- 1Department of Cardiovascular Medicine, The Second Xiangya Hospital of Central South University, Changsha, China
- 2Department of Cell Biology, School of Life Sciences, Central South University, Changsha, China
- 3Hunan Key Laboratory of Animal for Human Disease, School of Life Sciences, Central South University, Changsha, China
Arteriosclerotic cardiovascular disease (ASCVD) is one of the major causes of death worldwide and most commonly develops as a result of atherosclerosis (AS). As we all know, dyslipidemia is a leading pathogenic risk factor for ASCVD, which leads to cardiac ischemic injury and myocardial infarction. Dyslipidemias include hypercholesterolemia, hypertriglyceridemia, increased low-density lipoprotein cholesterol (LDL-c) and decreased high density lipoproteins cholesterol (HDL-c). Mutations of dyslipidemia related genes have been proved to be the crucial contributor to the development of AS and ASCVD. In this study, a Han-Chinese family with ASCVD was enrolled and the lipid testing discovered an obvious reduced levels of HDL-c in the affected members. We then performed whole exome sequencing to detect the candidate genes of the family. After data filtering, a novel heterozygous nonsense mutation (NM_007168: c.3460C>T; p.R1154X) of ABCA8 was detected and validated to be co-separated in the family members by Sanger sequencing. Previous studies have proved that deleterious heterozygous ABCA8 variants may disrupt cholesterol efflux and reduce HDL-c levels in humans and mice. This study may be the second report related to ABCA8 mutations in patients with reduced levels of HDL-c. Our study not only contributed to the genetic counseling and prenatal genetic diagnosis of patients with ASCVD caused by reduced HDL-c levels, but also provided a new sight among ABCA8, cholesterol efflux and HDL-c levels.
Introduction
Atherosclerosis is the major contributor of ischemic syndromes such as myocardial infarction or stroke, mainly resulted from plaque rupture and subsequent arterial blockade (Kobiyama and Ley, 2018). The incidence of AS is steadily rising along with an increasingly older population worldwide (Libby et al., 2016). The epidemiological survey shows that more than 20 million patients die from AS-related disorders worldwide every year (Herrington et al., 2016).
Previous studies have revealed that dyslipidemia was the predominant pathogenic factor of atherosclerotic plaque on the artery walls, which finally lead to AS and AS-related disorders (Hurtubise et al., 2016). And the main forms of dyslipidemia contains hypercholesterolemia, hypertriglyceridemia, and reduced high density lipoprotein (HDL-c). Dyslipidemia is a multi-factorial disease, which derives from complex interactions between genetic and environmental lesions. Many genes have been identified may be responsible for hypercholesterolemia and hypertriglyceridemia, for instance, Low-Density Lipoprotein Receptor, Proprotein Convertase Subtilisin/Kexin type 9, Lipoprotein lipase, Apolipoprotein C2, Reticulon 3 and et al. (Surendran et al., 2012; Xiang et al., 2017, 2018). Low levels of HDL-c increase the risk of atherosclerotic cardiovascular disorder and shorten life expectancy. However, the underlying cause of reduced HDL-c values was still not clear. Several genes including ATP binding cassette subfamily A member 1 (ABCA1) and ATP binding cassette subfamily A member 8 (ABCA8) have been identified in patients with reduced HDL-c (Trigueros-Motos et al., 2017; Maranghi et al., 2019).
Here, we enrolled a Han-Chinese family with Coronary Heart Disease (ASCVD) (Figure 1A). Lipid testing revealed that the levels of HDL-c were overt decreased, while the levels of low-density lipoprotein cholesterol (LDL-c), total cholesterol (TC) and triglycerides (TG) were close to normal standards. Whole exome sequencing and Sanger sequencing were employed to detect the genetic lesion of the family.
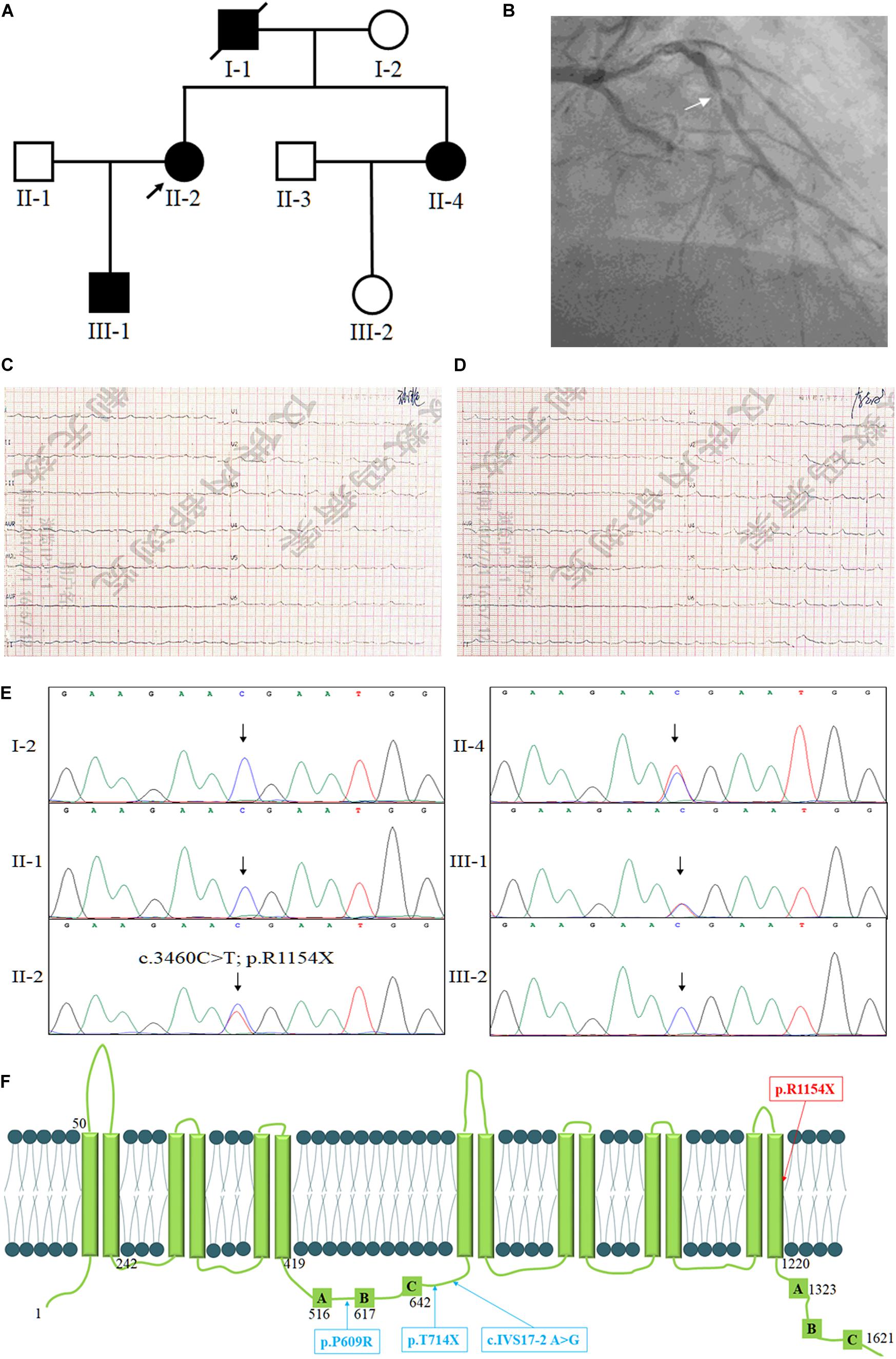
Figure 1. The clinical and genetic information of the family. (A) Pedigree of the family with low levels of HDL-c. Family members are identified by generations and numbers. Squares indicate male family members; circles, female members; closed symbols, affected members; open symbols, unaffected members; arrow, proband. (B) The coronary angiography of the proband, the arrow shows the stenosis of the anterior descending coronary artery. The ECG testing of the proband before percutaneous coronary intervention (C) and after percutaneous coronary intervention (D). (E) Sequencing results of the ABCA8 mutation. Sequence chromatogram indicates a C to T transition of nucleotide 3460. (F) The structure of ABCA8 and the summary of reported mutations of ABCA8.
Case Presentation
The proband (II-2), a 54-year-old lady, came to the hospital due to recurrent chest pain in last 2 years. Coronary angiography indicated approximately 60–80% stenosis of the anterior descending coronary artery (Figure 1B), ECG testing also suggested the patient suffered from CHD (Figure 1C). However, the lipid testing described the level of LDL-c (3.94 mmol/L; control: <3.12 mmol/L) and a distinctly reduced levels of HDL-c (0.41 mmol/L; control: 0.9–2.19 mmol/L) of the proband (Table 1). This discovery attracted our interest because most patients with ASCVD commonly presented high levels of LDL-c, TC, and TG (Hurtubise et al., 2016). We then investigated the family history of the proband (II-2), which indicated that her young sister (II-4) has been diagnosed as the occlusion of left iliac artery and her father (I-1) was died from myocardial infarction at 60-year-old. Lipid testing further described that both her son (III-1) and her young sister (II-4) showed an overt reduced levels of HDL-c and normal levels of LDL-c, TC, and TG (Table 1). And his young sister (II-4) also suffered from arterial plaque in the left lower limb. The blood pressure of the proband was 80–120 mmHg and the fasting blood-glucose was 5.2 mmol/L. The proband (II-2) accepted the treatment of percutaneous coronary intervention, the proband did not complain any uncomfortable after treatment. And breathing sound of the lungs was clearly, the heart rate was normal (Figure 1D), and the insertion site recovered well. The II-4 is accepting recovery treatment by exercise and diet control.
Laboratory Investigations
We supposed that the low levels of HDL-c may be the leading cause of AS and ASCVD in this family. However, what’s the genetic lesion underling the reduced levels of HDL-c in this family? We then isolated the genomic DNA of the proband and other family members (I-2, II-1, II-2, II-4, III-1, and III-2). Whole exome sequencing of the proband (II-2) was performed to detect the candidate gene of reduced levels of HDL-c.
In short, Exome capture and next-generation sequencing were conducted by Novogene Bioinformatics Institute (Beijing, China). One microgram of qualified genomic DNA from each person was captured by the Agilent’s SureSelect Human All Exon kit V5 (Agilent Technologies, Inc., Santa Clara, CA, United States) and sequenced by Illumina Hiseq4000 (Illumina Inc., San Diego, CA, United States). Shortly, genomic DNA were randomly carved by Covaris S220 sonicator (Covaris, Inc., Woburn, MA, United States) (Fan et al., 2019a). Then the fragmented DNAs underwent three enzymatic steps: end repair, A-tailing and adapters ligation. The adapter-ligated DNA fragments were amplified with Herculase II fusion DNA polymerase (Agilent). Later, the exomes in the pre-capture libraries were captured by SureSelect capture library kit (Agilent) (Fan et al., 2019b). After DNA quality estimation, the captured DNA library was used for next-generation sequencing on Illumina Hiseq4000 platform (Fan et al., 2019a). Downstream processing was carried out by Genome Analysis Toolkit (GATK), Varscan2 and Picard, and variant calls were performed by the GATK Haplotype Caller (Fan et al., 2019a). Variant annotation referred to Ensemble release 82, and filtering was conducted by ANNOVAR Documentation.
The filtered non-synonymous SNPs or INDELs with an alternative allele frequency more than 1% in public databases were kicked before further analysis. The public databases contains the NHLBI Exome Sequencing Project Exome Variant Server (ESP6500), dbSNP1441, the 1000 Genomes project2, the ExAC database3 and in-house exome databases of Novogene (2500 exomes) (Fan et al., 2019b). Then the filtered SNVs and INDELs, predicted by SIFT4, Polyphen25, and MutationTaster6 to be damaging, were remained (Fan et al., 2019a).
After data filtering, a novel nonsense mutation (NM_007168: c.3460C>T; p.R1154X) of ABCA8 was identified and validated by Sanger sequencing in the proband (Figure 1E). Previous studies have revealed that deleterious heterozygous ABCA8 mutations may disrupt cholesterol efflux and reduce HDL-c levels in humans and mice (Trigueros-Motos et al., 2017; Sasaki et al., 2018). No other meaningful mutations related to lipid metabolism has been identified. Sanger sequencing further confirmed that only the affected individuals (II-2, II-4, and III-1) carried the novel nonsense mutation (NM_007168: c.3460C>T; p.R1154X) of ABCA8 (Figure 1F). The novel mutation, resulting a truncated protein, was absent in the healthy members (I-2, II-1, and III-2) and 200 local people who were used as an internal control to exclude the SNP in local people (Fan et al., 2019a). Bioinformatics predicted that the newly identified mutation was deleterious and may disrupt the structure and function of ABCA8 (Schwarz et al., 2014). On the basis of ACMG guidelines (Richards et al., 2015), the novel variant meetings the following criteria from the ACMG guidelines: PVS1, PS3, and PM2.
Discussion
As the extremely crucial transmembrane proteins, the ABC (ATP-binding cassette) transporters were encoded by 48 ABC transporter genes which were divided in to seven subfamilies named A–G in human (Kim et al., 2013; Hedditch et al., 2014). The ABC transporters are responsible for transferring substrates such as lipids, peptides, ions, carbohydrates, and vitamins across membranes by employing the energy from the hydrolysis of ATP (Trigueros-Motos et al., 2017; Sasaki et al., 2018). The subfamily A (ABCA) consists of 12 members in two subgroups: ABCA6-like and ABCA1-like transporter.
The subfamily A (ABCA) has 12 members with two subgroups, i.e., ABCA6-like and ABCA1-like transporter. The ABC1–4, 7, and 12 belongs to the ABCA1-like subgroup which play an important role in transporting cholesterol and phospholipids transport (Tsuruoka et al., 2002). However, less is known about the functional roles of the ABCA6-like subgroup transporters, i.e., ABCA5–6, 8–10 in humans, although several reports have described the tissue mRNA and protein expressions (Kim et al., 2013; Hedditch et al., 2014). ABCA8 is expressed in the brain, heart, small intestine, liver, lung, pancreas, prostate, spleen, testicle in human tissue (Bleasel et al., 2013; Demidenko et al., 2015; Gidding et al., 2015). However, less is known about its functional roles in vivo.
The human ABCA8 gene encoding ATP-binding cassette-subfamily A, member 8 protein is located on chromosome 17q24.2, encoding 1621amino. ABCA8 contains 14 predicted transmembrane domains and 2 putative ATP-binding cassettes, but it lacks the common ABC transporter motif LSGGQ (Tsuruoka et al., 2002). At first, ABCA8 was classified into ABCA6-like transporters subgroup which was not responsible for lipid transporting in the ABCA family (Kaminski et al., 2001). However, ABCA8 was currently confirmed to associate with regulating cholesterol efflux and HDL-c levels in a similar fashion as the canonical cholesterol efflux proteins ABCA1 and adenosine triphosphate–binding cassette transporters G1 (Kim et al., 2008; Trigueros-Motos et al., 2017). Furthermore, ABCA8 has been suggested to associate with stimulating sphingomyelin production in oligodendrocytes (Bleasel et al., 2013; Kim et al., 2013). Meanwhile, the ABCA8 protein is reported to relate to ovarian cancer other than its role in anionic drugs transport across Xenopus laevis oocyte membranes (Hedditch et al., 2014; Liu et al., 2015). Here, we identified a novel nonsense mutation (NM_007168: c.3460C>T; p.R1154X) of ABCA8 in a family with very low levels of HDL-c which further confirmed that deleterious heterozygous ABCA8 mutations may disrupt cholesterol efflux and reduce HDL-c levels in humans. This discovery may be the second report related to ABCA8 mutations in patients with reduced levels of HDL-c.
Commonly, increased LDL-c and TG were recognized as the crucial risk factors of ASCVD (Fernandez et al., 2015). Mutations of the pathogenic genes related to hypercholesterolemia and hypertriglyceridemia probably cause AS (Surendran et al., 2012; Xiang et al., 2017, 2018). Although reducing LDL-c levels has been proved to be an effective therapy, some patients still remain a high risk of ASCVD. This “residual risk” is majority result from elevated TG and low HDL-c levels (Fruchart et al., 2008). Our study, together with other researches, further revealed that the metabolism of HDL-c levels also played a significant role in ASCVD.
At present, only Trigueros-Motos et al. (2017) reported three deleterious heterozygous ABCA8 mutations including p. P609R, c.IVS17-2 A>G and p. T741X in patients with reduced HDL-c levels (Figure 1F). In addition, the rs4148008 in ABCA8 was reported to be significantly related to an average of 0.42 mg/dL HDL-c levels (Teslovich et al., 2010). In our study, the EXAC database contains three heterozygous carriers of the novel mutation, but we still believe that the novel mutation is the genetic factor of the family. Because the nonsense mutation is co-separated in the family members, bioinformatics analysis predict that this mutation is pathogenic and the mutation belongs to PVS1, PS3, and PM2 underling ACMG classification. It is reasonable when the novel mutation existed with an extremely lower MAF in EXAC database, since the reduced levels of HDL-c have a high risk of AS, but most symptoms of AS do not show up until a blockage occurs (Herrington et al., 2016; Kobiyama and Ley, 2018).
In mice, knockout Abca8 may affect the efflux transporter for cholesterol and taurocholate. And the levels of HDL-c were significantly decreased in Abca8 knockout mice. On the contrary, hepatic overexpression of human ABCA8 in mice showed an obvious increased HDL-c in plasma (Trigueros-Motos et al., 2017). The phenotypes of human carried ABCA8 mutations were consistent with the presentation in Abca8 knockout mice. Here, the identified novel nonsense mutation (NM_007168: c.3460C>T; p.R1154X), resulting in the loss of function of ABCA8, also showed the same phenotypes with previous studies in human and Abca8 knockout mice (Trigueros-Motos et al., 2017; Sasaki et al., 2018).
Conclusion
In conclusion, we enrolled a Han-Chinese family with ASCVD. Lipid testing indicated overt reduced levels of HDL-c. Whole exome sequencing and Sanger sequencing detected a nonsense mutation (NM_007168: c.3460C>T; p.R1154X) of ABCA8 in the ASCVD patients and absent in the healthy members. This study may be the second report related to ABCA8 mutations in patients with reduced levels of HDL-c. Our study not only contributed to the genetic counseling and prenatal genetic diagnosis of patients with ASCVD caused by reduced HDL-c levels, but also confirmed the genetic lesion of the family with reduced HDL-c levels which suggested the family members with the novel mutation pay attention to ASCVD and accept medical examination regularly. In addition, our study also provided a new sight among ABCA8, cholesterol efflux and HDL-c levels.
Data Availability Statement
The datasets for this article are not publicly available due to concerns regarding participant/patient anonymity. Requests to access the datasets should be directed to the corresponding author.
Ethics Statement
The studies involving human participants were reviewed and approved by The Second Xiangya Hospital of the Central South University Ethics Committee. The patients/participants provided their written informed consent to participate in this study. Written informed consent was obtained from the individual(s) for the publication of any potentially identifiable images or data included in this manuscript.
Author Contributions
C-YW and L-LF performed genetic analysis. Y-QC enrolled the samples and clinical data. J-YJ and RD isolated the gDNA and performed PCR. C-YW, Y-QC, and L-LF wrote the manuscript. RX supported the study. All authors reviewed the manuscript.
Funding
This study was supported by the National Science and Technology Major Project of the Ministry of Science and Technology of China (2017ZX10103005-006), National Natural Science Foundation of China (81970403), the Fundamental Research Funds for the Central Universities of Hunan Province (CX20190104), the Emergency Project of Prevention and Control for COVID-19 of Central South University (160260003), and the Fundamental Research Funds for Central Universities of Central South University (2019zzts228).
Conflict of Interest
The authors declare that the research was conducted in the absence of any commercial or financial relationships that could be construed as a potential conflict of interest.
Acknowledgments
We thank all subjects for participating in this study. We also thank Dr. Shuai Guo from The University of Texas MD Anderson Cancer Center, United States for editing the language.
Abbreviations
ABCA1, ATP binding cassette subfamily A member 1; ABCA8, ATP binding cassette subfamily A member 8; AS, atherosclerosis; ASCVD, arteriosclerotic cardiovascular disease; HDL-c, high density lipoprotein; LDL-c, low-density lipoprotein cholesterol; TC, total cholesterol; TG, triglycerides.
Footnotes
- ^ http://www.ncbi.nlm.nih.gov/projects/SNP/index.html
- ^ http://www.1000genomes.org/
- ^ http://exac.broadinstitute.org
- ^ http://sift.jcvi.org/
- ^ http://genetics.bwh.harvard.edu/pph2/
- ^ http://www.mutationtaster.org/
References
Bleasel, J. M., Hsiao, J. H., Halliday, G. M., and Kim, W. S. (2013). Increased expression of ABCA8 in multiple system atrophy brain is associated with changes in pathogenic proteins. J. Parkinsons Dis. 3, 331–339. doi: 10.3233/jpd-130203
Demidenko, R., Razanauskas, D., Daniunaite, K., Lazutka, J. R., Jankevicius, F., and Jarmalaite, S. (2015). Frequent down-regulation of ABC transporter genes in prostate cancer. BMC Cancer 15:683. doi: 10.1186/s12885-015-1689-8
Fan, L. L., Chen, Y. Q., Huang, H., Yuan, Z. Z., Jin, J. Y., Hu, M., et al. (2019a). Exome sequencing identifies a novel nonsense mutation of ring finger protein 207 in a Chinese family with Long QT syndrome and syncope. J. Hum. Genet. 64, 233–238. doi: 10.1038/s10038-018-0549-1
Fan, L. L., Ding, D. B., Huang, H., Chen, Y. Q., Jin, J. Y., Xia, K., et al. (2019b). A de novo mutation of SMYD1 (p.F272L) is responsible for hypertrophic cardiomyopathy in a Chinese patient. Clin. Chem. Lab. Med. 57, 532–539. doi: 10.1515/cclm-2018-0578
Fernandez, R., Everett, B., Miranda, C., Rolley, J. X., Rajaratnam, R., and Davidson, P. M. (2015). Migratory implications for coronary heart disease risk prevention in Asian Indians: evidence from the leading health indicators. J. Cult. Divers. 22, 30–38.
Fruchart, J. C., Sacks, F., Hermans, M. P., Assmann, G., Brown, W. V., Ceska, R., et al. (2008). The residual risk reduction initiative: a call to action to reduce residual vascular risk in patients with dyslipidemia. Am. J. Cardiol. 102, 1K–34K. doi: 10.1016/j.amjcard.2008.10.002
Gidding, S. S., Champagne, M. A., De Ferranti, S. D., Defesche, J., Ito, M. K., Knowles, J. W., et al. (2015). The agenda for familial hypercholesterolemia: a scientific statement from the American heart association. Circulation 132, 2167–2192. doi: 10.1161/cir.0000000000000297
Hedditch, E. L., Gao, B., Russell, A. J., Lu, Y., Emmanuel, C., Beesley, J., et al. (2014). ABCA transporter gene expression and poor outcome in epithelial ovarian cancer. J. Natl. Cancer Inst. 106:dju149.
Herrington, W., Lacey, B., Sherliker, P., Armitage, J., and Lewington, S. (2016). Epidemiology of atherosclerosis and the potential to reduce the global burden of Atherothrombotic disease. Circ. Res. 118, 535–546. doi: 10.1161/circresaha.115.307611
Hurtubise, J., Mclellan, K., Durr, K., Onasanya, O., Nwabuko, D., and Ndisang, J. F. (2016). The different facets of dyslipidemia and hypertension in atherosclerosis. Curr. Atheroscler. Rep. 18:82.
Kaminski, W. E., Wenzel, J. J., Piehler, A., Langmann, T., and Schmitz, G. (2001). ABCA6, a novel a subclass ABC transporter. Biochem. Biophys. Res. Commun. 285, 1295–1301. doi: 10.1006/bbrc.2001.5326
Kim, W. S., Hsiao, J. H., Bhatia, S., Glaros, E. N., Don, A. S., Tsuruoka, S., et al. (2013). ABCA8 stimulates sphingomyelin production in oligodendrocytes. Biochem. J. 452, 401–410. doi: 10.1042/bj20121764
Kim, W. S., Weickert, C. S., and Garner, B. (2008). Role of ATP-binding cassette transporters in brain lipid transport and neurological disease. J. Neurochem. 104, 1145–1166. doi: 10.1111/j.1471-4159.2007.05099.x
Libby, P., Bornfeldt, K. E., and Tall, A. R. (2016). Atherosclerosis: successes, surprises, and future challenges. Circ. Res. 118, 531–534. doi: 10.1161/circresaha.116.308334
Liu, X., Gao, Y., Zhao, B., Li, X., Lu, Y., Zhang, J., et al. (2015). Discovery of microarray-identified genes associated with ovarian cancer progression. Int. J. Oncol. 46, 2467–2478. doi: 10.3892/ijo.2015.2971
Maranghi, M., Truglio, G., Gallo, A., Grieco, E., Verrienti, A., Montali, A., et al. (2019). A novel splicing mutation in the ABCA1 gene, causing Tangier disease and familial HDL deficiency in a large family. Biochem. Biophys. Res. Commun. 508, 487–493. doi: 10.1016/j.bbrc.2018.11.064
Richards, S., Aziz, N., Bale, S., Bick, D., Das, S., Gastier-Foster, J., et al. (2015). Standards and guidelines for the interpretation of sequence variants: a joint consensus recommendation of the American College of medical genetics and genomics and the association for molecular pathology. Genet. Med. 17, 405–424.
Sasaki, K., Tachikawa, M., Uchida, Y., Hirano, S., Kadowaki, F., Watanabe, M., et al. (2018). ATP-Binding cassette transporter a subfamily 8 is a sinusoidal efflux transporter for cholesterol and taurocholate in mouse and human liver. Mol. Pharm. 15, 343–355. doi: 10.1021/acs.molpharmaceut.7b00679
Schwarz, J. M., Cooper, D. N., Schuelke, M., and Seelow, D. (2014). MutationTaster2: mutation prediction for the deep-sequencing age. Nat. Methods 11, 361–362. doi: 10.1038/nmeth.2890
Surendran, R. P., Visser, M. E., Heemelaar, S., Wang, J., Peter, J., Defesche, J. C., et al. (2012). Mutations in LPL, APOC2, APOA5, GPIHBP1 and LMF1 in patients with severe hypertriglyceridaemia. J. Intern. Med. 272, 185–196. doi: 10.1111/j.1365-2796.2012.02516.x
Teslovich, T. M., Musunuru, K., Smith, A. V., Edmondson, A. C., Stylianou, I. M., Koseki, M., et al. (2010). Biological, clinical and population relevance of 95 loci for blood lipids. Nature 466, 707–713.
Trigueros-Motos, L., Van Capelleveen, J. C., Torta, F., Castano, D., Zhang, L. H., Chai, E. C., et al. (2017). ABCA8 regulates cholesterol efflux and high-density lipoprotein cholesterol levels. Arterioscler. Thromb. Vasc. Biol. 37, 2147–2155.
Tsuruoka, S., Ishibashi, K., Yamamoto, H., Wakaumi, M., Suzuki, M., Schwartz, G. J., et al. (2002). Functional analysis of ABCA8, a new drug transporter. Biochem. Biophys. Res. Commun. 298, 41–45. doi: 10.1016/s0006-291x(02)02389-6
Xiang, R., Fan, L. L., Huang, H., Chen, Y. Q., He, W., Guo, S., et al. (2018). Increased reticulon 3 (RTN3) leads to obesity and hypertriglyceridemia by interacting with heat shock protein family A (Hsp70) member 5 (HSPA5). Circulation 138, 1828–1838. doi: 10.1161/circulationaha.117.030718
Keywords: atherosclerosis, reduced HDL-c levels, cholesterol efflux, ABCA8, nonsense mutation
Citation: Wang C-Y, Chen Y-Q, Jin J-Y, Du R, Fan L-L and Xiang R (2020) A Novel Nonsense Mutation of ABCA8 in a Han-Chinese Family With ASCVD Leads to the Reduction of HDL-c Levels. Front. Genet. 11:755. doi: 10.3389/fgene.2020.00755
Received: 10 April 2020; Accepted: 25 June 2020;
Published: 15 July 2020.
Edited by:
Kirsten B. Holven, University of Oslo, NorwayReviewed by:
Rita Selvatici, University of Ferrara, ItalyMuhammad Jawad Hassan, National University of Medical Sciences (NUMS), Pakistan
Copyright © 2020 Wang, Chen, Jin, Du, Fan and Xiang. This is an open-access article distributed under the terms of the Creative Commons Attribution License (CC BY). The use, distribution or reproduction in other forums is permitted, provided the original author(s) and the copyright owner(s) are credited and that the original publication in this journal is cited, in accordance with accepted academic practice. No use, distribution or reproduction is permitted which does not comply with these terms.
*Correspondence: Liang-Liang Fan, c3dmYW5saWFuZ2xpYW5nQGNzdS5lZHUuY24=; Rong Xiang, c2hpcmxlc21pbGVAY3N1LmVkdS5jbg==
†These authors have contributed equally to this work