- Key Laboratory of Prevention and Control of Birth Defects, Department of Medical Genetics, Newborn Screening Center, Liuzhou Maternity and Child Health Care Hospital, Liuzhou Institute for Reproduction and Genetics, Affiliated Maternity Hospital and Affiliated Children’s Hospital of Guangxi University of Science and Technology, Liuzhou, China
Inborn errors of metabolism (IEMs) often causing progressive and irreversible neurological damage, physical and intellectual development lag or even death, and serious harm to the family and society. The screening of neonatal IEMs by tandem mass spectrometry (MS/MS) is an effective method for early diagnosis and presymptomatic treatment to prevent severe permanent sequelae and death. A total of 111,986 healthy newborns and 7,461 hospitalized high-risk infants were screened for IEMs using MS/MS to understand the characteristics of IEMs and related gene mutations in newborns and high-risk infants in Liuzhou. Positive samples were analyzed by Sanger sequencing or next-generation sequencing. The results showed that the incidence of IEMs in newborns in the Liuzhou area was 1/3,733, and the incidence of IEMs in high-risk infants was 1/393. Primary carnitine deficiency (1/9,332), phenylketonuria (1/18,664), and isovaleric acidemia (1/37,329) ranked the highest in neonates, while citrullinemia type II ranked the highest in high-risk infants (1/1,865). Further, 56 mutations of 17 IEMs-related genes were found in 49 diagnosed children. Among these, HPD c.941T > C, CBS c.1465C > T, ACADS c.337G > A, c.1195C > T, ETFA c.737G > T, MMACHC 1076bp deletion, PCCB c.132-134delGACinsAT, IVD c.548C > T, c.757A > G, GCDH c.1060G > T, and HMGCL c.501C > G were all unreported variants. Some related hotspot mutations were found, including SLC22A5 c.51C > G, PAH c.1223G > A, IVD c.1208A > G, ACADS c.625G > A, and GCDH c.532G > A. These results show that the overall incidence of IEMs in the Liuzhou area is high. Hence, the scope of IEMs screening and publicity and education should be expanded for a clear diagnosis in the early stage of the disease.
Introduction
Inborn errors of metabolism (IEMs), also known as inherited metabolic diseases, is a group of hereditary diseases caused by genetic defects, which lead to the functional defects of some enzymes, carriers, receptors, and other proteins needed to maintain normal metabolism. IEMs result in the disorder of biochemical metabolic pathways, accumulation of intermediate or bypass metabolites, or a lack of terminal metabolites. There are several forms of IEMs, including amino acid metabolism disorders (including urea cycle disorders, branched-chain amino acid metabolism abnormalities, and sulfur amino acid metabolism disorders), organic acidemia, fatty acid oxidation metabolism disorders, glucose metabolism disorders, and other diseases. Dozens of genetic metabolic diseases, such as amino acid metabolic disorders, organic acid metabolic disorders, and fatty acid oxidation disorders, can be screened for simultaneously with high flux and high accuracy by determining the amino acid and acylcarnitine content in neonatal dried blood on filter paper. This method has gradually become popular in China in recent years. A large number of genetic metabolic diseases can be detected at an early stage in newborns due to the wide popularization of tandem mass spectrometry in China. The incidence rate of IEMs varies among different countries and populations worldwide (Webster et al., 2003; Yoon et al., 2005; Sanderson et al., 2006; Dhondt, 2010; Lindner et al., 2011; Lim et al., 2014; Hassan et al., 2016; Karaceper et al., 2016; Yunus et al., 2016; Alfadhel et al., 2017; Shibata et al., 2018).
The incidence of IEMs also varies greatly among the different cities and regions within China (Guo et al., 2018; Wang et al., 2019). Significant differences in IEMs spectra, prevalence, and genetic characteristics exist among the different regions and populations in China. The Liuzhou area has 48 ethnic minorities, and the custom of intermarriage among these ethnic groups is widespread. In Liuzhou, newborn screening for congenital hypothyroidism and phenylketonuria (PKU) began 1994, screening for glucose-6-phosphate dehydrogenase deficiency, thalassemia, and congenital adrenocortical hyperplasia began in 2000, and tandem mass spectrometry screening for genetic metabolic diseases began in 2012. At present, the screening coverage rate of genetic metabolic diseases is about 50% of newborns in Liuzhou, and it is expected that the tandem mass spectrometry screening of IEMs in Liuzhou area will reach full coverage in newborns in 2025. In this study, the amino acid and acylcarnitine profiles of 111,986 newborns born in Liuzhou from December 2012 to June 2020 and 7,461 hospitalized children with suspected genetic metabolic diseases were screened, and then diagnoses were confirmed by first-generation sequencing or high-throughput sequencing. Finally, 30 cases of genetic metabolic diseases were diagnosed in 111,986 newborns, at an incidence rate of 1/3,733; 19 cases were diagnosed in high-risk infants, at an incidence rate of 1/393. Further, 56 mutations of 17 IEMs-related genes were found in 49 diagnosed children. What is noteworthy is that the incidence of primary carnitine deficiency (PCD) is the highest in Liuzhou, about 1/9,332, which is higher than that reported in other areas of China. SLC22A5 gene c.51C > G may be a hotspot mutation in Liuzhou, but whether it is related to ethnic groups in this area needs to be further explored in the future.
Materials and Methods
Ethical Approval
This study was approved by the Ethical Committee of Liuzhou Maternity and Child Health Hospital, and informed consent of the parents.
Study Cohort and Specimen Collection
From December 2012 to June 2020, The heel blood of newborns 3 days after birth and high-risk infants was collected, dripped on filter paper (Waterman Company, S&S903#, Britain), and dried naturally at room temperature. Dried blood samples from 111,986 newborns and 7,461 hospitalized children with suspected genetic metabolic diseases were collected. Among the 111,986 newborns, there were 53,753 Han, 40,763 Zhuang, 10,394 Dong, 6,217 Miao and 859 Yao. There were 5,596 Han, 4,594 Zhuang, 2,096 Zhuang, 543 Dong, 130 Miao and 98 Yao among the 7,461 high risk infants.
Tandem Mass Spectrometry Screening
Dried blood spots were pretreated using a non-derivatized MS/MS kit according to the manufacturer instructions (FengHua, China) and then analyzed using a tandem mass spectrometry system (ABI3200, United States). The screening indicators included: free carnitine (C0); 11 amino acids and 33 acylcarnitines (Table 1). Repeated MS/MS tests were performed on the recalled positive samples, and biochemical tests or genetic analysis were performed on the re-examined positive cases.
Genetic Analysis
For all positive samples, 2 mL of whole-blood samples were collected from the newborns and their parents after obtaining the informed consent of the guardian and the approval of the hospital ethics committee. Genomic DNA was extracted to amplify all exons and part of the intron region at the exon boundary. The amplified products were sequenced by Sanger sequencing and compared with the human genome gene sequence. Some genetic metabolic diseases, such as PKU and methylmalonic acidemia (MMA), which are caused by multiple genes, were screened by high-throughput sequencing by Jiajian Medicine (Guangzhou, China) and then verified by Sanger sequencing.
Bioinformatics Analysis
The Human Gene Mutation Database,1 single-nucleotide polymorphism,2 ExAC,3 and 1,000 Genomes Project4 were queried for the newly discovered variation sites, and protein functions were predicted using Sorting Intolerant from Tolerant and PolyPhen-2 bioinformatics software. At the same time, the pathogenicity was interpreted according to the standards and guidelines for the interpretation of gene variation issued by the American Society of Medical Genetics and Genomics in 2015.
Statistical Analysis
Statistical analysis was performed using SPSS17.0 version. The difference of categorical data was compared using Chi-square test. The difference of measurement data was compared by analysis of variance. p < 0.05 was considered to be statistical significance. Primary screening positive rate = number of positive cases at primary screening/number of children screened × 100%; positive predictive value = number of confirmed cases/number of positive recalls × 100%; incidence = number of confirmed cases/number of children screened × 100%.
Results
Screening Results of Amino Acids and Acylcarnitines in Newborns
Among the 111,986 newborns, 2,464 tested positive at the primary screening, with a positive rate of 2.2% (2,464/111,986), and 2,275 suspected positive cases were recalled, with a positive recall rate of 92.3% (2,275/2,464). Finally, 30 cases of genetic metabolic diseases were clinically diagnosed (the disease spectrum is shown in Table 2, and the MS/MS results are shown in Table 3), and the positive predictive value was 1.3%. Preliminary statistics showed that the incidence of genetic metabolic diseases in the Liuzhou area was 1/3,733. The total incidence of amino acid metabolic diseases was 1/13,998, of which there were six cases of phenylalanine hydroxylase deficiency (PHD) (1/18,664; highest), one case of hyperhomocysteinemia (1/111,986), and one case of citrullinemia type l (CTLN2; 1/111,986).
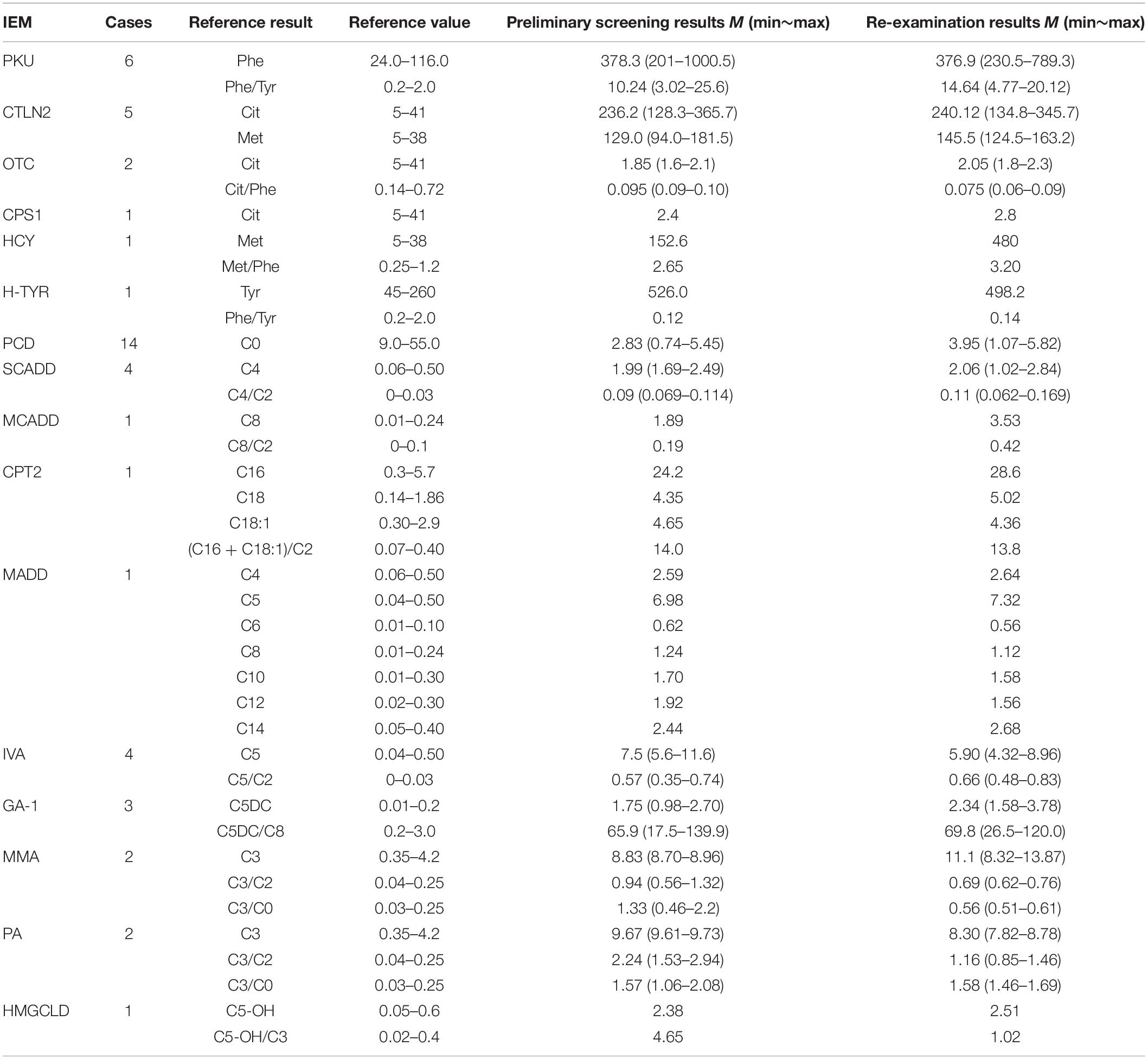
Table 3. Results of tandem mass spectrometry in 49 children with genetic metabolic diseases (μmol/L).
Seven cases of organic acidemia (1/15,998) were reported, of which isovaleric acidemia (IVA) was the highest (1/37,329); besides, there were one case of MMA (1/111,986), one case of glutaric acidemia type I (1/111,986), and one case of 3-hydroxy-3-methyl glutaric acidemia (1/111,986). Fifteen cases of fatty acid metabolism disorder (1/7,466) were found, of which PCD was the highest (1/9,332); besides, there were two cases of short-chain acyl-CoA dehydrogenase deficiency (SCADD; 1/55,993) and one case of medium-chain acyl-CoA dehydrogenase deficiency (MCADD; 1/111,986) (Table 2 and Figure 1).
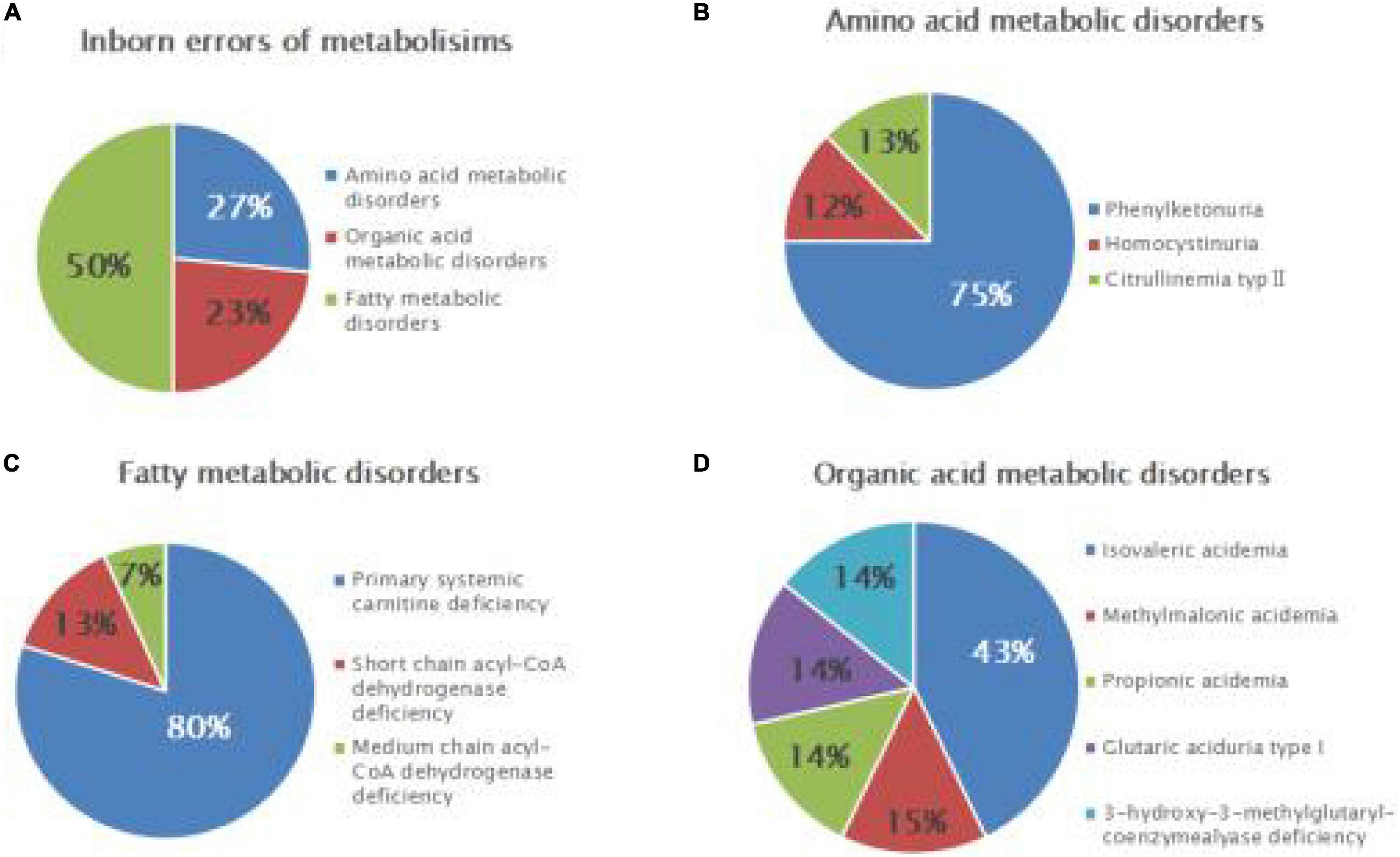
Figure 1. Disease spectrum and distribution of inborn errors of metabolism (IEMs) in newborns. Relative proportions of different categories of IEMs (A), amino acid metabolic disorders (B), fatty acid metabolic disorders (C), and organic acid metabolic disorders (D).
Diagnosis of IEMs in High-Risk Infants
Among 7,461 hospitalized high-risk infants with suspected genetic metabolic diseases, 19 cases were diagnosed as genetic metabolic diseases, including eight cases of amino acid metabolic diseases (1/933), of which there were four cases of CTLN2 (1/1,865), two cases of ornithine carbamoyltransferase deficiency (1/3,731), one case of tyrosinemia (H-TYR; 1/7,461), and one case of carbamyl phosphate synthase I deficiency (CPS1; 1/7,461). Further, six cases of fatty acid metabolism disorder (1/1,244) were found, including two cases of PCD (1/3,731), two cases of SCADD (1/3,731), one case of carnitine palmitoyltransferase II deficiency (CPT2; 1/7,461), and one case of glutaric acidemia type II (1//7,461). Moreover, five cases of organic acidemia (1/1,492) were reported, including two cases of glutaric acidemia type I (1/3,731), one case of propionic acidemia (PA; 1/7,461), one case of IVA (1/7,461), and one case of MMA (1/,7461) (Table 4 and Figure 2).
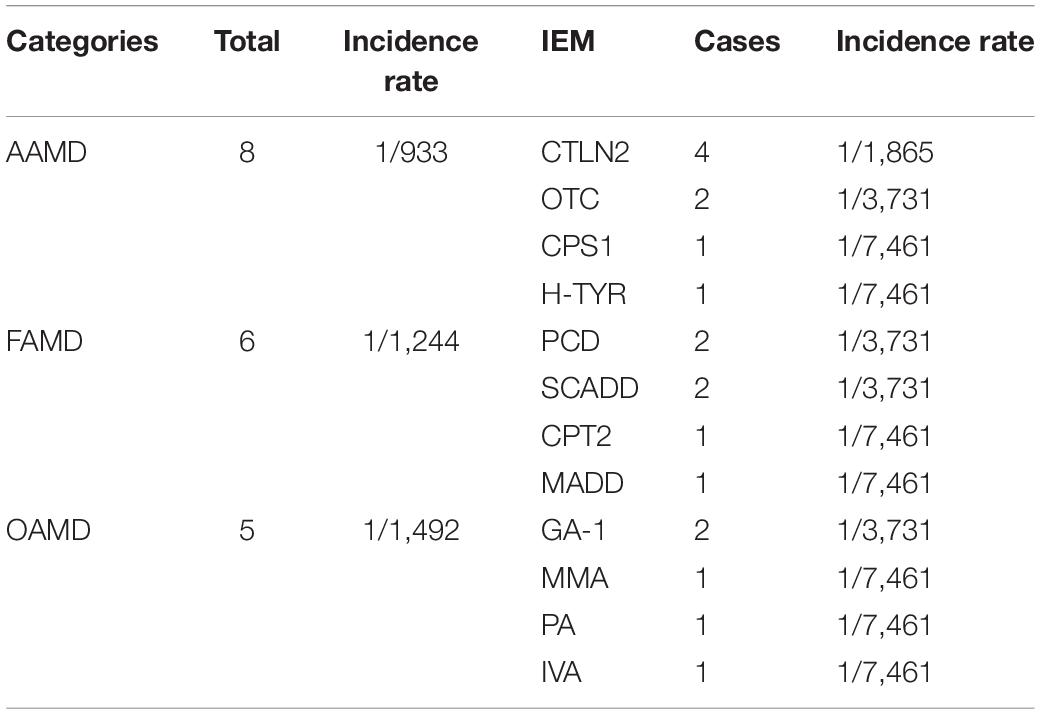
Table 4. Disease spectrum of 19 children with genetic metabolic diseases screened from 7,461 high-risk infants.
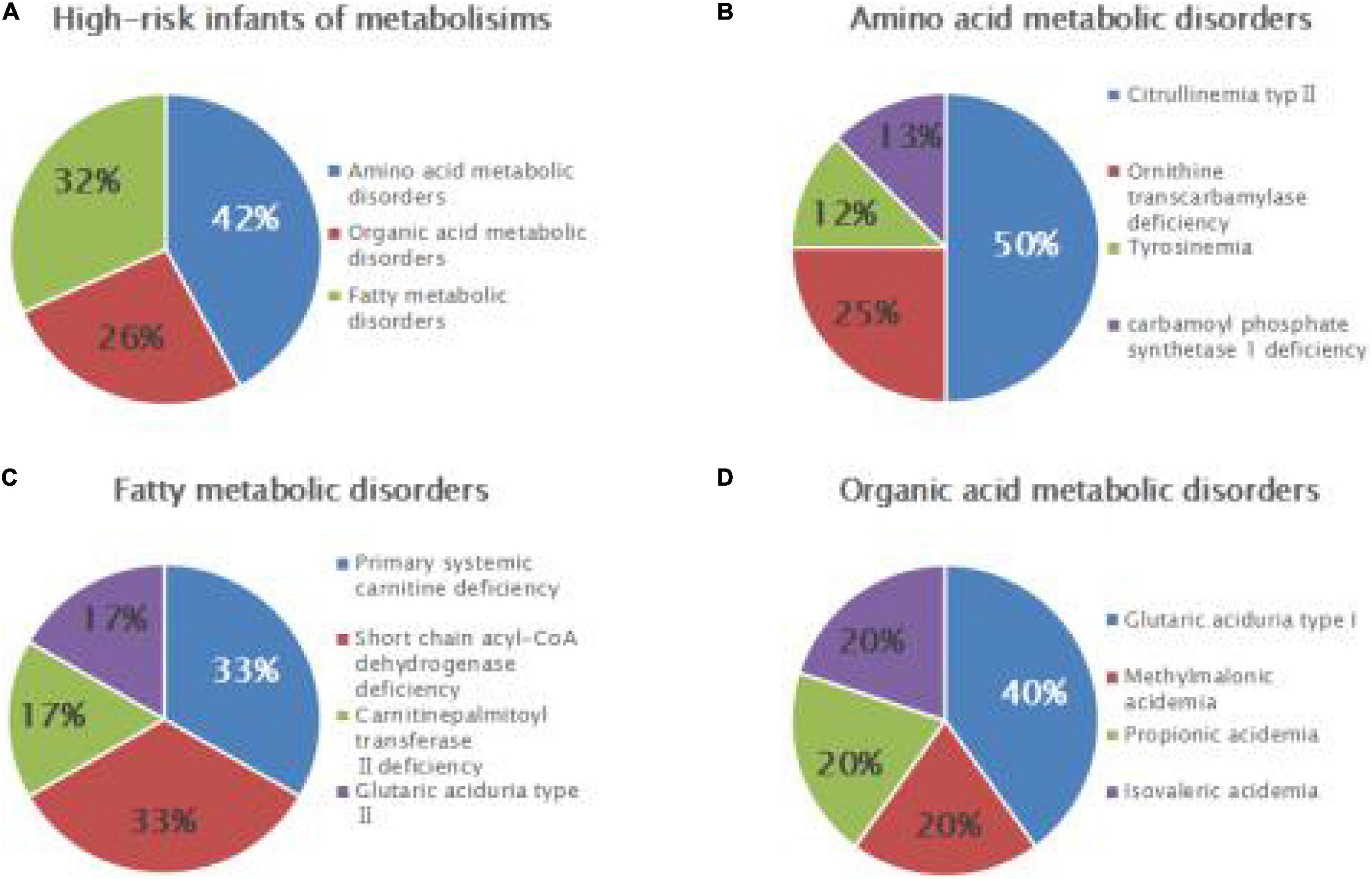
Figure 2. Disease spectrum and distribution of IEMs in high-risk infants. Relative proportions of different categories of IEMs (A), amino acid metabolic disorders (B), fatty acid metabolic disorders (C), and organic acid metabolic disorders (D) in high-risk infants.
Gene Detection Results
The gene detection results revealed 56 mutations of 17 IEMs-related genes in 49 diagnosed children. A total of 11 kinds of 26 mutation sites were detected in the SLC22A5 gene: c.51C > G, c.976C > T, c.1411C > T, c.1195C > T, c.760C > T, c.919delG, c.1400C > G, c.517delC, c.839C > T, c.505C > T, and c.338G > A. A total of eight kinds of 12 mutation sites were detected in the PAH gene: c.1174T > A, c.1223G > A, c.208_210del, c.611A > G, c.331C > T, c.28G > A, c.320A > G, and c.721C > T. Also, five mutation types and eight mutation sites were found in the IVD gene: c.158G > A, c.214G > A, c.548C > T, c.757A > G, and 1208A > G. The ACADS gene had six kinds of eight mutation sites: c.268G > A, c.320G > A, c.337G > A, c.625G > A, c.1031A > G, and c.1148G > A. A total of three kinds and six mutation sites were detected in the GCDH gene: c.532G > A, c.655G > A, and c.1060G > T. The detection of other disease-related genes is shown in Tables 5, 6. Among these, HPD gene c.941T > C, CBS gene c.1465C > T, ACADS gene c.337G > A and c.1195C > T, ETFA gene c.737G > T, MMACHC gene 1076-bp deletion, PCCB gene c.132-134delGACinsAT, IVD gene c.548C > T and c.757A > G, GCDH gene c.1060G > T, and HMGCL gene c.501C > G were all unreported variants.
Discussion
The incidence of genetic metabolic diseases varies across different countries and regions worldwide. In this study, the overall incidence of genetic metabolic diseases in Liuzhou, Guangxi, was about 1/3,733, which was similar to that reported in other domestic studies. PCD had the highest incidence of all genetic metabolic diseases in newborns in Liuzhou, and ranked first among the fatty acid metabolic disorders, at 1/9,332, which is higher than that reported in other parts of China. The global prevalence rate of PCD is about 1/20,000–1/120,000 (di San Filippo et al., 2008). At present, more than 180 kinds of SLC22A5 gene mutations have been reported, most of which are missense mutations, followed by non-sense mutations and frameshift mutations, while splice site mutations are relatively rare; the most frequent mutation is exon 1 (Li et al., 2010). The hotspot mutations of the SLC22A5 gene vary among different races and regions worldwide. R282X mutations are common in Caucasians (Burwinkel et al., 1999; Vaz et al., 1999; Wang et al., 1999), and W132X and W283C mutations are most common in East Asian populations (Koizumi et al., 1999; Nezu et al., 1999; Tang et al., 1999). R254X mutations were found in children with PCD in Taiwan and Shanghai (Tang et al., 2002; Lee et al., 2010). In this study, 11 kinds of 26 mutation sites were detected in newborns and high-risk infants: c.51C > G, c.976C > T, c.1411C > T, c.1195C > T, c.760C > T, c.919delG, c.1400C > G, c.517delC, c.839C > T, c.505C > T, and c.338G > A. Inconsistent with previously reported hotspots of mutations, c.51C > G mutations occurred as often as eight times in 14 children in this study, with a frequency of about 28.6%. At the same time, 2,093 newborns were screened for the SLC22A5 gene mutation, and 24 cases of reported mutation carriers were detected, of which the c.51C > G mutation occurred as often as 13 times, accounting for 54% of the carriers. The c.51C > G mutation had a high carrying rate in the population of Liuzhou and was the most frequent mutation of the SLC22A5 gene reported in this study. Primary screening of 119,986 newborns resulted in 2,464 positive tests, from these newborns 2,275 were examined for a second time and 30 positive cases were established. The main reasons for the high false positive rate of tandem mass spectrometry are as follows: The first is the quality of the blood tablets to be tested. For example, whether the blood collection time is standardized, whether the blood collection process is polluted, and the transportation time of blood filter paper is too long. The second is the quality control of the experimental process. Such as reagent preparation, quality control material selection. Finally, the quality audit and interpretation of the experimental results, there may be subjective factors in the interpretation of laboratory results.
PHD had the second highest incidence of all genetic metabolic diseases and ranked first among the amino acid metabolic disorders. In this study, PHD was detected in six newborns, at a rate of 1/18,664. Studies have shown that the incidence of PHD in China is 1/3,000–1/16,000 and incidences are higher in northern China than in southern China. The Liuzhou area of Guangxi is located in the south of China, and the detection rate of PKU is significantly lower than that in other areas in the north of China. So far, more than 800 PAH gene mutations have been found in patients with PAH deficiency. The PAH gene has some hotspot mutations, which vary among different populations. c.728G > A is the most common in China and Korea (Lee et al., 2008; Zhang et al., 2018), the c.1222C > T mutation is the most common in the United States (Kaul et al., 1994), c.1238G > C is common in Japan (Okano et al., 2011), and IVS10-11G > A is the most common in Iran and Spain (Bueno et al., 2013; Rastegar Moghadam et al., 2018). In the present study, eight kinds of 12 mutation sites were found in the PHA gene: c.1174T > A, c.1223G > A, c.208_210del, c.611A > G, c.331C > T, c.728G > A, c.320A > G, and c.721C > T. Further, c.1223G > A was the most common PAH mutation site, accounting for 25% of all mutation types, potentially representing a hot spot mutation in this region.
IVA had the third highest incidence of all genetic metabolic diseases and ranked first among organic acidemia disorders, with a detection rate of 1/37,329, which was higher than that reported in previous studies. IVA occurs due to the loss of the functional activity of isovaleryl-CoA dehydrogenase caused by IVD gene mutation, resulting in leucine metabolism disorder. The disease was first reported by Tanaka et al. (1966). Significant differences in the incidence of IVA exist among different regions and populations: about 1/250,000 in the United States (Frazier et al., 2006), about 1/365,000 in Taiwan (Lin et al., 2007), and about 1/67,000 in Germany (Ensenauer et al., 2011). Some thermal mutations were found in the IVD gene in different regions. The c.932C > T mutation is the main mutation in Germany and the United States (Vockley and Ensenauer, 2006). The c.457-3_2CA > GG mutation is common in Korea (Lee et al., 2007), and the c.149G > C and c.1208A > G mutations are common in Taiwan (Lin et al., 2007). The Chinese scholar Wenjuan Qiu reported a case of IVA with compound heterozygous mutations of the IVD gene, c.149G > A and c.466G > C (Qiu et al., 2008). Shiyue Mei reported two cases of IVA and detected compound heterozygous mutations of the IVD gene, c.1195G > C and c.466-3_466-2delinsGG, and the homozygous mutation c.1208A > G (Mei et al., 2018). Xiyuan Li reported three cases of children with atypical IVA (Li et al., 2014) in which six mutation types were detected: c.157C > T, c.214G > A, c. 1183C > G, c.1208A > G, c.1039G > A, and c.1076A > G. In this study, five mutation types and eight mutation sites of the IVD gene were detected in four children with IVA: c.158G > A, c.214G > A, c.548C > T, c.757A > G, and 1208A > G, of which c.548C > T and c.757A > G were not included in the database. The frequency of the 1208A > G mutation was the highest (appearing four times in this study). In a previous study, high-throughput sequencing was carried out in 2,095 normal newborns in this area, and 10 cases of IVD gene carriers were detected, of which as many as five cases were c.1208A > G mutation carriers. At present, no reports are available on the mutation spectrum and hot spots of patients with IVA in China. However, the c.1208A > G mutation has a high frequency in China, according to the IVD gene mutation types detected in four children with IVA in this study and other domestic studies. Whether the mutation is a hotspot mutation in the Chinese population or local population needs to be further verified by expanding the sample size.
In this study, the detection rate of SCADD in newborns was about 1/55,993, and the rate in Suzhou, China, was about 1/28,690 (Wang et al., 2019). Zytkovicz reported that the global incidence of SCADD was about 1/25,000–1/45,000 (Zytkovicz et al., 2001). More than 70 types of gene mutations have been reported in ACADS. Gregersen et al. (2008) and Jethva et al. (2008) found that c.625G > A and c.511C > T mutations were dominant in Europe and the United States. The two mutation sites were screened in 694 newborns in the United States, revealing that the c.625G > A mutation accounted for 22% of all detected alleles. C.511C > T accounted for 3%. The incidence of SCADD was significantly lower in the Asian population than in Caucasians, while the carrying rate of the c.625G > A mutation in Hispanics was as high as 30%. In this study, six mutation sites of the ACADS gene were detected in newborns and high-risk infants: c.268G > A, c.320G > A, c.337G > A, c.625G > A, c.1031A > G, c.1148G > A, and c.1195C > T, of which c.337G > A and c.1195C > T were unreported mutation sites; the c.625G > A mutation accounted for 25%, which was consistent with reports from Gregersen et al. (2008) and Jethva et al. (2008). At present, there is still controversy about whether to screen SCADD and some variants of ACADS gene. At the same time, whether the impact of SCADD on the population is the same in different countries or different regions needs to be further observed in the later stage.
One case of glutaric acidemia type I was detected in newborns, and the detection rate was 1/111,986. Two cases were detected in high-risk infants, and the detection rate was 1/3,731. The incidence of glutaric acidemia type I in newborns is about 1/100,000 (Kölker et al., 2011), with ethnic and regional differences. The incidence of glutaric acidemia type I was about 1/130,000 in the United States (Basinger et al., 2006). Of the 129,415 newborns screened, only 2 cases were diagnosed with glutaric acidemia type I in Zhejiang province (Yang et al., 2011). At present, more than 200 GCDH gene variants have been reported, with obvious heterogeneity in different regions and populations. The common variation in Pennsylvania is c.1296C > T (Korman et al., 2007), while the hotspot of variation in Taiwan and Hong Kong is IVS10-2A > C (Tang et al., 2000; Shu et al., 2003; Hsieh et al., 2008). At present, no hotspots of variation exist in the Chinese mainland area. Lin et al. (2018) analyzed gene variations in five cases of glutaric aciduria type I (GA-I); c.1244-2A > C mutation frequency was the highest. Three variants of the GCDH gene were found by Sanger sequencing: c.532G > A, c. 655G > A, and c.1060G > T, of which c.532G > A appeared four times, which might be a hotspot variation in this area; c.1060G > T variation has not been reported.
In addition, other types of genetic metabolic diseases were also detected, such as hyperhomocysteinemia, MCADD, MMA, propionemia, 3-hydroxy-3-methylglutaric acidemia, ornithine carbamoyltransferase deficiency, H-TYR, CPS1, CPT2, and glutaric acidemia type II. Due to the small number of cases, these diseases were not discussed in this study. In this study, only one mutation was detected in 2 cases of OTC gene and 1 case of PCCB gene, which may be caused by large deletion, deep intronic mutations or promoter mutations. To sum up, after screening newborns and some high-risk infants in this area for genetic metabolic diseases, the detection rate and disease spectrum of some diseases were preliminarily confirmed and some mutation hotspots and new mutations were detected. These mutation hotspots may be potential candidate sites for gene screening and may expand the gene mutation spectrum of genetic metabolic diseases. The findings of this study may have a certain reference value for genetic counseling and prenatal diagnosis of genetic metabolic diseases.
Data Availability Statement
The original contributions presented in the study are included in the article/supplementary material, further inquiries can be directed to the corresponding author/s.
Ethics Statement
The studies involving human participants were reviewed and approved by the Ethics Committee of Liuzhou Maternal and Child Health Hospital. Written informed consent to participate in this study was provided by the participants’ legal guardian/next of kin. Written informed consent was obtained from the individual(s), and minor(s)’ legal guardian/next of kin, for the publication of any potentially identifiable images or data included in this article.
Author Contributions
JT formulated the study, analyzed the data, wrote the manuscript, and designed the tables. DC, RoC, LP, JY, DY, LH, TY, HN, and JW performed screening by MS/MS and collected the data. ReC provided intellectual thoughts, revised the manuscript, and led the project. All authors contributed to the article and approved the submitted version.
Funding
This study was supported by grants from the Liuzhou Scientific Research and Technology Development Plan Project (2017BH20313), the Liuzhou Science and Technology Major Special Project (2018AH10501 and 2020NBAB0803), and the Guangxi Department of Health Project (Z20170530).
Conflict of Interest
The authors declare that the research was conducted in the absence of any commercial or financial relationships that could be construed as a potential conflict of interest.
Publisher’s Note
All claims expressed in this article are solely those of the authors and do not necessarily represent those of their affiliated organizations, or those of the publisher, the editors and the reviewers. Any product that may be evaluated in this article, or claim that may be made by its manufacturer, is not guaranteed or endorsed by the publisher.
Acknowledgments
We thank International Science Editing (http://www.internationalscienceediting.com) for editing this manuscript.
Abbreviations
CPS1, Carbamyl phosphate synthase 1 deficiency; CPT2, carnitine palmitoyltransferase II deficiency; CTLN2, citrullinemia; GA-I, glutaric aciduria type I; HCY, homocystinuria; HMGCLD, 3-hydroxy-3-methylglutaryl-coenzyme A lyase deficiency; H-TYR, tyrosinemia; IVA, isovaleric acidemia; MADD, multiple acyl-CoA dehydrogenase deficiency; MCADD, medium-chain acyl-CoA dehydrogenase deficiency; MMA, methylmalonic acidemia; OTC, ornithine transcarbamylase deficiency; PA, propionic acidemia; PCD, primary carnitine deficiency; PKU, phenylketonuria; SCADD, short-chain acyl-CoA dehydrogenase deficiency.
Footnotes
- ^ http://www.hgmd.cf.ac.uk/ac/
- ^ http://www.ncbi.nlm.nih.gov/
- ^ http://exac.broadinstitute.org/
- ^ https://www.internationalgenome.org/
References
Alfadhel, M., Al Othaim, A., Al Saif, S., Al Mutairi, F., Alsayed, M., Rahbeeni, Z., et al. (2017). Expanded newborn screening program in Saudi Arabia: incidence of screened disorders. J. Paediatr. Child. Health 53, 585–591. doi: 10.1111/jpc.13469
Basinger, A. A., Booker, J. K., Frazier, D. M., Koeberl, D. D., Sullivan, J. A., and Muenzer, J. (2006). Glutaric acidemia type 1 in patients of Lumbee heritage from North Carolina. Mol. Genet. Metab. 88, 90–92. doi: 10.1016/j.ymgme.2005.12.008
Bueno, M. A., González-Lamuño, D., Delgado-Pecellín, C., Aldámiz-Echevarría, L., Pérez, B., Desviat, L. R., et al. (2013). Molecular epidemiology and genotypephenotype correlation in phenylketonuria patients from South Spain. J. Hum. Genet. 58, 279–284. doi: 10.1038/jhg.2013.16
Burwinkel, B., Kreuder, J., Schweitzer, S., Vorgerd, M., Gempel, K., Gerbitz, K. D., et al. (1999). Carnitine transporter OCTN2 mutations in systemic primary carnitine deficiency: a novel Arg169Gln mutation and a recurrent Arg282ter mutation associated with an unconventional splicing abnormality. Biochem. Biophys. Res. Commun. 261, 484–487. doi: 10.1006/bbrc.1999.1060
Dhondt, J. L. (2010). Expanded newborn screening: social and ethical issues. J. Inherit. Metab. Dis. 33, S211–S217. doi: 10.1007/s10545-010-9138-y
di San Filippo, C. A., Taylor, M. R. G., Mestroni, L., Botto, L. D., and Longo, N. (2008). Cardiomyopathy and carnitine deficiency. Mol. Genet. Metab. 94, 162–166. doi: 10.1016/j.ymgme.2008.02.002
Ensenauer, R., Fingerhut, R., Maier, E. M., Polanetz, R., Olgemöller, B., Röschinger, W., et al. (2011). Newborn screening for isovaleric acidemia using tandem mass spectrometry: data from 1.6 million newborns. Clin. Chem. 57, 623–626. doi: 10.1373/clinchem.2010.151134
Frazier, D. M., Millington, D. S., McCandless, S. E., Koeberl, D. D., Weavil, S. D., Chaing, S. H., et al. (2006). The tandem mass spectrometry newborn screening experience in North Carolina: 1997–2005. J. Inherit. Metab. Dis. 29, 76–85. doi: 10.1007/s10545-006-0228-9
Gregersen, N., Andresen, B. S., Pedersen, C. B., Olsen, R. K. J., Corydon, T. J., and Bross, P. (2008). Mitochondrial fatty acid oxidation defects – remaining challenges. J. Inherit. Metab. Dis. 31, 643–657. doi: 10.1007/s10545-008-0990-y
Guo, K., Zhou, X., Chen, X., Wu, Y., Liu, C., and Kong, Q. (2018). Expanded newborn screening for inborn errors of metabolism and genetic characteristics in a Chinese population. Front. Genet. 9:122. doi: 10.3389/fgene.2018.00122
Hassan, F. A., El-Mougy, F., Sharaf, S. A., Mandour, I., Morgan, M. F., Selim, L. A., et al. (2016). Inborn errors of metabolism detectable by tandem mass spectrometry in Egypt: the first newborn screening pilot study. J. Med. Screen. 23, 124–129. doi: 10.1177/0969141315618229
Hsieh, C.-T., Hwu, W.-L., Huang, Y.-T., Huang, A.-C., Wang, S.-F., Hu, M.-H., et al. (2008). Early detection of glutaric aciduria type I by newborn screening in Taiwan. J. Formos. Med. Assoc. 107, 139–144. doi: 10.1016/S0929-6646(08)60127-8
Jethva, R., Bennett, M. J., and Vockley, J. (2008). Short-chain acyl-coenzyme A dehydrogenase deficiency. Mol. Genet. Metab. 95, 195–200. doi: 10.1016/j.ymgme.2008.09.007
Karaceper, M. D., Chakraborty, P., Coyle, D., Wilson, K., Kronick, J. B., Hawken, S., et al. (2016). The health system impact of false positive newborn screening results for medium-chain acyl-CoA dehydrogenase deficiency: a cohort study. Orphanet. J. Rare Dis. 11:12. doi: 10.1186/s13023-016-0391-5
Kaul, R., Matalon, R., Allen, R., Fisch, R. O., Michals, K., Petrosky, A., et al. (1994). Frequency of 12 mutations in 114 children with phenylketonuria in the Midwest region of the USA. J. Inherit. Metab. Dis. 17, 356–358. doi: 10.1007/BF00711829
Koizumi, A., Nozaki, J., Ohura, T., Kayo, T., Wada, Y., Nezu, J., et al. (1999). Genetic epidemiology of the carnitine transporter OCTN2 gene in a Japanese population and phenotypic characterization in Japanese pedigrees with primary systemic carnitine deficiency. Hum. Mol. Genet. 8, 2247–2254. doi: 10.1093/hmg/8.12.2247
Kölker, S., Christensen, E., Leonard, J. V., Greenberg, C. R., Boneh, A., Burlina, A. B., et al. (2011). Diagnosis and management of glutaric aciduria type I – revised recommendations. J. Inherit. Metab. Dis. 34, 677–694. doi: 10.1007/s10545-011-9289-5
Korman, S. H., Jakobs, C., Darmin, P. S., Gutman, A., van der Knaap, M. S., Ben-Neriah, Z., et al. (2007). Glutaric aciduria type 1: clinical, biochemical and molecular findings in patients from Israel. Eur. J. Paediatr. Neurol. 11, 81–89. doi: 10.1016/j.ejpn.2006.11.006
Lee, N.-C., Leung-Sang Tang, N., Chien, Y.-H., Chen, C.-A., Lin, S.-J., Chiu, P.-C., et al. (2010). Diagnoses of newborns and mothers with carnitine uptake defects through newborn screening. Mol. Genet. Metab. 100, 46–50. doi: 10.1016/j.ymgme.2009.12.015
Lee, Y. W., Lee, D. H., Kim, N. D., Lee, S. T., Ahn, J. Y., Choi, T. Y., et al. (2008). Mutation analysis of PAH gene and characterization of a recurrent deletion mutation in Korean patients with phenylketonuria. Exp. Mol. Med. 40, 533–540. doi: 10.3858/emm.2008.40.5.533
Lee, Y.-W., Lee, D. H., Vockley, J., Kim, N.-D., Lee, Y. K., and Ki, C.-S. (2007). Different spectrum of mutations of isovaleryl-CoA dehydrogenase (IVD) gene in Korean patients with isovaleric acidemia. Mol. Genet. Metab. 92, 71–77. doi: 10.1016/j.ymgme
Li, F.-Y., El-Hattab, A. W., Bawle, E. V., Boles, R. G., Schmitt, E. S., Scaglia, F., et al. (2010). Molecular spectrum of SLC22A5 (OCTN2) gene mutations detected in 143 subjects evaluated for systemic carnitine deficiency. Hum. Mutat. 31, E1632–E1651. doi: 10.1002/humu.21311
Li, X.-Y., Hua, Y., Ding, Y., Wu, T.-F., Song, J.-Q., Liu, Y., et al. (2014). Clinical and genetic features of three patients with non-classical isovaleric aciduria. Lin Chuang Er Ke Za Zhi. 32, 1107–1111. doi: 10.3969/j.issn.1000-3606.2014.12.03
Lim, J. S., Tan, E. S., John, C. M., Poh, S., Yeo, S. J., Ang, J. S., et al. (2014). Inborn Error of Metabolism (IEM) screening in Singapore by electrospray ionization-tandem mass spectrometry (ESI/MS/MS): An 8 year journey from pilot to current program. Mol. Genet. Metab. 113, 53–61. doi: 10.1016/j ymgme.2014.07.018
Lin, W.-D., Wang, C.-H., Lee, C.-C., Lai, C.-C., Tsai, Y., and Tsai, F.-J. (2007). Genetic mutation profile of isovaleric acidemia patients in Taiwan. Mol. Genet. Metab. 90, 134–139. doi: 10.1016/j.ymgme.2006.08.011
Lin, Y.-M., Han, M.-Y., Zheng, Z.-Z., Lin, W.-H., Yu, K., and Fu, Q. (2018). Detection of GCDH mutations in five Chinese patients with glutaric acidemia type I. Zhong Hua Yi Xue Yi Chuan Xue Za Zhi. 35, 39–42. doi: 10.3760/cma.j.issn.1003-9406.2018.01.008
Lindner, M., Gramer, G., Haege, G., Fang-Hoffmann, J., Schwab, K. O., Tacke, U., et al. (2011). Efficacy and outcome of expanded newborn screening for metabolic diseases – report of 10 years from South-West Germany. Orphanet. J. Rare Dis. 6:44. doi: 10.1186/1750-1172-6-44
Mei, S.-Y., Bai, N., Hu, S., Li, N., Liu, L.-N., and Kong, X.-D. (2018). Analysis of gene variation in two families with isovalerate acidemia and prenatal diagnosis of one case. Zhong Hua Wei Chan Yi Xue Za Zhi. 21, 31–35. doi: 10.3760/cma.j.issn.1007-9408.2018.01.007
Nezu, J., Tamai, I., Oku, A., Ohashi, R., Yabuuchi, H., Hashimoto, N., et al. (1999). Primary systemic carnitine deficiency is caused by mutations in a gene encoding sodium ion-dependent carnitine transporter. Nat. Genet. 21, 91–94. doi: 10.1038/5030
Okano, Y., Kudo, S., Nishi, Y., Sakaguchi, T., and Aso, K. (2011). Molecular characterization of phenylketonuria and tetrahydrobiopterin-responsive phenylalanine hydroxylase deficiency in Japan. J. Hum. Genet. 56, 306–312. doi: 10.1038/jhg.2011.10
Qiu, W.-J., Gu, X.-F., Ye, J., Han, L.-S., Bai, H.-T., Wang, X., et al. (2008). Clinical and mutational study of a Chinese infant with isovaleric acidemia. Zhong Hua Er Ke Za Zhi. 46:52630. doi: 10.3321/j.issn:0578-1310.2008.07.014
Rastegar Moghadam, M., Shojaei, A., Babaei, V., Rohani, F., and Ghazi, F. (2018). Mutation analysis of Phenylalanine hydroxylase gene in Iranian patients with Phenylketonuria. Med. J. Islam. Repub. Iran 32:21. doi: 10.14196/mjiri.32.21
Sanderson, S., Green, A., Preece, M. A., and Burton, H. (2006). The incidence of inherited metabolic disorders in the West Midlands, UK. Arch. Dis. Child. 91, 896–899. doi: 10.1136/adc.2005.091637
Shibata, N., Hasegawa, Y., Yamada, K., Kobayashi, H., Purevsuren, J., Yang, Y., et al. (2018). Diversity in the incidence and spectrum of organic acidemias, fatty acid oxidation disorders, and amino acid disorders in Asian countries: selective screening vs. expanded newborn screening. Mol. Genet. Metab. Rep. 16, 5–10. doi: 10.1016/j.ymgmr.2018.05.003
Shu, S. G., Tsai, C. R., Chen, L. H., and Chi, C. S. (2003). Type I glutaric aciduria: phenotypes and genotypes in 5 Taiwanese children. J. Formos. Med. Assoc. 102, 729–732.
Tanaka, K., Budd, M. A., Efron, M. L., and Isselbacher, K. J. (1966). Isovaleric acidemia: a new genetic defect of leucine metabolism. Proc. Natl. Acad. Sci. U S A. 56, 236–242. doi: 10.1073/pnas.56.1.236
Tang, N. L., Ganapathy, V., Wu, X., Hui, J., Seth, P., Yuen, P. M., et al. (1999). Mutations of OCTN2, an organic cation/carnitine transporter, lead to deficient cellular carnitine uptake in primary carnitine deficiency. Hum. Mol. Genet. 8, 655–660. doi: 10.1093/hmg/8.4.655
Tang, N. L., Hui, J., Law, L. K., Lam, Y. Y., Chan, K. Y., Yeung, W. L., et al. (2000). Recurrent and novel mutations of GCDH gene in Chinese glutaric acidemia type I families. Hum. Mutat. 16:446. doi: 10.1002/1098-1004(200011)16:5<446::AID-HUMU14<3.0.CO;2-Y
Tang, N. L. S., Hwu, W. L., Chan, R. T., Law, L. K., Fung, L. M., and Zhang, W. M. (2002). A founder mutation (R254X) of SLC22A5 (OCTN2) in Chinese primary carnitine deficiency patients. Hum. Mutat. 20:232. doi: 10.1002/humu.9053
Vaz, F. M., Scholte, H. R., Ruiter, J., Hussaarts-Odijk, L. M., Pereira, R. R., Schweitzer, S., et al. (1999). Identification of two novel mutations in OCTN2 of three patients with systemic carnitine deficiency. Hum. Genet. 105, 157–161. doi: 10.1007/s004399900105
Vockley, J., and Ensenauer, R. (2006). Isovaleric acidemia: new aspects of genetic and phenotypic heterogeneity. Am. J. Med. Genet. C Semin. Med. Genet. 142C, 95–103. doi: 10.1002/ajmg.c.30089
Wang, T., Ma, J., Zhang, Q., Gao, A., Wang, Q., Li, H., et al. (2019). Expanded newborn screening for inborn errors of metabolism by tandem mass spectrometry in Suzhou, China: disease spectrum, prevalence, genetic characteristics in a Chinese population. Front. Genet. 10:1052. doi: 10.3389/fgene.2019.01052
Wang, Y., Ye, J., Ganapathy, V., and Longo, N. (1999). Mutations in the organic cation/carnitine transporter OCTN2 in primary carnitine deficiency. Proc. Natl. Acad. Sci. U S A. 96, 2356–2360. doi: 10.1073/pnas.96.5.2356
Webster, D. Joint Newborn Screening Committee, Human Genetics Society of Australasia and Royal Australasian College of Physicians (2003). Newborn screening in Australia and New Zealand. Southeast Asian J. Trop. Med. Public Health 34, 69–70.
Yang, L., Yin, H., Yang, R., and Huang, X. (2011). Diagnosis, treatment and outcome of glutaric aciduria type I in Zhejiang Province, China. Med. Sci. Monit. 17, H55–H59. doi: 10.12659/msm.881834
Yoon, H. R., Lee, K. R., Kang, S., Lee, D. H., Yoo, H. W., Min, W. K., et al. (2005). Screening of newborns and high-risk group of children for inborn metabolic disorders using tandem mass spectrometry in South Korea: a three-year report. Clin. Chim. Acta. 354, 167–180. doi: 10.1016/j.cccn.2004.11.032
Yunus, Z. M., Rahman, S. A., Choy, Y. S., Keng, W. T., and Ngu, L. H. (2016). Pilot study of newborn screening of inborn error of metabolism using tandem mass spectrometry in Malaysia: outcome and challenges. J. Pediatr. Endocrinol. Metab. 29, 1031–1039. doi: 10.1515/jpem-2016-0028
Zhang, Z., Gao, J. J., Feng, Y., Zhu, L. L., Yan, H., Shi, X. F., et al. (2018). Mutational spectrum of the phenylalanine hydroxylase gene in patients with phenylketonuria in the central region of China. Scand. J. Clin. Lab. Invest. 78, 211–218. doi: 10.1080/00365513.2018.1434898
Zytkovicz, T. H., Fitzgerald, E. F., Marsden, D., Larson, C. A., Shih, V. E., Johnson, D. M., et al. (2001). Tandem mass spectrometric analysis for amino, organic, and fatty acid disorders in newborn dried blood spots: a two-year summary from the New England Newborn Screening Program. Clin. Chem. 47, 1945–1955.
Keywords: disease spectrum, genetic characteristics, genetic mutation, incidence of IEMs, inborn errors of metabolism, tandem mass spectrometry
Citation: Tan J, Chen D, Chang R, Pan L, Yang J, Yuan D, Huang L, Yan T, Ning H, Wei J and Cai R (2021) Tandem Mass Spectrometry Screening for Inborn Errors of Metabolism in Newborns and High-Risk Infants in Southern China: Disease Spectrum and Genetic Characteristics in a Chinese Population. Front. Genet. 12:631688. doi: 10.3389/fgene.2021.631688
Received: 20 November 2020; Accepted: 12 July 2021;
Published: 28 July 2021.
Edited by:
Mohamed A. Elmonem, Cairo University, EgyptReviewed by:
Aria Setoodeh, Tehran University of Medical Sciences, IranLambertus Petrus Van Den Heuvel, Radboud University Nijmegen Medical Centre, Netherlands
Copyright © 2021 Tan, Chen, Chang, Pan, Yang, Yuan, Huang, Yan, Ning, Wei and Cai. This is an open-access article distributed under the terms of the Creative Commons Attribution License (CC BY). The use, distribution or reproduction in other forums is permitted, provided the original author(s) and the copyright owner(s) are credited and that the original publication in this journal is cited, in accordance with accepted academic practice. No use, distribution or reproduction is permitted which does not comply with these terms.
*Correspondence: Ren Cai, bHpjYWlyZW5AMTI2LmNvbQ==