- 1 INSERM U636, Nice, France
- 2 Laboratoire de Génétique du Développement Normal et Pathologique, Université de Nice-Sophia Antipolis, Nice, France
Part of the heterodimeric P-TEF-b element of the Pol II transcription machinery, the cyclin-dependent kinase 9 plays a critical role in gene expression. Phosphorylation of several residues in the polymerase is required for elongation of transcript. It determines the rates of transcription and thus, plays a critical role in several differentiation pathways, best documented in heart development. The synthesis and activity of the protein are tightly regulated in a coordinated manner by at least three non-coding RNAs. First, its kinase activity is reversibly inhibited by formation of a complex with the 334 nt 7SK RNA, from which it is released under conditions of stress. Then, heart development requires a maximal rate of synthesis during cardiomyocyte differentiation, followed by a decrease in the differentiated state. The latter is insured by microRNA-mediated translational inhibition. In a third mode of RNA control, increased levels of transcription are induced by small non-coding RNA molecules with sequences homologous to the transcript. Designated paramutation, this epigenetic variation, stable during development, and hereditarily transmitted in a non-Mendelian manner over several generations, is thought to be a response to the inactivation of one of the two alleles by an abnormal recombination event such as insertion of a transposon.
Introduction
Understanding the controls of gene expression by non-coding RNAs, the major part of the “dark matter” of the genome (Kapranov et al., 2010; Mattick et al., 2010), is becoming a major goal in developmental biology, physiology, and pathology. Several mechanisms of such control have been uncovered – and the list is certainly not complete. Our current work is focused on a network involving at least three distinct small non-coding (snc) RNAs, which work synergistically to adjust the levels of synthesis and activity of a pleiotropic protein, the cyclin-dependent kinase 9 (Cdk9), part of the heterodimeric complex designated P-TEFb. Its serine–threonine kinase activity plays an essential role in RNA polymerase II transcription and is currently recognized as a critical actor in a number of physiological and pathological processes. It is therefore understandable that both synthesis and activity of Cdk9 are tightly regulated. The control of its translation, critical in heart differentiation, is exerted by several microRNAs, primarily miR-1 and miR-133. Its enzymatic activity is independently regulated by formation of a complex with a 332 nt non-coding RNA, 7SK, which promotes the reversible integration of protein inhibitors in P-TEFb. A third avenue of regulation is via activation of transcription by sequence-related oligoribonucleotides that we reported for Cdk9 and two other genes (Rassoulzadegan et al., 2006; Wagner et al., 2008; Grandjean et al., 2009).
Cdk9, a Protein at a Nodal Point in Development and Differentiation
The Cdk9 protein is associated with a cyclin from either the T or the K families in the heterodimer P-TEFb, which plays a key role in the Polymerase II transcription machinery (Kohoutek, 2009). The Cdk9 component functions as the catalytic domain, while the cyclin constitutes the regulatory subunit (Malumbres and Barbacid, 2005). P-TEFb is required after binding of the initiation complex to a promoter – the phosphorylation of a C-terminal domain of the polymerase by P-TEFb allows elongation of the nascent transcript. Levels of transcription are therefore determined by the recruitment of P-TEFb to promoters, in turn dependent on its interactions with other proteins such as the bromodomain protein Brd4 (Yang et al., 2005). Cdk9 is also part of the p300/GATA4 complex required for the expression of cardiac specific genes such as Nkx2.5, Anf, and ß-Myh (Kaichi et al., 2011 and references therein). P-TEFb interacts with Myosin skeletal muscle differentiation (Simone et al., 2002) and interactions were documented with a plethora of transcription factors (Kohoutek, 2009). Phosphorylation of substrates other than RNA Pol II are also likely to be important, for instance that of several sites in p53 (Radhakrishnan and Gartel, 2006). A role in the activation of quiescent B cells was reported (De Falco et al., 2008), and a general function has been proposed in transcription coupled alternative splicing (Barboric et al., 2009). While the P-TEFb complex as a whole is a functional kinase unit, its activity appears to be essentially modulated by variations of the synthesis and/or activity of the Cdk9 moiety. Several RNA-mediated regulatory circuits have been identified. Our knowledge is still, however, incomplete, as illustrated by the paucity of data on the differential expression of the two isoforms encoded at the Cdk9 locus and on their respective functions. In addition to the ubiquitous 42 kDa protein, a 55-kDa species transcribed from a distinct upstream promoter includes an additional N-terminal sequence of 117 aminoacids (Giacinti et al., 2008). Differential expression of the two proteins has been reported in various cell types and conditions (reviewed in Romano and Giordano, 2008). We observed that the longer isoform, initially not expressed in ES cells, is transcribed in the course of their differentiation into beating cardiac cells (Hossein Ghanbarian et al., unpublished).
Variations in Cdk9 expression, hence on P-TEFb activity, are associated with various diseases. Among them, cardiac hypertrophy was the most extensively documented. Cardiomyocyte differentiation normally involves an increase in cell size that requires a general activation of transcription together with that of the heart-specific genes. The kinase is required in normal differentiation and its overexpression results in cardiac hypertrophy (Sano et al., 2002; Wagner et al., 2008). Additional pathological developments are associated with abnormal Cdk9 expression (Kohoutek, 2009). One well-documented instance is viral immunodeficiency. A complex of Cdk9 with the Tat proteins of HIV1 and HIV2 viruses enhances their interaction with Tar RNA and thus, the transcription of the viral genome (Wei et al., 1998). In human gastric tumors (He et al., 2008), high levels of P-TEFb activity are generated by a mutation deleting part of LARP7, a protein that participates in inhibition of Cdk9 in the complex with 7SK RNA (see below). In addition to the immunodeficiency family, other viruses of clinical interest are dependent on the Cdk9 kinase, which therefore appears as a likely target for the development of new pharmacological strategies (reviewed by Romano and Giordano, 2008; Wang and Fischer, 2008).
Multiple Regulations by Small Non-Coding RNAs
Given its essential functions, it is not unexpected that both the synthesis and activity of the protein are under tight regulatory controls. Three distinct circuits enacted by sncRNAs illustrate their power and versatility. We will briefly review the first one, translation control by microRNAs for which excellent reviews are available (Kohoutek, 2009; Sayed and Abdellatif, 2011), and then the reversible inhibition of enzymatic activity in the 7SK complex. We will discuss in more detail the properties and possible function of the inherited epigenetic increase in Cdk9 transcription in the “Cdk9* paramutants” (Wagner et al., 2008).
Down Regulation by MicroRNA: The Case of MiR-1
Two of the microRNAs detected in heart (Callis and Wang, 2008) have been the subject of intensive studies, miR-1 and miR-133. miR-1, most important in heart development, targets the 3′ untranslated region of the Cdk9 transcript. In undifferentiated ES cells, miR-1 is either absent or barely detectable. When cardiac differentiation is induced by culture in suspension (Boheler et al., 2002), expression progressively increases. Ectopic expression in the initial ES cultures inhibits differentiation while reducing expression of Cdk9 (Takaya et al., 2009). Forced expression of miR-1 in embryonic cardiomyocytes inhibits their proliferation and increases differentiation (Zhao et al., 2005). Down regulation of Cdk9 is clearly critical to keep cardiac growth within the physiological limits, and the microRNA also targets Hand2 mRNA, which encodes a transcription factor required for ventricular myocyte expansion. miR-1 and miR-133 play opposite roles in cardiomyocyte growth and apoptosis. Unlike miR-1, which is apoptotic and targets the anti-apoptotic heat shock proteins HSP60 and HSP70, miR-133 is anti-apoptotic and represses caspase-9, a regulator of mitochondria-mediated apoptosis (Xu et al., 2007). It is expected that more miRNAs will be added to the growing list of cardiac regulators (Callis and Wang, 2008).
Regulation of P-TEFb Enzymatic Activity: The 7SK Complex
A fraction of the Cdk9 protein is stored in a reversibly inactivated form. Inhibition is achieved in a complex with the evolutionary conserved 7SK sncRNA (Nguyen et al., 2001; Yang et al., 2001). 7SK bridges the protein to either one of two proteins, Hexim1 and Hexim2, which inhibit the kinase activity (Yik et al., 2003; Byers et al., 2005). In addition, two other proteins, MePCE and LARP7 are required for the stabilization of the complex by inhibiting degradation of the 7SK RNA (Krueger et al., 2008). Complex formation is fully reversible: its dissociation results in a burst of Cdk9 activity under conditions of stress, UV irradiation, mechanical load, and pharmacological treatments by “hypertrophic agonists” such as endothelin-1, phenylephrine, calcineurin (Nguyen et al., 2001; Sano et al., 2002; Espinoza-Derout et al., 2009). As one additional enzyme was found associated with 7SK complexes (He et al., 2006), one may consider a more general type of regulation by the means of readily reversible inhibition of enzyme activity in complexes with inhibitory proteins mediated by non-coding RNAs.
Long Term Transcriptional Activation by Homologous Oligoribonucleotides and microRNAs
A third mode of regulation of Cdk9 expression directed by sncRNAs is epigenetic transcriptional activation during heart development. It results in a dramatic heart hypertrophy syndrome stably inherited over several generations (Wagner et al., 2008), designated “paramutation” by analogy with the phenomenon of plant hereditary epigenetic variations (Chandler, 2007). We previously reported a heritable epigenetic modification of the Kit gene induced by microinjecting mouse fertilized eggs with oligoribonucleotides with two types of sequences: (1) corresponding to short stretches of the Kit transcript and (2) corresponding to certain endogenous microRNAs (Rassoulzadegan et al., 2006). In a quest for other examples, we practiced a series of injections of other microRNAs and transcript fragments, among them oligoribonucleotides with a sequence from Cdk9 mRNA and the miR-1 microRNA. Their injection into one-cell embryos followed by reimplantation in foster mothers resulted in increased expression of Cdk9 in cardiomyocyte precursors at the E18.5 embryonic stage (Wagner et al., 2008).
All the treated embryos developed into newborns and adults showed increased heart size: 1.5- to 2-fold that of the controls, with a disrupted organization of the wall ultrastructure and TUNEL-positive cells. All these features are characteristic of the human hypertrophic cardiomyopathy, a severe condition with a poor prognosis and, remarkably, a frequent familial distribution. In the mouse, the cardiac phenotype was efficiently transmitted to the offspring in three generations of crosses with normal partners. Increased P-TEFb activity evidenced by Pol II phosphorylation resulted from elevated levels of Cdk9 mRNA resulting from increased transcriptional activity. Levels of the Cyclin T1 and 7SK RNAs remained unchanged.
Two remarkable features of the epigenetic variation are worth noting. First, the Cdk9 gene remains subject to the same developmental regulations as in the wild type controls. Overexpression, as seen in the cardiomyocyte precursors (E18.5) and normal development, affects only the level of the short isoform exclusively made in the wild type. The other remarkable feature, common to the different instances of paramutation so far analyzed, is the mode of inheritance described for other epigenetic variations as “rheostat transmission” (Beaudet and Jiang, 2002). The quantitative phenotypic modulation, in this case heart size, appears to be set separately for every individual at some early time in development. In other words, mice with large hearts will generate in the same litter animals with very large, large, more than average, and nearly normal heart sizes. Conversely, mice with nearly normal or normal hearts will generate the same assortment of phenotypes, including the very large ones (Wagner et al., 2008). This pattern of phenotypic distribution is more evocative of a continuous modulation such as that of a rheostat than of the on–off “switch” of most genetic controls.
Long Term Activation of Cdk9 in Embryonic Stem Cells
With the aim to limit the complexity of the experimental approach, we tested whether the same oligoribonucleotides would activate transcription of Cdk9 in embryonic stem cells in culture (ES lines) and affect their differentiation into cardiac precursors (Hossein Ghanbarian and Minoo Rassoulzadegan, manuscript in preparation). In order to be able to compare the cell culture and in vivo results, we made use of a mouse ES cell line that we have previously tested for being able to successfully incorporate in the embryo and give rise to whole spectrum of differentiated cell types. The first question was whether transcription of the genes paramutated in the mouse would be increased to the same extent upon RNA transfer by electroporation in ES cells and this turned out to be the case. We were then able to compare these results with the other instance of transcriptional activation by homologous RNAs, recently reported in human cell lines in culture (Place et al., 2008; Morris, 2009; Huang et al., 2010). A critical question was the stability of the activated state. Unlike the long term effects generated in the mouse, the activated state in human cells was maintained for only a limited number of cell doublings. Results in the ES cells show a 1.5- to 2-fold activation of Cdk9 transcription within the first days in the transfected culture. Cotransfer of a selectable marker allowed us to isolate clones maintaining elevated (1.5- to 3-fold) transcription rates, eventually reverting to the wild type level, but only after their propagation in culture for 15–20 cell generations. The field of RNA paramutation will thus now benefit from the availability of a cell culture system to hopefully reach a mechanistic explanation of RNA induction, an objective that was obviously more difficult to attain with the limited amounts of material offered by early mouse embryos. Several lines of research are now started on the cell culture system. One of them is a point by point comparison with the short term induction of transcription described in human cells. The two studies involved different genes and the possible induction of Kit in human cells, for instance, or paramutation of Cdh1 (E-cadherin), inducible in the mouse (Huang et al., 2010), would allow more significant comparisons. An important mechanistic aspect is that in human cells, induction requires the Argonaute system and mouse cells can now be tested in this respect. One most intriguing aspect is the fact that, in ES cells as in the mouse, oligoribonucleotides with fragments of the sense sequence start the efficient and highly specific paramutation process. Recognition by base pairing would be the most obvious explanation, leading to the assumption that natural antisense transcripts are present in the cell. This class of non-coding RNAs has been identified for various loci and their regulatory roles is currently a matter of attention in several laboratories (reviewed by Werner and Swan, 2010).
The modified ES clones showed another property of interest (Hossein Ghanbarian and Minoo Rassoulzadegan, manuscript in preparation). The cells do not appear as even partially differentiated. They express characteristic ES cell markers such as Nanog (Silva et al., 2009) and none of the genes characteristic of cardiomyocytes. Still, the epigenetic variation had conferred on the cells the capacity to differentiate faster and more efficiently into the cardiac lineage. This was clearly ascertained when cardiac differentiation was induced in the cultures (Boheler et al., 2002). Briefly, cells are first grown in suspended aggregates, designated embryoid bodies (“EB”), then EBs separately replated as individual small cultures. Over the next days, cardiac marker genes are expressed with a defined time course, until eventually the appearance of rhythmically beating cardiac cells. They appeared at a much earlier time and at higher frequencies in cultures of the Cdk9 paramutant cells than in the control and the same was true of the expression of cardiac markers such as the cardiac myosin genes. Interestingly expression of Cdk9 in the differentiated cardiomyocytes had returned to the value of the controls, as in the adult heart of the paramutant mice (Wagner et al., 2008). Directing differentiation of pluripotent stem cells to a differentiated state will be critical for their intended use in therapeutic and regenerative medicine. If generalized to other differentiation states, paramutation might offer one possible approach to this goal. A similar result, based on a different RNA-based technology, has been recently reported (Warren et al., 2010).
Distinct Regulations for Different Physiological Situations
An overall picture of Cdk9 regulation by sncRNAs, albeit still incomplete and requiring further refinement, is emerging from these results. Under different physiological conditions, expression levels are set by distinct RNA-mediated regulatory pathways summarized in Figure 1. During cardiac differentiation, involving both cell growth and cell division, a general activation of transcription is achieved only for a limited period, with continuing cell division leading to overgrowth and eventually to hypertrophy. Co-induction of miR-1 and other cardiac markers promotes a progressive decline in Cdk9 synthesis. This is clearly only part of the story and future work will surely make this simple scheme more complex. The additional angle of RNA-mediated regulation is to make the storage of the protein in a reversibly inactivated state respond to a distinct requirement of the system, namely to provide an immediate burst of active enzyme under stress conditions.
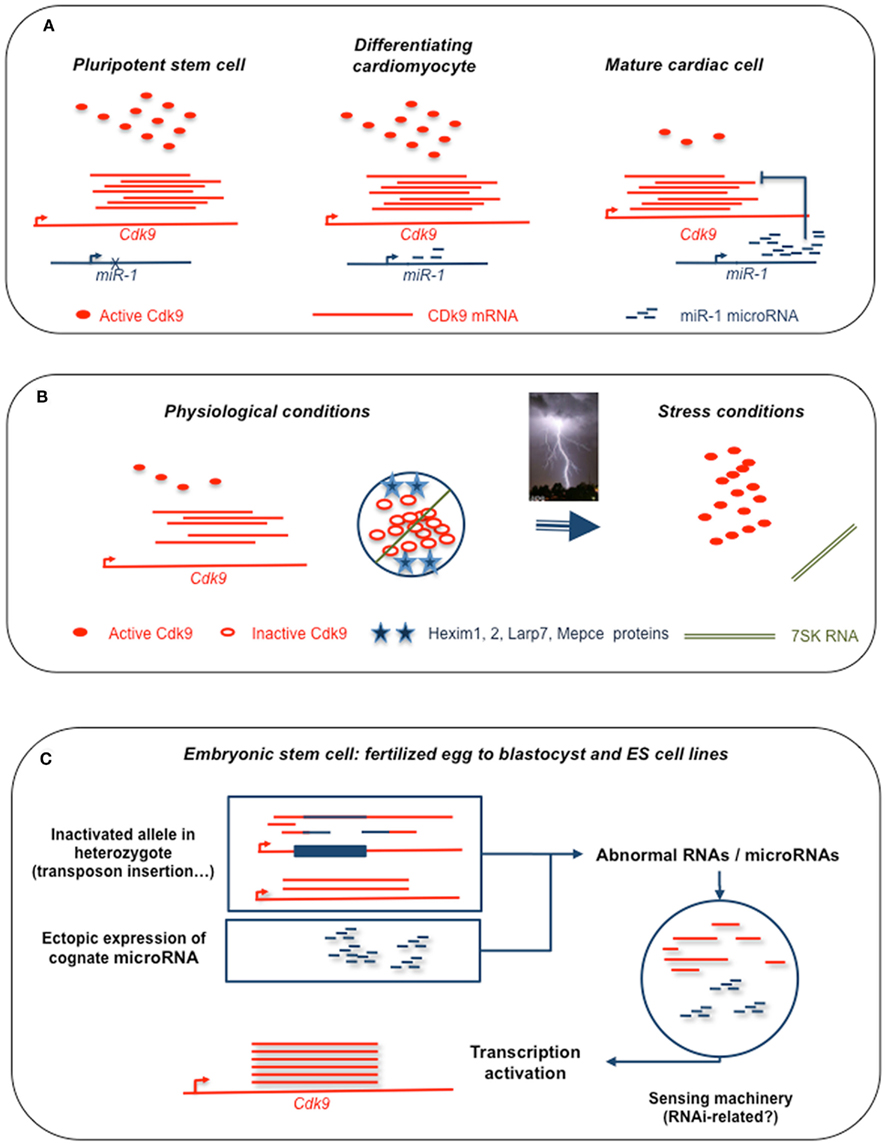
Figure 1. Schematic representation of sncRNA-mediated regulations of Cdk9 expression. (A) Down regulation by microRNA miR-1 of Cdk9 translation in differentiated cardiomyocytes following the high levels of expression required during the differentiation process. (B) Storage of inactive protein in a complex with 7SK RNA and inhibitory proteins, release of active kinase in stress conditions. (C) Summary of our current observations on the initiation of paramutation. Observed either in heterozygote genotypes with one allele disrupted by insertion as in the case of Kit* paramutants (Rassoulzadegan et al., 2006) or upon accumulation of a cognate microRNA, miR-1 in the case of Cdk9. Abnormal RNAs have to be detected by a sensing system in the embryonic cell. Suggestion that the sensor involves the RNAi machinery is based on our current data showing a requirement for Argonaut proteins. Transcription is then upregulated, possibly by a mechanism related to the RNA activation process reported in human cells (Place et al., 2008; Morris, 2009; Huang et al., 2010). As shown for miR-124 and the Sox9* paramutation (Grandjean et al., 2009), heritability is explained by the transfer of the inducing RNAs by oocyte and sperm (not shown).
Then, the possible “raison d’être” of the long term, hereditary form of regulation of gene expression induced by short RNAs with fragments of the sense sequence can only be at this stage a matter of speculation. Coming back to the initial description of the paramutation of the Kit gene (Rassoulzadegan et al., 2006), it is important to note that the modification was detected in the progeny of heterozygotes carrying a gene disrupted by exogenous sequences. Increased expression of the intact Kit+ allele in germ cells was stably maintained during development and inherited in serial crosses. Abnormal transcript fragments generated from the disrupted allele appeared as the likely inducers of the epigenetic change that led to the change in gene expression. Induction by the cognate miRNAs (miR-221 and -222 for Kit, miR-1 for Cdk9, miR-124 for Sox9) may result from the induction by the microRNAs of degradation of the transcript into the inducing RNA fragments in cells in which the miRNA is not normally present. Alternatively, the ectopically expressed microRNAs may be acting as inducers by themselves. Triggered by abnormal forms of the transcript, paramutation of the wild type locus may be, in teleonomic terms and under natural circumstances, a way to compensate for the inactivation of the other allele by a non-homologous recombination event such as insertion of a transposon.
One important question is whether paramutation could be considered as a general property of a mouse gene – or its establishment a general property of a miRNA. In addition to the consideration that in this case, it should have been recognized a long time ago, several observations clearly point to a negative answer. No clear phenotype was generated in our hands by a series of oligoribonucleotides tested in the microinjection assay and/or in transfected ES cells. It is also significant in this respect that miR-1 efficiently induced, both in the mouse and in ES cells, the long term overexpression of Cdk9, but not of Hand2, a recognized target of the microRNA (Wagner et al., 2008). One may then have to consider the process as characteristic of a subset of genes. It may be noteworthy that all three genes for which paramutation events have been detected, Kit, Cdk9, and Sox9, are critical for mouse development to the point that homozygous negative mutants are embryonic lethals. On a purely speculative basis, we would thus be tempted to consider that we are dealing with a rescue mechanism, offered to a limited number of loci, that compensates the defective state of one locus by increasing the expression of the allelic wild type gene.
Finally, one may wonder whether the biological processes underlying the observations of “paramutation” are identical in plants and in the mouse. Strictly speaking, they both follow Chandler’s definition as “RNA-mediated instructions passed across generations” (Chandler, 2007). The two processes appear, however, distinct in their most basic aspects – as distinct as mouse is from maize. Primarily, the plant paramutation phenomenon is a silencing process and the current thinking in the field considers RNA inducers, whose role was deduced from the requirements for plant-specific RNA polymerases, as guides for a DNA methylation process (Simon and Meyers, 2011). In the mouse, as discussed in the above sections, we and others reported, to the contrary, a transcriptional activation induced by sequence-related small RNAs – a clearly distinct process with, at the present time, no established relationship to DNA methylation (our unpublished data). Thus, a case can be made that a possible confusion is introduced by the use of the same word, “paramutation,” to describe the two phenomena, a confusion for which we are ourselves responsible for a significant part. It remains however that they have in common is the notion of that heredity may not be circumscribed to the strict domain of Mendelism, with possible important implications in multiple biological fields including pathology, developmental, and evolutionary biology.
Conflict of Interest Statement
The authors declare that the research was conducted in the absence of any commercial or financial relationships that could be construed as a potential conflict of interest.
References
Barboric, M., Lenasi, T., Chen, H., Johansen, E. B., Guo, S., and Peterlin, B. M. (2009). 7SK snRNP/P-TEFb couples transcription elongation with alternative splicing and is essential for vertebrate development. Proc. Natl. Acad. Sci. U.S.A. 106, 7798–7803.
Beaudet, A. L., and Jiang, Y. H. (2002). A rheostat model for a rapid and reversible form of imprinting-dependent evolution. Am. J. Hum. Genet. 70, 1389–1397.
Boheler, K. R., Czyz, J., Tweedie, D., Yang, H. T., Anisimov, S. V., and Wobus, A. M. (2002). Differentiation of pluripotent embryonic stem cells into cardiomyocytes. Circ. Res. 91, 189–201.
Byers, S. A., Price, J. P., Cooper, J. J., Li, Q., and Price, D. H. (2005). HEXIM2, a HEXIM1-related protein, regulates positive transcription elongation factor b through association with 7SK. J. Biol. Chem. 280, 16360–16367.
Chandler, V. L. (2007). Paramutation: RNA-mediated instructions passed across generations. Cell 23, 641–645.
De Falco, G., Leucci, E., Onnis, A., Bellan, C., Tigli, C., Wirths, S., Cerino, G., Cocco, M., Crupi, D., De Luca, A., Lanzavecchia, A., Tosi, P., Leoncini, L., and Giordano, A. (2008). Cdk9/Cyclin T1 complex: a key player during the activation/differentiation process of normal lymphoid B cells. J. Cell. Physiol. 215, 276–282.
Espinoza-Derout, J., Wagner, M., Salciccioli, L., Lazar, J. M., Bhaduri, S., Mascareno, E., Chaqour, B., and Siddiqui, M. A. (2009). Positive transcription elongation factor b activity in compensatory myocardial hypertrophy is regulated by cardiac lineage protein-1. Circ. Res. 104, 1347–1354.
Giacinti, C., Musaro, A., De Falco, G., Jourdan, I., Molinaro, M., Bagella, L., Simone, C., and Giordano, A. (2008). Cdk9-55: a new player in muscle regeneration. J. Cell. Physiol. 216, 576–582.
Grandjean, V., Gounon, P., Wagner, N., Martin, L., Wagner, K. D., Bernex, F., Cuzin, F., and Rassoulzadegan, M. (2009). The miR-124-Sox9 paramutation: RNA-mediated epigenetic control of embryonic and adult growth. Development 136, 3647–3655.
He, N., Jahchan, N. S., Hong, E., Li, Q., Bayfield, M. A., Maraia, R. J., Luo, K., and Zhou, Q. (2008). A La-related protein modulates 7SK snRNP integrity to suppress P-TEFb-dependent transcriptional elongation and tumorigenesis. Mol. Cell 29, 588–599.
He, W. J., Chen, R., Yang, Z., and Zhou, Q. (2006). Regulation of two key nuclear enzymatic activities by the 7SK small nuclear RNA. Cold Spring Harb. Symp. Quant. Biol. 71, 301–311.
Huang, V., Qin, Y., Wang, J., Wang, X., Place, R. F., Lin, G., Lue, T. F., and Li, L. C. (2010). RNAa is conserved in mammalian cells. PLoS ONE 5, e8848. doi: 10.1371/journal.pone.0008848
Kaichi, S., Takaya, T., Morimoto, T., Sunagawa, Y., Kawamura, T., Ono, K., Shimatsu, A., Baba, S., Heike, T., Nakahata, T., and Hasegawa, K. (2011). Cyclin-dependent kinase 9 forms a complex with GATA4 and is involved in the differentiation of mouse ES cells into cardiomyocytes. J. Cell. Physiol. 226, 248–254.
Kapranov, P., St Laurent, G., Raz, T., Ozsolak, F., Reynolds, C. P., Sorensen, P. H., Reaman, G., Milos, P., Arceci, R. J., Thompson, J. F., and Triche, T. J. (2010). The majority of total nuclear-encoded non-ribosomal RNA in a human cell is ‘dark matter’ un-annotated RNA. BMC Biol. 8, 149. doi: 10.1186/1741-7007-8-149
Krueger, B. J., Jeronimo, C., Roy, B. B., Bouchard, A., Barrandon, C., Byers, S. A., Searcey, C. E., Cooper, J. J., Bensaude, O., Cohen, E. A., Coulombe, B., and Price, D. H. (2008). LARP7 is a stable component of the 7SK snRNP while P-TEFb, HEXIM1 and hnRNP A1 are reversibly associated. Nucleic Acids Res. 36, 2219–2229.
Malumbres, M., and Barbacid, M. (2005). Mammalian cyclin-dependent kinases. Trends Biochem. Sci. 30, 630–641.
Mattick, J. S., Taft, R. J., and Faulkner, G. J. (2010). A global view of genomic information – moving beyond the gene and the master regulator. Trends Genet. 26, 21–28.
Morris, K. V. (2009). RNA-directed transcriptional gene silencing and activation in human cells. Oligonucleotides 19, 299–306.
Nguyen, V. T., Kiss, T., Michels, A. A., and Bensaude, O. (2001). 7SK small nuclear RNA binds to and inhibits the activity of CDK9/cyclin T complexes. Nature 414, 322–325.
Place, R. F., Li, L. C., Pookot, D., Noonan, E. J., and Dahiya, R. (2008). MicroRNA-373 induces expression of genes with complementary promoter sequences. Proc. Natl. Acad. Sci. U.S.A. 105, 1608–1613.
Radhakrishnan, S. K., and Gartel, A. L. (2006). CDK9 phosphorylates p53 on serine residues 33, 315 and 392. Cell Cycle 5, 519–521.
Rassoulzadegan, M., Grandjean, V., Gounon, P., Vincent, S., Gillot, I., and Cuzin, F. (2006). RNA-mediated non-mendelian inheritance of an epigenetic change in the mouse. Nature 441, 469–474.
Romano, G., and Giordano, A. (2008). Role of the cyclin-dependent kinase 9-related pathway in mammalian gene expression and human diseases. Cell Cycle 7, 3664–3668.
Sano, M., Abdellatif, M., Oh, H., Xie, M., Bagella, L., Giordano, A., Michael, L. H., Demayo, F. J., and Schneider, M. D. (2002). Activation and function of cyclin T-Cdk9 (positive transcription elongation factor-b) in cardiac muscle-cell hypertrophy. Nat. Med. 8, 1310–1317.
Sayed, D., and Abdellatif, M. (2011). MicroRNAs in development and disease. Physiol. Rev. 91, 827–887.
Silva, J., Nichols, J., Theunissen, T. W., Guo, G., Van Oosten, A. L., Barrandon, O., Wray, J., Yamanaka, S., Chambers, I., and Smith, A. (2009). Nanog is the gateway to the pluripotent ground state. Cell 138, 722–737.
Simon, S. A., and Meyers, B. C. (2011). Small RNA-mediated epigenetic modifications in plants. Curr. Opin. Plant Biol. 14, 148–155.
Simone, C., Stiegler, P., Bagella, L., Pucci, B., Bellan, C., De Falco, G., De Luca, A., Guanti, G., Puri, P. L., and Giordano, A. (2002). Activation of MyoD-dependent transcription by cdk9/cyclin T2. Oncogene 21, 4137–4148.
Takaya, T., Ono, K., Kawamura, T., Takanabe, R., Kaichi, S., Morimoto, T., Wada, H., Kita, T., Shimatsu, A., and Hasegawa, K. (2009). MicroRNA-1 and MicroRNA-133 in spontaneous myocardial differentiation of mouse embryonic stem cells. Circ. J. 73, 1492–1497.
Wagner, K. D., Wagner, N., Ghanbarian, H., Grandjean, V., Gounon, P., Cuzin, F., and Rassoulzadegan, M. (2008). RNA induction and inheritance of epigenetic cardiac hypertrophy in the mouse. Dev. Cell 14, 962–969.
Wang, S., and Fischer, P. M. (2008). Cyclin-dependent kinase 9: a key transcriptional regulator and potential drug target in oncology, virology and cardiology. Trends Pharmacol. Sci. 29, 302–313.
Warren, L., Manos, P. D., Ahfeldt, T., Loh, Y. H., Li, H., Lau, F., Ebina, W., Mandal, P. K., Smith, Z. D., Meissner, A., Daley, G. Q., Brack, A. S., Collins, J. J., Cowan, C., Schlaeger, T. M., and Rossi, D. J. (2010). Highly efficient reprogramming to pluripotency and directed differentiation of human cells with synthetic modified mRNA. Cell Stem Cell 7, 618–630.
Wei, P., Garber, M. E., Fang, S. M., Fischer, W. H., and Jones, K. A. (1998). A novel CDK9-associated C-type cyclin interacts directly with HIV-1 Tat and mediates its high-affinity, loop-specific binding to TAR RNA. Cell 92, 451–462.
Werner, A., and Swan, D. (2010). What are natural antisense transcripts good for? Biochem. Soc. Trans. 38, 1144–1149.
Xu, C., Lu, Y., Pan, Z., Chu, W., Luo, X., Lin, H., Xiao, J., Shan, H., Wang, Z., and Yang, B. (2007). The muscle-specific microRNAs miR-1 and miR-133 produce opposing effects on apoptosis by targeting HSP60, HSP70 and caspase-9 in cardiomyocytes. J. Cell. Sci. 120, 3045–3052.
Yang, Z., Yik, J. H., Chen, R., He, N., Jang, M. K., Ozato, K., and Zhou, Q. (2005). Recruitment of P-TEFb for stimulation of transcriptional elongation by the bromodomain protein Brd4. Mol. Cell 19, 535–545.
Yang, Z., Zhu, Q., Luo, K., and Zhou, Q. (2001). The 7SK small nuclear RNA inhibits the CDK9/cyclin T1 kinase to control transcription. Nature 414, 317–322.
Yik, J. H., Chen, R., Nishimura, R., Jennings, J. L., Link, A. J., and Zhou, Q. (2003). Inhibition of P-TEFb (CDK9/Cyclin T) kinase and RNA polymerase II transcription by the coordinated actions of HEXIM1 and 7SK snRNA. Mol. Cell 12, 971–982.
Keywords: mice, cardiac hypertrophy, epigenetic, paramutation, heredity, 7SK, Cdk9, non-coding RNA
Citation: Ghanbarian H, Grandjean V, Cuzin F and Rassoulzadegan M (2011) A network of regulations by small non-coding RNAs: the P-TEFb kinase in development and pathology. Front. Gene. 2:95. doi: 10.3389/fgene.2011.00095
Received: 04 October 2011; Paper pending published: 01 November 2011;
Accepted: 07 December 2011; Published online: 28 December 2011.
Edited by:
Philipp Kapranov, St. Laurent Institute, USAReviewed by:
Zhaohui Wang, University of Texas Southwestern Medical Center, USAPhilipp Kapranov, St. Laurent Institute, USA
Joseph Nadeau, Institute for Systems Biology, USA
Copyright: © 2011 Ghanbarian, Grandjean, Cuzin and Rassoulzadegan. This is an open-access article distributed under the terms of the Creative Commons Attribution Non Commercial License, which permits non-commercial use, distribution, and reproduction in other forums, provided the original authors and source are credited.
*Correspondence: Minoo Rassoulzadegan, INSERM U636, Université de Nice-Sophia Antipolis, Campus Valrose, 06108 Nice Cedex 2, France. e-mail:bWlub29AdW5pY2UuZnI=
†Present address: Hossein Ghanbarian, Department of Molecular Biology and Genetic Engineering, Stem Cell Technology Research Center, Tehran 15856364473, Iran.