- 1Research Department, National Institution for Academic Degrees and Quality Enhancement of Higher Education (NIAD-QE), Kodaira-shi, Tokyo, Japan
- 2Graduate School of Human Sciences, Sophia University, Chiyoda-ku, Tokyo, Japan
Syndromic autism spectrum conditions (ASC), such as Klinefelter syndrome, also manifest hypogonadism. Compared to the popular Extreme Male Brain theory, the Enhanced Perceptual Functioning model explains the connection between ASC, savant traits, and giftedness more seamlessly, and their co-emergence with atypical sexual differentiation. Overexcitability of primary sensory inputs generates a relative enhancement of local to global processing of stimuli, hindering the abstraction of communication signals, in contrast to the extraordinary local information processing skills in some individuals. Weaker inhibitory function through gamma-aminobutyric acid type A (GABAA) receptors and the atypicality of synapse formation lead to this difference, and the formation of unique neural circuits that process external information. Additionally, deficiency in monitoring inner sensory information leads to alexithymia (inability to distinguish one’s own emotions), which can be caused by hypoactivity of estrogen and oxytocin in the interoceptive neural circuits, comprising the anterior insular and cingulate gyri. These areas are also part of the Salience Network, which switches between the Central Executive Network for external tasks and the Default Mode Network for self-referential mind wandering. Exploring the possibility that estrogen deficiency since early development interrupts GABA shift, causing sensory processing atypicality, it helps to evaluate the co-occurrence of ASC with attention deficit hyperactivity disorder, dyslexia, and schizophrenia based on phenotypic and physiological bases. It also provides clues for understanding the common underpinnings of these neurodevelopmental disorders and gifted populations.
1 Introduction
Various neurodevelopmental atypicalities are overrepresented in males, and autism spectrum conditions (ASC), which have been the targets of primary attention, are approximately three times more prevalent in males than females (1) [however, some current arguments maintain equal prevalence of ASC across sexes (2)]. There are also global sex differences in professional interest and cognitive strength (3, 4). Classically, Geshwind et al. assumed that high prenatal androgen levels cause the suppression of “dominant” left cerebral cortex language field development, leading to learning disorders, left-handedness, and susceptibility to immune diseases and migraines (5, 6). In a more recent version, Baron-Cohen’s Extreme Male Brain (EMB) theory posits that systemizing-empathizing dimensions of cognitive preference explain ASC tendencies in parallel with male-female brain differences (7–9). There is a global sex difference in the 2nd to 4th digit ratio, and a smaller (< 0) ratio is regarded to reflect a stronger androgen effect in utero. The finger digit ratio is easy to measure and came to be the most popular putative biomarker of prenatal androgen effects, instead of handedness (10).
However, these premises are rebutted by many accounts that failed to find an association between various androgen measures and ASC symptoms (11), especially in ASC males (12). Brain differences between ASC versus typically developed (TD) in women somewhat overlap with male-female sex differences among TD, but they have little overlap with ASC-TD differences within men (13). The diagnosis of ASC does not imply a uniform tendency, which covers a multifaceted spectrum (14), questioning the plausibility of research strategies that investigate mechanisms in parallel with typical gender differences.
Therefore, we focused on multiple chromosomal/genetic syndromes associated with sex steroid deficiencies and the known risks of ASC. About 5–20% of ASC are considered syndromic (15), and many also show hypogonadism. Characteristically, 30–50% of individuals with Klinefelter syndrome (KS), one of the most prevalent syndromes with chromosomal aneuploidy, have ASC, in contrast to the prevalence rate of 1% in the general population (16). In other syndromes with hypogonadism, 11–80% of Prader-Willi syndromes (PWS) are associated with ASC (15). Fragile X and Down syndromes also show a high prevalence of ASC along with other neurodevelopmental disorder (NDD) symptoms and psychiatric status. Hundreds of genes related to developmental or psychiatric status overlap across different diagnostic categories (17, 18). The diagnostic criteria category is not as typological as previously believed. The viewpoint of capturing status as a spectrum covering diverse phenotypes has become predominant (14). When investigating the effect of sex steroids on cognitive function, it would be more promising to focus on syndromes with known physiological factors and common endophenotypes instead of being bound by the Diagnostic Statistical Manual criteria (19).
Another drawback of the EMB theory in explaining individual differences in strength and weakness across cognitive domains, in parallel with general sex differences, is that the prevalence of non-heterosexuals among the ASC population is nearly twice that of TD (20–22). At the same time, the prevalence of ASC in male and female transgender people is approximately 7–10 times than in the general population (23, 24). Furthermore, mediation analysis suggests that the non-heterosexuality of individuals with ASD is mediated by a higher incidence of gender dysphoria (20). Among KS individuals, some of whom experience hypogonadism in utero, and self-perceived gender nonconformity tends to co-emerge with NDDs (25). These pieces of evidence suggest a common underlying mechanism between NDD risk and atypical gender identity development.
In this paper, we have collected evidence that non-correlative biopharmacological studies support that hypoestrogenism is a more robust predictor of NDDs, and a cluster of outstanding abilities. The apparent correlation between hyperandrogenism and such cognitive traits can be explained as the result of negative feedback and a pseudo-relationship (26, 27) (see section 2.1.3). This paper connects evidence from atypical sexual development with the neurodevelopmental theory of ASC, which is based on the free energy principle by Karl Friston. Oxytocin plays an important role in early insular development by helping neural predictive signals synchronized with external and internal stimuli (28), leading to a sense of self-integration (29)(Figure 1). The insula is a central part of integrating external and internal sensations into self-image (28) and switches between the self-referential Default Mode and Central Executive Networks in the neural process of cognition (30). Steroids and oxytocin bidirectionally and synergistically affect early neural development and function in adults, and disruption of oxytocin activity has been reported in PWS, which shows hypogonadism and a strong tendency toward ASC (31, 32). In the rat brain, estrogen receptor beta (ERβ) is distributed over most cerebral cortex areas, mainly in layer V. Estrogen receptor beta is abundant in the primary motor and somatosensory fields, and has a lower density in the insula, followed by the cingulate cortex (33). The cingulate cortex works together with the insula and connects incoming sensation with emotional recognition and control. A recent hypothesis regarding schizophrenia suggested that insufficient estrogen and oxytocin levels are upstream etiologies. Moderate increases in these hormones ameliorate the positive and negative symptoms of schizophrenia by attenuating impairments in prepulse inhibition, resulting in the facilitation of emotion recognition and social interaction (34).
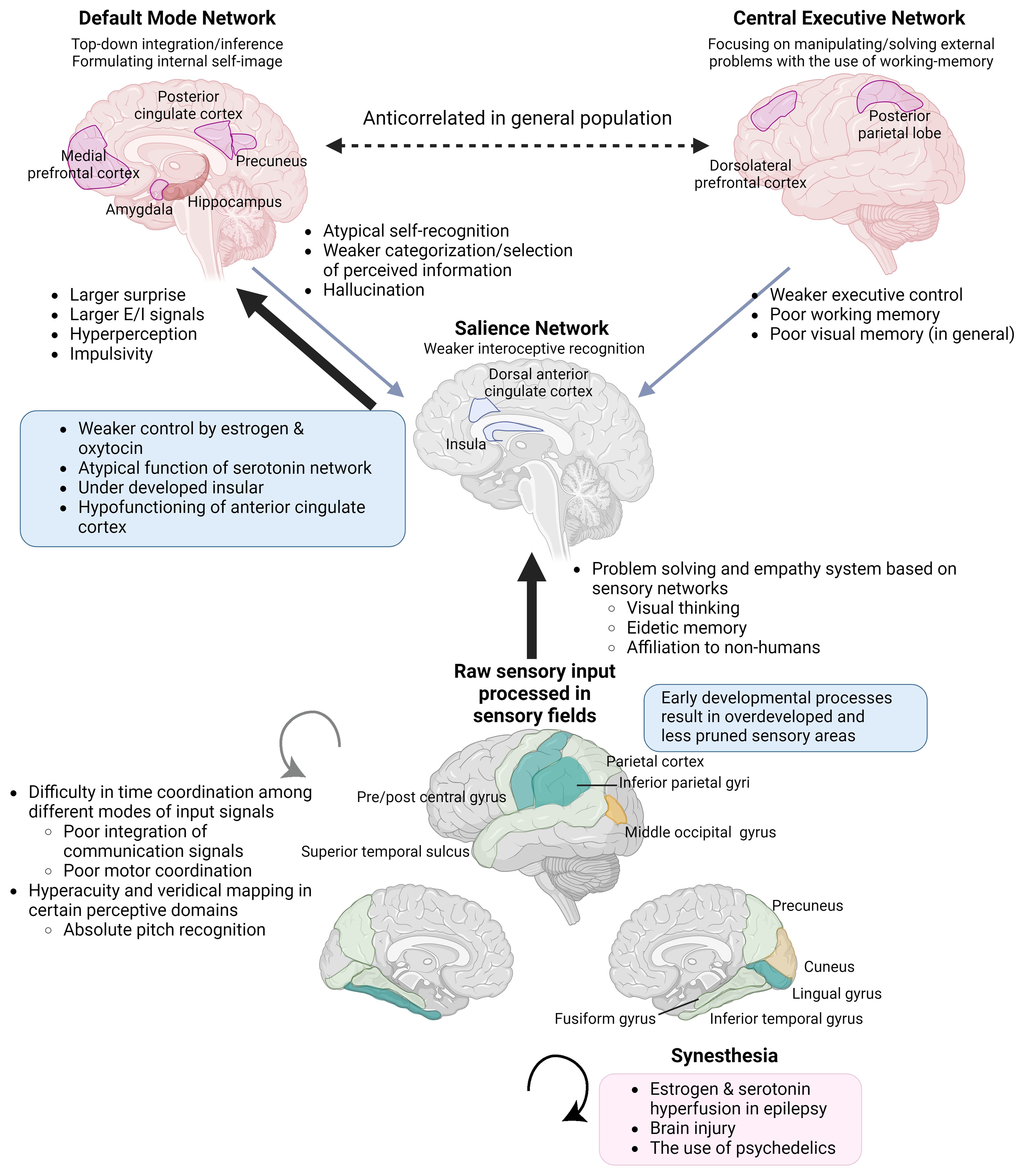
Figure 1 Integration of information processing theories and endocrinological characteristics underpinning endophenotypes of ASC, KS, and savant/gifted. The Enhanced Perceptual Functioning model and the neurodevelopmental theory based on the free energy principle, in combination with an understanding of the functions of brain networks and the interoceptive feedback system, can explain endophenotypes commonly observed across individuals with NDDs and savants/gifted individuals. The significance of each aspect varies across syndromes and subtypes within them. Brain images at the bottom. Yellow: the surface expanded areas in infants with high ASC risks. Light green: the thicker cortical areas in male to female transgenders. Green: the overdeveloped parts common to the two groups (Section 3.1.2). Individuals with KS also show similar overdevelopment patterns (Section 3.2.3). E/I, excitation/inhibition. (Created with BioRender.com).
The brains of individuals with KS (16), ASC (35, 36), and schizophrenia (37) commonly show the under-development or function of the insula. Additionally, KS (16), ASC (38), and also male-to-female transgender individuals (39) share commonalities in the overdevelopment of primary sensory areas (Figure 1). Underdevelopment of the insula can lead to overfocusing on raw sensory input processed in sensory areas and an inability to abstract perceptive stimuli, resulting in shortcomings in emotional regulation, motor coordination, and the processing of communication signals, including language (28, 40, 41). This paradigm also helps to focus on the co-occurrence of endophenotypes across different diagnostic categories, such as ASC, attention-deficit hyperactivity disorder (ADHD) (42), schizophrenia, dyslexia, along with atypical self-integration, and mystical thinking, which might become the source of wild imagination and creative inspiration (43).
2 Androgyny hypothesis of ASC and underlying mechanism of cognitive phenotype
2.1 Estrogen deficiency instead of androgen excess hypothesis of ASC
2.1.1 Syndromes with hypogonadism known as risk factors for ASC and other developmental uniqueness
The most notable drawback of the EMB is the high prevalence of ASC in several hypoandrogenic populations (44, 45). Klinefelter syndrome (XXY and its variations with >2 Xs) is the most prevalent sex chromosome aneuploidy (SCA), with a high end of prevalence rate of ≈1:500 in males (46). The lifetime diagnosis rate of KS is low, for example UK, 36% (47) and 23% (48); Australia, 50% (49); and Denmark, 25% (50), and approximately one-tenth of them are diagnosed prenatally (47). While typically known for tall stature, eunuchoid physique, learning difficulties, and impulsivity, the emergent phenotypes of KS are diverse. In a population who had been diagnosed irrespective of symptoms, the prevalence of gynecomastia and delay in school achievement did not differ from that in a control population (51). Common and robust characteristics include microorchidism, hypergonadotropic hypoandrogenism, and infertility (16). Hypoandrogenism is observed in > 75% of the diagnosed cases (16).
Among individuals diagnosed with KS, 30–50% are affected by ASC (16), and 63% are diagnosed with ADHD (52). The incidence of schizophrenia has been reported to be high; however, this observation was based on a small clinical sample (53). Psychosocial atypicality is generally considered to emerge together with hypogonadism features (54), whereas there have been case reports of ASC manifestation together with typical androgen production (55). A greater number of X-chromosomes leads to more pronounced physiological and cognitive characteristics (56, 57). In KS, ASC characteristics are mild, and restrictions of interest do not emerge (58). Verbal intelligence quotient (IQ) is typically in the low-normal range, whereas performance IQ measuring visuospatial ability is not impaired (59) and sometimes reaches a gifted range (>130) (60). Boys with KS show relative strength in arithmetic (59), although some report weakness in arithmetic problem solving. Executive function is affected by diminished cognitive flexibility and reduced working memory (53, 61). Testosterone supplementation improves language skills and concentration (53) (Table 1), but not visuospatial ability, which is generally considered to be promoted by androgens. Hypoandrogenism develops after puberty; however, speech and motor delays appear before that. Androgen secretion in the peripheral and central neural systems prenatally (165) and infantile mini-puberty (166) tends to be low, implying its influence on early developmental stages. Prenatal androgen production rate is diverse, as manifested in the micropenis manifestation in 10–25% of diagnosed cases (16). Hypogonadotropic hypogonadism cases are included in such a phenotype, and the percentage is as low as two in 160 KS individuals (167). Decrease in bone mineral density is observed in <40% individuals, indicating lower estrogen function (16).
The XYY karyotype is rare, with approximately 1:1,000 males. They are characterized by tall stature, hypotonia, and cognitive problems; however, apparent symptoms are rare. Therefore, diagnosed individuals comprise a minority, with 0.7% (48), 5.9% (47), or at most 20% of XYY cases (168). Circulating testosterone levels are comparable to those in controls (48); however, infertility rate is higher than that in typical males (169, 170). There are sporadic reports of intersex states (testicular feminization) (171, 172) and transgender individuals (173) living as females. Among diagnosed individuals, cognitive and psychiatric profiles are similar to those of individuals with KS (53), but impairments in language and social responsiveness are more severe (58). To assess subtle differences in testicular function, particularly during prepuberty, inhibin B is a sensitive measure of testicular function, and lower inhibin B levels are associated with more autistic and problematic behaviors. Interestingly, pubertal rise in inhibin B is blunted, and prepubertal anti-Müllerian hormone levels are high, similar to those in KS. In XYY individuals, higher inhibin B or testosterone levels predict better cognitive, academic, and behavioral outcomes (126), contrary to the old expectation that XYY individuals are super-males and aggressive. Estradiol levels are low-normal (174, 175), and the osteoporosis rate is not high (48), suggesting that peripheral estrogen action is typical.
Individuals prenatally diagnosed with XYY show a higher-than-average IQ, in contrast to those who are postnatally diagnosed because of physiological/psychobehavioral problems. Postnatally diagnosed individuals with XYY show lower than average IQ and more ASC symptoms; however, physiological symptoms are comparable between the two groups. Although KS is characterized by shrunken testes, XYY males tend to exhibit macroorchidism, indicating a disrupted hypothalamic pituitary gonadal (HPG) axis (174).
Another genetic condition that connects hypogonadism and autistic cognitive predisposition is PWS. This is a genomic imprinting disease that lacks a paternally imprinted 15q11-q13 gene region due to mutations or maternal disomy. In contrast to the KS variations and XYY, but similar to XXXXY (57), the stature is short. Disruption of food intake regulation leads to obesity. Both males and females are since prenatally hypogonadal, adrenarche starts early, and the ASC prevalence rate is 11–80% (15). Personality problems are severe, with multiple learning difficulties and an IQ of 30–70 (176). Distinct from SCA is the inclination toward obsessive-compulsive disorders. Language delay is typical, as observed in the case of syndromic ASC, whereas special characteristics of PWS include the numbness to pain, vomiting stimuli, and temperature sensation. Additionally, individuals with PWS are very good at jigsaw puzzle (177). Therefore, locus 15q11-q13 has been considered to be responsible for savant syndrome (178), which includes relevant genes, such as gamma-aminobutyric acid type A receptor subunit gamma3 (GABRG3). Mutation of the gene affects the activity of the GABAA receptor subunit, hindering inhibitory signaling in response to GABA. However, this finding was not replicated (179), implying that this region only explains a certain subtype of savant syndrome. Interestingly, individuals with PWS tend to be fond of caring for animals and babies (180), suggesting that they are not indifferent to their interactions with animate agents.
Fragile X syndrome (FXS) is a triplet repeat disease caused by the extension of CGG repeats in the fragile X messenger ribonucleoprotein 1 (FMR1) gene, which resides in the 5′ end noncoding region on the X chromosome. This is the most common cause of mental retardation in males, and the most common single genetic cause of ASC. Affected individuals show an elongated face, large ears, other physical malformations, and difficulty in swallowing food. The FMR1 protein (FMRP) is one of the most abundant proteins in the brain and is crucial for synapse formation, regulating mRNA translation within dendrites. The mutation of FMR1 targets GABA receptor subunits and downregulates GABAA receptors. The protein is not synthesized when the number of CGG repeats exceeds 200, leading to obesity and behavioral problems. Anxiety, ADHD, and sensory hypersensitivity are frequent symptoms. The perseverance on topics is also frequent, sometimes meeting the criteria for obsessive-compulsive disorder. The abnormal enlargement of the testes begins at puberty. Significant to the current argument is the Prader-Willi phenotype (PWP) of FXS, which comprises 10% of all affected individuals; individuals with PWP have a syndrome similar to PWS, with heightened prevalence of ASC (54%) compared to 30% among males with FXS (127). The imprinting pattern of 15q11-q13 is normal; however, the cytoplasmic FMRP interacting protein 1 encoded by CYFIP1 in 15q11-q13 colocalizes and works together with FMRP in dendrites to form neuronal structures, and the downregulation of CYFIP1 results in this phenotype. In PWP, congenital hypogenitalism and hypogonadism emerge in 6 of 13 cases, with later development of macroorchidism in general (127). The full-scale IQ, including verbal and performance IQ ranges from to 36–49. In the molecular biological exploration of the common factors between FXS and ASC, the delay in synapse maturation, under-regulation of GABAA receptors and subsequent imbalance of glutamine/GABA neural systems are considered primary causes (127).
Additionally, chromosome 21 trisomy (Down syndrome) shows comorbidities with ASC (1–42%) (130–132) and ADHD (34%) (130). Individuals with Down syndrome show characteristic facial features, low IQs, hypotonia, and poor motor coordination. In males with Down syndrome, approximately one-fourth show cryptorchism and 10% show hypospadias (181). Infertility is prevalent, and hypogonadism is also reported to be high; however, sex steroid levels are relatively normal in the postpubescent population. Approximately one-third of the individuals show elevated LH or FSH levels, indicating primary gonadal dysfunction (181, 182). Some individuals show savant skills, such as music (183), but considered to be rare. Symptomology related to ASC has eluded research attention in this syndrome.
In the general population, registry-based studies in Sweden demonstrated that individuals of both sexes with hypogonadotropic hypogonadism or delayed puberty showed a significant increase in the prevalence of ASC, ADHD, and intellectual disabilities compared to matched controls (184). The impact of hypogonadism remained significant even after excluding individuals with chromosomal abnormalities from the analyses. Kallman syndrome is a genetic disorder that manifests with outcomes associated with congenital hypogonadism. Affected individuals show hypogonadotropic hypogonadism, anosmia, an elongated face, large ears, and an increased risk of osteoporosis, hearing loss, mental handicap, and schizophrenia (151). Gonadotropin-releasing hormone-1 (GnRH-1) neurons originate from the nasal placode, and atypical development at this earliest neural development stage leads to disorganization of HPG axis. Subsequently, several ASC risk genes require the facilitation by androgen or estrogen to fulfill their typical neurodevelopmental functions, as discussed in the next section. Additionally, testosterone (185) and estrogen (see Section 2.1.3) directly modulate GABAA receptor function, and their deficiency leads to destabilized excitation in the limbic system.
Androgen deficiency affects hypogonadal populations mainly on impairing communication abilities. Weaker activation of the dopaminergic reward circuit (see section 3.3) and the amygdala (see section 2.1.3) likely induces interpersonal anxiety and weaker interpersonal motivation. Impairment in executive control of behavior through weaker control of the prefrontal cortex (see section 3.3.2) and in spatial memory through hippocampal underdevelopment (3.2.1) are also possible outcomes. The effect of androgen is partly through AR and partly through ERβ in non-aromatized or after aromatized form.
2.1.2 Cognitive characteristics explaining ASC-savant traits and major possible target gene expressions triggered by sex-steroid deficiency
The free energy principle is a broad theory in computational neuroscience that explains the formation of neural circuits and cognitive processes as the minimization of gaps between the expectation of signals and reception of input. As a comprehensive theory similar to Hebb’s rule, the free energy principle tries to explain how the information from stimuli is joined with the inner process representing phenomena in neural systems, and the closing gaps updates neural wiring. The principle was originally formulated to explain NDD phenomena, such as ASC and schizophrenia, and explains the characteristics of these two apparently different symptoms in the same model with different parameters. This principle has been demonstrated to be applicable to the decision-making process through the modulation of dopamine receptors (186), and to the learning process through cholinergic neuromodulation (187).
The neurodevelopmental hypothesis derived from the principle posits that a major causal factor of ASC symptoms is the disruption of GABA shift in early development (41) (Figure 2). The oxytocin-induced GABA shift changes GABAergic neuronal function from excitatory (depolarizing) to inhibitory (hyperpolarizing). Estradiol, especially its work on ERβ, is essential for organizing neural distribution in the developing brain (188, 189). Excessive estradiol delays GABA shift (190–192), and steroidogenesis dysfunction also delays this shift. For example, high concentrations of environmental disruptors, such as bisphenol A or bisphenol S hinder oxytocin function and GABA shift, likely by disrupting endogenous estradiol function (193). The delay or elimination of the shift leaves the brain in an immature state, leading to inflation of excitation/inhibition (E/I) ratio and a delay in closing the critical period postnatally, that is supposed to prune excess synapses, affecting neuronal plasticity (194). This leads to microstructural atypicality and hypoconnectivity of neural circuits around the amygdala, insula, and cingulate cortex, as well as psycho-behavioral atypicality. The GABA shift is an event that changes the brain from an organizational neural distribution phase to a synapse pruning phase in accordance with external sensory input (195).
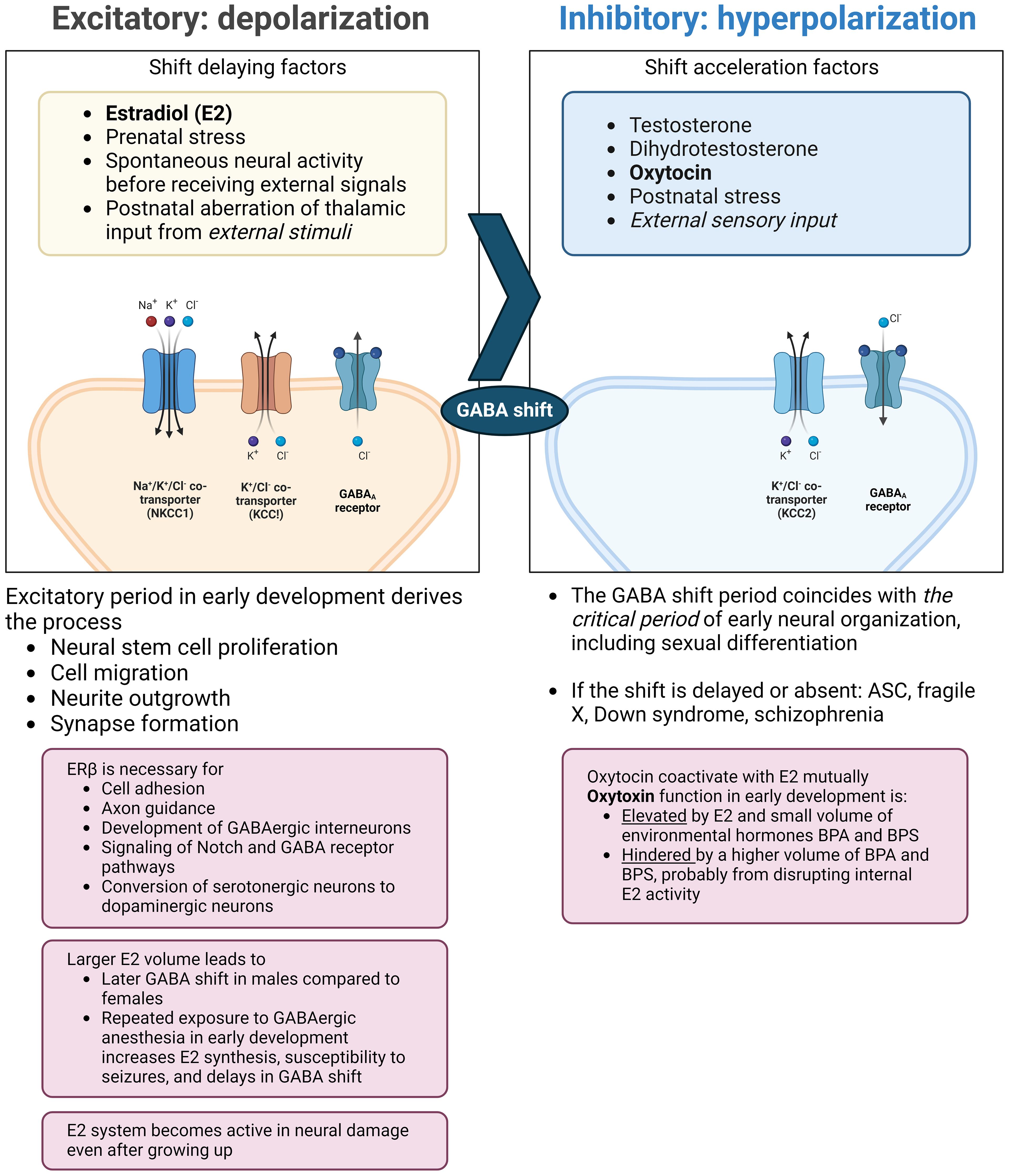
Figure 2 Endocrinological environment affecting the timing of GABA shift. Perturbation of ERβ activity hinders neural and glial cell development and adhesion. This also interrupts the function of oxytocin (right pane) and delays the timing of the GABA shift. The extended immature phase of the brain corresponds to weaker GABA inhibition and the skew to the serotonergic system compared to the dopaminergic system. Also, excessive estradiol doses by endogenous/exogenous causes a delay in GABA shift and can induce epilepsy, with possible subsequent distortion of steroidogenesis and functional pathways. ASC: autism spectrum condition; BPA: bisphenol A; BPS: bisphenol S; ER: estrogen receptor; GABA: γ-aminobutyric acid; KCC: K+-Cl- cotransporter; NKCC: sodium-potassium-chloride cotransporter. (Created with BioRender.com).
In contrast, the major psychological explanations of the cognitive characteristics of ASC, covering communication deficiency and higher performance in certain visual tasks, have been: 1) the Weak Central Coherence (196, 197); 2) the Enhanced Perceptual Functioning (EPF) (198, 199); 3) systemizing the brain through the EMB (7); and in a classic form, 4) the Geschwind–Galaburda hypothesis, which attributes the cause to the imbalance of hemispheric development (5, 6). The Geschwind–Galaburda hypothesis explains that higher prenatal androgen levels cause a delay in the development of left cerebral hemisphere, as manifested by an increase in non-right-handedness and atypical brain torque in individuals with ASC (200). The neurodevelopmental hypothesis indicates the order of development of these phenomena: the difficulty in closing gaps between internal and external perceptive stimuli and the expectation of received signals leads to non-optimal attenuation of perception, as manifested in hyper/hypoesthesia 2), and difficulty in summarizing perceived information 1), resulting in skewed strength/weakness in cognitive domains 3) and the skewed development of corresponding brain areas 4). Steroid deficiencies in early development and adulthood are possible causes of failure to attenuate neural signals. This could be manifested by overexcitation over primary perceptual input (201), overdevelopment of the visual cortex and primary sensory areas (38), glial cell atypicality perturbing synapses and neural circuit maturation (202–204). Delays in synapse maturation, reduced synapse pruning, and atypical neural networks of glutamine and GABA (205, 206) are hallmarks of ASC.
In higher-level cognitive processing, insular malformations are key to understanding the hindrance to integrating higher-order ego recognition and naming emotional experiences of self and others (36). The insula integrates multimodal sensations from the outside world and inner body into self-awareness. The posterior insula is an integral part of passing interoception to the brain, and it comprises visceral feelings and feedback from the autonomous nervous system. Disconnection from the interoception network leads to alexithymia (207). The inability to anticipate one’s own bodily responses leads to easy panicking and shyness (128). Although ERs are not densely distributed, estrogen infusion into the insula of the rat brain excites neurons by suppressing GABA release in this area and activates descending sympathoexcitatory pathways (145, 146). On the other hand, higher oxytocin concentrations correspond to stronger feelings of body ownership (29), sensitivity to the social environment (144), and emotional empathy in humans, possibly by strengthening the interoceptive network and attenuating physiological reactions to negative stimuli. Testosterone supplementation in individuals with KS improves communication and interpersonal skills, possibly by smoothing the expectation of perceptual input and helping integrate socially relevant information, thereby reducing anxiety about interacting with others (128, 208).
Major ASC-susceptible genes are modulated by estrogen and androgen, and steroid deficiencies can cause diverse cognitive symptoms. Forkhead box protein P2 gene (FOXP2), originally discovered in a family line with language developmental disorders, and its paralog, FOXP1, the fifth major risk factor for ASC and a common risk factor for other NDDs (209), are among them. FOXP2 is expressed in earliest-born cortical neurons in the subplate, and the protein binds to DNA to facilitate neural development and cell-type differentiation (210). Non-aromatizable androgens are necessary for normal expression and function of Foxp1 and Foxp2 in rats (211). Foxp2 is expressed in the sensory and motor-related cortices, cerebellum, and medial amygdala. Knockdown of Foxp2 in mice compromises social behavior processed in the medial amygdala, in dopamine-dependent manner (63). In female zebrafish, Foxp2 deficiency leads to disruption of the HPG axis (212), and FOXP2 is overexpressed in human prostate cancer cells (213). These results suggest that FOXP2 functions in the feedback on estrogen and androgen pathways.
Additionally, SH3 and multiple ankyrin repeat domains 3 (SHANK3) is another major gene factor against ASC, regulating neuronal and synaptic excitability (214). In line with SHANK1 and SHANK2, it functions on the N-methyl-D-aspartate (NMDA) and glutamate receptors, and is involved in dendritic spine maturation (215). SHANK3 is crucial for fixing and guiding the actin cytoskeleton of neurons before synaptic transmission; knockdown of SHANK3 reduces neuronal soma size, growth cone area, neurite length, and branch numbers (216). In macaque monkeys with the ASC-type mutant SHANK3, behavioral outcomes include sleep disturbances, motor deficits, repetitive behaviors, and social and learning impairments. Neuronal networks were also altered into hypo-connectivity in the Default Mode Network (DMN: see section 2.2.1) and local hyper-connectivity in areas including the somatosensory and posterior cingulate cortices (217). In human cell culture, dihydrotestosterone (DHT) increased the expression of SHANK by 35% and estradiol by 15%, indicating that both AR, ERα, and ERβ contribute to regulation (218). In contrast, Srancikova et al. found that testosterone downregulates SHANK1 and SHANK3, and that gene expression was lower in the hippocampus of male than of female rats (219).
In rodents, estrogen regulates sexual characteristics, puberty, and neurobiological reproductive systems through ERα. Contrary, estrogen modulates non-reproductive systems, such as anxiety, locomotion, fear, memory, and learning, through ERβ. If the action of estrogen on these neural systems during developmentally critical stages is insufficient, the typical development of neurons, synapses, and glial cells is hindered (220). The action of ERβ is not necessarily estrogen-dependent (188); the androgen metabolite androstanediol is also suggested to promote oxytocin function through ERβ (221). In human brain, areas with especially abundant ERβ are somatosensory cortex, hippocampus, thalamus, and cerebellum (222). Insufficient estrogen action is a possible causal factor for various psychiatric and NDDs that emerge with different sex ratios after puberty. In women, psychiatric syndromes, including schizophrenia, ASC, ADHD, and general anxiety disorders are exacerbated when estrogen levels are low (222).
2.1.3 Estrogen deficiency sometimes emerges with an increase in androgen markers in males
Differentially expressed gene analysis across different phenotypes of ASC revealed that both androgen and estrogen signaling pathways are related to these conditions. Androgen signaling is associated with the emergence of a savant tendency (223, 224). Among ASC related genes, scavenger receptor class B type 1 (SCARB1) which codes a membrane protein regulating cholesterol usage in cells is also involved in sexual differentiation, and 5α-reductase type 1 (SRD5A1) gene controls cellular cholesterol intake and testosterone metabolism (224). However, these two genes have relatively small explanatory power among ASC-related genes (209). An apparent increase in steroid biomarkers may be secondary to a deficiency in sex steroid action during critical developmental periods and the subsequent disruption of steroidogenesis. Additionally, steroids functions not only trigger genetic expression cascades through classical actions on nuclear steroid receptors. Instead, many estrogenic neuromodulating mechanisms seem to depend on receptors on the membrane, which directly and rapidly modulate the strength of GABA or glutamate signal transmission in neurons (225, 226), and the activities of glial cells (98, 227–229), which guide neural extension, synapse modulation, and information transmission.
The G protein-coupled estrogen receptor (GPER) is a major estrogen membrane receptor expressed in primate LH-releasing hormone neurons in the olfactory placode and hypothalamus, modulating the HPG axis (230). Male HPG axis negative feedback loop is mainly controlled by estrogen instead of non-aromatized androgen in pituitary, suggesting the insufficient estrogen action behind the gonadotropin increase (231). GPER plays an important role in the control of spermatogenesis in the testes (231). Individuals with KS typically show hypergonadotropic hypogonadism, and their testicular tissue shows a 12-fold increase in GPER and a decrease in ERβ mRNA expression compared to control males, indicating insufficient estrogen control for testicular function in this population (232). GPER is expressed in both the central nervous system and peripheral tissues, including the cardiovascular system. Among children with ASC, serum GPER levels decreased with an increase in symptom severity. However, serum estradiol levels did not correlate with GPER levels (233).
During the developmental phases in men, excess androgen levels trigger negative feedback or are buffered within the high-normal range, which does not necessarily deliver unique developmental characteristics, as expected in women. For example, fetuses with congenital androgen hyperplasia (CAH) are exposed to androgens at supranormal concentrations in the adrenal glands because of a lack of metabolic pathways. This causes physiological and psychological masculinization (234, 235) only in females, but does not increase the odds of ASC, according to a recent meta-analysis (133).
When mothers suffer from polycystic ovary syndrome (PCOS) and androgen production is elevated, the conceived babies’ risk of developing ASC increases (134). Animal models suggest that in girls, the elevation of prenatal exogenous androgen levels is likely to induce postnatal upregulation of androgen production and activity, whereas in males, this induces a decrease in luteinizing hormone (LH) and underdevelopment of the testes (236). Estrogen concentrations in affected mothers and offspring are not consistently altered, but aromatase activity decreases in the placenta of mothers (237). In mice, the offspring of androgen-exposed mothers show downregulation of AR or ERα in a sex-dependent manner in the hypothalamus, hippocampus, and amygdala, showing anxiety symptoms. The expression of serotonergic and GABAergic genes tends to increase in a sex-dependent manner (238). A survey of gifted boys, who would have a common physiological background with savants reported that they had smaller 2D:4D finger ratios, suggesting the influence of higher androgens in the prenatal environment. However, salivary testosterone levels were significantly lower, and performance on reading in the eye test was poorer (239). This case might correspond with the case of offsprings who have been exposed to exogenous androgen in utero, while in male neonates, the association between amniotic testosterone levels and the 2D:4D ratio is questioned (240). Additionally, an initial report of smaller 2D:4D ratios in individuals with autism (241) was not replicated (12), or was limited to syndromic cases among men (242).
In an attempt to distinguish adults with Asperger’s syndrome from those typically developed with 24 serum biomarkers, only four markers were common between men and women. An increase in LH and free testosterone levels was observed only in women; among men, the main characteristics were higher levels of cytokines and other inflammatory measures (135). Geschwind et al. pointed that ASC and gifted are often suffering from autoimmune diseases (5). Autoimmune diseases are more prevalent in females compared to males in the general population. However, long-term aromatase deficiency induces autoimmune diseases in mice (243).
Congenital estrogen deficiency results from the deletion of the aromatase gene cytochrome P450 family 19 subfamily A member 1 (CYP19A1) or abnormal function of ERs (estrogen resistance). The PWP of FXS, who are at high risk of ASC (127), shows aromatase deficiency, with eunuchoid proportions, early onset metabolic syndrome, and oligozoospermia, similar to KS, in combination with cryptorchidism or macroorchidism (see section 2.1.1). Among various endocrinological diseases with the depression of estradiol levels, aromatase deficiency causes a large drop in estradiol levels; testosterone levels are low in some cases, and high in others (26). Mothers with fetuses affected by aromatase deficiency show virilization during the third trimester. Congenital estrogen deficiency in women leads to virilization of the genitalia, decreased estrogen levels, and increased androgen levels (27). In rats, prenatal exposure to synthetic progesterone in the form of oral contraceptives results in ERβ suppression in the amygdala and ASC-like behavior (244). Estrogen deficiency leads to insulin resistance, which hinders synaptic plasticity and dopaminergic function in the ventral striatum, thereby inducing anxiety and depression. Hypoactivation of the mesocorticolimbic and nigrostriatal dopamine pathways has been suggested to correspond to low social interaction motivation and stereotyped behavior (245).
Deficiencies in ERβ, CYP19A1, and ER coactivators in the middle frontal gyrus can be a direct cause of ASC (136). Acid-related orphan receptor alpha (RORA) is a transcription factor that induces aromatase expression through a feedback loop at sex steroid concentrations (246). In ASC population, RORA and aromatase expression are greatly decreased (247, 248). In a survey of the Japanese population, single nucleotide polymorphisms (SNPs) of ESR 1/2 were found to be related to the severity of symptoms in ASC. Human ER genes, ESR1 and ESR2, encode receptors that are homologues of ERα and ER β respectively, and ESR1 was concerned with the impairment of social interactions, and ESR2 with emotional regulation. However, ESR1/2 SNPs did not predict the severity of social communication problems, stereotypies, or sensory abnormalities (137). Among male and female transgender populations, which tend to co-emerge with ASC, many gene variants correspond to estrogen signaling pathways in sexually dimorphic brain areas, but none to androgen pathways (249).
In the prenatal environment, low concentrations of unconjugated estriol (uE3) and high concentrations of maternal serum alpha-fetoprotein (MSAFP) in the maternal serum increased the odds ratio of ASC prevalence in offspring (138). A low uE3 concentration is an indication of insufficient production of adrenal steroid (dehydroepiandrosterone and others) in infants, and MSAFP suppresses estrogen activity. Additionally, prenatal exposure to progestin, which is prescribed to prevent threatened miscarriage, has been reported to suppress the expression of ERβ in the fetal brain, thereby increasing the risk of ASC in rat experiments and epidemiological studies (139).
The above evidence suggests that one of the main factors that induce ASC is the depression of estrogen action; if the potential for steroidogenesis in the fetus is intact, the androgen production rate would be increased to compensate for estrogen deficiency. In another case, an increase in exogenous androgen circulation downregulates endogenous androgen genesis in the fetus, that will cause estrogen deficiency subsequently; however, using a Danish cohort sample, Baron-Cohen et al. showed an increase in various estrogen and progesterone concentrations in amniocentesis fluids of boys with ASC (11, 140).
In addition to syndromic ASCs that lack steroid hormones during early development, there are known cases of externally caused shortages of sex steroids that induce ASC. The responsible environmental disruptors are dioxins and herbicides (141). An analysis of umbilical codes suggests that exposure to high levels of dioxins suppresses androgen production in male fetuses (250). Bisphenol A (BPA) is a blocker of AR (251) and ER, affecting the expression of multiple ASC related genes. Bisphenol A exposure in pregnant rats increased neurite length and the number of neurite branches in offspring of both sexes, while an increase in neuronal cell death, the impairment of neuronal development in the hippocampus and learning ability were observed only in male offspring (252). The prefrontal cortex of adult Long-Evans rats prenatally exposed to high concentrations of BPA showed an increase in the number of neurons and glia in layers 5/6, but only in males (253). In contrast, an epidemiological test using a public human cohort demonstrated that the effect of BPA on ASC susceptibility was more apparent in girls (254).
Studies have reported the disruption of steroidogenesis in individuals with ASC, particularly alterations in metabolic pathways before steroids are converted into sex hormones (255). Along with estrogen, neurosteroids such as the progesterone metabolite allopregnanolone, are crucial for neural cell proliferation, migration, myelination, synapse formation, and modulation of GABAA receptors in the cerebral cortex, thalamus, and hippocampus (256, 257). This function persists from the earliest stages of brain development to maturity. Androgens show similar functions or work in consort with estrogen, but their importance in early neural development and cognitive modulation seems to be secondary, as direct pharmacological evidence of the effects of developmental androgen alteration is limited. Such reasoning resolves the discrepancy, especially in affected males, where researchers have largely failed to find an increase in androgen markers prenatally or postnatally (133, 255).
One epidemiological support for the early idea that an abnormal increase in steroidogenesis causes ASC comes from the fact that peripubertal (15–19 years) individuals with ASC have a higher incidence of genital/ovarian cancer. Mothers of individuals with ASC also frequently experience sex hormone-responsive cancers (258). The increased vulnerability to disease, likely derived from the abundance of sex steroids, is more evident in female ASC than that in male ASC cases. Some ASC-related genes, such as FOXP1 and copy number variation, are known to suppress tumors or cancer if they are intact, requiring further examination to explore how such a genetic background interacts with steroid excess contributing to cancer risk.
2.2 Extreme success in “masculine” artistic-academic fields inversely correlate with masculinity indices among men
2.2.1 Connection between developmental disorders, savant/gifted, and atypical sexual differentiation
Approximately 50% of individuals with savant ability are from the ASC population (259), and the emerging ratio of sex differences is approximately three men to one woman (260). Savant ability is independent of total IQ scores. In the general population, prodigious savants who show distinguished talents that are difficult to interpret with common sense, are very rare, with only approximately 50 individuals worldwide. If the definition is expanded to talented savants who show some distinguished abilities relative to other domains of talent of their own, the prevalence among the ASC population is estimated to be from 28.5% (260) to 42% (261) or nearly 50% (262). In the early stages of academic notice, savant art was considered as an emotionless repetition of the gross amount of memory without original creativity. This notion was later corrected; savant art has its own originality, and is considered a model of the root of creativity (262, 263).
In contrast, gifted individuals are defined as “those who show exquisite talents in one or several fields (logical thinking, learning ability, etc.), or those who show abilities of top 10 scores of a population (264).” The greater the extraordinary ability, the greater tends to be the developmental unevenness across cognitive domains and social maladaptation. The behavioral output of gifted individuals is often difficult to distinguish from or co-exists with ADHD, obsessive-compulsive disorder, ASC, schizophrenia, and avoidant personality disorders (155). Whether an individual is judged as savant or gifted is largely influenced by the academic background of the report.
Using the Bayesian explanation of the free energy principle, hypopriors (having fewer internal models prior to processing stimuli) can explain hypersensitivity to sensory input, immunity to being distracted by contextual information (better at copying impossible figures), and incompetency in communication dependent on summarized inference (265) (Figure 1). Therefore, the EPF model in combination with the free energy principle does not require supposing a lack of empathic motivation in the first place, but suggests that the characteristics of perceptual processing are the basis of both unevenness in the strength of cognitive domains and communication inaptness.
The main premise of the EBM theory is that higher androgen action on the prenatal brain increases ASC traits between and within sexes. However, ASC traits are more observable among hypoandrogenism conditions, contrary to expectations, except for females with PCOS and offspring of mothers with PCOS (133). The effects of maternal PCOS on offspring are under debate, with complications from epigenetic effects, genetic inheritance, insulin resistance (266, 267), and the effects of metformin administration on mothers (268). Additionally, Baron-Cohen et al. admit that MRI voxel-based morphological ASC-TD brain differences within sex are not parallel between sexes, and the difference within men is especially discordant with TD sex differences (13). They also reported in adults, blood serum biomarkers, such as high LH and free androgen index could distinguish between women with Asperger’s syndrome and controls; however, the same marker set did not have discrimination power for men (135).
The DMN is the functional connection of brain areas involved in self-referential mind wandering, which becomes active when a person is not focused on performing tasks. Researchers have become increasingly interested in the DMN because of its ability to discriminate atypical cognitive statuses. Baron-Cohen et al. compared the connectivity strength of the DMN between sexes in TD and within sexes across ASC and TD groups. They reported that TD males and individuals with ASD had weaker connectivity in the DMN (269). In contrast, in an analysis of whole-brain connectivity without presupposed theories, the connectivity between social brain areas (fusiform gyrus, superior temporal sulcus, inferior parietal cortex, insula, and posterior cingulate cortex) and other areas was stronger in female ASC (male-like), but weaker in male ASC (female-like) (270). They argued that this result supports the atypical sexual differentiation theory of ASC genesis instead of the EMB theory.
Do gifted individuals tend to be hypermasculine or gender neutral? Several studies support the latter tendency. The fields of interest and strengths of gifted individuals tended to be gender-neutral instead of following stereotyped gender categories (155, 271). Their gender identities also tend to be neutral (272). Another survey showed that neutral gender identity was more evident among gifted women than in men (273). Additionally, more students in gifted classes recognized themselves as sexual minorities than their non-gifted counterparts (274).
3 Expectation mismatch on perceptual input will lead to uneven development of perceptual processing and difficulty in information integration
3.1 Oversensitive perceptual input as the basis of weak central coherence, extreme systemizing, and communication deficiency
3.1.1 Hyperacuity and overexcitability over sensory input likely start at early development
Widely observed cognitive underpinnings among ASC and other NDDs are the dominance of primary and local sensory information processing, the weakness of abstracting from that information, and having a global view. They also have difficulties combining information of different modalities over time. The peculiarities of vision perception have been extensively studied. This can lead to excellence in information processing in certain domains, such as in savants, and at the same time, difficulties in real-time responses needed for communication (201, 275). Perceptual distinctiveness observable daily is the inflation or suppression of senses in response to external stimuli (149, 276), a characteristic shared by the gifted (277). The high excitability of neurons because of insufficient suppression by GABA, and increased glutamine concentrations are considered physiological factors (201).
Brain development starts from lower-level sensory areas; subsequently, integration functions in the association areas and prefrontal lobes develop over a long period. The peculiarities of developmental trajectory in individuals with ASC are observable immediately after birth; their brain size is larger than that of TD individuals in the early stages of life (278), especially in the visual cortex and primary sensory areas, followed by the inferior temporal cortex (38) (Figure 1). Dominance of local visual processing seems to start in infancy (279), indicating that peculiarity in perception begins at the earliest development of primary sensory receptive fields, inducing the overdevelopment of certain perception-oriented information processing neural network traits, and neural excitability (280). Hypersensitivity of receptive fields and sensory processing in ASC individuals seems to be underlined by the peculiar density in neural cell packing; in ASC postmortem brains from infancy to young adults, mini-columns in the cerebral cortex were narrower in Brodmann area 3 (primary sensory area), 4 (primary motor area), 9 (prefrontal association area), 17 (V1), 21 (temporal visual association area), and 22 (temporal auditory area) (202, 281). In other specimens, ASC did not differ in cell structure in area V1 (Brodmann area 17), and narrowing of the mini-columns was most prominent in the ventral and orbitofrontal prefrontal lobes (282).
The function of estradiol on ERβ seems to be critical for early neural/glial distribution and closing the immature phase shifting to stimuli-dependent synapse modification (Figure 2). The time phases and pathways of neural maturation differ across perception modalities. In embryonic stem cell lines in which SHANK3 was mutated into the ASC type, olfactory placodal neurons first emerged at the earliest stage of neurogenesis, and then developed smaller cell bodies and more and longer neurites compared to those in controls. These shifts were not observed in cortical neurons, except for the shortening of neurites (283). Similar changes were observed in the auditory areas, but in the opposite direction, with a wider interval between mini-columns compared to that in controls in the primary auditory and association fields. This difference was particularly significant in the primary auditory field in younger populations (284).
As stated previously, hyperacuity in the visual senses appears to be underlined by differences in neural cell structures. Notable characteristics of individuals with ASC include larger numbers of near-distance neural connections (285), a larger proportion of intra-hemispheric in contrast to inter-hemispheric neural connections, an inclination to excitation in the neural E/I balance, and heterogeneity of development between brain areas. The incoherence of processing and communication speed across modalities makes it difficult to combine information in real-time, resulting in the creation of unique information-processing networks that detour neurotypical processing in language areas, which may manifest as a unique brain torque (200) or non-right-handedness (286) in parts of the population.
3.1.2 Visual imagery and synesthesia as the bases of savant ability
Typically appearing cognitive skills in savants are categorized as follows: 1) calendar calculation; 2) music; 3) art; 4) mathematical and number skills; 5) mechanical or spatial skills; and 6) “other obscure skills” which include exceptional multilingualism, sensory discrimination abilities, synesthesia, and knowledge in specific fields (121, 287). For ASC individuals, Temple Grandin grouped characteristic cognitive styles into the following: 1) visual thinkers; 2) music and math thinkers (pattern thinkers); and 3) verbal logic thinkers (288). Distinct cognitive strategies observable in high-functional ASC, savants, and gifted are calculation, architecture, and art creation by manipulating vivid visual imagery (68, 108–113). Enhanced pattern detection is likely to develop through exceptional sensory acuity and veridical mapping across isomorphic structures (289).
Individuals with ASC tend to preferentially use the visual cortex, in contrast to neurotypicals who use the prefrontal cortex to solve visuospatial tasks, such as mental rotation, under experimental conditions (93, 94). Furthermore, even in non-spatial tasks, such as the N-back working memory task which is typically processed verbally in neurotypicals, ASCs tend to use the right hemisphere and posterior cerebral regions, including the inferior temporal area and occipital lobe, indicating that they solve tasks visually (95). Inferior temporal gyrus comprises “what” ventral pathway in visual processing and responsible for object imagery handling color, texture, and patterns. The area stores single cells which index long-term memory, connecting semantic significance to the images, and also processes letters and manipulates numbers. Among individuals with ASC, visual imagery itself, focus on detail, and low communication ability were not related to savant abilities; however, high spatial mental imagery predicted a larger number of savant abilities (121). Spatial imagery is processed in the dorsal visual pathway leading to the occipito-parietal regions.
Synesthesia is another key to understanding savant phenomena through atypical sensory processing. In synesthesia, the senses of the different modalities are jointly perceived. Synesthesia is relatively common in ASC populations and closely associated with the emergence of savantism (290). Mental imagery ability is closely associated with sequence-space synesthesia, leading to savant abilities, such as calendar calculation (114, 115). The inferior temporal cortex and areas adjacent to the fusiform gyrus are responsible for grapheme-color synesthesia (291). In contrast, a survey of a larger population reported that the emergence of synesthesia was independent of the strength of visual imagery, and that individuals with weak visual imagery could possess synesthesia (292). Connectivity in the superior parietal or frontal cortex is responsible for the synesthesia irrespective of its subtype (291). Absolute pitch recognition is an aspect of veridical mapping and sound-related synesthesia that sometimes hinders language recognition, which requires the abstraction of information from similar phoneme patterns (289, 293).
In summary, the endophenotype connecting the cognitive characteristics commonly observed among ASC and other NDDs, savant and gifted individuals is heightened sensory sensitivity (121). The overdeveloped cortical parts in ASC high-risk infants overlap to those in male-to-female transgender individuals experiencing significant body dysphoria (transsexuals), in contrast to their cisgender counterparts in the postcentral gyrus (primary sensory area), left inferior temporal gyrus, and right lingual gyrus, respectively (38, 39). The other overdeveloped regions in the frontal and parietal occipital regions are located adjacent to each other (Figure 1). Lingual gyrus is responsible for visual imagery and creative thinking, in combination with other occipito-temporal-parietal complex areas (294). Another indicator that possibly reflects the early overdevelopment of sensory processing network is eidetic memory and memories from the early stages of life. Individuals with ASC have memories from an earlier age, not in an episodic form, but with sensory details (295).
3.1.3 Untypical olfactory sensitivity
Olfactory sensitivity is another cognitive domain in which individuals with ASD do not simply exaggerate their TD male traits. Among TD individuals, olfactory sensitivity is superior in females, and some individuals with ASC individuals are obsessed with smell stimuli (68). Among the sensory symptoms, atypical sensitivity to taste and smell is the most prominent in ASC, and is a strong predictor of social deficits (72). Experimentally, some studies supported heightened sensitivity in trace smell perception threshold tasks in ASC (69), while others did not find differences from TDs (296, 297) or found blunter olfactory sensitivity (hypotonia) (73) and lower activity in the olfactory cortex (74), leaving the argument inconclusive (298).
In TDs, arousal in the autonomous nervous system is increased by the sniffing of human sweat during fear, but the response of ASCs was the opposite (299). The authors argued that social dysosmia (the inability to discriminate socially relevant chemosensory signals) is a causal factor in ASC’s incorrect reading of others’ emotions (298). Experiments using general odorant substances have shown that ASCs are rather impaired in the discrimination ability of odorants, and that the evaluation of smell stimuli is atypical (72, 75, 76).
In any standardized olfactory perception task to identify sensitivity threshold, discrimination ability, and identification with known stimuli, TD females outperform TD males, and the difference is most evident at the threshold (62), culminating in the ovulatory period when estrogen and androgen concentrations are the highest (67). In contrast, hyperosmia in women (strong sense of smell) is associated with morning sickness, but the emergence of morning sickness does not coincide with fluctuation of sex steroids, but with an increase in human chorionic gonadotropin (66). Pregnant women with morning sickness perceive the smell of food to be unpleasant. This does not necessarily correspond to a lowered threshold of olfactory senses; discrimination ability is undermined for some odorant stimuli (71). Unusual olfactory perception in ASC seems to be similar, reflecting a hyperreaction to perceived stimuli, but not necessarily the manifestation of heightened sensitivity (76).
Notable changes in the olfactory sensitivity have been observed in patients with epileptic seizures. In some cases, olfactory hypersensitivity and phantom odors are perceived as precursors or aftereffects of epilepsy (70). Epilepsy is found in 4–46% of individuals with ASC, and is also common in syndromes that contribute to ASC. An imbalance between excitatory and inhibitory neural activity is thought to be a common mechanism, and excessive estradiol activity in the brain during the critical period is a risk factor for epilepsy (192). Synesthesia sometimes emerges after epilepsy, drug intake, or brain trauma which cause the shuffling of neural sensory mapping in cortical layer V. Brain trauma converts neural environments into immature patterns, resulting in atypical neural circuitry in adults. Excitation of pyramidal neurons with the serotonin receptor, 5-HT2A, induces destabilization of the thalamus, possibly resulting in reinnervation with neural inputs from different modalities (300). Researchers have suggested similarities between hyperosmia, phantom odor, and synesthesia (301). Weakened inhibition from the frontal lobe due to a lesion in the right inferior parietal lobule is suggested as a trigger of hyperosmia (302).
3.2 Savants/gifted individuals excel in visual imagery processing, but are poor in spatial memory
3.2.1 Cognitive and neurological profiles of individuals with SCA in reference to ASC
Brain morphology and cognitive characteristics in SCAs overlap with those in ASC. In studies on KS, the total cerebral volume was reduced, but the gray matter volume in the parieto-occipital and sensorimotor cortices were significantly increased (16, 303) or at least preserved (304), whereas the inferior frontal and temporal areas, especially in the dominant hemisphere, were reduced. A reduction in the frontal lobe corresponds to attenuated executive function, which is mostly evident in impaired mental flexibility (61). In the general male population with hypogonadism, there is evidence of an association between androgen deficiency and compromised executive function (151).
Although androgen therapy in adult KS is ineffective in improving mental flexibility, a mouse model suggested a combined negative effect of the number of X chromosomes and gonadectomy on the cognitive domain (77). Skews in cognitive domains (performance IQ>verbal IQ) overlap with other SCAs, except for Turner syndrome (karyotype XO) (53, 305). The ASC-like tendency was more severe in other SCA (XYY and XXYY) (58, 129) than in KS. Therefore, the general assumption is that the duplication of sex chromosomes, rather than sex steroid action mainly affects cognitive atypicality in SCAs (306). However, as suggested in Section 2.1.1, even XYY individuals which are generally categorized to possess typical steroid levels share some phenotypes suggestive of disruption of the steroid metabolism-feedback pathway, similar to other syndromes with hypogonadism. Chromosome-manipulated mouse models also suggest that the effect of chromosome number on cognitive differences is negligible, except when choosing same-sex conspecifics for mating, but is moderated by the organizational effect of sex steroids (307). A more comprehensive view is that the activational function of steroids is altered in individuals with SCA, as reported in animal models (308) and humans, probably through the alteration of organizational pathways. For example, the effect of CAG repeat on AR might be inverted in naturally hypogonadal populations, such as KS and female populations; in TD women, a longer CAG repeat, implying less active AR receptors, is associated with a higher circulating androgen concentration which is typically considered to be the marker of stronger masculinization (99).
Individuals with Turner syndrome are females with estrogen deficiency, characterized by superior verbal and poor visuospatial abilities (53). This pattern appears to be in contrast with KS and XYY, supporting the notion that sex chromosome allocation is crucial for cognitive deficiency and strength patterns. However, examination of task scores shows that they are more alike than the first look; impaired visual memory and visual attention are also observed in NDD with performance IQ strength, such as in individuals with ASC without superior IQ (82–84) or at older age (85). Visual memory corresponds to drawing complex figures through recall, using such as the Rey-Osterrieth complex figure test as a typical measuring tool. Androgen supplementation improves the scores in female-to-male transgender individuals experiencing significant body dysphoria (transsexuals) (78), and estradiol improves the scores in hypogonadal males (79), but is ineffective in women with Turner syndrome (86). In Turner syndrome, superior verbal ability is limited to certain domains, such as word knowledge, receptive/expressive abilities, verbal memory, coemerging with lower verbal fluency, articulation, and social skills. The prevalence rates of ASC, ADHD, and schizophrenia are greatly increased compared to those in TD women. Such overlaps of cognitive characteristics with KS and XYY suggest Turner syndrome’s similarity to ASC with hypergraphia (language production-strong subtype) (86).
A lack of optimal estrogen supply through critical periods throughout life leads to the suppression of verbal fluency, verbal memory, and executive function, and increases the risk of schizophrenia, ASC, ADHD, general anxiety disorder, and depression (222). Such cognitive profiles correspond to KS, XYY, and Turner syndromes. The general cognitive benefits of testosterone supplementation in KS (124) and general hypogonadal men (88, 125) are an increase in verbal fluency, but not in visuospatial ability. In a comparison between testosterone and DHT supplementation in hypogonadal men, only DHT was effective in improving spatial memory (route and spatial array learning tests), whereas testosterone improved verbal memory (story recall), suggesting that the latter effect occurs after conversion to estradiol (88).
Decreased brain volume in KS is known to occur in the hippocampus, insula, striatum, and amygdala (16). However, some studies did not find a reduction in the hippocampus (304). Spatial memories of complex figures and places can be attributed to the hippocampus. The hippocampus is abundant in both AR and ERβ (290), and estrogen deficiency impairs hippocampal development. In female mice, ERβ-KO causes a decrease in visuospatial learning ability (90). In KS, oral supplementation of the androgen, oxandrolone, in boys of 4–12 years was effective in increasing hippocampal volume to the TD range (80). The volume of the hippocampus positively correlated with scores on the Recall of Designs subtest of the Differential Ability Scale (81). Oxandrolone is a non-aromatizable androgen, but sometimes causes gynecomastia through an unknown loop. Sex steroid administration in gonadectomized male rats has revealed that the cognitive-enhancing effect of androgen on the hippocampus is at least partly through its action on ERβ (89).
A comprehensive review of the effect of estrogen on androgen-deprived males showed that estradiol treatment improved visual/verbal memory and behavioral inhibition (79). It should be noted that in the comparison of visuospatial tasks between animal models and humans, the latter depends on the manipulation of visual images, whereas the former depends on localization in actual spatial environments.
3.2.2 Effect of steroid hormones across different components of visuospatial abilities
The EBM theory gives the impression that visuospatial abilities, such as mental rotation, are derived from higher androgen levels, and that female-favored verbal abilities are more facilitated by an increase in estrogen levels. Although previous reports have supported these ideas, recent findings have refuted such a simple relationship. The most robust sex differences in cognitive ability have been found in mental rotations using two-dimensionally depicted polygons (92). Lesbians and women with CAH exposed to high levels of androgens during fetal life have higher scores for mental rotation among women, whereas men with CAH have lower scores than typical men (96). Two explanations are possible for the latter association: androgen negative feedback may be in effect, resulting in lower androgen action and visuospatial ability, or the highly androgenic environment in the early male developmental period reduces the capacity for mental rotation. Animal models have also shown inconsistent results regarding the effects of CAH on males, and it cannot be determined which model is more appropriate (104). Additionally, in a meta-analysis of CAH studies, 2D:4D digit ratio was found to be an ineffective predictor of spatial ability (96).
A notable finding was that although boys with CAH were masculinized in hobby choice compared to their siblings, their spatial and mechanical abilities were lower (104), suggesting that higher prenatal androgen levels compromised their spatial and mechanical abilities. Boys with androgen excess disorders (Familial male precocious puberty) did not show an ability difference in a virtual water maze task compared to controls (309). A study of adult TD males found that mental rotation and spatial visualization scores (paper folding and MacQuarrie blocks) were negatively correlated with salivary T levels, whereas shorter AR CAG repeat polymorphisms (stronger androgen action) were associated with higher scores on spatial visualization tasks (97).
Visuospatial abilities, such as mental rotation, may be best in populations with testosterone levels intermediate between those of women and men (99, 100). A longitudinal investigation of cycle effects for eight weeks reported a quadratic association between salivary T and mental rotation scores within each sex (101). Among boys, gifted individuals had superior mental rotation ability, and higher scores were associated with lower prenatal androgen action, as suggested by longer AR CAG repeat lengths and larger 2D:4D ratios (215). Contradictory to this picture is a report that heterosexual and gay men without childhood gender nonconformity scored better in mental rotation than those with childhood gender nonconformity (102).
Although various reports have found no association between circulating androgen levels (103, 239, 310–312) or androgen administration and visuospatial ability, particularly among men (313), a robust relationship has been reported in which high circulating estrogen levels suppress mental rotational capacity. The mental rotation scores of naturally cycling women increase during periods of low estrogen concentration (314), correlating negatively with salivary estradiol levels (105). In men, lower estrogen concentrations were associated with higher mental rotation scores (103).
In contrast, spatial memory, which uses visuospatial working memory to remember where things are located, is better in women, and higher estrogen levels are more advantageous. Androgen-suppressed, TD men undergoing androgen deprivation therapy for prostate cancer, and long-term abusers of exogenous androgens have reduced visuospatial working memory (91), which can be caused by estrogen deficiency.
3.2.3 Similarity of visual processing endophenotypes of dyslexia to ASC and savant/gifted in reference to KS
The most robust and prevalent cognitive profile of KS is a slightly inferior language ability, with dyslexia occurring in 50–70% of cases (52, 123); auditory processing and verbal memory are the weakest components (315). The cognitive characteristics of individuals with dyslexia have similarity with those of savants and gifted. A person may be gifted in mathematics, but has difficulty with sequential calculations and memorizing formulas (316). They also excel in various artistic activities and are superior at extracting patterns from visual noise (a measure of object imagery). Art students showed a higher rate of dyslexia (106, 122) and weaker speech perception skills.
In dyslexic brains, hypoactivation of the left occipital-temporal region during language tasks (317) and underdevelopment of the left frontal gyrus have been observed, especially among populations that use logographic letters, such as Chinese (318). Brains of KS have been reported to show a similar lack of dominance when processing language tasks (319, 320), although not all (321).
The total brain volume in KS is smaller than that in TD (322), with smaller volumes in the insula, frontal and temporal lobes, and subcortical regions, such as the amygdala, hippocampus (16, 51, 94, 95), and cerebellum (see Section 3.2.1). In contrast, the sensorimotor and parietal-occipital areas are enlarged, and the white matter volumes of the left cuneus and precuneus are the most robust, and extending to the inferior parietal regions in some cases (152, 303, 323). The inferior parietal lobule, which includes the angular gyrus (Brodmann area 39), is important for problem-solving through visual imagery, and is enlarged in mathematicians (107) and artists, especially in the non-dominant hemisphere (106). This region is responsible for connecting bodily sensations to spatial self-images. Stimulation of the angular gyrus induces out-of-body sensations, as is often observed in ASC, schizophrenia, and dissociative disorders (324).
It has been assumed that the blockage of language development and general intelligence might be complemented by other routes of information processing and outlets, as reported in the early cases of savants excelling in dancing or music (183). Well-known examples of paradoxical functional facilitation include acquired damage to the left hemisphere, resulting in savant-like abilities, including temporal lobe epilepsy, stroke in the dominant hemisphere, and frontotemporal dementia (287). It has been implied that damage to the frontal lobes enhances the function of the sensory areas in the posterior part of the brain (325).
A decrease in fine motor skills and visual-motor integration is also manifested in KS and XYY (53), and is ameliorated by androgen supplementation (142), which is probably also associated with improvements in verbal fluency. As observed in ASC, individuals with KS are weak at working memory (152), and they make more errors when presented with visual patterns that are difficult to discriminate, especially when presented sequentially (87). These phenotypes underlie the difficulties in language manipulation.
Autism spectrum conditions and predisposing syndromes of ASC often include a history of epilepsy (ASC: 4–46%, KS: 5–17%). Epileptic seizures alter neurotransmitter efficiency; however, only a few cases of acquired savants are directly attributable to epileptic seizures (325). Instead, endophenotypes that induce ASC, savant, or gifted individuals, in addition to syndromes with chromosomal abnormalities (326) and hypogonadism, should be considered to share a predisposition common to epilepsy. Atrophy of various brain regions has been observed in individuals with a long history of epilepsy (327). Epilepsy is caused by neuronal hyperexcitation, and astrocytic abnormalities are among its main factors. Sex steroid concentrations affect the severity and frequency of epileptic seizures. Notably, an overdose of estrogen aggravates neural overexcitation (328). Additionally, valproic acid, a major antiepileptic drug whose intake by the mother induces ASC in the fetus, disrupts the fetal HPG axis and causes autoimmune diseases in males (329).
3.3 High sensitivity to social cues, shyness, and impulsivity
3.3.1 Shyness and high social anxiety, weaker interoception network, and alexithymia lead to insufficient communication skills
Contrary to the impression that individuals with autistic tendency are “mind-blind” and inattentive to social cues, the narrative of affected individuals support the view that they shut down the flow of social information, because they are afraid of getting panicked by emotional disturbance caused by involvement with others and their gazes (330). Individuals with KS (128) and at least subsets of ASC (331, 332) show increased physiological arousal in response to social cues, such as the human eye and mouth, but less activation of the amygdala and insula (333). It is difficult for them to assess and label affective responses, that underlies alexithymia (128). This makes them easily panicked by social stimuli; thus, they avoid them.
When individuals perceive their own emotions, information from the peripheral nerves inside the body, including visceral sensations such as heartbeat and temperature, are input via the insular cortex to the cingulate gyrus or the ventral medial frontal cortex. Conscious access to internal receptive sensation (interoception) is carried by the anterior insula and anterior cingulate gyrus, and is central to simulating the emotional states of others (143), thereby enabling to empathize with others.
Alexithymia does not necessarily mean that affected individuals lack an interoceptive sense or emotion; however, it makes it more difficult to explicitly identify emotions and communicate one’s feelings to others, thereby reducing the capacity to empathize with others (28, 143, 332). The insular cortex and cingulate gyrus are atrophied in KS (334), which corresponds to difficulty in social interaction and language development. Insular volume in ASC is not different from that in TD, whereas hypoactivation in emotional empathy task corresponds to higher levels of alexithymia (36). For KS (333) and ASC (36), the identification of stimuli with negative emotional valence is difficult or hypoactivates brain areas for processing emotions, which might also correspond to a reduction in amygdala volume. To complicate the interpretation, the insula in ASC shows hyperactivation in different types of tasks.
The most robust cognitive profiles in KS are shyness, unassertiveness, withdrawal, impulsiveness, and anxiousness (306). That is similar to that of ASC (335). Androgen replacement therapy for KS improves verbal IQ, and evidence suggests that this is largely due to reduced social anxiety (208). The reasoning is opposite to general human population, whose right anterior insula is overactivated in generalized anxiety disorders and in cases with high neuroticism, and these individuals show high interoceptive sensitivity (147).
In contrast to the fear of evolutionarily relevant stimuli, which is adaptive, anxiety is a high-vigilance response to undefined threats. Oxytocin acts to reduce anxiety in humans and animals, which helps to discriminate between actual danger and safety (336), and the candidate for its major activation site is bed nucleus stria terminalis (220, 337, 338). In TD participants, intranasal oxytocin administration during imaging social embarrassment situation experienced by self or others reduced physiological arousal and activation in the right amygdala and insula, especially among participants with high trait anxiety. This also increases the empathic ratings of others’ embarrassment and their own embarrassment, supporting the idea that attenuation of maladaptive vigilance to social stimuli attenuates alexithymia and helps empathy with others (144). Although it is debatable how circulating oxytocin levels correspond to its activity in the brain, those with high concentrations of salivary oxytocin experience stronger body ownership during the rubber hand illusion, suggesting that oxytocin facilitates to joint top-down model of self and interoceptive awareness of one’s own body (29, 30).
Oxytocin receptors exist in the insula, but their numbers are not large, and their expression corresponding to social behaviors is more apparent in the anterior cingulate cortex and amygdala (339, 340). With regard to control by sex steroids, although ERs are relatively sparse in the insula, estrogen directly facilitates descending sympathoexcitatory information transfer by affecting GABAergic neurotransmission (145). In the majority of oxytocin receptors in the brain, such as in the paraventricular nucleus (PVN), the receptors are co-expressed with ERβ and facilitated by estrogen action (220). Additionally, the dihydrotestosterone metabolite, 5 -androstane-3,17 -diol (3 -diol), also activates the oxytocin promoter through action on ERβ (221). This could explain the pathway by which androgen supplementation in KS reduces anxiety and improves verbal ability.
3.3.2 Steroid deficiency decreases impulsive action, but increases impulsive choice
Impulsivity and aggression are generally considered greater in males and male prevalent NDD. Androgens are known to function in the limbic system to regulate reproductive function, aggressive behavior, and homeostasis. Specifically, AR is expressed in the brain reward system, which includes the mesocorticolimbic ventral tegmental area and nucleus accumbens, as well as the medial prefrontal cortex, in male and female rodents, non-human primates, and humans. Steroid hormone concentrations in the mesocorticolimbic system are more than double those in the circulatory system, and are still present six weeks after gonadectomy, indicating an important role for local androgen synthesis in the central nervous system (341). Androgens enhance perseverance toward activities for greater rewards and risk-oriented behaviors. Testosterone enhances tolerance to large uncertain rewards. In contrast, the predominant personalities of KS and ASC are shyness, social withdrawal, and clumsiness, and their impulsivity is the opposite of that of bold risk seekers.
Several theories have attempted to dissect the conceptualization of impulsivity. Sensation seeking by Zuckerman concerns the inclination toward reckless behavior to increase reproductive chances, and is higher in males (157), culminating in adolescence, and then decrease (154). The sensation-seeking behavioral list matches “masculine” risk-seeking behavior, and is suggested to be more strongly connected to reward processing than impulsivity (158). In contrast, impulsivity refers to a lack of self-control or deficiencies in response to inhibition. Impulsivity linearly decreases with age in the general population from approximately 10 years of age (154). Deficits in inhibitory executive function are commonly observed in patients with KS, ASC, ADHD, and gifted individuals. Typically developed males undergoing androgen deprivation therapy experience emotional lability and impulsivity (low inhibition) (156); a decrease in volume and neural activity in the prefrontal cortex likely underlies this effect (153).
In the experimental paradigm, impulsivity is measured separately using impulsive action and impulsive choice tasks (342). Both link to the dopamine system; impulsive action is assessed with stop signal and go/no-go tasks, which measure the reaction time that subjects must respond to ‘go’ stimuli, while inhibiting responses to occasional no-go/stop signals. In animal models, impulsive action is stronger in males (159) and impulsive action in females is positively correlated with circulating estradiol levels (160).
In contrast, impulsive choice concerns delay discounting, corresponding to a skew toward choosing an immediate small reward, in contrast to a delayed or uncertain larger reward. This is a model of ADHD and addiction, in which impulsive choices are stronger in females. This type of disinhibition is a model of impulsivity observed in syndromes with hypogonadism and male-prevalent NDD, and is attenuated by sex steroids. In laboratory animals, orchiectomy increases, and supraphysiological testosterone administration decreases impulsive choice in males (161). Although ovariectomy and estrous cycle do not affect the performance in female animals, women show a decrease of “now” bias in follicular phase, indicating dopamine signaling facilitated by estradiol rise, which speeds up subjective time perception and decrease impulsivity (164). In other words, the results suggest that estradiol deficiency affects frontoparietal circuits supporting working memory, and makes the subject feel that the passage of time is slower, combined with the effect on the hippocampus that is linked to discounting behavior, leading to impatient decision-making.
The developmental theory of ADHD also suggests that insufficient estradiol at the developmental stage leads to a hypofunctioning mesolimbic dopamine system and inefficient reinforcement, inducing the affected person to seek stronger stimulation (162). The authors suggested that suboptimal estrogen and dopamine activity decreases the opening time of NMDA receptors and hinders the development of association and behavioral learning.
4 Mismatch between top-down expectation and bottom-up perceptive input leads to unstable identity
4.1 Blockade of interoception leads to hallucination and diffusion of self-boundaries
The similarity between reports of unusual sensory/spiritual experiences and visual thinking of savant-gifted individuals was noted early in Gastaut–Geschwind syndrome (112, 116, 117). The inclination toward mysticism and excessive religiosity, which have often been seen in great individuals with special talents, are also characteristic of temporal/frontal lobe epilepsy, and some have suggested that these are features of ASC (68, 343–346). There is a category named the “hard-to-explain, paranormal sensations” among savant abilities (287), and gifted children show a tendency to have a precocious interest in self-transcendent questions and report hard-to-explain experiences (347).
As described in 3.3.1, KS and ASC show atypical activities of the insula and cingulate cortex, that joint internal perception (interoception) and the top-down concept of self, and constituting the Salience Network, which switches attention between executive function and internal mind-wandering. A decrease in activity in these regions has also been observed in individuals with schizophrenia, with an increase in the severity of hallucinations and heightened functional connectivity between the DMN and the Central Executive Network (37)(Figure 1). This implies that failure to switch to the executive network while attending to external stimuli constitutes the core mechanism that induces positive symptoms in schizophrenia (30, 348). In a typical conscious state, the projection pathway of interoceptive perception works independently of the neural pathways from the primary somatosensory cortex. Functional coupling between these factors using the hallucinogen, psilocybin, is a model of the unique perceptual experiences of savant-gifted individuals (118–120).
Bioactive compounds which work as psychedelics, such as serotonin, lysergic acid diethylamide (LSD), psilocybin, and dimethyltryptamine (DMT), act on the serotonin receptor, 5-HT2A, and mainly induce visual hallucinations and mystical feelings. Cortical hyperactivation of 5-HT2A has been suggested to disrupt the thalamic gating of sensory and cognitive information (349, 350). Approximately one-third of the autistic population show 50–70% increase in blood serotonin levels (351, 352), which is called hyperserotonemia. Hyperserotonemia is more prevalent in the prepubescent and male populations. The density of serotonin transporters and 5-HT2 is decreased in adult ASC brains, especially in the anterior cingulate cortex (353, 354), suggesting the dysregulation of serotonin function. Selective serotonin reuptake inhibitors work on subsets of individuals with ASC to ameliorate adverse behaviors (352). Serotonin dysregulation is also observed in individuals with FXS (355). A delay in GABA shift disturbs the shift from serotonergic to dopaminergic neurons (Figure 2).
While individuals with schizophrenia experience depersonalization feeling and the sense of being manipulated by others, ASC has the sensory peculiarity as “difficulty in understanding the boundaries between self and others” (149). The central area that generates the subjective awareness of “self” as a reference to the surrounding environment is the cortical mediomedial structure within the DMN, which is active during self-resuming mind wandering (148). Activity in the DMN (medial prefrontal cortex, anterior/medial cingulate, and inferior parietal lobule), while performing self-referential tasks was consistently lower in the ASC in various experimental paradigms, suggesting that the highest-order self-concept is difficult to form (150).
The Bayesian brain model based on the free energy principle suggests that disruption of the interoception/Salience Network results in overfocusing on sensory input, and vice versa (28, 40, 41), which manifests as sensory oversensitivity in various NDDs and savants/gifted individuals (155). At the same time, the failure to select and hand over that external information to internal references, that form the concept of the world and self, results in a conscious state similar to that under the effect of sensory deprivation and hallucinogens.
5 Discussion and future direction
In this paper, we reviewed the evidence supporting that ASC is likely to co-occur with atypical sex differentiation, androgyny, contrary to the prediction by the EMB. We discussed its physiological factors and specificity of perceptual processing shared by savants and gifted individuals, with reference to the conditions of various sex differentiation diversities.
Although different neurosteroids affect the regulation of GABA shift (Figure 2) and subsequent behaviors in a unique manner (257), estradiol and ERβ seem to be among the main upstream factors that control early neural cell development and distribution, and the maturation timing of the developing brain. Theoretically, excess estrogen during early development can delay this shift; however, the observation of syndromic NDD cases and emergence patterns of giftedness suggest that hypogonadism is a more prevalent cause of such atypicality. Apparent hyperandrogenism can be explained by a reflection of deficiency in estrogen regulation in animal studies and human clinical cases. Combining the neurodevelopmental hypothesis with the psychological EPF model, we suggest a new paradigm to explain putative sex differences in strength/weakness in cognitive traits. They might be influenced by the balance of steroid actions, but not in a simple dichotomized manner, such as male-favor/female-favor. As it is indicated by strong neurosteroid function of estradiol, many apparent “male-favor” cognitive function is likely to be affected by estrogen or ERβ action (Table 1).
Variations in the endophenotypes of NDDs can be explained by alterations in shift timing and weight differences in each neural factor, caused by genetic and environmental influences. For example, the same SCA states show diverse manifestations of symptoms, ranging from none to ASC, schizophrenia, ADHD, and their combination. The insufficient functionality of androgen and estrogen is known to hinder the typical function of ASC-related genes, such as FOXP2 and SHANK3, which can lead to an ASC-like phenotype. If only EPF is apparent, difficulties in language processing and symptoms of dyslexia may be more pronounced. If the imbalance between disinhibition of neural activity, control of executive function, and attention switching is more pronounced, it would lead to more ADHD-like features than ASC (356).
In humans, the critical period for the GABA shift extends postnatally to the first 12 months. The primary cause of shift delays and various NDDs is considered to be the downregulation of Potassium Chloride Cotransporter 2 (KCC2) (194). Steroid regulation of the shift varies across brain areas and with the subject’s sex, requiring a thorough examination of each endophenotype. Sex differences appear even before the peak of androgen secretion from the testes, suggesting a genetic background that affects endocrinological influences. Additionally, toxicological analysis has shown that the primary critical period for ASC is very early, starting from the time of neural tube closure to the end of second trimester. This occurs slightly later in schizophrenia, culminating between first and second trimesters (357). The risk periods extend to over three years of age for ADHD (357). The first and second trimesters are time windows for neurogenesis and neuronal migration (358). If estrogen deficiencies are critical in deriving NDD endophenotypes in hypogonadal individuals, as we suggested, the primary factor to produce ASC-like symptoms would be the insufficiency of ERβ functions in neural cell development, while each manifestation type will be later modified by the maturation shift in each neural system for individual timing.
The free energy principle suggests that in ASC, sensory perception is too precise, while top-down inference is weak, inducing a large Bayesian surprise at every time of perception, requiring recalculation (41). In contrast, perceptive information is less precise in schizophrenia, allowing inner models to go astray and elude from correcting inferences (359). Further studies are required to clarify when and how receptive precision diversifies across these two conditions.
The formation of an inner image and its extension to an atypical sensation, such as a feeling of dissociation or synesthesia, is a cue for understanding underlying mechanisms. Pharmacologically and epidemiologically, serotonergic hyperactivation of 5-HT2A induces a mismatch between top-down self-image inference and bottom-up perception, transcending the boundaries of input source areas, leading to deep hallucinations, including ego dissolution (119). Thus, ASC and gifted individuals, not only those with schizophrenia, tend to have unusual beliefs. Serotonergic regulation is disrupted in ASC, while intracellular concentration of 5-HT is high in many of them. The extended immature phase before the GABA shift may be the cause of the atypicality of the serotonergic pathway; however, its interaction with steroids and oxytocin function is yet to be elucidated.
Recent progress in the therapeutic use of psychedelics is likely to open the way for answering this question. A drug called ‘ecstasy,’ 3,4-Methylenedioxymethamphetamine (MDMA) promotes affiliative feeling toward others. While MDMA does not bind to the 5-HT2 receptor, it induces the release of serotonin and oxytocin. This drug is effective in alleviating anxiety in individuals with ASC or post-traumatic stress disorder, opening a critical period for social reward learning neural circuits for reinnervation (360). A rodent study demonstrated that the critical period opening characteristic is not limited to MDMA, but is shared with LSD and psylocibin, which worked on 5-HT2 (361). Psychedelics with various activating targets result in the modulation of extracellular matrix, such as fibronectin, receptors, and proteases, allowing the re-wiring of the social reward learning system. The extent to which such after-corrections have a durable effect in individuals with altered endocrinological environments, such as hypogonadism, requires further investigation.
Data availability statement
The original contributions presented in the study are included in the article/supplementary material. Further inquiries can be directed to the corresponding author/s.
Ethics statement
Ethical approval was not required for the study involving humans in accordance with the local legislation and institutional requirements. Written informed consent to participate in this study was not required from the participants or the participants’ legal guardians/next of kin in accordance with the national legislation and the institutional requirements.
Author contributions
KS: Writing – review & editing, Writing – original draft, Visualization, Investigation, Funding acquisition, Conceptualization. ST: Writing – review & editing, Investigation.
Funding
The author(s) declare financial support was received for the research, authorship, and/or publication of this article. This work was supported by the JSPS Topic-Setting Program to Advance Cutting-Edge Humanities and Social Sciences Research, Grant Number JPJS00122674991.
Acknowledgments
The authors thank Dr. Atsuko Saito from Sophia University for supervising ST and providing an environment to focus on the research project.
Conflict of interest
The authors declare that the research was conducted in the absence of any commercial or financial relationships that could be construed as a potential conflict of interest.
Publisher’s note
All claims expressed in this article are solely those of the authors and do not necessarily represent those of their affiliated organizations, or those of the publisher, the editors and the reviewers. Any product that may be evaluated in this article, or claim that may be made by its manufacturer, is not guaranteed or endorsed by the publisher.
Abbreviations
ASC, autism spectrum condition; KS, Klinefelter syndrome; PWS, Prader-Willi syndrome; FXS, Fragile X syndrome; PWP, Prader-Willi phenotype; TD, typically developed; NDD, neurodevelopmental disorder; IQ, intelligence quotient; EBM, extreme male brain theory; ADHD, attention deficit hyperactivity disorder; 2D:4D, 2nd to 4th digit ratio; SCA, sex chromosome aneuploidy; CAH, congenital adrenal hyperplasia; PCOS, polycystic ovarian syndrome; GABA, γ-aminobutyric acid; NMDA, N-methyl-D-aspartate; DMN, default mode network; HPG axis, hypothalamic pituitary gonadal axis; AR, androgen receptor; ER, estrogen receptor; GPER, G protein-coupled estrogen receptor; DHT, dihydrotestosterone; LH, luteinizing hormone; LSD, lysergic acid diethylamide; 5-HT, 5-hydroxytryptamine (serotonin); MDMA, 3,4-methylenedioxymethamphetamine.
References
1. Loomes R, Hull L, Mandy WPL. What is the male-to-female ratio in Autism Spectrum Disorder? A systematic review and meta-analysis. J Am Acad Child Adolesc Psychiatry. (2017) 56:466–74. doi: 10.1016/j.jaac.2017.03.013
2. Burrows CA, Grzadzinski RL, Donovan K, Stallworthy IC, Rutsohn J, St John T, et al. A data driven approach in an unbiased sample reveals equivalent sex ratio of autism spectrum disorder associated impairment in early childhood. Biol Psychiatry. (2022) 92:654–62. doi: 10.1016/j.biopsych.2022.05.027
3. Hines M. Brain Gender. Oxford, United Kingdom: Oxford University Press (2005). p. 295. doi: 10.1093/acprof:oso/9780195188363.001.1
4. Halpern DF, Benbow CP, Geary DC, Gur RC, Hyde JS, Gernsbacher MA. The science of sex differences in science and mathematics. Psychol Sci Public Interest. (2007) 8:1–51. doi: 10.1111/j.1529-1006.2007.00032.x
5. Geschwind N, Behan P. Left-handedness: association with immune disease, migraine, and developmental learning disorder. Proc Natl Acad Sci. (1982) 79:5097–100. doi: 10.1073/pnas.79.16.5097
6. Geschwind N, Galaburda A. Cerebral lateralization. Biological mechanisms, associations, and pathology: II. A hypothesis and a program for research. Arch Neurol. (1985) 42:521–2. doi: 10.1001/archneur.1985.04060060019009
7. Baron-Cohen S. The extreme male brain theory of autism. Trends Cognit Sci. (2002) 6:248–54. doi: 10.1016/S1364-6613(02)01904-6
8. Greenberg DM, Warrier V, Allison C, Baron-Cohen S. Testing the Empathizing–Systemizing theory of sex differences and the Extreme Male Brain theory of autism in half a million people. Proc Natl Acad Sci. (2018) 115:12152–7. doi: 10.1073/pnas.1811032115
9. Baron-Cohen S, Knickmeyer RC, Belmonte MK. Sex differences in the brain: implications for explaining autism. Science. (2005) 310:819–23. doi: 10.1126/science.1115455
10. Valla JM, Ceci SJ. Can sex differences in science be tied to the long reach of prenatal hormones? Brain organization theory, digit ratio (2D/4D), and sex differences in preferences and cognition. Perspect Psychol Sci. (2011) 6:134–46. doi: 10.1177/1745691611400236
11. Worsham W, Dalton S, Bilder DA. The prenatal hormone milieu in autism spectrum disorder. Front Psychiatry. (2021) 12:655438. doi: 10.3389/fpsyt.2021.655438
12. Falter CM, Plaisted KC, Davis G. Male brains, androgen, and the cognitive profile in autism: convergent evidence from 2D: 4D and congenital adrenal hyperplasia. J Autism Dev Disord. (2008) 38:997–8. doi: 10.1007/s10803-008-0552-z
13. Lai M-C, Lombardo MV, Suckling J, Ruigrok ANV, Chakrabarti B, Ecker C, et al. Biological sex affects the neurobiology of autism. Brain. (2013) 136:2799–815. doi: 10.1093/brain/awt216
14. Lenroot R, Yeung PK. Heterogeneity within autism spectrum disorders: what have we learned from neuroimaging studies? Front Hum Neurosci. (2013) 7:733. doi: 10.3389/fnhum.2013.00733
15. Kong X, Zhu J, Tian R, Liu S, Sherman HT, Zhang X, et al. Early screening and risk factors of autism spectrum disorder in a large cohort of Chinese patients with Prader-Willi syndrome. Front Psychiatry. (2020) 11:594934. doi: 10.3389/fpsyt.2020.594934
16. Gravholt CH, Chang S, Wallentin M, Fedder J, Moore P, Skakkebæk A. Klinefelter syndrome: integrating genetics, neuropsychology, and endocrinology. Endocr Rev. (2018) 39:389–423. doi: 10.1210/er.2017-00212
17. SFARI Gene - Welcome. SFARI Gene . Available online at: https://gene.sfari.org/ (Accessed 14, 2023).
18. Anney RJL, Ripke S, Anttila V, Grove J, Holmans P, Huang H, et al. Meta-analysis of GWAS of over 16,000 individuals with autism spectrum disorder highlights a novel locus at 10q24.32 and a significant overlap with schizophrenia. Mol Autism. (2017) 8:21. doi: 10.1186/s13229-017-0137-9
19. Argyropoulos A, Gilby K, Hill-Yardin E. Studying autism in rodent models: reconciling endophenotypes with comorbidities. Front Hum Neurosci. (2013) 7:417. doi: 10.3389/fnhum.2013.00417
20. George R, Stokes MA. Gender identity and sexual orientation in autism spectrum disorder. Autism. (2018) 22:970–82. doi: 10.1177/1362361317714587
21. Qualls LR, Hartmann K, Paulson JF. Broad autism phenotypic traits and the relationship to sexual orientation and sexual behavior. J Autism Dev Disord. (2018) 48:3974–83. doi: 10.1007/s10803-018-3556-3
22. Rudolph CES, Lundin A, Åhs JW, Dalman C, Kosidou K. Brief report: sexual orientation in individuals with autistic traits: population based study of 47,000 adults in Stockholm county. J Autism Dev Disord. (2018) 48:619–24. doi: 10.1007/s10803-017-3369-9
23. Janssen A, Huang H, Duncan C. Gender variance among youth with autism spectrum disorders: a retrospective chart review. Transgender Health. (2016) 1:63–8. doi: 10.1089/trgh.2015.0007
24. de Vries ALC, Noens ILJ, Cohen-Kettenis PT, van Berckelaer-Onnes IA, Doreleijers TA. Autism spectrum disorders in gender dysphoric children and adolescents. J Autism Dev Disord. (2010) 40:930–6. doi: 10.1007/s10803-010-0935-9
25. Tawata S, Sakaguchi K, Saito A. [Cognitive characteristics, gender dysphoria, and hormonal treatment options in Klinefelter’s syndrome]. J Jpn Soc Gend Identity Disord. (2022) 15:119–32.
26. Rochira V, Carani C. Aromatase deficiency in men: a clinical perspective. Nat Rev Endocrinol. (2009) 5:559–68. doi: 10.1038/nrendo.2009.176
27. Zirilli L, Rochira V, Diazzi C, Caffagni G, Carani C. Human models of aromatase deficiency. J Steroid Biochem Mol Biol. (2008) 109:212–8. doi: 10.1016/j.jsbmb.2008.03.026
28. Quattrocki E, Friston K. Autism, oxytocin and interoception. Neurosci Biobehav Rev. (2014) 47:410–30. doi: 10.1016/j.neubiorev.2014.09.012
29. Ide M, Wada M. Salivary oxytocin concentration associates with the subjective feeling of body ownership during the rubber hand illusion. Front Hum Neurosci. (2017) 11:166. doi: 10.3389/fnhum.2017.00166
30. Palaniyappan L, Liddle PF. Does the salience network play a cardinal role in psychosis? An emerging hypothesis of insular dysfunction. J Psychiatry Neurosci. (2012) 37:17–27. doi: 10.1503/jpn.100176
31. Swaab DF, Purba JS, Hofman MA. Alterations in the hypothalamic paraventricular nucleus and its oxytocin neurons (putative satiety cells) in Prader-Willi syndrome: a study of five cases. J Clin Endocrinol Metab. (1995) 80:573–9. doi: 10.1210/jcem.80.2.7852523
32. Johnson L, Manzardo AM, Miller JL, Driscoll DJ, Butler MG. Elevated plasma oxytocin levels in children with Prader–Willi syndrome compared with healthy unrelated siblings. Am J Med Genet A. (2016) 170:594–601. doi: 10.1002/ajmg.a.37488
33. Kritzer MF. Regional, laminar, and cellular distribution of immunoreactivity for ERα and ERβ in the cerebral cortex of hormonally intact, adult male and female rats. Cereb Cortex. (2002) 12:116–28. doi: 10.1093/cercor/12.2.116
34. Papadea D, Dalla C, Tata DA. Exploring a possible interplay between schizophrenia, oxytocin, and estrogens: a narrative review. Brain Sci. (2023) 13:461. doi: 10.3390/brainsci13030461
35. Caria A, de Falco S. Anterior insular cortex regulation in autism spectrum disorders. Front Behav Neurosci. (2015) 9:38. doi: 10.3389/fnbeh.2015.00038
36. Nomi JS, Molnar-Szakacs I, Uddin LQ. Insular function in autism: update and future directions in neuroimaging and interventions. Prog Neuropsychopharmacol Biol Psychiatry. (2019) 89:412–26. doi: 10.1016/j.pnpbp.2018.10.015
37. Manoliu A, Riedl V, Zherdin A, Mühlau M, Schwerthöffer D, Scherr M, et al. Aberrant dependence of default mode/central executive network interactions on anterior insular salience network activity in schizophrenia. Schizophr Bull. (2014) 40:428–37. doi: 10.1093/schbul/sbt037
38. Hazlett HC, Gu H, Munsell BC, Kim SH, Styner M, Wolff JJ, et al. Early brain development in infants at high risk for autism spectrum disorder. Nature. (2017) 542:348–51. doi: 10.1038/nature21369
39. Luders E, Sánchez F, Tosun D, Shattuck D, Gaser C, Vilain E, et al. Increased cortical thickness in male-to-female transsexualism. J Behav Brain Sci. (2012) 2:357–62. doi: 10.4236/jbbs.2012.23040
40. Lawson RP, Rees G, Friston KJ. An aberrant precision account of autism. Front Hum Neurosci. (2014) 8:302. doi: 10.3389/fnhum.2014.00302
41. Inui T, Kumagaya S, Myowa-Yamakoshi M. Neurodevelopmental hypothesis about the etiology of autism spectrum disorders. Front Hum Neurosci. (2017) 11:354. doi: 10.3389/fnhum.2017.00354
42. Trent S, Davies W. The influence of sex-linked genetic mechanisms on attention and impulsivity. Biol Psychol. (2012) 89:1–13. doi: 10.1016/j.biopsycho.2011.09.011
43. Eysenck HJ. Genius: The Natural History of Creativity. Cambridge: Cambridge University Press (1995). p. 354. doi: 10.1017/CBO9780511752247
44. Alsemari A. Hypogonadism and neurological diseases. Neurol Sci. (2013) 34:629–38. doi: 10.1007/s10072-012-1278-4
45. Wilson HA, Creighton C, Scharfman H, Choleris E, MacLusky NJ. Endocrine insights into the pathophysiology of autism spectrum disorder. Neuroscientist. (2021) 27:650–67. doi: 10.1177/1073858420952046
46. Boks MPM, de Vette MHT, Sommer IE, van Rijn S, Giltay JC, Swaab H, et al. Psychiatric morbidity and X-chromosomal origin in a Klinefelter sample. Schizophr Res. (2007) 93:399–402. doi: 10.1016/j.schres.2007.03.015
47. Abramsky L, Chapple J. 47,XXY (Klinefelter syndrome) and 47,XYY: estimated rates of an indication for postnatal diagnosis with implications for prenatal counselling. Prenat Diagn. (1997) 17:363–8. doi: 10.1002/(sici)1097-0223(199704)17:4<363::aid-pd79>3.0.co;2-o
48. Zhao Y, Gardner EJ, Tuke MA, Zhang H, Pietzner M, Koprulu M, et al. Detection and characterization of male sex chromosome abnormalities in the UK Biobank study. Genet Med. (2022) 24:1909–19. doi: 10.1016/j.gim.2022.05.011
49. Herlihy AS, Halliday JL, Cock ML, McLachlan RI. The prevalence and diagnosis rates of Klinefelter syndrome: an Australian comparison. Med J Aust. (2011) 194:24–8. doi: 10.5694/j.1326-5377.2011.tb04141.x
50. Bojesen A, Juul S, Gravholt CH. Prenatal and postnatal prevalence of Klinefelter syndrome: a national registry study. J Clin Endocrinol Metab. (2003) 88:622–6. doi: 10.1210/jc.2002-021491
51. Girardin CM, Lemyre E, Alos N, Deal C, Huot C, Van Vliet G. Comparison of adolescents with Klinefelter syndrome according to the circumstances of diagnosis: amniocentesis versus clinical signs. Horm Res. (2009) 72:98–105. doi: 10.1159/000232162
52. Bruining H, Swaab H, Kas M, van Engeland H. Psychiatric characteristics in a self-selected sample of boys with Klinefelter syndrome. Pediatrics. (2009) 123:e865–70. doi: 10.1542/peds.2008-1954
53. Hong DS, Reiss AL. Cognitive and neurological aspects of sex chromosome aneuploidies. Lancet Neurol. (2014) 13:306–18. doi: 10.1016/S1474-4422(13)70302-8
54. Bonomi M, Rochira V, Pasquali D, Balercia G, Jannini EA, Ferlin A, et al. Klinefelter syndrome (KS): genetics, clinical phenotype and hypogonadism. J Endocrinol Invest. (2017) 40:123–34. doi: 10.1007/s40618-016-0541-6
55. Jha P, Sheth D, Ghaziuddin M. Autism spectrum disorder and Klinefelter syndrome. Eur Child Adolesc Psychiatry. (2007) 16:305–8. doi: 10.1007/s00787-007-0601-8
56. Gropman A, Samango-Sprouse CA. Neurocognitive variance and neurological underpinnings of the X and Y chromosomal variations. Am J Med Genet C Semin Med Genet. (2013) 163:35–43. doi: 10.1002/ajmg.c.31352
57. Tartaglia N, Ayari N, Howell S, D’Epagnier C, Zeitler P. 48,XXYY, 48,XXXY and 49,XXXXY syndromes: not just variants of Klinefelter syndrome. Acta Paediatr. (2011) 100:851–60. doi: 10.1111/j.1651-2227.2011.02235.x
58. Cordeiro L, Tartaglia N, Roeltgen D, Ross J. Social deficits in male children and adolescents with sex chromosome aneuploidy: a comparison of XXY, XYY, and XXYY syndromes. Res Dev Disabil. (2012) 33:1254–63. doi: 10.1016/j.ridd.2012.02.013
59. Leggett V, Jacobs P, Nation K, Scerif G, Bishop DVM. Neurocognitive outcomes of individuals with a sex chromosome trisomy: XXX, XYY, or XXY: a systematic review. Dev Med Child Neurol. (2010) 52:119–29. doi: 10.1111/j.1469-8749.2009.03545.x
60. Walzer S, Graham J, Bashir A, Silbert A. Preliminary observations on language and learning in XXY boys. Birth Defects Orig Artic Ser. (1982) 18:185–92.
61. van Rijn S, Bierman M, Bruining H, Swaab H. Vulnerability for autism traits in boys and men with an extra X chromosome (47,XXY): the mediating role of cognitive flexibility. J Psychiatr Res. (2012) 46:1300–6. doi: 10.1016/j.jpsychires.2012.06.004
62. Sorokowski P, Karwowski M, Misiak M, Marczak MK, Dziekan M, Hummel T, et al. Sex differences in human olfaction: a meta-analysis. Front Psychol. (2019) 10:242. doi: 10.3389/fpsyg.2019.00242
63. Herrero MJ, Wang L, Hernandez-Pineda D, Banerjee P, Matos HY, Goodrich M, et al. Sex-specific social behavior and amygdala proteomic deficits in Foxp2+/– mutant mice. Front Behav Neurosci. (2021) 15:706079. doi: 10.3389/fnbeh.2021.706079
64. Cooke BM, Breedlove SM, Jordan CL. Both estrogen receptors and androgen receptors contribute to testosterone-induced changes in the morphology of the medial amygdala and sexual arousal in male rats. Horm Behav. (2003) 43:336–46. doi: 10.1016/S0018-506X(02)00047-8
65. Cao J, Patisaul HB. Sex-specific expression of estrogen receptors α and β and Kiss1 in the postnatal rat amygdala. J Comp Neurol. (2013) 521:465–78. doi: 10.1002/cne.23185
66. Cameron EL. Pregnancy and olfaction: a review. Front Psychol. (2014) 5:67. doi: 10.3389/fpsyg.2014.00067
67. Renfro KJ, Hoffmann H. The relationship between oral contraceptive use and sensitivity to olfactory stimuli. Horm Behav. (2013) 63:491–6. doi: 10.1016/j.yhbeh.2013.01.001
69. Ashwin C, Chapman E, Howells J, Rhydderch D, Walker I, Baron-Cohen S. Enhanced olfactory sensitivity in autism spectrum conditions. Mol Autism. (2014) 5:53. doi: 10.1186/2040-2392-5-53
70. Chen C, Shih Y-H, Yen D-J, Lirng J-F, Guo Y-C, Yu H-Y, et al. Olfactory auras in patients with temporal lobe epilepsy. Epilepsia. (2003) 44:257–60. doi: 10.1046/j.1528-1157.2003.25902.x
71. Fornazieri MA, Prina DMC, Favoreto JPM, Rodrigues e Silva K, Ueda DM, de Rezende Pinna F, et al. Olfaction during pregnancy and postpartum period. Chemosens Percept. (2019) 12:125–34. doi: 10.1007/s12078-019-09259-7
72. Galle SA, Courchesne V, Mottron L, Frasnelli J. Olfaction in the autism spectrum. Perception. (2013) 42:341–55. doi: 10.1068/p7337
73. Kumazaki H, Muramatsu T, Fujisawa TX, Miyao M, Matsuura E, Okada K, et al. Assessment of olfactory detection thresholds in children with autism spectrum disorders using a pulse ejection system. Mol Autism. (2016) 7:6. doi: 10.1186/s13229-016-0071-2
74. Koehler L, Fournel A, Albertowski K, Roessner V, Gerber J, Hummel C, et al. Impaired odor perception in autism spectrum disorder is associated with decreased activity in olfactory cortex. Chem Senses. (2018) 43:627–34. doi: 10.1093/chemse/bjy051
75. Sweigert JR, St. John T, Begay KK, Davis GE, Munson J, Shankland E, et al. Characterizing olfactory function in children with autism spectrum disorder and children with sensory processing dysfunction. Brain Sci. (2020) 10:362. doi: 10.3390/brainsci10060362
76. Wicker B, Monfardini E, Royet J-P. Olfactory processing in adults with autism spectrum disorders. Mol Autism. (2016) 7:4. doi: 10.1186/s13229-016-0070-3
77. Aarde SM, Hrncir H, Arnold AP, Jentsch JD. Reversal learning performance in the XY∗ mouse model of Klinefelter and Turner syndromes. Front Behav Neurosci. (2019) 13:201. doi: 10.3389/fnbeh.2019.00201
78. Gómez-Gil E, Cañizares S, Torres A, de la Torre F, Halperin I, Salamero M. Androgen treatment effects on memory in female-to-male transsexuals. Psychoneuroendocrinology. (2009) 34:110–7. doi: 10.1016/j.psyneuen.2008.08.017
79. Wibowo E. Cognitive impacts of estrogen treatment in androgen-deprived males: what needs to be resolved. Curr Neuropharmacol. (2017) 15:1043–55. doi: 10.2174/1570159X15666170313122555
80. Foland-Ross LC, Ross JL, Reiss AL. Androgen treatment effects on hippocampus structure in boys with Klinefelter syndrome. Psychoneuroendocrinology. (2019) 100:223–8. doi: 10.1016/j.psyneuen.2018.09.039
81. Elliott CD. Differential Ability Scales: Introductory and Technical Handbook. San Antonio: Harcourt Brace Jovanovich, Inc (1990). 354 p.
82. Chien Y-L, Gau SS-F, Shang C-Y, Chiu Y-N, Tsai W-C, Wu Y-Y. Visual memory and sustained attention impairment in youths with autism spectrum disorders. Psychol Med. (2015) 45:2263–73. doi: 10.1017/S0033291714003201
83. Minshew NJ, Goldstein G. The pattern of intact and impaired memory functions in autism. J Child Psychol Psychiatry. (2001) 42:1095–101. doi: 10.1017/S0021963001007867
84. Williams DL, Goldstein G, Minshew NJ. The profile of memory function in children with autism. Neuropsychology. (2006) 20:21–9. doi: 10.1037/0894-4105.20.1.21
85. Geurts HM, Vissers ME. Elderly with autism: executive functions and memory. J Autism Dev Disord. (2012) 42:665–75. doi: 10.1007/s10803-011-1291-0
86. Knickmeyer RC, Davenport M. Turner syndrome and sexual differentiation of the brain: implications for understanding male-biased neurodevelopmental disorders. J Neurodev Disord. (2011) 3:293–306. doi: 10.1007/s11689-011-9089-0
87. Bruining H, Swaab H, Sonneville LMJde, Rijn Sv, Engeland Hv, Kas MJH. In search for significant cognitive features in Klinefelter syndrome through cross-species comparison of a supernumerary X chromosome. Genes Brain Behav. (2011) 10:658–62. doi: 10.1111/gbb.2011.10.issue-6
88. Cherrier MM, Craft S, Matsumoto AH. Cognitive changes associated with supplementation of testosterone or dihydrotestosterone in mildly hypogonadal men: a preliminary report. J Androl. (2003) 24:568–76. doi: 10.1002/j.1939-4640.2003.tb02708.x
89. Edinger KL, Frye CA. Androgens’ effects to enhance learning may be mediated in part through actions at estrogen receptor-β in the hippocampus. Neurobiol Learn Mem. (2007) 87:78–85. doi: 10.1016/j.nlm.2006.07.001
90. Rissman EF, Heck AL, Leonard JE, Shupnik MA, Gustafsson J-Å. Disruption of estrogen receptor β gene impairs spatial learning in female mice. Proc Natl Acad Sci. (2002) 99:3996–4001. doi: 10.1073/pnas.012032699
91. McGinty HL, Phillips KM, Jim HSL, Cessna JM, Asvat Y, Cases MG, et al. Cognitive functioning in men receiving androgen deprivation therapy for prostate cancer: a systematic review and meta-analysis. Support Care Cancer. (2014) 22:2271–80. doi: 10.1007/s00520-014-2285-1
92. Neubauer AC, Bergner S, Schatz M. Two- vs. three-dimensional presentation of mental rotation tasks: sex differences and effects of training on performance and brain activation. Intelligence. (2010) 38:529–39. doi: 10.1016/j.intell.2010.06.001
93. Silk TJ, Rinehart N, Bradshaw JL, Tonge B, Egan G, O’Boyle MW, et al. Visuospatial processing and the function of prefrontal-parietal networks in autism spectrum disorders: a functional MRI study. Am J Psychiatry. (2006) 163:1440–3. doi: 10.1176/ajp.2006.163.8.1440
94. McGrath J, Johnson K, Ecker C, O’Hanlon E, Gill M, Gallagher L, et al. Atypical visuospatial processing in autism: insights from functional connectivity analysis. Autism Res. (2012) 5:314–30. doi: 10.1002/aur.1245
95. Koshino H, Carpenter PA, Minshew NJ, Cherkassky VL, Keller TA, Just MA. Functional connectivity in an fMRI working memory task in high-functioning autism. NeuroImage. (2005) 24:810–21. doi: 10.1016/j.neuroimage.2004.09.028
96. Puts DA, McDaniel MA, Jordan CL, Breedlove SM. Spatial ability and prenatal androgens: meta-analyses of congenital adrenal hyperplasia and digit ratio (2D: 4D) studies. Arch Sex Behav. (2008) 37:100–11. doi: 10.1007/s10508-007-9271-3
97. Sankar JS, Hampson E. Androgen receptor polymorphism, mental rotation, and spatial visualization in men. Psychoneuroendocrinology. (2021) 129:105239. doi: 10.1016/j.psyneuen.2021.105239
98. Arevalo M-A, Santos-Galindo M, Bellini M-J, Azcoitia I, Garcia-Segura LM. Actions of estrogens on glial cells: implications for neuroprotection. Biochim Biophys Acta BBA - Gen Subj. (2010) 1800:1106–12. doi: 10.1016/j.bbagen.2009.10.002
99. Nowak NT, Diamond MP, Land SJ, Moffat SD. Contributions of sex, testosterone, and androgen receptor CAG repeat number to virtual Morris water maze performance. Psychoneuroendocrinology. (2014) 41:13–22. doi: 10.1016/j.psyneuen.2013.12.003
100. Hampson E. Spatial cognition in humans: possible modulation by androgens and estrogens. J Psychiatry Neurosci. (1995) 20:397–404.
101. Courvoisier DS, Renaud O, Geiser C, Paschke K, Gaudy K, Jordan K. Sex hormones and mental rotation: an intensive longitudinal investigation. Horm Behav. (2013) 63:345–51. doi: 10.1016/j.yhbeh.2012.12.007
102. Folkierska-Żukowska M, Rahman Q, Marchewka A, Wypych M, Droździel D, Sokołowski A, et al. Male sexual orientation, gender nonconformity, and neural activity during mental rotations: an fMRI study. Sci Rep. (2020) 10:18709. doi: 10.1038/s41598-020-74886-0
103. Kozaki T, Yasukouchi A. Relationships between salivary estradiol and components of mental rotation in young men. J Physiol Anthropol. (2008) 27:19–24. doi: 10.2114/jpa2.27.19
104. Berenbaum SA, Bryk KLK, Beltz AM. Early androgen effects on spatial and mechanical abilities: evidence from congenital adrenal hyperplasia. Behav Neurosci. (2012) 126:86–96. doi: 10.1037/a0026652
105. Griksiene R, Monciunskaite R, Arnatkeviciute A, Ruksenas O. Does the use of hormonal contraceptives affect the mental rotation performance? Horm Behav. (2018) 100:29–38. doi: 10.1016/j.yhbeh.2018.03.004
106. Chakravarty A. Artistic talent in dyslexia—a hypothesis. Med Hypotheses. (2009) 73:569–71. doi: 10.1016/j.mehy.2009.05.034
107. Aydin K, Ucar A, Oguz KK, Okur OO, Agayev A, Unal Z, et al. Increased gray matter density in the parietal cortex of mathematicians: a voxel-based morphometry study. Am J Neuroradiol. (2007) 28:1859–64. doi: 10.3174/ajnr.A0696
108. Dehaene S. The Number Sense: How the Mind Creates Mathematics. New York: Oxford Univ Pr (1997). 274 p.
109. James IM. Asperger’s Syndrome and High Achievement: Some Very Remarkable People. London: Jessica Kingsley Publishers (2006). p. 226.
110. Luria AR. The Mind of a Mnemonist: A Little Book about a Vast Memory. New York: Basic Books (1968). 160 p.
111. Padgett J, Seaberg MA. Struck by Genius: How a Brain Injury Made Me a Mathematical Marvel. New ed. Boston: Houghton Mifflin Harcourt (2014). 256 p.
112. Ramachandran VS. Phantoms in the Brain: Probing the Mysteries of the Human Mind. New York: William Morrow (1998). 320 p.
113. Robison JE. Be Different: Adventures of a Free-Range Aspergian with Practical Advice for Aspergians, Misfits, Families & Teachers. New York: Crown (2011). 304 p.
114. Bouvet L, Amsellem F, Maruani A, Tonus-Vic Dupont A, Mathieu A, Bourgeron T, et al. Synesthesia & autistic features in a large family: evidence for spatial imagery as a common factor. Behav Brain Res. (2019) 362:266–72. doi: 10.1016/j.bbr.2019.01.014
115. Hughes JEA, Gruffydd E, Simner J, Ward J. Synesthetes show advantages in savant skill acquisition: training calendar calculation in sequence-space synaesthesia. Cortex. (2019) 113:67–82. doi: 10.1016/j.cortex.2018.11.023
117. Waxman SG, Geschwind N. Hypergraphia in temporal lobe epilepsy. Neurology. (1974) 24:629–9. doi: 10.1212/WNL.24.7.629
118. dos Santos RG, Osório FL, Crippa JAS, Hallak JEC. Classical hallucinogens and neuroimaging: a systematic review of human studies: hallucinogens and neuroimaging. Neurosci Biobehav Rev. (2016) 71:715–28. doi: 10.1016/j.neubiorev.2016.10.026
119. Tagliazucchi E, Roseman L, Kaelen M, Orban C, Muthukumaraswamy SD, Murphy K, et al. Increased global functional connectivity correlates with LSD-induced ego dissolution. Curr Biol. (2016) 26:1043–50. doi: 10.1016/j.cub.2016.02.010
120. Petri G, Expert P, Turkheimer F, Carhart-Harris R, Nutt D, Hellyer PJ, et al. Homological scaffolds of brain functional networks. J R Soc Interface. (2014) 11:20140873. doi: 10.1098/rsif.2014.0873
121. Hughes JEA, Ward J, Gruffydd E, Baron-Cohen S, Smith P, Allison C, et al. Savant syndrome has a distinct psychological profile in autism. Mol Autism. (2018) 9:53. doi: 10.1186/s13229-018-0237-1
122. Wolff U, Lundberg I. The prevalence of Dyslexia among art students. Dyslexia. (2002) 8:34–42. doi: 10.1002/dys.211
123. Boone KB, Swerdloff RS, Miller BL, Geschwind DH, Razani J, Lee A, et al. Neuropsychological profiles of adults with Klinefelter syndrome. J Int Neuropsychol Soc. (2001) 7:446–56. doi: 10.1017/S1355617701744013
124. Patwardhan AJ, Eliez S, Bender B, Linden MG, Reiss AL. Brain morphology in Klinefelter syndrome: extra X chromosome and testosterone supplementation. Neurology. (2000) 54:2218–23. doi: 10.1212/WNL.54.12.2218
125. Alexander GM, Swerdloff RS, Wang C, Davidson T, McDonald V, Steiner B, et al. Androgen–behavior correlations in hypogonadal men and eugonadal men. Horm Behav. (1997) 31:110–9. doi: 10.1006/hbeh.1997.1372
126. Davis SM, Bloy L, Roberts TPL, Kowal K, Alston A, Tahsin A, et al. Testicular function in boys with 47,XYY and relationship to phenotype. Am J Med Genet C Semin Med Genet. (2020) 184:371–85. doi: 10.1002/ajmg.c.31790
127. McLennan Y, Polussa J, Tassone F, Hagerman R. Fragile X syndrome. Curr Genomics. (2011) 12:216–24. doi: 10.2174/138920211795677886
128. van Rijn S, Barendse M, Goozen Sv, Swaab H. Social attention, affective arousal and empathy in men with Klinefelter syndrome (47, XXY): evidence from eyetracking and skin conductance. PloS One. (2014) 9:e84721. doi: 10.1371/journal.pone.0084721
129. Green T, Flash S, Reiss AL. Sex differences in psychiatric disorders: what we can learn from sex chromosome aneuploidies. Neuropsychopharmacology. (2019) 44:9–21. doi: 10.1038/s41386-018-0153-2
130. Oxelgren UW, Myrelid Å, Annerén G, Ekstam B, Göransson C, Holmbom A, et al. Prevalence of autism and attention-deficit–hyperactivity disorder in Down syndrome: a population-based study. Dev Med Child Neurol. (2017) 59:276–83. doi: 10.1111/dmcn.13217
131. Reilly C. Autism spectrum disorders in Down syndrome: a review. Res Autism Spectr Disord. (2009) 3:829–39. doi: 10.1016/j.rasd.2009.01.012
132. Grieco J, Pulsifer M, Seligsohn K, Skotko B, Schwartz A. Down syndrome: cognitive and behavioral functioning across the lifespan. Am J Med Genet C Semin Med Genet. (2015) 169:135–49. doi: 10.1002/ajmg.c.31439
133. May T, Yi KLJ, Loveland KL, Vollenhoven B, Williams K. Overlap of autism and conditions associated with atypical sex hormone levels or response: a systematic review and meta-analysis. Res Autism Spectr Disord. (2021) 80:101693. doi: 10.1016/j.rasd.2020.101693
134. Rotem RS, Nguyen VT, Chodick G, Davidovitch M, Shalev V, Hauser R, et al. Associations of maternal androgen-related conditions with risk of autism spectrum disorder in progeny and mediation by cardiovascular, metabolic, and fertility factors. Am J Epidemiol. (2021) 190:600–10. doi: 10.1093/aje/kwaa219
135. Schwarz E, Guest PC, Rahmoune H, Wang L, Levin Y, Ingudomnukul E, et al. Sex-specific serum biomarker patterns in adults with Asperger’s syndrome. Mol Psychiatry. (2011) 16:1213–20. doi: 10.1038/mp.2010.102
136. Crider A, Thakkar R, Ahmed AO, Pillai A. Dysregulation of estrogen receptor beta (ERβ), aromatase (CYP19A1), and ER co-activators in the middle frontal gyrus of autism spectrum disorder subjects. Mol Autism. (2014) 5:46. doi: 10.1186/2040-2392-5-46
137. Doi H, Fujisawa TX, Iwanaga R, Matsuzaki J, Kawasaki C, Tochigi M, et al. Association between single nucleotide polymorphisms in estrogen receptor 1/2 genes and symptomatic severity of autism spectrum disorder. Res Dev Disabil. (2018) 82:20–6. doi: 10.1016/j.ridd.2018.02.014
138. Windham GC, Lyall K, Anderson M, Kharrazi M. Autism spectrum disorder risk in relation to maternal mid-pregnancy serum hormone and protein markers from prenatal screening in California. J Autism Dev Disord. (2016) 46:478–88. doi: 10.1007/s10803-015-2587-2
139. Li L, Li M, Lu J, Ge X, Xie W, Wang Z, et al. Prenatal progestin exposure is associated with autism spectrum disorders. Front Psychiatry. (2018) 9:611. doi: 10.3389/fpsyt.2018.00611
140. Baron-Cohen S, Tsompanidis A, Auyeung B, Nørgaard-Pedersen B, Hougaard DM, Abdallah M, et al. Fetal estrogens and autism. Mol Psychiatry. (2020) 25:2970–8. doi: 10.1038/s41380-019-0454-9
141. Sealey LA, Hughes BW, Sriskanda AN, Guest JR, Gibson AD, Johnson-Williams L, et al. Environmental factors in the development of autism spectrum disorders. Environ Int. (2016) 88:288–98. doi: 10.1016/j.envint.2015.12.021
142. Ross JL, Kushner H, Kowal K, Bardsley M, Davis S, Reiss AL, et al. Androgen treatment effects on motor function, cognition, and behavior in boys with Klinefelter syndrome. J Pediatr. (2017) 185:193–199.e4. doi: 10.1016/j.jpeds.2017.02.036
143. Bird G, Viding E. The self to other model of empathy: providing a new framework for understanding empathy impairments in psychopathy, autism, and alexithymia. Neurosci Biobehav Rev. (2014) 47:520–32. doi: 10.1016/j.neubiorev.2014.09.021
144. Geng Y, Zhao W, Zhou F, Ma X, Yao S, Becker B, et al. Oxytocin facilitates empathic-and self-embarrassment ratings by attenuating amygdala and anterior insula responses. Front Endocrinol. (2018) 9:572. doi: 10.3389/fendo.2018.00572
145. Saleh TM, Connell BJ, Cribb AE. Sympathoexcitatory effects of estrogen in the insular cortex are mediated by GABA. Brain Res. (2005) 1037:114–22. doi: 10.1016/j.brainres.2005.01.010
146. Saleh TM, Connell BJ, Legge C, Cribb AE. Estrogen attenuates neuronal excitability in the insular cortex following middle cerebral artery occlusion. Brain Res. (2004) 1018:119–29. doi: 10.1016/j.brainres.2004.05.074
147. Terasawa Y, Shibata M, Moriguchi Y, Umeda S. Anterior insular cortex mediates bodily sensibility and social anxiety. Soc Cognit Affect Neurosci. (2013) 8:259–66. doi: 10.1093/scan/nss108
148. Northoff G. Neuro-philosophy and the Healthy Mind: Learning from the Unwell Brain. 1st ed. New York: W W Norton & Co Inc (2016). 242 p.
149. Robertson AE, Simmons DR. The relationship between sensory sensitivity and autistic traits in the general population. J Autism Dev Disord. (2013) 43:775–84. doi: 10.1007/s10803-012-1608-7
150. Lian F, Northoff G. The lost neural hierarchy of the autistic self—locked-out of the mental self and its default-mode network. Brain Sci. (2021) 11:574. doi: 10.3390/brainsci11050574
151. Akinola OB, Gabriel MO. Neuroanatomical and molecular correlates of cognitive and behavioral outcomes in hypogonadal males. Metab Brain Dis. (2018) 33:491–505. doi: 10.1007/s11011-017-0163-5
152. Skakkebæk A, Gravholt CH, Rasmussen PM, Bojesen A, Jensen JS, Fedder J, et al. Neuroanatomical correlates of Klinefelter syndrome studied in relation to the neuropsychological profile. NeuroImage Clin. (2014) 4:1–9. doi: 10.1016/j.nicl.2013.10.013
153. Wu LM, Tanenbaum ML, Dijkers MPJM, Amidi A, Hall SJ, Penedo FJ, et al. Cognitive and neurobehavioral symptoms in patients with non-metastatic prostate cancer treated with androgen deprivation therapy or observation: a mixed methods study. Soc Sci Med 1982. (2016) 156:80–9. doi: 10.1016/j.socscimed.2016.03.016
154. Steinberg L, Albert D, Cauffman E, Banich M, Graham S, Woolard J. Age differences in sensation seeking and impulsivity as indexed by behavior and self-report: evidence for a dual systems model. Dev Psychol. (2008) 44:1764–78. doi: 10.1037/a0012955
155. Webb JT, Amend ER, Beljan P. Misdiagnosis and Dual Diagnoses of Gifted Children and Adults: ADHD, Bipolar, OCD, Asperger’s, Depression, and Other Disorders. Tucson, AZ: Gifted Unlimited, LLC (2016). 420 p.
156. Cherrier M, Aubin S, Higano C. Cognitive and mood changes in men undergoing intermittent combined androgen blockade for non-metastatic prostate cancer. Psychooncology. (2009) 18:237–47. doi: 10.1002/pon.1401
157. Cross CP, Cyrenne D-LM, Brown GR. Sex differences in sensation-seeking: a meta-analysis. Sci Rep. (2013) 3:2486. doi: 10.1038/srep02486
158. Galvan A, Hare T, Voss H, Glover G, Casey B J. Risk-taking and the adolescent brain: who is at risk? Dev Sci. (2007) 10:F8–F14. doi: 10.1111/j.1467-7687.2006.00579.x
159. Jentsch JD, Taylor JR. Sex-related differences in spatial divided attention and motor impulsivity in rats. Behav Neurosci. (2003) 117:76–83. doi: 10.1037/0735-7044.117.1.76
160. Colzato LS, Hertsig G, van den Wildenberg WPM, Hommel B. Estrogen modulates inhibitory control in healthy human females: evidence from the stop-signal paradigm. Neuroscience. (2010) 167:709–15. doi: 10.1016/j.neuroscience.2010.02.029
161. Hernandez CM, Orsini C, Wheeler A-R, Ten Eyck TW, Betzhold SM, Labiste CC, et al. Testicular hormones mediate robust sex differences in impulsive choice in rats. eLife. (2020) 9:e58604. doi: 10.7554/eLife.58604
162. Sagvolden T, Johansen EB, Aase H, Russell VA. A dynamic developmental theory of attention-deficit/hyperactivity disorder (ADHD) predominantly hyperactive/impulsive and combined subtypes. Behav Brain Sci. (2005) 28:397–419. doi: 10.1017/S0140525X05000075
163. Wood RI, Armstrong A, Fridkin V, Shah V, Najafi A, Jakowec M, et al. ’Roid rage in rats? Testosterone effects on aggressive motivation, impulsivity and tyrosine hydroxylase. Physiol Behav. (2013) 111:6–12. doi: 10.1016/j.physbeh.2012.12.005
164. Smith CT, Sierra Y, Oppler SH, Boettiger CA. Ovarian cycle effects on immediate reward selection bias in humans: a role for estradiol. J Neurosci. (2014) 34:5468–76. doi: 10.1523/JNEUROSCI.0014-14.2014
165. Ross JL, Samango-Sprouse C, Lahlou N, Kowal K, Elder FF, Zinn A. Early androgen deficiency in infants and young boys with 47,XXY klinefelter syndrome. Horm Res Paediatr. (2005) 64:39–45. doi: 10.1159/000087313
166. Aksglaede L, Davis SM, Ross JL, Juul A. Minipuberty in Klinefelter syndrome: current status and future directions. Am J Med Genet C Semin Med Genet. (2020) 184:320–6. doi: 10.1002/ajmg.c.31794
167. Cangiano B, Indirli R, Profka E, Castellano E, Goggi G, Vezzoli V, et al. Central hypogonadism in Klinefelter syndrome: report of two cases and review of the literature. J Endocrinol Invest. (2021) 44:459–70. doi: 10.1007/s40618-020-01324-3
168. Berglund A, Stochholm K, Gravholt CH. Morbidity in 47,XYY syndrome: a nationwide epidemiological study of hospital diagnoses and medication use. Genet Med. (2020) 22:1542–51. doi: 10.1038/s41436-020-0837-y
169. Kim IW, Khadilkar AC, Ko EY, Sabanegh ES. 47,XYY Syndrome and male infertility. Rev Urol. (2013) 15:188–96.
170. Borjian Boroujeni P, Sabbaghian M, Dizaji A, Zarei Moradi S, Almadani N, Mohammadpour F, et al. Clinical aspects of infertile 47,XYY patients: a retrospective study. Hum Fertil. (2017) 22:1–6. doi: 10.1080/14647273.2017.1353143
171. Cuatrecasae-Membrado JM, Audi-Parera L, Zuasnabar A, Catald M, Pulg A, Bouch-Bnanyarak JM. 169 a case of male pseudohermaphroditism with XYY karyotype. Pediatr Res. (1985) 19:631. doi: 10.1203/00006450-198506000-00189
172. Westarp CV, Killinger DW, Volpé R. A case of testicular feminization with XYY karyotype. Clin Endocrinol (Oxf). (1974) 3:447–55. doi: 10.1111/j.1365-2265.1974.tb02816.x
173. Swaab D. Sexual differentiation of the human brain: relevance for gender identity, transsexualism and sexual orientation. Gynecol Endocrinol Off J Int Soc Gynecol Endocrinol. (2005) 19:301–12. doi: 10.1080/09513590400018231
174. Bardsley MZ, Kowal K, Levy C, Gosek A, Ayari N, Tartaglia N, et al. 47, XYY syndrome: clinical phenotype and timing of ascertainment. J Pediatr. (2013) 163:1085–94. doi: 10.1016/j.jpeds.2013.05.037
175. Aksglaede L, Skakkebaek NE, Juul A. Abnormal sex chromosome constitution and longitudinal growth: serum levels of insulin-like growth factor (IGF)-I, IGF binding protein-3, luteinizing hormone, and testosterone in 109 males with 47, XXY, 47, XYY, or sex-determining region of the Y chromosome (SRY)-positive 46, XX karyotypes. J Clin Endocrinol Metab. (2008) 93:169–76. doi: 10.1210/jc.2007-1426
176. Salehi P, Herzig L, Capone G, Lu A, Oron AP, Kim S-J. Comparison of Aberrant Behavior Checklist profiles across Prader-Willi syndrome, Down syndrome, and autism spectrum disorder. Am J Med Genet A. (2018) 176:2751–9. doi: 10.1002/ajmg.a.40665
177. Goldstone AP. Prader-Willi syndrome: advances in genetics, pathophysiology and treatment. Trends Endocrinol Metab. (2004) 15:12–20. doi: 10.1016/j.tem.2003.11.003
178. Nurmi EL, Dowd M, Tadevosyan-leyfer O, Haines JL, Folstein SE, Sutcliffe JS. Exploratory subsetting of autism families based on savant skills improves evidence of genetic linkage to 15q11-q13. J Am Acad Child Adolesc Psychiatry. (2003) 42:856–63. doi: 10.1097/01.CHI.0000046868.56865.0F
179. Ma D, Jaworski J, Menold M, Donnelly S, Abramson R, Wright H, et al. Ordered-subset analysis of savant skills in autism for 15q11-q13. Am J Med Genet B Neuropsychiatr Genet. (2005) 135B:38–41. doi: 10.1002/ajmg.b.30166
180. Dykens E. Toward a positive psychology of mental retardation. Am J Orthopsychiatry. (2006) 76:185–93. doi: 10.1037/0002-9432.76.2.185
181. Mercer ES, Broecker B, Smith EA, Kirsch AJ, Scherz HC, A. Massad C. Urological manifestations of Down syndrome. J Urol. (2004) 171:1250–3. doi: 10.1097/01.ju.0000112915.69436.91
182. Hawli Y, Nasrallah M, Fuleihan GE-H. Endocrine and musculoskeletal abnormalities in patients with Down syndrome. Nat Rev Endocrinol. (2009) 5:327–34. doi: 10.1038/nrendo.2009.80
183. Down JL. On Some of the Mental Affections of Childhood and Youth. London: J. & A. Churchill (1887). 324 p.
184. Ohlsson Gotby V, Söder O, Frisén L, Serlachius E, Bölte S, Almqvist C, et al. Hypogonadotrophic hypogonadism, delayed puberty and risk for neurodevelopmental disorders. J Neuroendocrinol. (2019) 31:e12803. doi: 10.1111/jne.12803
185. Bitran D, Kellogg CK, Hilvers RJ. Treatment with an anabolic-androgenic steroid affects anxiety-related behavior and alters the sensitivity of cortical GABAA receptors in the rat. Horm Behav. (1993) 27:568–83. doi: 10.1006/hbeh.1993.1041
186. Friston K, Schwartenbeck P, FitzGerald T, Moutoussis M, Behrens T, Dolan RJ. The anatomy of choice: dopamine and decision-making. Philos Trans R Soc B Biol Sci. (2014) 369:20130481. doi: 10.1098/rstb.2013.0481
187. Moran RJ, Campo P, Symmonds M, Stephan KE, Dolan RJ, Friston KJ. Free energy, precision and learning: the role of cholinergic neuromodulation. J Neurosci. (2013) 33:8227–36. doi: 10.1523/JNEUROSCI.4255-12.2013
188. Varshney MK, Inzunza J, Lupu D, Ganapathy V, Antonson P, Rüegg J, et al. Role of estrogen receptor beta in neural differentiation of mouse embryonic stem cells. Proc Natl Acad Sci. (2017) 114:E10428–37. doi: 10.1073/pnas.1714094114
189. Fan X, Warner M, Gustafsson J-Å. Estrogen receptor β expression in the embryonic brain regulates development of calretinin-immunoreactive GABAergic interneurons. Proc Natl Acad Sci. (2006) 103:19338–43. doi: 10.1073/pnas.0609663103
190. Perrot-Sinal TS, Davis AM, Gregerson KA, Kao JPY, McCarthy MM. Estradiol enhances excitatory gammabutyric acid-mediated calcium signaling in neonatal hypothalamic neurons. Endocrinology. (2001) 142:2238–43. doi: 10.1210/endo.142.6.8180
191. Perrot-Sinal TS, Sinal CJ, Reader JC, Speert DB, McCarthy MM. Sex differences in the chloride cotransporters, NKCC1 and KCC2, in the developing hypothalamus. J Neuroendocrinol. (2007) 19:302–8. doi: 10.1111/j.1365-2826.2007.01530.x
192. Wang J, Yang B, Ju L, Yang J, Allen A, Zhang J, et al. The estradiol synthesis inhibitor formestane diminishes the ability of sevoflurane to induce neurodevelopmental abnormalities in male rats. Front Syst Neurosci. (2020) 14:546531. doi: 10.3389/fnsys.2020.546531
193. Naderi M, Puar P, JavadiEsfahani R, Kwong RWM. Early developmental exposure to bisphenol A and bisphenol S disrupts socio-cognitive function, isotocin equilibrium, and excitation-inhibition balance in developing zebrafish. NeuroToxicology. (2022) 88:144–54. doi: 10.1016/j.neuro.2021.11.009
194. Pozzi D, Rasile M, Corradini I, Matteoli M. Environmental regulation of the chloride transporter KCC2: switching inflammation off to switch the GABA on? Transl Psychiatry. (2020) 10:1–11. doi: 10.1038/s41398-020-01027-6
195. Peerboom C, Wierenga CJ. The postnatal GABA shift: a developmental perspective. Neurosci Biobehav Rev. (2021) 124:179–92. doi: 10.1016/j.neubiorev.2021.01.024
196. Dakin S, Frith U. Vagaries of visual perception in autism. Neuron. (2005) 48:497–507. doi: 10.1016/j.neuron.2005.10.018
197. Happé F, Frith U. The weak coherence account: detail-focused cognitive style in autism spectrum disorders. J Autism Dev Disord. (2006) 36:5–25. doi: 10.1007/s10803-005-0039-0
198. Mottron L, Burack JA. Enhanced perceptual functioning in the development of autism. In: Burack JA, Charman N, Yirmiya N, Zelazo PR, editors. The Development of Autism: Perspectives from Theory and Research. Lawrence Erlbaum Associates Publishers, Mahwah, NJ, US (2001). p. 131–48.
199. Mottron L, Dawson M, Soulières I, Hubert B, Burack J. Enhanced perceptual functioning in autism: an update, and eight principles of autistic perception. J Autism Dev Disord. (2006) 36:27–43. doi: 10.1007/s10803-005-0040-7
200. Sha Z, van Rooij D, Anagnostou E, Arango C, Auzias G, Behrmann M, et al. Subtly altered topological asymmetry of brain structural covariance networks in autism spectrum disorder across 43 datasets from the ENIGMA consortium. Mol Psychiatry. (2022) 27:2114–25. doi: 10.1101/2021.05.05.442735
201. Robertson CE, Baron-Cohen S. Sensory perception in autism. Nat Rev Neurosci. (2017) 18:671–84. doi: 10.1038/nrn.2017.112
202. Casanova MF, van Kooten IAJ, Switala AE, van Engeland H, Heinsen H, Steinbusch HWM, et al. Minicolumnar abnormalities in autism. Acta Neuropathol (Berl). (2006) 112:287–303. doi: 10.1007/s00401-006-0085-5
203. Liu X, Ying J, Wang X, Zheng Q, Zhao T, Yoon S, et al. Astrocytes in neural circuits: key factors in synaptic regulation and potential targets for neurodevelopmental disorders. Front Mol Neurosci. (2021) 14:729273. doi: 10.3389/fnmol.2021.729273
204. Elçi̇ ME, Usta MB, Karabeki̇Roğlu K. Role of glia cells in autism spectrum disorders. Psikiyatr Güncel Yaklaşımlar. (2023) 15:577–88. doi: 10.18863/pgy.1189139
205. Marotta R, Risoleo MC, Messina G, Parisi L, Carotenuto M, Vetri L, et al. The neurochemistry of autism. Brain Sci. (2020) 10:163. doi: 10.3390/brainsci10030163
206. Mariani J, Coppola G, Zhang P, Abyzov A, Provini L, Tomasini L, et al. FOXG1-dependent dysregulation of GABA/glutamate neuron differentiation in autism spectrum disorders. Cell. (2015) 162:375–90. doi: 10.1016/j.cell.2015.06.034
207. Brewer R, Cook R, Bird G. Alexithymia: a general deficit of interoception. R Soc Open Sci. (2016) 3:150664. doi: 10.1098/rsos.150664
208. van Rijn S. Salivary testosterone in relation to social cognition and social anxiety in children and adolescents with 47,XXY (Klinefelter syndrome). PloS One. (2018) 13:e0200882. doi: 10.1371/journal.pone.0200882
209. Satterstrom FK, Kosmicki JA, Wang J, Breen MS, De Rubeis S, An J-Y, et al. Large-scale exome sequencing study implicates both developmental and functional changes in the neurobiology of autism. Cell. (2020) 180:568–584.e23. doi: 10.1016/j.cell.2019.12.036
210. Hickey S, Berto S, Konopka G. Chromatin decondensation by FOXP2 promotes human neuron maturation and expression of neurodevelopmental disease genes. Cell Rep. (2019) 27:1699–1711.e9. doi: 10.1016/j.celrep.2019.04.044
211. Bowers JM, Perez-Pouchoulen M, Roby CR, Ryan TE, McCarthy MM. Androgen modulation of Foxp1 and Foxp2 in the developing rat brain: impact on sex specific vocalization. Endocrinology. (2014) 155:4881–94. doi: 10.1210/en.2014-1486
212. Wang M, Luo J, Dai L, Feng M, Cao X, Zhang J, et al. Foxp2 deficiency impairs reproduction by modulating the hypothalamic–pituitary–gonadal axis in zebrafish. Biol Reprod. (2024), ioae019. doi: 10.1093/biolre/ioae019
213. Zhu X, Chen C, Wei D, Xu Y, Liang S, Jia W, et al. FOXP2 confers oncogenic effects in prostate cancer. eLife. (2023) 12:e81258. doi: 10.7554/eLife.81258
214. Yoo T, Cho H, Park H, Lee J, Kim E. Shank3 exons 14–16 deletion in glutamatergic neurons leads to social and repetitive behavioral deficits associated with increased cortical layer 2/3 neuronal excitability. Front Cell Neurosci. (2019) 13:458. doi: 10.3389/fncel.2019.00458
215. Andoh M, Ikegaya Y, Koyama R. Microglia in animal models of autism spectrum disorders. Prog Mol Biol Transl Sci. (2020) 173:239–73. doi: 10.1016/bs.pmbts.2020.04.012
216. Huang G, Chen S, Chen X, Zheng J, Xu Z, Doostparast Torshizi A, et al. Uncovering the functional link between SHANK3 deletions and deficiency in neurodevelopment using iPSC-derived human neurons. Front Neuroanat. (2019) 13:23. doi: 10.3389/fnana.2019.00023
217. Zhou Y, Sharma J, Ke Q, Landman R, Yuan J, Chen H, et al. Atypical behavior and connectivity in SHANK3-mutant macaques. Nature. (2019) 570:326–31. doi: 10.1038/s41586-019-1278-0
218. Berkel S, Eltokhi A, Fröhlich H, Porras-Gonzalez D, Rafiullah R, Sprengel R, et al. Sex hormones regulate SHANK expression. Front Mol Neurosci. (2018) 11:337. doi: 10.3389/fnmol.2018.00337
219. Srancikova A, Mihalj D, Bacova Z, Bakos J. The effects of testosterone on gene expression of cell-adhesion molecules and scaffolding proteins: the role of sex in early development. Andrologia. (2021) 53:e14153. doi: 10.1111/and.14153
220. Acevedo-Rodriguez A, Mani SK, Handa RJ. Oxytocin and estrogen receptor β in the brain: an overview. Front Endocrinol. (2015) 6:160. doi: 10.3389/fendo.2015.00160
221. Hiroi R, Lacagnina AF, Hinds LR, Carbone DG, Uht RM, Handa RJ. The androgen metabolite, 5α-androstane-3β, 17β-diol (3β-diol), activates the oxytocin promoter through an estrogen receptor-β pathway. Endocrinology. (2013) 154:1802–12. doi: 10.1210/en.2012-2253
222. Hwang WJ, Lee TY, Kim NS, Kwon JS. The role of estrogen receptors and their signaling across psychiatric disorders. Int J Mol Sci. (2021) 22:373. doi: 10.3390/ijms22010373
223. Hu VW, Bi C. Phenotypic subtyping and re-analyses of existing transcriptomic data from autistic probands in simplex families reveal differentially expressed and ASD trait-associated genes. Front Neurol. (2020) 11:578972. doi: 10.3389/fneur.2020.578972
224. Hu VW, Nguyen A, Kim KS, Steinberg ME, Sarachana T, Scully MA, et al. Gene expression profiling of lymphoblasts from autistic and nonaffected sib pairs: altered pathways in neuronal development and steroid biosynthesis. PloS One. (2009) 4:e5775. doi: 10.1371/journal.pone.0005775
225. Grassi S, Frondaroli A, Scarduzio M, Dutia MB, Dieni C, Pettorossi VE. Effects of 17β-estradiol on glutamate synaptic transmission and neuronal excitability in the rat medial vestibular nuclei. Neuroscience. (2010) 165:1100–14. doi: 10.1016/j.neuroscience.2009.11.039
226. Oberlander JG, Woolley CS. 17β-Estradiol acutely potentiates glutamatergic synaptic transmission in the hippocampus through distinct mechanisms in males and females. J Neurosci. (2017) 37:12314–27. doi: 10.1523/JNEUROSCI.3011-17.2017
227. Garcia-Ovejero D, Azcoitia I, DonCarlos LL, Melcangi RC, Garcia-Segura LM. Glia-neuron crosstalk in the neuroprotective mechanisms of sex steroid hormones. Brain Res Rev. (2005) 48:273–86. doi: 10.1016/j.brainresrev.2004.12.018
228. Micevych P, Bondar G, Kuo J. Estrogen actions on neuroendocrine glia. Neuroendocrinology. (2010) 91:211–22. doi: 10.1159/000289568
229. Murphy DD, Segal M. Morphological plasticity of dendritic spines in central neurons is mediated by activation of cAMP response element binding protein. Proc Natl Acad Sci. (1997) 94:1482–7. doi: 10.1073/pnas.94.4.1482
230. Noel SD, Keen KL, Baumann DI, Filardo EJ, Terasawa E. Involvement of G protein-coupled receptor 30 (GPR30) in rapid action of estrogen in primate LHRH neurons. Mol Endocrinol. (2009) 23:349–59. doi: 10.1210/me.2008-0299
231. Chimento A, Sirianni R, Casaburi I, Pezzi V. Role of estrogen receptors and G protein-coupled estrogen receptor in regulation of hypothalamus–pituitary–testis axis and spermatogenesis. Front Endocrinol. (2014) 5:1. doi: 10.3389/fendo.2014.00001
232. Bernardino R, Alves M, Silva J, Barros A, Ferraz L, Sousa M, et al. Expression of estrogen receptors alpha (ER-α), beta (ER-β), and G protein-coupled receptor 30 (GPR30) in testicular tissue of men with Klinefelter syndrome. Horm Metab Res. (2016) 48:413–5. doi: 10.1055/s-0042-105151
233. Altun H, Kurutaş EB, Şahin N, Sınır H, Fındıklı E. Decreased levels of G protein-coupled estrogen receptor in children with autism spectrum disorders. Psychiatry Res. (2017) 257:67–71. doi: 10.1016/j.psychres.2017.06.008
234. Pasterski VL, Geffner ME, Brain C, Hindmarsh P, Brook C, Hines M. Prenatal hormones and postnatal socialization by parents as determinants of male-typical toy play in girls with congenital adrenal hyperplasia. Child Dev. (2005) 76:264–78. doi: 10.1111/j.1467-8624.2005.00843.x
235. Hines M. Prenatal testosterone and gender-related behavior. Eur J Endocrinol. (2006) 155:S115–21. doi: 10.1530/eje.1.02236
236. Connolly F, Rae MT, Bittner L, Hogg K, McNeilly AS, Duncan WC. Excess androgens in utero alters fetal testis development. Endocrinology. (2013) 154:1921–33. doi: 10.1210/en.2012-2153
237. Maliqueo M, Lara HE, Sánchez F, Echiburú B, Crisosto N, Sir-Petermann T. Placental steroidogenesis in pregnant women with polycystic ovary syndrome. Eur J Obstet Gynecol Reprod Biol. (2013) 166:151–5. doi: 10.1016/j.ejogrb.2012.10.015
238. Hu M, Richard JE, Maliqueo M, Kokosar M, Fornes R, Benrick A, et al. Maternal testosterone exposure increases anxiety-like behavior and impacts the limbic system in the offspring. Proc Natl Acad Sci. (2015) 112:14348–53. doi: 10.1073/pnas.1507514112
239. Durdiaková J, Celec P, Laznibatová J, Minárik G, Lakatošová S, Kubranská A, et al. Differences in salivary testosterone, digit ratio and empathy between intellectually gifted and control boys. Intelligence. (2015) 48:76–84. doi: 10.1016/j.intell.2014.11.002
240. Richards G, Gomes M, Ventura T. Testosterone measured from amniotic fluid and maternal plasma shows no significant association with directional asymmetry in newborn digit ratio (2D:4D). J Dev Orig Health Dis. (2018) 10:1–6. doi: 10.1017/S2040174418000752
241. Manning JT, Baron-Cohen S, Wheelwright S, Sanders G. The 2nd to 4th digit ratio and autism. Dev Med Child Neurol. (2001) 43:160–4. doi: 10.1111/j.1469-8749.2001.tb00181.x
242. Schieve LA, Tian L, Dowling N, Croen L, Hoover-Fong J, Alexander A, et al. Associations between the 2nd to 4th digit ratio and autism spectrum disorder in population-based samples of boys and girls: findings from the Study to Explore Early Development. J Autism Dev Disord. (2018) 48:2379–95. doi: 10.1007/s10803-018-3495-z
243. Jones MEE, Chin Boon W, Proietto J, Simpson ER. Of mice and men: the evolving phenotype of aromatase deficiency. Trends Endocrinol Metab. (2006) 17:55–64. doi: 10.1016/j.tem.2006.01.004
244. Zou Y, Lu Q, Zheng D, Chu Z, Liu Z, Chen H, et al. Prenatal levonorgestrel exposure induces autism-like behavior in offspring through ERβ suppression in the amygdala. Mol Autism. (2017) 8:46. doi: 10.1186/s13229-017-0159-3
245. Dangmann R. A hypothesis to explain the potential influence of hormones on the severity of autism spectrum conditions in women. Med Hypotheses. (2023) 178:111136. doi: 10.1016/j.mehy.2023.111136
246. Hu VW. The expanding genomic landscape of autism: discovering the ‘forest’ beyond the ‘trees.’. Future Neurol. (2013) 8:29–42. doi: 10.2217/fnl.12.83
247. Hu VW, Sarachana T, Sherrard RM, Kocher KM. Investigation of sex differences in the expression of RORA and its transcriptional targets in the brain as a potential contributor to the sex bias in autism. Mol Autism. (2015) 6:7. doi: 10.1186/2040-2392-6-7
248. Sarachana T, Xu M, Wu R-C, Hu VW. Sex hormones in autism: androgens and estrogens differentially and reciprocally regulate RORA, a novel candidate gene for autism. PloS One. (2011) 6:e17116. doi: 10.1371/journal.pone.0017116
249. Theisen JG, Sundaram V, Filchak MS, Chorich LP, Sullivan ME, Knight J, et al. The use of whole exome sequencing in a cohort of transgender individuals to identify rare genetic variants. Sci Rep. (2019) 9:20099. doi: 10.1038/s41598-019-53500-y
250. Boda H, Nghi TN, Nishijo M, Thao PN, Tai PT, Van Luong H, et al. Prenatal dioxin exposure estimated from dioxins in breast milk and sex hormone levels in umbilical cord blood in Vietnamese newborn infants. Sci Total Environ. (2018) 615:1312–8. doi: 10.1016/j.scitotenv.2017.09.214
251. Wetherill Y, Akingbemi B, Kanno J, McLachlan J, Nadal A, Sonnenschein C, et al. In vitro molecular mechanisms of Bisphenol A action. Reprod Toxicol Elmsford N. (2007) 24:178–98. doi: 10.1016/j.reprotox.2007.05.010
252. Thongkorn S, Kanlayaprasit S, Panjabud P, Saeliw T, Jantheang T, Kasitipradit K, et al. Sex differences in the effects of prenatal bisphenol A exposure on autism-related genes and their relationships with the hippocampus functions. Sci Rep. (2021) 11:1241. doi: 10.1038/s41598-020-80390-2
253. Sadowski RN, Wise L, Park P, Schantz S, Juraska J. Early exposure to bisphenol A alters neuron and glia number in the rat prefrontal cortex of adult males, but not females. Neuroscience. (2014) 279:122–31. doi: 10.1016/j.neuroscience.2014.08.038
254. Hansen JB, Bilenberg N, Timmermann CAG, Jensen RC, Frederiksen H, Andersson A-M, et al. Prenatal exposure to bisphenol A and autistic- and ADHD-related symptoms in children aged 2 and5 years from the Odense Child Cohort. Environ Health. (2021) 20:24. doi: 10.1186/s12940-021-00709-y
255. Janšáková K, Hill M, Čelárová D, Celušáková H, Repiská G, Bičíková M, et al. Alteration of the steroidogenesis in boys with autism spectrum disorders. Transl Psychiatry. (2020) 10:340. doi: 10.1038/s41398-020-01017-8
256. Diviccaro S, Cioffi L, Falvo E, Giatti S, Melcangi RC. Allopregnanolone: an overview on its synthesis and effects. J Neuroendocrinol. (2022) 34:e12996. doi: 10.1111/jne.12996
257. Darbra S, Pallarès M. Developmental actions of neurosteroids in rodents: focus on allopregnanolone. Curr Opin Endocr Metab Res. (2022) 23:100317. doi: 10.1016/j.coemr.2022.100317
258. Chiang H-L, Liu C-J, Hu Y-W, Chen S-C, Hu L-Y, Shen C-C, et al. Risk of cancer in children, adolescents, and young adults with autistic disorder. J Pediatr. (2015) 166:418–423.e1. doi: 10.1016/j.jpeds.2014.10.029
259. Treffert DA. The savant syndrome: an extraordinary condition. A synopsis: past, present, future. Philos Trans R Soc B Biol Sci. (2009) 364:1351–7. doi: 10.1098/rstb.2008.0326
260. Howlin P, Goode S, Hutton J, Rutter M. Savant skills in autism: psychometric approaches and parental reports. Philos Trans R Soc B Biol Sci. (2009) 364:1359–67. doi: 10.1098/rstb.2008.0328
261. Bennett E, Heaton P. IIs talent in autism spectrum disorders associated with a specific cognitive and behavioral phenotype? J Autism Dev Disord. (2012) 42:2739–53. doi: 10.1007/s10803-012-1533-9
262. Treffert D. Extraordinary People: Understanding Savant Syndrome. Lincoln, NE: iUniverse (2006). 438 p.
263. Mottron L, Dawson M, Soulières I. Enhanced perception in savant syndrome: patterns, structure and creativity. Philos Trans R Soc B Biol Sci. (2009) 364:1385–91. doi: 10.1098/rstb.2008.0333
264. Gagné F. Transforming gifts into talents: the DMGT as a developmental theory1. High Abil Stud. (2004) 15:119–47. doi: 10.1080/1359813042000314682
265. Pellicano E, Burr D. When the world becomes ‘too real’: a Bayesian explanation of autistic perception. Trends Cognit Sci. (2012) 16:504–10. doi: 10.1016/j.tics.2012.08.009
266. Cesta CE, Öberg AS, Ibrahimson A, Yusuf I, Larsson H, Almqvist C, et al. Maternal polycystic ovary syndrome and risk of neuropsychiatric disorders in offspring: prenatal androgen exposure or genetic confounding? Psychol Med. (2020) 50:616–24. doi: 10.1017/S0033291719000424
267. Zhang F-F, Zhang Q, Wang Y-L, Wang F-F, Hardiman PJ, Qu F. Intergenerational influences between maternal polycystic ovary syndrome and offspring: an updated overview. J Pediatr. (2021) 232:272–81. doi: 10.1016/j.jpeds.2021.01.018
268. Hanem LGE, Salvesen Ø, Madsen A, Sagen JV, Mellgren G, Juliusson PB, et al. Maternal PCOS status and metformin in pregnancy: steroid hormones in 5–10 years old children from the PregMet randomized controlled study. PloS One. (2021) 16:e0257186. doi: 10.1371/journal.pone.0257186
269. Ypma RJF, Moseley RL, Holt RJ, Rughooputh N, Floris DL, Chura LR, et al. Default mode hypoconnectivity underlies a sex-related autism spectrum. Biol Psychiatry Cognit Neurosci Neuroimaging. (2016) 1:364–71. doi: 10.1016/j.bpsc.2016.04.006
270. Alaerts K, Swinnen SP, Wenderoth N. Sex differences in autism: a resting-state fMRI investigation of functional brain connectivity in males and females. Soc Cognit Affect Neurosci. (2016) 11:1002–16. doi: 10.1093/scan/nsw027
271. Hébert TP, Pagnani AR, Hammond DR. An examination of paternal influence on high-achieving gifted males. J Educ Gift. (2009) 33:241–74. doi: 10.1177/016235320903300205
272. Wilcove JL. Perceptions of masculinity, femininity, and androgyny among a select cohort of gifted adolescent males. J Educ Gift. (1998) 21:288–309. doi: 10.1177/016235329802100303
273. Miller NB, Falk RF, Huang Y. Gender identity and the overexcitability profiles of gifted college students. Roeper Rev. (2009) 31:161–9. doi: 10.1080/02783190902993920
274. Wexelbaum R, Hoover J. Gifted and LGBTIQ: a comprehensive research review. Int J Talent Dev Creat. (2014) 2:73–86.
275. Just MA. Cortical activation and synchronization during sentence comprehension in high-functioning autism: evidence of underconnectivity. Brain. (2004) 127:1811–21. doi: 10.1093/brain/awh199
276. Tavassoli T, Hoekstra RA, Baron-Cohen S. The Sensory Perception Quotient (SPQ): development and validation of a new sensory questionnaire for adults with and without autism. Mol Autism. (2014) 5:29. doi: 10.1186/2040-2392-5-29
277. Rinn AN, Mullet DR, Jett N, Nyikos T. Sensory processing sensitivity among high-ability individuals: a psychometric evaluation of the highly sensitive person scale. Roeper Rev. (2018) 40:166–75. doi: 10.1080/02783193.2018.1466840
278. Courchesne E, Campbell K, Solso S. Brain growth across the life span in autism: age-specific changes in anatomical pathology. Brain Res. (2011) 1380:138–45. doi: 10.1016/j.brainres.2010.09.101
279. Gliga T, Bedford R, Charman T, Johnson MH, Baron-Cohen S, Bolton P, et al. Enhanced visual search in infancy predicts emerging autism symptoms. Curr Biol. (2015) 25:1727–30. doi: 10.1016/j.cub.2015.05.011
280. Minshew NJ, Williams DL. The new neurobiology of autism: cortex, connectivity, and neuronal organization. Arch Neurol. (2007) 64:945–50. doi: 10.1001/archneur.64.7.945
281. Casanova MF, Buxhoeveden DP, Switala AE, Roy E. Minicolumnar pathology in autism. Neurology. (2002) 58:428–32. doi: 10.1212/WNL.58.3.428
282. Buxhoeveden DP, Semendeferi K, Buckwalter J, Schenker N, Switzer R, Courchesne E. Reduced minicolumns in the frontal cortex of patients with autism. Neuropathol Appl Neurobiol. (2006) 32:483–91. doi: 10.1111/j.1365-2990.2006.00745.x
283. Kathuria A, Nowosiad P, Jagasia R, Aigner S, Taylor RD, Andreae LC, et al. Stem cell-derived neurons from autistic individuals with SHANK3 mutation show morphogenetic abnormalities during early development. Mol Psychiatry. (2018) 23:735–46. doi: 10.1038/mp.2017.185
284. McKavanagh R, Buckley E, Chance SA. Wider minicolumns in autism: a neural basis for altered processing? Brain. (2015) 138:2034–45. doi: 10.1093/brain/awv110
285. Schipul SE, Keller TA, Just MA. Inter-regional brain communication and its disturbance in autism. Front Syst Neurosci. (2011) 5:10. doi: 10.3389/fnsys.2011.00010
286. Markou P, Ahtam B, Papadatou-Pastou M. Elevated levels of atypical handedness in autism: meta-analyses. Neuropsychol Rev. (2017) 27:258–83. doi: 10.1007/s11065-017-9354-4
287. Treffert DA, Tammet D, Leed P, Martinez R, Rancer SM. Islands of Genius: The Bountiful Mind of the Autistic, Acquired, and Sudden Savant. London: Jessica Kingsley Publishers (2010).
288. Grandin T. The Way I See It : A Personal Look at Autism & Asperger’s. Arlington, TX: Future Horizons Inc (2008). 260 p.
289. Mottron L, Bouvet L, Bonnel A, Samson F, Burack JA, Dawson M, et al. Veridical mapping in the development of exceptional autistic abilities. Neurosci Biobehav Rev. (2013) 37:209–28. doi: 10.1016/j.neubiorev.2012.11.016
290. Hughes JEA, Simner J, Baron-Cohen S, Treffert DA, Ward J. Is synesthesia more prevalent in autism spectrum conditions? Only where there is prodigious talent. Multisensory Res. (2017) 30:391–408. doi: 10.1163/22134808-00002558
291. Rouw R, Scholte H. Increased structural connectivity in Grapheme-Color Synesthesia. Nat Neurosci. (2007) 10:792–7. doi: 10.1038/nn1906
292. Dance CJ, Jaquiery M, Eagleman DM, Porteous D, Zeman A, Simner J. What is the relationship between aphantasia, synaesthesia and autism? Conscious Cognit. (2021) 89:103087. doi: 10.1016/j.concog.2021.103087
293. Bouvet L, Donnadieu S, Valdois S, Caron C, Dawson M, Mottron L. Veridical mapping in savant abilities, absolute pitch, and synesthesia: an autism case study. Front Psychol. (2014) 5. doi: 10.3389/fpsyg.2014.00106
294. Zhang L, Qiao L, Chen Q, Yang W, Xu M, Yao X, et al. Gray matter volume of the lingual gyrus mediates the relationship between inhibition function and divergent thinking. Front Psychol. (2016) 7. doi: 10.3389/fpsyg.2016.01532
295. Zamoscik V, Mier D, Schmidt SNL, Kirsch P. Early memories of individuals on the autism spectrum assessed using online self-reports. Front Psychiatry. (2016) 7:79. doi: 10.3389/fpsyt.2016.00079
296. Addo RN, Wiens S, Nord M, Larsson M. Olfactory functions in adults with autism spectrum disorders. Perception. (2017) 46:530–7. doi: 10.1177/0301006616686100
297. Tavassoli T, Baron-Cohen S. Olfactory detection thresholds and adaptation in adults with autism spectrum condition. J Autism Dev Disord. (2012) 42:905–9. doi: 10.1007/s10803-011-1321-y
298. Barros F, Soares SC. Giving meaning to the social world in autism spectrum disorders: olfaction as a missing piece of the puzzle? Neurosci Biobehav Rev. (2020) 116:239–50. doi: 10.1016/j.neubiorev.2020.06.008
299. Endevelt-Shapira Y, Perl O, Ravia A, Amir D, Eisen A, Bezalel V, et al. Altered responses to social chemosignals in autism spectrum disorder. Nat Neurosci. (2018) 21:111–9. doi: 10.1038/s41593-017-0024-x
300. Brogaard B. Serotonergic hyperactivity as a potential factor in developmental, acquired and drug-induced synesthesia. Front Hum Neurosci. (2013) 7:657. doi: 10.3389/fnhum.2013.00657
301. Sagiv N, Ilbeigi A, Ben-Tal O. Reflections on synesthesia, perception, and cognition. Intellectica Rev Assoc Pour Rech Cognit. (2011) 55:81–94. doi: 10.3406/intel.2011.1162
302. Kalita S, Mehdiratta N, Birwatkar D, Hirsch AR. Investigating a clinical case of deluded gustatory perception. New Adv Brain Crit Care. (2023) 4:62–5. doi: 10.33140/NABCC
303. Bryant DM, Hoeft F, Lai S, Lackey J, Roeltgen D, Ross J, et al. Neuroanatomical phenotype of klinefelter syndrome in childhood: A voxel-based morphometry study. J Neurosci. (2011) 31:6654–60. doi: 10.1523/JNEUROSCI.5899-10.2011
304. Itti E, Gaw Gonzalo IT, Pawlikowska-Haddal A, Boone KB, Mlikotic A, Itti L, et al. The structural brain correlates of cognitive deficits in adults with Klinefelter’s syndrome. J Clin Endocrinol Metab. (2006) 91:1423–7. doi: 10.1210/jc.2005-1596
305. Printzlau F, Wolstencroft J, Skuse DH. Cognitive, behavioral, and neural consequences of sex chromosome aneuploidy. J Neurosci Res. (2017) 95:311–9. doi: 10.1002/jnr.23951
306. van Rijn S, de Sonneville L, Swaab H. The nature of social cognitive deficits in children and adults with Klinefelter syndrome (47,XXY). Genes Brain Behav. (2018) 17:e12465. doi: 10.1111/gbb.12465
307. Arnold AP. Mouse models for evaluating sex chromosome effects that cause sex differences in non-gonadal tissues. J Neuroendocrinol. (2009) 21:377–86. doi: 10.1111/j.1365-2826.2009.01831.x
308. Lewejohann L, Damm OS, Luetjens CM, Hämäläinen T, Simoni M, Nieschlag E, et al. Impaired recognition memory in male mice with a supernumerary X chromosome. Physiol Behav. (2009) 96:23–9. doi: 10.1016/j.physbeh.2008.08.007
309. Mueller SC, Merke DP, Leschek EW, Fromm S, Grillon C, Cornwell BR, et al. Grey matter volume correlates with virtual water maze task performance in boys with androgen excess. Neuroscience. (2011) 197:225–32. doi: 10.1016/j.neuroscience.2011.09.022
310. Falter CM, Arroyo M, Davis GJ. Testosterone: activation or organization of spatial cognition? Biol Psychol. (2006) 73:132–40. doi: 10.1016/j.biopsycho.2006.01.011
311. Puts DA, Cárdenas RA, Bailey DH, Burriss RP, Jordan CL, Breedlove SM. Salivary testosterone does not predict mental rotation performance in men or women. Horm Behav. (2010) 58:282–9. doi: 10.1016/j.yhbeh.2010.03.005
312. Quaiser-Pohl C, Jansen P, Lehmann J, Kudielka BM. Is there a relationship between the performance in a chronometric mental-rotations test and salivary testosterone and estradiol levels in children aged 9–14 years? Dev Psychobiol. (2016) 58:120–8. doi: 10.1002/dev.21333
313. Haren MT, Wittert GA, Chapman IM, Coates P, Morley JE. Effect of oral testosterone undecanoate on visuospatial cognition, mood and quality of life in elderly men with low-normal gonadal status. Maturitas. (2005) 50:124–33. doi: 10.1016/j.maturitas.2004.05.002
314. Hampson E. Regulation of cognitive function by androgens and estrogens. Curr Opin Behav Sci. (2018) 23:49–57. doi: 10.1016/j.cobeha.2018.03.002
315. Geschwind DH, Dykens E. Neurobehavioral and psychosocial issues in Klinefelter syndrome. Learn Disabil Res Pract. (2004) 19:166–73. doi: 10.1111/j.1540-5826.2004.00100.x
316. Lambert R, Harriss E. Insider accounts of dyslexia from research mathematicians. Educ Stud Math. (2022) 111:89–107. doi: 10.1007/s10649-021-10140-2
317. Paulesu E, Danelli L, Berlingeri M. Reading the dyslexic brain: multiple dysfunctional routes revealed by a new meta-analysis of PET and fMRI activation studies. Front Hum Neurosci. (2014) 8. doi: 10.3389/fnhum.2014.00830
318. Siok WT, Niu Z, Jin Z, Perfetti CA, Tan LH. A structural-functional basis for dyslexia in the cortex of Chinese readers. Proc Natl Acad Sci. (2008) 105:5561–6. doi: 10.1073/pnas.0801750105
319. Itti E, Gaw Gonzalo IT, Boone KB, Geschwind DH, Berman N, Pawlikowska-Haddal A, et al. Functional neuroimaging provides evidence of anomalous cerebral laterality in adults with Klinefelter’s syndrome. Ann Neurol. (2003) 54:669–73. doi: 10.1002/ana.10735
320. van Rijn S, Aleman A, Swaab H, Vink M, Sommer I, Kahn RS. Effects of an extra X chromosome on language lateralization: an fMRI study with Klinefelter men (47,XXY). Schizophr Res. (2008) 101:17–25. doi: 10.1016/j.schres.2008.02.001
321. Wilson AC, Bishop DVM. Sex chromosome trisomies are not associated with atypical lateralization for language. Dev Med Child Neurol. (2018) 60:1132–9. doi: 10.1101/248286
322. Skakkebæk A, Gravholt CH, Chang S, Moore PJ, Wallentin M. Psychological functioning, brain morphology, and functional neuroimaging in Klinefelter syndrome. Am J Med Genet C Semin Med Genet. (2020) 184:506–17. doi: 10.1002/ajmg.c.31806
323. Hong DS, Hoeft F, Marzelli MJ, Lepage J-F, Roeltgen D, Ross J, et al. Influence of the X-chromosome on neuroanatomy: evidence from Turner and Klinefelter syndromes. J Neurosci. (2014) 34:3509–16. doi: 10.1523/JNEUROSCI.2790-13.2014
324. Pfeiffer C, Serino A, Blanke O. The vestibular system: a spatial reference for bodily self-consciousness. Front Integr Neurosci. (2014) 8:31. doi: 10.3389/fnint.2014.00031
325. Hughes JR. Ahmad SI, editor. Neurodegenerative Diseases. Advances in Experimental Medicine and Biology. Springer US, New York, NY (2012). p. 332–43. doi: 10.1007/978-1-4614-0653-2_25
326. Sorge G, Sorge A. Epilepsy and chromosomal abnormalities. Ital J Pediatr. (2010) 36:36. doi: 10.1186/1824-7288-36-36
327. Saniya K, Patil BG, Chavan MD, Prakash KG, Sailesh KS, Archana R, et al. Neuroanatomical changes in brain structures related to cognition in epilepsy: an update. J Nat Sci Biol Med. (2017) 8:139–43. doi: 10.4103/0976-9668.210016
328. Herzog AG. Psychoneuroendocrine aspects of temporolimbic epilepsy: Part I. Brain, reproductive steroids, and emotions. Psychosomatics. (1999) 40:95–101. doi: 10.1016/S0033-3182(99)71254-5
329. Jeon SJ, Gonzales EL, Mabunga DFN, Valencia ST, Kim DG, Kim Y, et al. Sex-specific behavioral features of rodent models of autism spectrum disorder. Exp Neurobiol. (2018) 27:321–43. doi: 10.5607/en.2018.27.5.321
330. Higashida N. The Wind I Become: The Landscape Where Myself with Autism Live in. Osaka, Japan: Big Issue Japan (2015). p. 189.
331. Corden B, Chilvers R, Skuse D. Avoidance of emotionally arousing stimuli predicts social–perceptual impairment in Asperger’s syndrome. Neuropsychologia. (2008) 46:137–47. doi: 10.1016/j.neuropsychologia.2007.08.005
332. Cuve HC, Gao Y, Fuse A. Is it avoidance or hypoarousal? A systematic review of emotion recognition, eye-tracking, and psychophysiological studies in young adults with autism spectrum conditions. Res Autism Spectr Disord. (2018) 55:1–13. doi: 10.1016/j.rasd.2018.07.002
333. van Rijn S, Swaab H, Baas D, de Haan E, Kahn RS, Aleman A. Neural systems for social cognition in Klinefelter syndrome (47,XXY): evidence from fMRI. Soc Cognit Affect Neurosci. (2011) 7:689–97. doi: 10.1093/scan/nsr041
334. Giedd JN, Clasen LS, Lenroot R, Greenstein D, Wallace GL, Ordaz S, et al. Puberty-related influences on brain development. Mol Cell Endocrinol. (2006) 254–255:154–62. doi: 10.1016/j.mce.2006.04.016
335. Reaven J. The treatment of anxiety symptoms in youth with high-functioning autism spectrum disorders: developmental considerations for parents. Brain Res. (2011) 1380:255–63. doi: 10.1016/j.brainres.2010.09.075
336. Puglia MH, Lillard TS, Morris JP, Connelly JJ. Epigenetic modification of the oxytocin receptor gene influences the perception of anger and fear in the human brain. Proc Natl Acad Sci. (2015) 112:3308–13. doi: 10.1073/pnas.1422096112
337. Duque-Wilckens N, Torres LY, Yokoyama S, Minie VA, Tran AM, Petkova SP, et al. Extrahypothalamic oxytocin neurons drive stress-induced social vigilance and avoidance. Proc Natl Acad Sci. (2020) 117:26406–13. doi: 10.1073/pnas.2011890117
338. Janecek M, Dabrowska J. Oxytocin facilitates adaptive fear and attenuates anxiety responses in animal models and human studies—potential interaction with the corticotropin-releasing factor (CRF) system in the bed nucleus of the stria terminalis (BNST). Cell Tissue Res. (2019) 375:143–72. doi: 10.1007/s00441-018-2889-8
339. Nasanbuyan N, Yoshida M, Takayanagi Y, Inutsuka A, Nishimori K, Yamanaka A, et al. Oxytocin–oxytocin receptor systems facilitate social defeat posture in male mice. Endocrinology. (2018) 159:763–75. doi: 10.1210/en.2017-00606
340. Yamagishi A, Lee J, Sato N. Oxytocin in the anterior cingulate cortex is involved in helping behavior. Behav Brain Res. (2020) 393:112790. doi: 10.1016/j.bbr.2020.112790
341. Tobiansky DJ, Wallin-Miller KG, Floresco SB, Wood RI, Soma KK. Androgen regulation of the mesocorticolimbic system and executive function. Front Endocrinol. (2018) 9:279. doi: 10.3389/fendo.2018.00279
342. Weafer J, de Wit H. Sex differences in impulsive action and impulsive choice. Addict Behav. (2014) 39:1573–9. doi: 10.1016/j.addbeh.2013.10.033
343. Bogdashina O. Autism and Spirituality: Psyche, Self and Spirit in People on the Autism Spectrum. London: Jessica Kingsley Publishers (2013). p. 274.
344. Devinsky O, Lai G. Spirituality and religion in epilepsy. Epilepsy Behav. (2008) 12:636–43. doi: 10.1016/j.yebeh.2007.11.011
345. Fitzgerald M. Autism and creativity: is there a link between autism in men and exceptional ability? New York: Routledge (2003). 304 p.
346. Fitzgerald M. The Genesis of Artistic Creativity: Asperger’s Syndrome and the Arts. London: Jessica Kingsley Publishers (2005). 256 p.
347. Lovecky DV. Spiritual sensitivity in gifted children. Roeper Rev. (1998) 20:178–83. doi: 10.1080/02783199809553887
348. Uddin LQ. Salience processing and insular cortical function and dysfunction. Nat Rev Neurosci. (2015) 16:55–61. doi: 10.1038/nrn3857
349. Rolland B, Jardri R, Amad A, Thomas P, Cottencin O, Bordet R. Pharmacology of hallucinations: several mechanisms for one single symptom? BioMed Res Int. (2014) 2014:e307106. doi: 10.1155/2014/307106
350. Russo M, Carrarini C, Dono F, Rispoli MG, Di Pietro M, Di Stefano V, et al. The pharmacology of visual hallucinations in synucleinopathies. Front Pharmacol. (2019) 10:1379. doi: 10.3389/fphar.2019.01379
351. Whitaker-Azmitia PM. Behavioral and cellular consequences of increasing serotonergic activity during brain development: a role in autism? Int J Dev Neurosci. (2005) 23:75–83. doi: 10.1016/j.ijdevneu.2004.07.022
352. Garbarino VR, Gilman TL, Daws LC, Gould GG. Extreme enhancement or depletion of serotonin transporter function and serotonin availability in autism spectrum disorder. Pharmacol Res. (2019) 140:85–99. doi: 10.1016/j.phrs.2018.07.010
353. Andersson M, Tangen Ä, Farde L, Bölte S, Halldin C, Borg J, et al. Serotonin transporter availability in adults with autism—a positron emission tomography study. Mol Psychiatry. (2021) 26:1647–58. doi: 10.1038/s41380-020-00868-3
354. Brandenburg C, Blatt GJ. Differential serotonin transporter (5-HTT) and 5-HT 2 receptor density in limbic and neocortical areas of adults and children with autism spectrum disorders: implications for selective serotonin reuptake inhibitor efficacy. J Neurochem. (2019) 151:642–55. doi: 10.1111/jnc.14832
355. Hanson AC, Hagerman RJ. Serotonin dysregulation in Fragile X Syndrome: implications for treatment. Intractable Rare Dis Res. (2014) 3:110–7. doi: 10.5582/irdr.2014.01027
356. Kennedy D. The ADHD-Autism Connection: A Step Toward More Accurate Diagnoses and Effective Treatments. Colorado Springs, CO: WaterBrook (2002). p. 224.
357. Heyer DB, Meredith RM. Environmental toxicology: sensitive periods of development and neurodevelopmental disorders. NeuroToxicology. (2017) 58:23–41. doi: 10.1016/j.neuro.2016.10.017
358. Guma E, Plitman E, Chakravarty MM. The role of maternal immune activation in altering the neurodevelopmental trajectories of offspring: a translational review of neuroimaging studies with implications for autism spectrum disorder and schizophrenia. Neurosci Biobehav Rev. (2019) 104:141–57. doi: 10.1016/j.neubiorev.2019.06.020
359. Inui T. [Cerebral mechanism through which incorrigible belief on the world derived from erroneous sensory perception]. Seishin Shinkeigaku Zasshi. (2012) 114:171–9.
360. Danforth AL, Grob CS, Struble C, Feduccia AA, Walker N, Jerome L, et al. Reduction in social anxiety after MDMA-assisted psychotherapy with autistic adults: a randomized, double-blind, placebo-controlled pilot study. Psychopharmacol (Berl). (2018) 235:3137–48. doi: 10.1007/s00213-018-5010-9
Keywords: savant syndrome, gender identity, free-energy principle, hyper/hypoesthesia, autism spectrum condition, Klinefelter syndrome, estrogen deficiency syndrome
Citation: Sakaguchi K and Tawata S (2024) Giftedness and atypical sexual differentiation: enhanced perceptual functioning through estrogen deficiency instead of androgen excess. Front. Endocrinol. 15:1343759. doi: 10.3389/fendo.2024.1343759
Received: 24 November 2023; Accepted: 15 April 2024;
Published: 01 May 2024.
Edited by:
Jean-Michel Le Melledo, University of Alberta, CanadaReviewed by:
Carolina Dalmasso, University of Kentucky, United StatesMaria Jesus Herrero, San Sebastian University, Chile
Copyright © 2024 Sakaguchi and Tawata. This is an open-access article distributed under the terms of the Creative Commons Attribution License (CC BY). The use, distribution or reproduction in other forums is permitted, provided the original author(s) and the copyright owner(s) are credited and that the original publication in this journal is cited, in accordance with accepted academic practice. No use, distribution or reproduction is permitted which does not comply with these terms.
*Correspondence: Kikue Sakaguchi, sakaguti@niad.ac.jp