further studies to clarify the efficacy of such challenging aggressive tumors. In Nelson’s syndrome, a sufficient effect on ACTH reduction and tumor shrinkage has been reported in a case report of pasireotide (109). A multicenter trial of pasireotide treatment for Nelson’s syndrome has been reported (110). In this trial, patients were treated with subcutaneous (s.c.) pasireotide twice daily for 1 month (n=8), followed by treatment with monthly pasireotide LAR for 6 months (n=5). ACTH reduction showed a complete response (CR) in five out of eight patients and partial response (PR) in two out of eight patients by s.c. pasireotide and further exhibited a CR in three out of five patients and PR in one out of five patients treated with pasireotide LAR. However, tumor shrinkage was not observed with hyperglycemia in six patients.
SCAs also exhibit a higher expression of SSTR2 and SSTR5 compared to null cell adenomas and SGAs by immunohistochemical analysis (40, 111). SSTR3 is also abundantly expressed in SCAs (40). Although the efficacy of somatostatin analogs for SCAs has not yet been established, pasireotide LAR (PASSILCORT; ClinicalTrial.gov identifier, NCT02749227) is under a phase II randomized clinical trial for residual or recurrent SCAs.
Dopamine Receptor Agonist
Since dopamine 2 receptor (D2R) is frequently expressed on ACTHomas, the dopamine receptor agonist cabergoline has shown to be an effective drug in approximately 20–30% of patients with Cushing’s disease (112–114). In a recent multicenter study, the efficacy of cabergoline in hormone reduction did not differ between microadenomas and macroadenomas (112). The effect of cabergoline on CCAs remains unclear (8). Although some case reports show that Nelson’s syndrome has been successfully treated with cabergoline (115, 116), the efficacy of cabergoline for such aggressive tumors is limited (79). In SCAs, a case report revealed that cabergoline has been shown to induce tumor shrinkage in a patient with SCA, in which D2R expression has been proved by in situ hybridization (117). However, SCAs have been reported to exhibit lower D2R mRNA levels than ACTH-negative nonfunctioning adenomas (118).
Temozolomide
Temozolomide is the drug with the most developed evidence for the treatment of aggressive pituitary adenomas and pituitary carcinomas. Although insurance is not covered in most countries, temozolomide is a promising therapeutic choice for refractory hormone-secreting and non-secreting pituitary tumors, including Cushing’s disease.
This drug was initially used in the treatment of glioblastoma multiforme (GBM) because of its significant clinical benefits. It is an alkylating agent that methylates specific guanine residue, leading to DNA damages by triggering tumor apoptosis. However, the existence of O6-methylguanine-DNA methyltransferase (MGMT), a DNA repair enzyme that can remove the methyl from the O6-methylguanine, works in contrast with temozolomide. Therefore, high MGMT expression levels in GBM tumors are related to drug resistance (119).
The first two cases of pituitary carcinomas were reported in 2006, who were successfully treated with temozolomide after initial therapy including surgery, dopamine agonists, somatostatin analogs, radiation, and chemotherapy (120). Following this report, more than 150 cases with pituitary carcinomas or aggressive pituitary adenomas have been treated with temozolomide, demonstrating a 69% (33–86%) response rate, which is defined as either a complete remission (CR), partial response (PR), or stable disease (SD). Additionally, 42% (29–69%) of significant tumor volume reduction has been shown to be either a CR or PR (88).
The reduction in tumor size occurred within 1–6 months after initiation of temozolomide therapy. Noting a report from a European cohort, after 3 (6) cycles of temozolomide, 23% (59%) achieved maximal radiological response, indicating that approximately 40% of patients experienced maximal radiological tumor response after 6 months (88, 121). Moreover, a German survey also reported that 52% of corticotroph tumors showed regression, 21% stability, and 26% progression at the end of temozolomide treatment. After a median follow-up of the 32-month radiological evaluation, disease stabilization in 37% and progression in 63% of patients were observed (121). These results are consistent with previous findings in Italy and France (122).
Although randomized prospective trials, or head-to-head studies of temozolomide compared to placebo or other treatment options, have not been performed yet, temozolomide can be a potential recommended therapy of choice for aggressive pituitary adenomas and pituitary carcinomas.
In aggressive pituitary tumors, Cushing’s disease is the most common type, with 45% of adenomas and 47% of carcinomas (88). Generally, functioning tumors, especially prolactinomas and corticotroph adenomas, have been reported to have a better response to temozolomide than nonfunctioning tumors. The response rate of temozolomide in corticotroph tumors is estimated to be 56%, compared to 44% in prolactinomas, 38% in somatotroph tumors, and only 22% in nonfunctioning tumors (89), indicating that aggressive corticotroph adenoma and corticotroph carcinomas are good candidates for temozolomide treatment. In fact, five cases of CCAs, which showed lower MGMT expression than noninvasive ACTHomas, treated with temozolomide, were shown to have a partial or complete reduction of tumor size in all cases. Plasma ACTH levels in these cases have also been suppressed, except for one case in which laboratory data were not documented (123). In addition, a German survey reported that ACTH decreased from 42 (9–794) pmol/L at baseline to a minimum of 23 (10–276) pmol/L after a median of 6 (3–10) months on temozolomide and then increased to 182 (12–671) pmol/L at the end of temozolomide treatment (122).
In children, temozolomide treatment for aggressive pituitary adenoma and carcinoma is quite rare, which leads to insufficient treatment data. In limited cases of DICER1 mutations with ACTH-secreting pituitary tumors, the effect of temozolomide has not been clearly shown (65, 124). Although there is no established course of treatment because of the paucity of data, the ESE guidelines suggest that temozolomide might be beneficial in adults (88). The common adverse events observed were similar to those observed in adults, including diarrhea, constipation, nausea, thrombocytopenia, headaches, syncope, and somnolence (36, 125–127).
In SCAs, temozolomide is considered a possible chemotherapeutic agent because of its low expression of MGMT (36, 126, 127). Several case reports of SCAs treated with temozolomide have been reported. Ceccato et al. reported two SCA patients treated with temozolomide: one associated with pasireotide treatment showed stable disease (SD) with 6% volume reduction and the other showed partial response (PR) with 49% volume reduction (128). A systematic review and meta-analysis reported that the recurrence rate of SCAs with a mean follow-up of <5 years or >5 years was 25% and 31%, respectively, and there was no significant difference in the recurrence rates between SCAs and other nonfunctioning pituitary adenomas (129). However, it should be noted that there are many unresolved points because of the rarity of SCAs.
When pituitary tumors show metastatic spread or are refractory to multiple treatments, temozolomide could be the last resort and salvage therapy. However, some recent studies have suggested that early use of temozolomide in these patients could result in a better outcome (88, 121). In this regard, high-grade tumors on MRI, such as invasiveness and increasing tumor size, and pathological findings, including high MIB1-labeling index, could be a sign of temozolomide initiation after surgery under RT (130, 131). However, patients administered with temozolomide in the early stage are relatively rare. Further clinical investigations are needed to determine whether early administration of temozolomide in patients with aggressive or metastatic pituitary tumors is associated with better outcomes.
Tumors resistant to TMZ chemotherapy have been shown in a certain number of refractory pituitary tumors (130). Thus, a predictive marker for resistance to temozolomide needs to be identified. As shown in GBM, low MGMT expression, a beneficial predictor of the response to temozolomide in glioblastoma (119), has been mostly associated with a positive response to temozolomide in pituitary tumors. However, some discrepancies, such as a high MGMT with a lack of response and no response despite low MGMT expression, have also been reported in pituitary tumors (88). Furthermore, no statistical association between MGMT expression levels and resistance to temozolomide has been shown (121, 122), indicating the limitation of MGMT as a predictive marker in these tumors. In addition to MGMT, several DNA mismatch repair (MMR) pathway proteins have been proposed, including MLH1, MSH2, MSH6, and PMS2, which recognize adducts including O6-methylguanin and remove them, leading to cell death (132). Therefore, the expression levels of MMR proteins may be critical to the cytotoxic effects of temozolomide (88). In fact, MSH6 immunopositivity has been associated with responsiveness to temozolomide in malignant pituitary neoplasms (133). Further analysis of the relationship between MMR pathway protein expression levels and temozolomide responsiveness is required (132). Overall, the expression of DNA repair proteins, including MGMT, may be associated with resistance to temozolomide treatment but is still controversial.
Chemotherapy
There are no established chemotherapy protocols for pituitary carcinomas. Mono- or combination therapy using chemotherapy, including capecitabine, carboplatin, etoposide, cisplatin, doxorubicin, 5-fluorouracil, tamoxifen, cyclophosphamide, lomustine, procarbazine, vincristine, oxaliplatin, dacarbazine, methotrexate, bleomycin, and cyclohexyl-chloroethyl-nitrosourea, has been used for several pituitary carcinoma cases, with some PR (20, 134–136).
Immune Therapy
As a successful immunotherapeutic strategy, immune checkpoint inhibitors (ICIs) for several cancers, has recently been developed. These targets include cytotoxic T lymphocyte antigen 4 (CTLA-4) and programmed cell death protein 1 (PD-1), located in T cells, and ligand for PD-1 (PD-L1), located in tumor cells. Ipilimumab, the first developed ICIs targeting CTLA-4; nivolumab, pembrolizumab, and cemiplimab targeting PD-1; and atezolizumab, avelumab, and durvalumab targeting PD-L1, have been approved and applied for the treatment of several cancers. For pituitary tumors, the first case treated with ICIs has been reported to have ACTH-secreting pituitary carcinoma exhibiting liver metastasis (137). In this patient, initial treatment, including TSSs, fractionated RT and pasireotide, and cabergoline, was performed followed by TMZ and a combination of TMZ and capecitabine. Since the tumor volume and hormonal hypersecretion were not controlled despite these treatments, the combination of ipilimumab (3 mg/kg every 3 weeks) and nivolumab (1 mg/kg every 3 weeks) was initiated, leading to regression of both sellar tumors and metastatic liver tumors (59% and 92%, respectively) with a 90% reduction in ACTH levels. In this report, genetic analysis of primary and metastatic tumors revealed several pathogenic somatic gene hypermutations possibly induced by medical therapy such as TMZ, which can be neoantigens for the targets of ICIs. Following this report, successful treatment of a second corticotroph carcinoma case derived from Nelson’s syndrome, with a combination of ipilimumab (3 mg/kg) and pembrolizumab (1 mg/kg) every 3 weeks, leading to stable disease, has been reported (138). Immunotherapy could be the next possible therapeutic candidate for aggressive ACTHomas.
Possible Targeted Therapy
Drug repositioning from several targeted therapies for cancers, including neuroendocrine tumors to aggressive pituitary tumors, has been investigated using receptors for tyrosine kinases, including EGFR, human epidermal growth factor receptor 2 (HER2), vascular endothelial growth factor receptor (VEGFR), intracellular signal transduction pathway proteins such as the mammalian target of rapamycin (mTOR), BRAF, and nuclear proteins such as cyclin-dependent kinase (CDK) (136, 139).
In ACTHomas, EGFR has been shown to be a tumorigenic factor, especially in USP8 variants (53, 56, 140). Although ACTHomas with USP8 mutations have been shown to be small and nonaggressive, EGFR overexpression in ACTHomas has been reported to be associated with aggressive ACTHomas via the activated MAPK pathway (141). Since EGFR can induce experimental corticotroph tumor proliferation both in vitro and in vivo, its tyrosine kinase inhibitor (TKI) gefitinib has been shown to reduce serum corticosterone levels with shrinking pituitary tumors of corticotroph-specific EGFR overexpressing mice, an animal model of Cushing’s disease (55, 56). EGFR TKI erlotinib and dual EGFR and HER2 TKI lapatinib have been used to treat aggressive pituitary tumors as a third-line treatment, revealing poor outcomes (142). Recently, lapatinib treatment for aggressive PRLomas has been reported to have a partial effect on tumor shrinkage and hormonal reduction (143). Further investigation of EGFR targets is required for aggressive corticotroph tumors.
VEGF inhibitors, including TKIs for VEGFR2, and monoclonal neutralizing antibodies against VEGF-A have been used for several vascular-rich cancers, including neuroendocrine neoplasms. The rationale of these drugs is to suppress angiogenesis, leading to the suppression of tumor growth and induction of shrinkage. Bevacizumab, a humanized monoclonal antibody for VEGF-A, has been used to treat SCA, leading to stable disease for at least 26 months (144). Eight years of PFS with RT, TMZ, and bevacizumab has been reported (145). Five more cases of ACTHoma treated with bevacizumab have been reported, showing some effectiveness (146) since VEGF can also modulate the tumor microenvironment, their inhibition can act as an antitumor immunity (147).
In pituitary adenomas, mTOR expression has been shown to be higher than those in a normal pituitary gland and is elevated in invasive tumors (148, 149), suggesting a potential therapeutic target of mTOR for aggressive ACTHomas. Everolimus, an mTOR inhibitor, has been approved for several cancer treatments, including neuroendocrine neoplasms (150). Everolimus has been reported as an effective therapy for STK11 mutated refractory ACTHoma with clinical improvement and stable disease for at least 6 months (151). In contrast, one case of corticotroph carcinoma was treated with everolimus with octreotide, exhibiting resistance in both tumoral growth and hormone secretion. According to the microarray investigation, regulatory associated protein of mTOR (RAPTOR) mRNA expression was low, suggesting the cause of everolimus resistance in corticotroph carcinoma (152).
In ACTHomas with BRAF, V600E mutation, a rare variant, has been shown to be a good candidate for the treatment with the BRAF inhibitor vemurafenib (57).
From the Cushing’s disease model of corticotroph-specific pituitary tumor transforming gene (PTTG) transgenic zebrafish, drug screening has been performed, identifying that the CDK2/cyclin E inhibitor, R-roscovitine, could be a potential drug for human ACTHomas (153, 154).
Adrenal Gland-Targeted Drugs
Steroidogenesis Inhibitors
Adrenal steroidogenesis inhibitors block cortisol synthesis by inhibiting various enzymes in steroidogenesis pathway while they have no evidence in corticotroph tumor shrinkage.
Ketoconazole is known as an anti-fungal biotics, which can inhibit cholesterol side-chain cleavage enzyme such as 17α-hydroxylase and 17, 20-lyase and 11β-hydroxylase (155). Ketoconazole which has numerous evidence in treating hypercortisolemia due to Cushing’s syndrome, exhibited high remission rate from 45 to 93% (91). Liver enzyme elevation is one of the most common side effects, which was observed in 13.5% of patients. As other adverse events, gastrointestinal disturbances and male hypogonadism should be considered (91).
Mitotane is currently approved for treating adrenocortical carcinoma, also widely used for Cushing’s syndrome, by inhibiting not only steroidogenesis but also inducing cell death of adrenocortical cells. According to a recent meta-analysis, mitotane exhibited high remission rate in treating Cushing’s syndrome (105), While dyslipidemia, gastrointestinal disturbances and neurological disorders are frequently observed adverse events, mitotane-induced adrenal insufficiency requires special caution, which demand more glucocorticoid dose than physiological setting.
Metyrapone is widely used as a steroidogenesis inhibitor for Cushing’s syndrome even though it has been still in off-label use in US. Metyrapone inhibits 11β-hydroxylase and converting from 11-deoxycortisol to cortisol, results in reducing cortisol level. Metyrapone showed a revised estimated average remission rate of 75.9% (105) The frequently reported adverse events were hirsutism in women, dizziness, arthralgias, gastrointestinal disturbances, adrenal insufficiency, hypokalemia and peripheral edema (156).
Novel Steroidogenesis Inhibitors
Levoketoconazole
Levoketoconazole which is an enantiomer of ketoconazole, was developed for achievement of better efficacy and safety. Levoketoconazole inhibits 21-hydroxylase, 17 alpha-hydroxylase, and 11 beta-hydroxylase steroidogenesis enzymes, resulted in exhibiting higher potency than ketoconazole (157). In phase 3 clinical trial, treated 81% patients with levoketoconazole achieved normalization of UFC level. While most of adverse events such as nausea and headache were acceptable, 13% of patients was obliged to discontinue the drug due to serious adverse events such as abnormal liver functio, prolonged QT interval, and adrenal insufficiency (158).
Osilodrostat
Osilodrostat is novel 11 beta-hydroxylase inhibitor that blocks the conversion from deoxycortisol to cortisol, which has similar action mechanism with metyrapone. Osilodrostat exhibited 3-fold higher affinity to 11 beta-hydroxylase and longer half-life than metyrapone. In please II clinical trial, osilodrostat treatment reduced UFC in 78.9% of patients at week 22 (159). Adverse events were very similar with those of other steroidogenesis inhibitors, including nausea, diarrhea, asthenia, adrenal insufficiency, and hirsutism in female (160).
Glucocorticoid Receptor-Directed Drugs
Mifepristone is officially approved non-selective GR antagonist for treating Cushing’s syndrome. The data from a multicenter, open-label, prospective clinical trial showed the improvement of clinical features associated with hypercortisolemia, psychiatric symptoms and glucose intolerance (161). On the other hand, specific inhibition of GR action causes hyperaldosteronism-like phenotype due to cortisol binding to MR, such as hypertension and hypokalemia. Apart from that, various adverse events also have been reported as follows: nausea, fatigue, and endometrial thickening in women (162).
Conclusions
In this review article, we introduced several aggressive types of ACTHomas, including CCAs, Nelson’s syndrome, and SCAs. The pathogenesis and treatment of these tumors have been introduced. Although numbers of genetic variants and mutations are implicated in ACTHomas, their mechanistic link to the aggressiveness and, more importantly, to therapeutical targeting are yet to be established. Future targeted drugs and immunotherapy are shown with their potential evidence. Further analysis and investigation are urgently required for this clinically serious disease.
Author Contributions
MY wrote the sections “Aggressive ACT Homas” and “Molecular Pathology”. TN wrote the section “Temozolomide”. The rest was written by HF. HF and WO planned and edited this review. All authors contributed to the article and approved the submitted version.
Funding
Grant-in-Aid for Scientific Research from the Japanese Ministry of Education, Culture, Sports, Science and Technology 15K09432, 19K09003.
Conflict of Interest
The authors declare that the research was conducted in the absence of any commercial or financial relationships that could be construed as a potential conflict of interest.
References
1. Di Ieva A, Rotondo F, Syro LV, Cusimano MD, Kovacs K. Aggressive Pituitary Adenomas–Diagnosis and Emerging Treatments. Nat Rev Endocrinol (2014) 10(7):423–35. doi: 10.1038/nrendo.2014.64
2. Crooke AC. Change in the Basophil Cells of the Pituitary Gland Common to Conditions Which Exhibit the Syndrome Attributed to Basophil Adenoma. J Pathol Bacteriol (1935) 41(2):339–49. doi: 10.1002/path.1700410215
3. Oldfield EH, Vance ML, Louis RG, Pledger CL, Jane JA Jr., Lopes MB. Crooke’s Changes in Cushing’s Syndrome Depends on Degree of Hypercortisolism and Individual Susceptibility. J Clin Endocrinol Metab (2015) 100(8):3165–71. doi: 10.1210/JC.2015-2493
4. Felix IA, Horvath E, Kovacs K. Massive Crooke’s Hyalinization in Corticotroph Cell Adenomas of the Human Pituitary. A Histological, Immunocytological, and Electron Microscopic Study of Three Cases. Acta Neurochir (Wien) (1981) 58(3-4):235–43. doi: 10.1007/BF01407130
5. Saeger W, Ludecke DK, Buchfelder M, Fahlbusch R, Quabbe HJ, Petersenn S. Pathohistological Classification of Pituitary Tumors: 10 Years of Experience With the German Pituitary Tumor Registry. Eur J Endocrinol (2007) 156(2):203–16. doi: 10.1530/eje.1.02326
6. George DH, Scheithauer BW, Kovacs K, Horvath E, Young WF Jr., Lloyd RV, et al. Crooke’s Cell Adenoma of the Pituitary: An Aggressive Variant of Corticotroph Adenoma. Am J Surg Pathol (2003) 27(10):1330–6. doi: 10.1097/00000478-200310000-00005
7. Holthouse DJ, Robbins PD, Kahler R, Knuckey N, Pullan P. Corticotroph Pituitary Carcinoma: Case Report and Literature Review. Endocr Pathol (2001) 12(3):329–41. doi: 10.1385/EP:12:3:329
8. Di Ieva A, Davidson JM, Syro LV, Rotondo F, Montoya JF, Horvath E, et al. Crooke’s Cell Tumors of the Pituitary. Neurosurgery (2015) 76(5):616–22. doi: 10.1227/NEU.0000000000000657
9. Nelson DH, Meakin JW, Dealy JB Jr, Matson DD, Emerson K Jr., Thorn GW. ACTH-Producing Tumor of the Pituitary Gland. N Engl J Med (1958) 259(4):161–4. doi: 10.1056/NEJM195807242590403
10. Nagesser SK, van Seters AP, Kievit J, Hermans J, Krans HM, van de Velde CJ. Long-Term Results of Total Adrenalectomy for Cushing’s Disease. World J Surg (2000) 24(1):108–13. doi: 10.1007/s002689910020
11. Kemink SA, Grotenhuis JA, De Vries J, Pieters GF, Hermus AR, Smals AG. Management of Nelson’s Syndrome: Observations in Fifteen Patients. Clin Endocrinol (Oxf) (2001) 54(1):45–52. doi: 10.1046/j.1365-2265.2001.01187.x
12. Barnett AH, Livesey JH, Friday K, Donald RA, Espiner EA. Comparison of Preoperative and Postoperative ACTH Concentrations After Bilateral Adrenalectomy in Cushing’s Disease. Clin Endocrinol (Oxf) (1983) 18(3):301–5. doi: 10.1111/j.1365-2265.1983.tb03215.x
13. Pereira MA, Halpern A, Salgado LR, Mendonca BB, Nery M, Liberman B, et al. A Study of Patients With Nelson’s Syndrome. Clin Endocrinol (Oxf) (1998) 49(4):533–9. doi: 10.1046/j.1365-2265.1998.00578.x
14. McCance DR, Russell CF, Kennedy TL, Hadden DR, Kennedy L, Atkinson AB. Bilateral Adrenalectomy: Low Mortality and Morbidity in Cushing’s Disease. Clin Endocrinol (Oxf) (1993) 39(3):315–21. doi: 10.1111/j.1365-2265.1993.tb02371.x
15. Banasiak MJ, Malek AR. Nelson Syndrome: Comprehensive Review of Pathophysiology, Diagnosis, and Management. Neurosurg Focus (2007) 23(3):E13. doi: 10.3171/foc.2007.23.3.15
16. Gil-Cardenas A, Herrera MF, Diaz-Polanco A, Rios JM, Pantoja JP. Nelson’s Syndrome After Bilateral Adrenalectomy for Cushing’s Disease. Surgery (2007) 141(2):147–51; discussion 51-2. doi: 10.1016/j.surg.2006.12.003
17. Leinung MC, Zimmerman D. Cushing’s Disease in Children. Endocrinol Metab Clin North Am (1994) 23(3):629–39. doi: 10.1016/S0889-8529(18)30089-6
18. Assie G, Bahurel H, Coste J, Silvera S, Kujas M, Dugue MA, et al. Corticotroph Tumor Progression After Adrenalectomy in Cushing’s Disease: A Reappraisal of Nelson’s Syndrome. J Clin Endocrinol Metab (2007) 92(1):172–9. doi: 10.1210/jc.2006-1328
19. Mete O, Lopes MB. Overview of the 2017 WHO Classification of Pituitary Tumors. Endocr Pathol (2017) 28(3):228–43. doi: 10.1007/s12022-017-9498-z
20. Yoo F, Kuan EC, Heaney AP, Bergsneider M, Wang MB. Corticotrophic Pituitary Carcinoma With Cervical Metastases: Case Series and Literature Review. Pituitary (2018) 21(3):290–301. doi: 10.1007/s11102-018-0872-8
21. Kaltsas GA, Nomikos P, Kontogeorgos G, Buchfelder M, Grossman AB. Clinical Review: Diagnosis and Management of Pituitary Carcinomas. J Clin Endocrinol Metab (2005) 90(5):3089–99. doi: 10.1210/jc.2004-2231
22. Veit JA, Boehm B, Luster M, Scheuerle A, Rotter N, Rettinger G, et al. Detection of Paranasal Ectopic Adrenocorticotropic Hormone-Secreting Pituitary Adenoma by Ga-68-DOTANOC Positron-Emission Tomography-Computed Tomography. Laryngoscope (2013) 123(5):1132–5. doi: 10.1002/lary.23867
23. Lopes MB, Scheithauer BW, Schiff D. Pituitary Carcinoma: Diagnosis and Treatment. Endocrine (2005) 28(1):115–21. doi: 10.1385/ENDO:28:1:115
24. Mete O, Asa SL. Clinicopathological Correlations in Pituitary Adenomas. Brain Pathol (2012) 22(4):443–53. doi: 10.1111/j.1750-3639.2012.00599.x
25. Mete O, Ezzat S, Asa SL. Biomarkers of Aggressive Pituitary Adenomas. J Mol Endocrinol (2012) 49(2):R69–78. doi: 10.1530/JME-12-0113
26. Colao A, Ochoa AS, Auriemma RS, Faggiano A, Pivonello R, Lombardi G. Pituitary Carcinomas. Front Horm Res (2010) 38:94–108. doi: 10.1159/000318499
27. Ragel BT, Couldwell WT. Pituitary Carcinoma: A Review of the Literature. Neurosurg Focus (2004) 16(4):E7. doi: 10.3171/foc.2004.16.4.8
28. Pernicone PJ, Scheithauer BW, Sebo TJ, Kovacs KT, Horvath E, Young WF Jr., et al. Pituitary Carcinoma: A Clinicopathologic Study of 15 Cases. Cancer (1997) 79(4):804–12. doi: 10.1002/(SICI)1097-0142(19970215)79:4<804::AID-CNCR18>3.0.CO;2-3
29. Heaney A. Management of Aggressive Pituitary Adenomas and Pituitary Carcinomas. J Neurooncol (2014) 117(3):459–68. doi: 10.1007/s11060-014-1413-6
30. Ben-Shlomo A, Cooper O. Silent Corticotroph Adenomas. Pituitary (2018) 21(2):183–93. doi: 10.1007/s11102-018-0864-8
31. Arnold PM, Ratnasingam D, O’Neil MF, Johnson PL. Pituitary Carcinoma Recurrent to the Lumbar Intradural Extramedullary Space: Case Report. J Spinal Cord Med (2012) 35(2):118–21. doi: 10.1179/2045772311Y.0000000055
32. Scheithauer BW, Kovacs K, Nose V, Lombardero M, Osamura YR, Lloyd RV, et al. Multiple Endocrine Neoplasia Type 1-Associated Thyrotropin-Producing Pituitary Carcinoma: Report of a Probable De Novo Example. Hum Pathol (2009) 40(2):270–8. doi: 10.1016/j.humpath.2008.06.013
33. Koyama J, Ikeda K, Shose Y, Kimura M, Obora Y, Kohmura E. Long-Term Survival With non-Functioning Pituitary Carcinoma - Case Report. Neurol Med Chir (Tokyo) (2007) 47(10):475–8. doi: 10.2176/nmc.47.475
34. Horvath E, Kovacs K, Killinger DW, Smyth HS, Platts ME, Singer W. Silent Corticotropic Adenomas of the Human Pituitary Gland: A Histologic, Immunocytologic, and Ultrastructural Study. Am J Pathol (1980) 98(3):617–38.
35. Raverot G, Wierinckx A, Jouanneau E, Auger C, Borson-Chazot F, Lachuer J, et al. Clinical, Hormonal and Molecular Characterization of Pituitary ACTH Adenomas Without (Silent Corticotroph Adenomas) and With Cushing’s Disease. Eur J Endocrinol (2010) 163(1):35–43. doi: 10.1530/EJE-10-0076
36. Cooper O. Silent Corticotroph Adenomas. Pituitary (2015) 18(2):225–31. doi: 10.1007/s11102-014-0624-3
37. Cho HY, Cho SW, Kim SW, Shin CS, Park KS, Kim SY. Silent Corticotroph Adenomas Have Unique Recurrence Characteristics Compared With Other Nonfunctioning Pituitary Adenomas. Clin Endocrinol (Oxf) (2010) 72(5):648–53. doi: 10.1111/j.1365-2265.2009.03673.x
38. Cheres AF, ElAsmar N, Rajpal A, Selman WR, Arafah BM. Perioperative Hypothalamic Pituitary Adrenal Function in Patients With Silent Corticotroph Adenomas. Pituitary (2017) 20(4):471–6. doi: 10.1007/s11102-017-0809-7
39. Yamada S, Ohyama K, Taguchi M, Takeshita A, Morita K, Takano K, et al. A Study of the Correlation Between Morphological Findings and Biological Activities in Clinically Nonfunctioning Pituitary Adenomas. Neurosurgery (2007) 61(3):580–4; discussion 4-5. doi: 10.1227/01.NEU.0000290906.53685.79
40. Oystese KA, Casar-Borota O, Normann KR, Zucknick M, Berg JP, Bollerslev J. Estrogen Receptor Alpha, a Sex-Dependent Predictor of Aggressiveness in Nonfunctioning Pituitary Adenomas: SSTR and Sex Hormone Receptor Distribution in NFPA. J Clin Endocrinol Metab (2017) 102(9):3581–90. doi: 10.1210/jc.2017-00792
41. Nishioka H, Inoshita N, Mete O, Asa SL, Hayashi K, Takeshita A, et al. The Complementary Role of Transcription Factors in the Accurate Diagnosis of Clinically Nonfunctioning Pituitary Adenomas. Endocr Pathol (2015) 26(4):349–55. doi: 10.1007/s12022-015-9398-z
42. Cazabat L, Dupuy M, Boulin A, Bernier M, Baussart B, Foubert L, et al. Silent, But Not Unseen: Multimicrocystic Aspect on T2-weighted MRI in Silent Corticotroph Adenomas. Clin Endocrinol (Oxf) (2014) 81(4):566–72. doi: 10.1111/cen.12443
43. Drummond J, Roncaroli F, Grossman AB, Korbonits M. Clinical and Pathological Aspects of Silent Pituitary Adenomas. J Clin Endocrinol Metab (2019) 104(7):2473–89. doi: 10.1210/jc.2018-00688
44. Thodou E, Argyrakos T, Kontogeorgos G. Galectin-3 as a Marker Distinguishing Functioning From Silent Corticotroph Adenomas. Horm (Athens) (2007) 6(3):227–32.
45. Mete O, Hayhurst C, Alahmadi H, Monsalves E, Gucer H, Gentili F, et al. The Role of Mediators of Cell Invasiveness, Motility, and Migration in the Pathogenesis of Silent Corticotroph Adenomas. Endocr Pathol (2013) 24(4):191–8. doi: 10.1007/s12022-013-9270-y
46. Horvath E, Kovacs K, Lloyd R. Pars Intermedia of the Human Pituitary Revisited: Morphologic Aspects and Frequency of Hyperplasia of POMC-peptide Immunoreactive Cells. Endocr Pathol (1999) 10(1):55–64. doi: 10.1007/BF02738816
47. Langlois F, Lim DST, Varlamov E, Yedinak CG, Cetas JS, McCartney S, et al. Clinical Profile of Silent Growth Hormone Pituitary Adenomas; Higher Recurrence Rate Compared to Silent Gonadotroph Pituitary Tumors, a Large Single Center Experience. Endocrine (2017) 58(3):528–34. doi: 10.1007/s12020-017-1447-6
48. Matsuno A, Okazaki R, Oki Y, Nagashima T. Secretion of High-Molecular-Weight Adrenocorticotropic Hormone From a Pituitary Adenoma in a Patient Without Cushing Stigmata. Case Report. J Neurosurg (2004) 101(5):874–7. doi: 10.3171/jns.2004.101.5.0874
49. Kovacs K, Horvath E, Bayley TA, Hassaram ST, Ezrin C. Silent Corticotroph Cell Adenoma With Lysosomal Accumulation and Crinophagy. A Distinct Clinicopathologic Entity. Am J Med (1978) 64(3):492–9. doi: 10.1016/0002-9343(78)90236-X
50. Artenstein AW, Opal SM. Proprotein Convertases in Health and Disease. N Engl J Med (2011) 365(26):2507–18. doi: 10.1056/NEJMra1106700
51. Tateno T, Izumiyama H, Doi M, Yoshimoto T, Shichiri M, Inoshita N, et al. Differential Gene Expression in ACTH -Secreting and non-Functioning Pituitary Tumors. Eur J Endocrinol (2007) 157(6):717–24. doi: 10.1530/EJE-07-0428
52. Righi A, Faustini-Fustini M, Morandi L, Monti V, Asioli S, Mazzatenta D, et al. The Changing Faces of Corticotroph Cell Adenomas: The Role of Prohormone Convertase 1/3. Endocrine (2017) 56(2):286–97. doi: 10.1007/s12020-016-1028-0
53. Reincke M, Sbiera S, Hayakawa A, Theodoropoulou M, Osswald A, Beuschlein F, et al. Mutations in the Deubiquitinase Gene USP8 Cause Cushing’s Disease. Nat Genet (2015) 47(1):31–8. doi: 10.1038/ng.3166
54. Ma ZY, Song ZJ, Chen JH, Wang YF, Li SQ, Zhou LF, et al. Recurrent Gain-of-Function USP8 Mutations in Cushing’s Disease. Cell Res (2015) 25(3):306–17. doi: 10.1038/cr.2015.20
55. Fukuoka H, Cooper O, Ben-Shlomo A, Mamelak A, Ren SG, Bruyette D, et al. EGFR as a Therapeutic Target for Human, Canine, and Mouse ACTH-secreting Pituitary Adenomas. J Clin Invest (2011) 121(12):4712–21. doi: 10.1172/JCI60417
56. Araki T, Liu X, Kameda H, Tone Y, Fukuoka H, Tone M, et al. Egfr Induces E2f1-Mediated Corticotroph Tumorigenesis. J Endocr Soc (2017) 1(2):127–43. doi: 10.1210/js.2016-1053
57. Chen J, Jian X, Deng S, Ma Z, Shou X, Shen Y, et al. Identification of Recurrent USP48 and BRAF Mutations in Cushing’s Disease. Nat Commun (2018) 9(1):3171. doi: 10.1038/s41467-018-05275-5
58. Sbiera S, Perez-Rivas LG, Taranets L, Weigand I, Flitsch J, Graf E, et al. Driver Mutations in USP8 Wild-Type Cushing’s Disease. Neuro Oncol (2019) 21(10):1273–83. doi: 10.1093/neuonc/noz109
59. Tanizaki Y, Jin L, Scheithauer BW, Kovacs K, Roncaroli F, Lloyd RV. P53 Gene Mutations in Pituitary Carcinomas. Endocr Pathol (2007) 18(4):217–22. doi: 10.1007/s12022-007-9006-y
60. Uzilov AV, Taik P, Cheesman KC, Javanmard P, Ying K, Roehnelt A, et al. USP8 and TP53 Drivers are Associated With CNV in a Corticotroph Adenoma Cohort Enriched for Aggressive Tumors. J Clin Endocrinol Metab (2020) 106:826–42. doi: 10.1210/clinem/dgaa853
61. Roussel-Gervais A, Couture C, Langlais D, Takayasu S, Balsalobre A, Rueda BR, et al. The Cables1 Gene in Glucocorticoid Regulation of Pituitary Corticotrope Growth and Cushing Disease. J Clin Endocrinol Metab (2016) 101(2):513–22. doi: 10.1210/jc.2015-3324
62. Casar-Borota O, Boldt HB, Engstrom BE, Andersen MS, Baussart B, Bengtsson D, et al. Corticotroph Aggressive Pituitary Tumours and Carcinomas Frequently Harbour ATRX Mutations. J Clin Endocrinol Metab (2020) 106:1183–94. doi: 10.1210/clinem/dgaa749
63. Hernandez-Ramirez LC, Gam R, Valdes N, Lodish MB, Pankratz N, Balsalobre A, et al. Loss-of-Function Mutations in the CABLES1 Gene are a Novel Cause of Cushing’s Disease. Endocr Relat Cancer (2017) 24(8):379–92. doi: 10.1530/ERC-17-0131
64. Chasseloup F, Pankratz N, Lane J, Faucz FR, Keil MF, Chittiboina P, et al. Germline CDKN1B Loss-of-Function Variants Cause Pediatric Cushing’s Disease With or Without an MEN4 Phenotype. J Clin Endocrinol Metab (2020) 105(6):1983–2005. doi: 10.1210/clinem/dgaa160
65. Martinez de LaPiscina I, Hernandez-Ramirez LC, Portillo N, Gomez-Gila AL, Urrutia I, Martinez-Salazar R, et al. Rare Germline Dicer1 Variants in Pediatric Patients With Cushing’s Disease: What Is Their Role? Front Endocrinol (Lausanne) (2020) 11:433. doi: 10.3389/fendo.2020.00433
66. Sahakitrungruang T, Srichomthong C, Pornkunwilai S, Amornfa J, Shuangshoti S, Kulawonganunchai S, et al. Germline and Somatic DICER1 Mutations in a Pituitary Blastoma Causing Infantile-Onset Cushing’s Disease. J Clin Endocrinol Metab (2014) 99(8):E1487–92. doi: 10.1210/jc.2014-1016
67. de Kock L, Sabbaghian N, Plourde F, Srivastava A, Weber E, Bouron-Dal Soglio D, et al. Pituitary Blastoma: A Pathognomonic Feature of Germ-Line DICER1 Mutations. Acta Neuropathol (2014) 128(1):111–22. doi: 10.1007/s00401-014-1285-z
68. Coli A, Asa SL, Fadda G, Scannone D, Chiloiro S, De Marinis L, et al. Minichromosome Maintenance Protein 7 as Prognostic Marker of Tumor Aggressiveness in Pituitary Adenoma Patients. Eur J Endocrinol (2016) 174(3):307–14. doi: 10.1530/EJE-15-0586
69. Garbicz F, Mehlich D, Rak B, Sajjad E, Maksymowicz M, Paskal W, et al. Increased Expression of the microRNA 106b~25 Cluster and its Host Gene MCM7 in Corticotroph Pituitary Adenomas is Associated With Tumor Invasion and Crooke’s Cell Morphology. Pituitary (2017) 20(4):450–63. doi: 10.1007/s11102-017-0805-y
70. Gicquel C, Le Bouc Y, Luton JP, Girard F, Bertagna X. Monoclonality of Corticotroph Macroadenomas in Cushing’s Disease. J Clin Endocrinol Metab (1992) 75(2):472–5. doi: 10.1210/jcem.75.2.1322426
71. Herman V, Fagin J, Gonsky R, Kovacs K, Melmed S. Clonal Origin of Pituitary Adenomas. J Clin Endocrinol Metab (1990) 71(6):1427–33. doi: 10.1210/jcem-71-6-1427
72. Dahia PL, Grossman AB. The Molecular Pathogenesis of Corticotroph Tumors. Endocr Rev (1999) 20(2):136–55. doi: 10.1210/edrv.20.2.0358
73. Raffin-Sanson ML, de Keyzer Y, Bertagna X. Proopiomelanocortin, a Polypeptide Precursor With Multiple Functions: From Physiology to Pathological Conditions. Eur J Endocrinol (2003) 149(2):79–90. doi: 10.1530/eje.0.1490079
74. de Keyzer Y, Bertagna X, Lenne F, Girard F, Luton JP, Kahn A. Altered Proopiomelanocortin Gene Expression in Adrenocorticotropin-Producing Nonpituitary Tumors. Comparative Studies With Corticotropic Adenomas and Normal Pituitaries. J Clin Invest (1985) 76(5):1892–8. doi: 10.1172/JCI112184
75. de Keyzer Y, Rene P, Lenne F, Auzan C, Clauser E, Bertagna X. V3 Vasopressin Receptor and Corticotropic Phenotype in Pituitary and Nonpituitary Tumors. Horm Res (1997) 47(4-6):259–62. doi: 10.1159/000185473
76. Huizenga NA, de Lange P, Koper JW, Clayton RN, Farrell WE, van der Lely AJ, et al. Human Adrenocorticotropin-Secreting Pituitary Adenomas Show Frequent Loss of Heterozygosity at the Glucocorticoid Receptor Gene Locus. J Clin Endocrinol Metab (1998) 83(3):917–21. doi: 10.1210/jc.83.3.917
77. Dahia PL, Honegger J, Reincke M, Jacobs RA, Mirtella A, Fahlbusch R, et al. Expression of Glucocorticoid Receptor Gene Isoforms in Corticotropin-Secreting Tumors. J Clin Endocrinol Metab (1997) 82(4):1088–93. doi: 10.1210/jcem.82.4.3861
78. Pinto EM, Siqueira SA, Cukier P, Fragoso MC, Lin CJ, de Mendonca BB. Possible Role of a Radiation-Induced p53 Mutation in a Nelson’s Syndrome Patient With a Fatal Outcome. Pituitary (2011) 14(4):400–4. doi: 10.1007/s11102-009-0194-y
79. Fountas A, Lim ES, Drake WM, Powlson AS, Gurnell M, Martin NM, et al. Outcomes of Patients With Nelson’s Syndrome After Primary Treatment: A Multicenter Study From 13 UK Pituitary Centers. J Clin Endocrinol Metab (2020) 105(5):dgz200. doi: 10.1210/clinem/dgz200
80. Bengtsson D, Joost P, Aravidis C, Askmalm Stenmark M, Backman AS, Melin B, et al. Corticotroph Pituitary Carcinoma in a Patient With Lynch Syndrome (LS) and Pituitary Tumors in a Nationwide Ls Cohort. J Clin Endocrinol Metab (2017) 102(11):3928–32. doi: 10.1210/jc.2017-01401
81. Hosoyama T, Nishijo K, Garcia MM, Schaffer BS, Ohshima-Hosoyama S, Prajapati SI, et al. A Postnatal Pax7 Progenitor Gives Rise to Pituitary Adenomas. Genes Cancer (2010) 1(4):388–402. doi: 10.1177/1947601910370979
82. Castellnou S, Vasiljevic A, Lapras V, Raverot V, Alix E, Borson-Chazot F, et al. SST5 Expression and USP8 Mutation in Functioning and Silent Corticotroph Pituitary Tumors. Endocr Connect (2020) 9:243–53. doi: 10.1530/endoabs.70.AEP617
83. Tani Y, Inoshita N, Sugiyama T, Kato M, Yamada S, Shichiri M, et al. Upregulation of CDKN2A and Suppression of Cyclin D1 Gene Expressions in ACTH-secreting Pituitary Adenomas. Eur J Endocrinol (2010) 163(4):523–9. doi: 10.1530/EJE-10-0245
84. Eieland AK, Normann KR, Sundaram AYM, Nyman TA, Oystese KAB, Lekva T, et al. Distinct Pattern of Endoplasmic Reticulum Protein Processing and Extracellular Matrix Proteins in Functioning and Silent Corticotroph Pituitary Adenomas. Cancers (Basel) (2020) 12(10):2980. doi: 10.3390/cancers12102980
85. Hammer GD, Tyrrell JB, Lamborn KR, Applebury CB, Hannegan ET, Bell S, et al. Transsphenoidal Microsurgery for Cushing’s Disease: Initial Outcome and Long-Term Results. J Clin Endocrinol Metab (2004) 89(12):6348–57. doi: 10.1210/jc.2003-032180
86. Sheehan JP, Niranjan A, Sheehan JM, Jane JA Jr., Laws ER, Kondziolka D, et al. Stereotactic Radiosurgery for Pituitary Adenomas: An Intermediate Review of its Safety, Efficacy, and Role in the Neurosurgical Treatment Armamentarium. J Neurosurg (2005) 102(4):678–91. doi: 10.3171/jns.2005.102.4.0678
87. Cordeiro D, Xu Z, Nasser M, Lopes B, Vance ML, Sheehan J. The Role of Crooke’s Changes in Recurrence and Remission After Gamma Knife Radiosurgery. J Neurooncol (2019) 142(1):171–81. doi: 10.1007/s11060-018-03078-8
88. Raverot G, Burman P, McCormack A, Heaney A, Petersenn S, Popovic V, et al. European Society of Endocrinology Clinical Practice Guidelines for the Management of Aggressive Pituitary Tumours and Carcinomas. Eur J Endocrinol (2018) 178(1):G1–G24. doi: 10.1530/EJE-17-0796
90. Valassi E, Franz H, Brue T, Feelders RA, Netea-Maier R, Tsagarakis S, et al. Preoperative Medical Treatment in Cushing’s Syndrome: Frequency of Use and its Impact on Postoperative Assessment: Data From ERCUSYN. Eur J Endocrinol (2018) 178(4):399–409. doi: 10.1530/EJE-17-0997
91. Pivonello R, De Leo M, Cozzolino A, Colao A. The Treatment of Cushing’s Disease. Endocr Rev (2015) 36(4):385–486. doi: 10.1210/er.2013-1048
92. Yoshida K, Fukuoka H, Odake Y, Nakajima S, Tachibana M, Ito J, et al. Multiple Salivary Cortisol Measurements are a Useful Tool to Optimize Metyrapone Treatment in Patients With Cushing’s Syndromes Treatment: Case Presentations. Front Endocrinol (Lausanne) (2017) 8:375. doi: 10.3389/fendo.2017.00375
93. Fukuoka H, Takeuchi T, Matsumoto R, Bando H, Suda K, Nishizawa H, et al. D-Dimer as a Significant Marker of Deep Vein Thrombosis in Patients With Subclinical or Overt Cushing’s Syndrome. Endocr J (2014) 61(10):1003–10. doi: 10.1507/endocrj.EJ14-0102
94. Stroud A, Dhaliwal P, Alvarado R, Winder MJ, Jonker BP, Grayson JW, et al. Outcomes of Pituitary Surgery for Cushing’s Disease: A Systematic Review and Meta-Analysis. Pituitary (2020) 23(5):595–609. doi: 10.1007/s11102-020-01066-8
95. Zhuang Z, Liu X, Bao X, Pan B, Deng K, Yao Y, et al. Invasive ACTH-secreting Pituitary Macroadenoma in Remission After Transsphenoidal Resection: A Case Report and Literature Review. Med (Baltimore) (2018) 97(46):e13148. doi: 10.1097/MD.0000000000013148
96. Barber TM, Adams E, Ansorge O, Byrne JV, Karavitaki N, Wass JA. Nelson’s Syndrome. Eur J Endocrinol (2010) 163(4):495–507. doi: 10.1530/EJE-10-0466
97. Tritos NA, Biller BM. Update on Radiation Therapy in Patients With Cushing’s Disease. Pituitary (2015) 18(2):263–8. doi: 10.1007/s11102-014-0615-4
98. Mehta GU, Ding D, Patibandla MR, Kano H, Sisterson N, Su YH, et al. Stereotactic Radiosurgery for Cushing Disease: Results of an International, Multicenter Study. J Clin Endocrinol Metab (2017) 102(11):4284–91. doi: 10.1210/jc.2017-01385
99. Jagannathan J, Sheehan JP, Pouratian N, Laws ER, Steiner L, Vance ML. Gamma Knife Surgery for Cushing’s Disease. J Neurosurg (2007) 106(6):980–7. doi: 10.3171/jns.2007.106.6.980
100. Sheehan JP, Xu Z, Salvetti DJ, Schmitt PJ, Vance ML. Results of Gamma Knife Surgery for Cushing’s Disease. J Neurosurg (2013) 119(6):1486–92. doi: 10.3171/2013.7.JNS13217
101. Kara M, Guduk M, Samanci Y, Yilmaz M, Sengoz M, Peker S. Gamma Knife Radiosurgery in Patients With Cushing’s Disease: Comparison of Aggressive Pituitary Corticotroph Tumor Versus Corticotroph Adenoma. Clin Neurol Neurosurg (2020) 197:106151. doi: 10.1016/j.clineuro.2020.106151
102. Gheorghiu ML, Fleseriu M. Stereotactic Radiation Therapy in Pituitary Adenomas, Is it Better Than Conventional Radiation Therapy? Acta Endocrinol (Buchar) (2017) 13(4):476–90. doi: 10.4183/aeb.2017.476
103. Lacroix A, Gu F, Gallardo W, Pivonello R, Yu Y, Witek P, et al. Efficacy and Safety of Once-Monthly Pasireotide in Cushing’s Disease: A 12 Month Clinical Trial. Lancet Diabetes Endocrinol (2018) 6(1):17–26. doi: 10.1016/S2213-8587(17)30326-1
104. Colao A, Petersenn S, Newell-Price J, Findling JW, Gu F, Maldonado M, et al. A 12-Month Phase 3 Study of Pasireotide in Cushing’s Disease. N Engl J Med (2012) 366(10):914–24. doi: 10.1056/NEJMoa1105743
105. Broersen LHA, Jha M, Biermasz NR, Pereira AM, Dekkers OM. Effectiveness of Medical Treatment for Cushing’s Syndrome: A Systematic Review and Meta-Analysis. Pituitary (2018) 21(6):631–41. doi: 10.1007/s11102-018-0897-z
106. Shimon I, Rot L, Inbar E. Pituitary-Directed Medical Therapy With Pasireotide for a Corticotroph Macroadenoma: Pituitary Volume Reduction and Literature Review. Pituitary (2012) 15(4):608–13. doi: 10.1007/s11102-012-0427-3
107. Cannavo S, Messina E, Albani A, Ferrau F, Barresi V, Priola S, et al. Clinical Management of Critically Ill Patients With Cushing’s Disease Due to ACTH-secreting Pituitary Macroadenomas: Effectiveness of Presurgical Treatment With Pasireotide. Endocrine (2016) 52(3):481–7. doi: 10.1007/s12020-015-0601-2
108. Greenman Y, Stern N. Paradoxical and Atypical Responses to Pasireotide in Aggressive ACTH-secreting Pituitary Tumors. Pituitary (2016) 19(6):605–11. doi: 10.1007/s11102-016-0755-9
109. Katznelson L. Sustained Improvements in Plasma ACTH and Clinical Status in a Patient With Nelson’s Syndrome Treated With Pasireotide LAR, a Multireceptor Somatostatin Analog. J Clin Endocrinol Metab (2013) 98(5):1803–7. doi: 10.1210/jc.2013-1497
110. Daniel E, Debono M, Caunt S, Girio-Fragkoulakis C, Walters SJ, Akker SA, et al. A Prospective Longitudinal Study of Pasireotide in Nelson’s Syndrome. Pituitary (2018) 21(3):247–55. doi: 10.1007/s11102-017-0853-3
111. Hanson PL, Aylwin SJ, Monson JP, Burrin JM. FSH Secretion Predominates In Vivo and In Vitro in Patients With non-Functioning Pituitary Adenomas. Eur J Endocrinol (2005) 152(3):363–70. doi: 10.1530/eje.1.01854
112. Ferriere A, Cortet C, Chanson P, Delemer B, Caron P, Chabre O, et al. Cabergoline for Cushing’s Disease: A Large Retrospective Multicenter Study. Eur J Endocrinol (2017) 176(3):305–14. doi: 10.1530/EJE-16-0662
113. Godbout A, Manavela M, Danilowicz K, Beauregard H, Bruno OD, Lacroix A. Cabergoline Monotherapy in the Long-Term Treatment of Cushing’s Disease. Eur J Endocrinol (2010) 163(5):709–16. doi: 10.1530/EJE-10-0382
114. Pivonello R, De Martino MC, Cappabianca P, De Leo M, Faggiano A, Lombardi G, et al. The Medical Treatment of Cushing’s Disease: Effectiveness of Chronic Treatment With the Dopamine Agonist Cabergoline in Patients Unsuccessfully Treated by Surgery. J Clin Endocrinol Metab (2009) 94(1):223–30. doi: 10.1210/jc.2008-1533
115. Casulari LA, Naves LA, Mello PA, Pereira Neto A, Papadia C. Nelson’s Syndrome: Complete Remission With Cabergoline But Not With Bromocriptine or Cyproheptadine Treatment. Horm Res (2004) 62(6):300–5. doi: 10.1159/000082235
116. Shraga-Slutzky I, Shimon I, Weinshtein R. Clinical and Biochemical Stabilization of Nelson’s Syndrome With Long-Term Low-Dose Cabergoline Treatment. Pituitary (2006) 9(2):151–4. doi: 10.1007/s11102-006-9290-4
117. Petrossians P, Ronci N, Valdes Socin H, Kalife A, Stevenaert A, Bloch B, et al. ACTH Silent Adenoma Shrinking Under Cabergoline. Eur J Endocrinol (2001) 144(1):51–7. doi: 10.1530/eje.0.1440051
118. Tateno T, Kato M, Tani Y, Oyama K, Yamada S, Hirata Y. Differential Expression of Somatostatin and Dopamine Receptor Subtype Genes in Adrenocorticotropin (ACTH)-Secreting Pituitary Tumors and Silent Corticotroph Adenomas. Endocr J (2009) 56(4):579–84. doi: 10.1507/endocrj.K08E-186
119. Hegi ME, Diserens AC, Gorlia T, Hamou MF, de Tribolet N, Weller M, et al. MGMT Gene Silencing and Benefit From Temozolomide in Glioblastoma. N Engl J Med (2005) 352(10):997–1003. doi: 10.1056/NEJMoa043331
120. Fadul CE, Kominsky AL, Meyer LP, Kingman LS, Kinlaw WB, Rhodes CH, et al. Long-Term Response of Pituitary Carcinoma to Temozolomide. Report of Two Cases. J Neurosurg (2006) 105(4):621–6. doi: 10.3171/jns.2006.105.4.621
121. Lasolle H, Cortet C, Castinetti F, Cloix L, Caron P, Delemer B, et al. Temozolomide Treatment can Improve Overall Survival in Aggressive Pituitary Tumors and Pituitary Carcinomas. Eur J Endocrinol (2017) 176(6):769–77. doi: 10.1530/EJE-16-0979
122. Elbelt U, Schlaffer SM, Buchfelder M, Knappe UJ, Vila G, Micko A, et al. Efficacy of Temozolomide Therapy in Patients With Aggressive Pituitary Adenomas and Carcinomas-A German Survey. J Clin Endocrinol Metab (2020) 105(3):dgz211. doi: 10.1210/clinem/dgz211
123. Tanaka S, Yamamoto M, Morita M, Takeno A, Kanazawa I, Yamaguchi T, et al. Successful Reduction of ACTH Secretion in a Case of Intractable Cushing’s Disease With Pituitary Crooke’s Cell Adenoma by Combined Modality Therapy Including Temozolomide. Endocr J (2019) 66(8):701–8. doi: 10.1507/endocrj.EJ18-0547
124. Scheithauer BW, Horvath E, Abel TW, Robital Y, Park SH, Osamura RY, et al. Pituitary Blastoma: A Unique Embryonal Tumor. Pituitary (2012) 15(3):365–73. doi: 10.1007/s11102-011-0328-x
125. Tatsi C, Stratakis CA. Aggressive Pituitary Tumors in the Young and Elderly. Rev Endocr Metab Disord (2020) 21(2):213–23. doi: 10.1007/s11154-019-09534-8
126. Salehi F, Scheithauer BW, Kovacs K, Horvath E, Syro LV, Sharma S, et al. O-6-methylguanine-DNA Methyltransferase (MGMT) Immunohistochemical Expression in Pituitary Corticotroph Adenomas. Neurosurgery (2012) 70(2):491–6; discussion 6. doi: 10.1227/NEU.0b013e318230ac63
127. Cohen-Inbar O, Xu Z, Lee CC, Wu CC, Chytka T, Silva D, et al. Prognostic Significance of Corticotroph Staining in Radiosurgery for non-Functioning Pituitary Adenomas: A Multicenter Study. J Neurooncol (2017) 135(1):67–74. doi: 10.1007/s11060-017-2520-y
128. Ceccato F, Lombardi G, Manara R, Emanuelli E, Denaro L, Milanese L, et al. Temozolomide and Pasireotide Treatment for Aggressive Pituitary Adenoma: Expertise at a Tertiary Care Center. J Neurooncol (2015) 122(1):189–96. doi: 10.1007/s11060-014-1702-0
129. Fountas A, Lavrentaki A, Subramanian A, Toulis KA, Nirantharakumar K, Karavitaki N. Recurrence in Silent Corticotroph Adenomas After Primary Treatment: A Systematic Review and Meta-Analysis. J Clin Endocrinol Metab (2018) 104:1039–48. doi: 10.1210/jc.2018-01956
130. Whitelaw BC. How and When to Use Temozolomide to Treat Aggressive Pituitary Tumours. Endocr Relat Cancer (2019) 26(9):R545–R52. doi: 10.1530/ERC-19-0083
131. Trouillas J, Roy P, Sturm N, Dantony E, Cortet-Rudelli C, Viennet G, et al. A New Prognostic Clinicopathological Classification of Pituitary Adenomas: A Multicentric Case-Control Study of 410 Patients With 8 Years Post-Operative Follow-Up. Acta Neuropathol (2013) 126(1):123–35. doi: 10.1007/s00401-013-1084-y
132. Syro LV, Rotondo F, Camargo M, Ortiz LD, Serna CA, Kovacs K. Temozolomide and Pituitary Tumors: Current Understanding, Unresolved Issues, and Future Directions. Front Endocrinol (Lausanne) (2018) 9:318. doi: 10.3389/fendo.2018.00318
133. Hirohata T, Asano K, Ogawa Y, Takano S, Amano K, Isozaki O, et al. DNA Mismatch Repair Protein (MSH6) Correlated With the Responses of Atypical Pituitary Adenomas and Pituitary Carcinomas to Temozolomide: The National Cooperative Study by the Japan Society for Hypothalamic and Pituitary Tumors. J Clin Endocrinol Metab (2013) 98(3):1130–6. doi: 10.1210/jc.2012-2924
134. Zacharia BE, Gulati AP, Bruce JN, Carminucci AS, Wardlaw SL, Siegelin M, et al. High Response Rates and Prolonged Survival in Patients With Corticotroph Pituitary Tumors and Refractory Cushing Disease From Capecitabine and Temozolomide (CAPTEM): A Case Series. Neurosurgery (2014) 74(4):E447–55; discussion E55. doi: 10.1227/NEU.0000000000000251
135. Heaney AP. Clinical Review: Pituitary Carcinoma: Difficult Diagnosis and Treatment. J Clin Endocrinol Metab (2011) 96(12):3649–60. doi: 10.1210/jc.2011-2031
136. Lin AL, Donoghue MTA, Wardlaw SL, Yang TJ, Bodei L, Tabar V, et al. Approach to the Treatment of a Patient With an Aggressive Pituitary Tumor. J Clin Endocrinol Metab (2020) 105(12):3807–20. doi: 10.1210/clinem/dgaa649
137. Lin AL, Jonsson P, Tabar V, Yang TJ, Cuaron J, Beal K, et al. Marked Response of a Hypermutated Acth-Secreting Pituitary Carcinoma to Ipilimumab and Nivolumab. J Clin Endocrinol Metab (2018) 103(10):3925–30. doi: 10.1210/jc.2018-01347
138. Sol B, de Filette JMK, Awada G, Raeymaeckers S, Aspeslagh S, Andreescu CE, et al. Immune Checkpoint Inhibitor Therapy for ACTH-secreting Pituitary Carcinoma: A New Emerging Treatment? Eur J Endocrinol (2021) 184(1):K1–5. doi: 10.1530/EJE-20-0151
139. Bedard PL, Hyman DM, Davids MS, Siu LL. Small Molecules, Big Impact: 20 Years of Targeted Therapy in Oncology. Lancet (2020) 395(10229):1078–88. doi: 10.1016/S0140-6736(20)30164-1
140. Cohen M, Persky R, Stegemann R, Hernandez-Ramirez LC, Zeltser D, Lodish MB, et al. Germline USP8 Mutation Associated With Pediatric Cushing Disease and Other Clinical Features: A New Syndrome. J Clin Endocrinol Metab (2019) 104(10):4676–82. doi: 10.1210/jc.2019-00697
141. Liu X, Feng M, Dai C, Bao X, Deng K, Yao Y, et al. Expression of EGFR in Pituitary Corticotroph Adenomas and Its Relationship With Tumor Behavior. Front Endocrinol (Lausanne) (2019) 10:785. doi: 10.3389/fendo.2019.00785
142. McCormack A, Dekkers OM, Petersenn S, Popovic V, Trouillas J, Raverot G, et al. Treatment of Aggressive Pituitary Tumours and Carcinomas: Results of a European Society of Endocrinology (ESE) Survey 2016. Eur J Endocrinol (2018) 178(3):265–76. doi: 10.1530/EJE-17-0933
143. Cooper O, Bonert VS, Rudnick J, Pressman BD, Lo J, Salvatori R, et al. Egfr/ErbB2 Targeting Lapatinib Therapy for Aggressive Prolactinomas. J Clin Endocrinol Metab (2020) 106:e917–25. doi: 10.1210/clinem/dgaa805
144. Ortiz LD, Syro LV, Scheithauer BW, Ersen A, Uribe H, Fadul CE, et al. Anti-VEGF Therapy in Pituitary Carcinoma. Pituitary (2012) 15(3):445–9. doi: 10.1007/s11102-011-0346-8
145. Rotman LE, Vaughan TB, Hackney JR, Riley KO. Long-Term Survival After Transformation of an Adrenocorticotropic Hormone-Secreting Pituitary Macroadenoma to a Silent Corticotroph Pituitary Carcinoma. World Neurosurg (2019) 122:417–23. doi: 10.1016/j.wneu.2018.11.011
146. Lamb LS, Sim HW, McCormack AI. Exploring the Role of Novel Medical Therapies for Aggressive Pituitary Tumors: A Review of the Literature- “Are We There Yet?” Cancers (Basel) (2020) 12(2):e917–25. doi: 10.3390/cancers12020308
147. Yang J, Yan J, Liu B. Targeting VEGF/VEGFR to Modulate Antitumor Immunity. Front Immunol (2018) 9:978. doi: 10.3389/fimmu.2018.00978
148. Monsalves E, Juraschka K, Tateno T, Agnihotri S, Asa SL, Ezzat S, et al. The PI3K/AKT/mTOR Pathway in the Pathophysiology and Treatment of Pituitary Adenomas. Endocr Relat Cancer (2014) 21(4):R331–44. doi: 10.1530/ERC-14-0188
149. Jia W, Sanders AJ, Jia G, Liu X, Lu R, Jiang WG. Expression of the mTOR Pathway Regulators in Human Pituitary Adenomas Indicates the Clinical Course. Anticancer Res (2013) 33(8):3123–31.
150. Yao JC, Fazio N, Singh S, Buzzoni R, Carnaghi C, Wolin E, et al. Everolimus for the Treatment of Advanced, non-Functional Neuroendocrine Tumours of the Lung or Gastrointestinal Tract (RADIANT-4): A Randomised, Placebo-Controlled, Phase 3 Study. Lancet (2016) 387(10022):968–77. doi: 10.1016/S0140-6736(15)00817-X
151. Donovan LE, Arnal AV, Wang SH, Odia Y. Widely Metastatic Atypical Pituitary Adenoma With mTOR Pathway STK11(F298L) Mutation Treated With Everolimus Therapy. CNS Oncol (2016) 5(4):203–9. doi: 10.2217/cns-2016-0011
152. Jouanneau E, Wierinckx A, Ducray F, Favrel V, Borson-Chazot F, Honnorat J, et al. New Targeted Therapies in Pituitary Carcinoma Resistant to Temozolomide. Pituitary (2012) 15(1):37–43. doi: 10.1007/s11102-011-0341-0
153. Liu NA, Jiang H, Ben-Shlomo A, Wawrowsky K, Fan XM, Lin S, et al. Targeting Zebrafish and Murine Pituitary Corticotroph Tumors With a Cyclin-Dependent Kinase (CDK) Inhibitor. Proc Natl Acad Sci USA (2011) 108(20):8414–9. doi: 10.1073/pnas.1018091108
154. Liu NA, Araki T, Cuevas-Ramos D, Hong J, Ben-Shlomo A, Tone Y, et al. Cyclin E-Mediated Human Proopiomelanocortin Regulation as a Therapeutic Target for Cushing Disease. J Clin Endocrinol Metab (2015) 100(7):2557–64. doi: 10.1210/jc.2015-1606
155. Daniel E, Newell-Price JD. Therapy of Endocrine Disease: Steroidogenesis Enzyme Inhibitors in Cushing’s Syndrome. Eur J Endocrinol (2015) 172(6):R263–80. doi: 10.1530/EJE-14-1014
156. Pivonello R, Ferrigno R, De Martino MC, Simeoli C, Di Paola N, Pivonello C, et al. Medical Treatment of Cushing’s Disease: An Overview of the Current and Recent Clinical Trials. Front Endocrinol (Lausanne) (2020) 11:648. doi: 10.3389/fendo.2020.00648
157. Guelho D, Grossman AB. Emerging Drugs for Cushing’s Disease. Expert Opin Emerg Drugs (2015) 20(3):463–78. doi: 10.1517/14728214.2015.1047762
158. Fleseriu M, Pivonello R, Elenkova A, Salvatori R, Auchus RJ, Feelders RA, et al. Efficacy and Safety of Levoketoconazole in the Treatment of Endogenous Cushing’s Syndrome (SONICS): A Phase 3, Multicentre, Open-Label, Single-Arm Trial. Lancet Diabetes Endocrinol (2019) 7(11):855–65. doi: 10.1016/S2213-8587(19)30313-4
159. Fleseriu M, Pivonello R, Young J, Hamrahian AH, Molitch ME, Shimizu C, et al. Osilodrostat, a Potent Oral 11beta-Hydroxylase Inhibitor: 22-Week, Prospective, Phase II Study in Cushing’s Disease. Pituitary (2016) 19(2):138–48. doi: 10.1007/s11102-015-0692-z
160. Cuevas-Ramos D, Lim DST, Fleseriu M. Update on Medical Treatment for Cushing’s Disease. Clin Diabetes Endocrinol (2016) 2:16. doi: 10.1186/s40842-016-0033-9
161. Castinetti F, Fassnacht M, Johanssen S, Terzolo M, Bouchard P, Chanson P, et al. Merits and Pitfalls of Mifepristone in Cushing’s Syndrome. Eur J Endocrinol (2009) 160(6):1003–10. doi: 10.1530/EJE-09-0098
Keywords: Cushing’s disease (CD), aggressiveness/physiology, pathology, medical treatment/surgical treatment, targeted therapy
Citation: Yamamoto M, Nakao T, Ogawa W and Fukuoka H (2021) Aggressive Cushing’s Disease: Molecular Pathology and Its Therapeutic Approach. Front. Endocrinol. 12:650791. doi: 10.3389/fendo.2021.650791
Received: 08 January 2021; Accepted: 26 May 2021;
Published: 16 June 2021.
Edited by:
Adam Mamelak, Cedars Sinai Medical Center, United StatesReviewed by:
Ning-Ai Liu, Cedars Sinai Medical Center, United StatesMônica Gadelha, Federal University of Rio de Janeiro, Brazil
Copyright © 2021 Yamamoto, Nakao, Ogawa and Fukuoka. This is an open-access article distributed under the terms of the Creative Commons Attribution License (CC BY). The use, distribution or reproduction in other forums is permitted, provided the original author(s) and the copyright owner(s) are credited and that the original publication in this journal is cited, in accordance with accepted academic practice. No use, distribution or reproduction is permitted which does not comply with these terms.
*Correspondence: Hidenori Fukuoka, fukuokah@med.kobe-u.ac.jp