- 1Department of General Surgery, State Key Laboratory of Complex Severe and Rare Diseases, Peking Union Medical College Hospital, China Academy of Medical Science & Peking Union Medical College, Beijing, China
- 2Gastroenterology Department, State Key Laboratory of Complex Severe and Rare Diseases, Peking Union Medical College Hospital, China Academy of Medical Science & Peking Union Medical College, Beijing, China
- 3Clinical Nutrition Department, State Key Laboratory of Complex Severe and Rare Diseases, Peking Union Medical College Hospital, China Academy of Medical Science & Peking Union Medical College, Beijing, China
Background: Bile acids are important signaling molecules that might activate hypothalamic neurons. This study aimed to investigate possible changes in hypothalamic pro-opiomelanocortin (POMC) neurons after biliary diversion in diabetic rats.
Methods: Ten GK rats were randomly divided into the biliary diversion (BD) and sham groups. The glucose metabolism, hypothalamic POMC expression, serum bile acid profiles, and ileal bile acid-specific receptors of the two groups were analyzed.
Results: Biliary diversion improved blood glucose (P = 0.001) and glucose tolerance (P = 0.001). RNA-Seq of the hypothalamus showed significantly upregulated expression of the POMC gene (log2-fold change = 4.1, P < 0.001), which also showed increased expression at the protein (P = 0.030) and mRNA (P = 0.004) levels. The POMC-derived neuropeptide α-melanocyte stimulating hormone (α-MSH) was also increased in the hypothalamus (2.21 ± 0.11 ng/g, P = 0.006). In addition, increased taurocholic acid (TCA) (108.05 ± 20.62 ng/mL, P = 0.003) and taurodeoxycholic acid (TDCA) (45.58 ± 2.74 ng/mL, P < 0.001) were found in the BD group and induced the enhanced secretion of fibroblast growth factor-15 (FGF15, 74.28 ± 3.44 pg/ml, P = 0.001) by activating farnesoid X receptor (FXR) that was over-expressed in the ileum.
Conclusions: Hypothalamic POMC neurons were upregulated after BD, and the increased TCA, TDCA, and the downstream gut-derived hormone FGF15 might activate POMC neurons.
Introduction
Type 2 diabetes mellitus (T2DM) is a major public health problem that causes vascular, renal, and neurologic complications (1). The number of T2DM patients is rising worldwide, although there are many treatment approaches available to control diabetes, such as sulphonylureas and metformin, exercise and diet. T2DM still causes high rates of disability and mortality year by year because of poor compliance of patients (2). Therefore, the search for new diabetes therapies is undoubtedly an active area of research and development.
Several randomized controlled trials and animal experiments have shown that bariatric surgery, including Roux-en-Y gastric bypass (RYGB) (3), sleeve gastrectomy (SG) (4) and biliopancreatic diversion (BPD) (5), is not only highly effective at producing weight loss but also results in significant improvement in T2DM (6). Moreover, many bariatric surgeries have been recommended as antidiabetes interventions for people with T2DM (7) and obesity (8), supported by the 2nd Diabetes Surgery Summit (DSS-II) guidelines (9).
The role bile acids (BAs) has been widely recognized recently (10). They are not only involved in the digestion of lipids but also act as signaling molecules that play an important role in glucose metabolism (11). It has been reported that BA levels are elevated following bariatric surgeries and could be potential mediators that contribute to metabolic homeostasis (12). In addition, biliary diversion surgery aimed at increasing enterohepatic circulation of BAs has been found to improve glucose levels (13), which supports the metabolic role of BAs. Farnesoid X receptor (FXR) and Takeda G-protein-coupled receptor 5 (TGR5) in the ileum are the BA-specific receptors that can be activated, leading to the secretion of gut-derived hormones, such as fibroblast growth factor-15 (FGF15) (14, 15) and glucagon-like peptide-1 (GLP-1) (16−18). BAs and downstream signaling molecules can activate the corresponding receptors that are present in the rat hypothalamus to regulate energy and glucose homeostasis (19, 20). Intracerebroventricular administration of FGF19 (ortholog of FGF15 in humans) has been found to increase energy expenditure and improve glucose tolerance through binding to the FGF receptor (21) stably mediated by Klotho (a transmembrane glycoprotein) (22).
The hypothalamus is critical for maintaining metabolic homeostasis. In particular, pro-opiomelanocortin (POMC) -expressing neurons (23) can produce a derived peptide, alpha-melanocyte-stimulating hormone (a-MSH) (24), leading to the activation of melanocortin 4 receptors (MC4Rs) (25) to exert a powerful effect on whole-body glucose regulation. According to Kalsbeek et al.'s reports, T2DM patients showed a decrease in the number of POMC-immunoreactive neurons (26). In addition, the prevalence of a specific heterozygous mutation in POMC was significantly higher in patients with early-onset obesity than in normal-weight controls (27), and heterozygous mutations in POMC may interfere with the effectiveness of bariatric surgeries (28). However, whether bariatric surgery leads to changes in these hypothalamic POMC neurons and the underlying mechanisms are unclear and imprecise.
Therefore, in an attempt to determine the changes in hypothalamic POMC neurons and the unique role of BA-related signaling pathways after bariatric surgery, we performed biliary diversion to the terminal ileum in Goto-Kakizaki (GK) rats.
Materials and methods
Animals
In this study, 10 GK rats (12-week-old male) weighing approximately 320 g were purchased from Junke Bioengineering Co., Ltd. (Nanjing, P.R. China). GK rats are a nonobese model of T2DM that present typical metabolic characteristics similar to those of human diabetes. The GK rats were housed in a climate-controlled environment with a 12-h light/dark cycle and were fed a standard chow diet and tap water in the laboratory animal center before being randomly allocated to the biliary diversion (BD) group (5 rats) or the sham group (5 rats). In addition, because rats are coprophagic animals and feces contain considerable amounts of bile acids, the rats were individually housed in metabolic cages during the study (Supplementary Figure 1). All experiments and surgical preparations were performed according to protocols approved by the Experimental Animal Welfare Ethics Committee.
Operations
The rats were fasted overnight (12 hours) and anesthetized by peritoneal injection of pentobarbital sodium that suited for experiments that focus on measurement of metabolic parameters (29) (the pentobarbital sodium concentration was 1%, and the injection dose was 35-40 mg per kilogram body weight). The biliary diversion operation is to transfer the bile in the common bile duct to the distal ileum (4 cm proximal to the ileocaecal valve) through a sterilized polytetrafluoroethylene (PTFE) tube with an inner diameter of 0.3 mm and outer diameter of 0.6 mm (Figure 1A). The abdominal wall was closed using simple, interrupted 3-0 polypropylene sutures. The sham operation involved the same procedures except that the distal end of the tube was fixed to the duodenum (parallel to the level of the ampulla of Vater) so that these rats also experienced surgical stress without physiologic biliary diversion (Figure 1B). All rats were fasted and deprived of water within 12 hours after surgery. The rats started to drink 12 hours after surgery and were fed enteral nutrient solution (10% ENSURE® Enteral Nutritional Powder (TP), ABBOTT LABORATORIES B.V.) from 24 hours after surgery to the third postoperative day. The surviving rats were maintained on a regular chow diet and water after surgery until the endpoint measurements.
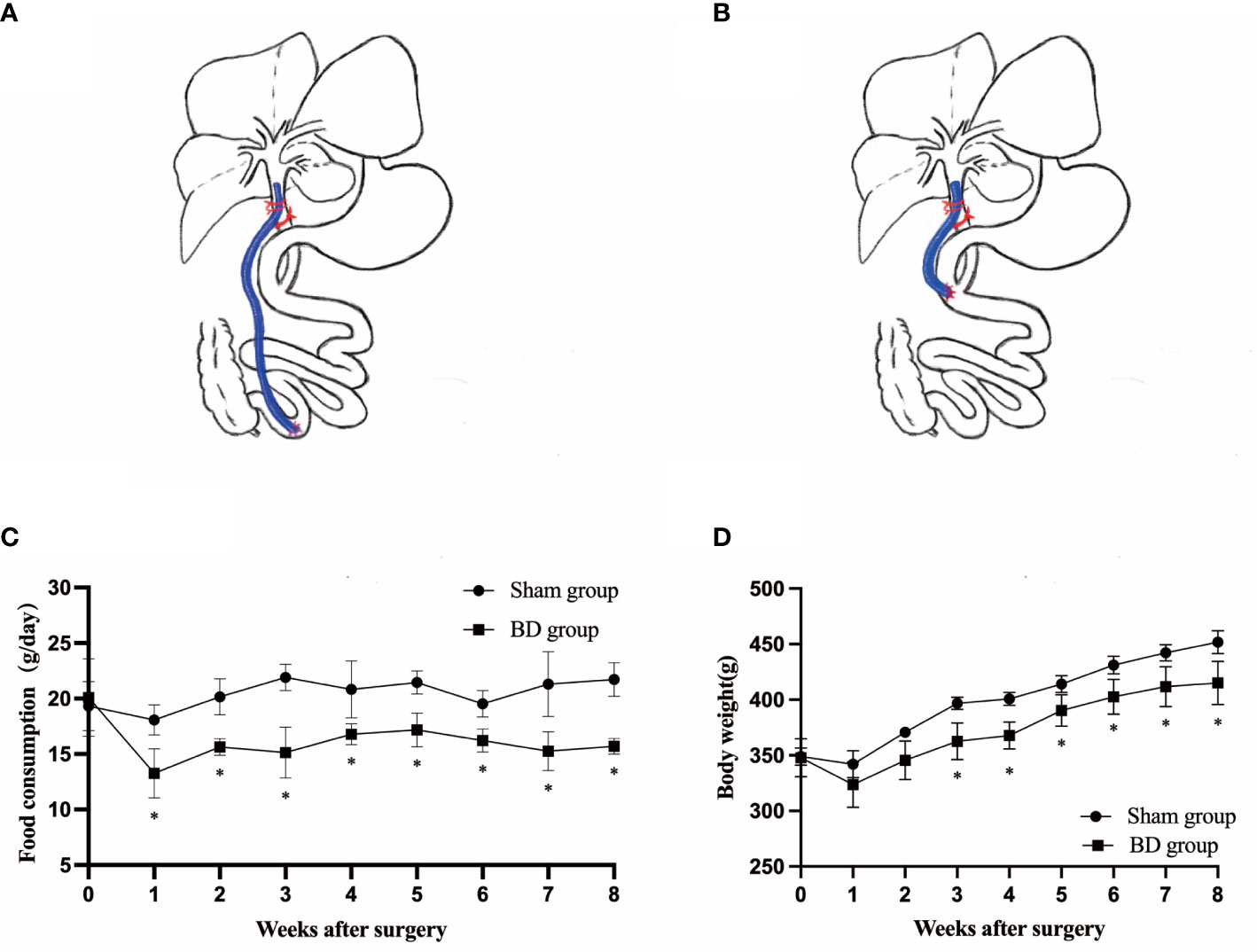
Figure 1 Surgical schematic and effects on body weight and food intake. (A) Sketch of biliary diversion of the common bile duct to the distal ileum. (B) Sketch of sham surgery with biliary diversion to the duodenum. (C) Average daily food intake in the two groups. (D) The body weight of the two groups. *P < 0.05.
Food consumption and body weight
Rats were pair-fed to maintain the same food intake between the two groups and reduce the potential impact of food on glucose metabolism. The maximum food intake for 24 hours was measured without restriction from 8 am to 8 am the next day. Body weight was measured at 8 am after an overnight fast using an electronic scale.
Glucose metabolism
Random blood glucose (RBG) levels in blood from the caudal vein were tested using a glucometer (Roche, Mannheim, Germany) at any time on any two days a week from 1 week before surgery to 8 weeks after surgery. Intraperitoneal glucose tolerance tests (IPGTT) were conducted before surgery and at 2, 4, 6, and 8 weeks after surgery. After fasting overnight (12 hours), the blood glucose levels of all rats were measured 0, 30, 60, 90 and 120 min using a glucometer after an intraperitoneal injection of dextrose (50%) at 2.0 mg/g body weight (30). The trapezoidal rule was used to determine the area under the curve (AUC) for the IPGTT (AUCIPGTT).
RNA-sequencing of the hypothalamus
The rats were euthanized by anesthesia overdose at 8 postoperative weeks after an overnight fast to obtain the hypothalamus. Total ribonucleic acid was extracted from hypothalamus samples using TRIzol reagent (Invitrogen, CA, USA) according to the manufacturer's instructions. Transcriptional sequencing was implemented based on Illumina methods. An Agilent 2100 bioanalyzer was used to determine the quality and quantity of the extracted RNA from the samples. Briefly, cDNA libraries were prepared using a NEBNext® UltraTM Directional RNA Library ep Kit for Illumina®, and paired-end sequencing was performed on an Illumina HiSeq2000 sequencer. Clean read data for subsequent analysis were obtained after filtration of the raw data, a sequencing error rate check and a GC content distribution check. The clean sequence reads were aligned to the rat genome (Rnor 6.0.104) using HISAT2 2.1.0. Differentially expressed genes (DEGs) were identified using featureCounts (2.0.1) and the DESeq2 package (1.34.0) and judged by log2 (fold-change) ≥ 1 or log2 (fold-change) ≤ −1 and a P-adjusted value of < 0.05. Kyoto Encyclopedia of Genes and Genomes (KEGG) pathway analysis was performed using the R package (v4.1.0). RNA-Seq clean data were uploaded to the National Center for Biotechnology Information Gene Expression Omnibus database with the accession number PRJNA795651.
Bile acid profiles
To identify the alteration in the bile acid profiles induced by biliary diversion, we measured and identified 18 bile acids in plasma samples collected from GK rats before surgery and 8 weeks postoperatively through targeted metabolomics analysis. At postoperative week 8, all rats were gavaged with 10% ENSURE® (1 ml/100 g body weight (31)) after fasting for 12 h. Blood was then collected from the angular vein at 2 hours after gavage. Targeted quantitative detection of serum bile acid was performed on an ultra-performance liquid chromatography-tandem mass spectrometry (UPLC−MS) platform (Waters Corporation, Milford, MA, USA) using the Waters ACQUITY UPLC I-class ULTRA high-performance liquid chromatography system combined with the Waters XEVO TQ-S tandem quadrupole mass spectrometry system. Data acquisition was carried out with MassLynx v 4.1 software (Waters Corporation, Milford, MA, USA).
Biochemical tests
Serum insulin and glucagon levels were analyzed using enzyme-linked immunosorbent assay (ELISA) kits (Solarbio Life Science, Beijing, P. R. China). Serum FGF15 was evaluated using a Rat FGF15 ELISA Kit (Solarbio Life Science, Beijing, P. R. China), and serum GLP-1 was analyzed using a Rat GLP-1 ELISA Kit (CUSABIO, Wuhan, P. R. China). Serum and hypothalamus (1 mg of tissue in 9 ml of phosphate-buffered saline) α-MSH levels were assessed using a Rat α-MSH ELISA Kit (FineTest, Wuhan, China). All kits were used according to the manufacturers' guidelines.
Western blotting
The hypothalamus and distal ileum were collected 8 weeks postsurgery and lysed in radioimmunoprecipitation assay buffer. Protein concentrations of the supernatant were quantified using the BCA Protein Assay Kit (Solarbio Life Science, Beijing, P. R. China), and equivalent amounts of protein were subjected to 10% sodium dodecyl sulfate−polyacrylamide gel electrophoresis and transferred to a 0.2 μm aperture polyvinylidene fluoride membrane. The membranes were blocked in 5% bovine serum albumin liquid and incubated with primary TGR5 (Abcam, Cambridge, UK), FXR (ABclonal, Wuhan, P.R. China), MC4R (ABclonal, Wuhan, P.R. China), Klotho (Proteintech, Wuhan, P.R. China), POMC (Wuhan, P.R. China) and β-actin (Cell Signaling Technology, Danvers, MA, USA) antibodies at 4 °C overnight. The membranes were washed three times with Tris-buffered saline and Tween 20, goat anti-rabbit (ABclonal, Wuhan, P.R. China) and goat anti-mouse (ABclonal, Wuhan, P.R. China) were incubated at room temperature for 1 hour with shaking. After three rinses with TBST solution, the membrane was scanned. The relative concentration of protein was quantified by densitometry using the Tanon Imaging System and ImageJ software.
Real-time qPCR
Complementary DNA (cDNA) was synthesized in a TaqMan-based assay from 5 μg of extracted total RNA. The primer sequences are listed in Table 1. Real-time quantitative polymerase chain reaction (qPCR) analysis was performed using SYBR Green (Roche) on a LightCycler® 480 instrument (Roche, Germany). The mRNA expression of GAPDH was used for normalization, and 2^-△△Ct values were analyzed to determine the relative mRNA expression levels.
Immunohistochemistry
Hypothalamic tissue was fixed in 4% paraformaldehyde for 24 hours. Then, these tissues were embedded in paraffin and cut into 4-μm slices. For immunohistochemistry (IHC), the slides were incubated with antibodies against POMC, MC4R and Klotho and goat anti-rabbit and goat anti-mouse antibodies. Images were acquired and analyzed using a microscope and Case Viewer 2.4 software.
Statistical analysis
The statistical analyses were performed using SPSS statistics software (version 25.0, IBM, Armonk, NY, USA) and Prism version 9 (GraphPad Prism, La Jolla, CA, USA). All quantitative data are expressed as the mean ± standard deviation. Student's t test (unpaired, two-tailed) and analysis of variance (ANOVA) test were used to compare the difference between the biliary diversion group and the sham group. The threshold for statistical significance was set at P < 0.05.
Results
Rat models
Biliary diversion and sham surgery were performed successfully in rat models. A BD rat died at postoperative week 2 because of intestinal obstruction due to abdominal adhesions, and a sham rat died at postoperative week 1 because of bile leakage. No other severe complications were observed.
Food intake and body weight
Before surgery, the daily food intake (20.10 ± 3.49 g vs. 19.33 ± 2.20 g, P = 0.722) and body weight (347.75 ± 17.24 g vs. 348.88 ± 7.77 g, P = 0.911) were not significantly different between the BD group (4 rats) and sham group (4 rats). The consumption in the BD group remained significantly lower than that in the sham group at postoperative week 8 (15.60 ± 0.59 g vs. 21.73 ± 1.52 g, P = 0.007, Figure 1C). The body weight of the BD group and the sham group was not statistically significant until the third week after surgery (362.50 ± 16.52 g vs. 396.75 ± 5.50 g, P = 0.020) Figure 1D and the difference between the two groups on food intake (P = 0.013) and body weight (P < 0.001) was also statistically significant over time through ANOVA test.
Effect on glucose metabolism
The RBG and the IPGTT were measured to evaluate the effects of biliary diversion on glucose homeostasis. The RBG levels in the BD group were significantly lower than those in the sham group (9.25 ± 0.72 mmol/L vs. 12.73 ± 0.84 mmol/L, P = 0.001) at postoperative week 8 (Figure 2A). The BD group also displayed improved glucose tolerance at 8 weeks postsurgery compared to the sham group (Figure 2B). In addition, the AUCIPGTT at 8 weeks (1729.00 ± 130.24 vs. 2292.00 ± 104.11, P = 0.001) postsurgery was significantly lower in the BD group than that in the sham group (Figure 2C), and the differences in RBG (P < 0.001) and AUCIPGTT (P < 0.001) between the two groups were also statistically significant over time. Moreover, the serum levels of insulin (0.29 ± 0.05 ng/ml vs. 0.28 ± 0.07 ng/ml, P = 0.823) and glucagon (100.26 ± 3.39 pg/ml vs. 103.63 ± 3.90 pg/ml, P = 0.269) did not differ significantly between the BD and sham groups (Figure 2D).
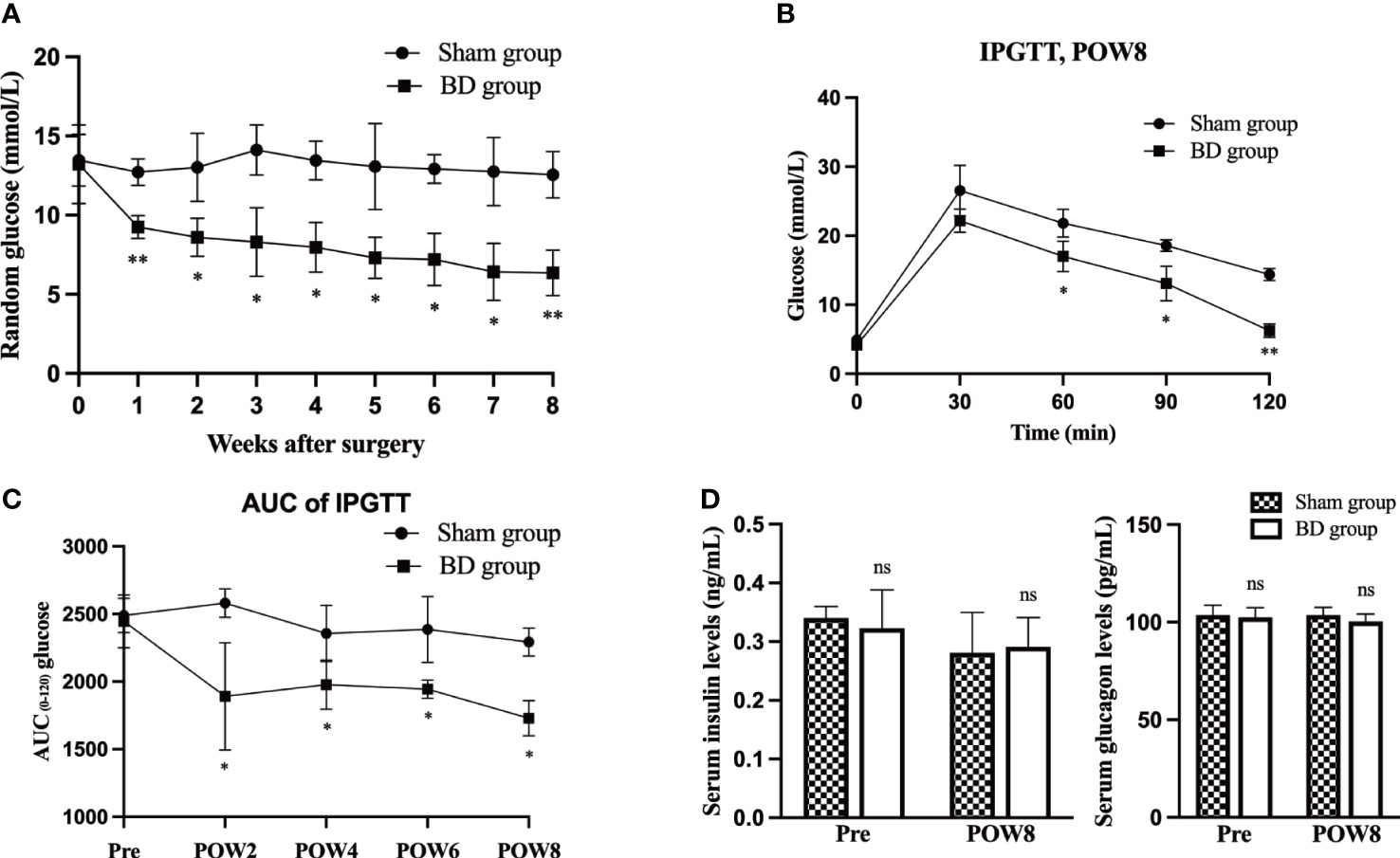
Figure 2 BD improved glucose homeostasis. (A) Random blood glucose (RBG) curves of the two groups presurgery and within 8 weeks after surgery. (B) Glycemic curves of intraperitoneal glucose tolerance tests (IPGTT) 8 weeks after surgery. (C) Area under the curve (AUC) measurements between 0 and 120 min in the IPGTT were calculated and compared between the two groups. (D) Serum insulin and glucagon levels in the two groups at presurgery and 8 weeks after surgery. *P < 0.05 and **P < 0.01. ns, not significant. POW, postoperative week. Pre, preoperation.
Changes in hypothalamic POMC neurons
Through RNA-seq of the hypothalamus (Figure 3A), we found that the POMC gene was significantly upregulated (log2-fold change = 4.1, P < 0.001) in the BD group compared to the sham group. According to KEGG pathway enrichment analysis (Figure 3A), the top four canonical pathways most increased in the BD group versus the sham group included cortisol synthesis and secretion, GABAergic synapse, aldosterone synthesis and secretion, and neuroactive ligand−receptor interaction signaling pathway.
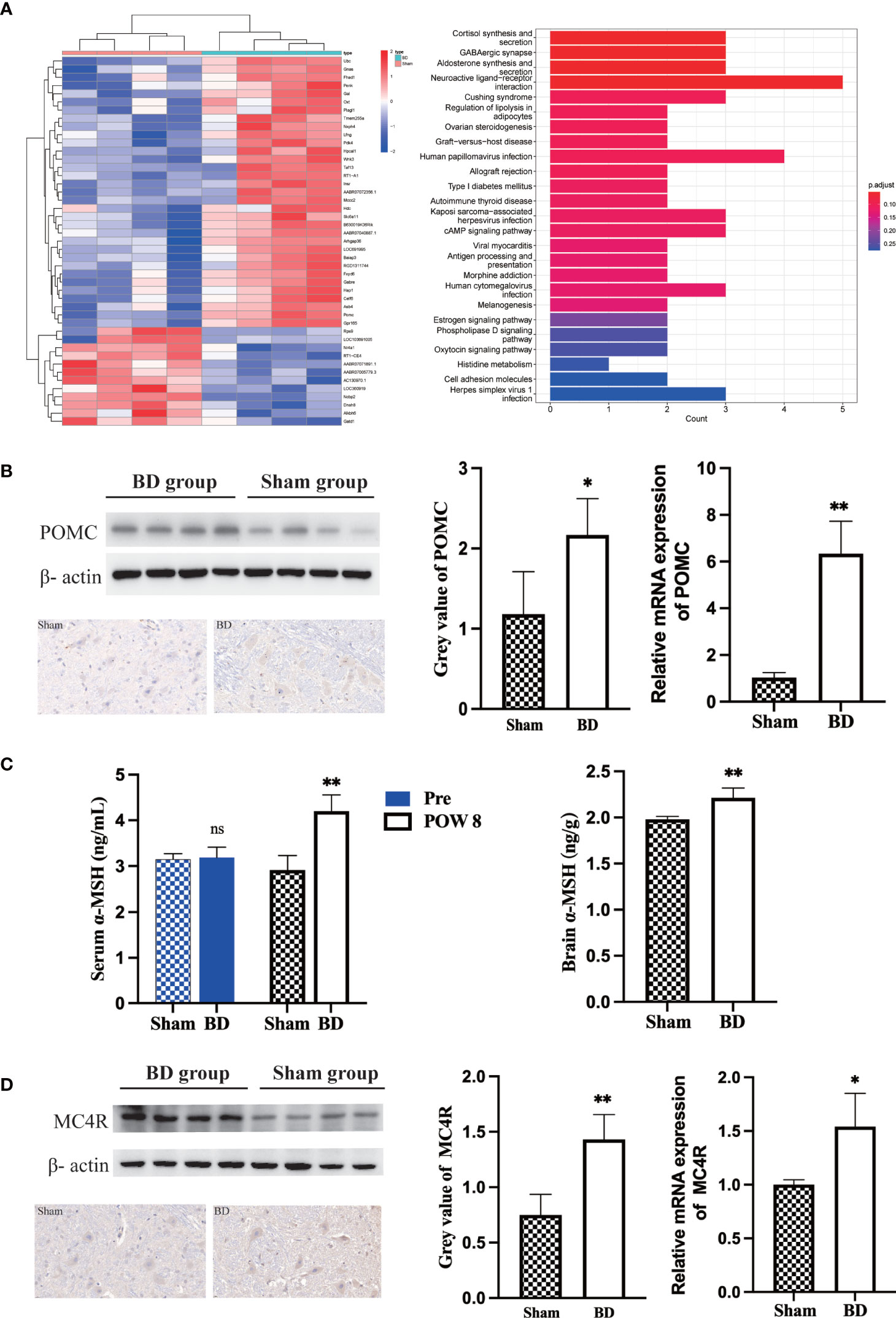
Figure 3 Biliary diversion altered hypothalamic gene expression and the related signaling pathway. (A) Left, clustered heatmap of the hypothalamic differentially expressed genes between the two groups. Right, the 25 most differentially expressed pathways between the two groups through KEGG analysis. (B) Western blots, IHC and RT−qPCR of POMC in the hypothalamus 8 weeks after surgery. (C) The concentration of α-MSH in the serum and hypothalamus 8 weeks after surgery in the two groups. (D) Western blots, IHC and RT−qPCR of MC4R in the hypothalamus 8 weeks after surgery. *P < 0.05. **P < 0.01. Scale bar = 10 μm. Sham, Sham group. BD, biliary diversion group.
The expression of POMC in the hypothalamus was measured by Western blotting. The relative expression level of hypothalamic POMC in the BD group (2.17 ± 0.45) was significantly higher than that in the sham group (1.18 ± 0.53, P = 0.030), and IHC also showed that the number of neuronal cells expressing POMC increased. For the BD group, RT−qPCR showed increased expression of the POMC gene in the hypothalamus (6.34 ± 1.29 vs. 1.03 ± 0.21, P = 0.004, Figure 3B). In addition, the concentrations of α-MSH, a POMC-derived neuropeptide, within the hypothalamus (2.21 ± 0.11 ng/g vs. 1.98 ± 0.03 ng/g, P = 0.006) and peripheral blood at 8 weeks postsurgery (4.20 ± 0.35 ng/mL vs. 2.91 ± 0.32 ng/mL, P = 0.002) were also significantly increased in the BD group (Figure 3C). The receptor of α-MSH in the hypothalamus-MC4R was also upregulated at the protein (1.43 ± 0.22 vs. 0.75 ± 0.19, P = 0.003) and mRNA levels (1.54 ± 0.31 vs. 1.00 ± 0.04, P = 0.038, Figure 3D).
Bile acid alteration and its effects on the ileum
The levels of serum total BA and 18 bile acid subclasses were not significantly different between the sham group and the BD group before surgery (Table 2). At 8 weeks after surgery, the levels of taurocholic acid (TCA, P = 0.003), taurodeoxycholic acid (TDCA, P < 0.001), and total bile acids (TBA, P = 0.035) were significantly higher in the BD group than in the sham group (Figure 4A).
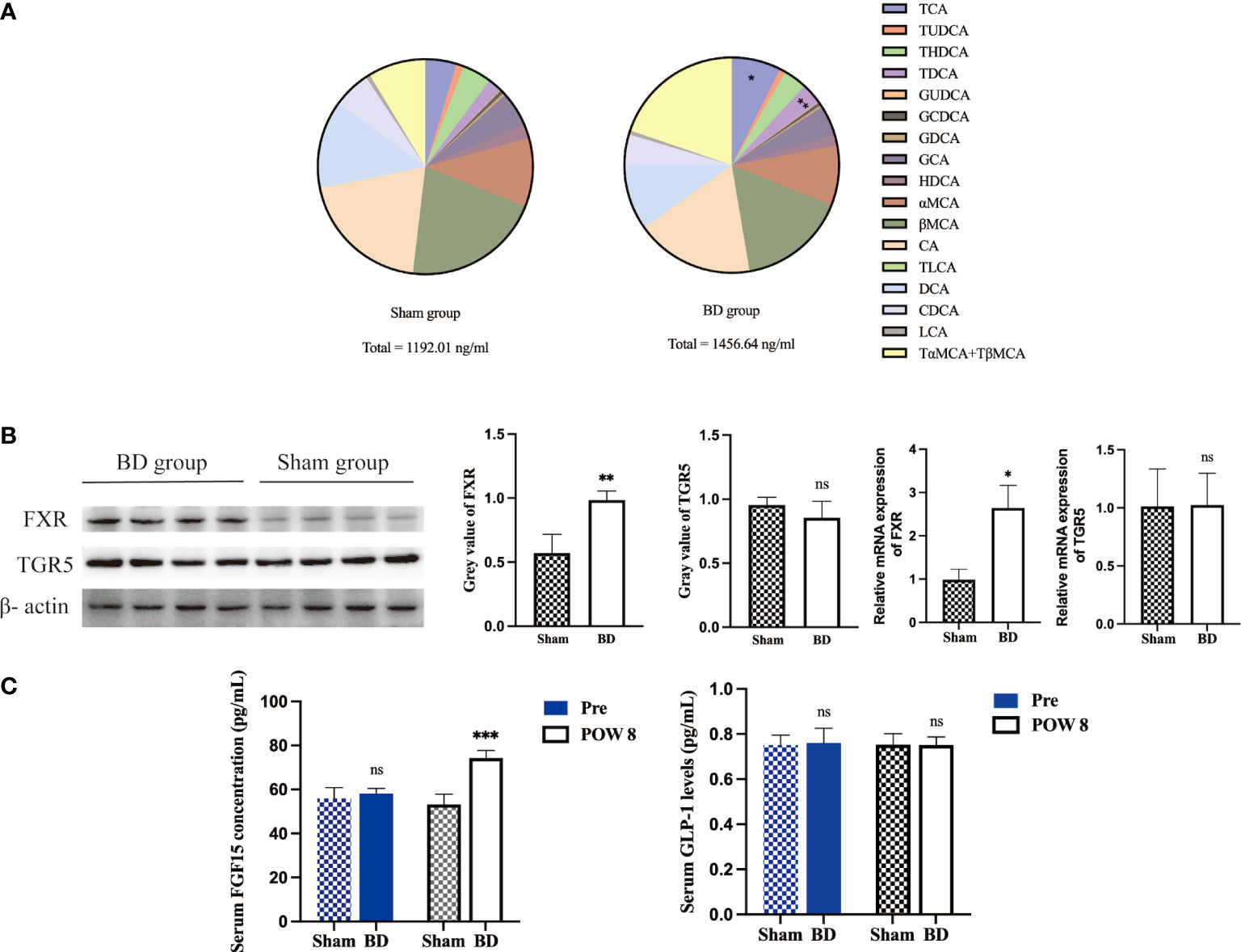
Figure 4 Altered bile acid pools and related signaling in the ileum after surgery. (A) Bile acid abundance and composition in the two groups 8 weeks after surgery. (B) Western blots and RT−qPCR of FXR and TGR5 in ileum tissue harvested from the two groups 8 weeks after surgery. (C) Serum FGF15 and GLP-1 concentrations presurgery and 8 weeks after surgery. *P < 0.05, **P < 0.01 and ***P < 0.001. ns, not significant. POW, postoperative week. Pre, preoperation. Sham, Sham group. BD, biliary diversion group.
The expression of the bile acid-specific receptors FXR and TGR5 in the ileum was measured by Western blotting and RT−qPCR. The expression of FXR at the protein level (0.98 ± 0.07 vs. 0.57 ± 0.15, P = 0.002) and mRNA level (2.65 ± 0.52 vs. 0.98 ± 0.25, P = 0.004) in the BD group increased, whereas that of TGR5 showed no significant change at the protein level (0.85 ± 0.13 vs. 0.95 ± 0.06, P = 0.209) and mRNA level (1.02 ± 0.27 vs. 1.01 ± 1.32, P = 0.956, Figure 4B). In addition, the BD group displayed increased serum levels of FGF15 (74.28 ± 3.44 pg/ml vs. 53.19 ± 4.72 pg/ml, P = 0.001), which is a gut-derived hormone induced by FXR agonists, compared to the sham group. However, the serum GLP-1 concentration showed no significant differences between the two groups (0.75 ± 0.04 pg/ml vs. 0.75 ± 0.05 pg/ml, P = 0.981, Figure 4C).
BAs-related receptors in the hypothalamus
Given the increased level of bile acids and upregulation of POMC genes, bile acid signaling proteins in the hypothalamus were measured. The expression of BA-specific receptors FXR and TGR5 in the hypothalamus at the protein level (0.78 ± 0.01 vs. 0.82 ± 0.04, P = 0.570; 0.84 ± 0.06 vs. 0.87 ± 0.08, P = 0.059) and mRNA level (0.81 ± 0.23 vs. 0.94 ± 0.21, P = 0.413; 0.97 ± 0.23 vs. 0.98 ± 0.17, P = 0.949) was not significantly changed between the BD group and the sham group (Figure 5A). In addition, there was also no change in the expression of these two receptors at the gene level, which refuted the hypothesis that specific bile acids that crossed the blood−brain barrier act directly on the hypothalamus.
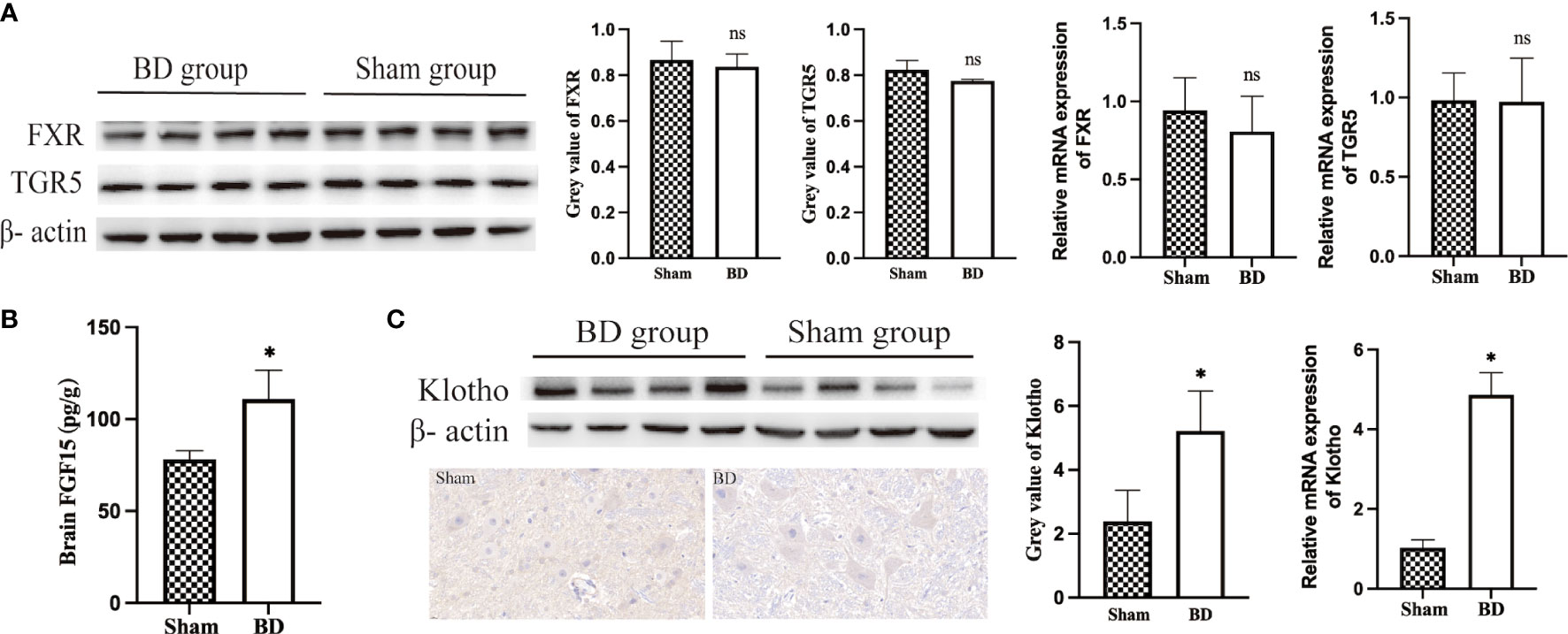
Figure 5 Bile acids and FGF15 receptors in the hypothalamus. (A) Western blots and RT−qPCR of bile acid receptors in the hypothalamus harvested from the two groups 8 weeks after surgery. (B) FGF15 concentration in brain tissue. (C) Western blots, IHC and RT−qPCR of Klotho in the hypothalamus harvested from the two groups 8 weeks after surgery. *P < 0.05. ns, not significant. Scale bar = 10 μm. Sham, Sham group. BD, biliary diversion group.
The BD group displayed significantly increased levels of FGF15 in serum (Figure 4C) and brain (110.91 ± 15.69 pg/g vs. 78.21 ± 4.58 pg/g, P = 0.007, Figure 5B), which could bind to the coreceptor Klotho in the hypothalamus. In view of the results of RNA-Seq that Klotho did not show a significant difference between the two groups, we detected the expression of Klotho through WB and RT−qPCR. Compared with the sham group, the BD group showed significantly increased expression of Klotho at the protein (5.22 ± 1.25 vs. 2.39 ± 0.97, P = 0.012) and mRNA levels (4.87 ± 0.56 vs. 1.03 ± 0.20, P < 0.001, Figure 5C). Moreover, IHC staining also showed that the expression of Klotho was increased in hypothalamic cells in the BD group compared with the sham group.
Discussion
Biliary diversion resulted in improvements in glucose homeostasis and reduced food consumption and body weight of GK rats, which agreed with previous findings (32, 33). The levels of RBG and AUCIPGTT in the BD group decreased significantly after surgery and were lower than those in the sham group at postoperative week 8. The model procedure eliminated other factors that may affect metabolism, such as stomach capacity (34), alimentary path and pancreatic juice.
The hypothalamus is an appetite regulation center, and we found that anorexigenic (appetite-reducing) POMC neurons were upregulated at the gene, mRNA and protein levels through RNA-seq, RT−qPCR, WB and IHC. Over the years, the recognition of the importance of the hypothalamus in glucose metabolism and appetite regulation has inspired a new wave of research on neural pathways (35). POMC neurons, as one of the most intensively studied populations of hypothalamic neurons, have been found to express receptors corresponding to different circulatory factors, such as leptin (36), insulin (37), PYY (38) and GLP-1/2 (39). Some animal and clinical studies have focused on the abovementioned gut hormones and reported related alterations caused by bariatric surgery (40) (41). Our results showed that the serum levels of GLP-1, its upstream receptor TGR5, and its downstream molecule insulin were not significantly changed in the BD group, which may be because biliary diversion to the ileum did not change the rate of nutrient entry into the intestine (42), which suggests that there are other signaling molecules and pathways responsible for the upregulation of the POMC gene, such as the bile acids that have significantly changed after bariatric surgery.
Several studies have identified the metabolic benefit of increased bile acid through FXR and TGR5 in bariatric surgery. Through bile acid-targeted metabolomics, the serum concentrations of TCA and TDCA were found to be significantly increased in the BD group (43). TCA and TDCA were identified as FXR agonists (44), and the activation of FXR signaling potently induced gut-derived hormone secretion and improved glucose homeostasis in subjects who received RYGB (45) and SG (46). The expression of FXR in the distal ileum was significantly increased in the BD group. In addition, the serum concentrations of FGF15, which is secreted from the intestine into the blood induced by the activation of FXR, were also increased. This proved that elevated TCA and TDCA levels upregulated the intestinal FXR-FGF15 signaling pathway. However, among the two identified bile acid subtypes of FXR agonists, which subtype acts as the determinant in the regulation of glucose metabolism was not determined. Thus, the effect of altered bile acids observed in the BD group was predominantly on the bile acid-specific receptor FXR and the downstream of the intestinal endogenous molecule FGF15.
FGF15/19, as an intestinal hormone, has been shown to regulate glucose homeostasis (47) by binding to FGF receptors and the coreceptor Klotho, which are located in different tissues, such as the liver (48), adipose tissue (49), and the brain (50). Some studies have reported that FGF19 exerts insulin-like activity that increases protein and glycogen synthesis independent of insulin through the FGF19-βKlotho axis in the liver (51, 52). Recent studies focused on the mechanism of the metabolic benefits of FGF15 indicated that FGF15 suppressed glucagon secretion (53) and silenced hypothalamic AGRP/NPY neurons (54), and the beneficial metabolic effects of FGFs on body weight and insulin sensitivity were absent in mice lacking Klotho in the nervous system (55). Our results showed that the level of FGF15 was significantly increased and that the coreceptor Klotho was also highly expressed in the hypothalamus. Therefore, it is reasonable to assume that the increased FGF15 was associated with the upregulation of POMC neurons.
POMC-derived peptide, α-MSH, is a well-known regulator of the central nervous system that can bind to and activate MC4R neurons (56), which leads to appetite inhibition and body weight loss (56) and is responsible for improved glucose homeostasis (57). Our results suggest that bile diversion may related to the activated hypothalamic POMC-α-MSH-MC4R signaling pathway, which exerts appetite-reducing effects that have been well accepted. In addition, BD not only increased the concentration of α-MSH in hypothalamic tissues but also increased α-MSH levels in the peripheral circulatory system. Peripheral α-MSH increases glucose uptake in skeletal muscles and enhances glucose clearance by activating muscle MC5R and protein kinase A (58). Subsequently, Rodrigues AR et al. (59) found that α-MSH promoted white adipose tissue (WAT) browning and upregulated insulin-dependent glucose transporter type 4 (GLUT4) in WAT, which also increased glucose clearance.
There are a few limitations in our study. One major limitation is that our animal and molecular studies do not allow us to identify the causal relation of increased BAs, FGF15 and the upregulation of the POMC gene in the hypothalamus. More studies are needed to investigate the underlying neuronal circuitry engaged by FGF15, such as the antagonism of FGF receptors or BAs. In addition, the other limitations of animal experiments is species differences and the small sample size. Thus, predicting the potential pathways in humans based on the results of GK rats is complicated, and more research and additional data are needed to prove the underlying glucose homeostatic mechanism of bariatric surgery in the future on the basis of an enlarged sample size.
In conclusion, biliary diversion to the distal ileum led to decreased appetite and weight loss, as well as sustained hyperglycemia improvements in GK rats. POMC neurons are significantly overexpressed in the hypothalamus and might be activated by increased TCA, TDCA, and FGF15 levels after BD.
Data availability statement
The original contributions presented in the study are included in the article/Supplementary Material, further inquiries can be directed to the corresponding author. The datasets of RNA-Seq in this study can be found in online repositories. The names of the repository/repositories and accession number(s) can be found below: https://dataview.ncbi.nlm.nih.gov/object/PRJNA795651.
Ethics statement
The animal study was reviewed and approved by Experimental Animal Welfare Ethics Committee of Peking Union Medical College Hospital.
Author contributions
SZ, WJC, and XH contributed to conception and design of the study. SZ, XB, QX, JC, and LD organized the database and performed the statistical analysis. SZ wrote the first draft of the manuscript. WC and QQ wrote sections of the manuscript. All authors contributed to the article and approved the submitted version.
Funding
This work was supported by the Program Focus Health of Liver and Gallbladder in Elder (ZYJ201912), China Academy of Medical Science Innovation Fund for Medical Sciences (2021-1-I2M-022) and National Natural Science Foundation of China (81970763).
Acknowledgments
We would like to thank Dr Kegong Tang and his colleagues, State Key Laboratory of Medical Molecular Biology, Institute of Basic Medical Sciences, Chinese Academy of Medical Sciences, Department of Biochemistry, Peking Union Medical College, for their invaluable technical efforts related to this project and the usage of the basic experimental facilities.
Conflict of interest
The authors declare that the research was conducted in the absence of any commercial or financial relationships that could be construed as a potential conflict of interest.
Publisher’s note
All claims expressed in this article are solely those of the authors and do not necessarily represent those of their affiliated organizations, or those of the publisher, the editors and the reviewers. Any product that may be evaluated in this article, or claim that may be made by its manufacturer, is not guaranteed or endorsed by the publisher.
Supplementary material
The Supplementary Material for this article can be found online at: https://www.frontiersin.org/articles/10.3389/fendo.2022.999928/full#supplementary-material
Supplementary Figure 1 | Metabolic cages used for housing GK rats.
References
2. Nauck MA, Wefers J, Meier JJ. Treatment of type 2 diabetes: challenges, hopes, and anticipated successes. Lancet Diabetes Endocrinol (2021) 9:525–44. doi: 10.1016/S2213-8587(21)00113-3
3. Osto E, Doytcheva P, Corteville C, Bueter M, Dorig C, Stivala S, et al. Rapid and body weight-independent improvement of endothelial and high-density lipoprotein function after roux-en-Y gastric bypass: role of glucagon-like peptide-1. Circulation (2015) 131:871–81. doi: 10.1161/CIRCULATIONAHA.114.011791
4. Jahansouz C, Xu H, Hertzel AV, Serrot FJ, Kvalheim N, Cole A, et al. Bile acids increase independently from hypocaloric restriction after bariatric surgery. Ann Surg (2016) 264:1022–8. doi: 10.1097/SLA.0000000000001552
5. Ferrannini E, Camastra S, Astiarraga B, Nannipieri M, Castro-Perez J, Xie D, et al. Increased bile acid synthesis and deconjugation after biliopancreatic diversion. Diabetes (2015) 64:3377–85. doi: 10.2337/db15-0214
6. Mingrone G, Panunzi S, De Gaetano A, Guidone C, Iaconelli A, Nanni G, et al. Bariatric-metabolic surgery versus conventional medical treatment in obese patients with type 2 diabetes: 5 year follow-up of an open-label, single-centre, randomised controlled trial. Lancet (2015) 386:964–73. doi: 10.1016/S0140-6736(15)00075-6
7. Syn NL, Cummings DE, Wang LZ, Lin DJ, Zhao JJ, Loh M, et al. Association of metabolic-bariatric surgery with long-term survival in adults with and without diabetes: a one-stage meta-analysis of matched cohort and prospective controlled studies with 174 772 participants. Lancet (2021) 397:1830–41. doi: 10.1016/S0140-6736(21)00591-2
8. Mingrone G, Panunzi S, De Gaetano A, Guidone C, Iaconelli A, Capristo E, et al. Metabolic surgery versus conventional medical therapy in patients with type 2 diabetes: 10-year follow-up of an open-label, single-centre, randomised controlled trial. Lancet (2021) 397:293–304. doi: 10.1016/S0140-6736(20)32649-0
9. Rubino F, Nathan DM, Eckel RH, Schauer PR, Alberti KG, Zimmet PZ, et al. Metabolic surgery in the treatment algorithm for type 2 diabetes: A joint statement by international diabetes organizations. Diabetes Care (2016) 39:861–77. doi: 10.2337/dc16-0236
10. Ahlin S, Cefalo C, Bondia-Pons I, Capristo E, Marini L, Gastaldelli A, et al. Bile acid changes after metabolic surgery are linked to improvement in insulin sensitivity. Br J Surg (2019) 106:1178–86. doi: 10.1002/bjs.11208
11. Browning MG, Pessoa BM, Khoraki J, Campos GM. Changes in bile acid metabolism, transport, and signaling as central drivers for metabolic improvements after bariatric surgery. Curr Obes Rep (2019) 8:175–84. doi: 10.1007/s13679-019-00334-4
12. Albaugh VL, Banan B, Ajouz H, Abumrad NN, Flynn CR. Bile acids and bariatric surgery. Mol Aspects Med (2017) 56:75–89. doi: 10.1016/j.mam.2017.04.001
13. Flynn CR, Albaugh VL, Cai S, Cheung-Flynn J, Williams PE, Brucker RM, et al. Bile diversion to the distal small intestine has comparable metabolic benefits to bariatric surgery. Nat Commun (2015) 6:7715. doi: 10.1038/ncomms8715
14. Ryan PM, Hayward NE, Sless RT, Garwood P, Rahmani J. Effect of bariatric surgery on circulating FGF-19: A systematic review and meta-analysis. Obes Rev (2020) 21:e13038. doi: 10.1111/obr.13038
15. Bozadjieva N, Heppner KM, Seeley RJ, Targeting FXR. And FGF19 to treat metabolic diseases-lessons learned from bariatric surgery. Diabetes (2018) 67:1720–8. doi: 10.2337/dbi17-0007
16. Chaudhari SN, Luo JN, Harris DA, Aliakbarian H, Yao L, Paik D, et al. A microbial metabolite remodels the gut-liver axis following bariatric surgery. Cell Host Microbe (2021) 29:408–424 e7. doi: 10.1016/j.chom.2020.12.004
17. Chaudhari SN, Harris DA, Aliakbarian H, Luo JN, Henke MT, Subramaniam R, et al. Bariatric surgery reveals a gut-restricted TGR5 agonist with anti-diabetic effects. Nat Chem Biol (2020) 17(1):20–9. doi: 10.1101/2020.01.10.902346
18. Scholtz S, Miras AD, Chhina N, Prechtl CG, Sleeth ML, Daud NM, et al. Obese patients after gastric bypass surgery have lower brain-hedonic responses to food than after gastric banding. Gut (2014) 63:891–902. doi: 10.1136/gutjnl-2013-305008
19. Castellanos-Jankiewicz A, Guzman-Quevedo O, Fenelon VS, Zizzari P, Quarta C, Bellocchio L, et al. Hypothalamic bile acid-TGR5 signaling protects from obesity. Cell Metab (2021) 33(7):1483–1492.e10. doi: 10.1016/j.cmet.2021.04.009
20. Fortin SM, Lipsky RK, Lhamo R, Chen J, Kim E, Borner T, et al. GABA neurons in the nucleus tractus solitarius express GLP-1 receptors and mediate anorectic effects of liraglutide in rats. Sci Transl Med (2020) 12(533):eaay8071. doi: 10.1126/scitranslmed.aay8071
21. Ryan KK, Kohli R, Gutierrez-Aguilar R, Gaitonde SG, Woods SC, Seeley RJ. Fibroblast growth factor-19 action in the brain reduces food intake and body weight and improves glucose tolerance in male rats. Endocrinology (2013) 154:9–15. doi: 10.1210/en.2012-1891
22. Jackson TC, Janesko-Feldman K, Carlson SW, Kotermanski SE, Kochanek PM. Robust RBM3 and beta-klotho expression in developing neurons in the human brain. J Cereb Blood Flow Metab (2019) 39:2355–67. doi: 10.1177/0271678X19878889
23. Harno E, Gali Ramamoorthy T, Coll AP, White A. POMC: The physiological power of hormone processing. Physiol Rev (2018) 98:2381–430. doi: 10.1152/physrev.00024.2017
24. Jais A, Bruning JC. Arcuate nucleus-dependent regulation of metabolism - pathways to obesity and diabetes mellitus. Endocr Rev (2021) 43(2):314–28. doi: 10.1210/endrev/bnab025
25. Krashes MJ, Lowell BB, Garfield AS. Melanocortin-4 receptor-regulated energy homeostasis. Nat Neurosci (2016) 19:206–19. doi: 10.1038/nn.4202
26. Kalsbeek MJ, Wolff SE, Korpel NL, la Fleur SE, Romijn JA, Fliers E, et al. The impact of antidiabetic treatment on human hypothalamic infundibular neurons and microglia. JCI Insight 5 (2020) 5(16):e133868. doi: 10.1172/jci.insight.133868
27. Challis BG, Pritchard LE, Creemers JW, Delplanque J, Keogh JM, Luan J, et al. A missense mutation disrupting a dibasic prohormone processing site in pro-opiomelanocortin (POMC) increases susceptibility to early-onset obesity through a novel molecular mechanism. Hum Mol Genet (2002) 11:1997–2004. doi: 10.1093/hmg/11.17.1997
28. Poitou C, Puder L, Dubern B, Krabusch P, Genser L, Wiegand S, et al. Long-term outcomes of bariatric surgery in patients with bi-allelic mutations in LEPR, and MC4R genes. Surg Obes Relat Dis (2021) 17:1449–56. doi: 10.1016/j.soard.2021.04.020
29. Sano Y, Ito S, Yoneda M, Nagasawa K, Matsuura N, Yamada Y, et al. Effects of various types of anesthesia on hemodynamics, cardiac function, and glucose and lipid metabolism in rats. Am J Physiol Heart Circ Physiol (2016) 311:H1360–6. doi: 10.1152/ajpheart.00181.2016
30. Andrikopoulos S, Blair AR, Deluca N, Fam BC, Proietto J. Evaluating the glucose tolerance test in mice. Am J Physiol Endocrinol Metab (2008) 295:E1323–32. doi: 10.1152/ajpendo.90617.2008
31. Wei M, Shao Y, Liu QR, Wu QZ, Zhang X, Zhong MW, et al. Bile acid profiles within the enterohepatic circulation in a diabetic rat model after bariatric surgeries. Am J Physiol Gastrointest Liver Physiol (2018) 314:G537–46. doi: 10.1152/ajpgi.00311.2017
32. Poland JC, Schrimpe-Rutledge AC, Sherrod SD, Flynn CR, McLean JA. Utilizing untargeted ion mobility-mass spectrometry to profile changes in the gut metabolome following biliary diversion surgery. Anal Chem (2019) 91:14417–23. doi: 10.1021/acs.analchem.9b02924
33. Ray K. Surgery: Bile diversion comparable to bariatric surgery in mice. Nat Rev Gastroenterol Hepatol (2015) 12:488. doi: 10.1038/nrgastro.2015.130
34. Zhang X, Yu B, Yang D, Qiao Z, Cao T, Zhang P. Gastric volume reduction is essential for the remission of type 2 diabetes mellitus after bariatric surgery in nonobese rats. Surg Obes Relat Dis (2016) 12:1569–76. doi: 10.1016/j.soard.2016.04.018
35. Kim KS, Seeley RJ, Sandoval DA. Signalling from the periphery to the brain that regulates energy homestasis. Nat Neurosci. (2018) 19(4):185–96. doi: 10.1038/nrn.2018.8
36. da Silva AA, do Carmo JM, Hall JE. CNS regulation of glucose homeostasis: Role of the leptin-melanocortin system. Curr Diabetes Rep (2020) 20:29. doi: 10.1007/s11892-020-01311-1
37. Dodd GT, Decherf S, Loh K, Simonds SE, Wiede F, Balland E, et al. Leptin and insulin act on POMC neurons to promote the browning of white fat. Cell (2015) 160:88–104. doi: 10.1016/j.cell.2014.12.022
38. Batterham RL, Cowley MA, Small CJ, Herzog H, Cohen MA, Dakin CL, et al. Gut hormone PYY(3-36) physiologically inhibits food intake. Nature (2002) 418:650–4. doi: 10.1038/nature00887
39. Shi X, Zhou F, Li X, Chang B, Li D, Wang Y, et al. Central GLP-2 enhances hepatic insulin sensitivity via activating PI3K signaling in POMC neurons. Cell Metab (2013) 18:86–98. doi: 10.1016/j.cmet.2013.06.014
40. Tsoli M, Chronaiou A, Kehagias I, Kalfarentzos F, Alexandrides TK. Hormone changes and diabetes resolution after biliopancreatic diversion and laparoscopic sleeve gastrectomy: a comparative prospective study. Surg Obes Relat Dis (2013) 9:667–77. doi: 10.1016/j.soard.2012.12.006
41. Kawasaki T, Ohta M, Kawano Y, Masuda T, Gotoh K, Inomata M, et al. Effects of sleeve gastrectomy and gastric banding on the hypothalamic feeding center in an obese rat model. Surg Today (2015) 45:1560–6. doi: 10.1007/s00595-015-1135-1
42. Douros JD, Tong J, D'Alessio DA. The effects of bariatric surgery on islet function, insulin secretion, and glucose control. Endocr Rev (2019) 40:1394–423. doi: 10.1210/er.2018-00183
43. So SSY, Yeung CHC, Schooling CM, El-Nezami H. Targeting bile acid metabolism in obesity reduction: A systematic review and meta-analysis. Obes Rev (2020) 21:e13017. doi: 10.1111/obr.13017
44. Monteiro-Cardoso VF, Corliano M, Singaraja RR. Bile acids: A communication channel in the gut-brain axis. Neuromol Med (2021) 23:99–117. doi: 10.1007/s12017-020-08625-z
45. Li K, Zou J, Li S, Guo J, Shi W, Wang B, et al. Farnesoid X receptor contributes to bidy weight-independent improvements in glycemic control after Roux-en-Y gastric bypass surgery in diet-induced obese mice. Mol Metab. 37:100980. doi: 10.016/j.molmet.2020.100980
46. McGavigan AK, Garibay D, Henseler ZM, Chen J, Bettaieb A, Haj FG, et al. TGR5 contributes to glucoregulatory improvements after vertical sleeve gastrectomy in mice. Gut (2017) 66:226–34. doi: 10.1136/gutjnl-2015-309871
47. Degirolamo C, Sabba C, Moschetta A. Therapeutic potential of the endocrine fibroblast growth factors FGF19, FGF21 and FGF23. Nat Rev Drug Discovery (2016) 15:51–69. doi: 10.1038/nrd.2015.9
48. Potthoff MJ, Boney-Montoya J, Choi M, He T, Sunny NE, Satapati S, et al. FGF15/19 regulates hepatic glucose metabolism by inhibiting the CREB-PGC-1alpha pathway. Cell Metab (2011) 13:729–38. doi: 10.1016/j.cmet.2011.03.019
49. Moron-Ros S, Uriarte I, Berasain C, Avila MA, Sabater-Masdeu M, Moreno-Navarrete JM, et al. FGF15/19 is required for adipose tissue plasticity in response to thermogenic adaptations. Mol Metab (2021) 43:101113. doi: 10.1016/j.molmet.2020.101113
50. Morton GJ, Matsen ME, Bracy DP, Meek TH, Nguyen HT, Stefanovski D, et al. FGF19 action in the brain induces insulin-independent glucose lowering. J Clin Invest (2013) 123:4799–808. doi: 10.1172/JCI70710
51. Kir S, Beddow SA, Samuel VT, Miller P, Previs SF, Suino-Powell K, et al. FGF19 as a postprandial, insulin-independent activator of hepatic protein and glycogen synthesis. Science (2011) 331:1621–4. doi: 10.1126/science.1198363
52. Kuro OM. The klotho proteins in health and disease. Nat Rev Nephrol (2019) 15:27–44. doi: 10.1038/s41581-018-0078-3
53. Picard A, Metref S, Tarussio D, Dolci W, Berney X, Croizier S, et al. Fgf15 neurons of the dorsomedial hypothalamus control glucagon secretion and hepatic gluconeogenesis. Diabetes (2021) 70:1443–57. doi: 10.2337/db20-1121
54. Liu S, Marcelin G, Blouet C, Jeong JH, Jo YH, Schwartz GJ, et al. A gut-brain axis regulating glucose metabolism mediated by bile acids and competitive fibroblast growth factor actions at the hypothalamus. Mol Metab (2018) 8:37–50. doi: 10.1016/j.molmet.2017.12.003
55. Owen BM, Mangelsdorf DJ, Kliewer SA. Tissue-specific actions of the metabolic hormones FGF15/19 and FGF21. Trends Endocrinol Metab (2015) 26:22–9. doi: 10.1016/j.tem.2014.10.002
56. Israeli H, Degtjarik O, Fierro F, Chunilal V, Gill AK, Roth NJ, et al. Structure reveals the activation mechanism of the MC4 receptor to initiate satiation signaling. Science (2021) 372:808–14. doi: 10.1126/science.abf7958
57. Zechner JF, Mirshahi UL, Satapati S, Berglund ED, Rossi J, Scott MM, et al. Weight-independent effects of roux-en-Y gastric bypass on glucose homeostasis via melanocortin-4 receptors in mice and humans. Gastroenterology (2013) 144:580–590 e7. doi: 10.1053/j.gastro.2012.11.022
58. Enriori PJ, Chen W, Garcia-Rudaz MC, Grayson BE, Evans AE, Comstock SM, et al. Alpha-melanocyte stimulating hormone promotes muscle glucose uptake via melanocortin 5 receptors. Mol Metab (2016) 5:807–22. doi: 10.1016/j.molmet.2016.07.009
Keywords: biliary diversion, bile acids, FGF15, POMC neurons, metabolism
Citation: Zhou S, Chen W, Bai X, Chen J, Xu Q, Dong L, Chen W, Qu Q and He X (2022) Upregulation of hypothalamic POMC neurons after biliary diversion in GK rats. Front. Endocrinol. 13:999928. doi: 10.3389/fendo.2022.999928
Received: 21 July 2022; Accepted: 16 September 2022;
Published: 07 October 2022.
Edited by:
Sanyuan Hu, Qianfoshan Hospital, Shandong University, ChinaReviewed by:
Yanmin Wang, California Medical Innovations Institute, United StatesYongtao Yu, General Hospital of Ningxia Medical University, China
Copyright © 2022 Zhou, Chen, Bai, Chen, Xu, Dong, Chen, Qu and He. This is an open-access article distributed under the terms of the Creative Commons Attribution License (CC BY). The use, distribution or reproduction in other forums is permitted, provided the original author(s) and the copyright owner(s) are credited and that the original publication in this journal is cited, in accordance with accepted academic practice. No use, distribution or reproduction is permitted which does not comply with these terms.
*Correspondence: Xiaodong He, hxdpumch@163.com