- 1Department of Obstetrics and Gynaecology, West China Second University Hospital, Sichuan University, Chengdu, China
- 2Key Laboratory of Birth Defects and Related Diseases of Women and Children, Ministry of Education, Sichuan University, Chengdu, China
Background: This study aims to evaluate prenatal diagnosis methods following positive noninvasive prenatal screening (NIPS) results.
Methods: According to the positive noninvasive prenatal screening results, 926 pregnant women were divided into three groups: main target disease group (high risk for trisomy 21, trisomy 18, or trisomy 13), sex chromosome aneuploidy (SCA) group, and other chromosomal abnormalities group [abnormal Z-scores for chromosomes other than trisomy (T)21/T18/T13 or SCAs]. The verification methods and results were then retrospectively analysed.
Results: In the main target disease group, the positive rate of chromosomal abnormalities confirmed by quantitative fluorescence polymerase chain reaction (QF-PCR) was 75.18% (212/282), which was not significantly different from that by karyotyping (79.36%, 173/218) and copy number variation (CNV) detection methods (71.43%, 65/91). The positive rate of additional findings confirmed by karyotyping and copy number variation detection methods in main target disease group was 0.46% (1/218) and 8.79% (8/91), respectively. The positive rate of chromosomal abnormalities confirmed by karyotyping and CNV detection methods were 27.11% (45/166) and 38.46% (95/247) in the SCA group and 4.17% (1/24) and 20% (36/180) in the other chromosomal abnormalities group, respectively. Fetal sex chromosome mosaicism was detected in 16.13% (20/124) of the confirmed SCA cases. There were no significant differences in the detection rates of chromosomal microarray analysis (CMA) and CNV sequencing (CNVseq) among the three groups (p > 0.05).
Conclusion: QF-PCR can quickly and accurately identify aneuploidies following NIPS-positive results for common aneuploidy, and in combination with karyotyping and CNV detection techniques can provide more comprehensive results. With the NIPS-positive results for SCA or other abnormalities, CMA and CNVseq may have the same effect on increasing the detection rate. The addition of fluorescence in situ hybridization assay may help to identify true fetal mosaicism.
Introduction
Noninvasive prenatal screening (NIPS) based on cell-free DNA in maternal circulation is used primarily to screen for trisomies 21, 18, and13, that has been shown to outperform conventional screening methods (Norton et al., 2015). In addition, NIPS may reveal other chromosomal abnormalities, such as sex chromosome aneuploidy (SCA) and other autosomal aberrations, by low-coverage whole-genome sequencing of maternal and placental fragments of DNA (Nicolaides et al., 2013; Lefkowitz et al., 2016; van der Meij et al., 2019). Not all positive results detected by NIPS represent fetal aberrations because of certain biological factors, such as confined placental mosaicism (Grati, 2014; Grati, 2016), ‘vanishing’ twins (Grömminger et al., 2014), and maternal genomic contribution (Ji et al., 2018; Zhang et al., 2020). Hence, further prenatal diagnostic testing is required to check NIPS-positive results.
Several frequently used prenatal diagnostic technologies vary in the testing principle, turnaround time, detection range, and cost. Cytogenetic karyotype testing enables the visual detection of chromosomal abnormalities such as full chromosome aneuploidies, polyploidy, mosaicism, and structural abnormalities of more than 5–10 million base pairs (Mb), including balanced and unbalanced rearrangements. Both quantitative fluorescence polymerase chain reaction (QF-PCR) and fluorescence in situ hybridization (FISH) provide a rapid prenatal diagnosis of chromosome aneuploidy, and FISH is a valuable complementary test for detecting mosaicism (Hultén et al., 2003; Su et al., 2015). Chromosomal microarray analysis (CMA) and copy number variation sequencing (CNVseq) assays, as the two major CNV detection techniques, offer a high-resolution approach to diagnose microdeletions or microduplications (Xu et al., 2014; Wang et al., 2020). CMA can be divided into two types: array-based comparative genomic hybridization (aCGH) and single nucleotide polymorphism (SNP) array.
During recent years, NIPS testing has considerably increased. While most studies have focused on the detection range and accuracy of NIPS(Nicolaides et al., 2013; Lefkowitz et al., 2016; Deng et al., 2019; van der Meij et al., 2019), only a few have investigated prenatal diagnostic techniques following positive NIPS results. In this study, we retrospectively analysed the prenatal diagnostic methods following different types of NIPS-positive results. This study, to the best of our knowledge, the first of its kind in southwest China, aims to provide more evidence for the clinical selection of prenatal diagnosis in patients with different types of NIPS-positive results.
Methods
Participants
This retrospective analysis involved 926 pregnant women with positive NIPS results who underwent amniocentesis between May 2015 and June 2019 at West China Second University Hospital of Sichuan University, Chengdu, Sichuan Province, China.
The NIPS-positive results were classified into three categories: 1) “main target disease” if NIPS was positive for trisomy (T)21, T18, or T13; 2) “SCA” when NIPS was positive for SCA; and 3) “other chromosomal abnormalities” when NIPS presented abnormal Z-scores for chromosomes other than T21/T18/T13 or SCAs. All participants received professional pre-test counselling before NIPS testing and amniocentesis. They all voluntarily chose to expand NIPS to obtain maximum fetal genomic information; and then selected one or more diagnostic methods for the ultimate report from karyotyping, CMA, and CNVseq, after knowing the scope of application, target diseases, limitations of these prenatal diagnostic techniques, and decided whether rapid testing (QF-PCR/FISH) would be performed. Statistical analyses of the diagnostic methods and results were conducted. Neonatal conditions of those diagnosed as negative by amniocentesis were followed up for growth and development via phone call.
The study was approved by the Institutional Ethics Committee of Sichuan University and conducted in accordance with the Declaration of Helsinki. All participants signed a written informed consent form before testing.
Noninvasive Prenatal Screening
Maternal peripheral blood (10 ml) was collected in a cell-free BCT tube (Streck, Omaha, NE, United States). The procedural details of the experimental operation and analysis methods have been published previously (Liu et al., 2021). The test results for all 24 chromosomes were presented using Z-scores (normal range, −3 < Z < 3).
Invasive Prenatal Diagnosis
Following written informed consent and performing a preoperative examination, 25–30 ml of amniotic fluid was collected by abdominal amniocentesis using ultrasonography guidance. The amniotic fluid was tested following strict instructions regarding the operation conditions and procedures for the corresponding diagnostic tests.
Chromosome Karyotyping
Upon standard metaphase conversion of cultured fetal cells, amniotic fluid cells were cultured using BIOAMFTM-3 medium (Biological Industries, Kibbutz Beit-Haemek, Israel) and AMINOPAN medium (PAN-biotech GmbH, Aidenbach, Germany). Each cultured cell was inoculated in parallel with the two bottles. More than 20 metaphase cells from each specimen were analysed by g-banding at a resolution of above 400 bands on average. Karyotypes were recorded as text written in the International System for Human Cytogenetic Nomenclature (ISCN) 2020.
QF-PCR
DNA was extracted from 5 ml of uncultured amniotic fluid samples using the QIAamp DNA Mini kit (QIAGEN, Dusseldorf, Germany). The concentration was adjusted to 30 ng/μL. PCR amplification was performed using a reaction kit (Guangzhou Darui Biotechnology Co., Ltd., Guangzhou, China). The PCR product and GeneScan™600 LIZ Size standard v2.0 was denatured, and electrophoretic analysis was performed using a POP4 gel (ABI) on the ABI 3500Dx Genetic Analyser (Applied Biosystems). There were four short tandem repeat (STR) markers for the X and Y chromosomes (AMXY, DXS981, DXS6809, DXS22), three for chromosome 21 (D21S1435, D21S11, D21S1411), four for chromosome 18 (D18S1002, D18S391, D18S535, D18S386) and four for chromosome 13 (D13S628, D13S742, D13S634, D13S305). Data collection and analysis were conducted using Gene Mapper ID Software V3.2 (Applied Biosystems, Inc., CA, United States).
FISH
The uncultured amniotic fluid was tested, following strict instructions of the operation conditions and procedure, using the AneuVysion Multicolor DNA Probe kit (Abbott Molecular, Inc., Des Plaines, United States), according to the manufacturer’s instructions. Two sets of CEP 18/X/Y and LSI 13/21 probes were used, which are located on the centromere of chromosome 18/X/Y, 13q14, and 21q22.13-q22.2. After hybridising the fluorescent-labelled DNA probe with the sample DNA, the fluorescence microscope OLYMPUS BX51 was used to assess chromosome aneuploidy.
CMA
The American Cytoscan®Affymetrix 750 K chip kit and reagent kit bundle (Beijing Guoku Biological Technology Co., Ltd., Beijing, China), and the Clontech Titanium DNA Amplification kit (Clontech, United States) were used for DNA extraction, fragmentation, and hybridization. Hybridization products were then washed, stained on Fluidics Station 450Dx v.2, and subsequently scanned on Gene Chip Scanner 3000Dxv.2 with Auto Loader. The obtained data were loaded into Chas V3.0 software for data processing.
CNVseq
DNA was extracted from amniotic fluid using a DNA extraction test kit (Hangzhou Berry Gene Diagnostic Technology Co., Ltd., Hangzhou, China). This was followed by DNA fragmentation, library construction using PCR-free technology, and index addition. The quality of the libraries was tested using the KAPA SYBERFAST qPCR kit (KAPA Biosystems, Wilmington, MA, United States) before and after pooling the libraries. The hybrid library was then subjected to 36 bp single-terminal sequencing with a sequencing depth of 0.1× using a Next Seq CN500 massively parallel sequencing kit (High-throughput sequencing kit) and the NextSeqCN500 high-throughput sequencing flow cell with four lanes (Illumina China, Shanghai, China). The sequences obtained were compared with those of the hg19 human genome.
Classification Criteria for CNVs
The results were determined by referring to the hg19 version of the human genome and the most recent data available on the Database of Genomic Variants, DECIPHER, Online Mendelian Inheritance in Man, University of California Santa Cruz, PubMed, and other public databases. According to the American College of Medical Genetics (ACMG) sequence variant classification guidelines for copy number variants (Richards et al., 2015), the clinical significance of CNVs was divided into five classes: pathogenic, likely pathogenic, benign, likely benign, and variant of uncertain significance (VUS).
Statistical Analysis
SPSS software v21 (IBM, Armonk, NY, United States) was used to analyse the data. Enumeration data are expressed as example and percentage (%). The chi-square (χ2) test was used to compare the difference in detection rates between the two methods. For a total number of <40 or a theoretical frequency <5, Fisher’s exact probability method was used. A p value of <0.05 was considered significant.
Results
According to the NIPS results, patients were divided into three groups: main target disease [T21 (n = 234), T18 (n = 44), and T13 (n = 31)], fetal sex chromosome abnormalities (n = 413), and other chromosomal abnormalities (n = 204) groups. The average maternal age, average gestational age, and true positive rate of each group are summarized in Table 1. In the main target disease group, 240/309 (77.70%) were confirmed as chromosome abnormalities including 233/240 (97.08%) with common trisomy (two of which were combined with other abnormalities) and 8/240 (3.33%) with CNV. In the SCAs group, 140/413 (33.90%) were confirmed as chromosome abnormalities including 115/140 (82.14%) with sex chromosome aneuploidy (one of which was combined with autosome CNV), 26/140 (18.57%) with only CNV and one with structural abnormality. In the other chromosomal abnormalities group, 37/214 (17.29%) were confirmed as chromosome abnormalities including 2/37 (5.41%) with chromosome aneuploidy, 28/37 (75.68%) with CNV and 7/37 (18.92%) with absence of heterozygosity (AOH). The NIPS exception types, diagnostic methods, and results of amniocentesis are presented in Table 2.
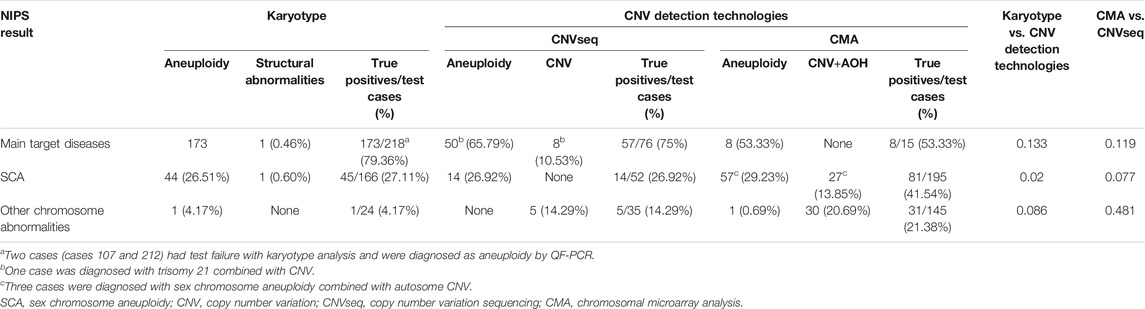
TABLE 2. Comparison of positive rates by different detection methods for the cases with different types of NIPS-positive results.
Prenatal Diagnosis of Cases With Positive NIPS Result for the Main Target Disease
A common trisomy was found in 233 (75.40%) cases (T21, 198; T18, 30; and T13, 5), including five cases of mosaic trisomy (three mosaic T21, one mosaic T18, and one mosaic T13) and five cases of robertsonian translocation [three 46,X?,rob(14;21)(q10;q10),+21, one 46,X?,rob(15;21)(q10;q10),+21, and one 46,X?,+21,rob(21;21)(q10;q10)]. Additional findings of nine cases are listed in Table 3. The levels of other exceptions detected ranged from 0.46%(1/218) to 8.79% (8/91) based on results of karyotyping and CNV detection technologies, respectively. Of these, 66.67% involved chromosomes with abnormal Z-values (n = 6) and 33.33% involved other autosomes (chromosomes 2 and 3). A total of 282 patients in the main target disease group underwent the QF-PCR test. Compared with the final reports, the QF-PCR assay correctly identified all 207 cases of target aneuploidies (178 T21, 25 T18, and 4 T13), including five mosaics, and helped confirm aneuploidies in two cases (cases 107 and 212) with culture failure. The QF-PCR result of case 282 showed that both D13S628 and D13S634 markers illustrated triallelic patterns, while the two markers D13S742, and D13S305 exhibited clear heterozygous patterns within the normal range. The karyotype of the pregnant woman was normal, and the karyotype of the fetal father was 46,XY,t(12;13)(q24;q14). No significant difference was observed in the positive rate between QF-PCR and karyotyping (p = 0.381), QF-PCR and CNV detection techniques (p = 0.330), karyotyping and CNV detection techniques (p = 0.133), and CNVseq and CMA (p = 0.119) for the main target disease group.
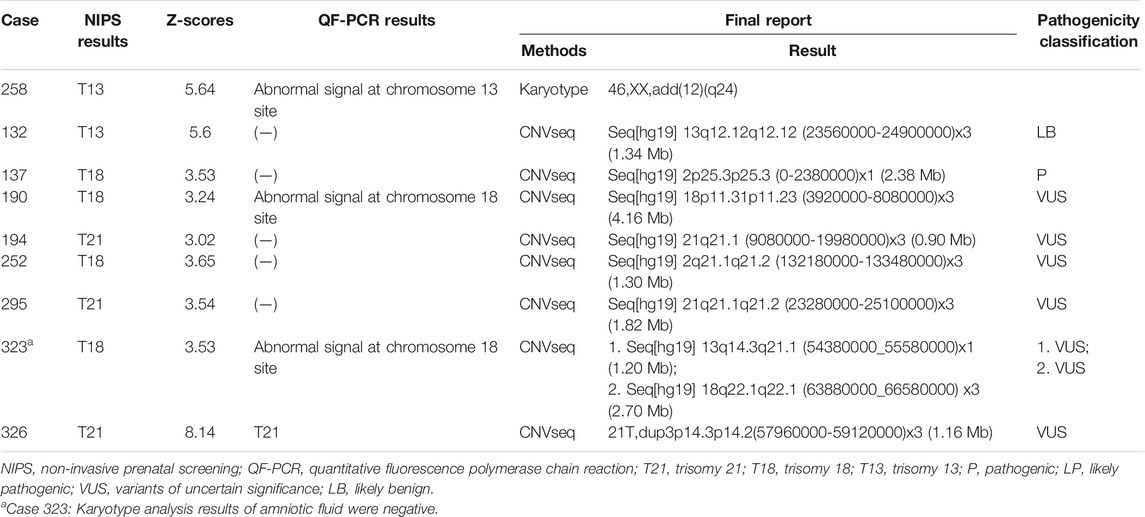
TABLE 3. Additional findings by amniocentesis in 9 cases with positive NIPS for main target diseases.
Prenatal Diagnosis of Cases With Positive NIPS Result for SCA
The positive karyotyping rate was lower than that of the CNV detection methods (p = 0.02). One hundred and fifteen (27.85%) cases were confirmed as sex chromosome aneuploidies by amniocentesis (two cases of monosomy X, 22 of XXX, 47 of XXY, 24 of XYY, one of XXYY, and 19 of sex chromosome mosaicism). The 19 cases with amniotic fluid findings involving sex chromosome mosaicisms are summarized in Table 4. There were eight other cases confirmed to involve sex chromosome abnormalities, including one sexual chromosome structural abnormality [45,X,del(X)(q24)] and seven sex chromosome CNVs [four were classified as pathogenic CNV (pCNV)]. Additionally, 20 fetuses were confirmed to have autosomal CNVs. Among the 27 CNVs found by CNV detection methods, eight were classified as pCNV or likely pCNV as shown in Table 5, including two CNVs with an abnormal size of >5 Mb and six with an abnormal size of <5 Mb. The other 19 CNVs with an abnormal size of <5 Mb were identified as two likely benign and 17 VUS.
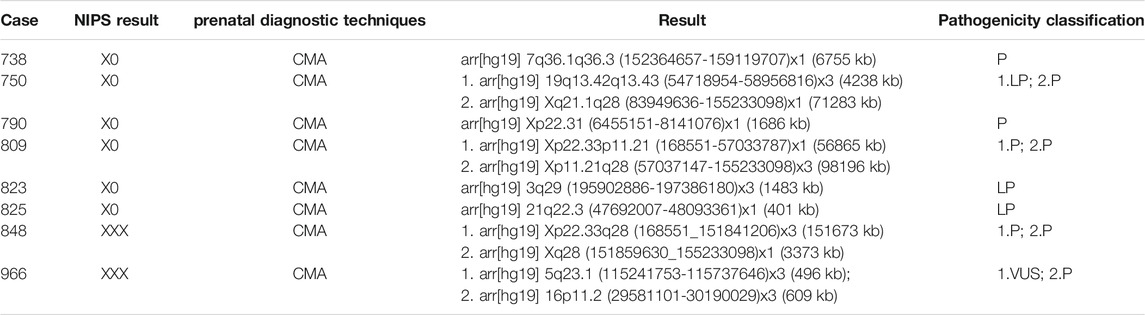
TABLE 5. Likely pathogenic and pathogenic CNVs by CNV detection techniques in 8 cases with positive NIPS for SCA.
Moreover, a sex chromosome mosaicism (case 840, Table 7) confirmed by peripheral karyotyping of the newborn was revealed upon follow-up investigation. The positive prediction value (PPV) of NIPS was 28.09% (116/413) for detecting fetal SCAs.
Prenatal Diagnosis of Cases With Positive NIPS Result for Other Chromosomal Abnormalities
Of 204 cases with positive NIPS result for other autosomal abnormalities, amniocentesis confirmed 37 cases with chromosomal abnormalities and only 23 with results corresponding to that of NIPS. The remaining 14 cases were confirmed with additional discoveries <5 Mb, including three pCNVs. The overall PPV was 11.27% (23/204); however, this percentage differed per chromosome. CNV detection techniques identified 28 cases of CNV, seven cases of AOH, and one T16 mosaicism. Of the total observed CNVs, 57.14% (16/28) involved CNVs with an abnormal size of <5 Mb, including three classified as pCNV, 12 as VUS, and 1 as likely benign. Furthermore, 42.86% (12/28) involved CNVs with an abnormal size of >5 Mb, which were classified as follows: six of pCNV, two of likely pathogenic (LP), and four of VUS. Likely pathogenic and pathogenic CNVs in 11 cases were shown in Table 6. One of seven AOHs was diagnosed as maternal uniparental disomy of chromosome 6 [upd (6)mat], following further testing of the parental samples. One case of mosaicism (47,XX,+9 [4]/46,XX [16]) was detected in 24 patients who underwent karyotyping.
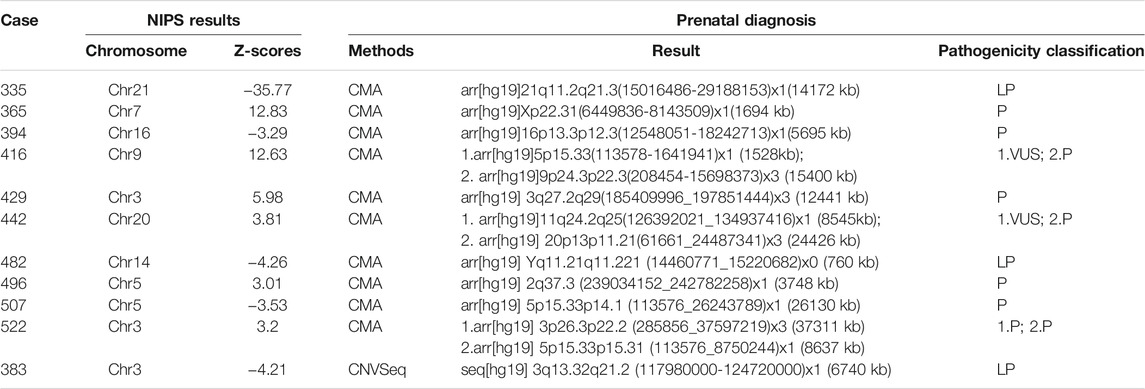
TABLE 6. Likely pathogenic and pathogenic CNVs by CNV detection techniques in 11 cases with positive NIPS for other chromosomal abnormalities.
Follow-up Investigation of 508 Cases With Initial Positive NIPS Results and Subsequent Negative Results in Amniocentesis
A total of 125 cases were lost to follow-up, and eight cases terminated the pregnancy due to complications during pregnancy, such as hypothyroidism and gestational hypertension. Among the 383 cases that were followed up, seven abnormal newborns were observed (Table 7). One abnormal newborn with perineal urethral stenosis underwent peripheral karyotyping and was diagnosed with a sex chromosome mosaicism (45,X [24]/46,XY [36]). Through the appraisal of growth and development by senior pediatricians for the rest neonates, there were no T21, T18, or T13; and no SCA or related abnormalities were reported during neonatal examination and follow-up.
Discussion
With the popularisation of medical knowledge, prenatal testing has been gaining increasing attention. The ACMG emphasises that detailed pre-test counselling and obtaining informed consent of pregnant women are the basis for expanding the scope of NIPS(Gregg et al., 2016). For an increasing number of pregnant women, NIPS is becoming an option to achieve high-resolution, sensitive, and specific detection of common trisomy and additional findings (van Schendel et al., 2015; van der Meij et al., 2019), as confirmed in this study. The PPV of NIPS was 75.40% for the main target disease, 28.09% for SCA, and 11.27% for other abnormalities, which was slightly lower than that predicted by previous studies (Norton et al., 2015; Deng et al., 2019; van der Meij et al., 2019). This might be relate to the fact that only pregnant women undergoing amniocentesis were included in this study.
Karyotyping is the most common validation method for positive NIPS results in many laboratories, followed by CMA, according to previous reports (Dobson et al., 2016; Cherry et al., 2017; Xu et al., 2020). Several problems have been identified in the actual application process. Culturing amniotic fluid cells and G-banding analysis make karyotyping time-consuming and requiring technical expertise; moreover, the long reporting period leads to anxiety in patients. In addition, karyotyping cannot reliably detect genomic segment rearrangements of <5–10 Mb. CMA can detect changes as small as 5–10 kb in size; however, its low throughput and high cost are obvious drawbacks.
Owing to the high PPV of NIPS for the main target disease, a rapid validation result is significant for pregnant women, and it could reduce parental anxiety and improve pregnancy management (Hultén et al., 2003; Ju et al., 2021). QF-PCR can be used to detect common aneuploidies within 24–48 h. When used alone, it helps to reduce parental anxiety and healthcare cost (Hills et al., 2010; de la Paz-Gallardo et al., 2015). Consistent with other clinical research reports (Voglino et al., 2002; Nicolini et al., 2004), the coincidence rate of QF-PCR and karyotyping in the diagnosis of common trisomies in this study was 100%. The results of QF-PCR did help these pregnant women to plan the next steps of pregnancy management more quickly. There has been controversy over whether QF-PCR can replace karyotyping as a stand-alone method in the general pregnant population, because a residual risk exists for clinically significant chromosomal abnormalities associated with normal QF-PCR results. QF-PCR is used in prenatal diagnostics exclusively for the analysis of numerical changes. Herein, the miss rate of QF-PCR tests at the karyotype detection level was 0.32% (1/309)—slightly higher than 0.069–0.12% reported in several studies with a large number of patients (Comas et al., 2010; Hills et al., 2010; Papoulidis et al., 2012); one of the primary causes for this result was the relatively small number of cases in our study. Theoretically, NIPS has a wider screening range and lower omission rate than traditional screening, which should translate to a lower residual risk of clinically significant chromosomal abnormalities associated with normal QF-PCR results in the main target disease group; however, further research is required to confirm this.
In some cases in the main target disease group, additional findings by karyotyping and CNV detection methods provided important information for effective genetic counseling. Robertsonian translocation accounts for approximately 2.31% (5/216) of all Down syndrome cases diagnosed by karyotyping in our study. QF-PCR is unable to distinguish the complete trisomy 21 from the trisomy as a consequence of translocation of chromosome 21, that is unlikely to affect present pregnancy management; however, it may affect the assessment of risk of recurrence. In case 258, considering the QF-PCR result (D13S628 and D13S634 markers both indicate the triallelic patterns) and karyotyping result (46,XX,add (12)(q24)), it suggested that there may be a balanced translocation carrier in fetal parents. Fetal father was identified as a carrier by further karyotyping, which provided an important basis for assessing the risk of offspring with an unbalanced structural abnormality. In a situation like this, karyotyping was a basic technique which cannot be replaced by other prenatal diagnostic techniques. Using CNV detection technology, we found that 6.59% (5/91) had corresponding chromosome microduplication rather than full trisomy, including case 190 and case 323 with ambiguous QF-PCR results. These findings demonstrated that submicroscopic duplications may result in NIPS showing a high-risk of trisomy, and CNV detection methods can aid in the clarification of abnormalities detected by NIPS. Furthermore, CNV detection technology has a 1.10% (1/91) higher yield of pathogenic CNVs than traditional karyotype analysis in women with positive NIPS resut for T21/T18/T13; this is in line with the findings in the general population of pregnant women (Wang et al., 2018). CNV detection techniques increased additional positive results for fetuses with trisomy 21/18/13 suspected by NIPS; however, the majority of additional findings (75%, 6/8) were classified as VUS, which increased the difficulty in genetic counselling and caused anxiety in pregnant women.
Here, the positive rate of the chromosomal aberration in the SCA group detected by karyotyping was lower than that by the CNV detection methods (p = 0.02), thus confirming that the performance of karyotyping is lower than that of CNV detection methods in confirming the SCAs predicted by NIPS. Sund et al. reported additional risks for structural abnormalities of sex chromosomes in fetuses with a noninvasive prenatal screen positive for SCA(Sund et al., 2020). A recent study has shown that the CNV detection method may provide additional findings for women with positive NIPS results for fetal SCA(Xu et al., 2020). In this study, 2.43% (6/247) fetuses in the SCA group were diagnosed with pCNV or likely pCNV (<5 Mb) by CNV detection methods (Table 5). This further highlights that the CNV detection technique can increase the diagnostic yield for women with positive NIPS result for SCA and should be recommended. In the other chromosomal abnormalities group, the detection rates of CNV detection methods and karyotyping were not significantly different. Nevertheless, caution should be exerted when interpreting the lack of statistical significance, as a relatively small number of people chose karyotyping in our study. Based on the assumption that the resolution of karyotyping is on the order of 5–10 Mb, 8.89% (16/180) anomalies diagnosed as <5 Mb by the CNV detection method would be missed by karyotyping in the other chromosomal abnormalities group, including 1.67% (3/180) classified as pathogenic CNV. Furthermore, there were seven (4.83%) cases of AOH identified by CMA, including one (0.69%) that was ultimately diagnosed with uniparental disomy (UPD). AOH provides clues to explore recessive diseases, and UPD for a clinically relevant chromosome (chromosomes 6, 7, 11, 14, 15, or 20) can result in abnormal phenotypes (Shaffer et al., 2001). The corresponding trisomy associated with UPD, which usually presents only in the placenta, can result in an abnormal NIPS Z-scores (Van Opstal et al., 2018). The ACMG statement indicated that any imprinted regions or chromosomes reportedly involved in positive NIPS cases with discordant results should consider undergoing specific UPD analyses (Cherry et al., 2017).
In the past few years, CMA has been widely recommended as the first-tier test for high-risk pregnancies to identify microscopic/submicroscopic CNVs(Chung et al., 2020; Wang et al., 2020). However, its low throughput, high cost, and complicated operation hinder widespread use. CNVseq based on massively parallel sequencing has several beneficial features, such as low cost, high throughput, low demand of DNA (10 ng), and high resolution (0.1 Mb). CNVseq has been demonstrated to be a suitable first-tier diagnostic test for the identification of clinically significant fetal chromosome anomalies, and it has shown similar effectiveness and advantages in the clinical diagnosis of aneuploidy and pCNVs(Ma et al., 2021). CNVseq could even detect some CNVs that were omitted by CMA because of insufficient probes within the corresponding regions and showed a higher sensitivity in detecting low-level mosaicism than CMA (Walker et al., 2019; Wang et al., 2020). Herein, CMA and CNVseq showed no significant differences in the positive rate in the three types of abnormalities indicated by NIPS, which further indicate that CNVseq represents an economic alternative to increase the abnormality detection rate in pregnant women with positive NIPS results. However, CNVseq cannot fully replace CMA. Except for aneuploidy, chromosomal CNV, and mosaicism, CMA based on the SNP platform can identify AOH and UPD; hence, cases with imprinted chromosome abnormalities predicted by NIPS may benefit more from CMA. However, neither CMA nor CNVseq can identify balanced translocations, and neither aCGH array nor CNVseq can identify triploidy. Neither of these chromosomal abnormalities can be detected by NIPS. Thus, karyotyping remains a valuable method for prenatal diagnosis and may provide unexpected yields for NIPS-positive pregnant women.
Additionally, the sex chromosome mosaicism was relatively frequently diagnosed in this study, accounting for 16.13% (20/124) of cases in the group with confirmed sex chromosome abnormalities. The frequency was similar to the rate of 13.74% (18/131) in a review of large case series of NIPS(Xie et al., 2020). Accurate identification of fetal mosaicism has clinical importance in guiding genetic counselling. FISH detection, using targeted locus-specific probes to observe the chromosomes of each cell without cell culture, demonstrates unique advantages in identifying chromosomal mosaicism. FISH can identify true fetal mosaicism by detecting uncultured amniocytes. In addition, FISH can be used to detect placental mosaicism. Confined placental mosaicism is one of the main reasons for the inconsistency between NIPS results and prenatal diagnosis results (Grati, 2016). A reflex FISH test is recommended to confirm chromosome mosaicism when a mosaic pattern is highly suspected (Hultén et al., 2003; Cherry et al., 2017; Wang et al., 2018; Chai et al., 2019). Compared with the FISH results, we found a higher proportion of the 45,X counted by karyotyping. Besides the different number of cells analysed, better growth of the abnormal cell type is likely to be the major contributor. Culture and harvesting of amniotic fluid cells may lead to changes in the mosaic rate of different cell lines and even cause pseudo-mosaicism (Grati, 2014; Chai et al., 2019). Moreover, overgrowth by the X0 cell line might explain why the FISH results for cases 561 and 568 were inconsistent with those of karyotyping. Contrary to the previous two cases, the karyotyping of case 588 revealed mosaicism, whereas all 100 cells analysed by FISH were X0. Maternal cell contamination is recognized as the most likely interpretation. However, the chance of true fetal mosaicism cannot be completely ruled out. Compared with karyotyping, CNV detection techniques can test uncultured amniotic fluid cells, thus reducing the influence of cell culture on the mosaic ratio and restrictions of the cell cycle on prenatal diagnosis. However, owing to fluctuations in the fluorescence signal intensity and background noise, CMA has a low sensitivity to a lower proportion of mosaic patterns than karyotype analysis and CNVseq (Chai et al., 2019; Hao et al., 2020). The CMA result of amniotic fluid cell testing for case 840 was inconsistent with that of karyotyping of neonatal peripheral blood; the false negative result of CMA may be caused by the above reasons or tissue-specific chimerism. Previous studies have confirmed that CNVseq with increased sensitivity for mosaicism can accurately and reproducibly quantitate mosaicism at levels of 20% (Ruttanajit et al., 2016; Wang et al., 2020). However, neither CMA nor CNVseq can recognize fetuses with balanced mosaics made up of different cell lines (such as 47,XXX/45,X) (Markus-Bustani et al., 2012). Therefore, the FISH assay should additionally be performed to derive a more accurate karyotype and more accurately define the levels of sex chromosome mosaicism.
Conclusion
For the majority of pregnant woman with a high-risk of common trisomy predicted by NIPS, QF-PCR stand-alone testing represents an economical and rapid method for prenatal diagnosis. Combined with karyotyping and CNV detection methods, some additional findings can be obtained, which can provide more comprehensive information for genetic counseling. When NIPS shows a positive result for SCA or other abnormalities, CNVseq can be employed as a valid and cost-effective alternative for CMA, which can improve the detection rate of pathogenic or potentially pathogenic chromosomal abnormalities. Considering the peculiarities of sex chromosomal mosaicism, the addition of the FISH assay can help identify true fetal mosaicism.
Data Availability Statement
The data analyzed in this study is subject to the following licenses/restrictions: The data for this article are not publicly available because of privacy concerns. Requests to access these datasets should be directed to the corresponding authors.
Ethics Statement
The studies involving human participants were reviewed and approved by the Institutional Ethics Committee of Sichuan University. The patients/participants provided their written informed consent to participate in this study.
Author Contributions
XJ and HL designed the study, analysed data, and wrote the manuscript. QiZ, SL, and JL analysed the experimental results. TB, CD, TX, YLi, JC, XW, LX, and YLu carried out the experiments. QuZ, LC, LL, and JW assisted with querying data. All authors reviewed and approved the final version of the manuscript.
Conflict of Interest
The authors declare that the research was conducted in the absence of any commercial or financial relationships that could be construed as a potential conflict of interest.
Publisher’s Note
All claims expressed in this article are solely those of the authors and do not necessarily represent those of their affiliated organizations, or those of the publisher, the editors and the reviewers. Any product that may be evaluated in this article, or claim that may be made by its manufacturer, is not guaranteed or endorsed by the publisher.
Acknowledgments
We thank all the pregnant women who participated in this study.
References
Chai, H., DiAdamo, A., Grommisch, B., Boyle, J., Amato, K., Wang, D., et al. (2019). Integrated FISH, Karyotyping and aCGH Analyses for Effective Prenatal Diagnosis of Common Aneuploidies and Other Cytogenomic Abnormalities. Med. Sci. 7 (2), 16. doi:10.3390/medsci7020016
Cherry, A. M., Akkari, Y. M., Barr, K. M., Kearney, H. M., Rose, N. C., South, S. T., et al. (2017). Diagnostic Cytogenetic Testing Following Positive Noninvasive Prenatal Screening Results: a Clinical Laboratory Practice Resource of the American College of Medical Genetics and Genomics (ACMG). Genet. Med. 19 (8), 845–850. doi:10.1038/gim.2017.91
Chung, C. C. Y., Chan, K. Y. K., Hui, P. W., Au, P. K. C., Tam, W. K., Li, S. K. M., et al. (2020). Cost-effectiveness Analysis of Chromosomal Microarray as a Primary Test for Prenatal Diagnosis in Hong Kong. BMC Pregnancy Childbirth 20 (1), 109. doi:10.1186/s12884-020-2772-y
Comas, C., Echevarria, M., Carrera, M., and Serra, B. (2010). Rapid Aneuploidy Testingversustraditional Karyotyping in Amniocentesis for Certain Referral Indications. J. Maternal-Fetal Neonatal Med. 23 (9), 949–955. doi:10.3109/14767050903334893
de la Paz-Gallardo, M. J., García, F. S. M., de Haro-Muñoz, T., Padilla-Vinuesa, M. C., Zafra-Ceres, M., Gomez-Capilla, J. A., et al. (2015). Quantitative-fluorescent-PCR versus Full Karyotyping in Prenatal Diagnosis of Common Chromosome Aneuploidies in Southern Spain. Clin. Chem. Lab. Med. 53 (9), 1333–1338. doi:10.1515/cclm-2014-0781
Deng, C., Zhu, Q., Liu, S., Liu, J., Bai, T., Jing, X., et al. (2019). Clinical Application of Noninvasive Prenatal Screening for Sex Chromosome Aneuploidies in 50,301 Pregnancies: Initial Experience in a Chinese Hospital. Sci. Rep. 9 (1), 7767. doi:10.1038/s41598-019-44018-4
Dobson, L. J., Reiff, E. S., Little, S. E., Wilkins-Haug, L., and Bromley, B. (2016). Patient Choice and Clinical Outcomes Following Positive Noninvasive Prenatal Screening for Aneuploidy with Cell-free DNA (cfDNA). Prenat Diagn. 36 (5), 456–462. doi:10.1002/pd.4805
Grati, F. (2014). Chromosomal Mosaicism in Human Feto-Placental Development: Implications for Prenatal Diagnosis. Jcm 3 (3), 809–837. doi:10.3390/jcm3030809
Grati, F. R. (2016). Implications of Fetoplacental Mosaicism on Cell-free DNA Testing: a Review of a Common Biological Phenomenon. Ultrasound Obstet. Gynecol. 48 (4), 415–423. doi:10.1002/uog.15975
Gregg, A. R., Skotko, B. G., Benkendorf, J. L., Monaghan, K. G., Bajaj, K., Best, R. G., et al. (2016). Noninvasive Prenatal Screening for Fetal Aneuploidy, 2016 Update: a Position Statement of the American College of Medical Genetics and Genomics. Genet. Med. 18 (10), 1056–1065. doi:10.1038/gim.2016.97
Grömminger, S., Yagmur, E., Erkan, S., Nagy, S., Schöck, U., Bonnet, J., et al. (2014). Fetal Aneuploidy Detection by Cell-free DNA Sequencing for Multiple Pregnancies and Quality Issues with Vanishing Twins. J. Clin. Med. 3 (3), 679–692. doi:10.3390/jcm3030679
Hao, M., Li, L., Zhang, H., Li, L., Liu, R., and Yu, Y. (2020). The Difference between Karyotype Analysis and Chromosome Microarray for Mosaicism of Aneuploid Chromosomes in Prenatal Diagnosis. J. Clin. Lab. Anal. 34 (12), e23514. doi:10.1002/jcla.23514
Hills, A., Donaghue, C., Waters, J., Waters, K., Sullivan, C., Kulkarni, A., et al. (2010). QF-PCR as a Stand-Alone Test for Prenatal Samples: the First 2 years' Experience in the London Region. Prenat. Diagn. 30 (6), a–n. doi:10.1002/pd.2503
Hultén, M., Dhanjal, S., and Pertl, B. (2003). Rapid and Simple Prenatal Diagnosis of Common Chromosome Disorders: Advantages and Disadvantages of the Molecular Methods FISH and QF-PCR. Reproduction 126 (3), 279–297. doi:10.1530/rep.0.1260279
Ji, X., Chen, F., Zhou, Y., Li, J., Yuan, Y., Mo, Y., et al. (2018). Copy Number Variation Profile in Noninvasive Prenatal Testing (NIPT) Can Identify Co-existing Maternal Malignancies: Case Reports and a Literature Review. Taiwanese J. Obstet. Gynecol. 57 (6), 871–877. doi:10.1016/j.tjog.2018.10.032
Ju, D., Li, X., Shi, Y., Ma, Y., Guo, L., Wang, Y., et al. (2021). Evaluation of the Practical Applications of Fluorescence In Situ Hybridization in the Prenatal Diagnosis of Positive Noninvasive Prenatal Screenings. J. Maternal-Fetal Neonatal Med. 1-8, 1–8. doi:10.1080/14767058.2021.1949449
Lefkowitz, R. B., Tynan, J. A., Liu, T., Wu, Y., Mazloom, A. R., Almasri, E., et al. (2016). Clinical Validation of a Noninvasive Prenatal Test for Genomewide Detection of Fetal Copy Number Variants. Am. J. Obstet. Gynecol. 215 (2), e1–227. doi:10.1016/j.ajog.2016.02.030
Liu, S., Liu, H., Liu, J., Bai, T., Jing, X., Xia, T., et al. (2021). Follow-up in Patients with Non-invasive Prenatal Screening Failures: A Reflection on the Choice of Further Prenatal Diagnosis. Front. Genet. 12, 666648. doi:10.3389/fgene.2021.666648
Ma, N., Xi, H., Chen, J., Peng, Y., Jia, Z., Yang, S., et al. (2021). Integrated CNV-Seq, Karyotyping and SNP-Array Analyses for Effective Prenatal Diagnosis of Chromosomal Mosaicism. BMC Med. Genomics 14 (1), 56. doi:10.1186/s12920-021-00899-x
Markus-Bustani, K., Yaron, Y., Goldstein, M., Orr-Urtreger, A., and Ben-Shachar, S. (2012). Undetected Sex Chromosome Aneuploidy by Chromosomal Microarray. Prenat Diagn. 32 (11), 1117–1118. doi:10.1002/pd.3979
Nicolaides, K. H., Syngelaki, A., Gil, M., Atanasova, V., and Markova, D. (2013). Validation of Targeted Sequencing of Single-Nucleotide Polymorphisms for Non-invasive Prenatal Detection of Aneuploidy of Chromosomes 13, 18, 21, X, and Y. Prenat Diagn. 33 (6), 575–579. doi:10.1002/pd.4103
Nicolini, U., Lalatta, F., Natacci, F., Curcio, C., and Bui, T.-H. (2004). The Introduction of QF-PCR in Prenatal Diagnosis of Fetal Aneuploidies: Time for Reconsideration. Hum. Reprod. Update 10 (6), 541–548. doi:10.1093/humupd/dmh046
Norton, M. E., Jacobsson, B., Swamy, G. K., Laurent, L. C., Ranzini, A. C., Brar, H., et al. (2015). Cell-free DNA Analysis for Noninvasive Examination of Trisomy. N. Engl. J. Med. 372 (17), 1589–1597. doi:10.1056/NEJMoa1407349
Papoulidis, I., Siomou, E., Sotiriadis, A., Efstathiou, G., Psara, A., Sevastopoulou, E., et al. (2012). Dual Testing with QF-PCR and Karyotype Analysis for Prenatal Diagnosis of Chromosomal Abnormalities. Evaluation of 13 500 Cases with Consideration of Using QF-PCR as a Stand-Alone Test According to Referral Indications. Prenat Diagn. 32 (7), 680–685. doi:10.1002/pd.3888
Richards, S., Aziz, N., Bale, S., Bick, D., Das, S., Gastier-Foster, J., et al. (2015). Standards and Guidelines for the Interpretation of Sequence Variants: a Joint Consensus Recommendation of the American College of Medical Genetics and Genomics and the Association for Molecular Pathology. Genet. Med. 17 (5), 405–424. doi:10.1038/gim.2015.30
Ruttanajit, T., Chanchamroen, S., Cram, D. S., Sawakwongpra, K., Suksalak, W., Leng, X., et al. (2016). Detection and Quantitation of Chromosomal Mosaicism in Human Blastocysts Using Copy Number Variation Sequencing. Prenat Diagn. 36 (2), 154–162. doi:10.1002/pd.4759
Shaffer, L. G., Agan, N., Goldberg, J. D., Ledbetter, D. H., Longshore, J. W., and Cassidy, S. B. (2001). American College of Medical Genetics Statement on Diagnostic Testing for Uniparental Disomy. Genet. Med. 3 (3), 206–211. doi:10.1097/00125817-200105000-00011
Su, S.-Y., Chueh, H.-Y., Li, C.-P., Chang, Y.-L., Chang, S.-D., and Chen, C.-P. (2015). Interphase Fluorescence In Situ Hybridization Assisting in Prenatal Counseling for Amniocentesis Karyotyping-Detected Fetal Mosaicism. Taiwanese J. Obstet. Gynecol. 54 (5), 588–591. doi:10.1016/j.tjog.2015.08.019
Sund, K. L., Khattar, D., Boomer, T., Caldwell, S., Dyer, L., Hopkin, R. J., et al. (2020). Confirmatory Testing Illustrates Additional Risks for Structural Sex Chromosome Abnormalities in Fetuses with a Non‐invasive Prenatal Screen Positive for Monosomy X. Am. J. Med. Genet. 184 (2), 294–301. doi:10.1002/ajmg.c.31783
van der Meij, K. R. M., Sistermans, E. A., Macville, M. V. E., Stevens, S. J. C., Bax, C. J., Bekker, M. N., et al. (2019). TRIDENT-2: National Implementation of Genome-wide Non-invasive Prenatal Testing as a First-Tier Screening Test in the Netherlands. Am. J. Hum. Genet. 105 (6), 1091–1101. doi:10.1016/j.ajhg.2019.10.005
Van Opstal, D., Diderich, K. E. M., Joosten, M., Govaerts, L. C. P., Polak, J., Boter, M., et al. (2018). Unexpected Finding of Uniparental Disomy Mosaicism in Term Placentas: Is it a Common Feature in Trisomic Placentas? Prenatal Diagn. 38 (12), 911–919. doi:10.1002/pd.5354
van Schendel, R. V., Dondorp, W. J., Timmermans, D. R. M., van Hugte, E. J. H., de Boer, A., Pajkrt, E., et al. (2015). NIPT-based Screening for Down Syndrome and beyond: what Do Pregnant Women Think? Prenat Diagn. 35 (6), 598–604. doi:10.1002/pd.4579
Voglino, G., Marongiu, A., Massobrio, M., Campogrande, M., and Todros, T. (2002). Rapid Prenatal Diagnosis of Aneuploidies. The Lancet 359 (9304), 442. doi:10.1016/s0140-6736(02)07572-4
Walker, L., Watson, C. M., Hewitt, S., Crinnion, L. A., Bonthron, D. T., and Cohen, K. E. (2019). An Alternative to Array-Based Diagnostics: a Prospectively Recruited Cohort, Comparing arrayCGH to Next-Generation Sequencing to Evaluate Foetal Structural Abnormalities. J. Obstet. Gynaecol. 39 (3), 328–334. doi:10.1080/01443615.2018.1522529
Wang, H., Dong, Z., Zhang, R., Chau, M. H. K., Yang, Z., Tsang, K. Y. C., et al. (2020). Low-pass Genome Sequencing versus Chromosomal Microarray Analysis: Implementation in Prenatal Diagnosis. Genet. Med. 22 (3), 500–510. doi:10.1038/s41436-019-0634-7
Wang, J., Chen, L., Zhou, C., Wang, L., Xie, H., Xiao, Y., et al. (2018). Prospective Chromosome Analysis of 3429 Amniocentesis Samples in China Using Copy Number Variation Sequencing. Am. J. Obstet. Gynecol. 219 (3), e1. doi:10.1016/j.ajog.2018.05.03
Xie, X., Tan, W., Li, F., Carrano, E., Ramirez, P., DiAdamo, A., et al. (2020). Diagnostic Cytogenetic Testing Following Positive Noninvasive Prenatal Screening Results of Sex Chromosome Abnormalities: Report of Five Cases and Systematic Review of Evidence. Mol. Genet. Genomic Med. 8 (7), e1297. doi:10.1002/mgg3.1297
Xu, H. B., Yang, H., Liu, G., and Chen, H. (2014). Systematic Review of Accuracy of Prenatal Diagnosis for Abnormal Chromosome Diseases by Microarray Technology. Genet. Mol. Res. 13 (4), 9115–9121. doi:10.4238/2014.October.31.27
Xu, J., Xue, Y., Wang, J., Zhou, Q., Zhang, B., Yu, B., et al. (2020). The Necessity of Prenatal Diagnosis by CMA for the Women with NIPS-Positive Results. Int. J. Genomics 2020, 1–7. doi:10.1155/2020/2145701
Keywords: aneuploidy, noninvasive prenatal screening, sex chromosome mosaicism, other chromosomal abnormalities, positive NIPS results, prenatal diagnostic techniques, sex chromosome aneuploidy
Citation: Jing X, Liu H, Zhu Q, Liu S, Liu J, Bai T, Deng C, Xia T, Liu Y, Cheng J, Wei X, Xing L, Luo Y, Zhou Q, Chen L, Li L and Wang J (2022) Clinical Selection of Prenatal Diagnostic Techniques Following Positive Noninvasive Prenatal Screening Results in Southwest China. Front. Genet. 12:811414. doi: 10.3389/fgene.2021.811414
Received: 08 November 2021; Accepted: 30 December 2021;
Published: 28 January 2022.
Edited by:
Damjan Glavač, University of Ljubljana, SloveniaReviewed by:
Thomas Liehr, Friedrich Schiller University Jena, GermanyFortunato Lonardo, Medical Genetics Unit, G. Rummo Hospital, Benevento, Italy
Copyright © 2022 Jing, Liu, Zhu, Liu, Liu, Bai, Deng, Xia, Liu, Cheng, Wei, Xing, Luo, Zhou, Chen, Li and Wang. This is an open-access article distributed under the terms of the Creative Commons Attribution License (CC BY). The use, distribution or reproduction in other forums is permitted, provided the original author(s) and the copyright owner(s) are credited and that the original publication in this journal is cited, in accordance with accepted academic practice. No use, distribution or reproduction is permitted which does not comply with these terms.
*Correspondence: Hongqian Liu, aG9uZ3FpYW4ubGl1QDE2My5jb20=; Qian Zhu, emh1cWlhbl8yMDA5QDE2My5jb20=