- 1Department of Cardiac Surgery, Fujian Medical University Union Hospital, Fuzhou, China
- 2Fujian Key Laboratory of Cardio-Thoracic Surgery, Fujian Medical University, Fuzhou, China
- 3Department of Anesthesiology, Xinyi People’s Hospital, Xuzhou, China
- 4Key Laboratory of Ministry of Education for Gastrointestinal Cancer, School of Basic Medical Sciences, Fujian Medical University, Fuzhou, China
- 5Department of Thoracic Surgery, Fujian Medical University Union Hospital, Fuzhou, China
RNA modification plays important roles in many biological processes such as gene expression control. Genetic variants that affect RNA modification may have functional roles in aortic dissection. The aim of this study was to identify RNA modifications related to spontaneous coronary artery dissection (SCAD). We examined the association of RNA modification-associated single-nucleotide polymorphisms (RNAm-SNPs) with SCAD in summary data from a genome-wide association study (GWAS) of European descent (270 SCAD cases and 5,263 controls). Furthermore, we performed expression quantitative loci (eQTL) and protein quantitative loci (pQTL) analyses for the RNAm-SNPs using publicly available data. Functional enrichment and protein–protein interaction analyses were performed for the identified proteins. We found 11,464 unique RNAm-SNPs in the SCAD GWAS dataset, and 519 were nominally associated with SCAD. Nine RNAm-SNPs were associated with SCAD at p < 0.001, and among them, seven were N6-methyladenosine (m6A) methylation-related SNPs, one (rs113664950 in HLA-DQB1) was m7G-associated SNP, and one [rs580060 in the 3′-UTR of Mitochondrial Ribosomal Protein S21 (MRPS21)] was A-to-I modification SNP. The genome-wide significant SNP rs3818978 (SCAD association p = 5.74 × 10–10) in the 5′-UTR of MRPS21 was related to m6A modification. These nine SNPs all showed eQTL effects, and six of them were associated with circulating protein or metabolite levels. The related protein-coding genes were enriched in specific Gene Ontology (GO) terms such as extracellular space, extracellular region, defense response, lymphocyte migration, receptor binding and cytokine receptor binding, and so on. The present study found the associations between RNAm-SNPs and SCAD. The findings suggested that RNA modification may play functional roles in SCAD.
Introduction
Aortic dissections (ADs) are complex disorders affecting the aorta. Typically, ADs begin with a tear in the aortic intima, and then blood will intrude into the aortic media and separate the mid-membrane, therefore separating a true from a false lumen. AD is a leading cause of morbidity and mortality. Recently, the Global Burden Disease 2010 project showed that the death rate of AD was increased to 2.78 per 100,000 in 2010 (Sampson et al., 2014a,b). The disease burden increases with age and is more common in men than in women (Sampson et al., 2014a).
Aortic dissection can be inherited, and genetic factors were involved. AD is a heterogeneous disease that can occur in association with syndromes (e.g., Marfan syndrome, Loeys–Dietz aortic aneurysm syndrome) genetically predisposed to AD (Finkbohner et al., 1995; van Karnebeek et al., 2001). Previous studies focused on the syndromes due to variations of TGFBR2 gene have confirmed the abnormality of the transforming growth factor-beta (TGF-β) pathway regulation as a mechanism contributing to aneurysm formation. Aortic disease in Marfan syndrome is due to the defects in the fibrillin-1 gene at chromosome 15q15-31 (Lee et al., 1991). Genetic studies also have demonstrated that ADs occur in familial aggregates and have identified many genetic loci for this disease (LeMaire et al., 2011; Guo et al., 2016; van ’t Hof et al., 2016; Turley et al., 2020). Identification of functional variants in these genetic loci for AD will increase our understanding of the pathological mechanism of AD.
Recently, genome-wide association studies (GWAS) have identified several genetic loci for spontaneous coronary artery dissection (SCAD), including chromosome 1q21.2 (ADAMTSL4), chromosome 6p24.1 (PHACTR1), chromosome 12q13.3 (LRP1), and chromosome 21q22.11 (MRP6/KCNE3) (Saw et al., 2020). This study identified novel genetic factors involved in AD and therefore supported the complex genetic basis of SCAD. However, although some of the identified variants have been shown to affect gene expression, most of their functions have remained unexplained.
To date, more than 170 types of chemical modifications present in RNA molecules have been reported. The RNA modifications are modifiable and are involved in regulations of diverse biological processes in living cells (Malbec et al., 2019). As sufficiently sensitive high-resolution transcriptome-wide techniques developed, several RNA modifications have been widely studied, including N6-methyladenosine (m6A), m6Am, m1A, 2′-O-Me, m5C, m5U, m7G, A-to-I, and pseudouridine. Among these known RNA modification types, m6A has been discovered as the first example. m6A is a type of reversible and widely conserved RNA methylation among eukaryotes. It is known to us because it is important in the regulation of gene expression (Meyer and Jaffrey, 2014) and mRNA stability (Wang et al., 2014) and homeostasis (Edupuganti et al., 2017). It also has been shown to play an important role in the etiology of various diseases (Visvanathan and Somasundaram, 2017). In recent years, researchers have shown that genetic variants would have impacts on all types of RNA modifications by changing the nucleotides at which the modifications occur or RNA sequences around the target sites (Zheng et al., 2018). Therefore, the RNA modification-associated single-nucleotide polymorphisms (RNAm-SNPs) may have impacts on gene expression regulation by influencing RNA modifications and may be important functional variants for SCAD. Currently, the relationship between RNAm-SNPs and SCAD is still unknown. Annotating the functional impacts of RNAm-SNPs on SCAD may help to decipher the pathogenicity mechanisms. This study will present the first effort of evaluating the impacts of the RNAm-SNPs on SCAD genome-wide.
Materials and Methods
Determination of RNA Modification-Associated Single-Nucleotide Polymorphisms for Spontaneous Coronary Artery Dissection
In this study, we made use of the novel RNA modification annotations to obtain more functional interpretation of results from the SCAD GWAS (Saw et al., 2020). The GWAS comprised 270 SCAD cases and 5,263 controls of European descent. Summary statistics from the initial GWAS (Saw et al., 2020), which included association p-values of 607,778 genotyped variants with SCAD, were downloaded and used as “original data” in the analysis of the present study. The SCAD GWAS summary datasets were publicly available at NHGRI-EBI GWAS Catalog (accession numbers GCST90000582 and GCST90000583).
To identify the RNAm-SNPs in the large amount of SNPs from the GWAS, we obtained a set of RNAm-SNPs in the RMVar database1. The RMVar database was an updated version of the m6AVar database. Currently, it contains 1,678,126 RNAm-SNPs for the nine types of RNA modifications (m6A, m6Am, m1A, 2′-O-Me, m5C, m5U, m7G, A-to-I, and pseudouridine), which is four times larger than m6Avar. There are three confidence levels for RNAm-SNPs in the RMVar database, ranging from low confidence to high confidence. RNAm-SNPs derived from single base resolution experiments were classified into high confidence level. Those with medium confidence levels were obtained from MeRIP-Seq experiments. The low-confidence level m6A-associated variants were predicted by a CNN model. Based on the annotation of the RNAm-SNP sets, we annotated the GWAS-tested SNPs with RNA modifications in the GWAS summary dataset. Then, RNAm-SNPs that were associated with SCAD were picked out (p-values < 0.05 were considered).
Expression Quantitative Loci Analysis for the Spontaneous Coronary Artery Dissection-Associated RNA Modification-Associated Single-Nucleotide Polymorphisms
The SCAD-associated RNAm-SNPs may affect SCAD risk via disturbing RNA modification. Because RNA modification is critical in gene expression regulation, the RNAm-SNPs may act through the regulation of gene expression and therefore may be associated with mRNA expression levels. We therefore performed gene expression quantitative loci (eQTL) analysis to find associations between RNAm-SNPs and mRNA expression levels in different types of cells and tissues. We focused on the association between RNAm-SNPs and the gene in which they are located (cis-acting eQTL). Data in the HaploReg browser2 were searched (Ward and Kellis, 2012). After RNAm-SNPs with cis-acting eQTL effects were found, we tried to identify their functionalities. Transcription regulation functions such as altering protein binding were searched in HaploReg and RegulomeDB3. We also looked for eQTL signals in 34 human tissues using data from the GTEx project (v7 release)4.
Protein Quantitative Loci Analysis for the Spontaneous Coronary Artery Dissection-Associated RNA Modification-Associated Single-Nucleotide Polymorphisms
We carried out protein quantitative loci (pQTL) analysis in peripheral blood for the identified RNAm-SNPs to find proteins related to SCAD. The associations between RNAm-SNPs and plasma protein levels were searched in three pQTL studies. The data included, first, the pQTL study that examined the associations between 509,946 SNPs and 1,124 proteins. The summary data were available in the pGWAS Server database5 (Suhre et al., 2017). The second pQTL study was the INTERVAL study containing 3,301 individuals of European descent. This study examined the associations between 10.6 million imputed autosomal variants and plasma levels of 2,994 proteins6 (Sun et al., 2018). The third pQTL study analyzed 83 proteins measured in 3,394 individuals7 (Folkersen et al., 2017). Functional enrichment analyses were performed in the DAVID database, and protein–protein interactions were found in the STRING database.
In addition, we looked for associations between RNAm-SNPs and concentrations of cytokines, lipids, and metabolites. The cytokine pQTL study examined the genome-wide associations for circulating levels of 41 cytokines in 8,293 Finns8 (Ahola-Olli et al., 2017). The summary data from a study that examined the associations between genome-wide SNPs and 123 metabolites in blood samples of 24,925 individuals were obtained from http://www.computationalmedicine.fi/data#NMR_GWAS (Kettunen et al., 2016).
Results
Spontaneous Coronary Artery Dissection-Associated RNA Modification-Associated Single-Nucleotide Polymorphisms
We first picked out RNAm-SNPs from the SCAD GWAS datasets based on the annotation of the nine types of RNAm-SNPs in the RMVar database (Table 1). For m6A modification, we found 11,464 unique RNAm-SNPs in coding and non-coding genes in the SCAD GWAS dataset. Among them, 519 (4.5%) were found to be nominally (p < 0.05) associated with SCAD (Table 1). Among these 519 SNPs, 213 were high-confidence RNAm-SNPs, 103 were medium-confidence RNAm-SNPs, and 203 were low-confidence RNAm-SNPs. The SNPs mainly located in exon (75), UTR (88), and intron (156). Specifically, nine RNAm-SNPs were associated with SCAD at p < 0.001, and rs3818978 in the 5′-UTR of MRPS21 was significantly associated with SCAD (p < 5.0 × 10–8) (Table 2). Seven of the nine identified RNAm-SNPs were m6A-associated SNPs (Figure 1). The genome-wide significant SCAD m6A-associated SNP rs3818978 (p = 5.74 × 10–10) belongs to the medium confidence category.
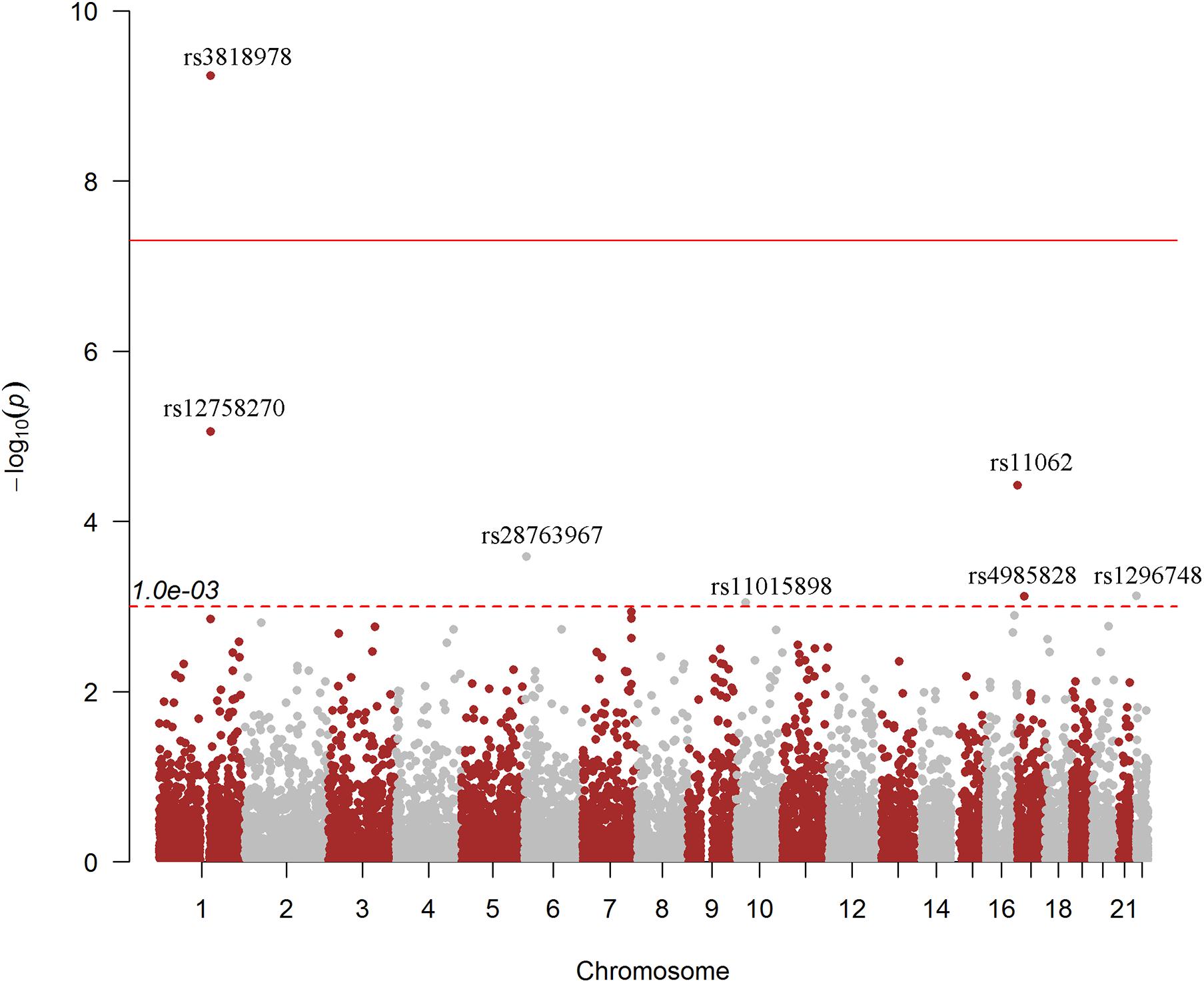
Figure 1. Genome-wide associations between RNA modification-associated single-nucleotide polymorphisms (RNAm-SNPs) and spontaneous coronary artery dissection (SCAD). This Manhattan plot shows the associations between RNAm-SNPs and SCAD. The x-axis indicates chromosome positions. The y-axis indicates –log10p-values of the associations. The information was extracted from the summary dataset of the SCAD genome-wide association study (GWAS) published in 2020 (Saw et al., 2020). The solid red line indicated the genome-wide significance level of 5.0 × 10–8, and the dotted red line indicated the suggested level of 1.0 × 10–3. The brown and gray color dots were used to separate them from each chromosome.
We identified 171 unique m7G modification SNPs from the SCAD GWAS dataset, and 14 of them were nominally associated with SCAD (Table 1). The functional loss m7G-associated SNP rs113664950 in an exon of HLA-DQB1 was associated with SCAD (p = 1.83 × 10–4) (Table 2). For A-to-I modification, we found 377 unique RNAm-SNPs from the SCAD GWAS dataset, and 14 of them were nominally associated with SCAD (Table 1). The functional loss A-to-I-associated SNP rs580060 in the 3′-UTR of MRPS21 was associated with SCAD (p = 2.97 × 10–6) (Table 2). Besides, we found one m6Am-associated SNP, 24 m1A-associated SNPs, and one m5C-associated SNP that were nominally associated with SCAD (Table 1).
Gene Expression Analysis
We further tried to identify gene expressions that were associated with the nine identified SCAD-associated RNAm-SNPs (Table 2). All of these RNAm-SNPs showed eQTL effects on protein-coding genes in various types of cells or tissues from GTEx project (Figure 2). The number of eQTL signals for the nine SNPs varied (from 29 to 540). A total of 540 eQTL signals were found for rs3818978 in MRPS21, which was significantly associated with SCAD (p = 5.74 × 10–10). According to the HaploReg database, rs3818978 overlapped promoter histone marks in 24 tissues related to 33 bound proteins and altered four regulatory motifs. This SNP was associated with gene expression of MRPS21 in aorta artery (p = 5.30 × 10–7) and appendage atrial tissues (p = 6.12 × 10–11) and associated with gene expression of ADAMTSL4 in aorta artery (p = 8.31 × 10–7) and tibial artery (p = 1.51 × 10–6). The m6A-associated SNP rs12758270 in the 3′-UTR of RPRD2 overlapped promoter histone marks in one tissue and enhancer histone marks in six tissues and altered two regulatory motifs. It was associated with gene expression of ECM1 in fibroblast cells (p = 2.79 × 10–5) and esophagus mucosa (p = 3.69 × 10–7) and ADAMTSL4 in esophagus mucosa (p = 9.84 × 10–12).
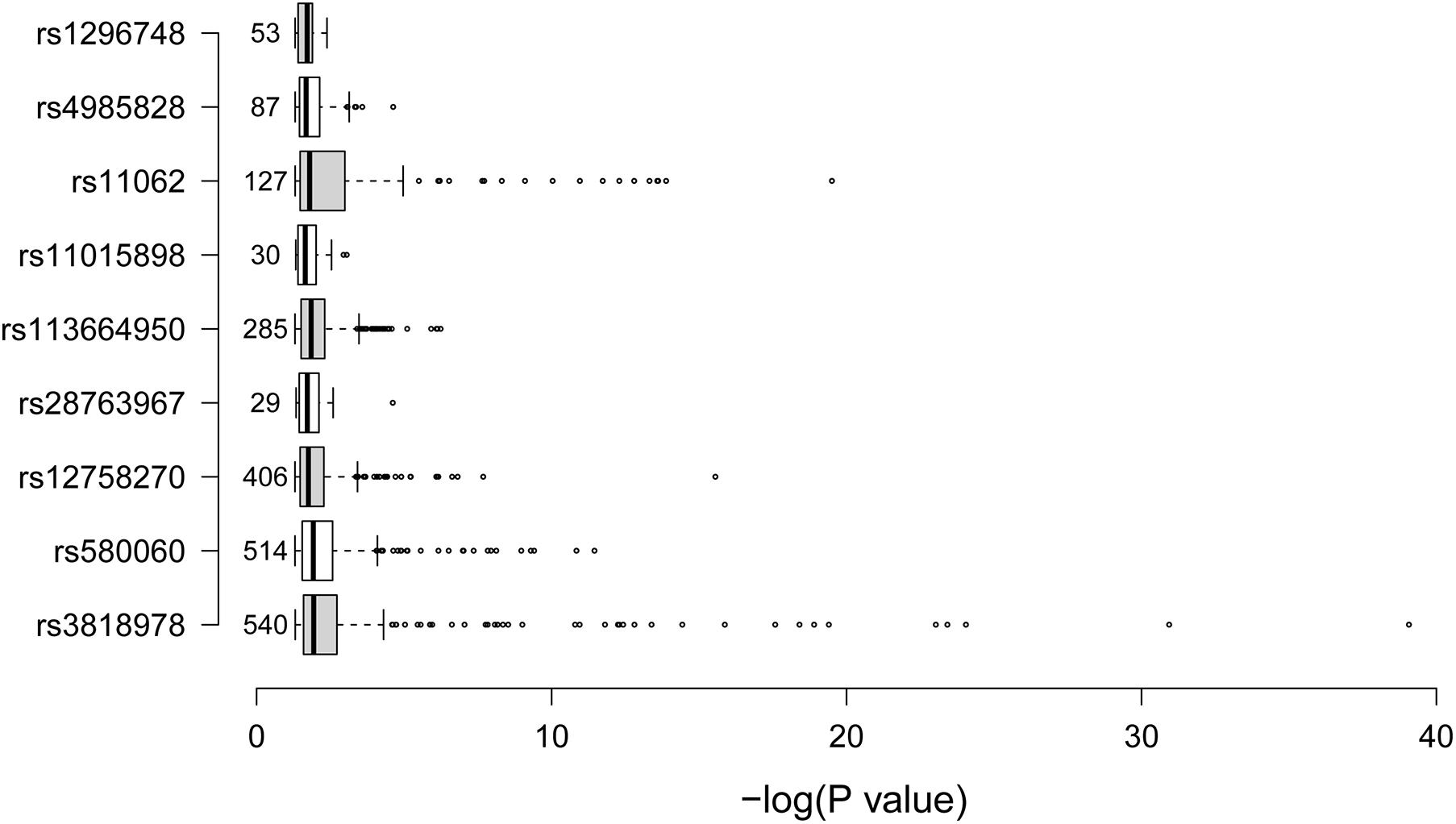
Figure 2. Summary of the expression quantitative loci (eQTL) signals. The boxplot summarized the –log10p-values for the eQTL signals of the nine spontaneous coronary artery dissection (SCAD)-associated RNA modification single-nucleotide polymorphism SNPs. The data were obtained from the GTEx project. The numbers on the left side of the boxes were the number of eQTL signals for the corresponding SNP.
Protein Quantitative Loci Analysis
We found 44 pQTL signals for six (rs12758270, rs28763967, rs11062, rs3818978, rs580060, and rs1296748) of the nine identified RNAm-SNPs (Table 3). A total of 42 proteins were found. Among these signals, 36 were found from the pGWAS Server (Suhre et al., 2017). The top signal was the association between rs12758270 and Extracellular matrix protein 1 (p = 2.66 × 10–7), followed by the association between rs11062 and Interleukin 4 (p = 6.78 × 10–5). Among the 44 pQTL signals, 43 signals were found for m6A-associated SNPs, and one signal was found for A-to-I-associated SNP rs580060, which was associated with Cystatin F (p = 4.69 × 10–4). In addition, we found that rs580060 (p = 1.41 × 10–5) and rs12758270 (p = 3.13 × 10–3) were associated with cytokine bNGF level. For lipid levels, rs3818978 was associated with plasma level of Glol (p = 4.62 × 10–4) and LDL-D (p = 2.63 × 10–4); rs580060 was associated with plasma level of XS-VLDL-PL (p = 2.89 × 10–4).
Functional Enrichment Analysis
The 42 associated proteins were enriched in 68 Gene Ontology (GO) terms, including cellular components such as extracellular space (p = 3.80 × 10–13) and extracellular region (p = 1.40 × 10–9); biological process such as defense response (p = 1.40 × 10–6) and lymphocyte migration (p = 1.40 × 10–6); molecular function such as receptor binding (p = 1.70 × 10–6) and cytokine receptor binding (p = 2.20 × 10–6); and so on (Figure 3A). Plasma levels of seven proteins coded by ADAMTS4, DDC, B2M, CCL20, CRISP3, ECM1, and TSHB were affected by rs12758270 (Table 3). These proteins were enriched in cellular component such as extracellular region (p = 9.30 × 10–5) and cytoplasmic vesicle (p = 5.90 × 10–3) (Figure 3B).
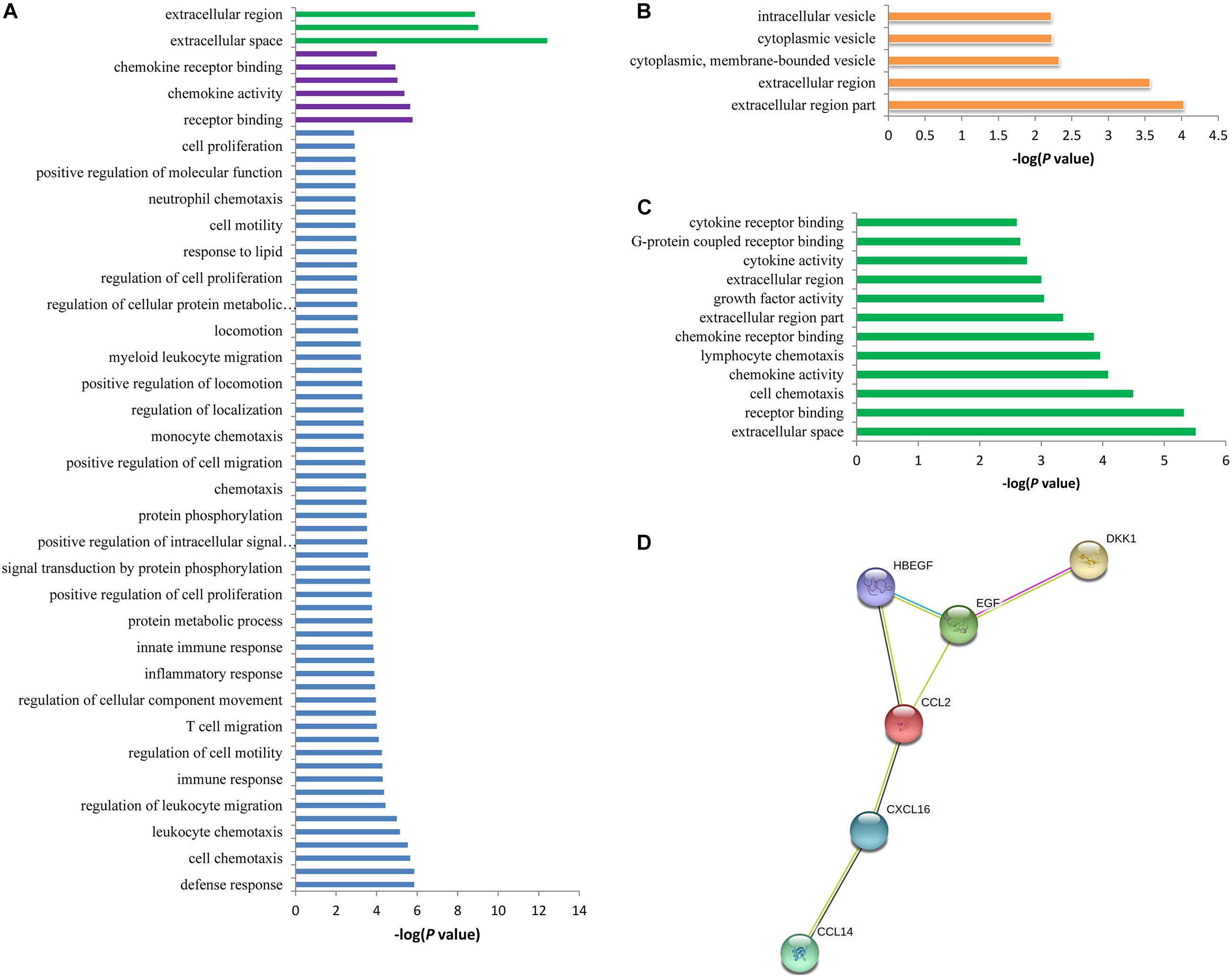
Figure 3. Functional annotation enrichment analysis of the spontaneous coronary artery dissection (SCAD)-related proteins. (A) There were 68 statistically significant Gene Ontology (GO) terms that were enriched in the SCAD-related proteins. (B) The seven proteins (ADAMTS4, DDC, B2M, CCL20, CRISP3, ECM1, and TSHB) that may be affected by rs12758270 were enriched in five GO terms. (C) The six proteins (CCL2, CXCL16, DKK1, HBEGF, EGF, and CCL14) that may be affected by rs3818978 were enriched in 12 GO terms. (D) Protein–protein interactions between the six proteins that may be affected by rs3818978.
The genome-wide significant SCAD-associated m6A SNP rs3818978 was associated with the plasma levels of CCL2, CXCL16, DKK1, HBEGF, EGF, and CCL14 (Table 3). These six proteins were enriched in specific biological processes (e.g., cell chemotaxis and lymphocyte chemotaxis), cellular component (e.g., extracellular space, extracellular region part, and extracellular region), and molecular function (e.g., receptor binding, chemokine activity, chemokine receptor binding, growth factor activity, and cytokine activity) (Figure 3C). These six proteins interacted directly with each other according to the STRING database (Figure 3D).
Discussion
This study showed that SCAD-associated SNPs identified in GWAS were related to RNA modification. These SNPs showed cis-acting eQTL effects in various types of cells and tissues, and some of them were found to be associated with circulating protein levels. Moreover, the associated proteins were enriched in specific functional annotation terms.
Increasing evidence has shown that m6A methylation is critical in gene expression regulation, as it modulates RNA processing such as nuclear export, translatability, splicing, and stability (Li et al., 2018; Visvanathan and Somasundaram, 2018). Variations in the methylation target sites can interrupt the modification and then disturb gene expression regulations (Zheng et al., 2018). Therefore, identification of RNAm-SNPs in diseases is a way to ascertain causal variants and therefore helpful for the interpretation of the GWAS findings. In the present study, we identified many SCAD-associated RNAm-SNPs and showed that these kinds of SNPs may have impacts on gene expression, including mRNA levels and protein levels. The findings suggested that RNA modification may play a role in SCAD.
One of the genome-wide significant RNAm-SNPs, rs3818978, has not been discussed in the SCAD GWAS paper. This m6A-associated SNP was close to the reported SCAD-associated SNP rs12740679 (4 Kb upstream of gene MRPS21). RNAm-SNP rs3818978 locates in the 5′-UTR of MRPS21. The function of MRPS21 gene has been less known. According our analysis, rs3818978 was associated with the expression of MRPS21 and ADAMTSL4 in the artery aorta and associated with the plasma level of several proteins with specific functions. In MRPS21, there was another SNP identified in this study, the A-to-I modification SNP rs580060, which locates in the 3′-UTR of MRPS21. This SNP was also associated with the expression of ADAMTSL4 in the artery aorta and associated with the plasma level of Cystatin F. ADAMTSL4 codes an extracellular matrix protein. The extracellular matrix protein binds to fibrillin-1 to form microfibrils in the matrix (Hubmacher and Apte, 2015). The original SCAD GWAS has demonstrated that ADAMTSL4 gene was expressed in the interarterial layer and vascular smooth muscle cells. It was suggested that ADAMTSL4 deficiency was involved in arterial fragility (Saw et al., 2020). The present study identified two RNA modification SNPs that may affect ADAMTSL4 expression as potential functional variants for SCAD.
In addition to the genome-wide significant signals, we found several RNAm-SNPs that did not reach the genome-wide significance threshold of 5.0 × 10–8 for SCAD. For example, the m6A-associated SNP rs12758270 that locates in the 3′-UTR of RPRD2 did not reach the genome-wide significant level for SCAD. Then, it has been ignored in the original GWAS. In the present study, we found that rs12758270 was associated with gene expression of ECM1 and ADAMTSL4 and associated with plasma levels of ADAMTS4, DDC, B2M, CCL20, CRISP3, ECM1, and TSHB, which have specific biological functions. Therefore, these RNAm-SNPs may also be potential functional candidates for SCAD.
The RNAm-SNPs could disturb RNA modification, and then the mRNA expression levels were changed and consequently affect SCAD risk. But further evidence is needed to prove that gene expression affected by these RNAm-SNPs was associated with SCAD risk. According to the result of a transcriptome sequencing study (GSE153434), DSP (fold change = 0.327, p = 2.30 × 10–4), MKX (fold change = 0.391, p = 3.83 × 10–8), and SERPINF1 (fold change = 0.066, p = 2.26 × 10–15) were differentially expressed between Stanford type A aortic dissection patients and controls in ascending aortic tissues (Zhou et al., 2020). SERPINF1 expression level was associated with rs11062 (m6A-associated SNP locates in the 3′-UTR of SMYD4) in transformed fibroblast cells, left ventricle, subcutaneous adipose, atrial appendage, and esophagus mucosa tissues. In addition, our analysis showed that RNAm-SNPs affected genes involved in specific biological functions, which were highly related to SCAD. SCAD is mediated by inflammasome activation, which exacerbates the secretion of pro-inflammatory cytokines, chemokines, and matrix metalloproteinases. The dysregulation of extracellular matrix results in progressive smooth muscle cell depletion and inflammation, leading to SCAD (Mayer et al., 2020; Shen et al., 2020). Therefore, gene expressions affected by the RNAm-SNPs play important roles in SCAD. This evidence may suggest that the identified RNAm-SNPs may participate in the pathogenesis of SCAD by altering RNA modification.
This study has some potential limitations. First, the sample size of the SCAD GWAS was still relatively small. Second, the m6A SNP set was large, but data for other types of RNA modification were still very rare. Third, although we identified associations between SCAD-associated SNPs and gene expression and plasma protein levels, the associations between the gene expression and plasma protein levels and SCAD have not been examined. Finally, the functions of these SNPs and the effects of modification of the detected genes on SCAD have not been examined experimentally. More evidence is needed to prove that these SNPs may indeed participate in the pathogenesis of SCAD by altering RNA modification. Further experiments determining the functions in related cells (e.g., vascular smooth muscle cells) are needed.
Conclusion
This study found SCAD-associated RNAm-SNPs and therefore suggested that RNA modification may play important roles in SCAD. The findings increased our understanding on the associations identified in the SCAD GWAS. These kinds of SNPs in susceptibility genes of SCAD may work by regulating gene expression, including mRNA levels and protein levels. No previous study has shown the relationship between RNA modification and SCAD. Therefore, this study may add valuable clues for further understanding of functional mechanisms underlying the development of SCAD.
Data Availability Statement
The original contributions presented in the study are included in the article/supplementary material, further inquiries can be directed to the corresponding author.
Author Contributions
TC, MT, and LC contributed to the conceptualization. TC contributed to data curation and writing of the original draft. MT contributed to the formal analysis. TC, MT, XY, ZQ, and XL contributed to the investigation. TC, MT, and XL contributed to the methodology. TC and MT contributed to the visualization. MT and LC contributed to the writing, review, and editing. All authors contributed to the article and approved the submitted version.
Funding
This work was supported by the National Natural Science Foundation of China (U2005202), the Fujian Province Major Science and Technology Program (2018YZ001-1), the Natural Science Foundation of Fujian Province (2020J01998 and 2020J02056), and the Fujian Provincial Health Technology Project (2019-ZQN-50).
Conflict of Interest
The authors declare that the research was conducted in the absence of any commercial or financial relationships that could be construed as a potential conflict of interest.
Footnotes
- ^ http://rmvar.renlab.org/download.html
- ^ http://archive.broadinstitute.org/mammals/haploreg/haploreg.php
- ^ http://regulome.stanford.edu/
- ^ http://www.gtexportal.org
- ^ http://metabolomics.helmholtz-muenchen.de/pgwas/index.php?task=download
- ^ http://www.phpc.cam.ac.uk/ceu/proteins/
- ^ https://zenodo.org/record/264128#.X3u5ga95uUk
- ^ http://www.computationalmedicine.fi/data#Cytokine_GWAS
References
Ahola-Olli, A. V., Würtz, P., Havulinna, A. S., Aalto, K., Pitkänen, N., Lehtimäki, T., et al. (2017). Genome-wide association study identifies 27 loci influencing concentrations of circulating cytokines and growth factors. Am. J. Hum. Genet. 100, 40–50. doi: 10.1016/j.ajhg.2016.11.007
Edupuganti, R. R., Geiger, S., Lindeboom, R. G. H., Shi, H., Hsu, P. J., Lu, Z., et al. (2017). N(6)-methyladenosine (m(6)A) recruits and repels proteins to regulate mRNA homeostasis. Nat. Struct. Mol. Biol. 24, 870–878. doi: 10.1038/nsmb.3462
Finkbohner, R., Johnston, D., Crawford, E. S., Coselli, J., and Milewicz, D. M. (1995). Marfan syndrome. Long-term survival and complications after aortic aneurysm repair. Circulation 91, 728–733. doi: 10.1161/01.cir.91.3.728
Folkersen, L., Fauman, E., Sabater-Lleal, M., Strawbridge, R. J., Frånberg, M., Sennblad, B., et al. (2017). Mapping of 79 loci for 83 plasma protein biomarkers in cardiovascular disease. PLoS Genet. 13:e1006706. doi: 10.1371/journal.pgen.1006706
Guo, D. C., Grove, M. L., Prakash, S. K., Eriksson, P., Hostetler, E. M., LeMaire, S. A., et al. (2016). Genetic variants in LRP1 and ULK4 are associated with acute aortic dissections. Am. J. Hum. Genet. 99, 762–769. doi: 10.1016/j.ajhg.2016.06.034
Hubmacher, D., and Apte, S. S. (2015). ADAMTS proteins as modulators of microfibril formation and function. Matrix Biol. 47, 34–43. doi: 10.1016/j.matbio.2015.05.004
Kettunen, J., Demirkan, A., Wurtz, P., Draisma, H. H., Haller, T., Rawal, R., et al. (2016). Genome-wide study for circulating metabolites identifies 62 loci and reveals novel systemic effects of LPA. Nat. Commun. 7:11122. doi: 10.1038/ncomms11122
Lee, B., Godfrey, M., Vitale, E., Hori, H., Mattei, M. G., Sarfarazi, M., et al. (1991). Linkage of marfan syndrome and a phenotypically related disorder to two different fibrillin genes. Nature 352, 330–334. doi: 10.1038/352330a0
LeMaire, S. A., McDonald, M. L., Guo, D. C., Russell, L., Miller, C. C. III, Johnson, R. J., et al. (2011). Genome-wide association study identifies a susceptibility locus for thoracic aortic aneurysms and aortic dissections spanning FBN1 at 15q21.1. Nat. Genet. 43, 996–1000. doi: 10.1038/ng.934
Li, L. J., Fan, Y. G., Leng, R. X., Pan, H. F., and Ye, D. Q. (2018). Potential link between m(6)A modification and systemic lupus erythematosus. Mol. Immunol. 93, 55–63. doi: 10.1016/j.molimm.2017.11.009
Malbec, L., Zhang, T., Chen, Y. S., Zhang, Y., Sun, B. F., Shi, B. Y., et al. (2019). Dynamic methylome of internal mRNA N(7)-methylguanosine and its regulatory role in translation. Cell Res. 29, 927–941. doi: 10.1038/s41422-019-0230-z
Mayer, L., Pechlaner, R., Barallobre-Barreiro, J., Boehme, C., Toell, T., Lynch, M., et al. (2020). Extracellular matrix protein signature of recurrent spontaneous cervical artery dissection. Neurology 95, e2047–e2055. doi: 10.1212/WNL.0000000000010710
Meyer, K. D., and Jaffrey, S. R. (2014). The dynamic epitranscriptome: N6-methyladenosine and gene expression control. Nat. Rev. Mol. Cell Biol. 15, 313–326. doi: 10.1038/nrm3785
Sampson, U. K., Norman, P. E., Fowkes, F. G., Aboyans, V., Song, Y., and Harrell, F. E. Jr., et al. (2014a). Estimation of global and regional incidence and prevalence of abdominal aortic aneurysms 1990 to 2010. Glob. Heart 9, 159–170. doi: 10.1016/j.gheart.2013.12.009
Sampson, U. K., Norman, P. E., Fowkes, F. G., Aboyans, V., Yanna, S., and Harrell, F. E. Jr., et al. (2014b). Global and regional burden of aortic dissection and aneurysms: mortality trends in 21 world regions, 1990 to 2010. Glob. Heart 9, 171–180.e10. doi: 10.1016/j.gheart.2013.12.010
Saw, J., Yang, M. L., Trinder, M., Tcheandjieu, C., Xu, C., Starovoytov, A., et al. (2020). Chromosome 1q21.2 and additional loci influence risk of spontaneous coronary artery dissection and myocardial infarction. Nat. Commun. 11:4432. doi: 10.1038/s41467-020-17558-x
Shen, Y. H., LeMaire, S. A., Webb, N. R., Cassis, L. A., Daugherty, A., and Lu, H. S. (2020). Aortic aneurysms and dissections series. Arterioscler. Thromb. Vasc. Biol. 40, e37–e46. doi: 10.1161/ATVBAHA.120.313991
Suhre, K., Arnold, M., Bhagwat, A. M., Cotton, R. J., Engelke, R., Raffler, J., et al. (2017). Connecting genetic risk to disease end points through the human blood plasma proteome. Nat. Commun. 8:14357. doi: 10.1038/ncomms14357
Sun, B. B., Maranville, J. C., Peters, J. E., Stacey, D., Staley, J. R., Blackshaw, J., et al. (2018). Genomic atlas of the human plasma proteome. Nature 558, 73–79. doi: 10.1038/s41586-018-0175-2
Turley, T. N., O’Byrne, M. M., Kosel, M. L., de Andrade, M., Gulati, R., Hayes, S. N., et al. (2020). Identification of susceptibility loci for spontaneous coronary artery dissection. JAMA Cardiol. 5, :929–938. doi: 10.1001/jamacardio.2020.0872
van Karnebeek, C. D., Naeff, M. S., Mulder, B. J., Hennekam, R. C., and Offringa, M. (2001). Natural history of cardiovascular manifestations in marfan syndrome. Arch. Dis. Child 84, 129–137. doi: 10.1136/adc.84.2.129
van ’t Hof, F. N., Ruigrok, Y. M., Lee, C. H., Ripke, S., Anderson, G., de Andrade, M., et al. (2016). Shared genetic risk factors of intracranial, abdominal, and thoracic aneurysms. J. Am. Heart Assoc. 5:e002603. doi: 10.1161/JAHA.115.002603
Visvanathan, A., and Somasundaram, K. (2017). mRNA traffic control reviewed: N6-methyladenosine (m(6) A) takes the driver’s seat. Bioessays 40:1700093. doi: 10.1002/bies.201700093
Visvanathan, A., and Somasundaram, K. (2018). mRNA traffic control reviewed: N6-methyladenosine (m(6) A) takes the driver’s seat. Bioessays 40:1700093. doi: 10.1002/bies.201700093
Wang, X., Lu, Z., Gomez, A., Hon, G. C., Yue, Y., Han, D., et al. (2014). N6-methyladenosine-dependent regulation of messenger RNA stability. Nature 505, 117–120. doi: 10.1038/nature12730
Ward, L. D., and Kellis, M. (2012). HaploReg: a resource for exploring chromatin states, conservation, and regulatory motif alterations within sets of genetically linked variants. Nucleic Acids Res. 40, D930–D934. doi: 10.1093/nar/gkr917
Zheng, Y., Nie, P., Peng, D., He, Z., Liu, M., Xie, Y., et al. (2018). m6AVar: a database of functional variants involved in m6A modification. Nucleic Acids Res. 46, D139–D145. doi: 10.1093/nar/gkx895
Keywords: artery dissection, RNA methylation, genome-wide association study, circulating protein, gene expression
Citation: Chai T, Tian M, Yang X, Qiu Z, Lin X and Chen L (2021) Genome-Wide Identification of RNA Modifications for Spontaneous Coronary Aortic Dissection. Front. Genet. 12:696562. doi: 10.3389/fgene.2021.696562
Received: 23 April 2021; Accepted: 01 June 2021;
Published: 02 July 2021.
Edited by:
Y-h. Taguchi, Chuo University, JapanCopyright © 2021 Chai, Tian, Yang, Qiu, Lin and Chen. This is an open-access article distributed under the terms of the Creative Commons Attribution License (CC BY). The use, distribution or reproduction in other forums is permitted, provided the original author(s) and the copyright owner(s) are credited and that the original publication in this journal is cited, in accordance with accepted academic practice. No use, distribution or reproduction is permitted which does not comply with these terms.
*Correspondence: Liangwan Chen, Y2hlbmxpYW5nd2FuQGZqbXUuZWR1LmNu
†These authors have contributed equally to this work