- 1Department of Traditional Chinese Medicine, Shanghai Jiao Tong University Affiliated Sixth People’s Hospital, Shanghai, China
- 2The Second Clinical Medical College, Heilongjiang University of Chinese Medicine, Harbin, China
The incidence of sporadic Alzheimer’s disease (AD) is increasing in recent years. Studies have shown that in addition to some genetic abnormalities, the majority of AD patients has a history of long-term exposure to risk factors. Neuroendocrine related risk factors have been proved to be strongly associated with AD. Long-term hormone disorder can have a direct detrimental effect on the brain by producing an AD-like pathology and result in cognitive decline by impairing neuronal metabolism, plasticity and survival. Traditional Chinese Medicine(TCM) may regulate the complex process of endocrine disorders, and improve metabolic abnormalities, as well as the resulting neuroinflammation and oxidative damage through a variety of pathways. TCM has unique therapeutic advantages in treating early intervention of AD-related neuroendocrine disorders and preventing cognitive decline. This paper reviewed the relationship between neuroendocrine and AD as well as the related TCM treatment and its mechanism. The advantages of TCM intervention on endocrine disorders and some pending problems was also discussed, and new insights for TCM treatment of dementia in the future was provided.
1 Introduction
Alzheimer’s disease (AD), a neurodegenerative disease with worldwide increasing prevalence, morbidity and mortality in recent years, is the fifth leading cause of death in adults over 65 years old now (1). The number of reported deaths from AD has increased by more than 145% in the past decade (2). In addition to the sharply rising number of deaths,AD is also burdening patients socially and economically year by year with the social aging process. In recent years, the prevention and treatment of AD has become a hot topic in medical research (3).
Since the neurological damage at late stage of AD is difficult to reverse, the current interest of AD treatment is mainly on early diagnosis, symptomatic treatment, slowing down the development of AD and improving cognitive function. AD as defined in the 2011 NIA-AA guidelines has three stages. Among them, MCI identifies individuals who do not have dementia, but who do have some deficits in cognition (4). 40%-75% of MCI patients may develop AD, and the risk of AD increases with the accumulation of amyloid beta (Aβ) and neurodegeneration. Although AD has a certain genetic tendency, the incidence of AD caused by the combination of multiple etiologies is increasing in recent years (5). Studies have shown that cardiovascular diseases, metabolic disorders, endocrine disorders, anxiety and depression are all risk factors for the development of AD. Early intervention of related risk factors have been proved to have an effect on reducing the risk of AD, even reversing the cognitive loss of MCI patients and delaying the progress of the disease (6, 7).
Previous studies on AD have found that cognitive loss is a cumulative process. Although human brain has a great potential and sufficient cognitive reserve, the onset of sporadic AD is often the result of the joint action of genes and environment, and the continuous accumulation of risk factors often leads to worse outcomes (8). Despite the complex pathogenesis of AD, studies have shown that some endocrine related factors show a significant correlation in the early stage of the disease. The effects of the nervous system and the endocrine system are bidirectional, and both of them jointly regulate brain functions such as nervous system homeostasis, emotion and cognition (9). On the one hand, many metabolic disorders appear before cognitive disorders, so some scholars believe that endocrine disorders are one of the pathogenesis of AD. On the other hand, other scholars believe that due to the regulation effects of the nervous system on endocrine, neurological diseases themselves can also lead to abnormal hormone levels, and some endocrine specific markers have been used for early diagnosis of AD (10). Regulating neuroendocrinology related factors is a promising treatment for people who need to control AD symptoms or reduce the risk of suffering from AD (11).
In traditional Chinese medicine(TCM), Alzheimer’s disease is classified as “forgetfulness”, “fatigue” and “ idiocy”. Based on TCM theory, marrow is an essence for the formation and maintenance of the human body, and is directly related to the functions of the five viscera such as kidney and spleen. Some scholars believe that the marrow can be understood as the connection between endocrine organ and nervous system, and it follows the dynamic balancing process of extraordinary fu organs. Modern studies have also found that the functions of kidney and spleen in TCM are closely related to neuro-endocrine-immune functions. Studies have shown that the deficiency of kidney essence is related to the dysfunction of HPA axis. The role of HPA-mediated BDNF in regulating neuroplasticity is consistent with the traditional notion that the kidney intervenes in brain function by affecting the brain marrow (12). In TCM, the spleen governs transportation and transformation of grain and water and distribution of its essence. Spleen and stomach disorders to cause phlegm and dampness, which blocks the operation of qi and blood. When phlegm and blood stasis stays in the brain for a long time, it is easy to develop AD. Here, the function of the spleen is adapted to the digestive and endocrine functions of the pancreas in modern medicine. Both of them regulate the metabolism of substances and energy in the body. The abnormal metabolism of substances and energy in AD also leads to the deposition of metabolic wastes in the brain, affecting neurological functions and leading to the pathogenesis of AD, which is also consistent with the theory of TCM (13). Therefore, the relevant treatments in traditional medicine are likely to have a good treatment effect on AD. Screening effective TCM treatment by modern technology and further studying of relevant mechanisms can promote TCM modern application.
The course from neuroendocrine disorders to cognitive impairment is a long and cumulative process (14). A variety of factors interact with each other in this process. It is difficult to treat a single target and achieve specific efficacy without having any side effects. This makes TCM have unique advantages over other treatments in AD. Natural chemicals have the characteristics of multi-target and multi-mechanism in living body, and can control the various nerve injury caused by AD. Mechanisms of action of TCM have become the focus of academic attention in recent years (15). Compared with the existing treatment methods of Western medicine for AD, TCM can intervene in early stage of cognitive decline and prevent the progression of AD without causing side effects such as sleep disorders, hallucinations and movement disorders, so it is widely welcomed by most patients (16).
Nerve cells are very sensitive to stress, and the immediate reaction of the nervous and endocrine systems is the survival mechanism during evolution. These organisms respond to changes in the outside world. Chronic stress leads to endocrine disorders, promotes inflammation and oxidative stress, and makes harmful environment continuously act on nerve cells. This also results in the accumulation of toxic substances in the nervous system. Many natural ingredients of TCM can not only restore hormone levels, but also have anti-inflammatory and antioxidant features. This exactly corresponds precisely to the role TCM played in cutting off the vicious cycle in AD (17). Flavonoids are rich in many TCMs. They can reduce neuroinflammatory reactions and regulate oxidative stress related reactions by regulating the autosecretory of nerve cells and the active factors secreted by glial cells. Albiflorin (AL) can reduce AD pathology and improve cognitive impairment by regulating oxidative stress and inflammation in the brain (18). Nobiletin(NOB) can regulate microglia and relieve neuroinflammation (19). Interestingly, TCM can also regulate the level of inflammation in circulation through systemic action, and cut off the harmful communication between peripheral and central organs, thus achieving a fundamental effect in treating AD (20). Studies have shown that some flavonoids themselves have antioxidant and inhibitory effects on amyloid protein aggregation, which is generally related to the position of flavonoid hydroxyl group (21). Abnormal amyloid protein aggregation is also an important pathology of islet dysfunction and AD (22). Flavonoids anthocyanins can enhance insulin sensitivity, weaken insulin resistance at target tissue, and inhibit free aliphatic acid oxidation (23–25). Overlapping mechanisms create preconditions for the dual effects of TCM in endocrine and nervous system (26, 27). In addition to improving the downstream effects of the endocrine axis, some TCM recipes such as ZiBuPiYin Recipe can also ameliorate the imbalance of the hypothalamic-pituitary-adrenal (HPA) axis. On the one hand, it can reduce the hormone level released by the hypothalamus. On the other hand, it can regulate the adrenal gland and peripheral immune organs, and effectively restore the normal hormone level (28).
Apart from regulating endocrine related levels, TCM also has a valid neuroprotective effect (29, 30). For one thing, bioactive compounds in TCM or TCM compound can reduce the synthetize of neurotoxic substances such as Aβ and Tau phosphorylation (31). For another thing, they can promote the clearance of toxic substances by increasing the toxic substance degradation enzyme, improving the blood-brain barrier function and promoting microglia phagocytosis (32, 33). TCM also reduces neuronal apoptosis and loss of neurons caused by excessive autophagy (34, 35). Beyond that, TCM can improve the pathways related to memory storage, and relieve the symptoms of memory loss by protecting the normal energy metabolism of the brain, restoring the structure and function of synapses and restoring neurotransmitter related neurotransmission (36, 37).
TCM can not only reduce memory loss of AD, but also treat mood disorders of AD, such as anxiety and depression, by regulating the stress-related endocrine axis (38). Studies have shown that acupuncture can also restore the circadian rhythm of the HPA axis well, and is an ideal TCM treatment for sleep disorders and emotional anxiety in AD (39). Some Chinese medicines can further regulate the intestinal flora of AD patients and relieve memory disorders through brain-gut axis (40).
2 Manuscript formatting
2.1 The relationship between neuroendocrine and Alzheimer’s disease
2.1.1 HPA axis and AD
Patients with AD often have HPA axis dysfunction. On the one hand, the release of glucocorticoid (GC), the main product of the HPA axis, promotes changes in metabolic processes in the body by increasing inflammatory response and oxidative stress (41). On the other hand, the brain is highly sensitive to stress, and anxiety enhances amygdala function, alters neural circuits, and leads to structural changes in the frontal lobe and hippocampus (42). These can all be reversed when the physiological level of corticosterone recovers to normal (43). Studies have shown that the elevated cortisol of the AD patient has diagnostic and prognostic value for AD (44).
The hippocampus and frontal lobe are important structures in the brain for learning and memory (45). When the brain is overloaded with glucocorticoids, hippocampal Rab35 (the enzyme required for Tau degradation by endlysosomal sorting) is down-regulated, and Aβ and highly phosphorylated Tau accumulate in large quantities (46). Accumulated toxic substances can eventually lead to cognitive loss by promoting inflammatory response, oxidative stress, energy metabolism disorders and memory related neuron atrophy and synaptic loss (47). GC in the brain is a key regulator of synaptic plasticity and microglial cell activity (48). Excessive release of GC under stress can lead to reduction of glucocorticoid receptor (GR) and down-regulation of its regulated anti-inflammatory effect, promoting neuroinflammation (49). These changes often occur before the onset of AD symptoms. Studies have shown that the HPA axis, circadian, and episodic memory are impaired in the early symptomatic stages of AD (50). Studies have also shown that the application of partial glucocorticoid receptor antagonists can improve neurotransmitter dysfunction and synaptic dysfunction, thus promoting memory storage (51, 52).
2.1.2 Cerebral insulin signal transduction and AD
The insulin signaling pathway itself as well plays an important role in memory and cognition. Insulin receptors(IRs) are widely distributed in the nervus centralis and parts of brain are able to secrete insulin themselves (53). Recent studies have shown that IRs play an important role in the significant links related to AD, including emotion, behavior, cognition, regulation of energy metabolism, regulation of neuronutrition and synaptic plasticity (54). A growing number of clinical studies have also shown that the longer the course of type 2 diabetes (T2D), the higher the risk of getting AD (55). Careful management of cholesterol and glucose from early adulthood can reduce the risk of AD (56). Improving glucose metabolism in MCI patients can reverse cognitive impairment and reduce the risk of developing dementia (57).
Activation of the cerebral insulin signaling pathway begins with the binding of ligand insulin to IR, and subsequent phosphorylation of IRS-1 affects PI3K/AKT signaling. PI3K/AKT signaling is regulated by hormones and connects to a variety of downstream AD-related effects by regulating a variety of transcription factors and cellular functions.PI3K/AKT signaling, which regulates many transcription factors such as CREB and NFκB, is associated with neuronal survival and inflammation. In addition, the following pathways are also involved: autophagy pathways like mTOR; apoptosis pathways like FoxO1, Bax, Bcl-2, JNK, ERK1/2; cerebral vasodilation factors like eNOS and NO. All of these effects can mediate neuron survival and synaptic plasticity, which is a popular pathway related to cognition in recent years (58). PI3K/AKT signaling is also related to pathways that mediate energy metabolism. Various factors that block PI3K/AKT signaling can lead to inactivation of GSK-3β, which further leads to tau phosphorylation and NFT formation. The activation of GSK-3β leads to CDK5 activation through regulation of P25. It is known that these two signal cascades are closely related to abnormal phosphorylation of Tau and play a main role in the pathological process of AD. Under normal circumstances, GSK3β inactivation causes Glut4 to be released from storage vesicles and move to the cell membrane. Hippocampal neurons increase glucose uptake and memory-related activities through this way (59). It has also been confirmed that when AD occurs, inflammation of the nervous system and activation of cellular stress can damage insulin signal transduction and result in brain BBB insulin resistance (60). Insulin resistance affects glucose metabolism and energy homeostasis in the brain, which constitute a vicious cycle and promote the pathological development of AD (61).
Some active factors associated with the insulin pathway are also involved in the development of AD. Glucagon-like peptide-1 (GLP-1) can be produced in the brain and acts like GSK-3β inhibitor (62, 63). GLP-1 activates the GLP-1 receptor signaling pathway and enhances hippocampus learning and memory, promoting neurogenesis, reducing inflammation and apoptosis by activating protein kinase A (PKA), phosphorylating Akt and CREB and reducing Aβ (64, 65). Decreased peripheral and cerebrospinal fluid IGF-1 levels may be a potential marker of cognitive decline and progression in AD (66). Insulin-degrading enzyme (IDE) has a great ability to degrade insulin and Aβ42 (67). IDE acts as an important regulator of Aβ clearance and diabetes, which is associated with neurodegeneration in AD (68).
2.1.3 HPG axis and AD
Being female is second only to advanced age as a risk factor for AD. AD affects more women than men, approaching 2:1 in many countries. Studies have shown that HPG axis changes are an important risk factor for AD. HPG axis hormones include Gonadotropinreception hormone(Gn RH), Luteinizing HRMone (LH), human Chorionic gonadotropin(h CG) and sex hormones. They are extensively involved in neuronal development, structure and brain function.
Studies in animal models and in patients with AD have shown that GnRH administration increases local estrogen levels, protects neural function from amyloid beta (Aβ) neurotoxicity, and prevents cognitive decline (69). Estrogen has been proved to have good neuroprotective effects and to prevent the pathologic development of Alzheimer’s disease (70). On one hand, estrogen has ideal neurotrophic effects. It can reduce neuroinflammation by inhibiting glial inflammatory activation, and thus reduce Aβ accumulation and pathological conformational changes of Tau to prevent memory disorders (71). On the other hand, estrogen, as a regulator of brain energy metabolism, restores normal glucose metabolism and brain mitochondrial function in AD, and significantly improves brain structural abnormalities and cognitive impairment (72, 73).
Recent studies have suggested that lifetime cumulative estrogen exposure may be related to the occurrence of AD. Although most of the brain is local synthetic estrogen, and the added exogenous estrogen therapy by factors such as age, family history, brain health, existing research shows that the menopausal women can improve cognitive function by taking supplementary estrogen. This suggests that individualized assessment is key to the successful prevention of AD in estrogen therapy (74). Similarly, androgens such as testosterone are regulated by the HPG axis. As the physiological level of testosterone decreases with aging in AD, androgen can prevent Aβ plaque formation and reduce Tau phosphorylation (75). The protective effect of testosterone has been proved to be related to the glycogen synthase pathway (76). In addition, LH has also been confirmed to be involved in APP metabolism and Aβ plaque formation in the hippocampus, and reducing LH can improve cognitive impairment (77, 78).
2.1.4 Brain gut axis and AD
The functions of the brain and gastrointestinal system are interlinked with each other. This bi-directional communication involves neuro-endocrine-immune changes and is strongly associated with the onset of AD (79). Studies have shown that improving intestinal flora can improve glucose tolerance, intestinal barrier dysfunction and dyslipidemia in AD model mice. This delayed brain pathological changes in AD model mice in many ways and relieved spatial learning and memory disorders, showing a bright prospect for treating AD (80).
Intestinal microbiome studies in patients with AD suggest that the unique microbiome changes in AD can be a predictor. Studies on the mechanism of AD induced by the microbiome - gut - brain axis changes suggest that intestinal microbes can create an inflammatory environment, promote protein misfolding, and cause inflammation to spread to the brain and cause pathological changes of AD (81). On the other hand, neuroinflammation can also affect the vagus nerve, resulting in intestinal dysbiosis (82). The microbiome - gut - brain axis is also an important link in the metabolism of substances in AD and can serve as an important link between glucolipid metabolism, insulin sensitivity, inflammation and the pathology of AD (83). Microbiome metabolism can also regulate the biosynthesis of neurotransmitters or their precursors, thus affecting the microbiome - gut - brain axis through the neuroendocrine pathway. Tryptophan metabolism regulates brain neurotransmitter signaling through the microbiome - gut - brain axis.
2.1.5 Interaction of neuroendocrine axes in AD
Recent studies have shown that imbalances in various neuroendocrine related systems, such as the HPA axis, HPG axis, insulin and brain-gut axis, can be observed in people with early stage AD, while related hormone therapy has been proved to achieve better results only in the early stages of aging (84, 85). This suggests that the intervention of AD through the neuroendocrine related axis needs to be carried out before the irreversible accumulation of neurotoxic substances, and there is still a large gap in the relevant early drug treatment in western medicine intervention.
During the process of AD, various neuroendocrine related axes interact and connect with each other through energy metabolism, oxidative stress and inflammation. There is a strong association between diurnal cortisol imbalance and insulin resistance (86). Glucocorticoids can mobilize liver nutrient metabolism and inhibit insulin secretion, and targeting stress-mediated glucocorticoid oversecretion is an effective way to restore the balance of insulin secretion (87). In the brain, hippocampal corticosteroid exposure promotes Tau phosphorylation by activating glycogen synthase kinase 3β (GSK3β), a pathway that intersects with insulin signaling (88, 89). Similarly, the HPG axis intersects with the HPA axis and insulin-related pathways. Estrogen inhibits 11β -hydroxysteroid dehydrogenase type 1, an enzyme involved in the synthesis of bioactive glucocorticoids from its inactive precursor. Estrogen-mediated neuroprotection also interconnects with the IGF-1 signaling pathway (90). Peripherally, either HPA axis imbalance, islet dysfunction or HPA axis imbalance can affect intestinal flora balance and promote chronic expression of peripheral inflammatory markers (91, 92) (Blue circle in Figure 1).
Brain-gut axis, peripheral-central immune communication or neuroendocrine related factors can act directly on the brain and alter the internal environment of the nervous system, leading to energy metabolism disorders, oxidative stress, and inflammation in nerve cells (93, 94). In this case, AD-related mitochondrial dysfunction, ER stress, and autophagy related pathways are activated (95). Amyloid precursor protein (APP) secretion increases, while Aβ-degrading enzymes such as enkephalin and insulin-degrading enzymes decrease. This contributes to the accumulation of toxic substances such as Aβ plaques and hyperphosphorylated Tau, resulting in the progression of AD pathology (Pink circle in Figure 1).
2.2 The neuroendocrine mechanism of TCM treating AD
2.2.1 The neuroendocrine mechanism of TCM regulating HPA axis
2.2.1.1 TCM alleviates the toxic effects of glucocorticoids
Many researches have proved that TCM can regulate the HPA axis, and different medicines can interfere with different sites of glucocorticoid action to improve cognition. Dawn saponin D (ASD) is a widely used TCM monomer that can resist AD, hyperlipidemia, diabetes and osteoporosis. Studies have shown that ASD can reduce the plasma corticosterone and ACTH levels in AD rats, improve memory deficits and anxiety symptoms. ASD shows a good brain protection effect by regulating the HPA axis hormone level (96). Astragaloside (AST) is the main active ingredient extracted from Chinese traditional herb Astragalus membranaceus. Studies have shown that AST can protect hippocampal nerves from glucocorticoid and Aβ25-35 injury, and improve learning and memory disorders. Its mechanism is related to the down-regulation of APP and β secretase mRNA levels. They subsequently caused the decrease of APP and Aβ expression in the hippocampus (97).
Mitochondrial regulation is also an important function of glucocorticoids (98). Chronic cellular stress will activate apoptosis signal transduction, which can promote Aβ production and lead to nerve cell death (99). Some TCMs have been confirmed to protect mitochondrial function and reduce nerve cell apoptosis by fighting ER stress. Ginsenoside Rd(Rd) can inhibit ACTH-induced corticosterone production by blocking the MC2R-cAMP/PKA/CREB pathway in adrenal cortical cells (100). Water extract of ginseng(WEG) can up-regulate the expression of GR and its related functional proteins HDAC6 and Hsp90 to restore mitochondrial function and reduce the succedent expression of ER stress-related proteins. This has followed with reductive nerve cell apoptosis and has shown treatment effects on anxiety and other mental disorders (101). The water extract of Sedum Takesimense (WEST) also has a similar effect (102) (Table 1 and Figure 2).
2.2.1.2 TCM regulates glucocorticoid receptors and related pathways
Glucocorticoid receptors (GR) in the hippocampus are important for memory formation (108). TCM can regulate toxic Aβ -producing enzymes to protect the brain with elevated stress hormones. They can also promote the production of brain-derived neurotrophic factors(BDNF), which promotes neuronal survival, through glucocorticoid-receptor (GR) related signaling pathways. Icaritin (ICA), the active component of Herba epimedium, has the properties of nerve cell regeneration and anti-apoptosis. Studies have shown that ICA promotes the expression of BDNF and Bcl-2 through GR, thereby inhibiting Bax and caspase-3 and reducing nerve cell apoptosis. ICA can also inhibit the release of lactate dehydrogenase (LDH) and promotes the activity of superoxidase dismutase (SOD), thereby reducing the level of oxidative stress in nerve cells. Its effect can be abolished by GR inhibitors (109). Puerarin (PU) has also been proved to be able to effectively improve learning and memory deficits in mice by promoting GR and BDNF gene expression in the hippocampus and induce neurogenesis (110). Existing studies have reduced the neurotoxic effects of glucocorticoids through selective inhibition of GR, but it has poor application prospects due to the wide distribution and diversity of glucocorticoid receptors. TCM can promote the recovery of normal pathways through the GR pathway. This may be related to the limited activation of the GR can promote cell survival, indicating that the treatment of AD regulated by TCM may be a promising means to reverse brain aging.
Reducing the Chronic activation of the HPA axis can maintain the normal operation of the neurotransmitter system and keep the structure and function of synapses in good condition. Dendrobium officinale alkaloid (DNLA) is an extract of Dendrobium officinale, which has been used in AD, anxiety and depression. DNLA can reduce neuron loss and increase Nissl bodies in the hippocampus CA2 and cortex by attenuating HPA activation and increasing GR expression. Beyond that, DNLA also has reduced the activity of monoamine neurotransmitters and metabolic enzymes elevated by CUS in the brain, and has alleviated the anxiety and depression-like behavior of rats under chronic stress (104). Yokukansan is also a traditional herbal medicine that can counteract the effects of corticosterone, reverse its cytotoxicity to hippocampal neurons and increase neuronal survival in chronic stress rats (111). Its effect of anti-anxiety and alleviation of aggressive symptoms may be mediated by 5-HT receptors (105). Glutamatergic neurological dysfunction is an important link in the pathology of AD, and glutamate receptor is related to nerve excitability. Accumulation of toxic substances in AD will damage receptor function, resulting in calcium imbalance and excitability toxicity. In the end, these will cause autophagy and apoptosis of neurons (103). Tenuifolin (TEN), the main component of Polygala tenuifolia, has neuroprotective performance. TEN can up-regulate the expressions of glucocorticoid receptor, glutamate receptor 1 and synaptic related proteins (107).
Some TCMs can also reduce stress-related inflammation, showing a good effect against inflammatory damage. TEN can reduce serum adrenocorticotropic hormone and corticosterone levels, inhibits Toll-like receptor 4(TLR4)/NFκB mediated inflammation, and regulates the levels of IL-6 and IL-10 in the hippocampus. TEN can also promote the increase of brain-derived neurotrophic factor and myosin kinase B, showing great improvement effect on memory loss caused by chronic stress (107). Ginsenoside Rg1 (Rg1) is the active ingredient in ginseng. Rg1 can protect cerebrovascular endothelium and increases neuronal survival through the GR-ERK signaling pathway, indicating its possible anti-glucocorticoid injury effect (112). In addition, Rg1 decreased NLRP1 inflammasome and ASC and reduced the expression of apoptosis-related factors by activating GR, showing a good cognitive protective effect (113).
It is worth mentioning that in the pathological development of AD, HPA axis and insulin-related pathway show extensive cross-influence. Some Traditional Chinese medicines regulate these cellular endocrinology by affecting their overlapping downstream pathways. JiAmarogentin (AMA) is a dicyclic glycoside isolated from Gentiana rigescens Franch, which has similar effects of nerve growth factor (NGF). Studies have shown that AMA may reduce neuroinflammation and nerve apoptosis through Ras/Raf/ERK and PI3K/AKT signaling pathways regulated by insulin. Interestingly, GR/PLC/PKC signaling pathway has also been found to be involved in the neurogenic effects of AMA. This has indicated possible interactions between GR and insulin-related pathways (114). 5,6,7,4 ‘-tetramethoxylflavanone (TMF) is one of the active ingredients in Chromolaena odorata (l.),and it can reduce BACE1 and PS1 expression to reduce toxic production and synaptic protection. In dexamethasone(DEX) model mice, TMF promoted the expression of proteins associated with neurogenesis, proliferation, differentiation, and maturation through a mechanism similar to AMA (115). Studies have shown that quercetin also decreased serum corticosterone and serum insulin in mice, and increased the expression of GLUT4 in neurons. This indicates its potential to promote neuronal survival by improving energy metabolism, which happens to be an important part of the co-regulation of HPA axis and insulin (116). These studies suggest that TCM intervention in the tandem between glycoskin and insulin pathways may be a better medical option for endocrine-related cognitive risks than mono-acting drugs. Liuwei Dihuang decoction (LW) is a classical TCM prescription, which has potential therapeutic effect on AD. The addition of LW-AFC restores the balance of HPA and HPG axis, enhances the proliferation of spleen cells and corrects the disorder of lymphocyte subsets. It can also regulate abnormal cytokine production in SAMP8 mice, showing superior neuroendocrine immune network regulation over memantine and Donepezil (117) (Table 1).
2.2.2 The neuroendocrine mechanism of TCM regulating insulin-related pathways
2.2.2.1 TCM promotes neuronal survival through insulin signaling pathway and related downstream pathways
TCM can reduce oxidative stress. Insulin signaling pathway is related to oxidative stress pathways. TCM can reduce oxidative stress by regulating insulin signaling pathway and activating antioxidant downstream pathways, thus reducing the accumulation of toxic substances and promoting neuronal survival (Figure 2). Studies have shown that Lychee seed and Astragalus Membranaceus have beneficial effects on regulating blood glucose, lipids, and anti-oxidation. They can alleviate insulin resistance and oxidative stress in the brain, and show sufficient cognitive benefits (118, 119). Xuefu Zhuyu decoction (XZD) is a tried and tested TCM formula for treating metabolic syndrome, cardiovascular and cerebrovascular diseases. XZD can reduce body weight, insulin resistance, and leptin levels in APP/PS1 mice, thereby improving neuroinflammation and AD-related pathology, demonstrating favorable neuroendocrine regulatory effects (120). In AD patients, insulin receptor substrate 1 (IRS-1) deficiency down-regulates Nrf2/HO-1 signaling, thereby increasing oxidative stress in the brain and ultimately causing nerve cell damage. Thymol is a monoterpene phenol isolated from herb, which has strong neuroprotective effect. Thymol has shown beneficial effects on high fat diet induced cognitive deficits by improving insulin resistance in the hippocampus and activating Nrf2/HO-1 signaling (121). Peganum Harmala (P. Harmala) enhanced Nrf2 through insulin signaling, while reducing lipid peroxidation and adding glutathione (122). Dianxianning (DXN) is a traditional Chinese formula that has been reported to have anti-Alzheimer’s disease activity. Studies have shown that Aβ -induced pathologic features are improved by the insulin-like pathway in transgenic worms treated by DXN (123) (Table 2 and Figure 2).
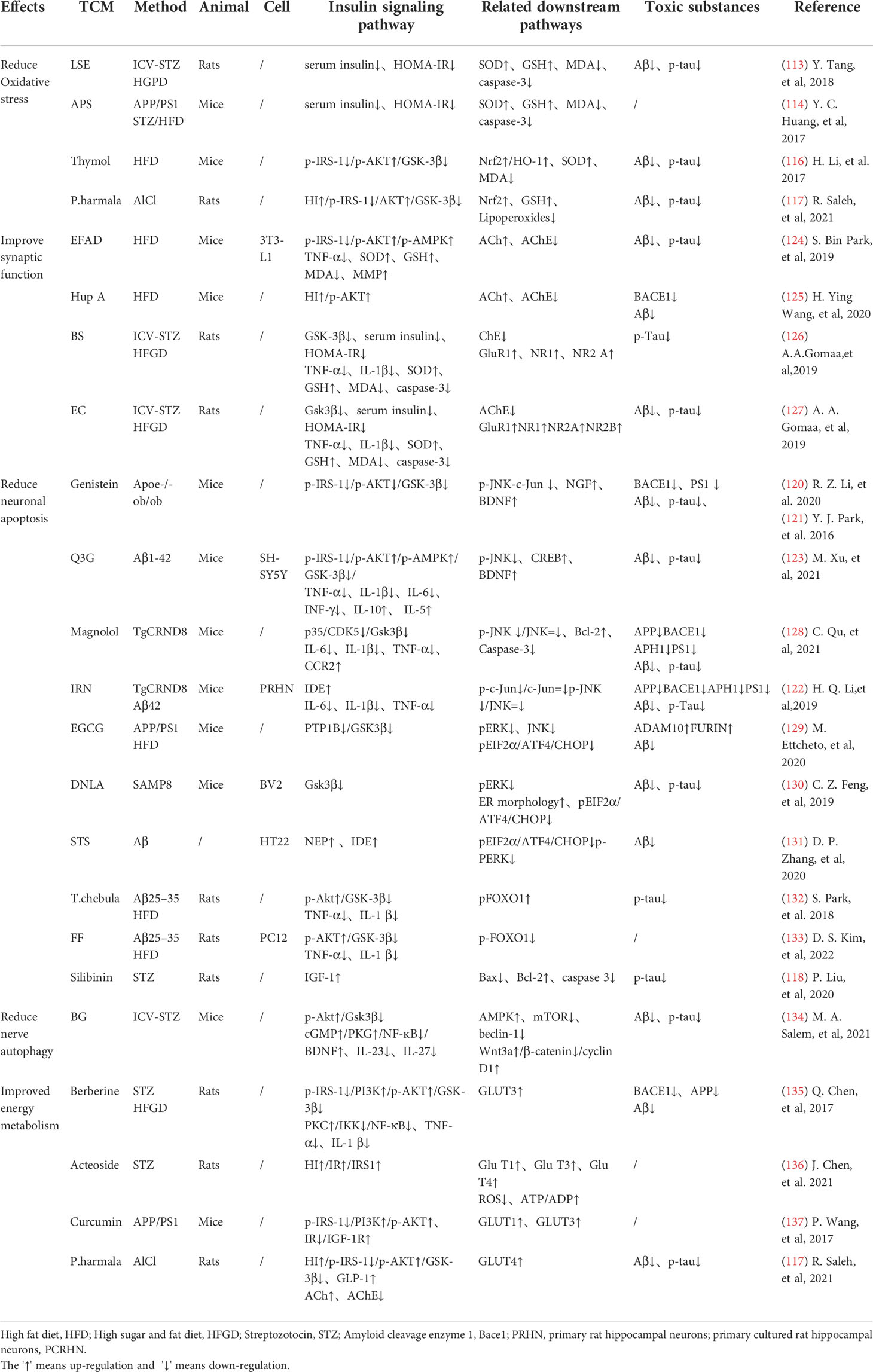
Table 2 TCM promotes neuronal survival through insulin signaling pathway and related downstream pathways.
TCM can reduce neuronal apoptosis. Neuronal apoptosis is an important part of AD pathology. Neuroinflammation can activate insulin signaling pathway and promote the initiation of apoptosis process. TCM can simultaneously intervene the insulin signaling pathway and its downstream endocrine mechanism about apoptosis, showing a good prospect in the treatment of AD. Silibinin, a polyphenolic flavonoid extracted from silythistle seeds, can significantly inhibit streptozotocin(STZ)-induced neuronal apoptosis, up-regulate insulin signal transduction pathway, and reduce the morphological and structural damage of hippocamal neurons (128). JNK/c-Jun pathway promotes pro-apoptotic protein transcription in AD brain neurons. Treatment with Genistein can increase insulin sensitivity and the expression levels of the neurotrophic factors nerve growth factor (NGF) and brain-derived neurotrophic factor (BDNF) (138). Genistein can also reduce c-Jun N-terminal kinase(JNK) activity and alleviated AD-related pathology in ApoE knockout high fat diet mice (133). Isorhynchophylline (IRN) can inhibit JNK signaling in primary hippocampal neurons treated with Aβ, reducing Aβ production and deposition, and Tau hyperphosphorylation (139). Quercetin-3-o-glucuronide (Q3G), a flavonol Glucuronide, also has similar down-regulation of JNK/c-Jun pathway. In addition, Q3G can also regulate intestinal microbiome ecological imbalance, alleviates cerebral insulin resistance and improves cognitive dysfunction through intestinal brain axis (132). Magnolol also has been proved to have a similar effect on reducing apoptosis and regulating intestinal microbes (140). FoxO1 regulates the transcriptional activity of apoptotic proteins in the nucleus. Studies have also shown that FoxO1 overexpression promotes GSK-3β activity and ERK activity by increasing phosphorylation of GSK-3β (S9), and participates in the process of Tau phosphorylation (131). Forsythia fruit (FF) is a Chinese medicine widely used in treating inflammation. Studies have shown that FF regulates insulin-related pathways and reduces neuronal apoptosis and injury by decreasing FoxO1 activity, thereby improving cognition in rats (129). Luteolin also has a similar effect (130, 141).Endoplasmic reticulum(ER) stress is a major factor of AD nerve apoptosis. ERK activation increases mitochondrial superoxide production and impairs mitochondrial homeostasis. This induces ER stress through the pERK-eIF2α-ATF4-CHOP axis, ultimately leading to neuronal apoptosis (134). ATF4 can guide the transcription of autophagy genes such as Beclin-1, CHOP. The upregulation of CHOP transcription factors is related to neuronal degeneration. The inhibition of insulin signaling pathway caused by increased PTP1B protein is also related to the activation of this pathway. Sodium tanshinone IIA sulfonate (STS) can reduce the level of oxidative stress in SH-SY5Y cells, and reduce ER stress levels by decreasing pERK-eIF2α-ATF4-CHOP axis activation. By protecting ER structure and function, STS has provided cognitive protection (142). Dendrobium officinale can also reduce endoplasmic reticulum stress and Aβ production through a similar pathway (135). EGCG can increase PTP1B protein and reduces activation of this pathway (143) (Table 2 and Figure 2).
TCM can reduce excessive autophagy. Excessive autophagy of nerve cells is a pathogenesis of AD, and the Beclin-1 complex is indispensable in the formation of autophagosome (144). Bergapten (BG) is a TCM with neuroprotective potential. Studies have shown that BG regulates the insulin pathway by stimulating Wnt3a, reduces the activity of GSK-3β, and increases the expression of BDNF. In addition, BG reduces autophagy of nerve cells through the AMPK/mTOR/Beclin-1 pathway, reverses intracerebellar (ICV) STZ injection-induced cognitive impairment, and alleviates AD related pathological manifestations. Huang-Lian-Jie-Du formulae (HLJDD) is a traditional Chinese formula, which has been used in diabetes and Alzheimer’s disease for a long time. HLJDD has been shown to take neuroprotective effects through regulation of glucose and lipid metabolism, up-regulation of autophagy and inhibition of NLRP3 inflammasome signaling pathways (145) (Table 2).
TCM can improved energy metabolism. The Glucose transporter protein (GLUTs) family consists of a large group of membrane proteins that transport glucose along a concentration gradient without energy expenditure. GLUT1 and GLUT3 are widely expressed in the central nervous system and are responsible for most glucose uptake and utilization in the brain. Decreased glucose metabolism and energy deficiency associated with GLUTs were observed in AD pathology, which was associated with high Tau phosphorylation and oligomer Aβ production (146). Insulin signaling pathway is closely related to glucose metabolism, and TCM induces GLUTs expression through insulin signaling pathway, alleviates the accumulation of toxic substances induced by energy metabolism disorders, and protects neurotransmitters. Memory impairment has been proved to be related to glucose uptake or metabolism in the medial prefrontal cortex. Berberine can cross the blood-brain barrier and up-regulate GLUT3, promote glucose uptake in the brain, and reduce the expression of amyloid precursor protein and BACE-1, as well as oligomeric Aβ42 production (136, 147, 148). Its cognitive improvement benefit may also be related to the activation of PI3K/Akt/mTOR and MAPK signaling pathways (137, 149). Acteoside can ameliorate STZ-induced oxidative stress and learning and memory impairment caused by GLUT1, GLUT3, and GLUT4 pathways and regulate intracranial metabolism (150). Curcumin has antioxidant and anti-inflammatory properties and can reduce amyloid pathology in AD. Curcumin can increase glucose levels in plasma and brain, and significantly increase GLUT3 and GLUT4 levels (151). Micropositron emission tomography (PET) has shown that curcumin treatment improved cerebral glucose uptake in AD mice (124, 125). Banxia Xiexin decoction (BXD) treatment can not only restore insulin signal transduction, but also increase the expression of glucose transporter 1 (GLUT1) and GLUT3 levels, which had a good effect on the improvement of cognitive ability, synaptic volume and ultrastructure of AD (126) (Table 2).
TCM can protect synaptic function. Synaptic loss caused by inflammation and oxidative stress in AD is a major factor related to the progression of the disease. Loss of cholinergic neurons leads to memory and attention deficits, and neurotransmission of glutamate is crucial for synaptic plasticity and neuronal survival (127). TCM can improve chronic stress induced neuroendocrine, neuromicroenvironment, synaptic structure and function disorders through insulin signaling pathway, and relieve patients’ memory loss. Aruncus Dioicus var. kamtschaticus (EFAD) and Huperzine A (Hup A) are both extracts of TCM, which can improve cerebral insulin resistance and cognitive impairment in AD animals. Both of them can increase Ach and decrease AchE and improve synaptic function (152, 153). Polyphenol rich frankincense(BS) gum has been proven to have anti-inflammatory, anticancer and anti-apoptotic effects. BS extracts can significantly enhance glutamate receptor expression (GluR, NR1, NR2 A and NR2B) and reduced Aβ deposition and Tau phosphorylation (154). Cardamom extract (EC) has anti-diabetic, antioxidant and anti-inflammatory properties. In addition to improving cognitive function in rats, EC can also increase the expression of suppressed glutamate receptors (AMPA GluR1 subunit and NMDA receptor subunit NR1, NR2A, NR2B) (155) (Table 2).
2.2.2.2 TCM regulates the generation and metabolism of insulin-related enzymes and toxic substances
In the transmission of insulin signaling pathway, the activation of GSK-3β leads to CDK5 activation through the regulation of P25, and both of these signaling cascades are closely related to the abnormal phosphorylation of Tau and play a key role in the pathological process of AD. The total alkaloids from Coptis chinensis Franch (ACF) are widely used in traditional Chinese medicine to treat diabetes and dementia. ACF treatment can significantly increase pIRS, PI3K, and pAkt, restrain GSK3β overactivation, thus reducing Aβ deposition (156). Catechins, proanthocyanidins A1, A2 in Lychee seed and Huperzine A can inhibit hyperphosphorylated Tau through A similar pathway (153, 157). Ginseng has many active components against AD. Pseudoginsenoside-f11 (PF11) is a root and leaf extract of ginseng subspecies. It can ameliorate STZ-induced learning and memory deficits and reduce neuronal loss by regulating insulin signaling and calproteinase I/CDK5 signaling pathways in the hippocampus and thus reducing phosphorylated Tau (158). Dendrobium officinale and ginsenoside Rb1(Rb1) have similar effects (143). CDK5 mediates phosphorylation and inactivation of PPARγ, which has antioxidant and neuroprotective effects (140). Ginsenoside Rg1(Rg1) is one of the main components of ginseng. Studies have shown that Ginsenoside Rg1 can act like CDK5 inhibitor and inhibit PPARγ phosphorylation, thus down-regulating BACE1 and APP expression (159). Notoginsenoside R1 (NTR1), the main active ingredient of ginseng, a well-known traditional Chinese herb, can induce increased levels of peroxisome proliferator-activated receptor γ (PPARγ) and decreases Aβ production (160) (Figure 2).
Overexpression of PTP1B negatively regulates insulin signaling by binding to specific phosphorylation residues of IRS-1 (161). Researches have shown that it is also possible that PTP1B itself regulates central nervous system processes associated with neurological diseases. Ferulic Acid(FA) is a phenolic compound can commonly found in a series of plants. Through reducing PTP1B, Ferulic Acid can promote insulin signal transmission, improve spatial memory of middle diabetic rats, increase hippocampal capillary density and reduce aAD-like pathological changes in hippocampus (162, 163) (Table 3 and Figure 2).
Insulin-degrading enzyme(IDE) and enkephalinase(NEP) have strong ability to degrade insulin and Aβ42, which are associated with neurodegeneration in Alzheimer’s disease (132). EGCG can inhibit NEP expression in astrocytes by activating extracellular signal-regulated kinase (ERK) and phosphoinositol 3-kinase (PI3K) (38, 165). This has promoted Aβ degradation (166). Paeoniflorin (PF) significantly inhibited NO production and secretion of IL-6, IL-1β and tumor necrosis factor-α(TNF-α) in glial cells by up-regulating the NF-κB pathway and the activity of Aβ -degrading enzymes such as IDE and NEP (164). Isorhynchophylline (IRN) has been shown to have significant anti-Alzheimer’s disease activity. In addition to increasing IDE expression, Isorhynchophylline can also inhibit the activation of microglia and astrocytes, reduce tau hyperphosphorylation and neuroinflammation, and improve cognitive impairment, which has a good potential for further development into drug therapy for AD (139). Sarsasapogenin-AA13 can convert pro-inflammatory M1 microglia into anti-inflammatory M2 microglia and increase the expression of IDE and NEP, thus reducing toxic substance deposition (167). A new kind of pectin RP02-1 extracted from the roots of Polygala Tenuifolia, pectin LBP1C-2 purified from the fruits of Lycium berries, Notoginseng Saponin Rg1(Rg1), Rb1 and ginsenoside F1 can up-regulate the expression of Aβ -degrading enzyme in a similar way (106, 152, 168–171) (Table 4). LBP1C-2 and Rg1can also decrease the expression of APP, BACE1 and PS1 and increase the expression of α secretase(ADAM10) (172). This leads to the reduction in the APP processing and effectively saves cognitive impairment and neuronal loss (173). Cornel Iridoid Glycoside (CIG) is an active ingredient from cornus officinalis that can also increase ADAM10, NEP and IDE levels in the brain of AD model mice. CIG can also increase the expressions of NGF, BDNF and phosphorylated camp-reactive element binding protein (p-CREB) in the brain of 3×Tg mice, alleviating plaque deposition and cognitive impairment (174). Kai Xin San, a Chinese Herbal formula composed of Radix Ginseng, Poria, Radix Polygalae and Acorus Tatarinowii Rhizome, can induce IDE and result in at least partial remission of hippocampal neuronal injury in rats (175) (Table 4).
2.2.3 The neuroendocrine mechanism of TCM regulating HPG axis
Estrogen treatment has been proved to have potential nerve protective effect. But except the nervous system, it may endanger the cardiovascular system and other system. In recent years, some traditional Chinese medicines have been proved to be rich in phytoestrogens, which have a natural similarity with estradiol structure. They can selectively activate estrogen receptors and activate specific neuroprotective effects such as anti-inflammatory, antioxidant and anti-apoptosis, and can be used in AD treatment.
Chinese medicine can reduce toxic substances by activating estrogen receptors. Curcumin can selectively activate estrogen receptor β (Erβ), inhibit IκBα degradation, and reduce BACE1 expression and Aβ level through the NFκB pathway (176). Calycosin, a typical phytoestrogen derived from astragalus membranaceus, binds with estrogen receptors to activate the protein kinase C pathway, reducing oxidative stress and inflammatory response, thereby improving the deposition of toxic substance such as amyloid beta and Tau in the hippocampus of APP/PS1 transgenic mice, and has a good cognitive improvement effect (177).
Some estrogen receptor selective activators have shown great anti-apoptotic effects and can protect nerve cells and synaptic functions. Silibinin, a flavonoid phytoestrogen derived from milk thistle, can improve cognition in AD rats by inhibiting the PI3K-Akt pathway and is a potential drug candidate for the treatment of Alzheimer’s disease (178). Erzhi pills, a classical Chinese medicine prescription that can up-regulate estrogen levels, can also increase the number of Erβ receptors, reduce nerve cell apoptosis and relieve AD pathology by the PI3K-Akt pathway similar to Silibinin (179). Kaempferol can protect PC-12 cells from the apoptotic process by activating estrogen receptors through the ER/ERK-related MAPK signaling pathway (180). Guanghopin alcohol (PTA) is a selective ERβ agonist that can improve oxidative stress and synaptic integrity by enhancing BDNF/TrkB/CREB signaling, and has shown a good neuroprotective effect (181). Interestingly, the quercetin, luteolin, and EGCG mentioned above also have phytoestrogenic properties, which will not be described further in this section due to their similar effects.
In addition, phytoestrogens can also protect endoplasmic reticulum and mitochondrial functions in AD model, showing a good regulation of cell metabolism. Resveratrol (Res) can increase estrogen levels and antioxidant capacity in AD models through the Nrf2/HO-1 signaling pathway, showing a role in regulating mitochondrial function (182). Biochanin A (BCA) is a phytoestrogens isolated from Pratense L. clover that has been commonly used to relieve postmenopausal problems in women. BCA can increase the expression of mitochondrial stability and morphology-related proteins like phosphorylated Drp1, OPA1 and Mfn2, as well as the expression of mitochondrial autophagy related proteins Beclin1, LC3B, Pink1 and Parkin. This shows that BCA can rescue mitochondrial abnormalities, thereby restoring cognitive decline and reducing Aβ deposition and BACE1 expression in oophorectomized APP/PS1 mice (183). Both phytoestrogens α -Zearalanol (α -Zal) and estrogens can effectively reduce the death of AD-like apoptotic neurons, but the side effects of α -ZAl on breast and endometrial tissues are significantly less than that of estrogens. α -ZAl has been proved to have the potential to stabilize ER function by reducing intracellular calcium overload, showing at least partial effects of neuronprotective effect against AD-like apoptosis (184), (Figure 3).
There are also studies shows that the activation of estrogen receptors can promote the expression of neurotransmitters and receptors and improve the neurotransmission function. Genistein is a neuroprotective phytoestrogens that can activate estrogen receptor subtypes, regulate NR2B and GLUTamate receptor subunit GluR2 (185), (Table 5).
2.2.4 The neuroendocrine mechanism of TCM regulating brain gut axis correlation
Most TCM treatments need to be taken orally. Recent studies have shown that some TCM can play an ideal role in the treatment of AD by affecting neuroendocrine factors related to brain-gut axis, such as pro-inflammatory factors, neurotransmitters and active metabolites. Some other TCM require intestinal conversion to become active forms for their neuroprotective effects (186). Some studies have shown that acupuncture can also affect intestinal flora, regulate levels of anti-inflammatory factors, and improve cognition in AD model animals (187). In patients with mild to moderate AD, the combination of abdominal acupoint catenet embedding therapy and Donepezil hydrochloride tablets was superior to monotherapy (188). However, the related mechanism is still unclear and needs further exploration.
Chinese medicine regulates intestinal flora and plays a neuroprotective role by improving inflammatory environment. Gastrodin (Gas) can reduce LPS and pro-inflammatory cytokines in the brain of AD model animals and improve memory by interfering with some intestinal microbiota (189). A novel selenium peptide (SE-PS) with neuroprotective effects obtained from Cordyceps militaris has shown significant protective effects on LPS-induced colon and brain inflammation and oxidative stress and has reduced cognitive impairment in mice by inhibiting the production of pro-inflammatory mediators and malondialdehyde, as well as promoting anti-inflammatory cytokines and the activity of antioxidant enzymes (190). Qisheng Wan Formula (QWF) has been widely used since ancient times to treat patients with amnesia or dementia. QWF has also been proved to improve hippocampal structure in AD rats by inhibiting pro-inflammatory factors and regulating gut microbiota (191).
TCM can regulate the metabolites derived from intestinal microorganisms, improve the metabolism of neurotransmitters and play a neuroprotective role. Xanthoceraside (XAN) is derived from the shell of Xanthoceras sorbifolia Bunge and has anti-AD activity. Although XAN is hardly absorbed by the BBB, it can significantly regulate metabolic disorders including neurotransmitter, amino acid, bile acid and SCFAs metabolism directly or indirectly induced by Aβ1-42 in the intestinal tract. In this way, XAN improves learning and memory deficits in AD rats (192). Similarly, fructose-oligosaccharides from Morinda Officinalis (OMO) can not only maintain the diversity and stability of the gut microbiome, but also have a similar effect in regulating neurotransmitters (193). Through metabonomics correlation analysis, it is beneficial for us to understand the mechanism of action of TCM by studying metabolic pathways related to metabolites derived from intestinal microorganisms. However, specific mechanisms need to be further explored, and relevant studies have been listed in Table 6.
2.3 Discussion
With the development of modern society, there are more and more sporadic AD patients, many of whom have neuroendocrine related dementia risk factors. Existing western medicine treatment methods for AD mostly focus on a single target to improve neurological function, but due to the complex etiology of AD, a single treatment method often cannot play an ideal role, and some drugs will produce dangerous side effects, which is not well accepted by patients. TCM has unique advantages in treating AD. First of all, as endocrine is A complex organic whole, it is sometimes difficult for drugs with A single target to take into account multiple causes. TCM can simultaneously take into account multiple pathologies of AD in the nervous system and peripheral organs through multi-target action, such as regulating the aggregation of Aβ and pTau in the whole process from generation to metabolism. Regulates inflammation and oxidative stress associated with central and peripheral insulin resistance; Regulate intestinal flora to reduce systemic inflammation, improve the internal environment of the nervous system from the root, and treat AD. Secondly, the use of natural ingredients in TCM have been proved to be safer. Currently western medicine such as Donepezil, lisdamine, galantamine and memantine, are commonly used in AD patients and often have side effects such as headache, dizziness, weight loss, abnormal blood pressure and confusion. None of them are recommended for the prevention of cognitive decline in early stage patients. Many TCM prescriptions, including Liuwei Dihuang decoction, Xuefu Zhuyu decoction and many other recipes mentioned above, have been applied for thousands of years in China. With the development of modern medicine, more evidence-based medical studies have shown satisfying safety and therapeutic benefits of these herbs (199, 200). In view of the objective factors such as the mixture of TCM components in traditional medical treatment, in recent years, researchers have tried to clarify the mechanism of TCM by isolating the active ingredients in formulations or natural Chinese medicinal materials and conducting cell or animal experiments (201). Relevant studies have shown that TCM is an option for patients with AD-related risk factors and mild cognitive decline (202). Some clinical studies have also shown that the combination of TCM and western medicine can have long-term effects on patients with mild cognitive impairment, but larger clinical studies are still needed to enhance the level of evidence (203, 204). Finally, for patients in the early stage of cognitive deficit, timely reduction of various risk factors to promote neurological compensation can greatly improve the quality of life of patients in the later stage. There is a gap in western medicine in this aspect, and Chinese medicine can play a good supplement in this aspect.
In the process of data collection, it was found that some active ingredients of Traditional Chinese medicines such as ginseng, dendrobium, EGCG, silibinin, berberine, curcumin, genistein and so on can regulate various neuroendocrine pathways. This suggests that these drugs have multiple targets in AD, making them more powerful drugs. However, the majority of these studies can not fully explain whether the effects of these drugs on hormone receptors can fully cover their effects, and further experiments are needed. We also found that TCM prescriptions often regulate a variety of neuroendocrine pathways, but the related mechanisms are rarely studied. It was also found that these neuroendocrine related axes often affect mitochondria and endoplasmic reticulum related functions to promote AD susceptibility. More effective drugs and treatments can be found by screening TCM treatments that affect the above related pathways. At present, most of the relevant mechanisms of TCM regulation of the neuroendocrine axis are still not in-depth, such as immune regulation mechanism, metabolism mechanism and other studies need to be further supplemented. Since the neuroendocrine disorders of AD patients vary from person to person, exploring the unique effects of TCMs can provide a basis for personalized treatment.
At present, there are still many deficiencies in the research on the neuroendocrine therapy of AD regulated by TCM. The research on the endocrine regulation mechanism of TCM is still at the surface phenomenon, lacking in-depth mechanism discussion. This is partly due to the use of natural products in TCM, which has the promiscuous characteristics. On the other hand, due to the complexity of endocrine system itself, further research is needed. Due to the differences between animal endocrine physiology and human neuroendocrine physiology, human neuroendocrine physiology has unique characteristics. Although existing animal research models can represent certain characteristics of AD to a certain extent, for sporadic AD with mixed etiology, a model closer to the actual situation still needs to be developed. Although TCM has been used in China and other Asian countries for thousands of years, in the era of modern medicine, more standardized and larger scale clinical studies are necessary to establish widely accepted and recommended TCM treatment plans for dementia.
Author contributions
CD, HC, and ZM have contributed equally to this work and share first authorship. All authors contributed to the article and approved the submitted version.
Funding
This work was supported by Project of COVID-19 Emergency Response Project of Shanghai Sixth People’s Hospital in 2022(ynxg202218), Project of Shanghai Science and Technology Commission (19401970600) and Project of Shanghai Science and Technology Commission (19401932500), and Shanghai will further accelerate the 3-year action plan for the development of TCM (2018–2020) for major clinical research on TCM (ZY (2018–2020)-CCCX-4010), the Innovation Fund of Integrated Traditional Chinese and Western Medicine, School of Medicine, Shanghai Jiao Tong University (18zxy002), the 2019 Teacher Training and Development Project of Medical School of Shanghai Jiao Tong University (JFXM201909), and the Experimental Project of Scientific and Technological Innovation for College Students of Heilongjiang University of Traditional Chinese Medicine (16041200019).
Conflict of interest
The authors declare that the research was conducted in the absence of any commercial or financial relationships that could be construed as a potential conflict of interest.
Publisher’s note
All claims expressed in this article are solely those of the authors and do not necessarily represent those of their affiliated organizations, or those of the publisher, the editors and the reviewers. Any product that may be evaluated in this article, or claim that may be made by its manufacturer, is not guaranteed or endorsed by the publisher.
References
1. Marasco RA. Economic burden of Alzheimer disease and managed care considerations. Am J Manag Care (2020) 26:S171–83. doi: 10.37765/AJMC.2020.88482
2. Tahami Monfared AA, Byrnes MJ, White LA, Zhang Q. The humanistic and economic burden of alzheimer’s disease. Neurol Ther (2022) 11:525–51. doi: 10.1007/s40120-022-00335-x
3. 2022 alzheimer’s disease facts and figures. Alzheimers Dement (2022) 18:327–406. doi: 10.1002/ALZ.12638
4. Albert MS, DeKosky ST, Dickson D, Dubois B, Feldman HH, Fox NC, et al. The diagnosis of mild cognitive impairment due to alzheimer’s disease: Recommendations from the national institute on aging-alzheimer’s association workgroups on diagnostic guidelines for alzheimer’s disease. Alzheimer’s Dement (2011) 7:270–9. doi: 10.1016/J.JALZ.2011.03.008
5. Tahami Monfared AA, Byrnes MJ, White LA, Zhang Q. Alzheimer’s disease: Epidemiology and clinical progression. Neurol Ther (2022) 11:553–69. doi: 10.1007/s40120-022-00338-8
6. Kellar D, Register T, Lockhart SN, Aisen P, Raman R, Rissman RA, et al. Intranasal insulin modulates cerebrospinal fluid markers of neuroinflammation in mild cognitive impairment and alzheimer’s disease: a randomized trial. Sci Rep (2022) 12:1–11. doi: 10.1038/s41598-022-05165-3
7. Siafarikas N, Kirsebom BE, Srivastava DP, Eriksson CM, Auning E, Hessen E, et al. Cerebrospinal fluid markers for synaptic function and Alzheimer type changes in late life depression. Sci Rep (2021) 11:1–9. doi: 10.1038/s41598-021-99794-9
8. van Loenhoud AC, Groot C, Bocancea DI, Barkhof F, Teunissen C, Scheltens P, et al. Association of education and intracranial volume with cognitive trajectories and mortality rates across the Alzheimer disease continuum. Neurology (2022) 98:e1679–91. doi: 10.1212/WNL.0000000000200116
9. Angoff R, Himali JJ, Maillard P, Aparicio HJ, Vasan RS, Seshadri S, et al. Relations of metabolic health and obesity to brain aging in young to middle-aged adults. J Am Heart Assoc (2022) 11:e022107. doi: 10.1161/JAHA.121.022107
10. Duong MT, Das SR, Lyu X, Xie L, Richardson H, Xie SX, et al. Dissociation of tau pathology and neuronal hypometabolism within the ATN framework of alzheimer’s disease. Nat Commun (2022) 13:1495. doi: 10.1038/S41467-022-28941-1
11. Allen AP, Curran EA, Duggan Á, Cryan JF, Chorcoráin AN, Dinan TG, et al. A systematic review of the psychobiological burden of informal caregiving for patients with dementia: Focus on cognitive and biological markers of chronic stress. Neurosci Biobehav Rev (2017) 73:123–64. doi: 10.1016/J.NEUBIOREV.2016.12.006
12. Huang J, Xie W, Yun C, Danli Z, Xiaoqing L, Yufan Z, et al. From the perspective of hypothalamic-pituitary-adrenal axsis to explore the neural mechanism of post-stroke aphasia under the guidance of TCM"brain-kidney-sui"theory. Glob Tradit Chin Med (2022) 15:786–91.
13. Lijuan S, Yonglin L, Hongyan W. Treatment of alzheimer’s disease from the five zang viscera. J Pract Tradit Chin Intern Med (2020) 34:12–4. doi: 10.13729/j.issn.1671-7813.z20190962
14. Geng X, Wu H, Li Z, Li C, Chen D, Zong J, et al. Jie-Yu-He-Huan capsule ameliorates anxiety-like behaviours in rats exposed to chronic restraint stress via the cAMP/PKA/CREB/BDNF signalling pathway. Oxid Med Cell Longev (2021) 2021:1703981. doi: 10.1155/2021/1703981
15. Qin G, Wang Y, Liu Z, Mana L, Huang S, Wang P. Shenzhiling oral solution promotes myelin repair through PI3K/Akt-mTOR pathway in STZ-induced SAD mice. 3 Biotech (2021) 11:361. doi: 10.1007/S13205-021-02900-X
16. Mana L, Feng H, Dong Y, Wang Y, Shi J, Tian J, et al. Effect of Chinese herbal compound GAPT on the early brain glucose metabolism of APP/PS1 transgenic mice. Int J Immunopathol Pharmacol (2019) 33:2058738419841482. doi: 10.1177/2058738419841482
17. Patel R, Kaur K, Singh S. Protective effect of andrographolide against STZ induced alzheimer’s disease in experimental rats: possible neuromodulation and aβ (1-42) analysis. Inflammopharmacology (2021) 29:1157–68. doi: 10.1007/S10787-021-00843-6
18. Ma X, Song M, Yan Y, Ren G, Hou J, Qin G, et al. Albiflorin alleviates cognitive dysfunction in STZ-induced rats. Aging (Albany NY) (2021) 13:18287–97. doi: 10.18632/AGING.203274
19. Qi G, Mi Y, Fan R, Li R, Liu Z, Liu X. Nobiletin protects against systemic inflammation-stimulated memory impairment via MAPK and NF-κB signaling pathways. J Agric Food Chem (2019) 67:5122–34. doi: 10.1021/ACS.JAFC.9B00133
20. Chen T, Shou L, Guo X, Wei M, Zheng H, Tao T. Magnolol attenuates the locomotor impairment, cognitive deficit, and neuroinflammation in alzheimer’s disease mice with brain insulin resistance via up-regulating miR-200c. Bioengineered (2022) 13:531–43. doi: 10.1080/21655979.2021.2009975
21. Sakalauskas A, Ziaunys M, Snieckute R, Smirnovas V. Autoxidation enhances anti-amyloid potential of flavone derivatives. Antioxidants (Basel) (2021) 10:1428. doi: 10.3390/ANTIOX10091428
22. Karmakar A, Mallick T, Fouzder C, Mukhuty A, Mondal S, Pramanik A, et al. Unfolding the role of a flavone-based fluorescent antioxidant towards the misfolding of amyloid proteins: An endeavour to probe amyloid aggregation. J Phys Chem B (2020) 124:11133–44. doi: 10.1021/ACS.JPCB.0C08729
23. Menezes JCJMDS, Diederich MF. Bioactivity of natural biflavonoids in metabolism-related disease and cancer therapies. Pharmacol Res (2021) 167:105525. doi: 10.1016/J.PHRS.2021.105525
24. Khan MS, Ikram M, Park TJ, Kim MO. Pathology, risk factors, and oxidative damage related to type 2 diabetes-mediated alzheimer’s disease and the rescuing effects of the potent antioxidant anthocyanin. Oxid Med Cell Longev (2021) 2021:4051207. doi: 10.1155/2021/4051207
25. Akhtar A, Dhaliwal J, Sah SP. 7,8-dihydroxyflavone improves cognitive functions in ICV-STZ rat model of sporadic alzheimer’s disease by reversing oxidative stress, mitochondrial dysfunction, and insulin resistance. Psychopharmacol (Berl) (2021) 238:1991–2009. doi: 10.1007/S00213-021-05826-7
26. Zhao L, Duan Z, Wang Y, Wang M, Liu Y, Wang X, et al. Protective effect of terminalia chebula retz. extract against aβ aggregation and aβ-induced toxicity in caenorhabditis elegans. J Ethnopharmacol (2021) 268:113640. doi: 10.1016/J.JEP.2020.113640
27. Sakalauskas A, Ziaunys M, Smirnovas V. Gallic Acid oxidation products alter the formation pathway of insulin amyloid fibrils. Sci Rep (2020) 10:14466. doi: 10.1038/S41598-020-70982-3
28. Bi T, Zhan L, Zhou W, Sui H. Effect of the ZiBuPiYin recipe on diabetes-associated cognitive decline in zucker diabetic fatty rats after chronic psychological stress. Front Psychiatry (2020) 11:272. doi: 10.3389/FPSYT.2020.00272
29. DanQing L, YuJie G, ChengPeng Z, HongZhi D, Yi H, BiSheng H, et al. N-butanol extract of hedyotis diffusa protects transgenic caenorhabditis elegans from aβ-induced toxicity. Phyther Res (2021) 35:1048–61. doi: 10.1002/PTR.6871
30. Chen W, Lin HR, Wei CM, Luo XH, Sun ML, Yang ZZ, et al. Echinacoside, a phenylethanoid glycoside from cistanche deserticola, extends lifespan of caenorhabditis elegans and protects from aβ-induced toxicity. Biogerontology (2018) 19:47–65. doi: 10.1007/S10522-017-9738-0
31. Yang C, Mo YS, Chen HF, Huang YH, Li SL, Wang H, et al. The effects of danggui-Shaoyao-San on neuronal degeneration and amyloidosis in mouse and its molecular mechanism for the treatment of alzheimer’s disease. J Integr Neurosci (2021) 20:255–64. doi: 10.31083/J.JIN2002025
32. Liu F, Dong B, Yang X, Yang Y, Zhang J, Jin DQ, et al. NO inhibitors function as potential anti-neuroinflammatory agents for AD from the flowers of inula japonica. Bioorg Chem (2018) 77:168–75. doi: 10.1016/J.BIOORG.2018.01.009
33. Wu JS, Meng QY, Shi XH, Liu LX, Zhang ZK, Guan HS, et al. The oxygenated products of cryptotanshinone by biotransformation with cunninghamella elegans exerting anti-neuroinflammatory effects by inhibiting TLR 4-mediated MAPK signaling pathway. Bioorg Chem (2020) 104:104246. doi: 10.1016/J.BIOORG.2020.104246
34. Austin SA. Katusic ZS. partial loss of endothelial nitric oxide leads to increased cerebrovascular beta amyloid. J Cereb Blood Flow Metab (2020) 40:392–403. doi: 10.1177/0271678X18822474
35. Huang HJ, Huang CY, Lee M, Lin JY, Hsieh-Li HM. Puerariae radix prevents anxiety and cognitive deficits in mice under oligomeric aβ-induced stress. Am J Chin Med (2019) 47:1459–81. doi: 10.1142/S0192415X19500757
36. YK K, IV P, OL L, YV G, A C, ED K, et al. NLRP3 inflammasome blocking as a potential treatment of central insulin resistance in early-stage alzheimer’s disease. Int J Mol Sci (2021) 22:11588. doi: 10.3390/IJMS222111588
37. Fu Y, Wei J, Li B, Gao L, Xia P, Wen Y, et al. CGA ameliorates cognitive decline by regulating the PI3K/AKT signaling pathway and neurotransmitter systems in rats with multi-infarct dementia. Exp Ther Med (2020) 20:1–1. doi: 10.3892/ETM.2020.9198
38. Luo Y, Yang M, Guo M, Zhong X, Hu Y. Huang Qin hua shi decoction for high-temperature- and high-humidity-induced cognitive-behavioral disorder in rats is associated with deactivation of the hypothalamic-pituitary-adrenal axis. J Int Med Res (2019) 47:5752–66. doi: 10.1177/0300060519875369
39. Liu C, Zheng S, Wu W, Wang X, Qin S, Zhao Y, et al. Effects of acupuncture on the hypothalamus-pituitary-adrenal axis in chronic insomnia patients: a study protocol for a randomized controlled trial. Trials (2019) 20:810. doi: 10.1186/S13063-019-3964-5
40. Kim DS, Byoung-Seob K, Ryuk JA, Park S. Tetragonia tetragonioides protected against memory dysfunction by elevating hippocampal amyloid-β deposition through potentiating insulin signaling and altering gut microbiome composition. Int J Mol Sci (2020) 21:2900. doi: 10.3390/IJMS21082900
41. Thomson EM. Air pollution, stress, and allostatic load: Linking systemic and central nervous system impacts. J Alzheimers Dis (2019) 69:597–614. doi: 10.3233/JAD-190015
42. Gerritsen L, Twait EL, Jonsson PV, Gudnason V, Launer LJ, Geerlings MI. Depression and dementia: The role of cortisol and vascular brain lesions. AGES-Reykjavik study. J Alzheimers Dis (2022) 85:1677–87. doi: 10.3233/JAD-215241
43. Si MW, Yang MK, Fu XD. Effect of hypothalamic-pituitary-adrenal axis alterations on glucose and lipid metabolism in diabetic rats. Genet Mol Res (2015) 14:9562–70. doi: 10.4238/2015.AUGUST.14.19
44. Yao Z, Liu B, Wang Y, Dong X. High cortisol and the risk of Alzheimer disease: A protocol for systematic review and meta-analysis. Med (Baltimore) (2021) 100:e27319. doi: 10.1097/MD.0000000000027319
45. Antypa D, Basta M, Vgontzas A, Zaganas I, Panagiotakis S, Vogiatzi E, et al. The association of basal cortisol levels with episodic memory in older adults is mediated by executive function. Neurobiol Learn Mem (2022) 190:107600. doi: 10.1016/J.NLM.2022.107600
46. Zhuravleva V, Vaz-Silva J, Zhu M, Gomes P, Silva JM, Sousa N, et al. Rab35 and glucocorticoids regulate APP and BACE1 trafficking to modulate aβ production. Cell Death Dis (2021) 12:1137. doi: 10.1038/S41419-021-04433-W
47. Saeedi M, Rashidy-Pour A. Association between chronic stress and alzheimer’s disease: Therapeutic effects of saffron. BioMed Pharmacother (2021) 133:110995. doi: 10.1016/J.BIOPHA.2020.110995
48. Vaz-Silva J, Gomes P, Jin Q, Zhu M, Zhuravleva V, Quintremil S, et al. Endolysosomal degradation of tau and its role in glucocorticoid-driven hippocampal malfunction. EMBO J (2018) 37:e99084. doi: 10.15252/EMBJ.201899084
49. Pedrazzoli M, Losurdo M, Paolone G, Medelin M, Jaupaj L, Cisterna B, et al. Glucocorticoid receptors modulate dendritic spine plasticity and microglia activity in an animal model of alzheimer’s disease. Neurobiol Dis (2019) 132:104568. doi: 10.1016/J.NBD.2019.104568
50. Lanté F, Chafai M, Raymond EF, Salgueiro Pereira AR, Mouska X, Kootar S, et al. Subchronic glucocorticoid receptor inhibition rescues early episodic memory and synaptic plasticity deficits in a mouse model of alzheimer’s disease. Neuropsychopharmacology (2015) 40:1772–81. doi: 10.1038/NPP.2015.25
51. Canet G, Pineau F, Zussy C, Hernandez C, Hunt H, Chevallier N, et al. Glucocorticoid receptors signaling impairment potentiates amyloid-β oligomers-induced pathology in an acute model of alzheimer’s disease. FASEB J (2020) 34:1150–68. doi: 10.1096/FJ.201900723RRR
52. Chen H, Lombès M, Le Menuet D. Glucocorticoid receptor represses brain-derived neurotrophic factor expression in neuron-like cells. Mol Brain (2017) 10:12. doi: 10.1186/S13041-017-0295-X
53. Nguyen TTL, Chan LC, Borreginne K, Kale RP, Hu C. Tye SJ. a review of brain insulin signaling in mood disorders: From biomarker to clinical target. Neurosci Biobehav Rev (2018) 92:7–15. doi: 10.1016/J.NEUBIOREV.2018.05.014
54. Reagan LP, Cowan HB, Woodruff JL, Piroli GG, Erichsen JM, Evans AN, et al. Hippocampal-specific insulin resistance elicits behavioral despair and hippocampal dendritic atrophy. Neurobiol Stress (2021) 15:100354. doi: 10.1016/J.YNSTR.2021.100354
55. Zhang X, Tong T, Chang A, Ang TFA, Tao Q, Auerbach S, et al. Midlife lipid and glucose levels are associated with alzheimer’s disease. Alzheimers Dement (2022). doi: 10.1002/ALZ.12641
56. Li Z, Heckman MG, Kanekiyo T, Martens YA, Day GS, Vassilaki M, et al. Clinicopathologic factors associated with reversion to normal cognition in patients with mild cognitive impairment. Neurology (2022) 98:e2036–45. doi: 10.1212/WNL.0000000000200387
57. Barbiellini Amidei C, Fayosse A, Dumurgier J, MacHado-Fragua MD, Tabak AG, Van Sloten T, et al. Association between age at diabetes onset and subsequent risk of dementia. JAMA (2021) 325:1640–9. doi: 10.1001/JAMA.2021.4001
58. Li W, Wu M, Zhang Y, Wei X, Zang J, Liu Y, et al. Intermittent fasting promotes adult hippocampal neuronal differentiation by activating GSK-3β in 3xTg-AD mice. J Neurochem (2020) 155:697–713. doi: 10.1111/JNC.15105
59. McNay EC, Pearson-Leary J. GluT4: A central player in hippocampal memory and brain insulin resistance. Exp Neurol (2020) 323:113076. doi: 10.1016/J.EXPNEUROL.2019.113076
60. De Felice FG, Gonçalves RA, Ferreira ST. Impaired insulin signalling and allostatic load in Alzheimer disease. Nat Rev Neurosci (2022) 23:215–30. doi: 10.1038/S41583-022-00558-9
61. Zhou AL, Sharda N, Sarma VV, Ahlschwede KM, Curran GL, Tang X, et al. Age-dependent changes in the plasma and brain pharmacokinetics of amyloid-β peptides and insulin. J Alzheimers Dis (2022) 85:1031–44. doi: 10.3233/JAD-215128
62. Xu LZ, Li FY, Li BQ, Cao SM, Li Y, Xu J, et al. Decreased levels of insulin-like growth factor-1 are associated with alzheimer’s disease: A meta-analysis. J Alzheimers Dis (2021) 82:1357–67. doi: 10.3233/JAD-210516
63. Aghanoori MR, Agarwal P, Gauvin E, Nagalingam RS, Bonomo R, Yathindranath V, et al. CEBPβ regulation of endogenous IGF-1 in adult sensory neurons can be mobilized to overcome diabetes-induced deficits in bioenergetics and axonal outgrowth. Cell Mol Life Sci (2022) 79:193. doi: 10.1007/S00018-022-04201-9
64. Diz-Chaves Y, Herrera-Pérez S, González-Matías LC, Mallo F. Effects of glucagon-like peptide 1 (GLP-1) analogs in the hippocampus. Vitam Horm (2022) 118:457–78. doi: 10.1016/BS.VH.2021.12.005
65. Li Z, Zhang Y, Meng X, Li M, Cao W, Yang J, et al. A novel DPP-4 inhibitor gramcyclin a attenuates cognitive deficits in APP/PS1/tau triple transgenic mice via enhancing brain GLP-1-dependent glucose uptake. Phytother Res (2022) 36:1297–309. doi: 10.1002/PTR.7387
66. Aguado-Llera D, Canelles S, Frago LM, Chowen JA, Argente J, Arilla E, et al. The protective effects of IGF-I against β-amyloid-related downregulation of hippocampal somatostatinergic system involve activation of akt and protein kinase a. Neuroscience (2018) 374:104–18. doi: 10.1016/J.NEUROSCIENCE.2018.01.041
67. Zheng Q, Kebede MT, Lee B, Krasinski CA, Islam S, Wurfl LA, et al. Differential effects of polyphenols on insulin proteolysis by the insulin-degrading enzyme. Antioxidants (Basel) (2021) 10:1342. doi: 10.3390/ANTIOX10091342
68. Zhang Y, Wang P. Age-related increase of insulin-degrading enzyme is inversely correlated with cognitive function in APPswe/PS1dE9 mice. Med Sci Monit (2018) 24:2446–55. doi: 10.12659/MSM.909596
69. Marbouti L, Zahmatkesh M, Riahi E, Shafiee Sabet M. GnRH protective effects against amyloid β-induced cognitive decline: A potential role of the 17β-estradiol. Mol Cell Endocrinol (2020) 518:110985. doi: 10.1016/J.MCE.2020.110985
70. Yun J, Yeo IJ, Hwang CJ, Choi DY, Im HS, Kim JY, et al. Estrogen deficiency exacerbates aβ-induced memory impairment through enhancement of neuroinflammation, amyloidogenesis and NF-ĸB activation in ovariectomized mice. Brain Behav Immun (2018) 73:282–93. doi: 10.1016/J.BBI.2018.05.013
71. Guglielmotto M, Manassero G, Vasciaveo V, Venezia M, Tabaton M, Tamagno E. Estrogens inhibit amyloid-β-Mediated paired helical filament-like conformation of tau through antioxidant activity and miRNA 218 regulation in hTau mice. J Alzheimers Dis (2020) 77:1339–51. doi: 10.3233/JAD-200707
72. Taxier LR, Philippi SM, York JM, LaDu MJ, Frick KM. APOE4 genotype or ovarian hormone loss influence open field exploration in an EFAD mouse model of alzheimer’s disease. Horm Behav (2022) 140:105124. doi: 10.1016/J.YHBEH.2022.105124
73. Mosconi L, Berti V, Dyke J, Schelbaum E, Jett S, Loughlin L, et al. Menopause impacts human brain structure, connectivity, energy metabolism, and amyloid-beta deposition. Sci Rep (2021) 11:10867. doi: 10.1038/S41598-021-90084-Y
74. Schelbaum E, Loughlin L, Jett S, Zhang C, Jang G, Malviya N, et al. Association of reproductive history with brain MRI biomarkers of dementia risk in midlife. Neurology (2021) 97:E2328–39. doi: 10.1212/WNL.0000000000012941
75. Marriott RJ, Murray K, Flicker L, Hankey GJ, Matsumoto AM, Dwivedi G, et al. Lower serum testosterone concentrations are associated with a higher incidence of dementia in men: The UK biobank prospective cohort study. Alzheimers Dement (2022). doi: 10.1002/ALZ.12529
76. Yao M, Rosario ER, Soper JC, Pike CJ. Androgens regulate tau phosphorylation through phosphatidylinositol 3-Kinase-Protein kinase b-glycogen synthase kinase 3β signaling. Neuroscience (2022). doi: 10.1016/J.NEUROSCIENCE.2022.06.034
77. Kling JM, Miller VM, Tosakulwong N, Lesnick T, Kantarci K. Associations of pituitary-ovarian hormones and white matter hyperintensities in recently menopausal women using hormone therapy. Menopause (2020) 27:872–8. doi: 10.1097/GME.0000000000001557
78. Bhatta S, Blair JA, Casadesus G. Luteinizing hormone involvement in aging female cognition: Not all is estrogen loss. Front Endocrinol (Lausanne) (2018) 9:544. doi: 10.3389/FENDO.2018.00544
79. Sohrabi M, Sahu B, Kaur H, Hasler W, Prakash A, Combs CK. Gastrointestinal changes and alzheimer’s disease. Curr Alzheimer Res (2022) 19:335–50. doi: 10.2174/1567205019666220617121255
80. Ou Z, Deng L, Lu Z, Wu F, Liu W, Huang D, et al. Protective effects of akkermansia muciniphila on cognitive deficits and amyloid pathology in a mouse model of alzheimer’s disease. Nutr Diabetes (2020) 10:20. doi: 10.1038/S41387-020-0115-8
81. Haran JP, Bhattarai SK, Foley SE, Dutta P, Ward DV, Bucci V, et al. Alzheimer’s disease microbiome is associated with dysregulation of the anti-inflammatory p-glycoprotein pathway. MBio (2019) 10:e00632-19. doi: 10.1128/MBIO.00632-19
82. Ishioh M, Nozu T, Igarashi S, Tanabe H, Kumei S, Ohhira M, et al. Activation of central adenosine A2B receptors mediate brain ghrelin-induced improvement of intestinal barrier function through the vagus nerve in rats. Exp Neurol (2021) 341:113708. doi: 10.1016/J.EXPNEUROL.2021.113708
83. Bonfili L, Cuccioloni M, Gong C, Cecarini V, Spina M, Zheng Y, et al. Gut microbiota modulation in alzheimer’s disease: Focus on lipid metabolism. Clin Nutr (2022) 41:698–708. doi: 10.1016/J.CLNU.2022.01.025
84. Mesa-Herrera F, Marín R, Torrealba E, Santos G, Díaz M. Neuronal ER-signalosome proteins as early biomarkers in prodromal alzheimer’s disease independent of amyloid-β production and tau phosphorylation. Front Mol Neurosci (2022) 15:879146. doi: 10.3389/FNMOL.2022.879146
85. Christensen A, Liu J, Pike CJ. Aging reduces estradiol protection against neural but not metabolic effects of obesity in female 3xTg-AD mice. Front Aging Neurosci (2020) 12:113. doi: 10.3389/FNAGI.2020.00113
86. Joseph JJ, Golden SH. Cortisol dysregulation: the bidirectional link between stress, depression, and type 2 diabetes mellitus. Ann N Y Acad Sci (2017) 1391:20–34. doi: 10.1111/NYAS.13217
87. Sharma VK, Singh TG. Chronic stress and diabetes mellitus: Interwoven pathologies. Curr Diabetes Rev (2020) 16:546–56. doi: 10.2174/1573399815666191111152248
88. Dey A, Hao S, Wosiski-Kuhn M, Stranahan AM. Glucocorticoid-mediated activation of GSK3β promotes tau phosphorylation and impairs memory in type 2 diabetes. Neurobiol Aging (2017) 57:75–83. doi: 10.1016/J.NEUROBIOLAGING.2017.05.010
89. Notarianni E. Cortisol: Mediator of association between alzheimer’s disease and diabetes mellitus? Psychoneuroendocrinology (2017) 81:129–37. doi: 10.1016/J.PSYNEUEN.2017.04.008
90. Zeibich L, Koebele SV, Bernaud VE, Ilhan ZE, Dirks B, Northup-Smith SN, et al. Surgical menopause and estrogen therapy modulate the gut microbiota, obesity markers, and spatial memory in rats. Front Cell Infect Microbiol (2021) 11:702628. doi: 10.3389/FCIMB.2021.702628
91. Akel H, Csóka I, Ambrus R, Bocsik A, Gróf I, Mészáros M, et al. In vitro comparative study of solid lipid and PLGA nanoparticles designed to facilitate nose-to-Brain delivery of insulin. Int J Mol Sci (2021) 22:13258. doi: 10.3390/IJMS222413258
92. Tran S, Kuruppu S, Rajapakse NW. Chronic renin-angiotensin system activation induced neuroinflammation: Common mechanisms underlying hypertension and dementia? J Alzheimers Dis (2022) 85:943–55. doi: 10.3233/JAD-215231
93. Cosarderelioglu C, Nidadavolu LS, George CJ, Marx-Rattner R, Powell L, Xue Q-L, et al. Higher angiotensin II type 1 receptor levels and activity in the postmortem brains of older persons with alzheimer’s dementia. J Gerontol A Biol Sci Med Sci (2022) 77:664–72. doi: 10.1093/GERONA/GLAB376
94. Urmila A, Rashmi P, Nilam G, Subhash B. Recent advances in the endogenous brain renin-angiotensin system and drugs acting on it. J Renin Angiotensin Aldosterone Syst (2021) 2021:9293553. doi: 10.1155/2021/9293553
95. Cervera-Juanes R, Darakjian P, Ball M, Kohama SG, Urbanski HF. Effects of estradiol supplementation on the brain transcriptome of old rhesus macaques maintained on an obesogenic diet. GeroScience (2022) 44:229–52. doi: 10.1007/S11357-021-00453-8
96. Wang Y, Shen J, Yang X, Jin Y, Yang Z, Wang R, et al. Akebia saponin d reverses corticosterone hypersecretion in an alzheimer’s disease rat model. BioMed Pharmacother (2018) 107:219–25. doi: 10.1016/J.BIOPHA.2018.07.149
97. Li WZ, Wu WY, Huang DK, Yin YY, Kan HW, Wang X, et al. Protective effects of astragalosides on dexamethasone and Aβ25-35 induced learning and memory impairments due to decrease amyloid precursor protein expression in 12-month male rats. Food Chem Toxicol (2012) 50:1883–90. doi: 10.1016/J.FCT.2012.03.064
98. Liu XJ, Wei J, Shang YH, Huang HC, Lao FX. Modulation of AβPP and GSK3β by endoplasmic reticulum stress and involvement in alzheimer’s disease. J Alzheimers Dis (2017) 57:1157–70. doi: 10.3233/JAD-161111
99. Ghemrawi R, Khair M. Endoplasmic reticulum stress and unfolded protein response in neurodegenerative diseases. Int J Mol Sci (2020) 21:1–25. doi: 10.3390/IJMS21176127
100. Jin W, Ma R, Zhai L, Xu X, Lou T, Huang Q, et al. Ginsenoside Rd attenuates ACTH-induced corticosterone secretion by blocking the MC2R-cAMP/PKA/CREB pathway in Y1 mouse adrenocortical cells. Life Sci (2020) 245:117337. doi: 10.1016/J.LFS.2020.117337
101. Jiang Y, Li Z, Liu Y, Liu X, Chang Q, Liao Y, et al. Neuroprotective effect of water extract of panax ginseng on corticosterone-induced apoptosis in PC12 cells and its underlying molecule mechanisms. J Ethnopharmacol (2015) 159:102–12. doi: 10.1016/J.JEP.2014.10.062
102. Yun HY, Jeong Y. Sedum takesimense protects PC12 cells against corticosterone-induced neurotoxicity by inhibiting neural apoptosis. Nutrients (2020) 12:1–16. doi: 10.3390/NU12123713
103. Yeung JHY, Walby JL, Palpagama TH, Turner C, Waldvogel HJ, Faull RLM, et al. Glutamatergic receptor expression changes in the alzheimer’s disease hippocampus and entorhinal cortex. Brain Pathol (2021) 31:e13005. doi: 10.1111/BPA.13005
104. Xiong TW, Liu B, Wu Q, Xu YY, Liu P, Wang Y, et al. Beneficial effects of dendrobium nobile lindl. Alkaloids (DNLA) on anxiety and depression induced by chronic unpredictable stress in rats. Brain Res (2021) 1771:147647. doi: 10.1016/J.BRAINRES.2021.147647
105. Yamaguchi T, Tsujimatsu A, Kumamoto H, Izumi T, Ohmura Y, Yoshida T, et al. Anxiolytic effects of yokukansan, a traditional Japanese medicine, via serotonin 5-HT1A receptors on anxiety-related behaviors in rats experienced aversive stress. J Ethnopharmacol (2012) 143:533–9. doi: 10.1016/J.JEP.2012.07.007
106. Liu XQ, Deng YX, Dai Z, Hu T, Cai WW, Liu HF, et al. Sodium tanshinone IIA sulfonate protects against aβ 1-42-induced cellular toxicity by modulating aβ-degrading enzymes in HT22 cells. Int J Biol Macromol (2020) 151:47–55. doi: 10.1016/J.IJBIOMAC.2020.02.040
107. Wang H, Huang H, Jiang N, Zhang Y, Lv J, Liu X. Tenuifolin ameliorates chronic restraint stress-induced cognitive impairment in C57BL/6J mice. Phytother Res (2022) 36:1402–12. doi: 10.1002/PTR.7402
108. Lv J, Chen L, Zhu N, Sun Y, Pan J, Gao J, et al. Beta amyloid-induced time-dependent learning and memory impairment: involvement of HPA axis dysfunction. Metab Brain Dis (2020) 35:1385–94. doi: 10.1007/S11011-020-00613-3
109. Tang C, Liu X, Zhu H, Lu Q. Antagonizing effect of icaritin on apoptosis and injury of hippocampal neurocytes induced by amyloid beta via GR/BDNF signaling pathway. J Recept Signal Transduct Res (2020) 40:550–9. doi: 10.1080/10799893.2020.1768547
110. Li XY, Ma J, Xu J, Zhu DS, Li A, Che YZ, et al. Puerarin and amlodipine improvement of d-Galactose-Induced impairments of behaviour and neurogenesis in mouse dentate gyrus: Correlation with glucocorticoid receptor expression. Neurochem Res (2017) 42:3268–78. doi: 10.1007/S11064-017-2366-X
111. Nakatani Y, Tsuji M, Amano T, Miyagawa K, Miyagishi H, Saito A, et al. Neuroprotective effect of yokukansan against cytotoxicity induced by corticosterone on mouse hippocampal neurons. Phytomedicine (2014) 21:1458–65. doi: 10.1016/J.PHYMED.2014.06.004
112. Yan J, Liu Q, Dou Y, Hsieh Y, Liu Y, Tao R, et al. Activating glucocorticoid receptor-ERK signaling pathway contributes to ginsenoside Rg1 protection against β-amyloid peptide-induced human endothelial cells apoptosis. J Ethnopharmacol (2013) 147:456–66. doi: 10.1016/J.JEP.2013.03.039
113. Zhang Y, Hu W, Zhang B, Yin Y, Zhang J, Huang D, et al. Ginsenoside Rg1 protects against neuronal degeneration induced by chronic dexamethasone treatment by inhibiting NLRP-1 inflammasomes in mice. Int J Mol Med (2017) 40:1134–42. doi: 10.3892/IJMM.2017.3092
114. Cheng L, Osada H, Xing T, Yoshida M, Xiang L, Qi J. The insulin receptor: A potential target of amarogentin isolated from gentiana rigescens franch that induces neurogenesis in PC12 cells. Biomedicines (2021) 9:581. doi: 10.3390/BIOMEDICINES9050581
115. Pakdeepak K, Chokchaisiri R, Govitrapong P, Tocharus C, Suksamrarn A, Tocharus J. 5,6,7,4’-tetramethoxyflavanone alleviates neurodegeneration in a dexamethasone-induced neurodegenerative mouse model through promotion of neurogenesis via the Raf/ERK1/2 pathway. Phytother Res (2020) 35:2536–44. doi: 10.1002/PTR.6983
116. Mehta V, Parashar A, Sharma A, Singh TR, Udayabanu M. Quercetin ameliorates chronic unpredicted stress-mediated memory dysfunction in male Swiss albino mice by attenuating insulin resistance and elevating hippocampal GLUT4 levels independent of insulin receptor expression. Horm Behav (2017) 89:13–22. doi: 10.1016/J.YHBEH.2016.12.012
117. Wang J, Zhang X, Cheng X, Cheng J, Liu F, Xu Y, et al. Ameliorates cognitive deterioration and modulates neuroendocrine-immune system in SAMP8 mouse. Curr Alzheimer Res (2017) 14:221–38. doi: 10.2174/1567205013666160603001637
118. Tang Y, Yu C, Wu J, Chen H, Zeng Y, Wang X, et al. Lychee seed extract protects against neuronal injury and improves cognitive function in rats with type II diabetes mellitus with cognitive impairment. Int J Mol Med (2018) 41:251–63. doi: 10.3892/IJMM.2017.3245
119. Huang YC, Tsay HJ, Lu MK, Lin CH, Yeh CW, Liu HK, et al. Astragalus membranaceus-polysaccharides ameliorates obesity, hepatic steatosis, neuroinflammation and cognition impairment without affecting amyloid deposition in metabolically stressed APPswe/PS1dE9 mice. Int J Mol Sci (2017) 18:2746. doi: 10.3390/IJMS18122746
120. Yeh CW, Liu HK, Lin LC, Liou KT, Huang YC, Lin CH, et al. Xuefu zhuyu decoction ameliorates obesity, hepatic steatosis, neuroinflammation, amyloid deposition and cognition impairment in metabolically stressed APPswe/PS1dE9 mice. J Ethnopharmacol (2017) 209:50–61. doi: 10.1016/J.JEP.2017.07.036
121. Li H, Qin T, Li M, Ma S. Thymol improves high-fat diet-induced cognitive deficits in mice via ameliorating brain insulin resistance and upregulating NRF2/HO-1 pathway. Metab Brain Dis (2017) 32:385–93. doi: 10.1007/S11011-016-9921-Z
122. Saleh RA, Eissa TF, Abdallah DM, Saad MA, El-Abhar HS. Peganum harmala enhanced GLP-1 and restored insulin signaling to alleviate AlCl 3-induced Alzheimer-like pathology model. Sci Rep (2021) 11:12040. doi: 10.1038/S41598-021-90545-4
123. Zhi D, Wang D, Yang W, Duan Z, Zhu S, Dong J, et al. Dianxianning improved amyloid β-induced pathological characteristics partially through DAF-2/DAF-16 insulin like pathway in transgenic c. elegans Sci Rep (2017) 7:11408. doi: 10.1038/S41598-017-11628-9
124. Wang P, Su C, Feng H, Chen X, Dong Y, Rao Y, et al. Curcumin regulates insulin pathways and glucose metabolism in the brains of APPswe/PS1dE9 mice. Int J Immunopathol Pharmacol (2017) 30:25–43. doi: 10.1177/0394632016688025
125. Thota RN, Rosato JI, Dias CB, Burrows TL, Martins RN, Garg ML. Dietary supplementation with curcumin reduce circulating levels of glycogen synthase kinase-3β and islet amyloid polypeptide in adults with high risk of type 2 diabetes and alzheimer’s disease. Nutrients (2020) 12:1032. doi: 10.3390/NU12041032
126. Chen F, He Y, Wang P, Wei P, Feng H, Rao Y, et al. Banxia xiexin decoction ameliorated cognition via the regulation of insulin pathways and glucose transporters in the hippocampus of APPswe/PS1dE9 mice. Int J Immunopathol Pharmacol (2018) 32:2058738418780066. doi: 10.1177/2058738418780066
127. Wang R, Reddy PH. Role of glutamate and NMDA receptors in alzheimer’s disease. J Alzheimers Dis (2017) 57:1041–8. doi: 10.3233/JAD-160763
128. Liu P, Cui L, Liu B, Liu W, Hayashi T, Mizuno K, et al. Silibinin ameliorates STZ-induced impairment of memory and learning by up- regulating insulin signaling pathway and attenuating apoptosis. Physiol Behav (2020) 213:112689. doi: 10.1016/J.PHYSBEH.2019.112689
129. Kim DS, Zhang T, Park S. Protective effects of forsythiae fructus and cassiae semen water extract against memory deficits through the gut-microbiome-brain axis in an alzheimer’s disease model. Pharm Biol (2022) 60:212–24. doi: 10.1080/13880209.2022.2025860
130. Daily JW, Kang S, Park S. Protection against alzheimer’s disease by luteolin: Role of brain glucose regulation, anti-inflammatory activity, and the gut microbiota-liver-brain axis. Biofactors (2021) 47:218–31. doi: 10.1002/BIOF.1703
131. Zhang W, Bai S, Yang J, Zhang Y, Liu Y, Nie J, et al. FoxO1 overexpression reduces aβ production and tau phosphorylation in vitro. Neurosci Lett (2020) 738:135322. doi: 10.1016/J.NEULET.2020.135322
132. Xu M, Huang H, Mo X, Zhu Y, Chen X, Li X, et al. Quercetin-3-O-Glucuronide alleviates cognitive deficit and toxicity in aβ 1-42 -induced AD-like mice and SH-SY5Y cells. Mol Nutr Food Res (2021) 65:e2000660. doi: 10.1002/MNFR.202000660
133. Park YJ, Ko J, Jeon S, Kwon YH. Protective effect of genistein against neuronal degeneration in ApoE -/- mice fed a high-fat diet. Nutrients (2016) 8:692. doi: 10.3390/NU8110692
134. Gundu C, Arruri VK, Sherkhane B, Khatri DK, Singh SB. GSK2606414 attenuates PERK/p-eIF2α/ATF4/CHOP axis and augments mitochondrial function to mitigate high glucose induced neurotoxicity in N2A cells. Curr Res Pharmacol Drug Discovery (2022) 3:100087. doi: 10.1016/J.CRPHAR.2022.100087
135. Ettcheto M, Cano A, Manzine PR, Busquets O, Verdaguer E, Castro-Torres RD, et al. Epigallocatechin-3-Gallate (EGCG) improves cognitive deficits aggravated by an obesogenic diet through modulation of unfolded protein response in APPswe/PS1dE9 mice. Mol Neurobiol (2020) 57:1814–27. doi: 10.1007/S12035-019-01849-6
136. Chen Q, Mo R, Wu N, Zou X, Shi C, Gong J, et al. Berberine ameliorates diabetes-associated cognitive decline through modulation of aberrant inflammation response and insulin signaling pathway in DM rats. Front Pharmacol (2017) 8:334. doi: 10.3389/FPHAR.2017.00334
137. Wu N, Liu W, Wang J, Han Y, Ye Y, Liu X, et al. Berberine ameliorates neuronal AD-like change via activating Pi3k/PGCϵ pathway. Biofactors (2021) 47:587–99. doi: 10.1002/BIOF.1725
138. Li RZ, Ding XW, Geetha T, Al-Nakkash L, Broderick TL, Babu JR. Beneficial effect of genistein on diabetes-induced brain damage in the ob/ob mouse model. Drug Des Devel Ther (2020) 14:3325–36. doi: 10.2147/DDDT.S249608
139. Li HQ, Ip SP, Yuan QJ, Zheng GQ, Tsim KKW, Dong TTX, et al. Isorhynchophylline ameliorates cognitive impairment via modulating amyloid pathology, tau hyperphosphorylation and neuroinflammation: Studies in a transgenic mouse model of alzheimer’s disease. Brain Behav Immun (2019) 82:264–78. doi: 10.1016/J.BBI.2019.08.194
140. Qu C, Li QP, Su ZR, Ip SP, Yuan QJ, Xie YL, et al. Nano-honokiol ameliorates the cognitive deficits in TgCRND8 mice of alzheimer’s disease via inhibiting neuropathology and modulating gut microbiota. J Adv Res (2021) 35:231–43. doi: 10.1016/J.JARE.2021.03.012
141. Park S, Kim DS, Kang S, Kim HJ. The combination of luteolin and l-theanine improved Alzheimer disease-like symptoms by potentiating hippocampal insulin signaling and decreasing neuroinflammation and norepinephrine degradation in amyloid-β-infused rats. Nutr Res (2018) 60:116–31. doi: 10.1016/J.NUTRES.2018.09.010
142. Zhang DP, Lu XY, He SC, Li WY, Ao R, Leung FCY, et al. Sodium tanshinone IIA sulfonate protects against aβ-induced cell toxicity through regulating aβ process. J Cell Mol Med (2020) 24:3328–35. doi: 10.1111/JCMM.15006
143. Feng CZ, Cao L, Luo D, Ju LS, Yang JJ, Xu XY, et al. Dendrobium polysaccharides attenuate cognitive impairment in senescence-accelerated mouse prone 8 mice via modulation of microglial activation. Brain Res (2019) 1704:1–10. doi: 10.1016/J.BRAINRES.2018.09.030
144. Salem MA, Budzyńska B, Kowalczyk J, El Sayed NS, Mansour SM. Tadalafil and bergapten mitigate streptozotocin-induced sporadic alzheimer’s disease in mice via modulating neuroinflammation, PI3K/Akt, wnt/β-catenin, AMPK/mTOR signaling pathways. Toxicol Appl Pharmacol (2021) 429:115697. doi: 10.1016/J.TAAP.2021.115697
145. Tian R, Liu X, Jing L, yang L, Xie N, Hou Y, et al. Huang-Lian-Jie-Du decoction attenuates cognitive dysfunction of rats with type 2 diabetes by regulating autophagy and NLRP3 inflammasome activation. J Ethnopharmacol (2022) 292:115196. doi: 10.1016/J.JEP.2022.115196
146. Szablewski L. Glucose transporters in brain: In health and in alzheimer’s disease. J Alzheimers Dis (2017) 55:1307–20. doi: 10.3233/JAD-160841
147. Wang S, He B, Hang W, Wu NH, Xia L, Wang X, et al. Berberine alleviates tau hyperphosphorylation and axonopathy-associated with diabetic encephalopathy via restoring PI3K/Akt/GSK3β pathway. J Alzheimers Dis (2018) 65:1385–400. doi: 10.3233/JAD-180497
148. Zhang HN, Sun YJ, He HQ, Li HY, Xue QL, Liu ZM, et al. Berberine promotes nerve regeneration through IGFR−mediated JNK−AKT signal pathway. Mol Med Rep (2018) 18:5030–6. doi: 10.3892/MMR.2018.9508
149. Zhang JH, Zhang JF, Song J, Bai Y, Deng L, Feng CP, et al. Effects of berberine on diabetes and cognitive impairment in an animal model: The mechanisms of action. Am J Chin Med (2021) 49:1399–415. doi: 10.1142/S0192415X21500658
150. Chen J, Gao L, Zhang Y, Su Y, Kong Z, Wang D, et al. Acteoside-improved streptozotocin-induced learning and memory impairment by upregulating hippocampal insulin, glucose transport, and energy metabolism. Phytother Res (2021) 35:392–403. doi: 10.1002/PTR.6811
151. Das TK, Chakrabarti SK, Zulkipli IN, Abdul Hamid MRW. Curcumin ameliorates the impaired insulin signaling involved in the pathogenesis of alzheimer’s disease in rats. J Alzheimer’s Dis Rep (2019) 3:59–70. doi: 10.3233/ADR-180091
152. Bin PS, JY K, JM K, SK P, SK Y, Lee U, et al. Effect of aruncus dioicus var. kamtschaticus extract on neurodegeneration improvement: Ameliorating role in cognitive disorder caused by high-fat diet induced obesity. Nutrients (2019) 11:1319. doi: 10.3390/NU11061319
153. Wang Hy, Wu M, Diao Jl, Li Jb, Sun Yx, Xiao Xq. Huperzine a ameliorates obesity-related cognitive performance impairments involving neuronal insulin signaling pathway in mice. Acta Pharmacol Sin (2020) 41:145–53. doi: 10.1038/S41401-019-0257-1
154. Gomaa AA, Makboul RM, Al-Mokhtar MA, Nicola MA. Polyphenol-rich boswellia serrata gum prevents cognitive impairment and insulin resistance of diabetic rats through inhibition of GSK3β activity, oxidative stress and pro-inflammatory cytokines. BioMed Pharmacother (2019) 109:281–92. doi: 10.1016/J.BIOPHA.2018.10.056
155. Gomaa AA, Makboul RM, El-Mokhtar MA, Abdel-Rahman EA, Ahmed IA, Nicola MA. Terpenoid-rich elettaria cardamomum extract prevents Alzheimer-like alterations induced in diabetic rats via inhibition of GSK3β activity, oxidative stress and pro-inflammatory cytokines. Cytokine (2019) 113:405–16. doi: 10.1016/J.CYTO.2018.10.017
156. Li JC, Shen XF, Shao JA, Tao MM, Gu J, Li J, et al. The total alkaloids from coptis chinensis franch improve cognitive deficits in type 2 diabetic rats. Drug Des Devel Ther (2018) 12:2695–706. doi: 10.2147/DDDT.S171025
157. Xiong R, Wang XL, Wu JM, Tang Y, Qiu WQ, Shen X, et al. Polyphenols isolated from lychee seed inhibit alzheimer’s disease-associated tau through improving insulin resistance via the IRS-1/PI3K/Akt/GSK-3β pathway. J Ethnopharmacol (2020) 251:112548. doi: 10.1016/J.JEP.2020.112548
158. Zhu L, Hou Xj, Che Xh, Zhou Ts, Liu X, Wu C, et al. Pseudoginsenoside-F11 attenuates cognitive dysfunction and tau phosphorylation in sporadic alzheimer’s disease rat model. Acta Pharmacol Sin (2021) 42:1401–8. doi: 10.1038/S41401-020-00562-8
159. Quan Q, Li X, Feng J, Hou J, Li M, Zhang B. Ginsenoside Rg1 reduces β−amyloid levels by inhibiting CDK5−induced PPARγ phosphorylation in a neuron model of alzheimer’s disease. Mol Med Rep (2020) 22:3277–88. doi: 10.3892/MMR.2020.11424
160. Li Z, Li H, Zhao C, Lv C, Zhong C, Xin W, et al. Protective effect of notoginsenoside R1 on an APP/PS1 mouse model of alzheimer’s disease by up-regulating insulin degrading enzyme and inhibiting aβ accumulation. CNS Neurol Disord Drug Targets (2015) 14:360–9. doi: 10.2174/1871527314666150225141521
161. Wang H, Sun X, Zhang N, Ji Z, Ma Z, Fu Q, et al. Ferulic acid attenuates diabetes-induced cognitive impairment in rats via regulation of PTP1B and insulin signaling pathway. Physiol Behav (2017) 182:93–100. doi: 10.1016/J.PHYSBEH.2017.10.001
162. Wang NY, Li JN, Liu WL, Huang Q, Li WX, Tan YH, et al. Ferulic acid ameliorates alzheimer’s disease-like pathology and repairs cognitive decline by preventing capillary hypofunction in APP/PS1 mice. Neurotherapeutics (2021) 18:1064–80. doi: 10.1007/S13311-021-01024-7
163. Sneideris T, Sakalauskas A, Sternke-Hoffmann R, Peduzzo A, Ziaunys M, Buell AK, et al. The environment is a key factor in determining the anti-amyloid efficacy of EGCG. Biomolecules (2019) 9:855. doi: 10.3390/BIOM9120855
164. Cho EJ, Kim HY, Lee AY. Paeoniflorin ameliorates aβ-stimulated neuroinflammation via regulation of NF-κB signaling pathway and aβ degradation in C6 glial cells. Nutr Res Pract (2020) 14:593–605. doi: 10.4162/NRP.2020.14.6.593
165. Yamamoto N, Shibata M, Ishikuro R, Tanida M, Taniguchi Y, Ikeda-Matsuo Y, et al. Epigallocatechin gallate induces extracellular degradation of amyloid β-protein by increasing neprilysin secretion from astrocytes through activation of ERK and PI3K pathways. Neuroscience (2017) 362:70–8. doi: 10.1016/J.NEUROSCIENCE.2017.08.030
166. Ziaunys M, Mikalauskaite K, Sakalauskas A, Smirnovas V. Interplay between epigallocatechin-3-gallate and ionic strength during amyloid aggregation. PeerJ (2021) 9:e12381. doi: 10.7717/PEERJ.12381
167. Huang C, Dong D, Jiao Q, Pan H, Ma L, Wang R. Sarsasapogenin-AA13 ameliorates aβ-induced cognitive deficits via improving neuroglial capacity on aβ clearance and antiinflammation. CNS Neurosci Ther (2017) 23:498–509. doi: 10.1111/CNS.12697
168. Zeng H, Li P, Zhou L, Ding K. A novel pectin from polygala tenuifolia blocks aβ 42 aggregation and production by enhancing insulin-degradation enzyme and neprilysin. Int J Biol Macromol (2020) 161:35–43. doi: 10.1016/J.IJBIOMAC.2020.05.212
169. Zhou L, Liao W, Zeng H, Yao Y, Chen X, Ding K. A pectin from fruits of lycium barbarum l. decreases β-amyloid peptide production through modulating APP processing. Carbohydr Polym (2018) 201:65–74. doi: 10.1016/J.CARBPOL.2018.08.050
170. Yang R, Yang R, Yang R, Jiang X, He X, Liang D, et al. Ginsenoside Rb1 improves cognitive impairment induced by insulin resistance through Cdk5/p35-NMDAR-IDE pathway. BioMed Res Int (2020) 2020:3905719. doi: 10.1155/2020/3905719
171. Pi T, Lang G, Liu B, Shi J. Protective effects of dendrobium nobile lindl. alkaloids on alzheimer’s disease-like symptoms induced by high-methionine diet. Curr Neuropharmacol (2022) 20:983–97. doi: 10.2174/1570159X19666210809101945
172. Liu Sz, Cheng W, Shao Jw, Gu Yf, Zhu Y, Dong Qj, et al. Notoginseng saponin Rg1 prevents cognitive impairment through modulating APP processing in aβ 1-42-injected rats. Curr Med Sci (2019) 39:196–203. doi: 10.1007/S11596-019-2019-1
173. Yun YJ, Park BH, Hou J, Oh JP, Han JH, Kim SC. Ginsenoside F1 protects the brain against amyloid beta-induced toxicity by regulating IDE and NEP. Life (2022) 12:58. doi: 10.3390/life12010058
174. Yang C, Bao X, Zhang L, Li Y, Li L, Zhang L. Cornel iridoid glycoside ameliorates cognitive deficits in APP/PS1/tau triple transgenic mice by attenuating amyloid-beta, tau hyperphosphorylation and neurotrophic dysfunction. Ann Transl Med (2020) 8:328–8. doi: 10.21037/ATM.2020.02.138
175. Wang N, Jia YM, Zhang B, Xue D, Reeju M, Li Y, et al. Neuroprotective mechanism of kai xin San: upregulation of hippocampal insulin-degrading enzyme protein expression and acceleration of amyloid-beta degradation. Neural Regener Res (2017) 12:654–9. doi: 10.4103/1673-5374.205107
176. Huang P, Zheng N, Zhou Hb, Huang J. Curcumin inhibits BACE1 expression through the interaction between ERβ and NFκB signaling pathway in SH-SY5Y cells. Mol Cell Biochem (2020) 463:161–73. doi: 10.1007/S11010-019-03638-0
177. Song L, Li X, Bai XX, Gao J, Wang CY. Calycosin improves cognitive function in a transgenic mouse model of alzheimer’s disease by activating the protein kinase c pathway. Neural Regener Res (2017) 12:1870–6. doi: 10.4103/1673-5374.219049
178. Song X, Liu B, Cui L, Zhou B, Liu L, Liu W, et al. Estrogen receptors are involved in the neuroprotective effect of silibinin in aβ 1-42-Treated rats. Neurochem Res (2018) 43:796–805. doi: 10.1007/S11064-018-2481-3
179. Xie Y, Yan B, Hou M, Zhou M, Liu C, Sun M, et al. Erzhi pills ameliorate cognitive dysfunction and alter proteomic hippocampus profiles induced by d-galactose and aβ 1-40 injection in ovariectomized alzheimer’s disease model rats. Pharm Biol (2021) 59:1402–14. doi: 10.1080/13880209.2021.1990353
180. Zhang N, Xu H, Wang Y, Yao Y, Liu G, Lei X, et al. Protective mechanism of kaempferol against aβ 25-35-mediated apoptosis of pheochromocytoma (PC-12) cells through the ER/ERK/MAPK signalling pathway. Arch Med Sci (2020) 17:406–16. doi: 10.5114/AOMS.2020.98199
181. Yan Qy, Lv J, Shen X, Ou-Yang Xn, Yang Jz, Nie Rf, et al. Patchouli alcohol as a selective estrogen receptor β agonist ameliorates AD-like pathology of APP/PS1 model mice. Acta Pharmacol Sin (2022) 43:2226–41. doi: 10.1038/S41401-021-00857-4
182. Kong D, Yan Y, He XY, Yang H, Liang BY, Wang J, et al. Effects of resveratrol on the mechanisms of antioxidants and estrogen in alzheimer’s disease. BioMed Res Int (2019) 2019:8983752. doi: 10.1155/2019/8983752
183. Hou Y, Zhao W, Yu H, Zhang F, Zhang HT, Zhou Y. Biochanin a alleviates cognitive impairment and hippocampal mitochondrial damage in ovariectomized APP/PS1 mice. Phytomedicine (2022) 100:154056. doi: 10.1016/J.PHYMED.2022.154056
184. Yilong D, Ruiqian L, Aimei J, Zhong X, Yanmei W. Phytoestrogen alpha-zearalanol attenuate endoplasmic reticulum stress to against cultured rat hippocampal neurons apoptotic death induced by amyloid beta25-35. Neuro Endocrinol Lett (2017) 38:353–9.
185. Wang Yx, Xia Zh, Jiang X, Li Lx, Wang Hg, An D, et al. Genistein inhibits amyloid peptide 25-35-induced neuronal death by modulating estrogen receptors, choline acetyltransferase and glutamate receptors. Arch Biochem Biophys (2020) 693:108561. doi: 10.1016/J.ABB.2020.108561
186. Oh JM, Jeong JH, Park SY, Chun S. Ginsenoside compound K induces adult hippocampal proliferation and survival of newly generated cells in young and elderly mice. Biomolecules (2020) 10:484. doi: 10.3390/BIOM10030484
187. Yang B, He M, Chen X, Sun M, Pan T, Xu X, et al. Acupuncture effect assessment in APP/PS1 transgenic mice: On regulating learning-memory abilities, gut microbiota, and microbial metabolites. Comput Math Methods Med (2022) 2022:1527159. doi: 10.1155/2022/1527159
188. Yang S-Q, Fan D-H, Yuan Z-X, Su M-Y, Zhang Z-N, Lin Y, et al. Abdominal acupoint thread embedding therapy based on "brain-intestinal connection" for mild-to-moderate alzheimer’s disease and its effects on serum levels of APP and aβ 1-42. Zhongguo Zhen Jiu (2021) 41:1231–5. doi: 10.13703/J.0255-2930.20201106-K0002
189. Fasina OB, Wang J, Mo J, Osada H, Ohno H, Pan W, et al. Gastrodin from gastrodia elata enhances cognitive function and neuroprotection of AD mice via the regulation of gut microbiota composition and inhibition of neuron inflammation. Front Pharmacol (2022) 13:814271. doi: 10.3389/FPHAR.2022.814271
190. Wu S, Wu Q, Wang J, Li Y, Chen B, Zhu Z, et al. Novel selenium peptides obtained from selenium-enriched cordyceps militaris alleviate neuroinflammation and gut microbiota dysbacteriosis in LPS-injured mice. J Agric Food Chem (2022) 70:3194–206. doi: 10.1021/ACS.JAFC.1C08393
191. Xiong W, Zhao X, Xu Q, Wei G, Zhang L, Fan Y, et al. Qisheng wan formula ameliorates cognitive impairment of alzheimer’s disease rat via inflammation inhibition and intestinal microbiota regulation. J Ethnopharmacol (2022) 282:114598. doi: 10.1016/J.JEP.2021.114598
192. Zhou H, Zhao J, Liu C, Zhang Z, Zhang Y, Meng D. Xanthoceraside exerts anti-alzheimer’s disease effect by remodeling gut microbiota and modulating microbial-derived metabolites level in rats. Phytomedicine (2022) 98:153937. doi: 10.1016/J.PHYMED.2022.153937
193. Chen D, Yang X, Yang J, Lai G, Yong T, Tang X, et al. Prebiotic effect of fructooligosaccharides from morinda officinalis on alzheimer’s disease in rodent models by targeting the microbiota-Gut-Brain axis. Front Aging Neurosci (2017) 9:403. doi: 10.3389/FNAGI.2017.00403
194. Li G, Zhang N, Geng F, Liu G, Liu B, Lei X, et al. High-throughput metabolomics and ingenuity pathway approach reveals the pharmacological effect and targets of ginsenoside Rg1 in alzheimer’s disease mice. Sci Rep (2019) 9:7040. doi: 10.1038/S41598-019-43537-4
195. Li SP, Wang YW, Qi SL, Zhang YP, Deng G, Ding WZ, et al. Analogous β-carboline alkaloids harmaline and harmine ameliorate scopolamine-induced cognition dysfunction by attenuating acetylcholinesterase activity, oxidative stress, and inflammation in mice. Front Pharmacol (2018) 9:346. doi: 10.3389/FPHAR.2018.00346
196. Li J, Liu Y, Li W, Wang Z, Guo P, Li L, et al. Metabolic profiling of the effects of ginsenoside re in an alzheimer’s disease mouse model. Behav Brain Res (2018) 337:160–72. doi: 10.1016/J.BBR.2017.09.027
197. Zhang Z, Yi P, Yang J, Huang J, Xu P, Hu M, et al. Integrated network pharmacology analysis and serum metabolomics to reveal the cognitive improvement effect of bushen tiansui formula on alzheimer’s disease. J Ethnopharmacol (2020) 249:112371. doi: 10.1016/J.JEP.2019.112371
198. Gu X, Zhao H, Zhou J, Zhou Y, Wei X, Wang H, et al. Effects of Huang-Lian-Jie-Du decoction on oxidative stress and AMPK-SIRT1 pathway in alzheimer’s disease rat. Evid Based Complement Alternat Med (2020) 2020:6212907. doi: 10.1155/2020/6212907
199. Cheng X, Huang Y, Zhang Y, Zhou W. LW-AFC, a new formula from the traditional Chinese medicine liuwei dihuang decoction, as a promising therapy for alzheimer's disease: Pharmacological effects and mechanisms. Adv Pharmacol (2020) 87:159–77. doi: 10.1016/bs.apha.2019.10.005
200. Wang D, Wang P, Zhang R, Xi X. Efficacy and safety of xuefu zhuyu decoction combined with Western medicine for angina pectoris in coronary heart disease: A protocol for systematic review and meta-analysis. Med (Baltimore) (2020) 99(50):e23195. doi: 10.1097/MD.0000000000023195
201. Wang P, Xiong X, Li S. Efficacy and safety of a traditional Chinese herbal formula xuefu zhuyu decoction for hypertension: A systematic review and meta-analysis. Med (Baltimore) (2015) 94(42):e1850. doi: 10.1097/MD.0000000000001850
202. Zhang M, Huang W, Yuan D. Efficacy and safety of banxia xiexin decoction as a complementary treatment for gastric cancer: A protocol for systematic review and meta-analysis. Med (Baltimore) (2021) 100(17):e25747. doi: 10.1097/MD.0000000000025747
203. Zhang J, Yang C, Wei D, Li H, Leung EL, Deng Q, et al. Long-term efficacy of Chinese medicine bushen capsule on cognition and brain activity in patients with amnestic mild cognitive impairment. Pharmacol Res (2019) 146:104319. doi: 10.1016/j.phrs.2019.104319
Keywords: neuroendocrinology, alzheimer disease, traditional chinese medicine, hypothalamic–pituitary–adrenal axis (HPA) and hypothalamic-pituitary-gonad axis (HPG) axes, insulin, brain gut axis
Citation: Deng C, Chen H, Meng Z and Meng S (2022) Roles of traditional chinese medicine regulating neuroendocrinology on AD treatment. Front. Endocrinol. 13:955618. doi: 10.3389/fendo.2022.955618
Received: 29 May 2022; Accepted: 01 September 2022;
Published: 21 September 2022.
Edited by:
Xiaohua Li, Seventh People’s Hospital of Shanghai, ChinaReviewed by:
Yunxia Li, Huazhong University of Science and Technology, ChinaXudong Li, Beijing Tiantan Hospital, Capital Medical University, China
Copyright © 2022 Deng, Chen, Meng and Meng. This is an open-access article distributed under the terms of the Creative Commons Attribution License (CC BY). The use, distribution or reproduction in other forums is permitted, provided the original author(s) and the copyright owner(s) are credited and that the original publication in this journal is cited, in accordance with accepted academic practice. No use, distribution or reproduction is permitted which does not comply with these terms.
*Correspondence: Shengxi Meng, mengsx163@163.com
†These authors have contributed equally to this work and share first authorship