- 1Department of Psychological Medicine, University of Otago, Christchurch, New Zealand
- 2Department of Health Sciences, School of Human and Health Sciences, University of Huddersfield, Huddersfield, UK
- 3Department of Pathology, University of Otago, Christchurch, New Zealand
- 4Division of Pharmacy and Pharmaceutical Sciences, School of Applied Sciences, University of Huddersfield, Huddersfield, UK
Dysfunction in the dopaminergic and serotonergic neurotransmitter systems has been demonstrated to be important in the etiology of borderline personality disorder (BPD). We investigated the relationship of two BPD risk factors, the HTR1A promoter polymorphism -1019C > G (rs6295) and the dopamine transporter (DAT1) repeat allele, with BPD in a major depressive disorder cohort of 367 patients. Out-patients with major depressive disorder were recruited for two treatment trials and assessed for personality disorders, including BPD. DNA samples were collected and the rs6295 polymorphism was detected with a TaqMan® assay. The DAT1 repeat allele was genotyped using a modified PCR method. The impact of polymorphisms on BPD was statistically analyzed using uncontrolled logistic and multiple logistic regression models. BPD patients had higher frequencies of the DAT1 9,9 (OR = 2.67) and 9,10 (OR = 3.67) genotypes and also those homozygous HTR1A G allele (OR = 2.03). No significant interactions between HTR1A and DAT1 genotypes, were observed; however, an increased risk of BPD was observed for those patients who were either 9,10; G,G (OR = 6.64) and 9,9; C,G (OR = 5.42). Furthermore, the odds of BPD in patients exhibiting high-risk variants of these two genes differed from those of patients in low-risk groups by up to a factor of 9. Our study provides evidence implicating the importance of the serotonergic and dopaminergic systems in BPD and that the interaction between genes from different neurotransmitters may play a role in the susceptibility to BPD.
Introduction
Borderline personality disorder (BPD) is a psychiatric diagnosis in the Diagnostic and Statistical Manual of Mental Disorders, Fourth Edition (DSM-IV) manual that describes a prolonged disturbance of personality function characterized by depth and variability of moods. These disturbances can have a pervasive negative impact on many or all of the psychosocial facets of life. Attempted suicide and completed suicide are possible outcomes, especially without proper care and effective therapy (Black et al., 2004). Onset of symptoms typically occurs during adolescence or young adulthood. In the USA, BPD is estimated to affect 2% of the population [National Institute of Mental Health, (NIMH)]. Impulsivity and impulsive-aggression are core characteristics of patients with BPD. These are heritable traits of temperament associated with suicidal and aggressive behaviors in patients with BPD (Oquendo and Mann, 2000; Soloff et al., 2000a).
The etiology of BPD is still not clear. However, twin studies have found that if one identical twin met the criteria for BPD, the other also met the criteria in 35–69% of cases (Loranger et al., 1982; Torgersen, 2000; Zanarini et al., 2004; Distel et al., 2008b). Several studies have suggested that traits related to BPD are influenced by genes (Torgersen, 2000; Torgersen et al., 2000; Joyce et al., 2006, 2009; Distel et al., 2008a) including genes relating to serotonergic and dopaminergic neurotransmission. The dopaminergic system is involved in cognitive and emotional processing and the regulation of impulsive behaviors: dysfunction of these processes have been related to BPD symptoms (Friedel, 2004), which may stem from multiple factors, including a genetic component. Several studies have illustrated the role of dopaminergic genes in the etiology of BPD and associated traits, including the dopamine transporter (DAT1; Joyce et al., 2006, 2009; Giegling et al., 2008). Indeed, in a previous study, we demonstrated that the DAT1 9-repeat allele to be a significant genetic risk factor for BPD (Joyce et al., 2006). Furthermore, animal research suggests that repeated exposure to social stress has long-term effects on DAT1 density (Lucas et al., 2004) and that DAT1 knock-out mice demonstrate abnormalities of social interaction (Rodriguiz et al., 2004). These studies highlight the importance of the dopaminergic system, in particular DAT1, in the neurobiology of BPD.
Many studies have also reported serotonergic dysfunction in BPD including genetic, behavioral, and neuroimaging studies investigating neurobiological correlates in BPD individuals (Hollander et al., 1994; Soloff et al., 2000b, 2007; Leyton et al., 2001; Bennett et al., 2002; Paris et al., 2004; Retz et al., 2004; Gabbard, 2005; Ni et al., 2006a,b, 2009; Pascual et al., 2007; Zetzsche et al., 2008). Moreover, the effectiveness of SSRIs, such as fluoxetine, in the treatment of depression in patients with BPD lends support to the role that serotonin dysfunction plays in BPD (Goodman et al., 2004). Serotonergic autoreceptors, including the serotonin 1A receptor (HTR1A) have been linked to BPD (Hansenne et al., 2002; Ni et al., 2006a; Soloff et al., 2007; Zetzsche et al., 2008). Reduced HTR1A activity has been linked to BPD and suicidal behavior (Hansenne et al., 2002). Furthermore, a polymorphism in the HTR1A promoter region (rs6295) has been investigated in BPD through genetic and neuroimaging studies (Serretti et al., 2007; Zetzsche et al., 2008; Ni et al., 2009). Although two of these reports failed to find a clear link between the rs6295 polymorphism and BPD (Serretti et al., 2007; Ni et al., 2009), Zetzsche et al. (2008) found that in BPD patients with the G allele, there were significant structural changes in the limbic system when compared to individuals with a C,C genotype.
Although both serotonergic and dopaminergic pathways have been implicated in BPD, no previous genetic studies have examined the joint (interactive) effects of both the DAT1 and HTR1A variants simultaneously on BPD. Due to the relationships of these neurotransmitter pathways with BPD etiology, we sought to determine if there was an interaction between the BPD risk factor polymorphisms, DAT1 repeat allele and HTR1A 1019C > G (rs6295), to see if there was an increase in the risk of BPD. Genetic analyses were performed using a cohort of 367 major depressive disorder patients measured for personality disorders including BPD.
Materials and Methods
Subjects
Patients with a principal diagnosis of major depressive disorder were recruited for two depression treatment studies aged ≥18 years from a wide variety of sources, including mental health out-patient clinics, general practitioners, self-referral, and psychiatric emergency services. No advertising for patients was involved. Exclusion criteria were minimized, but included a history of schizophrenia or mania, a principal current diagnosis of severe alcohol or drug dependence, or severe current medical illness. In addition, patients needed to be free of psychotropic medication for a minimum of 2 weeks, or five drug half-lives, except for an occasional hypnotic for sleep. Patients also could not have received in the past 12 months an adequate treatment trial of one of the trial treatments to which they could be randomized. All patients were from New Zealand of European descent. In the first depression treatment trial, 194 patients were randomized to fluoxetine or nortriptyline (Joyce et al., 2002); and in the second trial 173 patients were randomized to interpersonal psychotherapy or cognitive behavior therapy (Luty et al., 2007), giving a total of 367 patients for the current study.
The two trials ran sequentially, in the same university-based out-patient Clinical Research Unit, and were the only depression treatment studies being undertaken in the Department at the time. Both treatment studies had been approved by the Canterbury (New Zealand) Ethics Committee. After being referred, patients were screened over the telephone by a research nurse who confirmed depressive symptoms and checked inclusion and exclusion criteria. Those who appeared suitable for inclusion were seen by a psychiatrist, senior psychiatric registrar, or clinical psychologist for an initial assessment. After giving consent, the patient then attended for a detailed clinical assessment. Assessment included the Structured Clinical Interview for DSM (SCID; Spitzer et al., 1992), which had been expanded to include assessment of all DSM-III-R and DSM-IV melancholic and atypical depression symptoms. Approximately 6 weeks after commencing treatment a trained psychiatrist or clinical psychologist completed the Structured Clinical Interview for Axis II Personality Disorders (SCID-II; Spitzer, 1987). Other clinician ratings were the Montgomery-Asberg Depression Rating Scale (MADRS; Montgomery and Asberg, 1979), the Hamilton Rating Scale for Depression (HRSD; Hamilton, 1960), and the Mental State Examination (MSE; Parker et al., 1994). An independent research nurse completed the MADRS, the HRSD, and the MSE. During the baseline assessment patients completed a series of self-report questionnaires, which included the Hopkins Symptom Checklist (SCL-90; Derogatis et al., 1973), the Structured Clinical Interview for Personality Disorders Questionnaire (SCID-PQ; First et al., 1997), the Parental Bonding Instrument (PBI; Parker, 1979) and the Temperament and Character Inventory (TCI; Svrakic et al., 1993). After this assessment patients were randomized to fluoxetine or nortriptyline in the first treatment trial (Joyce et al., 2002); and interpersonal psychotherapy or cognitive behavior therapy for the second treatment trial (Luty et al., 2007). However, in the current study, the two sample cohorts were considered to comprise a single sample, with no distinction made between the two cohorts, as the treatment variable in each cohort was not of direct interest and did not affect the relationship between allele status and the criterion variable of interest, BPD diagnosis. DNA was also collected for genetic studies.
Genotyping
Genomic DNA was obtained from peripheral blood using sodium chloride extraction (Ciulla et al., 1988) and re-suspended in Tris–EDTA buffer (pH 8.0) and stored at -20°C until analysis. For all genotyping positive and negative controls were included. For DAT1 repeat allele genotyping, data from a previous study was obtained (Joyce et al., 2006), but for validation purposes 25% of the sample were re-genotyped using a previously described polymerase chain reaction (PCR) method (Sullivan et al., 1997). The single-nucleotide polymorphism (SNP), located in the promoter region of the HTR1A gene rs6295 (1019C > G), was genotyped using a TaqMan® probe (Applied Biosystems, Scoresby, Vic, Australia). One allelic probe was labeled with FAM dye, and the other with fluorescent VIC dye. PCRs were performed in a 384-well plate format within a total volume of 5 μl containing 2.73 μl of 2× ABgene Universal PCR Master Mix (Advanced Biotechnologies Ltd, Surrey, UK), 0.07 μl of 40× TaqMan® probe (Applied Biosystems) and 20 ng of genomic DNA. Plates were placed in the LightCycler® 480 real-time PCR System (Roche Diagnostics, Auckland, New Zealand), and heated at 95°C for 10 min, followed by 40 cycles of 95°C for 15 s, 60°C for 1 min and 72°C for 1 s. The fluorescence intensity in each well was measured and analyzed using the LightCycler® (Roche Diagnostics). Genotypes were assigned using the end-point genotyping analysis software. The genotypes of 15% of the samples were verified by sequencing (Genetics Analysis Services, University of Otago). The web-based analysis program SHEsis1 (Shi and He, 2005) was used to check for deviations in Hardy–Weinberg equilibrium (HWE).
Statistical Analysis
All data was entered into a relational database and subsequently transferred to the statistical package IBM SPSS (version 20.0) for statistical analysis. The sample was summarized descriptively to consider the incidence of BPD in various sub-groups of patients. Logistic regression analyses were conducted to investigate the association between BPD and the presence of the G allele of the rs6295 -1019C > G polymorphism; the presence of the DAT1 9-repeat allele; and the demographic factors of age and gender. Initially a series of uncontrolled analyses were conducted. Patients were classified according to their HTR1A genotype, with those homozygous for the C allele (subsequently referred to as the C,C variant) considered to be the reference category and those homozygous for the G allele (subsequently referred to as the G,G variant) and those heterozygous with the C and G alleles (subsequently referred to as the C,G variant) tested against the reference category. Patients were also classified according to the status of the 9-repeat allele of the DAT1 gene, with the 10-repeat polymorphism on both alleles (subsequently referred to as the 10,10 variant) considered to be the reference category and the 9-repeat polymorphism on both alleles (subsequently referred to as the 9,9 variant), and the 9-repeat and 10-repeat polymorphisms on each allele (subsequently referred to as the 9,10 variant) being tested against the reference category. Four patients were found to exhibit the 11-repeat or 12-repeat polymorphism: these were deleted from the data set before further analysis.
Any factor appearing to be important from the uncontrolled analyses was considered for inclusion in a subsequent multiple logistic regression model. As the analysis was primarily concerned with associations between BPD and the DAT1 and HTR1A genotypes in conjunction, these variables were forced into a multiple model. Statistical significance, odds ratio, and associated confidence intervals were estimated for each remaining parameter from this multiple model. Interactions between gene variants were also tested in this model.
Results
The two sample cohorts for this study were combined and consisted of a total of 367 patients (131 males, 236 females) with a mean age of 33.3 years (SD 10.9 years). There was no significant difference in the age distribution of males and females [mean male age 33.1 years (SD 11.0 years); mean female age 33.5 years (SD 10.9 years)]. The range of the data was 18–63 years in both males and females. Out of the full combined cohort, a valid diagnosis of whether or not the patient had BPD was recorded for 347 patients, with 50 (14.4%) having a positive diagnosis of BPD and 297 (85.6%) having no diagnosis of BPD. Table 1 gives a summary of the frequencies of patients for whom the HTR1A genotypes were recorded, and for whom the DAT1 genotype was recorded and validated from a previous study. The variants were in HWE (HTR1A, p = 0.52; DAT1; p = 0.77).
The relative frequencies of BPD in patients with certain allele combinations were compared. The incidence of BPD in patients with the 9,9, variant (five out of 19; 26.3%); or the 9,10 variant of the DAT1 gene (21 out of 106; 19.8%) was 2.36 times greater than the incidence of BPD in patients with the 10,10 variant (15 out of 179; 8.4%). The incidence of BPD in patients with the G,G variant of the HTR1A gene (12 out of 60; 20.0%) was 1.75 times the incidence of BPD in patients with the C,C variant (eight out of 70; 11.4%) or the C,G variant of this gene (13 out of 136; 9.6%).P-values, odds ratios, and associated 95% confidence intervals (CIs) for all parameters considered in uncontrolled logistic regression models are summarized in Table 2.
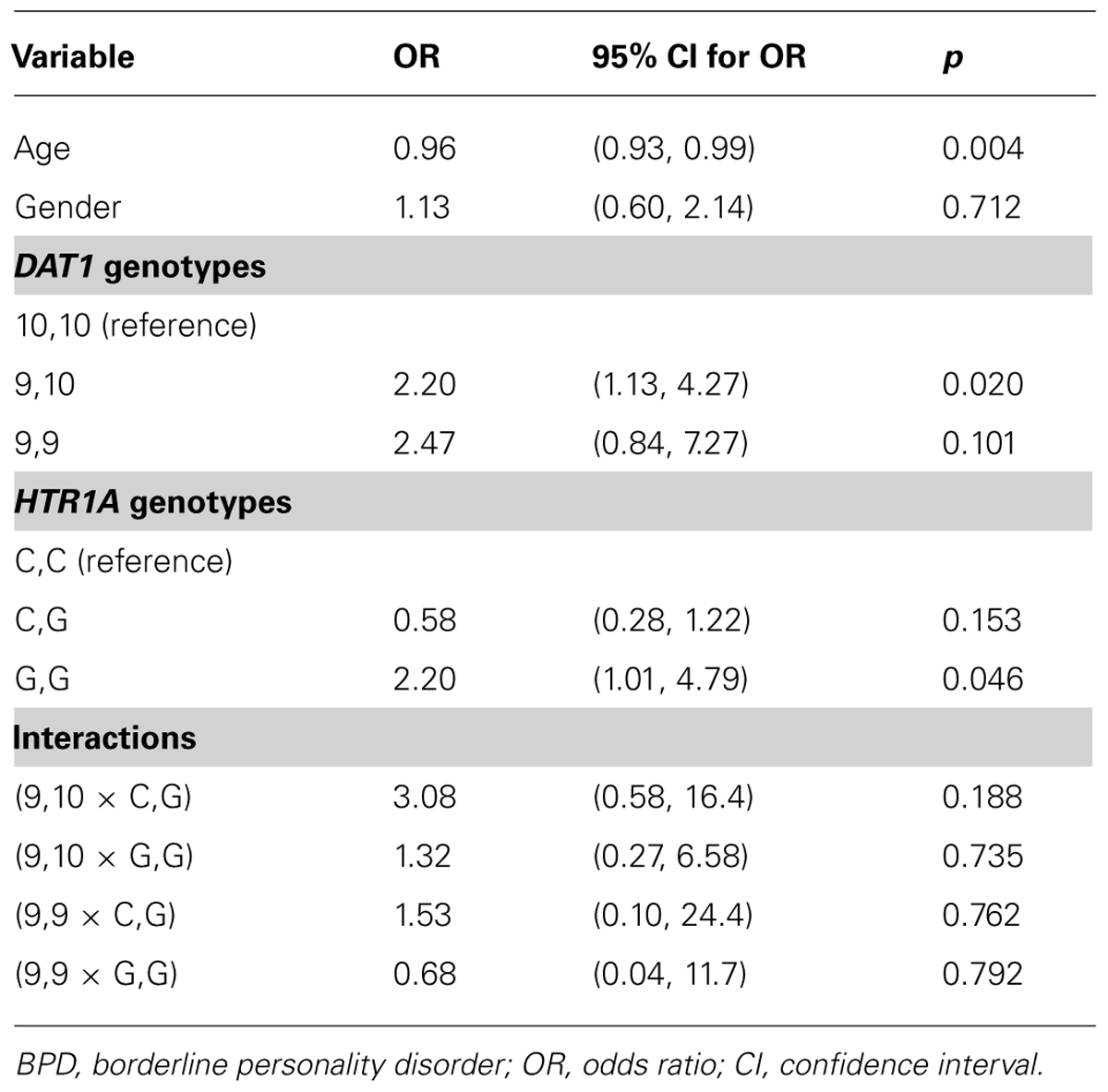
TABLE 2. Results from an uncontrolled logistic regression models (all considered parameters) predicting BPD.
The Age parameter exhibited a substantive or statistically significant association with the outcome and were carried forward for inclusion in a multiple logistic regression model, together with all indicator variables modelling the DAT1 and HTR1A genotypes. Gender was not found to exhibit a substantive or statistically significant association with the outcome and was not considered in a multiple model. No interactions were found to be significantly associated with the outcome: following standard procedures, all interactions were removed and the model re-cast as a main effects model. The output from this model is summarized in Table 3.
When controlling for other factors and covariates, age and HTR1A, DAT1 variants were significantly associated with the incidence of BPD (Table 2). For HTR1A, a comparison of the reference category of the HTR1A gene with the other two categories (C,G and G,G) appears to show non-significance of the variant with BPD. The odds of the BPD in patients with G,G genotypes [2.02; 95% CI: (1.10, 4.79)] are significantly greater than the odds in patients who have the C,G genotype (0.58; 95% CI 0.28, 1.22): however, this comparison is not shown in Table 2 as C,G was not selected as the reference category. Furthermore, the G,G genotype shows some degree of substantive significance with respect to the reference category of C,C (p = 0.171), while failing to reach statistical significance at the 5% level.
Higher incidences of BPD are found in younger patients, with the odds of BPD incidence reducing by about 5% for each year of age. Higher incidences of BPD are also found in patients with the 9,9 and 9,10 variants of the DAT1 gene than in patients with the 10,10 variant. Similarly, higher incidences of BPD are found in patients with the G,G variant of the HTR1A gene than in patients with the C,G and C,C variants of this gene.
To explore the risk of BPD from both HTR1A and DAT1 genotypes, the effect associated with specific combinations of these genotypes were considered. The effect of both the DAT1 and HTR1A variants results in a compounding of odds ratios (Table 4). The highest odds of BPD occurred in patients homozygous with the G,G allele of the HTR1A gene and either the 9,10 (OR, 6.64) or 9,9 (OR, 5.42) of the DAT1 gene. The lowest risk category, which was below the baseline risk of 1 or reference category (10,10; C,C), was 10,10; C,G. The risk ratio between the highest (9,10; G,G) and lowest (10,10; C;G) risk categories was 9.22. Conversely the effect of the presence of a particular variant of the DAT1 gene may “cancel” the effect of another variant of the HTR1A gene: for example the odds of BPD occurrence in patients with the 9,10 variants of the DAT1 gene, and the C,G variants of the HTR1A gene are within 18% of the odds of BPD occurrence in a patient with the reference categories of the 10,10 variant of the DAT1 gene and the G,G variant of the HTR1A gene.
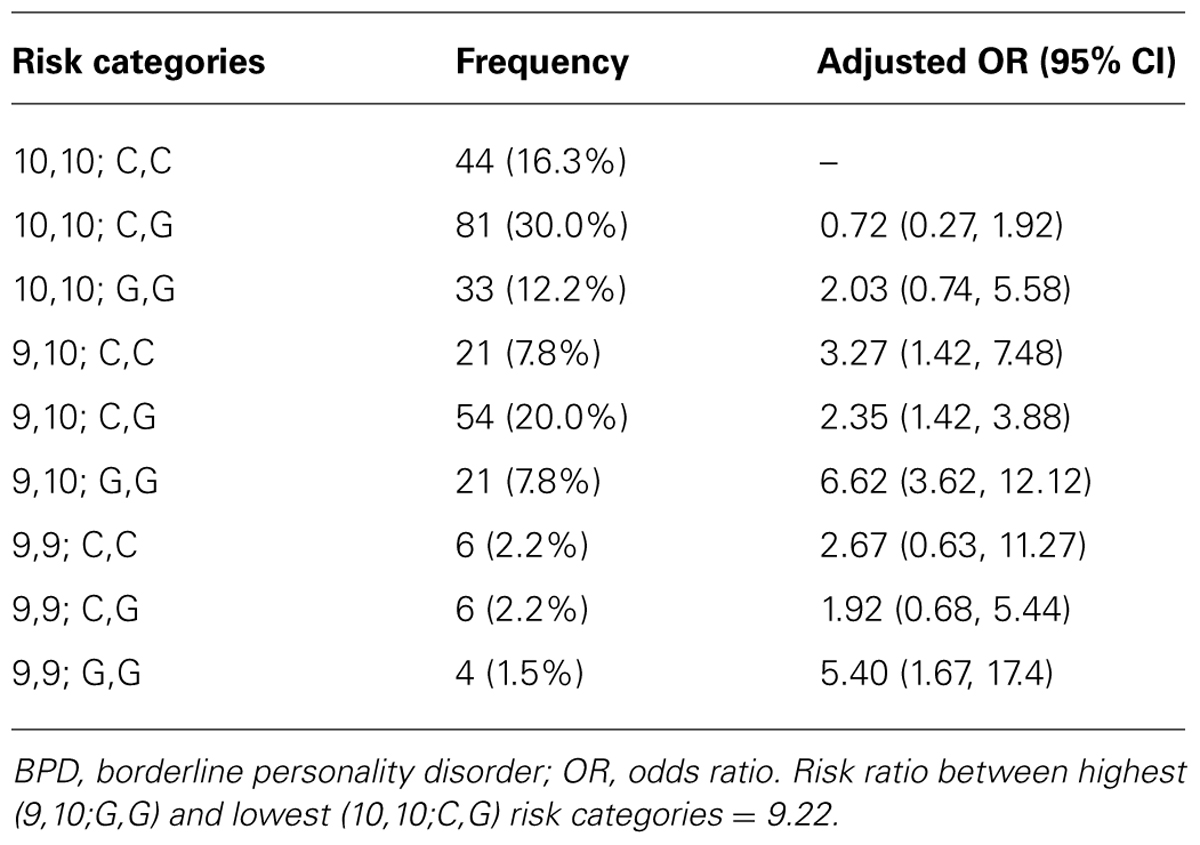
TABLE 4. Baseline risk of BPD (DAT1 10,10 and HTR1A C,C) = 1.00, with frequencies of gene combinations.
Discussion
In a previous study we identified that BPD was significantly associated with the DAT1 9-repeat allele variant (Joyce et al., 2006). In this study, the odds ratios associated with the DAT1 9-repeat allele (either 9,9 or 9,10) are consistent with our previous study, which was an analysis of a similar (but not identical) data set (Joyce et al., 2006). In the current study, however, the effect of the 9-repeat allele is considered as a controlling variable when assessing the effect of the HTR1A rs6295 allele in BPD. By contrast, the analysis of Joyce et al. (2006) focused on the DAT1 allele as the key experimental variable and did not consider its effect in conjunction with the HTR1A allele.
The current study confirms that the HTR1A G allele increased the risk of BPD when controlling for the presence of the DAT1 9,9 variant. Two previous studies failed to find a positive link between the HTR1A G allele and BPD (Serretti et al., 2007; Ni et al., 2009), and although our study did not reach statistical significance, individuals with the G,G genotype showed an increase risk of BPD. The analysis also revealed a significant increase in BPD when the two risk alleles were present on both genes. The odds of BPD in patients in highest risk categories were those with the G,G variant of HTR1A and either 9,9 (OR, 5.42) or 9,10 (OR 6.64) genotypes of DAT1. Moreover, the odds of BPD in patients with the highest risk category (9,10; G,G) were about nine times greater than those in the lowest risk category (10,10; C,G). The sub-group including patients at highest risk had an incidence of BPD of 25.3% (18 out of 71): nearly double the overall incidence rate in the full cohort.
Neither the number of 9-repeats of the DAT1 gene, nor the number of instances of the G allele in the HTR1A gene, seems to be additive effects. This suggests that a simpler model, in which the 9,10 and 9,9 variants of the DAT1 gene are merged together, and the C,G and C,C variants of the HTR1A gene are merged together, may provide an adequate summary of the data. While both the 9,9 and 9,10 variants of the DAT1 allele were found to be associated with BPD to a significantly greater extent than the 10,10 variant in an uncontrolled model, in a multiple model the incidence of BPD associated with the 9,9 variant was not significantly greater than the incidence of BPD associated with the 10,10 variant. This may be due to the relationships between gene variants.
One potential limitation of these findings is that the association has been found in clinical samples of depressed patients. In the community, the prevalence of BPD is about 1–2%, but in out-patient samples it is often 10–15% (Hyman, 2002). Mood disorders increase the risk of BPD. Any depression sample is thus an “enriched” sample, and it is possible that our findings in two combined depressed samples may not extend to the minority of BPD patients who do not suffer from depression. Another consideration is samples size and the possibility of false positives. However, with 367 patients, including 50 cases of BPD, the study was deemed adequately powered to detect any significant effects. Simulation studies by Peduzzi et al. (1996) suggest that with the number of positive cases, up to five variables may be considered in a multiple logistic regression model without compromising stability of parameter estimates. The sample size and number of positive BPD cases in our model is hence within acceptable limits.
Genetic factors contributing to BPD are thought to be only modest (Torgersen, 2000) and that the environment may have a significant impact on the development of BPD. However, twin studies of BPD have shown that 35–69% of the variance in BPD is caused by genetics (meaning that respectively 65% to 31% of the variance in BPD is caused by other factors, such as environment; Loranger et al., 1982; Torgersen, 2000; Zanarini et al., 2004; Distel et al., 2008b). This suggests that BPD has at least moderate genetic cause and is most likely an interaction of genes and environment leading to BPD. From our observations it is apparent that genetic factors contributing to BPD may be as a result of more than one risk variant, and consequently the involvement of more than one neurotransmitter system in traits underlying BPD. The complexity of BPD therefore cannot be delineated by single gene effects alone, which may explain, in part, why previous studies investigating the HTR1A G allele and BPD have been negative (Serretti et al., 2007; Ni et al., 2009). However, Zetzsche et al. (2008) revealed that BPD patients harboring the HTR1A G allele were significantly more likely to have a decrease in amygdala volume. The amygdala is part of the limbic system and controls negative emotion. Hyperactivation of the amygdala observed in BPD (Herpertz et al., 2001; Guitart-Masip et al., 2009), may reflect the heightened response to emotionally relevant environmental stressors in individuals with this disorder (Herpertz et al., 2001). Loss of inhibitory regulation in the amygdala results in disinhibited fear response, anger, and impulsive-aggressive behavior: core characteristics of BPD (Phillips and LeDoux, 1992). Promoter studies have shown that the minor G allele increases HTR1A activity (Czesak et al., 2012), and as an autoreceptor could conceivably reduce serotonergic function. Indeed, low levels of the serotonin metabolite, 5-hydroxyindoleacetic acid, have been detected in the cerebrospinal fluid of BPD patients (Brown et al., 1982). Reduced serotonergic function could conceivably inhibit the ability of this neurotransmitter system to modulate or control impulsive and aggressive behavior in BPD individuals. When combined with dysfunction in the dopaminergic system, potentially through DAT1, this could increase the susceptibility to BPD. Therefore, to fully understand the etiology of BPD, there is a clear need to interrogate multiple genetic factors and/or neurotransmitter systems in more depth.
Conflict of Interest Statement
The authors declare that the research was conducted in the absence of any commercial or financial relationships that could be construed as a potential conflict of interest.
Acknowledgments
We thank for all the patients and control subjects who volunteered to the study. This work was supported by grants from the Health Research Council of New Zealand, Canterbury Medical Research Foundation. We also wish to thank the University of Huddersfield Research Fund.
Footnotes
References
Bennett, A. J., Lesch, K. P., Heils, A., Long, J. C., Lorenz, J. G., Shoaf, S. E., et al. (2002). Early experience and serotonin transporter gene variation interact to influence primate CNS function. Mol. Psychiatry 7, 118–122. doi: 10.1038/sj.mp.4000949
Black, D. W., Blum, N., Pfohl, B., and Hale, N. (2004). Suicidal behavior in borderline personality disorder: prevalence, risk factors, prediction, and prevention. J. Pers. Disord. 18, 226–239. doi: 10.1521/pedi.18.3.226.35445
Brown, G. L., Ebert, M. H., Goyer, P. F., Jimerson, D. C., Klein, W. J., Bunney, W. E., et al. (1982). Aggression, suicide, and serotonin: relationships to CSF amine metabolites. Am. J. Psychiatry 139, 741–746.
Ciulla, T. A., Sklar, R. M., and Hauser, S. L. (1988). A simple method for DNA purification from peripheral blood. Anal. Biochem. 174, 485–488. doi: 10.1016/0003-2697(88)90047-4
Czesak, M., Le Francois, B., Millar, A. M., Deria, M., Daigle, M., Visvader, J. E., et al. (2012). Increased serotonin-1A (5-HT1A) autoreceptor expression and reduced raphe serotonin levels in deformed epidermal autoregulatory factor-1 (deaf-1) gene knock-out mice. J. Biol. Chem. 287, 6615–6627. doi: 10.1074/jbc.M111.293027
Derogatis, L. R., Lipman, R. S., and Covi, L. (1973). SCL-90: an outpatient psychiatric rating scale: preliminary report. Psychopharmacol. Bull. 9, 913–928.
Distel, M. A., Hottenga, J. J., Trull, T. J., and Boomsma, D. I. (2008a). Chromosome 9: linkage for borderline personality disorder features. Psychiatric Genet. 18, 302–307. doi: 10.1097/YPG.0b013e3283118468
Distel, M. A., Trull, T. J., Derom, C. A., Thiery, E. W., Grimmer, M. A., Martin, N. G., et al. (2008b). Heritability of borderline personality disorder features is similar across three countries. Psychol. Med. 38, 1219–1229. doi: 10.1017/S0033291707002024
First, M. B., Spitzer, R. L., Gibbon, M., and Williams, J. B. W. (1997). Structured Clinical Interview for DSM-IV Personality Disorders (SCID-II). American Psychiatric Press.
Friedel, R. O. (2004). Dopamine dysfunction in borderline personality disorder: a hypothesis. Neuropsychopharmacology 29, 1029–1039. doi: 10.1038/sj.npp.1300424
Gabbard, G. O. (2005). Mind, brain, and personality disorders. Am. J. Psychiatry 162, 648–655. doi: 10.1176/appi.ajp.162.4.648
Giegling, I., Moreno-De-Luca, D., Rujescu, D., Schneider, B., Hartmann, A. M., Schnabel, A., et al. (2008). Dopa decarboxylase and tyrosine hydroxylase gene variants in suicidal behavior. Am. J. Med. Genet. B Neuropsychiatr. Genet. 147, 308–315. doi: 10.1002/ajmg.b.30599
Goodman, M., New, A., and Siever, L. (2004). Trauma, genes, and the neurobiology of personality disorders. Ann. N.Y. Acad. Sci. 1032, 104–106. doi: 10.1196/annals.1314.008
Guitart-Masip, M., Pascual, J. C., Carmona, S., Hoekzema, E., Berge, D., Perez, V., et al. (2009). Neural correlates of impaired emotional discrimination in borderline personality disorder: an fMRI study. Prog. Neuropsychopharmacol. Biol. Psychiatry, 33, 1537–1545. doi: 10.1016/j.pnpbp.2009.08.022
Hamilton, M. (1960). A rating scale for depression. J. Neurol. Neurosurg. Psychiatry 2356–2362. doi: 10.1136/jnnp.23.1.56
Hansenne, M., Pitchot, W., Pinto, E., Reggers, J., Scantamburlo, G., Fuchs, S., et al. (2002). 5-HT1A dysfunction in borderline personality disorder. Psychol. Med. 32, 935–941. doi: 10.1017/S0033291702005445
Herpertz, S. C., Dietrich, T. M., Wenning, B., Krings, T., Erberich, S. G., Willmes, K., et al. (2001). Evidence of abnormal amygdala functioning in borderline personality disorder: a functional MRI study. Biol. Psychiatry 50, 292–298. doi: 10.1016/S0006-3223(01)01075-7
Hollander, E., Stein, D. J., DeCaria, C. M., Cohen, L., Saoud, J. B., Skodol, A. E., et al. (1994). Serotonergic sensitivity in borderline personality disorder: preliminary findings. Am. J. Psychiatry 151, 277–280. doi: 10.1176/appi.ajp.2013.12040518
Hyman, S. E. (2002). A new beginning for research on borderline personality disorder. Biol. Psychiatry 51, 933–935. doi: 10.1016/S0006-3223(02)01408-7
Joyce, P. J., McHugh, P. C., Light, K. J., Rowe, S., Miller, A., and Kennedy, M. A. (2009). The relationships between angry-impulsive personality traits, borderline personality disorder and genetic polymorphism of the dopamine transporter. Biol. Psychiatry 66, 717–721. doi: 10.1016/j.biopsych.2009.03.005
Joyce, P. R., McHugh, P. C., McKenzie, J. M., Sullivan, P. F., Mulder, R. T., Luty, S. E., et al. (2006). A dopamine transporter polymorphism is a risk factor for borderline personality disorder in depressed patients. Psychol. Med. 36, 807–813. doi: 10.1017/S0033291706007288
Joyce, P. R., Mulder, R. T., Luty, S. E., Sullivan, P. F., McKenzie, J. M., Abbott, R. M., et al. (2002). Patterns and predictors of remission, response and recovery in major depression treated with fluoxetine or nortriptyline. Aust. N.Z. J. Psychiatry 36, 384–391. doi: 10.1046/j.1440-1614.2001.01026.x
Leyton, M., Okazawa, H., Diksic, M., Paris, J., Rosa, P., Mzengeza, S., et al. (2001). Brain Regional alpha-[11C]methyl-L-tryptophan trapping in impulsive subjects with borderline personality disorder. Am. J. Psychiatry 158, 775–782. doi: 10.1176/appi.ajp.158.5.775
Loranger, A. W., Oldham, J. M., and Tulis, E. H. (1982). Tulis. Familial transmission of DSM-III borderline personality disorder. Arch. Gen. Psychiatry 39, 795–799. doi: 10.1001/archpsyc.1982.04290070031007
Lucas, L. R., Celen, Z., Tamashiro, K. L., Blanchard, R. J., Blanchard, D. C., Markham, C., et al. (2004). Repeated exposure to social stress has long-term effects on indirect markers of dopaminergic activity in brain regions associated with motivated behavior. Neuroscience 124, 449–457. doi: 10.1016/j.neuroscience.2003.12.009
Luty, S. E., Carter, J. D., McKenzie, J. M., Rae, A. M., Frampton, C. M., Mulder, R. T., et al. (2007). Randomised controlled trial of interpersonal psychotherapy and cognitive-behavioural therapy for depression. Br. J. Psychiatry 190, 496–502. doi: 10.1192/bjp.bp.106.024729
Montgomery, S. A., and Asberg, M. (1979). A new depression scale designed to be sensitive to change. Br. J. Psychiatry 134, 382–389. doi: 10.1192/bjp.134.4.382
Ni, X., Bismil, R., Chan, K., Sicard, T., Bulgin, N., McMain, S., et al. (2006a). Serotonin 2A receptor gene is associated with personality traits, but not to disorder, in patients with borderline personality disorder. Neurosci. Lett. 408, 214–219. doi: 10.1016/j.neulet.2006.09.002
Ni, X., Chan, K., Bulgin, N., Sicard, T., Bismil, R., McMain, S., et al. (2006b). Association between serotonin transporter gene and borderline personality disorder. J. Psychiatr. Res. 40, 448–453. doi: 10.1016/j.jpsychires.2006.03.010
Ni, X., Chan, D., Chan, K., McMain, S., and Kennedy, J. L. (2009). Serotonin genes and gene–gene interactions in borderline personality disorder in a matched case–control study. Prog. Neuropsychopharmacol. Biol. Psychiatry 33, 128–133. doi: 10.1016/j.pnpbp.2008.10.022
Oquendo, M. A., and Mann, J. J. (2000). The biology of impulsivity and suicidality. Psychiatr. Clin. North Am. 23, 11–25. doi: 10.1016/S0193-953X(05)70140-4
Paris, J., Zweig-Frank, H., Kin, N. M., Schwartz, G., Steiger, H., and Nair, N. P. (2004). Neurobiological correlates of diagnosis and underlying traits in patients with borderline personality disorder compared with normal controls. Psychiatry Res. 121, 239–252. doi: 10.1016/S0165-1781(03)00237-3
Parker, G. (1979). Parental characteristics in relation to depressive disorders. Br. J. Psychiatry 134, 138–147. doi: 10.1192/bjp.134.2.138
Parker, G., Hadzi-Pavlovic, D., Wilhelm, K., Hickie, I., Brodaty, H., Boyce, P., et al. (1994). Defining melancholia: properties of a refined sign-based measure. Br. J. Psychiatry 164, 216–226. doi: 10.1192/bjp.164.3.316
Pascual, J. C., Soler, J., Baiget, M., Cortes, A., Menoyo, A., Barrachina, J., et al. (2007). Association between the serotonin transporter gene and personality traits in borderline personality disorder patients evaluated with Zuckerman–Zuhlman personality questionnaire (ZKPQ). Actas Esp. Psiquiatr. 35, 382–386.
Peduzzi, P., Concato, J., Kemper, E., Holford, T. R., and Feinstein, A. R. (1996). A simulation study of the number of events per variable in logistic regression analysis. J. Clin. Epidemiol. 49, 1373–1379. doi: 10.1016/S0895-4356(96)00236-3
Phillips, R. G., and LeDoux, J. E. (1992). Differential contribution of amygdala and hippocampus to cued and contextual fear conditioning. Behav. Neurosci. 106, 274–285. doi: 10.1037/0735-7044.106.2.274
Retz, W., Retz-Junginger, P., Supprian, T., Thome, J., and Rosler, M. (2004). Association of serotonin transporter promoter gene polymorphism with violence: relation with personality disorders, impulsivity, and childhood ADHD psychopathology. Behav. Sci. Law 22, 415–425. doi: 10.1002/bsl.589
Rodriguiz, R. M., Chu, R., Caron, M. G., and Wetsel, W. C. (2004). Aberrant responses in social interaction of dopamine transporter knockout mice. Behav. Brain Res. 148, 185–198. doi: 10.1016/S0166-4328(03)00187-6
Serretti, A., Mandelli, L., Giegling, I., Schneider, B., Hartmann, A. M., Schnabel, A., et al. (2007). HTR2C and HTR1A gene variants in German and Italian suicide attempters and completers. Am. J. Med. Genet. B Neuropsychiatr. Genet. 144, 291–299. doi: 10.1002/ajmg.b.30432
Shi, Y. Y., and He, L. (2005). SHEsis, a powerful software platform for analyses of linkage disequilibrium, haplotype construction, and genetic association at polymorphism loci. Cell Res. 15, 97–98. doi: 10.1038/sj.cr.7290272
Soloff, P. H., Lynch, K. G., Kelly, T. M., Malone, K. M., and Mann, J. J. (2000a). Characteristics of suicide attempts of patients with major depressive episode and borderline personality disorder: a comparative study. Am. J. Psychiatry 157, 601–618. doi: 10.1176/appi.ajp.157.4.601
Soloff, P. H., Meltzer, C. C., Greer, P. J., Constantine, D., and Kelly, T. M. (2000b). A fenfluramine-activated FDG-PET study of borderline personality disorder. Biol. Psychiatry 47, 540–547. doi: 10.1016/S0006-3223(99)00202-4
Soloff, P. H., Price, J. C., Meltzer, C. C., Fabio, A., Frank, G. K., and Kaye, W. H. (2007). 5HT2A receptor binding is increased in borderline personality disorder. Biol. Psychiatry 62, 580–587. doi: 10.1016/j.biopsych.2006.10.022
Spitzer, M. (1987). [Clinical aspects and practical applications of DSM-III]. Psychiatr. Prax 14, 212–217.
Spitzer, R. L., Williams, J. B., Gibbon, M., and First, M. B. (1992). The Structured clinical interview for DSM-III-R (SCID). I: history, rationale, and description. Arch. Gen. Psychiatry 49, 624–629. doi: 10.1001/archpsyc.1992.01820080032005
Sullivan, P. F., Fifield, W. J., Kennedy, M. A., Mulder, R. T., Sellman, J. D., and Joyce, P. R. (1997). Novelty seeking and a dopamine transporter gene polymorphism (DAT1). Biol. Psychiatry 42, 1070–1072. doi: 10.1016/S0006-3223(97)00346-6
Svrakic, D. M., Whitehead, C., Przybeck, T. R., and Cloninger, C. R. (1993). Differential diagnosis of personality disorders by the seven-factor model of temperament and character. Arch. Gen. Psychiatry 50, 991–999. doi: 10.1001/archpsyc.1993.01820240075009
Torgersen, S. (2000). Genetics of patients with borderline personality disorder. Psychiatr. Clin. North Am. 23, 1–9. doi: 10.1016/S0193-953X(05)70139-8
Torgersen, S., Lygren, S., Oien, P. A., Skre, I., Onstad, S., Edvardsen, J., et al. (2000). A twin study of personality disorders. Compr. Psychiatry 41, 416–425. doi: 10.1053/comp.2000.16560
Zanarini, M. C., Frankenburg, F. R., Yong, L., Raviola, G., Bradford Reich, D., Hennen, J., et al. (2004). Borderline psychopathology in the first-degree relatives of borderline and axis II comparison probands. J. Pers. Disord. 18, 439–447. doi: 10.1521/pedi.18.5.439.51327
Keywords: personality disorder, depression, 5-HT, dopamine, genetics
Citation: Joyce PR, Stephenson J, Kennedy M, Mulder RT and McHugh PC (2014) The presence of both serotonin 1A receptor (HTR1A) and dopamine transporter (DAT1) gene variants increase the risk of borderline personality disorder. Front. Genet. 4:313. doi: 10.3389/fgene.2013.00313
Received: 12 June 2013; Paper pending published: 10 July 2013;
Accepted: 22 December 2013; Published online: 07 January 2014.
Edited by:
Andrew M. McIntosh, The University of Edinburgh, UKReviewed by:
Indrani Halder, University of Pittsburgh, USAPhilip Bowden Mitchell, University of New South Wales, Australia
Ellen Grunewald, University of Edinburgh, UK
Copyright © 2014 Joyce, Stephenson, Kennedy, Mulder and McHugh. This is an open-access article distributed under the terms of the Creative Commons Attribution License (CC BY). The use, distribution or reproduction in other forums is permitted, provided the original author(s) or licensor are credited and that the original publication in this journal is cited, in accordance with accepted academic practice. No use, distribution or reproduction is permitted which does not comply with these terms.
*Correspondence: Patrick C. McHugh, Division of Pharmacy and Pharmaceutical Sciences, School of Applied Sciences, University of Huddersfield, Huddersfield HD1 3DH, UK e-mail: p.c.mchugh@hud.ac.uk