- 1Université de Paris, UMR-S 1266 INSERM, Institute of Psychiatry and Neuroscience of Paris, Paris, France
- 2Laboratory of Neurophysiology of the Multidisciplinary Institute of Cell Biology [IMBICE, Argentine Research Council (CONICET) and Scientific Research Commission, Province of Buenos Aires (CIC-PBA). National University of La Plata], La Plata, Buenos Aires, Argentina
- 3Department of Internal Medicine, Section of Endocrinology and Metabolism, Leiden University Medical Center, Leiden, Netherlands
- 4Institut de génétique et de biologie moléculaire et cellulaire (IGBMC), UMR7104 CNRS/U1258 INSERM, Université de Strasbourg, Illkirch, France
- 5UMR CNRS/MNHN 7179, Mécanismes Adaptatifs et Evolution, Brunoy, France
- 6Université de Lille, Faculté des Sciences et Technologies, Villeneuve d’Ascq, France
- 7Marrow Adiposity and Bone Lab - MABLab ULR 4490, Univ. Littoral Côte d’Opale, Boulogne-sur-Mer, Univ. Lille and CHU Lille, Lille, France
Using preproghrelin-deficient mice (Ghrl-/-), we previously observed that preproghrelin modulates pulsatile growth hormone (GH) secretion in post-pubertal male mice. However, the role of ghrelin and its derived peptides in the regulation of growth parameters or feeding in females is unknown. We measured pulsatile GH secretion, growth, metabolic parameters and feeding behavior in adult Ghrl-/- and Ghrl+/+ male and female mice. We also assessed GH release from pituitary explants and hypothalamic growth hormone-releasing hormone (GHRH) expression and immunoreactivity. Body weight and body fat mass, linear growth, spontaneous food intake and food intake following a 48-h fast, GH pituitary contents and GH release from pituitary explants ex vivo, fasting glucose and glucose tolerance were not different among adult Ghrl-/- and Ghrl+/+ male or female mice. In vivo, pulsatile GH secretion was decreased, while approximate entropy, that quantified orderliness of secretion, was increased in adult Ghrl-/- females only, defining more irregular GH pattern. The number of neurons immunoreactive for GHRH visualized in the hypothalamic arcuate nucleus was increased in adult Ghrl-/- females, as compared to Ghrl+/+ females, whereas the expression of GHRH was not different amongst groups. Thus, these results point to sex-specific effects of preproghrelin gene deletion on pulsatile GH secretion, but not feeding, growth or metabolic parameters, in adult mice.
Introduction
The ghrelin gene (GHRL) encodes proghrelin prohormone, which in turn gives rise to acylated and non-acylated ghrelin as well as obestatin, which are mainly secreted from cells of the stomach (1). The most studied proghrelin-derived product is the 28-residue acylated peptide ghrelin that acts via the growth hormone secretagogue receptor (GHSR). Ghrelin administration in humans and rodents induces a plethora of effects (2, 3) including stimulation of GH secretion (2, 4). In rodents, this effect is principally mediated through GHRH neurons of the hypothalamic arcuate nucleus (ArcN) (5). Also, ghrelin treatment rapidly increases food intake (6), glycaemia (7–9), glucocorticoid secretion (10) and affects cognitive behaviors (11). Strikingly, the notion that endogenous ghrelin plays a leading physiological role has been challenged by studies showing that genetically-modified mice lacking ghrelin display minor alterations (12). In particular, adult Ghrl-/- mice fed on a regular diet show food intake, body weight, body size and body composition indistinguishable from those observed in wild-type littermates (13–17). Also, mice lacking the enzyme ghrelin-O-acyl-transferase (GOAT), which n-acetylates ghrelin, and mice with ablation of proghrelin-expressing cells show no food intake, body growth nor body weight alterations (18–22). Despite that ghrelin system is believed to play a major role under energy deficit conditions, such as fasting, when plasma ghrelin levels increase, fasted Ghrl-/- mice show no alterations in plasma parameters (e.g., glucose, insulin, leptin) nor in compensatory hyperphagia when refed (23–25). Still, young Ghrl-/- male mice show reduced amplitude of GH secretory pulses (26). Moreover, some studies found that Ghrl-/- mice fed on a high-fat diet (HFD) show lower body weight and fat mass (24, 27, 28). Interestingly, GHSR-deficient (Ghsr-/-) mice usually show more robust alterations, as compared to Ghrl-/- mice, which has been attributed to the fact that the latter retain ligand-independent effects of GHSR and/or signaling of other proghrelin-derived peptides (25, 29).
The vast majority of the research investigating Ghrl-/- mice has been conducted in males. Specifically, some of the above referred studies did not include females at all (24, 25, 30). Other studies merely assessed body weight or food intake whereas the assessment of plasma hormone levels was exclusively performed in males (17, 23). Notably, several studies suggest a modulatory role of sex on ghrelin actions (31–34), but sex differences in Ghrl-/- mice, and specifically on GH secretion, have not been investigated thoroughly. In GHSR-deficient mice, only females fed on standard chow diet had lower body weight and adiposity, as compared to wild-type littermates (35). Furthermore, Ghsr-/- female but not male mice fed on a HFD exhibited reduced taste responsiveness to linoleic acid compared to wild-type littermates (36). Finally, adult Ghsr-/- female but not male mice had reduced pulsatile GH secretion (37). We thus questioned a possible role of ghrelin gene in regulating GH secretion and its physiological consequences in female mice during adulthood, when the changes in pulsatile GH secretion and pattern associated with the rapid linear growth and pubertal maturation has ended (38, 39). Specifically, we investigated if ghrelin gene deletion induces sex-specific dimorphic effects on GH secretion, feeding behavior or body and metabolic parameters in adult 20-40-week-old mice.
Materials and Methods
Animals
Ghrl-/- mice were originally obtained from Dr Tomasetto (IGBMC, France) and backcrossed on a C57BL/6J genetic background (26). Heterozygous mice were raised at the Institute of Psychiatry and Neuroscience of Paris (INSERM UMR-S 1266) and bred to obtain Ghrl-/- mice and wild-type littermates (Ghrl+/+ mice). Offspring were genotyped as previously described (26) and housed in a room under controlled illumination (7:00 to 19:00) and temperature (22–24°C). Mice had free access to chow diet (3% fat, 16% protein, 60% carbohydrate, 4% fibers, 2.79 kcal/g, Safe A04, France) and water, except when indicated. Experiments were conducted in 20-40-week-old male and random cycling female mice.
Assessment of Body Weight, Body Length and Body Composition
A cohort of anesthetized mice (6 Ghrl+/+ and 7 Ghrl-/- males; 8 Ghrl+/+ and 7 Ghrl-/- females) was used to assess naso-anal distance. Another cohort of mice (4-7 Ghrl+/+ and 4-6 Ghrl-/- males; 4-9 Ghrl+/+ and 4-6 Ghrl-/- females) was dissected to weigh pituitary gland, liver, heart, spleen, pancreas, testis/ovaries, kidneys, adrenals and fat depots (mesenteric, inguinal, perirenal, perigonadal, interscapular brown adipose tissue).
Repeated Blood Sampling for GH Assay
Ultradian GH secretion was assessed as previously described (26, 40). Briefly, mice (5 Ghrl+/+ and 8 Ghrl-/- males; 8 Ghrl+/+ and 6 Ghrl-/- females) were acclimated to handling and blood sampling collection to minimize stress. Then, sequential tail-tip whole venous blood samples (2 μl/sample) were collected every 10-min over a 6-h-sampling period (9:00 to 15:00) (See Supplementary File for details).
Assessment of Pulsatile GH Secretion
GH concentration time series were analyzed using an automated deconvolution method following established parameters (41). Measures include the number of pulses (over the 6-h sampling period) and mean pulse mass (i.e., mean of the summed pulses) as well as basal (i.e., non-pulsatile), pulsatile (i.e., sum of individual GH pulses) and total GH secretion (i.e., sum of basal plus pulsatile). The orderliness of GH secretion was calculated by Jack-Knife Approximate Entropy (JkApEn) as described earlier (42, 43) (See Supplementary File for details).
GH Pituitary Content and GH Release From Pituitary Explants
Two different cohorts of mice were used to assess total GH pituitary contents (12 Ghrl+/+ and 7 Ghrl-/- males; 5 Ghrl+/+ and 5 Ghrl-/- females) and basal and GHRH-stimulated GH release from perifused pituitary explants (4 Ghrl+/+ and 4 Ghrl-/- males; 4 Ghrl+/+ and 4 Ghrl-/- females) as previously described (26) (See Supplementary File for details). Samples were frozen at -20°C until GH determination.
GH Enzyme Immuno-Assay
GH concentrations in whole blood, pituitary contents and media were determined using an in-house mouse GH ELISA (40). (See Supplementary File for details). The assay sensitivity was 0.038 ng/mL, and intra- and inter-assay coefficients of variations were 3.2% and less than 8.75%, respectively.
Assessment of Spontaneous Food Intake
Mice (8 Ghrl+/+ and 6 Ghrl-/- males; 7 Ghrl+/+ and 8 Ghrl-/- females) were individually housed in automated feeding stations equipped with high precisions sensors (LabMaster System, TSE Systems, Germany) to record spontaneous food intake and meal patterns as previously reported (44) (See Supplementary File for details).
Fasting-Refeeding Protocol
As described before (25), individually housed mice (5 Ghrl+/+ and 5 Ghrl-/- males; 7 Ghrl+/+ and 6 Ghrl-/- females) were fasted at 10:00 and refed 48-h later. Body weight and food intake was daily monitored at 10:00 for four days after refeeding. Food intake was calculated by subtracting the weight of the remaining food at 10:00 to the weight of the initial food. Importantly, mice fully tolerated a 48-h fasting period (25, 45).
Assessment of Blood Glucose and Glucose Tolerance Test
Blood glucose was measured with a glucometer (GlucoFix Premium, Menarini Diagnostics) at 10:00 and at 18:00 in fed conditions as well as at 10:00 following a 24-h fast (8 Ghrl+/+ and 6 Ghrl-/- males; 8 Ghrl+/+ and 8 Ghrl-/- females). After fasting, glucose was measured before and 15-, 30-, 60- and 120-min after glucose intraperitoneal injection (2 g/kg body weight).
GHRH Immunostaining and Quantification
Brains of perfused mice (3 Ghrl+/+ and 5 Ghrl-/- males; 5 Ghrl+/+ and 8 Ghrl-/- females) were post-fixed, frozen and coronally cut at 40 µm into four equal series. Chromogenic immunohistochemistry against GHRH was performed as described before (46), using a previously validated rabbit anti-GHRH antibody (47) (immunoserum L0851, 1:10000) for 48-h at 4°C. Then, sections were sequentially incubated with a biotinylated anti-rabbit antibody and the avidin-biotin-peroxidase complex. A visible black/purple signal was developed with a diaminobenzidine/nickel solution (See Supplementary File for details). Quantifications were performed in the ArcN between bregma -1.58 and -2.06 mm, using the anatomical limits, according to the Paxinos mouse brain atlas (48). Total GHRH-immunoreactive cells were quantified, and data were expressed as positive cells (GHRH+) per section. Blind quantitative analysis was performed independently by two observers.
Real-Time Quantitative PCR Measurement
Hypothalami were quickly dissected, frozen in liquid nitrogen and stored at -80°C. The mRNAs were extracted with Trizol reagent and cDNAs were obtained from the reverse transcription of total RNA (ThermoFisher, Waltham, MA, USA). The mRNA levels of Ghrh and Ghsr were quantified relative to the housekeeping genes Ppia and Gapdh. Relative quantification (RQ) was calculated relative to the Ghrl+/+ males (See Supplementary File for details).
Statistical Analysis
Data are expressed as mean ± SEM, and statistical analyses were performed using Statview software (SAS institute) or GraphPad Prism (GraphPad Software). Differences across sex and genotype were identified by 2-way ANOVA, followed by multiple comparisons, using Bonferroni post-hoc analysis. Differences were considered significant when p<0.05.
Results
Body Weight, Organ Weights, Body Fat Partitioning, Metabolic Parameters, Locomotor Activity, Spontaneous Food Intake and Fasting-Induced Food Intake Are Unaltered in Adult Ghrl-/- Mice
As shown in Table 1, body weight, naso-anal distance and organ weights were not different in adult Ghrl-/- as compared to Ghrl+/+ males and females, respectively. Moreover, fed morning, fed evening and 24-h fasted blood glucose levels as well as glucose tolerance were not different in adult Ghrl-/- as compared to Ghrl+/+ males and females. Spontaneous diurnal and nocturnal food intake, fasting-induced food intake (Figure 1) and home cage ambulatory activity (not shown) were not different in adult Ghrl-/- as compared to Ghrl+/+ males and females. In addition, meal number, mean meal size and mean meal duration in ad libitum fed mice were not different amongst genotypes (not shown). Finally, parameters of bones architecture measured by micro-CT were not different in adult Ghrl-/- as compared to Ghrl+/+ males and females (not shown), although a tendency toward reduction of bone volume fraction was observed (volume of mineral bone per unit volume of the sample, BV/TV; ANOVA, genotype effect: p=0.0894, sex effect: p<0.0001, sex x genotype effect: p=0.9014) in 80-week-old mice only.
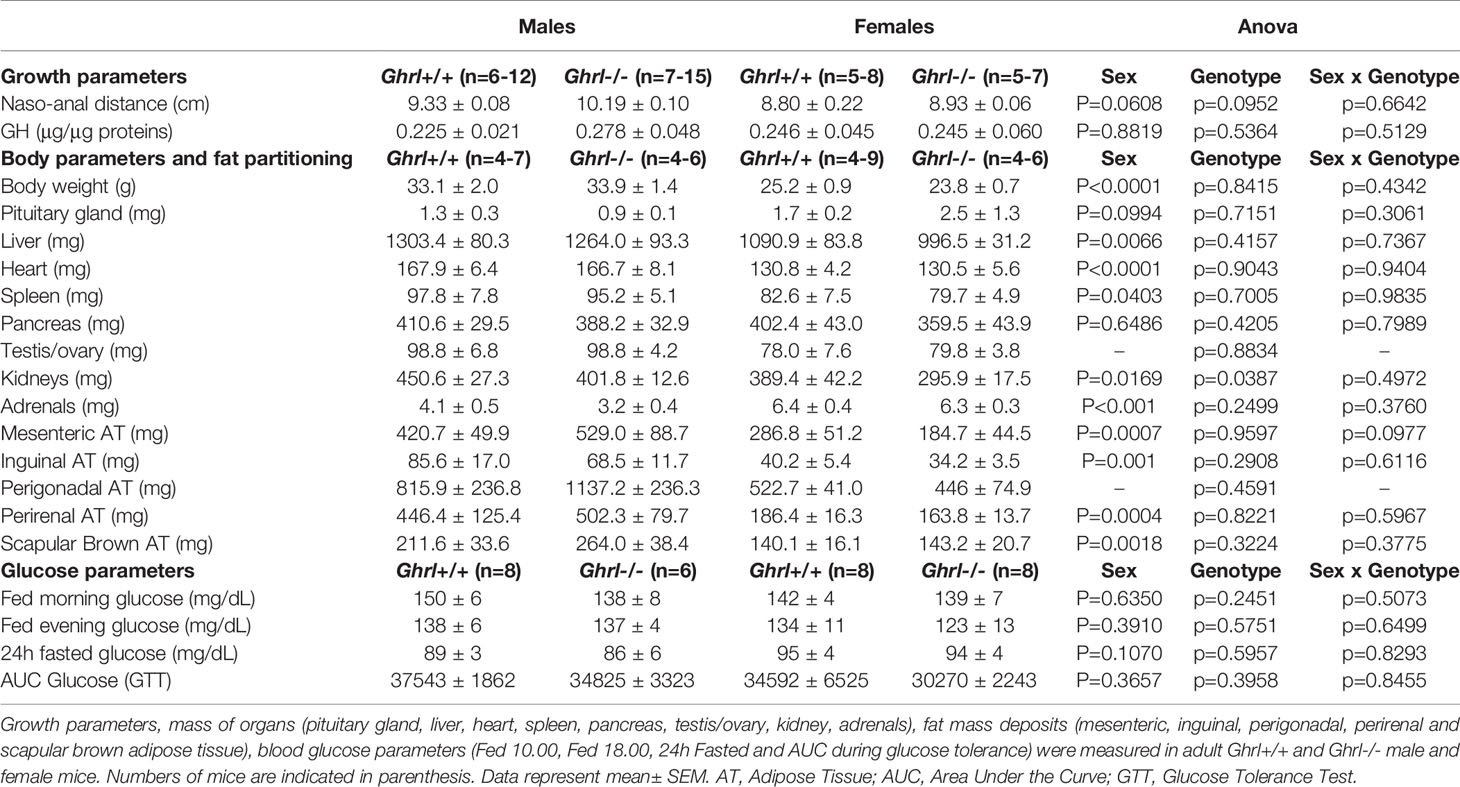
Table 1 Growth and body parameters, body fat partitioning and metabolic parameters in male and female Ghrl-/- mice.
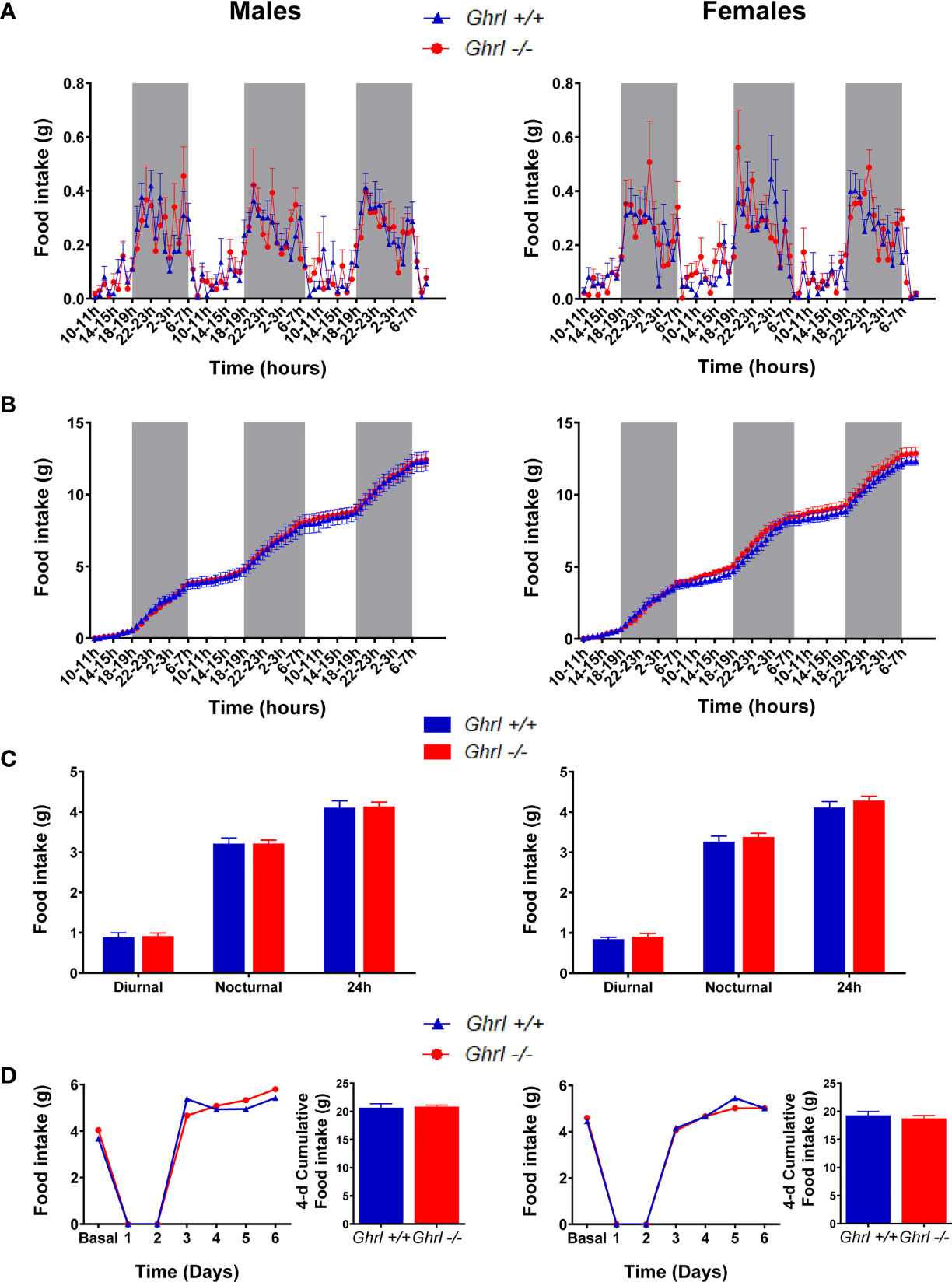
Figure 1 Spontaneous food intake and food intake in a fast-refeeding protocol in male and female Ghrl-/- mice. Feeding parameters in adult male and female Ghrl+/+ and Ghrl-/- mice showing spontaneous food intake (A) and cumulative food intake (B) measured every hour over 3 days as well as diurnal, nocturnal and 24-h food intake averaged over 3 days (C). Daily food intake and 4-days cumulative food intake during re-feeding (D) in mice fasted for 48-h and then allowed free access to food. Light and dark phases are denoted by white and grey rectangles on the x-axis. Data represent mean ± SEM. Number of mice in panels (A–C) males (n=8 Ghrl+/+ males and 7 Ghrl-/-), females (n=8 Ghrl+/+ and 8 Ghrl-/-). Number of mice in panel D: males (n=5 Ghrl+/+ males and 5 Ghrl-/-), females (n=7 Ghrl+/+ and 6 Ghrl-/-).
The Ultradian Pattern of GH Secretion Is Altered in Adult Ghrl-/- Females, but Not in Ghrl-/- Males
GH pituitary contents were not different in adult Ghrl-/-, as compared to Ghrl+/+ males and females (Table 1). As reported before (37), we observed a sexually dimorphic GH secretion pattern. Indeed, mean pulse mass was higher in males than in females (p<0.05 in both Ghrl+/+ and Ghrl-/- mice, post-hoc test), whereas basal GH secretion and JkApEn values were lower in males than in females (p<0.05 in both Ghrl+/+ and Ghrl-/- mice for basal GH, p<0.01 in Ghrl+/+ mice and p<0.0001 in Ghrl-/- mice for JkApEn, post-hoc test) (Figure 2). An increase in the number of GH pulses in females as compared to males was observed in Ghrl-/- mice only (p<0.01, post-hoc test). Furthermore, we observed that the regularity of GH secretion was decreased in Ghrl-/- female mice when compared to Ghrl+/+ female mice (Figure 2). More precisely, pulsatile GH secretion and mean pulse mass were reduced by 43% and 56% respectively in Ghrl-/- females as compared to Ghrl+/+ females (p<0.05 and p<0.01 for pulsatile GH and mean pulse mass, respectively, post-hoc test) while total and basal GH secretion were not significantly affected by ghrelin deletion. The maximal pulse amplitude was also reduced by 50% in Ghrl-/- compared to Ghrl+/+ females (26 ± 4 versus 13 ± 3 ng/mL, p<0.05, post-hoc test). In contrast, JkApEn values increased by 27% in Ghrl-/- females as compared to Ghrl+/+ females (p<0.01, post-hoc test). In males, we did not find genotype differences for any of the GH secretion parameters, however, the inter-individual variability of pulsatile GH, maximal pulse amplitude and mean pulse mass was higher than in females (see representative GH profiles).
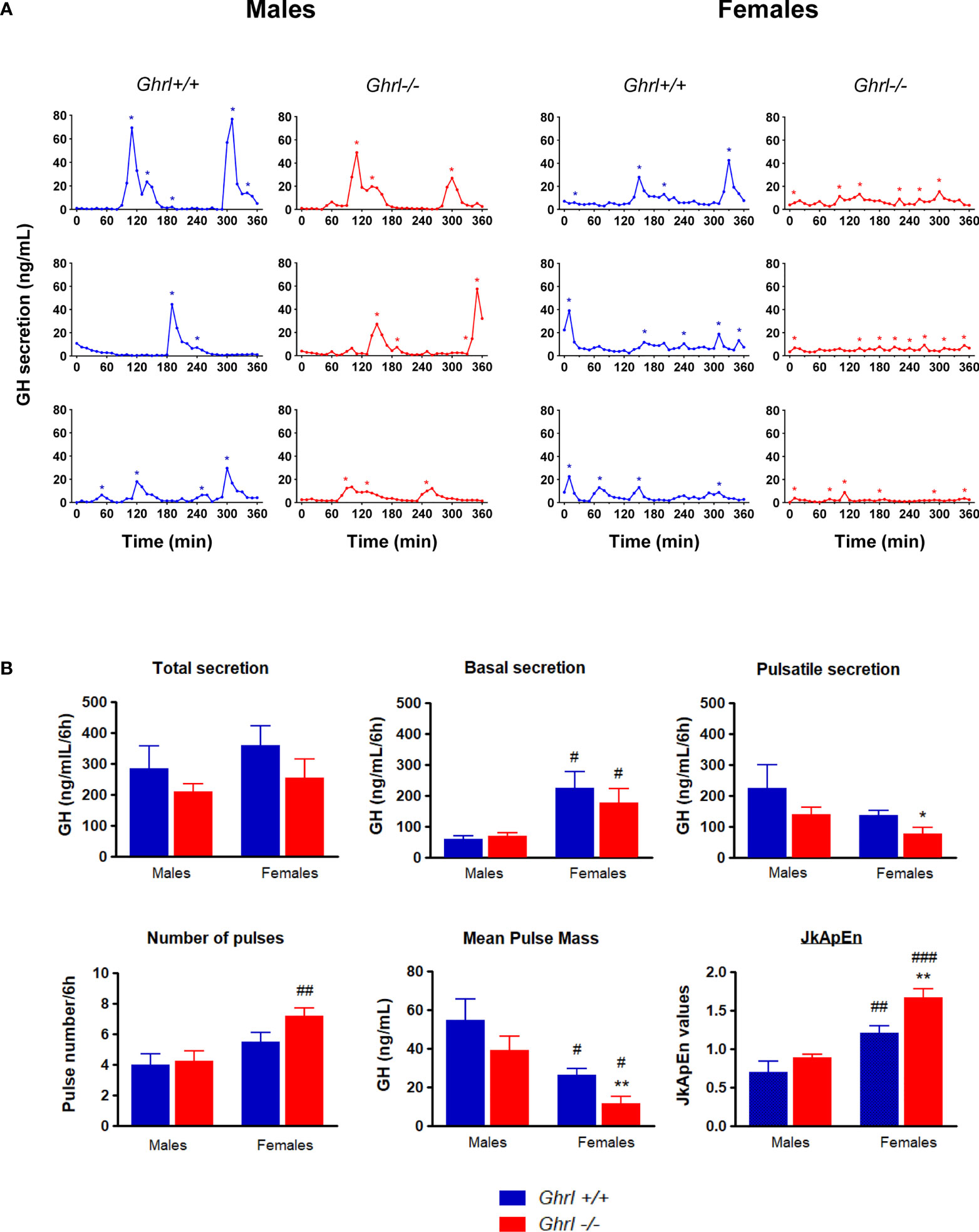
Figure 2 Analyses of ultradian GH secretion in male and female Ghrl-/- mice. Representative individual plasma GH secretory profiles in 36-week-old male and female Ghrl+/+ and Ghrl-/- mice (A). Deconvolution analyses and regularity parameters in adult Ghrl+/+ and Ghrl-/- male and female mice showing total GH secretion, basal GH secretion, pulsatile GH secretion, number of pulses, mean pulse mass and JkApEn values (B). Asterisks indicate the location of secretory peaks. Data represent mean ± SEM. Number of mice: males (n=5 Ghrl+/+ males and 8 Ghrl-/-), females (n=8 Ghrl+/+ and 6 Ghrl-/-). *p < 0.05, **p < 0.01 Ghrl-/- versus Ghrl+/+ mice of the same sex. #p < 0.05, ##p < 0.01, ###p < 0.0001 females versus males of the same genotype.
In Vitro Release of GH Is Unaltered in Pituitary Explants From Adult Male and Female Ghrl-/- and Ghrl+/+ Mice
To test if the alteration of the ultradian GH pattern in adult Ghrl-/- females was due to modifications at the pituitary level, we assessed basal and stimulated GH secretion from pituitary gland explants (Figure 3A). As reported earlier (26), basal and GHRH-induced GH release were significantly higher in males than in females (ANOVA, sex effect: p<0.0001). However, basal GH release or GH release stimulated by GHRH or KCl was not different in pituitary explants from Ghrl+/+ and Ghrl-/- mice in either sex.
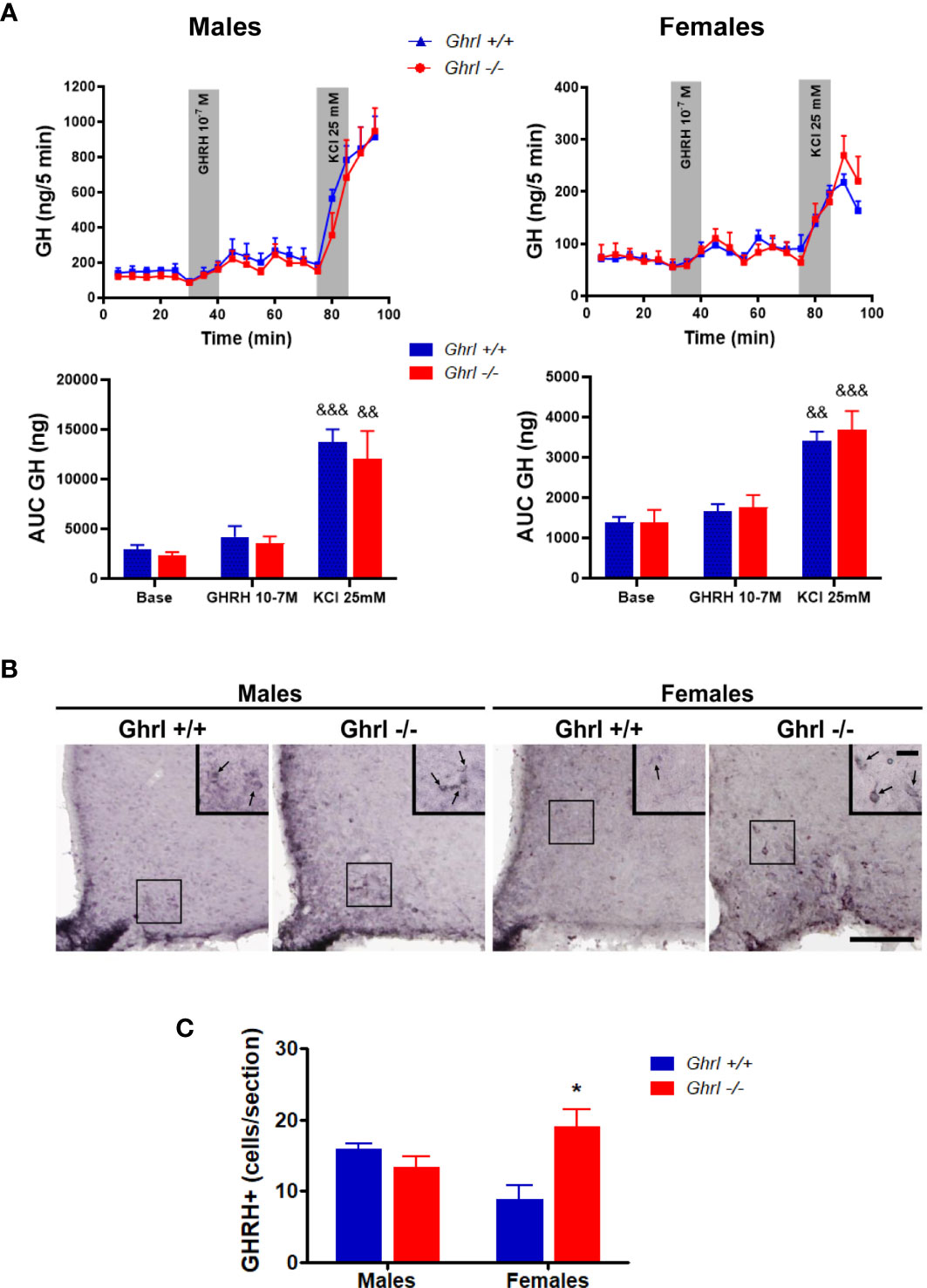
Figure 3 GH release from pituitary explants and GHRH immunoreactivity in the hypothalamus of male and female Ghrl-/- mice. Basal GH release, 10-7 M GHRH-induced GH release and 25 mM KCl induced GH release measured in adult male and female Ghrl+/+ and Ghrl-/- mice. Note that the Y-axes scales are different for males and females (A). Photomicrographs of GHRH immunoreactivity staining (B) and GHRH positive cells (C) in the hypothalamus of 36-week-old male and female Ghrl+/+ and Ghrl-/- mice. Data represent mean ± SEM. Number of mice in panel (A) males (n=4 Ghrl+/+ males and 4 Ghrl-/-), females (n=4 Ghrl+/+ and 4 Ghrl-/-). Number of mice in panel (C) males (n=3 Ghrl+/+ males and 5 Ghrl-/-), females (n=5 Ghrl+/+ and 8 Ghrl-/-) *p < 0.05 Ghrl-/- versus Ghrl+/+ mice of the same sex, &&p < 0.01, &&&p <0 .0001 KCl versus base and GHRH.
The Number of GHRH+ Neurons Increased in ArcN of Adult Female, but Not Male, Ghrl-/- Mice
The hypophysiotropic GHRH neurons of the ArcN control the pulsatile pattern of GH secretion (39) and are sexually dimorphic (47). Thus, we estimated the sex and genotype effects on GHRH immunoreactive signal in the ArcN. Our analysis measuring the visualized GHRH+ cells in the ArcN revealed a significant interaction between sex and genotype (ANOVA, sex effect: p=0.7901, genotype effect: p=0.1185, sex x genotype interaction: p=0.0145) (Figures 3B, C). The number of visualized GHRH+ neurons increased in Ghrl-/- females, as compared to Ghrl+/+ females (p<0.05, post-hoc test), whereas the number of GHRH+ neurons of Ghrl-/- and Ghrl+/+ males was not different. In the hypothalamus, no genotype differences were found in the mRNA levels of Ghrh (1.00 ± 0.14 in Ghrl+/+ males, 1.18 ± 0.14 in Ghrl-/- males, 0.74 ± 0.10 in Ghrl+/+ females, 0.82 ± 0.19 in Ghrl-/- females) (ANOVA, sex effect: p=0.0599, genotype effect: p=0.3961, sex x genotype interaction: p=0.7589), or Ghsr (1.00 ± 0.08 in Ghrl+/+ males, 0.92 ± 0.10 in Ghrl-/- males, 0.83 ± 0.07 in Ghrl+/+ females, 0.69 ± 0.02 in Ghrl-/- females) (ANOVA, sex effect: p=0.0149, genotype effect: p=0.1482, sex x genotype interaction: p=0.6274; with p<0.05 only for Ghrl-/- females vs. Ghrl+/+ males, post-hoc test).
Discussion
As compared to wild-type littermates, Ghrl-/- mice of both sexes lack major alterations in body growth, metabolic parameters or feeding behavior at adulthood. Adult Ghrl-/- females display, however, decreased amplitude of GH secretion and increased irregularity of secretion accompanied by increased immunoreactive signal for GHRH in the ArcN without change in the pituitary GH content or GH secretion from pituitary explants.
Ghrl Deletion Affects Pulsatile GH Secretion and Regularity of Ultradian GH Pattern in Adult Female, but Not Male, Mice
Among the proghrelin-derived peptides, ghrelin seems to play the most powerful control on GH secretion. Indeed, ghrelin treatment potently increases GH secretion in humans and rodents. However, the role of endogenous ghrelin on GH secretion has been more difficult to demonstrate. Endogenous ghrelin seems to regulate the amplitude of GH secretion in healthy men since ghrelin levels and GH pulse amplitude strongly correlate (49). Pharmacological blockage of GHSR in male rats decreased the amplitude of GH release but did not affect the pattern of GH secretion (50). Also, no correlation was found between acyl ghrelin or total ghrelin and GH plasma peaks in freely behaving male rats (1, 51). In male rats, we previously showed that ghrelin displays an ultradian pattern of secretion through the light and dark periods with pulses occurring with a frequency similar to GH (1). In mice, however, due to the small volume of blood and the absence of ghrelin assays with sufficient sensitivity, determining the ultradian pattern of ghrelin secretion and a possible sexual dimorphic pattern of secretion are challenging. To our knowledge, few studies have investigated the link between endogenous ghrelin and GH secretion in women or female rodents. Transgenic female, not male, rats with reduced hypothalamic GHSR expression display reduced pulsatile GH secretion (52). In contrast to adult males, adult Ghsr-/- female mice showed a reduction of total, basal and pulsatile GH secretion (37). Furthermore, Ghrl-/- and Goat-/- male mice lack alterations of pulsatile GH secretion at adulthood, although some transitory modifications are seen in young 7-8-week-old males, a period of rapid growth rate (26, 53), suggesting that ghrelin-gene derived peptides regulate pulsatile GH secretion in an age-dependent manner.
Here, we observed that adult Ghrl-/- males lack significant alterations in GH secretion whereas adult Ghrl-/- females display a reduction of pulsatile GH secretion, maximal pulse amplitude and regularity of GH secretory pattern (i.e., higher ApEn values). ApEn is considered a barometer of GH negative feedback mechanism on GH release. As observed in all species investigated so far (39), we confirmed that females show higher ApEn values and reduced pulsatile GH secretion as compared to males. The increased ApEn values in Ghrl-/- females are in line with the reduced pulsatile GH secretion, whereas the unchanged ApEn values in Ghrl-/- males point to unchanged pulsatile GH secretion, as observed herein. Of note, the higher interindividual variability observed in males for several parameters of GH secretion may have prevented from unmasking statistical genotype differences in this sex. In any case, our data demonstrate a significant effect of endogenous proghrelin-derived peptides on GH secretion in adult female mice. Since both Ghsr-/- females and Ghrl-/- females show a reduction of the pulsatile GH secretion, it seems reasonable to hypothesize that endogenous ghrelin-evoked GHSR signaling increases GH secretion in adult female mice mainly. It is important to highlight that stress is known to reduce plasma GH levels (54). The blood sampling procedure used in the current study induces only a modest rise in plasma corticosterone levels that are within the physiological range of endogenous diurnal variations (53). Furthermore, Ghrl-/- mice displayed similar plasma corticosterone levels than Ghrl+/+ mice in non-stressed conditions and in response to restrain-stress (unpublished observations). Thus, it seems unlikely that differential responses to the experimental blood sampling procedure may have contributed to reduce GH secretion in Ghrl-/- females.
Neuroendocrine Basis by Which Ghrl Deletion Affects GH Pulse Amplitude and Regularity of Ultradian GH Pattern in Female Mice
Ghrelin-induced GH secretion involves pituitary and hypothalamic mechanisms (39). In vitro, ghrelin acts on somatotropic cells of the pituitary to release GH (55). Also, ghrelin enhances GHRH-induced GH release and impairs somatostatin-mediated inhibition of GH release. Notably, ghrelin treatment does not induce GH secretion in male mice lacking GHSR exclusively in somatotropic cells (56). Ghrelin also indirectly acts at the pituitary by stimulating GHRH neurons and inhibiting somatostatin neurons via multiple mechanisms. For instance, ghrelin directly excites GHRH neurons, and also indirectly increases GHRH neuron excitability by decreasing their inhibitory GABA inputs (57). Here, we found that Ghrl-/- and Ghrl+/+ females show similar pituitary GH contents as well as a similar basal, KCl-induced and GHRH-induced secretion of GH in vitro. Conversely, a higher number of GHRH+ neurons was visualized in the ArcN of Ghrl-/- females, as compared to Ghrl+/+ females, and no changes in the GHRH levels in the median eminence (not shown) nor in the hypothalamic levels of Ghrh mRNA. Thus, hypothalamic, rather than pituitary dysfunctions are more likely associated to the reduction of the pulsatile GH secretion in Ghrl-/- females. Transgenic female rats with reduced GHSR expression in hypothalamic tyrosine hydroxylase-expressing neurons show reduced GH secretion and lower number of GHRH neurons (58), also suggesting hypothalamic basis for altered GH secretion induced by a deficit of ghrelin action in female rodents. Importantly, the GHRH neurons are poorly visualized in naive rodents because the GHRH peptide is rapidly transported to the neuron terminals for release (59). Thus, the above referred study in rats used colchicine treatment to estimate the number of GHRH neurons. Here, however, the GHRH immunolabeling was performed in brain sections of naive mice undermining our capacity to estimate the number of GHSR neurons in each experimental group. Since Ghrl-/- females display a reduction of pulsatile GH secretion, it seems reasonable to hypothesize that GHRH neurons were more easily visualized in Ghrl-/- females because GHRH secretion was reduced. Such hypothesis could be further tested by assessing plasma GH levels in Ghrl+/+ and Ghrl-/- females following somatostatin withdrawal, which triggers GHRH-dependent GH secretion in vivo (60). In this regard, it is possible that somatostatin neurons, which control the timing of GH secretory pulses and whose release activity is also under the control of ghrelin (4), are differentially affected in Ghrl-/- females and contribute to modulate GH secretion. Of note, expression of somatostatin receptor 2 on GHRH neurons displays sexual dimorphism in mice, suggesting that somatostatin interacts with GHRH neurons to control sexual dimorphic GH responses (47). Further studies are required to precisely determine the hypothalamic dysfunctions affecting the GH secretion in Ghrl-/- females. Also, the reason why alterations of GH secretion only take place in females remain uncertain. Of note, the ghrelin system displays important dimorphic responses (34). Indeed, studies in women showed that estradiol potentiates ghrelin-stimulated pulsatile GH secretion and GHRH/ghrelin synergy (61–63). In mice, females were also more sensitive to the GH-releasing effects of ghrelin treatment than males (34). Strikingly, we found that Ghsr mRNA levels in the hypothalamus were not different among genotypes, although Ghsr mRNA levels tended to be lower in Ghrl-/- than Ghrl+/+ females. Previously, Ghsr mRNA levels were found similar in the ArcN of 8-week-old male and female rats (64) and slightly lower in the hypothalamus of 6-month-old female rats, as compared to males (65).
Ghrl Deletion in Mice Does Not Significantly Affect Food Intake, Growth or Metabolism at Adulthood
As previously reported (12, 23, 25), we confirmed that adult Ghrl-/- mice – male and female - lack alterations of spontaneous food intake, meal pattern or fast-refeeding hyperphagia. Also, adult Ghrl-/- mice show no major growth or metabolic deficits, as previously shown (26), despite adult Ghrl-/- females show alterations of their GH secretion pattern. Notably, previous studies also showed that young Ghrl-/- males display alterations in ultradian GH secretion patterns that are not associated with changes in growth or metabolic parameters (26). Similarly, young Goat-/- mice show reduced GH pulse amplitude but unaltered body weight or body growth, although an increase in plasma insulin-like growth factor I levels has been proposed to compensate the alterations in GH secretion in these mice (53). Thus, the lack of proghrelin-derived peptides seems to induce some sexually dimorphic alterations in the GH axis that are insufficient to affect eating behaviors, body growth and metabolism at adulthood. Still, altered GH secretion during adulthood could contribute to specific phenotypes associated with ageing or pathophysiological conditions. Indeed, we observed a tendency to reduced volume of mineralized bone and significant reductions in the mass of soleus muscle in older 80-week-old Ghrl-/- mice that may be linked to ghrelin since this hormone plays a protective effect on bones structure in older mice (66) and both acyl and des-acyl ghrelin enhance muscle anabolism (67) and prevent skeletal muscle atrophy (68).
The Absence of Evident Dysfunctions in Adult Mice Lacking Ghrl Gene Must Be Interpreted With Caution
The study of mice with genetic modifications of different elements of the ghrelin system, such as proghrelin, GOAT or GHSR, has been instrumental to reveal some aspects of their physiological role. For instance, the observation that Goat-/- mice, in contrast to WT mice, suffer severe hypoglycemia and become moribund in a starvation protocol indicates that ghrelin plays essential functions under energy deficit (21). In this regard, it is interesting to stress that Ghrl-/- mice exhibit less evident alteration than mice lacking GHSR [as review in (12)]. For instance, GHSR-deficient mice, but not Ghrl-/- mice, show reduced hyperphagia after fasting (25). GHSR-deficient mice show some alterations, as compared to Ghrl-/- mice, even under ad libitum feeding conditions. In contrast to Ghrl-/- mice, adult Ghsr-/- mice fed on a regular chow displayed reduced body weight and linear growth, regardless of the sex (37). Furthermore, meal frequency was reduced in Ghsr-/- male mice (37). The exact molecular mechanisms underlying such distinct phenotypes in Ghsr-/- mice, as compared to Ghrl-/- mice, are uncertain. On one hand, GHSR acts via several ligand-independent mechanisms that include its capacity to induce constitutive intracellular signaling or its ability to cross-talk with other receptors (69). Also, the deletion of Ghrl gene not only eliminates ghrelin but also obestatin and des-acyl-ghrelin, which seem to impair the effect of ghrelin. For instance, obestatin treatment antagonizes ghrelin-induced GH secretion in rats (70), although these observations were not confirmed by others (71, 72). Des-acyl ghrelin appears to block some of the effects of ghrelin via either GHSR-dependent or GHSR-independent mechanisms (12, 73, 74). Finally, the liver-expressed antimicrobial peptide 2 (LEAP2) was recently recognized as another endogenous peptide ligand for GHSR (75). LEAP2, which is released by endocrine cells of the liver and the intestinal tract, abrogates ghrelin-evoked and constitutive GHSR activities (75, 76). Notably, LEAP2 displays a binding affinity to GHSR similar to ghrelin, but its level in plasma is ~10-fold higher than ghrelin level in ad libitum fed conditions (69). Thus, LEAP2 may play a more important role than ghrelin on GHSR under the tested conditions, masking some of the consequences of Ghrl gene deletion.
In conclusion, present data suggest that ghrelin plays a more prominent role in the regulation of pulsatile GH secretion in adult female than male mice. The mechanism of the sex-specific effect of preproghrelin deletion on GH pulsatility still needs to be refined. The physiological implications of the altered GH pulsatility in preproghrelin deleted mice would also need to be clarified since it is not associated with major growth, feeding or metabolic phenotypes.
Data Availability Statement
The raw data supporting the conclusions of this article will be made available by the authors, without undue reservation.
Ethics Statement
The animal study was reviewed and approved by Animal Experimentation Committee CEEA.34 of University of Paris.
Author Contributions
RH, GF, MP, JE, and VT designed experiments. RH, GF, NL, AL, OF, PZ, and OV performed experiments. FR performed jkApEn and deconvolution analysis of GH pulsatility parameters. CC performed all studies and analysis on bone parameters. CT provided the colony of preproghrelin deficient mice. RH, GF, NL, MP, and VT analyzed data. MP and VT wrote the manuscript. JE edited the manuscript. All authors contributed to the article and approved the submitted version.
Funding
This work was supported by an Agence Nationale de la Recherche Jeunes Chercheuses Jeunes Chercheurs Grant ANR-12-JSV1–0013-01 (to VT), Institut National de la Santé et de la Recherche Médicale (INSERM), grants from the Fondo para la Investigación Científica y Tecnológica (FONCyT, PICT2016-1084 and PICT2017-3196) and from CONICET (PUE-2017) to MP. GF was supported by CONICET.
Conflict of Interest
The authors declare that the research was conducted in the absence of any commercial or financial relationships that could be construed as a potential conflict of interest.
Publisher’s Note
All claims expressed in this article are solely those of the authors and do not necessarily represent those of their affiliated organizations, or those of the publisher, the editors and the reviewers. Any product that may be evaluated in this article, or claim that may be made by its manufacturer, is not guaranteed or endorsed by the publisher.
Acknowledgments
We are grateful to the “Plateforme d’Expérimentation Fonctionnelle du Petit Animal” at UMR894 INSERM and to the animal facility and PhenoBrain Platform at UMR-S 1266 INSERM for the care of the animals and to Alice Cougnon and Chloé Got for genotyping and technical support. We are grateful to Dr A. F. Parlow (National Hormone and Peptide Program, Los Angeles, California) for providing the GH assay reagents. We are also grateful to Dr Johannes Veldhuis (Mayo School of Graduate Medical Education, Mayo Clinic, Rochester, USA) for providing his support in setting up analyses’ parameters for GH profiles. We are thankful to Flore Miellot (Marrow Adiposity and Bone Lab ULR4490, Univ. Littoral Côte d’Opale, Boulogne/Mer France) for micro-computed tomography analysis. We thank the Argentinian Bec.AR program for the financial support to cover the exchange of GF.
Supplementary Material
The Supplementary Material for this article can be found online at: https://www.frontiersin.org/articles/10.3389/fendo.2021.754522/full#supplementary-material
References
1. Zizzari P, Hassouna R, Grouselle D, Epelbaum J, Tolle V. Physiological Roles of Preproghrelin-Derived Peptides in GH Secretion and Feeding. Peptides (2011) 32:2274–82. doi: 10.1016/j.peptides.2011.04.014
2. Kojima M, Hosoda H, Date Y, Nakazato M, Matsuo H, Kangawa K. Ghrelin Is a Growth-Hormone-Releasing Acylated Peptide From Stomach. Nature (1999) 402:656–60. doi: 10.1038/45230
3. Tomasetto C, Karam SM, Ribieras S, Masson R, Lefebvre O, Staub A, et al. Identification and Characterization of a Novel Gastric Peptide Hormone: The Motilin-Related Peptide. Gastroenterology (2000) 119:395–405. doi: 10.1053/gast.2000.9371
4. Tolle V, Zizzari P, Tomasetto C, Rio MC, Epelbaum J, Bluet-Pajot MT. In Vivo and In Vitro Effects of Ghrelin/Motilin-Related Peptide on Growth Hormone Secretion in the Rat. Neuroendocrinology (2001) 73:54–61. doi: 10.1159/000054620
5. Tannenbaum GS, Lapointe M, Beaudet A, Howard AD. Expression of Growth Hormone Secretagogue-Receptors by Growth Hormone-Releasing Hormone Neurons in the Mediobasal Hypothalamus. Endocrinology (1998) 139:4420–3. doi: 10.1210/endo.139.10.6330
6. Nakazato M, Murakami N, Date Y, Kojima M, Matsuo H, Kangawa K, et al. A Role for Ghrelin in the Central Regulation of Feeding. Nature (2001) 409:194–8. doi: 10.1038/35051587
7. Broglio F, Arvat E, Benso A, Gottero C, Muccioli G, Papotti M, et al. Ghrelin, a Natural GH Secretagogue Produced by the Stomach, Induces Hyperglycemia and Reduces Insulin Secretion in Humans. J Clin Endocrinol Metab (2001) 86:5083–6. doi: 10.1210/jcem.86.10.8098
8. Broglio F, Gottero C, Benso A, Prodam F, Destefanis S, Gauna C, et al. Effects of Ghrelin on the Insulin and Glycemic Responses to Glucose, Arginine, or Free Fatty Acids Load in Humans. J Clin Endocrinol Metab (2003) 88:4268–72. doi: 10.1210/jc.2002-021940
9. Chuang JC, Sakata I, Kohno D, Perello M, Osborne-Lawrence S, Repa JJ, et al. Ghrelin Directly Stimulates Glucagon Secretion From Pancreatic Alpha-Cells. Mol Endocrinol (2011) 25:1600–11. doi: 10.1210/me.2011-1001
10. Cabral A, Suescun O, Zigman JM, Perello M. Ghrelin Indirectly Activates Hypophysiotropic CRF Neurons in Rodents. PloS One (2012) 7:e31462. doi: 10.1371/journal.pone.0031462
11. Perello M, Sakata I, Birnbaum S, Chuang JC, Osborne-Lawrence S, Rovinsky SA, et al. Ghrelin Increases the Rewarding Value of High-Fat Diet in an Orexin-Dependent Manner. Biol Psychiatry (2010) 67:880–6. doi: 10.1016/j.biopsych.2009.10.030
12. Uchida A, Zigman JM, Perello M. Ghrelin and Eating Behavior: Evidence and Insights From Genetically-Modified Mouse Models. Front Neurosci (2013) 7:121. doi: 10.3389/fnins.2013.00121
13. Ma X, Lin L, Qin G, Lu X, Fiorotto M, Dixit VD, et al. Ablations of Ghrelin and Ghrelin Receptor Exhibit Differential Metabolic Phenotypes and Thermogenic Capacity During Aging. PloS One (2011) 6:e16391. doi: 10.1371/journal.pone.0016391
14. Pfluger PT, Kirchner H, Gunnel S, Schrott B, Perez-Tilve D, Fu S, et al. Simultaneous Deletion of Ghrelin and its Receptor Increases Motor Activity and Energy Expenditure. Am J Physiol Gastrointestinal Liver Physiol (2008) 294:G610–8. doi: 10.1152/ajpgi.00321.2007
15. Sato T, Kurokawa M, Nakashima Y, Ida T, Takahashi T, Fukue Y, et al. Ghrelin Deficiency Does Not Influence Feeding Performance. Regul Pept (2008) 145:7–11. doi: 10.1016/j.regpep.2007.09.010
16. Sun Y, Ahmed S, Smith RG. Deletion of Ghrelin Impairs Neither Growth Nor Appetite. Mol Cell Biol (2003) 23:7973–81. doi: 10.1128/MCB.23.22.7973-7981.2003
17. Wortley KE, Anderson KD, Garcia K, Murray JD, Malinova L, Liu R, et al. Genetic Deletion of Ghrelin Does Not Decrease Food Intake But Influences Metabolic Fuel Preference. Proc Natl Acad Sci USA (2004) 101:8227–32. doi: 10.1073/pnas.0402763101
18. Gutierrez JA, Solenberg PJ, Perkins DR, Willency JA, Knierman MD, Jin Z, et al. Ghrelin Octanoylation Mediated by an Orphan Lipid Transferase. Proc Natl Acad Sci USA (2008) 105:6320–5. doi: 10.1073/pnas.0800708105
19. Yang J, Brown MS, Liang G, Grishin NV, Goldstein JL. Identification of the Acyltransferase That Octanoylates Ghrelin, an Appetite-Stimulating Peptide Hormone. Cell (2008) 132:387–96. doi: 10.1016/j.cell.2008.01.017
20. Kirchner H, Gutierrez JA, Solenberg PJ, Pfluger PT, Czyzyk TA, Willency JA, et al. GOAT Links Dietary Lipids With the Endocrine Control of Energy Balance. Nat Med (2009) 15:741–5. doi: 10.1038/nm.1997
21. Zhao TJ, Liang G, Li RL, Xie X, Sleeman MW, Murphy AJ, et al. Ghrelin O-Acyltransferase (GOAT) Is Essential for Growth Hormone-Mediated Survival of Calorie-Restricted Mice. Proc Natl Acad Sci USA (2010) 107:7467–72. doi: 10.1038/nm.1997
22. McFarlane MR, Brown MS, Goldstein JL, Zhao TJ. Induced Ablation of Ghrelin Cells in Adult Mice Does Not Decrease Food Intake, Body Weight, or Response to High-Fat Diet. Cell Metab (2014) 20:54–60. doi: 10.1016/j.cmet.2014.04.007
23. Sun Y, Wang P, Zheng H, Smith RG. Ghrelin Stimulation of Growth Hormone Release and Appetite Is Mediated Through the Growth Hormone Secretagogue Receptor. Proc Natl Acad Sci USA (2004) 101:4679–84. doi: 10.1073/pnas.0305930101
24. Sun Y, Butte NF, Garcia JM, Smith RG. Characterization of Adult Ghrelin and Ghrelin Receptor Knockout Mice Under Positive and Negative Energy Balance. Endocrinology (2008) 149:843–50. doi: 10.1210/en.2007-0271
25. Fernandez G, Cabral A, Andreoli MF, Labarthe A, M’Kadmi C, Ramos JG, et al. Evidence Supporting a Role for Constitutive Ghrelin Receptor Signaling in Fasting-Induced Hyperphagia in Male Mice. Endocrinology (2018) 159:1021–34. doi: 10.1210/en.2017-03101
26. Hassouna R, Zizzari P, Tomasetto C, Veldhuis JD, Fiquet O, Labarthe A, et al. An Early Reduction in GH Peak Amplitude in Preproghrelin-Deficient Male Mice has a Minor Impact on Linear Growth. Endocrinology (2014) 155:3561–71. doi: 10.1210/en.2014-1126
27. Wortley KE, del Rincon JP, Murray JD, Garcia K, Iida K, Thorner MO, et al. Absence of Ghrelin Protects Against Early-Onset Obesity. J Clin Invest (2005) 115:3573–8. doi: 10.1172/JCI26003
28. Dezaki K, Sone H, Koizumi M, Nakata M, Kakei M, Nagai H, et al. Blockade of Pancreatic Islet-Derived Ghrelin Enhances Insulin Secretion to Prevent High-Fat Diet-Induced Glucose Intolerance. Diabetes (2006) 55:3486–93. doi: 10.2337/db06-0878
29. Kern A, Albarran-Zeckler R, Walsh HE, Smith RG. Apo-Ghrelin Receptor Forms Heteromers With DRD2 in Hypothalamic Neurons and Is Essential for Anorexigenic Effects of DRD2 Agonism. Neuron (2012) 73:317–32. doi: 10.1016/j.neuron.2011.10.038
30. De Smet B, Depoortere I, Moechars D, Swennen Q, Moreaux B, Cryns K, et al. Energy Homeostasis and Gastric Emptying in Ghrelin Knockout Mice. J Pharmacol Exp Ther (2006) 316:431–9. doi: 10.1124/jpet.105.091504
31. Butera PC, Clough SJ, Bungo A. Cyclic Estradiol Treatment Modulates the Orexigenic Effects of Ghrelin in Ovariectomized Rats. Pharmacol Biochem Behav (2014) 124:356–60. doi: 10.1016/j.pbb.2014.07.004
32. Clegg DJ, Brown LM, Zigman JM, Kemp CJ, Strader AD, Benoit SC, et al. Estradiol-Dependent Decrease in the Orexigenic Potency of Ghrelin in Female Rats. Diabetes (2007) 56:1051–8. doi: 10.2337/db06-0015
33. Matsubara M, Sakata I, Wada R, Yamazaki M, Inoue K, Sakai T. Estrogen Modulates Ghrelin Expression in the Female Rat Stomach. Peptides (2004) 25:289–97. doi: 10.1016/j.peptides.2003.12.020
34. Yamada C, Iizuka S, Nahata M, Hattori T, Takeda H. Vulnerability to Psychological Stress-Induced Anorexia in Female Mice Depends on Blockade of Ghrelin Signal in Nucleus Tractus Solitarius. Br J Pharmacol (2020) 177:4666–82. doi: 10.1111/bph.15219
35. Zigman JM, Nakano Y, Coppari R, Balthasar N, Marcus JN, Lee CE, et al. Mice Lacking Ghrelin Receptors Resist the Development of Diet-Induced Obesity. J Clin Invest (2005) 115:3564–72. doi: 10.1172/JCI26002
36. Calder AN, Yu T, Dahir NS, Sun Y, Gilbertson TA. Ghrelin Receptors Enhance Fat Taste Responsiveness in Female Mice. Nutrients (2021) 13(4):1045. doi: 10.3390/nu13041045
37. Labarthe A, Zizzari P, Fiquet O, Lebrun N, Veldhuis JD, Roelfsema F, et al. Effect of GHS-R Deletion on Growth, Pulsatile GH Secretion and Meal Pattern in Male and Female Mice. Neuroendocrinology (2021). doi: 10.1159/000516147
38. Huang L, Huang Z, Chen C. Rhythmic Growth Hormone Secretion in Physiological and Pathological Conditions: Lessons From Rodent Studies. Mol Cell Endocrinol (2019) 498:110575. doi: 10.1016/j.mce.2019.110575
39. Steyn FJ, Tolle V, Chen C, Epelbaum J. Neuroendocrine Regulation of Growth Hormone Secretion. Compr Physiol (2016) 6:687–735. doi: 10.1002/cphy.c150002
40. Steyn FJ, Huang L, Ngo ST, Leong JW, Tan HY, Xie TY, et al. Development of a Method for the Determination of Pulsatile Growth Hormone Secretion in Mice. Endocrinology (2011) 152:3165–71. doi: 10.1210/en.2011-0253
41. Keenan DM, Roelfsema F, Veldhuis JD. Dose-Response Downregulation Within the Span of Single Interpulse Intervals. Am J Physiol Regulatory Integr Comp Physiol (2010) 299:R11–8. doi: 10.1152/ajpregu.00201.2010
42. Meyfroidt G, Keenan DM, Wang X, Wouters PJ, Veldhuis JD, Van den Berghe G. Dynamic Characteristics of Blood Glucose Time Series During the Course of Critical Illness: Effects of Intensive Insulin Therapy and Relative Association With Mortality. Crit Care Med (2010) 38:1021–9. doi: 10.1097/CCM.0b013e3181cf710e
43. Veldhuis JD, Johnson ML, Veldhuis OL, Straume M, Pincus SM. Impact of Pulsatility on the Ensemble Orderliness (Approximate Entropy) of Neurohormone Secretion. Am J Physiol Regulatory Integr Comp Physiol (2001) 281:R1975–85. doi: 10.1152/ajpregu.2001.281.6.R1975
44. Hassouna R, Zizzari P, Viltart O, Yang SK, Gardette R, Videau C, et al. A Natural Variant of Obestatin, Q90L, Inhibits Ghrelin’s Action on Food Intake and GH Secretion and Targets NPY and GHRH Neurons in Mice. PloS One (2012) 7:e51135. doi: 10.1371/journal.pone.0051135
45. Luque RM, Huang ZH, Shah B, Mazzone T, Kineman RD. Effects of Leptin Replacement on Hypothalamic-Pituitary Growth Hormone Axis Function and Circulating Ghrelin Levels in Ob/Ob Mice. Am J Physiol Endocrinol Metab (2007) 292:E891–9. doi: 10.1152/ajpendo.00258.2006
46. Cabral A, Fernandez G, Tolosa MJ, Rey Moggia A, Calfa G, De Francesco PN, et al. Fasting Induces Remodeling of the Orexigenic Projections From the Arcuate Nucleus to the Hypothalamic Paraventricular Nucleus, in a Growth Hormone Secretagogue Receptor-Dependent Manner. Mol Metab (2020) 32:69–84. doi: 10.1016/j.molmet.2019.11.014
47. Bouyer K, Loudes C, Robinson IC, Epelbaum J, Faivre-Bauman A. Sexually Dimorphic Distribution of Sst2a Somatostatin Receptors on Growth Hormone-Releasing Hormone Neurons in Mice. Endocrinology (2006) 147:2670–4. doi: 10.1210/en.2005-1462
48. Paxinos G, Franklin KBJ, Franklin KBJ. The Mouse Brain in Stereotaxic Coordinates. San Diego: Academic Press (2001).
49. Nass R, Gaylinn BD, Thorner MO. The Role of Ghrelin in GH Secretion and GH Disorders. Mol Cell Endocrinol (2011) 340:10–4. doi: 10.1016/j.mce.2011.03.021
50. Zizzari P, Halem H, Taylor J, Dong JZ, Datta R, Culler MD, et al. Endogenous Ghrelin Regulates Episodic Growth Hormone (GH) Secretion by Amplifying GH Pulse Amplitude: Evidence From Antagonism of the GH Secretagogue-R1a Receptor. Endocrinology (2005) 146:3836–42. doi: 10.1210/en.2005-0212
51. Tolle V, Bassant M-H, Zizzari P, Poindessous-Jazat F, Tomasetto C, Epelbaum J, et al. Ultradian Rhythmicity of Ghrelin Secretion in Relation With GH, Feeding Behavior, and Sleep-Wake Patterns in Rats. Endocrinology (2002) 143:1353–61. doi: 10.1210/endo.143.4.8712
52. Shuto Y, Shibasaki T, Otagiri A, Kuriyama H, Ohata H, Tamura H, et al. Hypothalamic Growth Hormone Secretagogue Receptor Regulates Growth Hormone Secretion, Feeding, and Adiposity. J Clin Invest (2002) 109:1429–36. doi: 10.1172/JCI0213300
53. Xie TY, Ngo ST, Veldhuis JD, Jeffery PL, Chopin LK, Tschop M, et al. Effect of Deletion of Ghrelin-O-Acyltransferase on the Pulsatile Release of Growth Hormone in Mice. J Neuroendocrinol (2015) 27:872–86. doi: 10.1111/jne.12327
54. Xu J, Bekaert AJ, Dupont J, Rouve S, Annesi-Maesano I, De Magalhaes Filho CD, et al. Exploring Endocrine GH Pattern in Mice Using Rank Plot Analysis and Random Blood Samples. J Endocrinol (2011) 208:119–29. doi: 10.1677/JOE-10-0317
55. Reichenbach A, Steyn FJ, Sleeman MW, Andrews ZB. Ghrelin Receptor Expression and Colocalization With Anterior Pituitary Hormones Using a GHSR-GFP Mouse Line. Endocrinology (2012) 153:5452–66. doi: 10.1210/en.2012-1622
56. Gupta D, Patterson AM, Osborne-Lawrence S, Bookout AL, Varshney S, Shankar K, et al. Disrupting the Ghrelin-Growth Hormone Axis Limits Ghrelin’s Orexigenic But Not Glucoregulatory Actions. Mol Metab (2021) 53:101258. doi: 10.1016/j.molmet.2021.101258
57. Feng DD, Yang SK, Loudes C, Simon A, Al-Sarraf T, Culler M, et al. Ghrelin and Obestatin Modulate Growth Hormone-Releasing Hormone Release and Synaptic Inputs Onto Growth Hormone-Releasing Hormone Neurons. Eur J Neurosci (2011) 34:732–44. doi: 10.1111/j.1460-9568.2011.07787.x
58. Mano-Otagiri A, Nemoto T, Sekino A, Yamauchi N, Shuto Y, Sugihara H, et al. Growth Hormone-Releasing Hormone (GHRH) Neurons in the Arcuate Nucleus (Arc) of the Hypothalamus Are Decreased in Transgenic Rats Whose Expression of Ghrelin Receptor Is Attenuated: Evidence That Ghrelin Receptor Is Involved in the Up-Regulation of GHRH Expression in the Arc. Endocrinology (2006) 147:4093–103. doi: 10.1210/en.2005-1619
59. Kuwahara S, Kesuma Sari D, Tsukamoto Y, Tanaka S, Sasaki F. Age-Related Changes in Growth Hormone (GH)-Releasing Hormone and Somatostatin Neurons in the Hypothalamus and in GH Cells in the Anterior Pituitary of Female Mice. Brain Res (2004) 1025:113–22. doi: 10.1016/j.brainres.2004.08.012
60. Clark RG, Carlsson LM, Rafferty B, Robinson IC. The Rebound Release of Growth Hormone (GH) Following Somatostatin Infusion in Rats Involves Hypothalamic GH-Releasing Factor Release. J Endocrinol (1988) 119:397–404. doi: 10.1677/joe.0.1190397
61. Kok P, Paulo RC, Cosma M, Mielke KL, Miles JM, Bowers CY, et al. Estrogen Supplementation Selectively Enhances Hypothalamo-Pituitary Sensitivity to Ghrelin in Postmenopausal Women. J Clin Endocrinol Metab (2008) 93:4020–6. doi: 10.1210/jc.2008-0522
62. Norman C, Rollene N, Weist SM, Wigham JR, Erickson D, Miles JM, et al. Short-Term Estradiol Supplementation Potentiates Low-Dose Ghrelin Action in the Presence of GHRH or Somatostatin in Older Women. J Clin Endocrinol Metab (2014) 99:E73–80. doi: 10.1210/jc.2013-3043
63. Veldhuis JD, Keenan DM, Iranmanesh A, Mielke K, Miles JM, Bowers CY. Estradiol Potentiates Ghrelin-Stimulated Pulsatile Growth Hormone Secretion in Postmenopausal Women. J Clin Endocrinol Metab (2006) 91:3559–65. doi: 10.1210/jc.2006-0948
64. Bennett PA, Thomas GB, Howard AD, Feighner SD, van der Ploeg LH, Smith RG, et al. Hypothalamic Growth Hormone Secretagogue-Receptor (GHS-R) Expression Is Regulated by Growth Hormone in the Rat. Endocrinology (1997) 138:4552–7. doi: 10.1210/endo.138.11.5476
65. Priego T, Sanchez J, Pico C, Palou A. Sex-Associated Differences in the Leptin and Ghrelin Systems Related With the Induction of Hyperphagia Under High-Fat Diet Exposure in Rats. Hormones Behav (2009) 55:33–40. doi: 10.1016/j.yhbeh.2008.07.010
66. van der Velde M, van der Eerden BC, Sun Y, Almering JM, van der Lely AJ, Delhanty PJ, et al. An Age-Dependent Interaction With Leptin Unmasks Ghrelin’s Bone-Protective Effects. Endocrinology (2012) 153:3593–602. doi: 10.1210/en.2012-1277
67. Wu CS, Wei Q, Wang H, Kim DM, Balderas M, Wu G, et al. Protective Effects of Ghrelin on Fasting-Induced Muscle Atrophy in Aging Mice. J Gerontol A Biol Sci Med Sci (2020) 75:621–30. doi: 10.1093/gerona/gly256
68. Porporato PE, Filigheddu N, Reano S, Ferrara M, Angelino E, Gnocchi VF, et al. Acylated and Unacylated Ghrelin Impair Skeletal Muscle Atrophy in Mice. J Clin Invest (2013) 123:611–22. doi: 10.1172/JCI39920
69. Cornejo MP, Mustafa ER, Barrile F, Cassano D, De Francesco PN, Raingo J, et al. The Intriguing Ligand-Dependent and Ligand-Independent Actions of the Growth Hormone Secretagogue Receptor on Reward-Related Behaviors. Neurosci Biobehav Rev (2021) 120:401–16. doi: 10.1016/j.neubiorev.2020.10.017
70. Zizzari P, Longchamps R, Epelbaum J, Bluet-Pajot MT. Obestatin Partially Affects Ghrelin Stimulation of Food Intake and Growth Hormone Secretion in Rodents. Endocrinology (2007) 148:1648–53. doi: 10.1210/en.2006-1231
71. Bresciani E, Rapetti D, Dona F, Bulgarelli I, Tamiazzo L, Locatelli V, et al. Obestatin Inhibits Feeding But Does Not Modulate GH and Corticosterone Secretion in the Rat. J Endocrinol Invest (2006) 29:RC16–8. doi: 10.1007/BF03344175
72. Yamamoto D, Ikeshita N, Daito R, Herningtyas EH, Toda K, Takahashi K, et al. Neither Intravenous Nor Intracerebroventricular Administration of Obestatin Affects the Secretion of GH, PRL, TSH and ACTH in Rats. Regul Pept (2007) 138:141–4. doi: 10.1016/j.regpep.2006.09.001
73. Inhoff T, Monnikes H, Noetzel S, Stengel A, Goebel M, Dinh QT, et al. Desacyl Ghrelin Inhibits the Orexigenic Effect of Peripherally Injected Ghrelin in Rats. Peptides (2008) 29:2159–68. doi: 10.1016/j.peptides.2008.09.014
74. Fernandez G, Cabral A, Cornejo MP, De Francesco PN, Garcia-Romero G, Reynaldo M, et al. Des-Acyl Ghrelin Directly Targets the Arcuate Nucleus in a Ghrelin-Receptor Independent Manner and Impairs the Orexigenic Effect of Ghrelin. J Neuroendocrinol (2016) 28:12349. doi: 10.1111/jne.12349
75. Ge X, Yang H, Bednarek MA, Galon-Tilleman H, Chen P, Chen M, et al. LEAP2 Is an Endogenous Antagonist of the Ghrelin Receptor. Cell Metab (2018) 27:461–469 e6. doi: 10.1016/j.cmet.2017.10.016
Keywords: ghrelin, growth hormone, food intake, sexual dimorphism, pulsatility
Citation: Hassouna R, Fernandez G, Lebrun N, Fiquet O, Roelfsema F, Labarthe A, Zizzari P, Tomasetto C, Epelbaum J, Viltart O, Chauveau C, Perello M and Tolle V (2021) Ghrelin Gene Deletion Alters Pulsatile Growth Hormone Secretion in Adult Female Mice. Front. Endocrinol. 12:754522. doi: 10.3389/fendo.2021.754522
Received: 06 August 2021; Accepted: 16 September 2021;
Published: 13 October 2021.
Edited by:
Hubert Vaudry, Université de Rouen, FranceReviewed by:
Laurent Gautron, University of Texas Southwestern Medical Center, United StatesTimothy Wells, Cardiff University, United Kingdom
Copyright © 2021 Hassouna, Fernandez, Lebrun, Fiquet, Roelfsema, Labarthe, Zizzari, Tomasetto, Epelbaum, Viltart, Chauveau, Perello and Tolle. This is an open-access article distributed under the terms of the Creative Commons Attribution License (CC BY). The use, distribution or reproduction in other forums is permitted, provided the original author(s) and the copyright owner(s) are credited and that the original publication in this journal is cited, in accordance with accepted academic practice. No use, distribution or reproduction is permitted which does not comply with these terms.
*Correspondence: Virginie Tolle, virginie.tolle@inserm.fr; Mario Perello, mperello@imbice.gov.ar
†Present Address: Rim Hassouna, Danone Nutricia Research, Palaiseau, France
Oriane Fiquet, Innate Immunity Unit, Department of Immunology, INSERM U1223, Institut Pasteur, Paris, France
Philippe Zizzari, Univ. Bordeaux, INSERM, Magendie, U1215, Bordeaux, France
‡These authors have contributed equally to this work and share first authorship
§These authors have contributed equally to this work and share senior authorship