Acid leaching of hydrothermally carbonized sewage sludge: phosphorus recovery and hydrochar characteristics
- 1Department of Waste and Resource Management, Faculty of Agricultural and Environmental Sciences, University of Rostock, Rostock, Germany
- 2Deutsches Biomasseforschungszentrum (DBFZ), Leipzig, Germany
- 3School of Data Science, Indian Institute of Science Education and Research, Thiruvananthapuram, India
The options for managing sewage sludge and its utilization as fertilizer are becoming progressively limited as a result of stringent environmental regulations imposed by the European Union over the past 10 years. The limitation of moisture present in sewage sludge that affects conventional treatment options like incineration can be obviated by using the hydrothermal carbonization (HTC) process. This research investigates the acid leaching of hydrochar produced by hydrothermally carbonizing sewage sludge. The objective is to investigate and compare the effects of formic acid (HCOOH), sulfuric acid (H2SO4), and acetic acid (CH3COOH) at varying pH levels on total phosphorus (TP) mobilization and hydrochar properties. The impact of independent parameters such as carbonization temperature of hydrochar, acid type, acid concentration, and acid leaching retention time on the TP mobilization, chemical oxygen demand (COD) of the leachate, and the fuel characteristic of the hydrochar was explored. A quadratic and cubic model was proposed to correlate the effects of independent parameters on TP and ortho-P mobilization, acid need, COD of leachate, and fuel characteristics of hydrochar using Design of Experiments and Response Surface Modeling. This approach was chosen in order to maximize the amount of data from a constrained number of experimental trials. The outcome of the study indicated a fractional amount of H2SO4 was enough to reach and maintain the lower pH in hydrochar slurry compared to HCOOH and CH3COOH. TP mobilization from solid to liquid is highly favorable in the presence of H2SO4 at lower pH compared to HCOOH and CH3COOH under similar reaction conditions. In addition, it was discovered that lowering the pH using HCOOH and H2SO4 to acid-leach the hydrochar boosted the caloric value of the hydrochar. However, CH3COOH has a contradictory effect.
1 Introduction
Phosphorus (P) is a critical element whose availability is vital for meeting agricultural and industrial demands across the world. In recent years, global phosphate demand has steadily increased due to the accelerated growth of the economy (Cieślik and Piotr Konieczka, 2017). However, the global phosphate mineral resource is non-renewable, limited to a few countries, and projected to be exhausted in 50–100 years (Poirier et al., 2022). As a result, there is an urgent need for the efficient management and recycling of phosphorus-rich by-products in order to meet future global demands. Recovering P from sewage sludge produced in a wastewater treatment plant (WWTP) is one such potential approach. Municipal WWTPs often convert phosphate from wastewater into sludge, making sewage sludge the most popular by-product with a substantial source of P. Recent estimates indicate that the quantity of industrial and domestic wastewater generated annually on a global scale is in the order of billions of tons and is anticipated to increase due to population growth and rising standards of living (Bora et al., 2019). The production of sewage sludge in Europe (EU27) is estimated to be approximately 10 million tonnes of dry matter (DM) annually (Domini et al., 2022). Germany alone has produced 1.72 million tonnes of sewage sludge DM in 2022, which demonstrates the potential of sewage sludge as an alternative source of P (Statistisches Bundesamt Destatis, 2022). Most WWTPs treat phosphorus-containing wastewater with enhanced biological phosphorus removal or chemical precipitation, which transfers >90% of the phosphorus from the unprocessed wastewater to the sewage sludge (Liang et al., 2019). The primary utilization pathway for sewage sludge includes thermal disposal in mono- and co-incineration facilities, recycling in agriculture, recycling in landscaping, and recycling of other materials through humification and composting. The management of sewage sludge varies significantly among EU Member States. In Spain, Ireland, Finland, Hungary, and Cyprus, land-based utilization or composting is the most popular method, whereas the Netherlands, Belgium, Germany, and Austria mostly employ incineration (Domini et al., 2022). The availability of agricultural areas and local regulations are the key factors affecting the decision to recover or dispose of sludge. The direct use of sewage sludge for agricultural purposes is, however, restricted in many countries due to the presence of harmful substances such as heavy metals, organic residues, microplastics, and various pathogens. The sustainable management of sewage sludge is a global concern due to its potential for negative environmental effects. Nevertheless, the organic matter and nutrients present in sewage sludge have the potential to be used to produce renewable energy and are essential for the production of fertilizers.
The German Sewage Sludge Ordinance (AbfKlärV) is a particular regulation under German waste law that specifies the application and utilization of sewage sludge (AbfKlärV, 2017). The new rule set by the German sewage sludge ordinance restricts the direct use of sewage sludge in agriculture and also mandates the recovery of phosphorus from sewage sludge in Germany (AbfKlärV, 2017). Following the 1999 ban on landfilling in the European Union (EU), incineration has become the preferred waste disposal method in the EU-15 (Raheem et al., 2018). In 2022, 1.3 million tonnes DM (approximately 77% of the total generated sewage sludge) have been thermally recycled (mono-incineration, co-incineration, and other thermal disposals), and the trend of sewage sludge being managed by land-based utilization/landscaping is decreasing (Statistisches Bundesamt Destatis, 2022). Although thermal treatment of sewage sludge is generally accepted, the most significant drawback of incineration is the high energy requirements of thermal dewatering (Werther and Ogada, 1991). In this regard, hydrothermal carbonization (HTC) has received increased attention as an environmentally acceptable and viable approach for treating sewage sludge without dewatering it. The HTC process uses the excess moisture in sewage sludge as a catalyst and converts the sewage sludge into a carbonaceous product at reaction temperatures ranging from 180°C to 260°C at 0.5–24 h retention time and at elevated autogenous pressures (Jellali et al., 2022).
In recent years, there has been considerable interest in the investigation of HTC as a potential treatment option for sewage sludge, as well as studies examining the P transformation during and after HTC. Several studies reported the distribution and transformation of P during HTC of sewage sludge (Wang et al., 2017; Ovsyannikova et al., 2019; Shi et al., 2019), acid leaching of sewage sludge and hydrochar using 2.5 M acid solutions of H2SO4 and HCl (Pérez, Boily, and Jansson, et al., 2021), the influence of organic acid (citrate and oxalate) leaching on the P transformation from sewage sludge hydrochar (Pérez, Boily, and Skoglund, et al., 2022), migration and transformation of phosphorus during hydrothermal carbonization of sewage sludge (Zheng et al., 2020b), and phosphorus recovery from sewage sludge incineration ash (Liang et al., 2019). Numerous previous studies have demonstrated that a significant amount of P from sewage sludge is retained in the hydrochar after HTC (Heilmann and Valentas, 2014; Wang et al., 2017; Wang et al., 2019). The previously published results indicate that 97.7%–98.7% of total P (TP) is retained in the resulting hydrochar after HTC treatment of sewage sludge (Shettigondahalli Ekanthalu et al., 2021). Variation in pH has a major effect on the transformation of P from the solid to the liquid phase, and the alteration of pH after HTC was found to be highly efficient (Shettigondahalli Ekanthalu et al., 2022). Prior research has examined the independent effects of organic and inorganic acids on the acid leaching of sewage sludge hydrochar. The effect of acid leaching of sewage sludge hydrochar utilizing organic and inorganic acids under varying conditions of acid strength and time has not been investigated yet, to the authors’ knowledge.
This study focuses on acid leaching the sewage sludge hydrochar with formic acid (HCOOH), sulfuric acid (H2SO4), and acetic acid (CH3COOH) at varying pH to determine the effects on P mobilization, hydrochar characteristics, and leachate characteristics. In addition to comprehending the impact of pH on P leaching, the purpose of this study is to investigate what other acid properties influence P leaching from solids to liquids. A Design of Experiments (DoE)/Response Surface Model (RSM) method facilitates the investigation of the selected reaction conditions within a defined reaction space. The DoE/RSM has been widely used in HTC (Mäkelä et al., 2015; Mäkelä et al., 2016; Román et al., 2018), for sewage sludge dewatering (Danso-Boateng et al., 2015; Lühmann and Wirth, 2020), optimization of hydrochar production (Zheng et al., 2020a; Guo et al., 2021; Akbari et al., 2022), and the influence of pH on P release and transformation in hydrothermally carbonized sewage sludge (Lühmann and Wirth, 2020). To date, acid-leaching hydrochar process parameters such as acid usage and the role of pH in P transformation, as well as hydrochar characteristics, have only been studied separately with particular acid utilization. The DoE/RSM methodology employed in this study seeks to comprehend the impacts of various acids and their interactions with hydrochar on the characteristics of hydrochar and leachate, with a specific emphasis on P mobilization. The results of this study offer an in-depth overview of the impact of pH and acid characteristics on the leaching of phosphorus from the solid to liquid phase as well as the properties of hydrochar.
2 Materials and methods
2.1 Materials
The digested and mechanically dewatered sewage sludge used in this investigation was directly obtained from Nordwasser GmbH in Rostock, Germany. The primary wastewater treatment facility in Rostock can handle the wastewater from 320,000 inhabitants and treats both industrial (1/3) and municipal (2/3) wastewater (UBC Sustainable Cities Commission, 2017). This WWTP produced 4,482 tonnes of dry sewage sludge per year on average between the years of 2014 and 2018, and all of the generated sewage sludge is currently incinerated (Tränckner, 2023). Sewage sludge was only sampled once during the treatment plant’s steady operation state to ensure consistency of results throughout the investigation. The sewage sludge sample was collected in a sealed sample container and immediately transferred to the laboratory, where it was refrigerated at 4°C until it was used for HTC. The dry matter content of the sewage sludge was 22.0% dry matter content (DM), and the ash content was 7.4% (at 815°C in % original substance). The refrigerated sewage sludge samples were allowed to warm to room temperature before HTC.
A LECO Thermogravimetric Analyzer (TGA) unit TGA701 was used to evaluate the moisture content, volatile organic compound, fixed carbon (FC), and ash content of sewage sludge. The final analysis was carried out using an organic element analyzer in accordance with EN ISO 16948, 2015 (EN ISO 16948 2015). The caloric value of the sample material was evaluated using a Parr 6400 calorimeter (Parr Instruments Inc., United States) in accordance with EN 14918, 2010 (EN 14918 2010). The total phosphate content of the sewage sludge was determined in an external laboratory using EN ISO 11885, 2009 (EN ISO 11885 2009).
Acid-leaching of hydrochar is facilitated using either formic acid 98% pure, sulfuric acid 72%, or acetic acid 100% (all the acid reagents were obtained from AppliChem GmbH, Darmstadt). The amount of acid required to attain the targeted pH was determined by following the methodology of DIN EN 15933:2012 (Deutsches Institut für Normung, 2012).
2.2 Experimental method
An overview of the experimental methodology is provided in Figure 1. The experimental methodology was planned in four stages: stage 1: HTC of sewage sludge, stage 2: DoE using Stat-Ease Design-Expert Software (Version 22.0.0), stage 3: acid leaching of hydrochar, and Stage 4: product recovery, experimental analysis, and regression surface modeling.
2.2.1 Hydrothermal carbonization of sewage sludge
A Parr 4523 reactor (Parr Instrument GmbH, Zeilweg 15, 60439 Frankfurt, Germany) was used to hydrothermally carbonize sewage sludge. The reactor operates at autogenic pressure and is equipped with a 1-L reaction vessel that can tolerate a maximum pressure of 138 bar. The heat for the reactor is provided by a 2 kW heating coil, and the stirring is facilitated by a stirring unit with a connected drive. The pressure and temperature sensors of the reactor are controlled and monitored using a Parr 4848 PID controller unit.
To understand the influence of HTC temperature on the acid leaching of consequently produced hydrochar, HTC was carried out at temperatures of 200°C, 220°C, and 240°C and a retention time of 2 h while continuously stirring the substrate at 150 rpm. Before beginning HTC, certain volumes of sewage sludge and distilled water were combined within the reactor and mixed to generate a homogenous slurry of 10% DM, and the initial pH was noted using a pH meter (WTW pH 3310, Xylem Analytics Germany Sales GmbH). The HTC of sewage sludge slurry was carried out at an autogenic pressure with a continuous heating rate of 4 K/min. Following HTC, the reactor vessel was left to cool to room temperature, and the final pH was recorded. Following the top-feeding method, solids and liquids were separated from the HTC slurry in a Büchner funnel. The hydrochar produced at three different temperatures was oven-dried at 105°C for 24 h. The dried hydrochar was collected separately (according to the temperature at which it was produced), crushed to produce fine powder, and stored in an airtight container at room temperature until used.
2.2.2 Design of experiments
The Design-Expert program from Stat-Ease, Inc., was used to design the experiments, perform regression analysis, and model the response surface. The experiments were designed to reveal the interactions of three independent variables with the targeted responses. The three independent variables selected include the following process conditions: 1) the carbonization temperature at which the hydrochar was produced: 200°C, 220°C, and 240°C, 2) pH during the acid leaching process: 1.5, 2.125, and 2.75, and 3) acid leaching time: 15 min, 30 min, and 45 min. Using Box–Behnken design, three replications at the central points and one point in the middle of each edge of the cubical surface were employed, resulting in 15 experimental runs for every acid used. The product of each experimental run was recovered and experimentally analyzed to generate six different targeted responses. The following responses were considered in this study: TP in leachate, ortho-P in leachate, COD of leachate, acid needed, %TP leached, and higher heating value (HHV) of hydrochar. Figure 1 (stage 2) is a graphical representation of the experimental design space, and Supplementary Material S1 includes the randomized experimental design.
2.2.3 Acid leaching process
In the experimental design described in Figure 1, three different acids were used to achieve the targeted pH, and the effects of different acids (HCOOH, H2SO4, and CH3COOH) on the TP-transformation, ortho-P transformation, COD, acid need, and HHV of hydrochar were experimentally analyzed. The experimentally analyzed results of the defined responses were fitted into the RSM model to generate respective 3D-surface models. During the acid-leaching process, the amount of acid required to attain the targeted pH was determined following the methodology of DIN EN 15933:2012 (Deutsches Institut für Normung, 2012). The standard DIN EN 1593:2012 specifies a method for determining the pH in suspension of sludge within the pH range of 2–12. However, to maintain uniformity in the experimental procedure, the same standards were used to determine and achieve a pH of 1.5.
A measure of 5 g of hydrochar (produced at 200°C, 220°C, and 240°C) was mixed with 45 mL of deionized water, and a homogeneous slurry was prepared by mixing the content for 15 min on a magnetic stirrer at 250 rpm. After preparing the homogeneous slurry, concentrated acids (one each of HCOOH, H2SO4, or CH3COOH) were slowly titrated against the slurry whilst constantly stirring the content until the desired pH was attained and held constant for 15 min. Later, the pH was held constant for 15 min, 30 min, and 45 min to facilitate the acid leaching of the hydrochar.
2.2.4 Product recovery experimental analysis and regression modeling
2.2.4.1 Product recovery experimental analysis
The hydrochar slurry produced after the acid leaching was sent directly to the polysulfone bottletop vacuum filter for the separation of solids and liquids. The following process conditions were maintained during the solid–liquid separation of the hydrochar slurry: 1) the entire content of the hydrochar slurry after acid-leaching was poured into the polysulfone bottletop vacuum filter and 2) the vacuum pump was turned on to create sufficient vacuum pressure for separating solids and liquids. After the filtering, the liquid was collected in a volumetric flask and kept at 4°C until it was tested for TP, ortho-P, and COD. Similarly, the resulting solids (hydrochar) were oven-dried at 105°C for 24 h before being kept in sealed containers for further examination.
Following the procedure outlined in EN 15170, 2010, the caloric value of the hydrochar was analyzed using a Parr 6400 calorimeter in a manner identical to that of sewage sludge. Liquid (leachate) was analyzed spectrophotometrically for TP and ortho-P using the NANOCOLOR ortho- and total-Phosphate tube test kits in accordance with DIN EN ISO 6878-D11. Lastly, following the standards specified in DIN ISO 15705, the COD of the leachate was analyzed using a NANOCOLOR COD 1500 tube test kit. All the spectrophotometric testing kits were obtained from MACHEREY-NAGEL GmbH & Co. KG, Germany. The studied findings were obtained in triplicate, and the mean value is presented.
2.2.4.2 Regression modeling
The DoE/RSM approach was chosen to understand the interaction between selected independent variables and their resulting responses. A DoE/RSM strategy was used in order to maximize the amount of data from a constrained number of experimental trials (Lühmann and Wirth, 2020). The DoE was designed with a Box–Behnken design of RSM methodology using Design-Expert software. Box–Behnken design is an approach that uses the minimum square technique to fit the model and defines the interactions of parameters and their influence on responses (Akbari et al., 2022).
The regression modeling was performed as described by Montgomery (2013), and the interactions between the selected variables and the resulting responses were modeled using quadratic Eq. 1 and cubic Eq. 2.
where
A specific model was chosen for every particular response to reduce the lack of fit value in relation to the pure error. For the quadratic and cubic models, the respective lack of fit values were analyzed with respect to the pure error. A non-significant lack of fit value (p-value greater than 0.05) indicates good predictive generalizability of the regression model. In some cases, reduced versions of the original quadratic and cubic models were used to ensure that the lack of fit value was non-significant. This ensures that the potential for overfitting of simpler or reduced versions of the original models decreases by improving their overall predictive reliability on an unseen data space. Table 1 and Supplementary Material S2 illustrate the specifications of the quadratic and cubic regression models that were obtained in the current study.
3 Results and discussion
3.1 Characteristics of sewage sludge and consequently produced hydrochar
The results of the initial and final analysis of sewage sludge are shown in Table 2. The sewage sludge used in this study had a moisture content of 78.00% and a total solid content of 22.00%. The initial analysis depicted that the sewage sludge had an ash content of 33.64% DM at 815°C and volatile solids (VS) of 65.00% DM, which was consistent with the previous investigation ranges (Peng et al., 2016; Wang et al., 2017; Shettigondahalli Ekanthalu et al., 2020). The final analysis results had a typical value for C-H-N-S-O content for sewage sludge in Germany (Roskosch and Heidecke, 2018), with C: 34.70%; H: 4.90%; N: 4.80%; S: 1.60%; and O: 17.60% on a dry basis. The dry sewage sludge is known to contain a high concentration of phosphorus and moderate heating value. The TP content in the feedstock was determined to be 34.4 g/kg, accounting for 3.4% of total dry sludge, and the heating value was observed to be relatively higher, at 14.72 MJ/kg (HHV), than was observed in previous studies (Peng et al., 2016; Wang et al., 2017; Roskosch and Heidecke, 2018). The overall characteristics of the feedstock have the typical composition of sewage sludge in Germany.
3.2 RSM process optimization
Table 3 shows the analysis of variance (ANOVA) and fit statistics for the regression model. These quantities signify the reliability of the regression model based on the experimental data used to model the response surface. Fischer (F) test results and probability (p-value) can be used to gauge the regression model’s effectiveness. An F-value is the ratio of two group variations used to determine the statistical significance between the means of the groups. A p-value is a statistical measurement used to validate a hypothesis against the observed data. A regression model with larger F-values and smaller p-values is more reliable. As depicted in Table 3, all the analyzed responses have p-values <0.05 and a larger F-value, indicating a greater statistical significance of the designed models.
The ANOVA of the residual data depicts unexplained variations in the response. Lack of fit refers to the situation where a statistical model fails to adequately fit the underlying data. In other words, it occurs when a regression model does not capture the relationship between the independent variables and the dependent variables. A strong lack of fit (p-value greater than 0.05) is an undesirable property because it indicates that the model does not fit the data well. It is desirable to have an insignificant lack of fit (p-value smaller than 0.05). As shown in Table 3, the lack-of-fit p-values for all the obtained responses were not significant relative to the pure error, meaning the model fits well, and the parameters have a significant effect on the output response.
The fit statistics shown in Table 3 are intended to provide the reader with an understanding of the quality of the regression model. Fit statistics include the data on
Adequate precision is a measure of the range in predicted response relative to its associated error; in other words, it is a signal-to-noise ratio. A ratio greater than four is desirable (Stat-Ease, 2022). All the obtained adequate precision values in this study were >4, confirming an adequate signal and the possibility of using the model to navigate the design space with greater reliability.
3.3 Acid requirement in achieving the targeted pH
Figure 2 displays the response surface plot of the amount of acid required to achieve and maintain the desired pH in the hydrochar slurry for 30 min of acid leaching. The demand for acid was unaffected by a 15-min change in leaching time either way (See Supplementary Figure S3). In comparison to organic acids, using inorganic acid required less acid to achieve and maintain the desired pH. Among the organic acids, acetic acid required ∼seven-fold more acid than formic acid to achieve a similar pH. For instance, ∼1.5 mL of H2SO4 (72% sulfuric acid) is required to achieve and maintain the pH of 1.5 in a 50 g hydrochar slurry of 10% dry matter with an initial pH of ∼5.73 (+/− 0.2); ∼15 mL of formic acid (98% formic acid) is required to achieve the same conditions, and the acid requirement for acetic acid (100% acetic acid) increases significantly to ∼100 mL. Several parameters influence the target of achieving and maintaining a lower pH during acid leaching of hydrochar. The primary influencing parameter is the type of acid; acid strength and other parameters include the HTC process and diverse chemical reaction that occurs within the process.
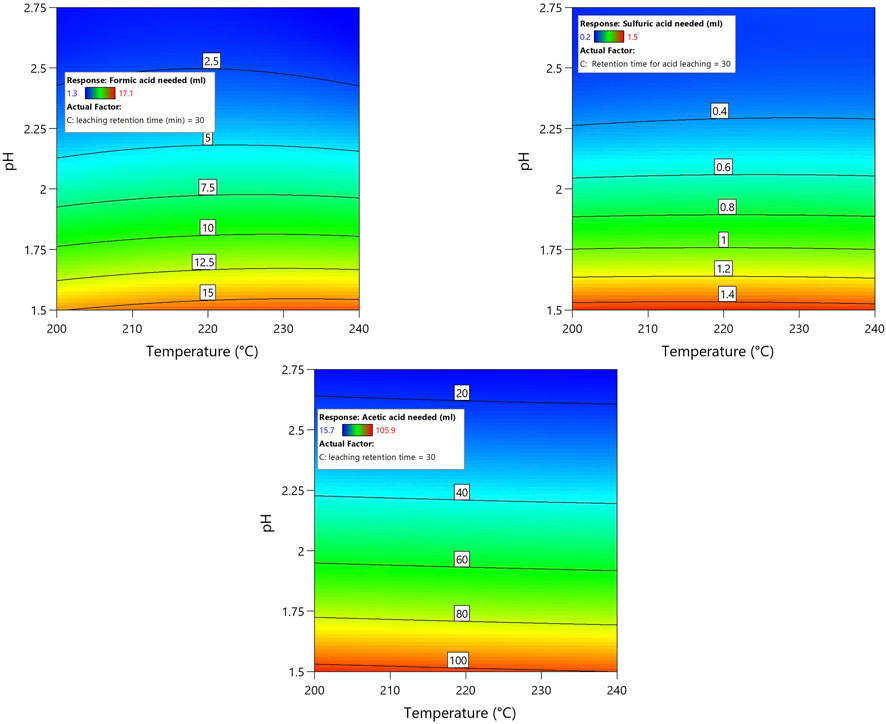
FIGURE 2. Regression model contour plot depicting the influence of acids on reaching the targeted pH.
It was seen in the experimental analysis that H2SO4 needed a fractional amount of acid compared to CH3COOH and HCOOH to reach and maintain the lower pH. Similarly, HCOOH also needed less acid than CH3COOH. This phenomenon can be explained using the strength of the acid. One approach for describing the strength of any acid is its pKa value. The negative log of the Ka value, also known as the acid dissociation constant, is the pKa value (Helmenstine, 2020). A stronger acid is indicated by a lower pKa value, and the lower pKa value indicates that the acid can be fully dissociated in water (Helmenstine, 2020). Of the different acids used in this investigation, H2SO4 is the strongest acid with a pKa value of −3.0, indicating the presence of highly acidic components that can readily donate protons in water (Stumm and Morgan, 1981). Formic acid, a simple carboxylic acid with a pKa value of 3.75, is a weaker acid than H2SO4. However, formic acid is relatively stronger than acetic acid, which has a higher pKa value of 4.7 (Stumm and Morgan, 1981). Hence, H2SO4, with its lower pKa value, needs only a fraction of the amount of acid required to achieve and maintain lower pH in hydrochar slurry compared to the amount needed for CH3COOH and HCOOH with their higher pKa values.
When half of the acid has dissociated, pKa and pH are equal. When the pKa and pH values are close, the buffering capacity of a species, or its ability to maintain the pH of a solution, is greatest. Therefore, the optimal choice for a buffer is one with a pKa value close to the desired pH of the chemical solution (Helmenstine, 2020). In the hydrolysis phase of the HTC process, volatile fatty acids (VFAs) are created, which results in the production of organic acid molecules and contributes to acidification (Danso-Boateng et al., 2015; Woriescheck, 2019). The presence of increased VFAs after the HTC of sewage sludge could also be one parameter influencing the larger amount of an organic acid (CH3COOH and HCOOH) required to achieve a lower pH compared to the amount of inorganic acids (H2SO4) required.
3.4 Effect of different acid utilization and acid leaching retention time on COD of leachate
The HTC process water is organically and inorganically contaminated, and COD is characterized as one of several organic contaminants. The organic contaminants in the process water produced by HTC usually have high levels of COD that depend upon the HTC process conditions and input materials (Woriescheck, 2019). During the HTC of sewage sludge, the decomposition of the organic acidic compounds and their displacement from the solid to the liquid phase contributes to the acidification of the liquid phase and simultaneous increase in COD (Jellali et al., 2022). In general, the HTC process decreases the pH and increases the soluble COD content, particularly for the digested and dewatered sludge (Merzari et al., 2020). Several organic compounds, especially the VFAs, create a major organic load in the process water after HTC, in turn increasing the COD of the process water. VFAs are formed during the hydrolysis stage of the HTC process and produce acids such as acetic acid, propionic acid, butyric acid, valeric acid, and levulinic acid (Danso-Boateng et al., 2015). The presence of these acids in the liquid phase increases the concentration of protons or hydroxide ions, further catalyzing decarboxylation and dehydration reactions (Reza et al., 2015; Guo et al., 2021). Among VFAs, acetic acid is the main organic acid produced during HTC through the hydrolysis and dehydration of straight-chain polymers, such as cellulose and hemicellulose, or simple monomers in the presence of subcritical water (Liu et al., 2020).
Figure 3 depicts the response surface plot of the COD of the leachate obtained after acid leaching the hydrochars using different acids at different pH levels with a 30 min retention time. The influence of acid leaching retention time on COD was insignificant with varying acid leaching retention time (+/−15 min) (see Supplementary Figure S4). In general, during the acid leaching process, using organic acids (HCOOH and CH3COOH) to achieve the targeted pH results in higher COD in the leachate compared to the use of an inorganic acid (H2SO4). COD was found to increase with increasing pH when H2SO4 and HCOOH were used for acid leaching. However, the use of CH3COOH for acid leaching did not have a greater influence on the COD, irrespective of varying pH. Compared to the use of organic acids, the use of inorganic acids might have leached a larger amount of organic material, furfural, and 5-HMF, together with nutrients. This could explain the significantly increased COD with H2SO4 compared to organic acid utilization. Additionally, an increased COD can be seen with formic acid utilization as well as an increase in the acid concentration. However, there is no increase in COD with the use of acetic acid despite the increase in the pH. One reason for such phenomena could be that acetic acid is the main organic acid produced during the HTC process, and further addition of acetic acid during acid leaching processes decreases the pH but does not increase the organic load or influence the COD concentration.
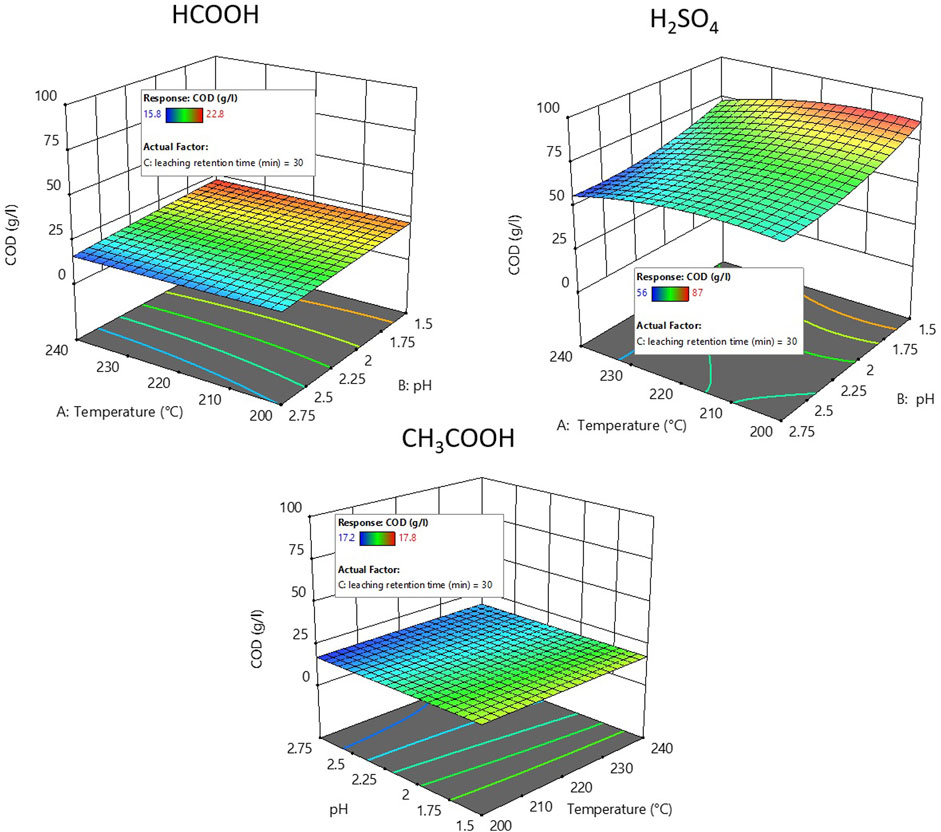
FIGURE 3. Response surface plot showing COD of the leachate produced after acid leaching using H2SO4, HCOOH, and CH3COOH.
3.5 Effect of different acids on P-transformation from solid to the liquid phase
Figure 4 displays the response surface plot showing the effects of the different acid types and their concentration (pH) on the transformation of TP from solid to liquid phase during an acid-leaching process. TP in the leachate was analyzed spectrophotometrically after acid hydrolysis and oxidation of the sample, and the results were used to plot the RSM plot. Figure 4 depicts the results from an acid leaching retention time of 30 min. Altering the acid leaching retention time by +/−15 min did not have a great influence on TP transformation (See Supplementary Figure S5, S6). In brief, the results show that acid leaching the hydrochar slurry using H2SO4 at pH 1.5 transformed approximately 90% (+/−1%) of TP from the solid to the liquid phase, whereas HCOOH and CH3COOH transformed only approximately 36% (+/−6%) and 2.5% (+/−1%), respectively, under similar reaction conditions. The ortho-P transformation from solid to liquid also had a similar trend to that of TP and is depicted in the response surface plot of Figure 5.
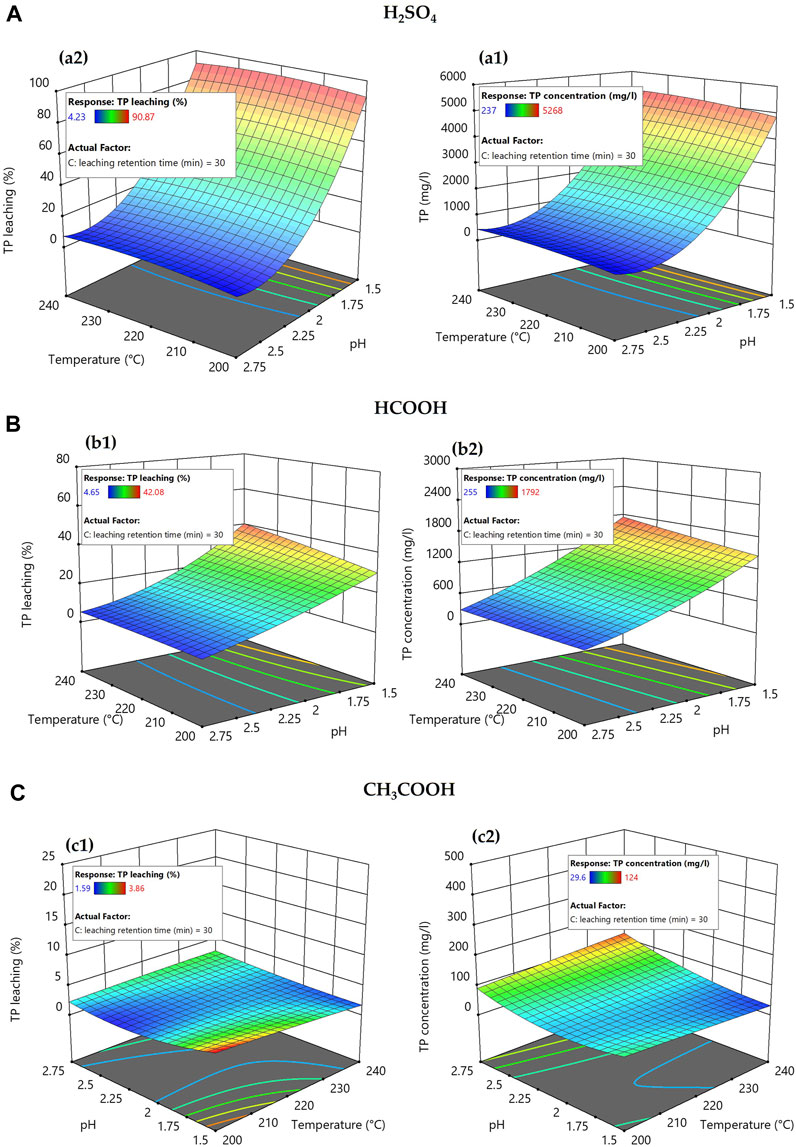
FIGURE 4. Response surface plot showing the effect of carbonization temperature of hydrochar and acid leaching pH on the transformation of TP from solid to liquid phase.
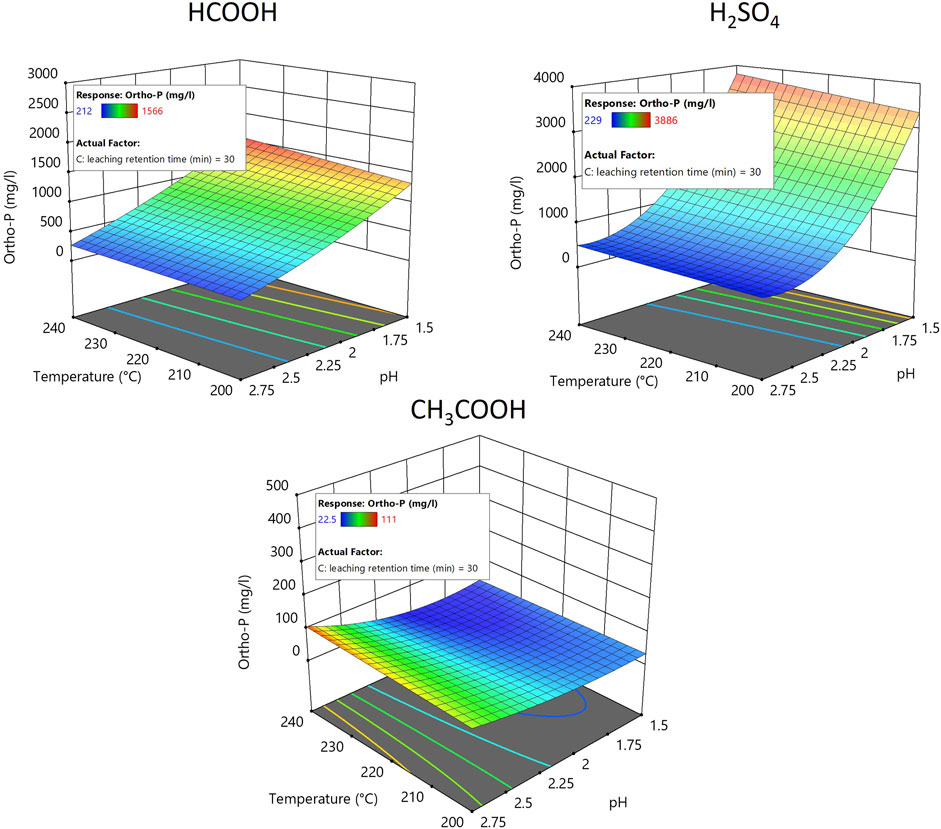
FIGURE 5. Response surface plot showing the effect of the carbonization temperature of hydrochar and acid leaching pH on the transformation of ortho-P from solid to liquid phase.
Following the acid leaching of hydrochar, the highest TP transformation from solid to liquid (5,268 mg/L or 90.87%) was observed by using H2SO4 and acid leaching at pH 1.5. The maximum TP transformation from solid to liquid following acid leaching at pH 1.5 using HCOOH and CH3COOH was 1,721 mg/L (42.08%) and 59.5 mg/L (3.36%), respectively. The results suggested that compared to inorganic acid (H2SO4), organic acids (HCOOH and CH3COOH) with similar pH have a limited impact on transforming TP from solid to liquid, which agrees with the previous studies (Ekpo et al., 2016; Wang et al., 2017; Shettigondahalli Ekanthalu et al., 2021). In addition to the acid used and the pH, the reaction temperature at which the hydrochar was produced also had a slight influence on the TP transformation. The hydrochar generated at 240°C had an 8.7% greater TP transition from solid to liquid than the hydrochar produced by acid-leaching (H2SO4, pH 1.5) at 200°C. The TP transformation of HCOOH was 11.5% higher under identical reaction circumstances, while the impacts of CH3COOH were too small to support any conclusion.
During HTC of sewage sludge, the presence of a higher concentration of multivalent metal ions, such as Al3+, Ca2+, Fe3+, and Mg2+, is responsible for forming phosphate with low solubility and, in turn, enabling the phosphate to be retained in subsequently produced hydrochar (Huang et al., 2018). The solubility of these multivalent metal ions has a significant impact on the TP mobility from a solid to a liquid during the acid-leaching procedure. Factors influencing the TP transformation from solid to liquid include the strength of the acid (Dai et al., 2017), the pH of the leaching medium (Petzet et al., 2012), and the ability of the acid to mobilize the phosphorus-holding compounds (ferric phosphate, ferrous phosphate, aluminum phosphate, calcium phosphate, and magnesium phosphate). The primary chemical reactions for the TP transformation from solid to liquid are included in the following equation.
The reactions in Eqs 3–6 are highly favorable under strongly acidic conditions and, particularly, the presence of strong acids like H2SO4. To facilitate the primary reactions for TP transformation, there is a requirement for greater acid concentration to overcome the buffering resistance provided by the hydrochar slurry (Shettigondahalli Ekanthalu et al., 2022). Figure 6 displays the concentration of Al3+ and Fe3+ ions in the leachate produced by acid-leaching hydrochar with HCOOH, H2SO4, and CH3COOH at pH values of 1.5, 2.125, and 2.75. The use of stronger acids or acids with lower pKa values has a substantially greater effect on the dissolution of Al3+ and Fe3+ ions and their corresponding P, as demonstrated by the experimental findings. Using H2SO4, which has a pKa value of −3.0, resulted in the greatest Al3+ and Fe3+ ion discharge, followed by HCOOH and CH3COOH, which have pKa values of 3.75 and 4.70, respectively. The use of CH3COOH for acid leaching has shown visibly poor dewaterability compared to H2SO4 and HCOOH. The hydrophilic nature of hydrochar slurry produced by the addition of CH3COOH can also hinder the migration of phosphorus-containing compounds from solid to liquid.
With the utilization of H2SO4, a slight decrease in the pH of the acid-leaching medium significantly increased the TP mobilization from solids to liquid. This is because at lower acid concentrations, most of the acid present in the reaction medium is utilized to offset the buffer resistance provided by acid-consuming compounds present in the reaction medium with relatively higher neutral points; see Eqs 5, 6.
3.6 Effects of different acid utilization on the caloric value of hydrochar produced after acid leaching
The caloric value of the hydrochar produced after acid leaching using different acids at different pH levels is illustrated in Figure 7. The illustrated Figure 7 particularly shows the caloric value of hydrochar produced at an acid leaching retention time of 30 min. Increasing or decreasing the leaching time by 15 min did not have any greater influence on the caloric value of the hydrochar produced (See Supplementary Figure S7). Treating the hydrochar with different acid-leaching conditions influenced its caloric value. Prior to acid leaching, hydrochar had a caloric value ranging between 13.43 MJ/kg and 13.95 MJ/kg, and after acid leaching, the caloric value of hydrochar ranged between 10.97 MJ/kg and 15.64 MJ/kg. The caloric value for the hydrochar after acid leaching using HCOOH and H2SO4 ranged between 15.64 MJ/kg and 13.43 MJ/kg and between 14.16 MJ/kg and 12.19 MJ/kg, respectively, which is comparable to the findings of other research examining the caloric value of hydrochar produced by hydrothermally carbonizing digested sewage sludge (He et al., 2013; Shettigondahalli Ekanthalu et al., 2021; Shettigondahalli Ekanthalu et al., 2022). However, the caloric value for the hydrochar after treatment with CH3COOH was in the range of 13.49–10.97 MJ/kg, which is less than that of hydrochar treated using different acids in this study. Furthermore, it was also noticed that decreasing the pH during acid leaching using HCOOH and H2SO4 increased the caloric value of hydrochar. In contrast, the use of CH3COOH for acid leaching adversely affected the caloric value of hydrochar with decreasing pH.
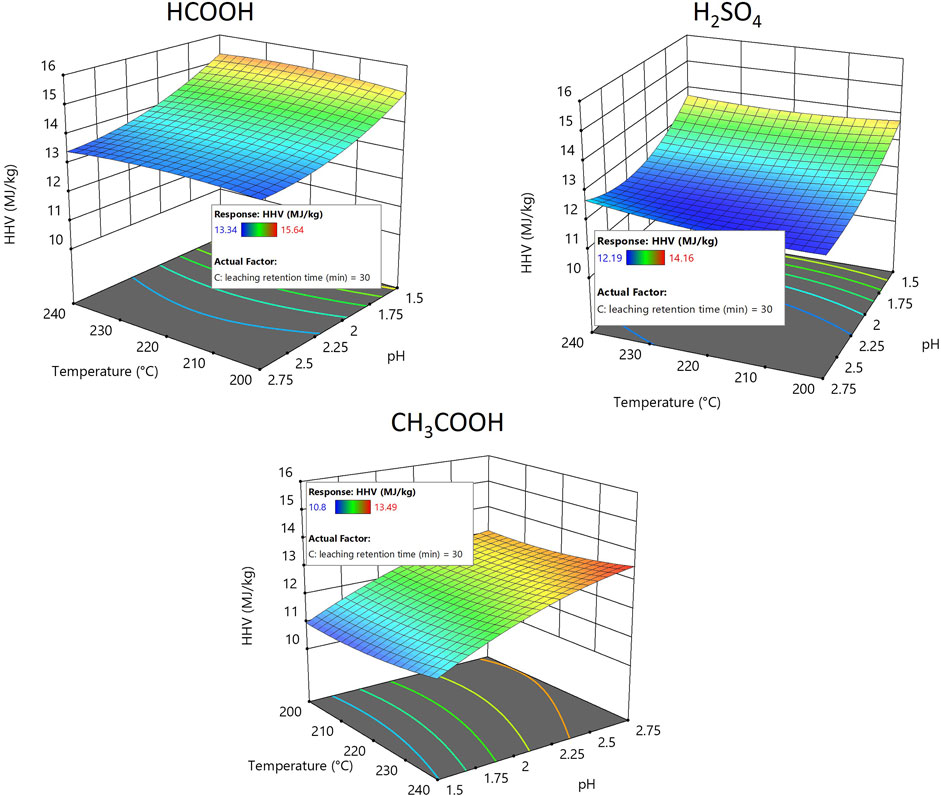
FIGURE 7. Response surface plot showing the influence of acid leaching using H2SO4, HCOOH, and CH3COOH on the caloric value of hydrochar.
Several previous studies showed a strong correlation between the increase in HTC reaction temperature and the caloric value (Danso-Boateng et al., 2015); similar results were found during this research. An increase in reaction temperature increased the caloric value of the produced hydrochar (see Table 1). The heating value of the sewage sludge tends to increase after HTC due to aromatization, polymerization, and condensation reactions that occur during the HTC process (Mazumder et al., 2020).
Numerous studies have demonstrated a significant correlation between FC content and caloric value. FC is the remaining combustible material that is present after the volatile solids in the hydrochar are burnt. The caloric value of hydrochar increases with the increasing FC concentration of hydrochar (Putra et al., 2018; Anshariah and Irvan, 2020; Shettigondahalli Ekanthalu et al., 2021). Before HTC, sewage sludge typically has high VS and low FC content, but a series of reactions that occurs during HTC considerably increases both FC and the caloric value of hydrochar (Putra et al., 2018). The initial analysis and caloric value of selected hydrochar samples obtained after 30 min acid leaching time are shown in Table 4.
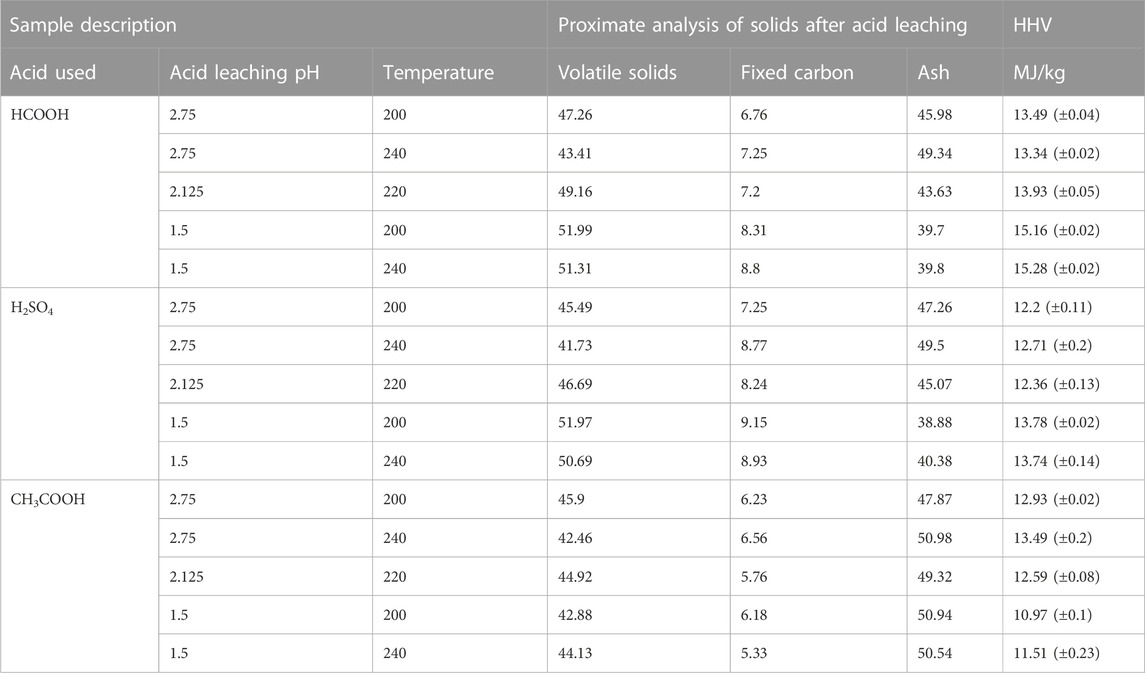
TABLE 4. Proximate analysis and caloric value of hydrochar samples obtained after 30 min acid leaching time.
When H2SO4 was used for acid leaching, the FC value of the hydrochar was found to increase with decreasing pH. Acid leaching the hydrochar using H2SO4 and decreasing the pH from 2.75 to 1.5 increased the FC content of the hydrochar from 7.25% to 9.15%. A similar effect was observed when HCOOH was used for acid leaching: decreasing the pH from 2.75 to 1.5 increased the FC content of the hydrochar from 6.76% to 8.93%. In contrast, when CH3COOH was used, decreasing pH from 2.75 to 1.5 adversely affected the FC content of hydrochar by decreasing it from 6.23% to 5.33% and decreasing the caloric value from 12.93 MJ/kg to 10.97 MJ/kg.
4 Conclusion
The acid leaching of hydrothermally carbonized sewage sludge was studied to understand and compare the effects of independent parameters like carbonization temperature of hydrochar, acid type (H2SO4, HCOOH, and CH3COOH), acid concentration (pH), and acid leaching retention time on the P mobilization, hydrochar properties, acid need, and COD of the leachate. The results of the study indicated that the use of acids with lower pKa values has a significantly greater impact on the solubility and mobility of phosphorus-containing compounds from solids to liquids. TP mobilization from solid to liquid is highly favorable in the presence of H2SO4 (∼90%) at lower pH (1.5) in comparison with HCOOH (∼36%) and CH3COOH (∼2.5%) at similar acid-leaching reaction conditions. It was also observed that a smaller amount of acid (H2SO4) with a lower pKa value is enough to achieve and maintain a lower pH in hydrochar slurry when compared to acids with a higher pKa value (CH3COOH and HCOOH). Regarding the COD of the leachate, the use of organic acid, specifically CH3COOH, tends to induce additional organic acidic compounds, which simultaneously contributes to an increase in COD. Concerning the energy characteristic of hydrochar, the utilization of CH3COOH negatively impacted the FC content of hydrochar while concurrently decreasing its caloric value. In contrast, the use of H2SO4 and HCOOH had positive effects on the FC content and the caloric value of hydrochar. Future research should evaluate and compare the economic effectiveness of using sulfuric acid to leach sewage sludge hydrochar with sewage sludge ash in order to provide an economic viewpoint of P recovery.
Data availability statement
The original contributions presented in the study are included in the article/Supplementary Material; further inquiries can be directed to the corresponding author.
Author contributions
VS: conceptualization, methodology, writing—original draft, formal analysis, validation, visualization, and writing—review and editing. TE: writing—review and editing. SN: supervision. EA: writing—review and editing. SB: writing—review and editing. MN: supervision. All authors contributed to the article and approved the submitted version.
Funding
This publication was funded by the German Research Foundation (DFG) and the Open Access Publication Fund of the University of Rostock.
Acknowledgments
The authors would like to thank Ruth Gebauer, Jan Sprafke, Kersten Eckermann, and Annabell Reiner for their kind assistance during the preparation of this article.
Conflict of interest
The authors declare that the research was conducted in the absence of any commercial or financial relationships that could be construed as a potential conflict of interest.
Publisher’s note
All claims expressed in this article are solely those of the authors and do not necessarily represent those of their affiliated organizations, or those of the publisher, the editors, and the reviewers. Any product that may be evaluated in this article, or claim that may be made by its manufacturer, is not guaranteed or endorsed by the publisher.
Supplementary material
The Supplementary Material for this article can be found online at: https://www.frontiersin.org/articles/10.3389/fenve.2023.1223247/full#supplementary-material
References
AbfKlärV, (2017). Verordnung über die Verwertung von Klärschlamm, Klärschlammgemisch und Klärschlammkompost. Rep. Ger. Sew. sludge Ord.
Akbari, Hamed, Akbari, Hamed, Fanaei, Farzaneh, and Adibzadeh, Amir (2022). Optimization of parameters affecting the hydrothermal carbonization of wastewater treatment plant sewage sludge. Biomass Convers. Biorefinery. doi:10.1007/s13399-022-03427-8
Anshariah, A. M. Imran, Imran, A., Widodo, S., and Irvan, U. R. (2020). Correlation of fixed carbon content and calorific value of South Sulawesi Coal, Indonesia. IOP Conf. Ser. Earth Environ. Sci. 473, 012106. doi:10.1088/1755-1315/473/1/012106
Bora, Akash Pratim, Gupta, Dipanshu Prakash, and Durbha, K. S. (2020). Sewage sludge to bio-fuel: a review on the sustainable approach of transforming sewage waste to alternative fuel. Fuel 259 (73352), 116262. doi:10.1016/j.fuel.2019.116262
Cieślik, Bartłomiej, and Konieczka, Piotr (2017). A review of phosphorus recovery methods at various steps of wastewater treatment and sewage sludge management. The concept of “no solid waste generation” and analytical methods. J. Clean. Prod. 142 (4), 1728–1740. doi:10.1016/j.jclepro.2016.11.116
Dai, Lichun, Yang, Bo, Li, H., Tan, Furong, Zhu, Nengmin, Zhu, Qili, et al. (2017). A synergistic combination of nutrient reclamation from manure and resultant hydrochar upgradation by acid-supported hydrothermal carbonization. Bioresour. Technol. 243, 860–866. doi:10.1016/j.biortech.2017.07.016
Danso-Boateng, E., Shama, G., Wheatley, A., Martin, S., and Holdich, R. G. (2015). Hydrothermal carbonisation of sewage sludge: effect of process conditions on product characteristics and methane production. Bioresour. Technol. 177, 318–327. doi:10.1016/j.biortech.2014.11.096
Deutsches Institut für Normung (2012). Sludge, treated biowaste and soil—determination of pH (EN 15933:2012). Berlin: Deutsches Institut für Normung.
Domini, M., Bertanza, G., Vahidzadeh, R., and Pedrazzani., R. (2022). Sewage sludge quality and management for circular economy opportunities in lombardy. Appl. Sci. 12, 10391. doi:10.3390/app122010391
Ekpo, U., Ross, A. B., Camargo-Valero, M. A., and Fletcher, L. A. (2016). Influence of pH on hydrothermal treatment of swine manure: impact on extraction of nitrogen and phosphorus in process water. Bioresour. Technol. 214, 637–644. doi:10.1016/j.biortech.2016.05.012
En 14918, (2010). Solid biofuels - determination of calorific value. Geneva, Switzerland: International Organization for Standardization.
En Iso 11885, (2009). Determination of selected elements by inductively coupled plasma optical emission spectrometry (ICP-OES). Geneva, Switzerland: International Organization for Standardization.
En Iso 16948, (2015). Solid biofuels — determination of total content of carbon, hydrogen and nitrogen. Geneva, Switzerland: International Organization for Standardization.
Guo, Shuai, Xu, DanDan, Guo, Xin, Li, Xingcan, and Zhao, Chenchen (2021). Hydrothermal carbonization of sewage sludge: multi-response optimization of hydrochar production and CO2-assisted gasication performance. Res. Square. doi:10.21203/rs.3.rs-954940/v1
He, Chao, Giannis, Apostolos, and Wang, Jing-Yuan (2013). Conversion of sewage sludge to clean solid fuel using hydrothermal carbonization: hydrochar fuel characteristics and combustion behavior. Appl. Energy 111, 257–266. doi:10.1016/j.apenergy.2013.04.084
Heilmann, Steven M., Molde, J. S., Timler, J. G., Wood, B. M., Mikula, A. L., Vozhdayev, G. V., et al. (2014). Phosphorus reclamation through hydrothermal carbonization of animal manures. Environ. Sci. Technol. 48 (17), 10323–10329. doi:10.1021/es501872k
Helmenstine, Anne Marie (2020). pKa definition in chemistry. https://www.thoughtco.com/what-is-pka-in-chemistry-605521 (Accessed April 17, 2023).
Huang, Rixiang, Fang, Ci, Zhang, Bei, and Tang, Yuanzhi (2018). Transformations of phosphorus speciation during (Hydro)thermal treatments of animal manures. Environ. Sci. Technol. 52 (5), 3016–3026. doi:10.1021/acs.est.7b05203
Jellali, S., Zorpas, A. A., Alhashmi, S., and Jeguirim, M. (2022). Recent advances in hydrothermal carbonization of sewage sludge. Energies 15 (18), 6714. doi:10.3390/en15186714
Liang, Sha, Chen, Haoming, Zeng, Xiaohui, Li, Zhibin, Yu, Wenbo, Xiao, Keke, et al. (2019). A comparison between sulfuric acid and oxalic acid leaching with subsequent purification and precipitation for phosphorus recovery from sewage sludge incineration ash. Water Res. 159, 242–251. doi:10.1016/j.watres.2019.05.022
Liu, Xiangmin, Zhai, Yunbo, Li, Shanhong, Wang, Bei, Wang, Tengfei, Liu, Yali, et al. (2020). Hydrothermal carbonization of sewage sludge: effect of feed-water ph on hydrochar’s physicochemical properties, organic component and thermal behavior. J. Hazard. Mater. 388, 122084. doi:10.1016/j.jhazmat.2020.122084
Lühmann, Taina, and Wirth, Benjamin (2020). Sewage sludge valorization via hydrothermal carbonization: optimizing dewaterability and phosphorus release. Energies 13 (17), 4417. doi:10.3390/en13174417
Mäkelä, M., Benavente, V., and Fullana, A. (2015). Hydrothermal carbonization of lignocellulosic biomass: effect of process conditions on hydrochar properties. Appl. Energy 155, 576–584. doi:10.1016/j.apenergy.2015.06.022
Mäkelä, Mikko, Benavente, Verónica, and Fullana, Andrés (2016). Hydrothermal carbonization of industrial mixed sludge from a pulp and paper mill. Bioresour. Technol. 200, 444–450. doi:10.1016/j.biortech.2015.10.062
Mazumder, Shanta, Saha, Pretom, and Toufiq Reza, M. (2020). Co-Hydrothermal carbonization of coal waste and food waste: fuel characteristics. Biomass Conversion and Biorefinery. Springer, Berlin, Germany, doi:10.1007/s13399-020-00771-5
Merzari, F., Goldfarb, J., Andreottola, G., Mimmo, T., Volpe, M., and Fiori, L. (2020). Hydrothermal carbonization as a strategy for sewage sludge management: influence of process withdrawal point on hydrochar properties. Energies 13 (11), 2890. doi:10.3390/en13112890
Montgomery, Douglas C. (2013). Design and analysis of experiments. Singapore: John Wiley & Sons Inc.
Ovsyannikova, Ekaterina, Arauzo, Pablo J., Becker, Gero С., and Kruse, Andrea (2019). Experimental and thermodynamic studies of phosphate behavior during the hydrothermal carbonization of sewage sludge. Sci. Total Environ. 692, 147–156. doi:10.1016/j.scitotenv.2019.07.217
Pérez, Carla, Boily, Jean-François, Skoglund, Nils, Jansson, Stina, and Fick, Jerker (2022). Phosphorus release from hydrothermally carbonized digested sewage sludge using organic acids. Waste Manag. 151, 60–69. doi:10.1016/j.wasman.2022.07.023
Peng, Chuan, Zhai, Yunbo, Zhu, Y. U. N., Xu, Bibo, Wang, Tengfei, Li, Caiting, et al. (2016). Production of char from sewage sludge employing hydrothermal carbonization: char properties, combustion behavior and thermal characteristics. Fuel 176, 110–118. doi:10.1016/j.fuel.2016.02.068
Pérez, Carla, Boily, Jean-François, Jansson, Stina, Gustafsson, Tomas, and Fick, Jerker (2021). Acid-induced phosphorus release from hydrothermally carbonized sewage sludge. Waste Biomass Valorization 12, 6555–6568. doi:10.1007/s12649-021-01463-5
Petzet, Sebastian, Peplinski, Burkhard, and Cornel, Peter (2012). On wet chemical phosphorus recovery from sewage sludge ash by acidic or alkaline leaching and an optimized combination of both. Water Res. 46 (12), 3769–3780. doi:10.1016/j.watres.2012.03.068
Poirier, Yves, Jaskolowski, A., and Clúa, Joaquín (2022). Phosphate acquisition and metabolism in plants. Curr. Biol. 32, 623–629. doi:10.1016/j.cub.2022.03.073
Putra, Herlian Eriska, Damanhuri, Enri, and Dewi, KaniaAri Darmawan Pasek (April 2018). Hydrothermal carbonization of biomass waste under low temperature condition. Proceedings of the 2nd International Conference on Engineering and Technology for Sustainable Development. Samara, Russia, doi:10.1051/matecconf/201815401025
Raheem, A., Sikarwar, V. S., He, J., Dastyar, W., Dionysiou, D. D., Wang, W., et al. (2018). Opportunities and challenges in sustainable treatment and resource reuse of sewage sludge: a review. Chem. Eng. J. 337, 616–641. doi:10.1016/j.cej.2017.12.149
Reza, M. Toufiq, Rottler, Erwin, Herklotz, Laureen, and Wirth, Benjamin (2015). Hydrothermal carbonization (HTC) of wheat straw: influence of feedwater pH prepared by acetic acid and potassium hydroxide. Bioresour. Technol. 182, 336–344. doi:10.1016/j.biortech.2015.02.024
Román, S., Libra, J., Berge, N., Sabio, E., Ro, K., Li, L., et al. (2018). Hydrothermal carbonization: modeling, final properties design and applications: a review. Energies 11, 216. doi:10.3390/en11010216
Roskosch, Andrea, and Heidecke, Patric (2018). Sewage sludge disposal in the Federal Republic of Germany. Dessau-Roßlau, Germany: German Environment Agency.
Shettigondahalli Ekanthalu, V., Morscheck, Gert, Narra, S., and Nelles, Michael (2020). Hydrothermal carbonization—a sustainable approach to deal with the challenges in sewage sludge management. Urban Min. Sustain. Waste Manag. 293, 293–302. doi:10.1007/978-981-15-0532-4_29
Shettigondahalli Ekanthalu, V., Narra, S., Ender, Tommy, Antwi, E., and Nelles, M. (2022). Influence of post- and pre-acid treatment during hydrothermal carbonization of sewage sludge on P-transformation and the characteristics of hydrochar. Processes 10 (151), 151. doi:10.3390/pr10010151
Shettigondahalli Ekanthalu, V., Narra, S., Sprafke, J., and Nelles, M. (2021). Influence of acids and alkali as additives on hydrothermally treating sewage sludge: effect on phosphorus recovery, yield, and energy value of hydrochar. Processes 9 (4), 618. doi:10.3390/pr9040618
Shi, Yan, Luo, Gang, Rao, Y., Chen, Huihui, and Zhang, Shicheng (2019). Hydrothermal conversion of dewatered sewage sludge: focusing on the transformation mechanism and recovery of phosphorus. Chemosphere 228, 619–628. doi:10.1016/j.chemosphere.2019.04.109
Stat-Ease, (2022). Adequate precision - tutorial. Minneapolis, Minnesota , United States: Stat-Ease, Inc.
Stat-Ease, Inc, (2022). Design-expert V22.0, tutorials. https://www.statease.com/docs/latest/tutorials/(Accessed June 03, 2023).
Statistisches Bundesamt Destatis, (2022). Abwasserbehandlung – klärschlamm. https://www-genesis.destatis.de/genesis/online?operation=ergebnistabelleUmfang&levelindex=2&levelid=1682687234807&downloadname=32214-0001-DLAND#abreadcrumb (Accessed April 28, 2023).
Stumm, Werner, and Morgan, James J. (1981). Aquatic chemistry - an introduction emphasizing chemical equilibria in natural water. Hoboken, New Jersey, United States: Wiley.
UBC Sustainable Cities Commission (2017). Enhanced nutrient removal with bio-filter at Rostock WWTP. https://www.balticwaterhub.net/solutions/bio-filter-wwtp-rostock.
Wang, Liping, Chang, Yuzhi, and Li, Aimin (2019). Hydrothermal carbonization for energy-efficient processing of sewage sludge: a review. Renew. Sustain. Energy Rev. 108, 423–440. doi:10.1016/j.rser.2019.04.011
Wang, Tao, Zhai, Yunbo, Zhu, Yun, Peng, C., Wang, Tengfei, Xu, Bibo, et al. (2017). Feedwater pH affects phosphorus transformation during hydrothermal carbonization of sewage sludge. Bioresour. Technol. 245, 182–187. doi:10.1016/j.biortech.2017.08.114
Werther, J., and Ogada, T. (1999). Sewage sludge combustion. Prog. Energy Combust. Sci. 25 (1), 55–116. doi:10.1016/S0360-1285(98)00020-3
Woriescheck, Tim (2019). PhD thesis. Oldenburg, Germany: Institut für Chemie, Carl von Ossietzky Universität Oldenburg.Charakterisierung, Aufreinigung und Wertstoffgewinnung von Prozesswasser der Hydrothermalen Carbonisierung
Zheng, Xiaoyuan, Jiang, Zhengwei, Ying, Zhi, Song, Jiaxing, Chen, Wei, and Wang, Bo (2020a). Role of feedstock properties and hydrothermal carbonization conditions on fuel properties of sewage sludge-derived hydrochar using multiple linear regression technique. Fuel 271, 117609. doi:10.1016/j.fuel.2020.117609
Zheng, Xiaoyuan, Jiang, Zhengwei, Ying, Zhi, Ye, Yutong, Chen, Wei, Wang, Bo, et al. (2020b). Migration and transformation of phosphorus during hydrothermal carbonization of sewage sludge: focusing on the role of pH and calcium additive and the transformation mechanism. ACS Sustain. Chem. Eng. 8 (21), 7806–7814. doi:10.1021/acssuschemeng.0c00031
Keywords: hydrothermal carbonization, response surface model, design of experiments, regression model, sewage sludge treatment, phosphorus recovery, pKa, organic and inorganic acids
Citation: Shettigondahalli Ekanthalu V, Ender T, Narra S, Antwi E, Bej S and Nelles M (2023) Acid leaching of hydrothermally carbonized sewage sludge: phosphorus recovery and hydrochar characteristics. Front. Environ. Eng. 2:1223247. doi: 10.3389/fenve.2023.1223247
Received: 15 May 2023; Accepted: 01 August 2023;
Published: 23 August 2023.
Edited by:
Christian Kennes, University of A Coruña, SpainReviewed by:
Przemysław Seruga, Wroclaw University of Economics, PolandYuanzhi Tang, Georgia Institute of Technology, United States
Copyright © 2023 Shettigondahalli Ekanthalu, Ender, Narra, Antwi, Bej and Nelles. This is an open-access article distributed under the terms of the Creative Commons Attribution License (CC BY). The use, distribution or reproduction in other forums is permitted, provided the original author(s) and the copyright owner(s) are credited and that the original publication in this journal is cited, in accordance with accepted academic practice. No use, distribution or reproduction is permitted which does not comply with these terms.
*Correspondence: Vicky Shettigondahalli Ekanthalu, vicky.ekanthalu@uni-rostock.de
†Present address: Edward Antwi, Independent Researcher, Schmarl, Germany