- 1Faculty of Medicine and Health Sciences, Norwegian University of Science and Technology (NTNU), Trondheim, Norway
- 2Department of Clinical and Molecular Medicine, Faculty of Medicine and Health Sciences, Norwegian University of Science and Technology (NTNU), Trondheim, Norway
- 3Center for Oral Health Services and Research, Mid-Norway (TkMidt), Trondheim, Norway
- 4Children’s Clinic, St. Olavs Hospital, Trondheim University Hospital, Trondheim, Norway
- 5Department of Rehabilitation Science and Health Technology, Faculty of Health Sciences, Oslo Metropolitan University, Oslo, Norway
- 6Department of Public Health and Nursing, Faculty of Medicine and Health Sciences, Norwegian University of Science and Technology (NTNU), Trondheim, Norway
- 7Department of Endocrinology, St. Olavs Hospital, Trondheim University Hospital, Trondheim, Norway
- 8Department of Obstetrics and Gynecology, St. Olavs Hospital, Trondheim University Hospital, Trondheim, Norway
- 9Clinic of Rehabilitation, St. Olavs Hospital, Trondheim University Hospital, Trondheim, Norway
Introduction: There is increasing evidence that the in utero environment affects the health and disease risk of offspring throughout their lives. The long-term effect of maternal hyperglycaemia on offspring glucose metabolism is of interest in a public health perspective. The aim of this study was to examine the association between in utero exposure to maternal glycaemia and offspring glucose metabolism.
Methods: Mother-child pairs were recruited from an RCT to prevent gestational diabetes mellitus where 855 healthy pregnant women were randomised to exercise or standard antenatal care. The original RCT detected no group differences in gestational diabetes mellitus prevalence or insulin resistance. The two groups were analysed as one group in the present study. Maternal glucose levels were assessed after 2-hour 75-gram oral glucose tolerance tests in pregnancy week ~34. Offspring outcomes were evaluated at ~9 years of age and included fasting glucose and homeostatic model assessment of insulin resistance. Multivariable regression models were performed, controlling for potential hereditary and lifestyle confounding factors.
Results: Complete data were available for 105 mother-child pairs. The regression analysis showed a positive association between maternal and offspring fasting glucose that was borderline significant (beta=0.18, 95% CI [-0.00027, 0.37], p=0.050). We did not find significant associations between maternal fasting glucose and offspring insulin resistance (beta=0.080, 95% CI [-0.087, 0.25], p=0.34), or between maternal 2-hour glucose and offspring fasting glucose (beta=0.016, 95% CI [-0.038, 0.070], p=0.56) or insulin resistance (beta=0.017, 95% CI [-0.032, 0.065], p=0.49).
Conclusions: Assessing a homogeneous group of healthy mother-child pairs, we found a borderline significant positive association between maternal and offspring fasting glucose, which persisted after adjustment for potential hereditary and lifestyle confounding factors. Our findings support other similar studies and highlight that improving the metabolic health of pregnant women, and women in childbearing age, should remain a key public health priority.
Clinical trial registration: ClinicalTrials.gov, identifier NCT00476567.
Introduction
There is increasing evidence that the in utero environment affects the health and disease risk of offspring throughout their lives (1–3). Hyperglycaemia and gestational diabetes mellitus (GDM) are common pregnancy complications, and consequences of different glycaemic levels in pregnancy have been widely studied. It is well established that GDM increases the risk of future type 2 diabetes in the mother and adverse pregnancy outcomes (4–6). Studies also indicate potential long-term effects on the offspring, including obesity, hypertension, and abnormal glucose metabolism (7–13). These associations are, however, less clear, and susceptible to confounders due to the long-term perspective and multifactorial aetiology.
The incidence and prevalence of type 2 diabetes among children and adolescents are increasing (14, 15), and associations between maternal glucose metabolism and offspring risk of childhood diabetes have been investigated. A large Canadian retrospective cohort study reported higher incidence of diabetes in offspring of GDM mothers, when adjusted for lifestyle confounders, but not hereditary factors (16). However, a systematic review by Kawasaki et al. did not detect increased risk of diabetes in children of mothers with GDM (10).
Childhood glucose metabolism is a more frequently studied outcome than childhood diabetes. As this is a strong predictor of future risk of type 2 diabetes (17–19), it is a highly relevant outcome to assess. The relationship between maternal and offspring glucose metabolism has been examined in multiple studies, but the conclusions are divergent, possibly due to methodologic differences and quality. Two systematic reviews reported an association between GDM and offspring glucose metabolism, but systematic adjustments for potential hereditary and lifestyle confounders were not performed (10, 20). Three small to medium-sized studies detected no independent association between maternal glycaemia and offspring fasting glucose (21, 22) or insulin resistance (21, 23). However, other studies report significant associations between maternal diabetes and offspring glucose metabolism persisting after adjustment for maternal and offspring BMI (24–26) and one when also adjusting for family history of diabetes (24). Notably, a large multicentre study including 4160 mother-child pairs, the Hyperglycaemia and Pregnancy Adverse Outcomes Follow-up Study (HAPO FUS), found associations between maternal and childhood glucose metabolism (7, 27), including levels of glycaemia below the diagnostic criteria of GDM (7). The findings were independent of family history of diabetes and maternal and offspring BMI, though not adjusted for potential lifestyle confounders. A separate follow-up study conducted from the Hong Kong HAPO cohort, also reported associations between maternal glucose levels and abnormal glucose tolerance in the offspring (11). These associations were independent of maternal pre-pregnancy BMI, children’s exercise level, and maternal and paternal diabetes status.
In the present study we aimed to investigate whether there is an association between in utero exposure to maternal glycaemia and offspring glucose metabolism at 9 years of age. We hypothesized that such an association would be present, consistent with the HAPO findings.
Materials and methods
Study design
Mother-child pairs were recruited from the RCT Training in pregnancy which was designed to investigate the effects of offering a regular exercise program during pregnancy on GDM prevalence (28). In this study no differences were observed between the intervention and control group in GDM prevalence or insulin resistance (28). Pregnant women booking appointments for their ~18-week routine ultrasound scans at St. Olavs Hospital (Trondheim University Hospital) and Stavanger University Hospital in Norway were invited to participate in the trial from 2007 to 2009. A total of 855 women were included, 660 in Trondheim and 195 in Stavanger. White women ≥ 18 years of age with a singleton live foetus who lived less than a 30-minute drive from the hospitals were eligible for inclusion. Exclusion criteria were history of diabetes or severe chronic diseases, known alcohol or drug misuse, previous severe pregnancy complications (preterm birth before 34 weeks, severe foetal growth retardation or severe preeclampsia with delivery before 34 weeks, eclampsia or HELLP syndrome), hypertension (systolic blood pressure ≥ 140 mmHg and/or diastolic blood pressure ≥ 90 mmHg) at antenatal care visit before study entry or foetal malformations, placenta previa or identified risk of preterm birth in current pregnancy (short cervix, amniotic fluid leakage or profuse vaginal bleeding with proven retroplacental hematoma) at ~18-week ultrasound scan.
The participating women were randomised to receive a 12-week exercise program or standard antenatal care. At the end of intervention (week 32–36 of pregnancy), participants were examined and underwent a 75-gram OGTT, where fasting and 2-hour glucose were analysed. Demographic and lifestyle characteristics were collected via questionnaires at two time points in pregnancy and 3 months post-partum. Pregnancy outcomes and newborn data were retrieved from medical charts after delivery.
At follow-up, questionnaire data were collected between October 2014 and December 2016 when the child was ~7 years old, by using the safe electronic solution CheckWare. Children and parents residing in Trondheim who had consented at the follow-up questionnaire to be invited to an in-person study visit, were invited to this study visit between November 2016 and December 2018, when the child was ~9 years old. The aim was assess the long-term effects on children’s health. For the women participating in this follow-up, we repeated the primary analyses from the original RCT of potential differences in GDM prevalence between the intervention and control group, using the revised WHO 2013 criteria (29), and supplemented by analysing for group differences in fasting and 2-hour glucose. If no new group differences were detected, the intervention and control group would be analysed as one cohort in this follow-up study.
Parents received written detailed information about the study. The children received a separate easy to read information letter, and parents and children were encouraged to discuss participation. The parents gave written consent on behalf of their children. The study was approved by The Regional Committee for Medical and Health Research Ethics in Central Norway (REK no. 2015/2028, application date 09-03-2016).
Outcomes
The primary outcome in this study was offspring glucose metabolism at ~9 years of age, as expressed by fasting serum glucose and estimated insulin resistance. At the study visit, fasting blood samples were collected from the children between 07:30 and 09:00. The blood samples were collected by standard venepuncture in vacuum tubes and sat for 30 minutes at room temperature before centrifugation (3000g/4°C/10 minutes). Sera were aliquoted and stored at -80°C until further analyses. Sera from all children were analysed simultaneously for glucose and insulin with accredited analyses at St. Olavs Hospital (Trondheim University Hospital) in September 2021. Insulin resistance was estimated by HOMA-IR according to the formula (fasting glucose in mmol/L)*(fasting insulin in pmol/L)/135 (30).
Predictors and covariates
Primary predictors were maternal fasting and 2-hour serum glucose following the OGTT at ~34 weeks of gestation (28). Covariates were chosen based on known associations with the predictors and outcomes from previous literature, and based on covariates chosen in similar studies to enable comparison with these studies. Children’s height and weight were measured at the ~9-year study visit. BMI was calculated as weight in kilograms divided by the square value of height in meters (kg/m2). Offspring BMI was further classified into iso-BMI categories, i.e. categories adjusted for age and sex, with the use of a calculator published by the Norwegian Institute of Public Health (31). Information about the child’s physical activity was collected by questionnaire at the ~7-year follow-up. Parents were asked if their child on average participated in moderate to vigorous physical activity ≥ 60 minutes per day (32), according to the WHO (33) and Norwegian Directorate of Health (34) recommendations for physical activity for children. Gestational age was estimated at the ~18-week routine ultrasound scan, and estimated gestational age at the OGTT was included as a covariate. Maternal pre-pregnancy BMI was calculated based on height and pre-pregnancy weight self-reported at study inclusion. Socioeconomic status was calculated based on the mother’s education and occupation reported in the questionnaire at study inclusion, according to Hollingshead Two-Factor Index of Social Position (35). Information about diabetes among the mother’s first-degree relatives (mother, father, siblings, or previous children) was collected at the questionnaire 3 months post-partum. The mother’s group allocation in the original RCT was also included as a covariate.
Statistical analyses
Descriptive statistics for continuous variables are presented with mean, standard deviation (SD) and minimum and maximum values. Categorical variables are presented with frequencies and percentages. Histograms and Q-Q plots for continuous variables were reviewed to assess normality, and maternal insulin and HOMA-IR were logarithmically transformed before further analyses due to non-normal distribution. Independent samples t-tests were used to analyse group differences for continuous variables. For categorical variables differences were analysed using Chi-square tests or Fishers exact tests as appropriate.
Simple and multivariable linear regression analyses were performed to assess associations between outcome and exposure variables. Mother-child pairs with missing values for one or more of the predictors, covariates, or outcomes were excluded from the regression analyses. Linearity was assessed by scatterplots, and multicollinearity was evaluated by reviewing pairwise correlations and variance inflation factors. The normality of residuals was assessed with residual plots. For the multivariable regression, covariate adjustments were performed in three separate models. Model 1 adjusted for offspring age (continuous), offspring sex (girl/boy), offspring physical activity (on average ≥ 60 minutes moderate to vigorous physical activity per day: yes/no), maternal group allocation in the original RCT (intervention group/control group), maternal socioeconomic position (continuous, class 1-5), maternal age (continuous), diabetes among first degree relatives of the mother (yes/no or uncertain) and gestational age at OGTT (continuous). Model 2 adjusted for the same variables as model 1, in addition to offspring BMI (continuous). Model 3 adjusted for the same variables as model 2, in addition to maternal pre-pregnancy BMI (continuous). Statistical analyses were performed using IBM SPSS Statistics version 28. P-values less than 0.05 were considered statistically significant.
Results
This 9-year follow-up study included a total of 118 mother-child pairs (Figure 1). This accounts for 18% of the 660 mothers who were included in the original RCT in Trondheim during pregnancy, and 74% of the 160 eligible mother-child pairs who were included in the 7-year follow-up and had given their consent to be invited to participate in an in-person study visit. 13 mother-child pairs were excluded from the regression analyses due to missing values of one or more of the predictors, covariates, or outcomes. The remaining 105 (16%) mother-child pairs were included. Of these, 59 mothers (56%) were randomised to the intervention group in the original RCT. When the glucose metabolisms of the women who participated in this follow-up study were analysed, we detected no differences between the intervention and control groups in terms of the prevalence of GDM (3 of 59 (5.1%) in the intervention group and 2 of 46 (4.3%) in the control group (p=0.86)), mean fasting glucose (4.3 mmol/L in both the intervention (SD=0.3) and the control (SD=0.4) group, p=0.50), or mean 2-hour glucose (5.5 mmol/L (SD=1.4) in the intervention group and 5.7 mmol/L (SD=1.2) in the control group, p=0.60). Therefore, the intervention and control groups from the original RCT were analysed as one cohort in this follow-up study.
Mother-child pairs who did and did not participate in the 9-year follow-up are compared in Table 1. Characteristics were similar in the two groups, except for a slightly higher maternal age (p=0.016), higher socioeconomic status (p=0.0021), and lower fasting glucose (p=0.022) among participants. Mean maternal fasting and 2-hour glucose was 4.3 mmol/L (SD ± 0.4) and 5.6 mmol/L (SD ± 1.3), respectively. Table 2 shows characteristics of the children at the ~9-year study visit and reported physical activity at the ~7-year follow-up. Mean fasting glucose was 4.7 mmol/L (SD ± 0.3), and mean HOMA-IR was 1.08 (SD ± 0.30).
Model diagnostics for linear regression analyses showed a good fit. Scatterplots indicated linearity between predictors and outcomes, and residual plots indicated reasonably normal distributions. Collinearity assessment showed pairwise correlations ranging between 0.0039 and 0.28 for covariates, except for the correlation between maternal fasting glucose and maternal pre-pregnancy BMI, which was 0.47. Although higher than ideal for the regression analyses, it was acceptable, and variance inflation factors were satisfactory; 1.31 for maternal fasting glucose and 1.36 for maternal pre-pregnancy BMI.
Results of the linear regression analyses are presented in Tables 3, 4. The analyses examined associations of maternal fasting and 2-hour glucose with offspring fasting glucose (Table 3) and offspring insulin resistance (Table 4). In the unadjusted analysis, a positive, though borderline significant association was observed between maternal and offspring fasting glucose. Adjusting for possible confounders and other covariates in model 1, and further adjustment for offspring BMI in model 2, strengthened the association. Adjusting for pre-pregnancy BMI in model 3, weakened the association. Other investigated associations were not significant in any of the models.
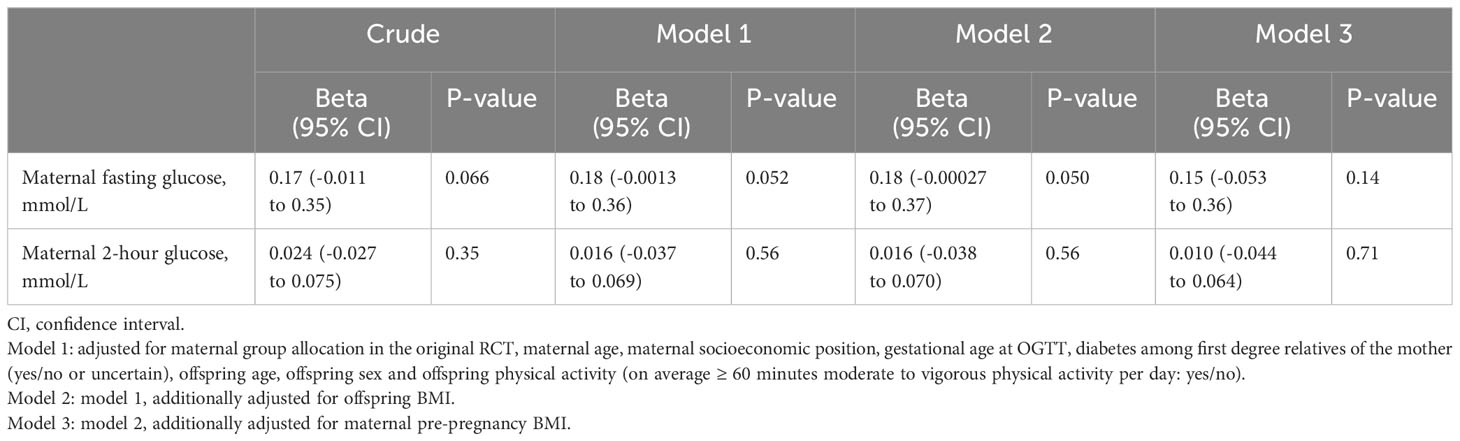
Table 3 Associations between maternal glucose and offspring fasting glucose (n=105 mother-child pairs).
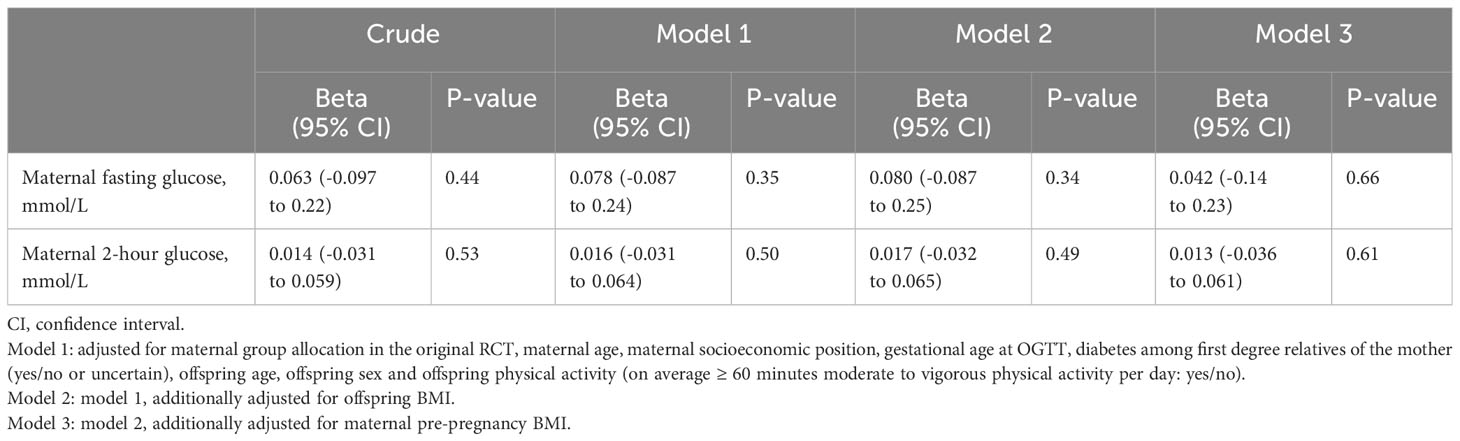
Table 4 Associations between maternal glucose and offspring insulin resistance (HOMA-IR) (n=105 mother-child pairs).
Discussion
Main findings
In this study, assessing 105 mother-child pairs, we observed a borderline significant positive association (beta=0.18, 95% CI [-0.00027, 0.37], p=0.050) between maternal and offspring fasting glucose when adjusted for potential confounders (model 2). The interpretation of this is that a 1 mmol/L increase in maternal fasting glucose, keeping everything else constant, would lead to a 0.18 mmol/L increase in offspring fasting glucose. We did not find significant associations between maternal fasting glucose and offspring insulin resistance, or between maternal 2-hour glucose and offspring fasting glucose or insulin resistance.
Strengths and limitations
This study has two main strengths in its investigation of the association between maternal and offspring metabolism. First, we examined serum levels of glucose and insulin which are objective measures of the offspring outcomes and maternal exposures. Second, a variety of relevant covariates were collected through study visits and questionnaires during pregnancy and at 7- and 9-year follow-ups, including both potential hereditary and lifestyle confounding factors.
There are inherent limitations to the analysis. The sample size is small, which limits the study’s power to demonstrate associations. Though associations between investigated exposures and outcomes were positive, confidence intervals were wide, and p-values did not meet the set significance level at < 0.05. Moreover, the study was not originally designed to examine the long-term effects of maternal hyperglycaemia in pregnancy, and no a-priori power calculation was performed. Though the study is generally well adjusted for confounders, we did not have access to relevant paternal factors, such as diabetes, BMI, and socioeconomic status.
Offspring fasting blood samples were analysed in this study. It is possible that glucose tolerance (1- or 2-hour glucose), obtained following a 75-gram glucose load, would be more sensitive in identifying abnormal glucose metabolism in children, as argued in a systematic review by Kawasaki et al. (10). However, the HAPO FUS detected associations between maternal 2-hour glucose and both offspring fasting and 2-hour glucose (7). The disadvantage of performing glucose tolerance tests is the increased discomfort for the participating children, and in this study, we therefore preferred fasting blood samples.
The OGTT was performed at ~34 weeks of gestation in the present study, in contrast to many other studies, and screening programs in most countries, where testing in week 24-28 is recommended. This is of importance when comparing results with other studies, as glucose metabolism changes during a normal pregnancy, with decreasing fasting glucose and increasing non-fasting glucose and insulin resistance with advancing gestation (36).
It is possible that the present study might be exposed to some degree of selection bias, in favour of healthier mother-child pairs. 74% of the eligible mother-child pairs from the 7-year follow-up participated in this 9-year follow-up study. However, only 18% of the mother-child pairs included in the original study in Trondheim participated in the present follow-up study. Participating mothers had higher socioeconomic position and lower third trimester fasting glucose than non-participants, though other characteristics were similar between the groups. It is also reasonable to assume that women who agreed to participate in the initial RCT might be healthier than the general population, as it was an exercise study. Compared with data from the medical birth registry of Norway (MBRN) in 2021 (37) the women in our study had slightly better metabolic health. The percentage of women with GDM (4.4% in this study and 6.3% in MBRN) and pre-pregnancy overweight or obesity (BMI ≥ 25) (21% in this study and 38% in MBRN) was lower in our study, even though MBRN utilized slightly stricter GDM criteria and OGTTs were performed earlier in pregnancy. Compared to the HAPO FUS participants (7), the women in the current study had slightly lower mean fasting glucose (4.3 mmol/L in this study and 4.5 mmol/L in HAPO FUS), lower mean 2-hour glucose (5.6 mmol/L in this study and 6.1 mmol/L in HAPO FUS), and a considerably lower percentage had GDM, 4.4%, vs 14.1% in HAPO FUS, using the same GDM criteria (29). Notably, the glucose analyses were performed at a mean gestational age of 28 weeks in the HAPO study, i.e. 6 weeks earlier than in the present study, indicating that the genuine difference in metabolic health might be even larger than displayed by these numbers. The present study and the HAPO FUS had different ethnic compositions, which may also have influenced the results.
Comparison with the ungKan3 study (38), reporting health and physical activity of Norwegian children in 2018, indicates that the children as well as the mothers in the present study are healthier than the Norwegian average. A considerably lower percentage in the present study were overweight or obese (6% in this study and 21% in ungKan3), both studies with a mean participant age of 9 years. The children in our study were, however, equally or slightly less physically active, with 66% in total meeting the WHO recommendation of ≥ 60 minutes of moderate to vigorous physical activity per day, vs 64% of the girls and 81% of the boys in the ungKan3 study. This difference might be caused by different measuring techniques (accelerometers in UngKan3 vs reported by parents in our study), as it has been shown that parents tend to under-report children’s physical activity (39). To conclude, the study population of both mothers and children seemed to be healthier than average and relatively homogeneous, which might have decreased the chance of detecting potential associations in our analyses. It may also decrease the generalizability of our findings to the general population. However, it would be unexpected for detected associations to be stronger when investigating a healthier study population.
Interpretation
In the HAPO FUS, significant associations between maternal and offspring glucose levels were detected, which were suggested by the authors to be clinically important, possibly contributing to the increasing prevalence of type 2 diabetes in children (7). In the present study comparable beta-estimates (in fully adjusted models, with child BMI, but without maternal BMI) were similar or higher than in the HAPO FUS. For the association between maternal and offspring fasting glucose, our beta-estimate of 0.18 (95% CI [-0.00027, 0.37]) was higher than in the HAPO FUS (0.042, 95% CI [0.031, 0.054]), and for the association between maternal 2-hour glucose and offspring fasting glucose, beta-estimates were similar (0.016 in the present study and 0.014 in the HAPO FUS). However, confidence intervals were wider in our study, as expected with a smaller sample size. Although borderline significant, our observed association between maternal and fasting glucose supports and aligns with the HAPO FUS findings.
The HAPO FUS observed the strongest associations between maternal and offspring fasting glucose, and maternal and offspring 2-hour glucose. The authors argued that this suggested that hereditary factors, not captured by family history, contributed to the associations (7). Consistent with HAPO FUS, the strongest association in our study was the association between maternal and offspring fasting glucose.
The children in our study were examined at ~9 years of age. There are indications that associations between maternal and offspring glucose metabolism might be more evident in older children. Most studies detecting significant associations have examined children older than in the current study (7, 16, 24–26, 40). The systematic review by Kawasaki et al. discovered significant associations between GDM and offspring fasting glucose among 20-year-olds, but not among 15-year-olds or 7-10-year-olds (10). However, the Hong Kong HAPO cohort found significant associations between maternal glucose levels and offspring risk of abnormal glucose tolerance among 7-year-old children (11).
When evaluating the relationship between maternal and offspring glucose metabolism it is relevant to assess whether an association is mediated by the in utero environment or by the influence of hereditary factors or postnatal environment. To assess the in utero exposure effects, the analyses in models 1-3 in this study were adjusted for potential confounding factors related to heredity or similar lifestyle. Diabetes among first-degree relatives of the mother was included in the analyses for this purpose. Socioeconomic status may be associated with lifestyle factors of both mother and child, which in turn may affect their respective glucose metabolisms, and several studies support the relationship between socioeconomic status and child metabolic health (41, 42). Physical activity of the children is also known to affect their glucose metabolism (43, 44), and may be associated with maternal glucose through similar mechanisms as socioeconomic status.
Previous literature does not provide clear evidence on how maternal BMI affects the relationship between maternal and offspring glucose metabolism. BMI is an established risk factor for hyperglycaemia in pregnancy (45). Several studies have also detected associations between pre-pregnancy BMI and offspring glucose levels, however, not after adjustment for offspring BMI (22, 26, 46, 47). This indicates that the relationship between pre-pregnancy BMI and offspring glucose levels is largely mediated by offspring BMI, and it is therefore not necessary to adjust for both maternal and offspring BMI. In the current study, analyses without pre-pregnancy BMI were performed in models 1 and 2, and the model 2 analyses were adjusted for offspring BMI to minimise the risk of confounding. Offspring BMI was included in the model 2 analyses with the additional purpose of examining if offspring BMI had a role as a mediator in the relationship between maternal and offspring glucose metabolism. In the HAPO FUS (7) and in the Effect of Preeclampsia On Cardiovascular Health (EPOCH) study (26), results were not attenuated when adjusting for offspring BMI, indicating that the associations were mediated by other mechanisms. Though insufficient to firmly conclude, the associations in our study were not attenuated when adjusting for offspring BMI, thus pointing in the same direction. Most studies investigating similar exposures and outcomes as this study have included maternal BMI or overweight in their analyses (7, 11, 21–24, 27), and maternal pre-pregnancy BMI was included in the model 3 analyses in our study to enable comparison with relevant studies. The model 3 analyses show that the associations were attenuated when adjusting for maternal BMI, but the reason cannot be determined with certainty. It is possible that the previously mentioned collinearity between maternal BMI and maternal fasting glucose, though below commonly used cut-of values, is affecting the precision of the model 3 analyses. The attenuated results might also be caused by maternal BMI mediating the association between maternal and offspring glucose levels.
In the current study, adjustments were also performed for selected additional covariates. Gestational age at the time of the OGTT was included as a covariate because of the known physiological changes in glucose metabolism over the course of pregnancy (36). Maternal age was considered a possible confounder because it affects the risk of hyperglycaemia in pregnancy (45) and might affect the long-term health of the offspring (48). Smoking during pregnancy and maternal hypertension were also assessed to be interesting covariates, but not included in the final analyses due to the few cases.
Other studies investigating associations between maternal and offspring glucose metabolism are generally less comprehensively adjusted than the present study, missing adjustments for hereditary and/or lifestyle confounding factors (7, 16, 21, 24–27, 40, 49). However, most of the studies conclude that in utero exposure probably constitutes part of the association between maternal and offspring glucose metabolism. This hypothesis is also supported by studies of Pima Indians, comparing the risk of diabetes between siblings born before and after their mother was recognised as having diabetes (50). Furthermore, it is important to note that the Hong Kong HAPO cohort found that maternal glucose levels were associated with the risk of offspring abnormal glucose tolerance, when adjusted for both lifestyle and hereditary factors (11). Though borderline significant and with a lower sample size, the results in the present study were not attenuated by adjustment for confounders, thus supporting previous studies.
Future perspectives
Previous literature and our study indicate that maternal hyperglycaemia negatively impact the offspring’s long-term health. Optimising metabolic health before conception and during pregnancy may therefore lead to offspring with improved metabolic health, and this should be a focus point for improving public health. Further studies should be performed to confirm the associations, with greater power and adjustment for as many covariates. Moreover, the effects of maternal interventions on long-term offspring outcomes are not yet well documented and future high-quality studies are needed to address this gap.
Conclusion
In the present study, assessing a small group of healthy mothers and children at low risk of metabolic diseases, we observed a borderline significant positive association between maternal and offspring fasting glucose, which persisted after adjustment for potential hereditary and lifestyle confounding factors. Our findings support other similar studies and highlight that improving the metabolic health of pregnant women, and women of childbearing age, should remain a key public health priority.
Data availability statement
The raw data supporting the conclusions of this article will be made available by the authors, without undue reservation.
Ethics statement
The studies involving humans were approved by The Regional Committee for Medical and Health Research Ethics in Central Norway. The studies were conducted in accordance with the local legislation and institutional requirements. Written informed consent for participation in this study was provided by the participants’ legal guardians/next of kin.
Author contributions
SN: Formal Analysis, Writing – original draft, Writing – review & editing. AS: Conceptualization, Data curation, Methodology, Supervision, Writing – review & editing. KE: Conceptualization, Data curation, Methodology, Writing – review & editing. TB: Data curation, Methodology, Writing – review & editing. US: Conceptualization, Data curation, Methodology, Writing – review & editing. KS: Methodology, Project administration, Writing – review & editing. SM: Conceptualization, Funding acquisition, Investigation, Methodology, Project administration, Writing – review & editing. SS: Conceptualization, Data curation, Formal Analysis, Funding acquisition, Investigation, Methodology, Project administration, Supervision, Writing – original draft, Writing – review & editing.
Funding
The author(s) declare financial support was received for the research, authorship, and/or publication of this article. The study was funded by the Norwegian Fund for Post-Graduate Training in Physiotherapy, the Joint Research Committee of St. Olavs Hospital HF and the Faculty of Medicine and Health Sciences, Norwegian University of Science and Technology (NTNU).
Acknowledgments
We would like to thank all women and children participating in this study.
Conflict of interest
The authors declare that the research was conducted in the absence of any commercial or financial relationships that could be construed as a potential conflict of interest.
Publisher’s note
All claims expressed in this article are solely those of the authors and do not necessarily represent those of their affiliated organizations, or those of the publisher, the editors and the reviewers. Any product that may be evaluated in this article, or claim that may be made by its manufacturer, is not guaranteed or endorsed by the publisher.
References
1. Godfrey KM, Reynolds RM, Prescott SL, Nyirenda M, Jaddoe VW, Eriksson JG, et al. Influence of maternal obesity on the long-term health of offspring. Lancet Diabetes Endocrinol (2017) 5(1):53–64. doi: 10.1016/S2213-8587(16)30107-3
2. Barbour LA. Changing perspectives in pre-existing diabetes and obesity in pregnancy: maternal and infant short- and long-term outcomes. Curr Opin Endocrinol Diabetes Obes (2014) 21(4):257–63. doi: 10.1097/MED.0000000000000079
3. Barker DJ, Thornburg KL. The obstetric origins of health for a lifetime. Clin Obstet Gynecol (2013) 56(3):511–9. doi: 10.1097/GRF.0b013e31829cb9ca
4. Wendland EM, Torloni MR, Falavigna M, Trujillo J, Dode MA, Campos MA, et al. Gestational diabetes and pregnancy outcomes–a systematic review of the World Health Organization (WHO) and the International Association of Diabetes in Pregnancy Study Groups (IADPSG) diagnostic criteria. BMC Pregnancy Childbirth (2012) 12:23. doi: 10.1186/1471-2393-12-23
5. Metzger BE, Lowe LP, Dyer AR, Trimble ER, Chaovarindr U, Coustan DR, et al. Hyperglycemia and adverse pregnancy outcomes. N Engl J Med (2008) 358(19):1991–2002. doi: 10.1056/NEJMoa0707943
6. Vounzoulaki E, Khunti K, Abner SC, Tan BK, Davies MJ, Gillies CL. Progression to type 2 diabetes in women with a known history of gestational diabetes: systematic review and meta-analysis. BMJ (2020) 369:m1361. doi: 10.1136/bmj.m1361
7. Scholtens DM, Kuang A, Lowe LP, Hamilton J, Lawrence JM, Lebenthal Y, et al. Hyperglycemia and adverse pregnancy outcome follow-up study (HAPO FUS): maternal glycemia and childhood glucose metabolism. Diabetes Care (2019) 42(3):381–92. doi: 10.2337/dc18-2021
8. Miranda JO, Cerqueira RJ, Barros H, Areias JC. Maternal diabetes mellitus as a risk factor for high blood pressure in late childhood. Hypertension (2019) 73(1):e1–7. doi: 10.1161/HYPERTENSIONAHA.118.11761
9. Lowe WL Jr., Lowe LP, Kuang A, Catalano PM, Nodzenski M, Talbot O, et al. Maternal glucose levels during pregnancy and childhood adiposity in the Hyperglycemia and Adverse Pregnancy Outcome Follow-up Study. Diabetologia (2019) 62(4):598–610. doi: 10.1007/s00125-018-4809-6
10. Kawasaki M, Arata N, Miyazaki C, Mori R, Kikuchi T, Ogawa Y, et al. Obesity and abnormal glucose tolerance in offspring of diabetic mothers: A systematic review and meta-analysis. PloS One (2018) 13(1):e0190676. doi: 10.1371/journal.pone.0190676
11. Tam WH, Ma RCW, Ozaki R, Li AM, Chan MHM, Yuen LY, et al. In utero exposure to maternal hyperglycemia increases childhood cardiometabolic risk in offspring. Diabetes Care (2017) 40(5):679–86. doi: 10.2337/dc16-2397
12. Bendor CD, Bardugo A, Rotem RS, Derazne E, Gerstein HC, Tzur D, et al. Glucose intolerance in pregnancy and offspring obesity in late adolescence. Diabetes Care (2022) 45(7):1540–8. doi: 10.2337/dc21-2634
13. Zhu Y, Olsen SF, Mendola P, Yeung EH, Vaag A, Bowers K, et al. Growth and obesity through the first 7 y of life in association with levels of maternal glycemia during pregnancy: a prospective cohort study. Am J Clin Nutr (2016) 103(3):794–800. doi: 10.3945/ajcn.115.121780
14. Mayer-Davis EJ, Lawrence JM, Dabelea D, Divers J, Isom S, Dolan L, et al. Incidence trends of type 1 and type 2 diabetes among youths, 2002-2012. N Engl J Med (2017) 376(15):1419–29. doi: 10.1056/NEJMoa1610187
15. Dabelea D, Mayer-Davis EJ, Saydah S, Imperatore G, Linder B, Divers J, et al. Prevalence of type 1 and type 2 diabetes among children and adolescents from 2001 to 2009. JAMA (2014) 311(17):1778–86. doi: 10.1001/jama.2014.3201
16. Blotsky AL, Rahme E, Dahhou M, Nakhla M, Dasgupta K. Gestational diabetes associated with incident diabetes in childhood and youth: a retrospective cohort study. CMAJ (2019) 191(15):E410–e7. doi: 10.1503/cmaj.181001
17. Weiss R, Santoro N, Giannini C, Galderisi A, Umano GR, Caprio S. Prediabetes in youth - mechanisms and biomarkers. Lancet Child Adolesc Health (2017) 1(3):240–8. doi: 10.1016/S2352-4642(17)30044-5
18. Tabák AG, Herder C, Rathmann W, Brunner EJ, Kivimäki M. Prediabetes: a high-risk state for diabetes development. Lancet (2012) 379(9833):2279–90. doi: 10.1016/S0140-6736(12)60283-9
19. Nichols GA, Hillier TA, Brown JB. Progression from newly acquired impaired fasting glusose to type 2 diabetes. Diabetes Care (2007) 30(2):228–33. doi: 10.2337/dc06-1392
20. Pathirana MM, Lassi ZS, Roberts CT, Andraweera PH. Cardiovascular risk factors in offspring exposed to gestational diabetes mellitus in utero: systematic review and meta-analysis. J Dev Orig Health Dis (2020) 11(6):599–616. doi: 10.1017/S2040174419000850
21. Landon MB, Mele L, Varner MW, Casey BM, Reddy UM, Wapner RJ, et al. The relationship of maternal glycemia to childhood obesity and metabolic dysfunction(‡). J Matern Fetal Neonatal Med (2020) 33(1):33–41. doi: 10.1080/14767058.2018.1484094
22. Kaseva N, Vääräsmäki M, Sundvall J, Matinolli HM, Sipola M, Tikanmäki M, et al. Gestational diabetes but not prepregnancy overweight predicts for cardiometabolic markers in offspring twenty years later. J Clin Endocrinol Metab (2019) 104(7):2785–95. doi: 10.1210/jc.2018-02743
23. Gingras V, Rifas-Shiman SL, Derks IPM, Aris IM, Oken E, Hivert MF. Associations of gestational glucose tolerance with offspring body composition and estimated insulin resistance in early adolescence. Diabetes Care (2018) 41(12):e164–e6. doi: 10.2337/dc18-1490
24. Clausen TD, Mathiesen ER, Hansen T, Pedersen O, Jensen DM, Lauenborg J, et al. High prevalence of type 2 diabetes and pre-diabetes in adult offspring of women with gestational diabetes mellitus or type 1 diabetes: the role of intrauterine hyperglycemia. Diabetes Care (2008) 31(2):340–6. doi: 10.2337/dc07-1596
25. Grunnet LG, Hansen S, Hjort L, Madsen CM, Kampmann FB, Thuesen ACB, et al. Adiposity, dysmetabolic traits, and earlier onset of female puberty in adolescent offspring of women with gestational diabetes mellitus: A clinical study within the danish national birth cohort. Diabetes Care (2017) 40(12):1746–55. doi: 10.2337/dc17-0514
26. Sauder KA, Hockett CW, Ringham BM, Glueck DH, Dabelea D. Fetal overnutrition and offspring insulin resistance and β-cell function: the Exploring Perinatal Outcomes among Children (EPOCH) study. Diabetes Med (2017) 34(10):1392–9. doi: 10.1111/dme.13417
27. Lowe WL Jr., Scholtens DM, Kuang A, Linder B, Lawrence JM, Lebenthal Y, et al. Hyperglycemia and adverse pregnancy outcome follow-up study (HAPO FUS): maternal gestational diabetes mellitus and childhood glucose metabolism. Diabetes Care (2019) 42(3):372–80. doi: 10.2337/dc18-1646
28. Stafne SN, Salvesen K, Romundstad PR, Eggebø TM, Carlsen SM, Mørkved S. Regular exercise during pregnancy to prevent gestational diabetes: a randomized controlled trial. Obstet Gynecol (2012) 119(1):29–36. doi: 10.1097/AOG.0b013e3182393f86
29. Diagnostic criteria and classification of hyperglycaemia first detected in pregnancy. Geneva: World Health Organization (2013).
30. Matthews DR, Hosker JP, Rudenski AS, Naylor BA, Treacher DF, Turner RC. Homeostasis model assessment: insulin resistance and β-cell function from fasting plasma glucose and insulin concentrations in man. Diabetologia (1985) 28(7):412–9. doi: 10.1007/BF00280883
31. Norsk Helseinformatikk [Norwegian Health information]. Beregning av KMI og iso-KMI [Calculation of BMI and iso-BMI] . Available at: https://nhi.no/skjema-og-kalkulatorer/kalkulatorer/diverse/bmi-kalkulator-kroppsmasseindeks/.
32. Bjøntegaard KA, Stafne SN, Mørkved S, Salvesen K, Evensen KAI. Body mass index and physical activity in seven-year-old children whose mothers exercised during pregnancy: follow-up of a multicentre randomised controlled trial. BMC Pediatr (2021) 21(1):496. doi: 10.1186/s12887-021-02952-1
33. WHO. Guidelines on physical activity and sedentary behaviour. Geneva: World Health Organization (2020).
34. Helsedirektoratet [Norwegian Directorate of Health]. Fysisk aktivitet for barn, unge, voksne, eldre og gravide [Physical activity for children, adolescents, adults, elderly and pregnant] (2019). Available at: https://www.helsedirektoratet.no/faglige-rad/fysisk-aktivitet-i-forebygging-og-behandling.
35. Hollingshead AB. Two Factor Index of Social Position. New Haven, Conneticut: Yale University Press (1957).
36. Lain KY, Catalano PM. Metabolic changes in pregnancy. Clin Obstet Gynecol (2007) 50(4):938–48. doi: 10.1097/GRF.0b013e31815a5494
37. Folkehelseinstituttet [Norwegian institute of public health]. Medisinsk fødselsregister [Medical Birth Registry of Norway] (2021). Available at: http://statistikkbank.fhi.no/mfr/.
38. Steene-Johannessen J, Anderssen SA, Bratteteig M, Dalhaug EM, Andersen ID, Andersen OK, et al. Kartlegging av fysisk aktivitet, sedat tid og fysisk form blant barn og unge 2018 (ungKan3) [Mapping of physical activity, sedentary time and physical fitness among children and adolescents 2018]. Norwegian: Norges idrettshøyskole [Norwegian School of Sport Sciences] and Folkehelseinstituttet [Norwegian Institute of Public Health] (2019).
39. Sarker H, Anderson LN, Borkhoff CM, Abreo K, Tremblay MS, Lebovic G, et al. Validation of parent-reported physical activity and sedentary time by accelerometry in young children. BMC Res Notes (2015) 8:735. doi: 10.1186/s13104-015-1648-0
40. Holder T, Giannini C, Santoro N, Pierpont B, Shaw M, Duran E, et al. A low disposition index in adolescent offspring of mothers with gestational diabetes: a risk marker for the development of impaired glucose tolerance in youth. Diabetologia (2014) 57(11):2413–20. doi: 10.1007/s00125-014-3345-2
41. Poulain T, Vogel M, Kiess W. Review on the role of socioeconomic status in child health and development. Curr Opin Pediatr (2020) 32(2):308–14. doi: 10.1097/MOP.0000000000000876
42. Poulain T, Vogel M, Sobek C, Hilbert A, Körner A, Kiess W. Associations between socio-economic status and child health: findings of a large german cohort study. Int J Environ Res Public Health (2019) 16(5):677. doi: 10.3390/ijerph16050677
43. Alves JM, Zink J, Chow T, Luo S, Belcher BR, Xiang AH, et al. Contributions of prenatal exposures and child lifestyle to insulin sensitivity. J Clin Endocrinol Metab (2020) 105(7):2413–21. doi: 10.1210/clinem/dgaa201
44. Pozuelo-Carrascosa DP, Cavero-Redondo I, Herráiz-Adillo Á, Díez-Fernández A, Sánchez-López M, Martínez-Vizcaíno V. School-based exercise programs and cardiometabolic risk factors: A meta-analysis. Pediatrics (2018) 142(5):e20181033. doi: 10.1542/peds.2018-1033
45. Lee KW, Ching SM, Ramachandran V, Yee A, Hoo FK, Chia YC, et al. Prevalence and risk factors of gestational diabetes mellitus in Asia: a systematic review and meta-analysis. BMC Pregnancy Childbirth (2018) 18(1):494. doi: 10.1186/s12884-018-2131-4
46. Gaillard R, Welten M, Oddy WH, Beilin LJ, Mori TA, Jaddoe VW, et al. Associations of maternal prepregnancy body mass index and gestational weight gain with cardio-metabolic risk factors in adolescent offspring: a prospective cohort study. BJOG (2016) 123(2):207–16. doi: 10.1111/1471-0528.13700
47. Hochner H, Friedlander Y, Calderon-Margalit R, Meiner V, Sagy Y, Avgil-Tsadok M, et al. Associations of maternal prepregnancy body mass index and gestational weight gain with adult offspring cardiometabolic risk factors: the Jerusalem Perinatal Family Follow-up Study. Circulation (2012) 125(11):1381–9. doi: 10.1161/CIRCULATIONAHA.111.070060
48. Barclay K, Myrskylä M. Maternal age and offspring health and health behaviours in late adolescence in Sweden. SSM Popul Health (2016) 2:68–76. doi: 10.1016/j.ssmph.2016.02.012
49. Wroblewska-Seniuk K, Wender-Ozegowska E, Szczapa J. Long-term effects of diabetes during pregnancy on the offspring. Pediatr Diabetes (2009) 10(7):432–40. doi: 10.1111/j.1399-5448.2009.00507.x
Keywords: blood glucose, child, follow-up, gestational diabetes, hyperglycaemia, insulin resistance, prenatal exposure
Citation: Nyen SL, Stunes AK, Evensen KAI, Børsting T, Syversen U, Salvesen KÅ, Mørkved S and Stafne SN (2024) Associations between maternal and offspring glucose metabolism: a 9-year follow-up of a randomised controlled trial. Front. Endocrinol. 14:1324925. doi: 10.3389/fendo.2023.1324925
Received: 20 October 2023; Accepted: 18 December 2023;
Published: 10 January 2024.
Edited by:
Bassem Refaat, Umm Al-Qura University, Saudi ArabiaReviewed by:
Ewa Wender-Ozegowska, Poznan University of Medical Sciences, PolandMaleesa Pathirana, Lyell McEwin Hospital, Australia
Copyright © 2024 Nyen, Stunes, Evensen, Børsting, Syversen, Salvesen, Mørkved and Stafne. This is an open-access article distributed under the terms of the Creative Commons Attribution License (CC BY). The use, distribution or reproduction in other forums is permitted, provided the original author(s) and the copyright owner(s) are credited and that the original publication in this journal is cited, in accordance with accepted academic practice. No use, distribution or reproduction is permitted which does not comply with these terms.
*Correspondence: Sigrid L. Nyen, sigridln@stud.ntnu.no; Signe N. Stafne, signe.n.stafne@ntnu.no
†ORCID: Astrid Kamilla Stunes, orcid.org/0000-0003-1074-5199
Kari Anne I. Evensen, orcid.org/0000-0002-0129-0164
Torunn Børstning, orcid.org/0000-0002-8807-3807
Unni Syversen, orcid.org/0000-0002-3607-7486
Kjell Å. Salvesen, orcid.org/0000-0002-1788-4063
Siv Mørkved, orcid.org/0000-0002-7250-107X
Signe N. Stafne, orcid.org/0000-0003-4922-477X