- 1College of Life Sciences and Food Engineering, Inner Mongolia Minzu University, Tongliao, China
- 2Network Center, Inner Mongolia Minzu University, Tongliao, China
- 3Affiliated Hospital of Inner Mongolia Minzu University, Tongliao, China
As one of common and severe mental illnesses, schizophrenia is difficult to be diagnosed exactly. Both its pathogenesis and the causes of its development are still uncertain because of its etiology complexity. At present, the diagnosis of schizophrenia is mainly based on the patient’s symptoms and signs, lacking reliable biomarkers that can be used for diagnosis. Circular RNAs in extracellular vesicles (EV circRNAs) can be used as promising candidate biomarkers for schizophrenia and other diseases, for they are not only high stability and disease specificity, but also are rich in contents and easy to be detected. The review is to focus on the research progress of the correlation between circRNAs and schizophrenia, and then to explores the possibility of EV circRNAs as new biomarkers for the schizophrenia diagnosis.
Introduction
Schizophrenia is a severe mental disease which is caused by both environmental and genetics factors (Ershova et al., 2019). Schizophrenia affects about 1% of world’s population (Mueser and McGurk, 2004). More than 80%–90% of the inpatients in mental hospitals belong to schizophrenia patients (Mueser and McGurk, 2004). However, the status quo of schizophrenia diagnosis is that doctors primarily rely on their subjective perceptions and assessments of patients’ symptoms and signs. There are no biochemical or genetic biomarkers detected for the doctors to rely on. Therefore, to ascertain schizophrenia biomarkers constitutes a research hotspot in psychiatry. CircRNAs are endogenous and closed loop structure RNA molecules, which can regulate mammalian gene expression at different levels. CircRNAs are particularly abundant in mammalian brains, and participate in mammalian neurodevelopment and function, brain health maintenance, and prevention of neuropsychiatric diseases (Mahmoudi et al., 2019). It has been confirmed that abnormally expressed circRNAs are present in the different tissues of schizophrenia patients, and they may play important roles in the occurrence of schizophrenia (Mahmoudi et al., 2019). EVs are membranous vesicles that are actively secreted by cells, and are widely present in various body fluids. Since EV circRNAs have the characteristics of disease specificity, high stability, abundant contents, and easy to be detected, they can be used as potential biomarkers for many diseases including schizophrenia. Li, et al. (2020a) briefly reviewed circRNAs potential as diagnostic biomarkers for schizophrenia and depression (Li et al., 2020b). Singh, et al. (2022) carried out a review focusing on the circRNA expression and activities in different tissue samples (Singh et al., 2022). The present review is to provide a detailed study summary of circRNAs in schizophrenia and to explore the feasibility of EV circRNAs as new biomarkers for the diagnosis of schizophrenia.
Circular RNAs
CircRNAs are a special class of endogenous circular single-stranded RNA molecules are formed in the process of splicing. Sanger et al. (1976) first discovered circRNAs in plants (Sanger et al., 1976) and they were initially considered to be typical by-products of mRNA post-transcriptional modification (Cocquerelle et al., 1993). With the evidence gathered, it has been found that circRNAs can not only be detected in different tissue samples and body fluids, but also are found to participate in the normal developmental processes, physiology, and disease states.
Despite the fact that the expression levels of some exceptionally abundant circRNAs are higher than that of their cognate linear mRNAs (Salzman et al., 2013; Rybak-Wolf et al., 2015), the abundance of most of circRNAs are relatively lower than that of mRNAs in cytoplasm and circRNAs which exhibit diverse expression patterns in mammal tissues and cell types (Chen, 2020). A significant enrichment of circRNAs was observed in brains (Rybak-Wolf et al., 2015; Veno et al., 2015). Unlike linear RNAs, circRNAs contain covalently closed loops, which make them resistant to RNase R’s digestion. They are relatively stable molecules with a longer half-life and resistance to degradation than other RNA molecules (Enuka et al., 2016). According to their origin, they can be divided into exon circRNA (EcircRNA), intron circRNA (ciRNA) and exon-intron circRNA (EIcircRNA) (Figure 1). Therefore, they have potential to become the biomarkers and therapeutic targets for human diseases.
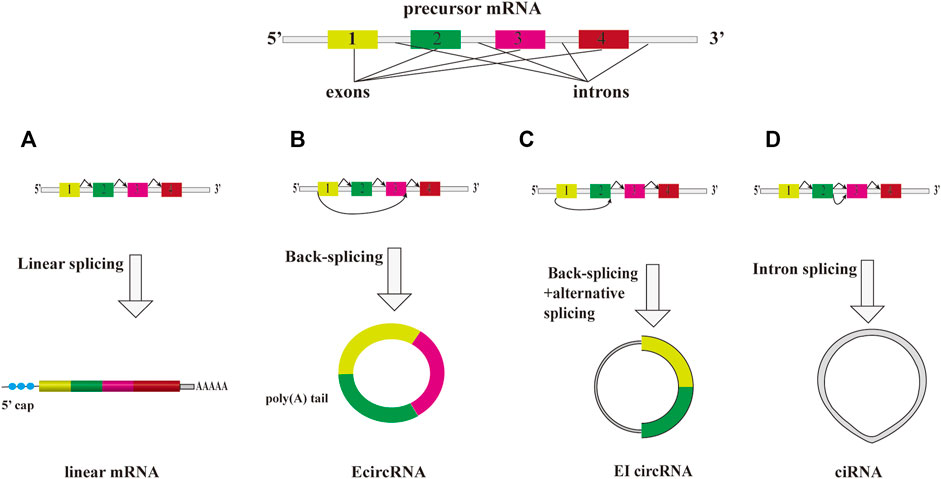
FIGURE 1. The overview diagram shows biosynthetic pathways of three major subclasses of circRNAs. A precursor mRNA (pre-mRNA) can produce a linear mRNA and circRNAs. (A) A precursor mRNA (pre-mRNA) can produce a linear mRNA undergo canonical splicing. Under back-splicing, it can produce a circRNA. They are three major subclasses of circRNAs, EcircRNA, ciRNA and EIcircRNA. (B) EcircRNA is the main group of circRNA class that consist of only exon(s). (C) cirRNA is derived from intron lariat. (D) EIcircRNA is composed of at least two exons and one retained intron.
Extracellular vesicles
Extracellular vesicles (EVs) are small diverse membrane vesicles limited by a lipid bilayer that are secreted by almost all kinds of cells into the extracellular space under both physiological and pathological states (Robbins and Morelli, 2014; van Niel et al., 2018; Gassama and Favereaux, 2021). The subtypes of EVs include exosomes, microvesicles, retrovirus like particles, and apoptotic bodies, etc. These EVs contain many kinds of factors including proteins, lipids and the genetic material from secreted cells to recipient cells. These factors are able to modify gene expression and activate immune responses of recipient cells at a distance (Robbins and Morelli, 2014; Gassama and Favereaux, 2021). EV is an additional mechanism for intercellular communication and can trigger multiple physiological and pathological processes such as immune surveillance and regulation of inflammation (Record et al., 2018).
The diameter of exosomes is about 30–150 nm that are actively secreted by cells to the outside and exist in most body fluids. Exosomes originate from the endosomal network. During endosomal maturation, intraluminal vesicles (ILVs) are generated by invagination of the limiting membrane of endosomes which result in the apparent selective sequestration of a small portion of cytosol (Stahl and Raposo, 2019). The endosomes that include ILVs are named multivesicular bodies (MVEs). MVBs can fuse with lysosomes for degradation of their contents or some multivesicular bodies fuse with the plasma membrane to release the ILVs into the extracellular environment. Once released, they are termed exosomes. Many different types of cells can secrete exosomes and it is a way of intercellular communication in normal and pathological states (Colombo et al., 2014). As a new mode of intercellular communication, exosomes can include biologically active macromolecules, transport from secreted cell to receptor cells, thereby finishing the exchange of genetic information between cells (Kalishwaralal et al., 2019). Many cells of the nervous system can release exosomes, such as neurons and glial cells can release exosomes (Von Bartheld and Altick, 2011; Chivet et al., 2013). Exosomes participate in the nervous system development and functions and the occurrence of neurological diseases (Osier et al., 2018; Zhang and Yang, 2018).
Microvesicles are another type of EVs which have different modes of the biogenesis of exosomes. Their formation occurs at the cellular plasma membrane during cell activation, apoptosis and mechanical injury (Deolindo et al., 2013). Microvesicles arise by outward budding and fission of plasma membrane that induced by translocation of phosphatidylserine to the outer-membrane leaflet (Akers et al., 2013; Raposo and Stoorvogel, 2013). Relative to exosomes, the size of microvesicles is large with the diameter range of 50–2,000 nm. Because they are also membranous vesicles, they can protect the contents from degradation and help them maintain biological activities in the process of long-term and long-distance transportation. By affecting autophagy, apoptosis and inflammation pathways, microvesicles and the cargos can change the biological effects of receptor cells (Lv et al., 2019). Neurons, astrocytes, microglia, and neural stem cells, have been described to release microvesicles and they involve in some central nervous diseases (Porro et al., 2015).
Apoptotic bodies are typically released from membrane blebs of apoptotic cells with the diameter ranging from 1,000 to 5,000 nm (Caruso and Poon, 2018). Apoptotic bodies are formed during the process of cell apoptosis process along with many intrinsic changes (Lu et al., 2005; Seo and Rhee, 2018). Apoptotic bodies are different from other subtypes of EVs and they encapsulate a spectrum of cargos, ranging from DNA fragments to intracellular components such as mitochondria (Xu et al., 2019; Kang et al., 2021). Retrovirus-like particles (RLPs) are non-infectious particles which resemble retroviral vesicles under Electron Microscope (EM). RLPs arise by direct budding from the plasma membrane (Bieda et al., 2001) and their diameter range are from 90 to 100 nm. A subset of retroviral proteins can be encapsulated in RLPs (Bronson et al., 1979; Mueller-Lantzsch et al., 1993; Dewannieux et al., 2005).
EVs are present in various biological fluids including peripheral blood, cerebrospinal fluid, urine, and saliva (Yuana et al., 2013). They can not only invade various tissues with the bloodstream, but also act as multimolecular messengers by an autocrine and paracrine manner. The uptake mode of EVs may be dependent on the type of cell and its physiologic state, and ligands on the surface of the EV and different cell types have different mechanisms of internalization (Abels and Breakefield, 2016).
It is well-known that intercellular communication is a vital event for multicellular organisms. Research about the intercellular communication mediated by EVs has attracted an increasing attention in recent years. EVs can play many roles in multiple biological processes. It is necessary to determine the potential physiological and pathological roles, clinical applications, and their relevance to disease. Because of their molecular cargos and easy accessibility, EVs have been suggested as enormous potential of biomarkers for the diagnosis of various diseases. EVs that released by cells of nervous system have been recognized as important modulators in the physiology of central nervous system and in neurodegenerative and neuroinflammatory disease states (Rufino-Ramos et al., 2017). At the same time, emerging evidences also indicates the potential utilities of EVs as early biomarkers for several brain disorders (Cheng et al., 2015; Zhao and Yang, 2021). EVs can pass the blood-brain barrier (Alvarez-Erviti et al., 2011). Recent studies suggested that brain-derived EVs were detected in rodent and human peripheral blood (Dickens et al., 2017; Mullins et al., 2017). Schizophrenia is generally considered to be a neurodevelopmental disorder of the brain. Increasing evidences suggested that inflammation contributes to occurrence and development of schizophrenia (van Beveren et al., 2014; Muller, 2018). EVs may contribute to the regulation of immune responses such as in autoimmune (Sáenz-Cuesta et al., 2014) and inflammatory diseases (Buzas et al., 2014) and infectious diseases (Silverman and Reiner, 2011). So, EVs are excellent candidate biomarkers for schizophrenia.
Correlations between circRNAs and schizophrenia
CircRNAs play important role in neurodevelopment and function, maintaining brain health, and preventing neuropsychiatric diseases (Zhuo et al., 2020). Multiple studies have confirmed that circRNAs are important regulators in normal developmental processes, physiology, and disease states, including cancer, mental illness, and cardiovascular disease (Floris et al., 2017; Liu et al., 2017; Han et al., 2018). At the same time, some studies have also revealed the relationship between circRNAs and neurological diseases (Errichelli et al., 2017; Bai et al., 2018). It has been confirmed that circRNAs are involved in the occurrence and development of a variety of neuropsychiatric diseases, such as schizophrenia (Mahmoudi et al., 2019), depression (Cui et al., 2016), Alzheimer’s disease (AD) (Akhter, 2018; Huang et al., 2018), epilepsy (Gong et al., 2018; Li et al., 2018) and Parkinson’s disease (PD) (Kumar et al., 2018).
It has been confirmed that there are abnormally expressed circRNAs in the brains of schizophrenia patients, and dysregulation circRNAs are involved in the occurrence of schizophrenia (Table 1). Piwecka, et al. (2017) have demonstrated that CDR1as regulates microRNA levels in the mammalian brain and knocked out CDR1as could make abnormal neural activity and behavioral disorders in mice and show impaired prepulse inhibition (PPI) (Piwecka et al., 2017). PPI is an important behavioral parameter for the measurement of the sensorimotor gating function is perfect. Sensorimotor gating dysregulation has been found in patients with neuropsychiatric disorders. In addition, knockout of the CDR1as locus of modeled mice also affected mRNAs encoding proteins involved in the maintenance of the mouse sleep-wake cycle. The study provides indirect evidence for the possible involvement of CDR1as in the pathogenesis of schizophrenia (Piwecka et al., 2017). As a large class of post-transcriptional regulators, circRNAs have been shown to act as a miRNA sponge/inhibitor to reduce miRNA activities, they are so-called competing endogenous RNAs. CDR1as was verified to act as a powerful miR-7 sponge to reduce miR-7 activity in developing midbrain of embryonic zebrafish and mouse brain (Hansen et al., 2013; Memczak et al., 2013). Mahmoudi, et al. (2019) performed transcriptome sequencing of the dorsal lateral prefrontal cortex (DLPFC) of 17 schizophrenia patients and 18 healthy controls and found that the overall expression of circRNAs in the DLPFC of schizophrenia showed a downward trend, and verified that hsa_circ_HP1BP3-7, hsa_circ_PPP2CA-3, hsa_circ_LONP2-6, hsa_circ_TOP1-10, hsa_circ_VCAN-2, hsa_circ_GPR137B-3, hsa_circ_ZNF236-2, and hsa_circ_MYO9A-66 were significantly downregulated in post-mortem cortex of schizophrenia patients by qRT-PCR (Mahmoudi et al., 2019). The expression of circHomer1a was verified to downregulated in the prefrontal cortex (PFC) of schizophrenia patients and schizophrenia patient-derived neural cells (Zimmerman et al., 2020).
At present, the study of schizophrenia-related peripheral blood circRNAs is still in its infancy. Abnormally expressed circRNAs were found in the peripheral blood of schizophrenic patients. Yao et al. (2019) verified that the expression level of hsa_circRNA_102101, hsa_circRNA_101836, and hsa_ circRNA_104597 are significantly downregulated, whereas those of hsa_circRNA_103102 and hsa_circRNA_103704 are significantly upregulated in peripheral blood mononuclear cells (PBMCs) of 102 schizophrenia patients compared with 103 healthy controls by RT-qPCR (Yao et al., 2019). After 8-week antipsychotic treatment, hsa_circRNA_104597 has changed to significant upregulation. So, the results indicated that hsa_circRNA_104597 can be schizophrenia diagnostic and therapeutic biomarker (Yao et al., 2019). Mahmoudi, et al. (2021) used deep RNA-seq technology to analyzed circRNA expression in PBMCs from 20 patients with schizophrenia, 19 patients with bipolar disorder as well as 20 controls. It showed that 22 and 33 circRNAs were significantly altered in PBMCs from individuals with schizophrenia and bipolar depression, respectively (Mahmoudi et al., 2021). Tan et al. (2021) found 44 differentially expressed circRNAs in the plasma exosomes of schizophrenia patients by high-throughput sequencing technology and verified that the expression levels of chr3_196488683_196483770_-4913, hsa_circ_0077837, hsa_circ_0001495, hsa_circ_0074371 and hsa_circ_0042174 were significantly downregulated in plasma EVs of six schizophrenia patients compared with six healthy controls by RT-qPCR (Tan et al., 2021). Liao, et al. (2022) used the whole transcriptome sequence technology to assess the expression profiles of circRNAs in the peripheral blood of three schizophrenia patients and three healthy controls, they found that 450 differentially expressed circRNAs were found to aberrantly express in the peripheral blood of schizophrenia patients (Liao et al., 2022). They further confirmed five circRNAs aberrantly expressed by RT-qPCR (Liao et al., 2022).
The lack of commonality was found in previous studies. The reasons for discordant results between previous studies are as follows. Firstly, the samples that used in these researches are different, some being peripheral blood, others PBMCs and still others plasma EVs. Although Yao et al. (2019) (Yao et al., 2019) and Mahmoudi et al. (2021) (Mahmoudi et al., 2021) used the same samples, the methods of them were different. Secondly, the sample numbers are small in Tan et al. (2021) and Liao et al. (2022).
Although the above studies have confirmed that circRNAs are significantly associated with schizophrenia, the roles in the pathogenesis of schizophrenia that circRNAs play are still a mystery and needs to be studied systematically. In addition, the associations of differentially expressed circRNAs with schizophrenia were mostly confirmed in brain. Since it is difficult to obtain samples from living human brain, biomarkers that abnormally expressed in brain tissue are difficult to be used for early warning and diagnosis of schizophrenia. They prompted scientists to verify biomarkers of schizophrenia from peripheral blood, especially from the EVs of peripheral blood. However, whether circRNAs in peripheral blood can accurately reflect the states of the brain and whether they are as specific biomarkers for brain-related diseases need further examination.
EV circRNAs as schizophrenia biomarkers
Biomarkers are biochemical and physiological indicators that have a very wide range of uses including change marking in the structure or function of cells, tissues, organs, and systems. The contents of EVs mirror the aspects of the secreting cell, including genetic and proteomic aspects (Akers et al., 2013). As a new mode of intercellular communication, EVs carry diverse molecules such as DNA, RNAs, protein, lipids, metabolites, and others, which transport from host cells to recipient cells, thereby affecting the function of recipient cells (van Niel et al., 2018; Kalishwaralal et al., 2019; Mathieu et al., 2019). In recent years, studies have shown that EVs are important carriers of circRNAs. The abnormally expressed circRNAs in the brain of schizophrenia patients can be transported through EVs, cross the blood-brain barrier, enter the circulatory system and exist stably (Zhuo et al., 2020). Due to the protective effect of EV membrane, the existence of circRNAs is more stable and abundant, and can better reflect the local state of the disease. RNA sequencing revealed that circRNAs are abundant in EVs of human Blood (Li et al., 2019). EV circRNAs have such characteristics, as disease specificity, high stability, content abundancy, and easy detection; these features make them to be used as promising candidate biomarkers for many diseases and have great potential and important significance in the development of new disease diagnosis and treatment methods. The overview diagram showing multiple characteristics and biological functions of EV circRNAs can be seen in Figure 2. Existing studies have linked EV circRNAs with tumors, confirming that they are excellent biomarkers for tumor diagnosis (Pan et al., 2019; Li et al., 2020a).
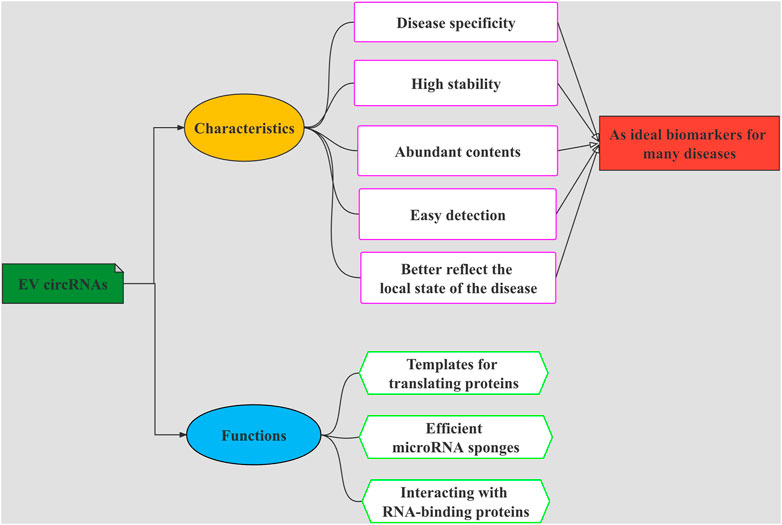
FIGURE 2. The overview diagram shows multiple characteristics and biological functions of EVs-circRNAs. The functions of EV circRNAs are as templates for translating proteins, efficient microRNA sponges, and interacting with RNA-binding proteins. EV circRNAs have such characteristics, as disease specificity, high stability, abundant contents, and easy to be detected. These functions and characteristics make them to be used as promising candidate biomarkers for many diseases.
Most of the research on EV circRNAs focusing on tumors, and their correlations with psychiatric diseases is still in its infancy. Although the fact that the expression of EV circRNAs synchronizing with that of the brain and can accurately reflecting the abnormal pathological state of the brain need to further verify, we must admit that EV circRNAs are promising candidates as biomarkers for neuropsychiatric diseases, especially for schizophrenia. However, the research on EV circRNAs as the biomarkers of psychiatric and neurological disorders is in its infancy, only a small number of literatures paying attention to EV circRNAs as biomarkers of schizophrenia. Only the study of Tan et al. (2021) explored plasma exosomal circRNAs as diagnostic biomarkers for schizophrenia and found that some circRNAs have the potential as diagnostic biomarkers and the therapeutic strategy for schizophrenia (Tan et al., 2021).
EV circRNAs participate in the occurrence and development of schizophrenia, so they have important scientific and clinical application values. Further and in-depth research on them will not only be conducive to the diagnosis, treatment and prognosis of schizophrenia, but also help to reveal the pathogenic mechanism of schizophrenia. With the further discoveries of more and more psychiatric disease-related EV circRNAs and the identification of their regulatory mechanisms, the role of EV circRNAs in the pathological mechanisms of schizophrenia can be clarified. Therefore, it is important to carry out the research of investigating the expression profile of circRNAs in EVs of patients with schizophrenia, exploring the feasibility of differentially expressed EV circRNAs as diagnostic markers for schizophrenia, and verifying biological pathways related to the occurrence of schizophrenia that EV circRNAs participate. Despite the fact that biology and utility of EVs have attracted much attention, the researches of EVs is still in the initial stage. Further research on EV circRNAs in schizophrenia may help to understand intercellular communications in diseased brains, and to find novel biomarkers and new therapeutic strategies in schizophrenia.
Conclusion
EV circRNAs participate in the pathogenesis of schizophrenia, have important scientific and clinical application values. Exploring EV circRNAs involvement in the occurrence and development of schizophrenia has increasingly become the direction of the diagnosis and treatment of schizophrenia. With the continuous improvement of detection, evaluation and intervention technologies, the relation studies between EV circRNAs and schizophrenia will not only help the diagnosis and treatment of schizophrenia, but also provide reference for the diagnosis and treatment of other psychiatric diseases. It is expected to become a research hotspot of mental illness.
Author contributions
KH designed the review and revised the manuscript. CG, HL, YB, and MG wrote the first draft of the manuscript. All authors contributed to and approved the final manuscript.
Funding
The study was financially supported by the Natural Science Foundations of China (Nos. 82060256 and 81660234) the Natural Science Foundation of Inner Mongolia (Nos. 2020MS08028 and 2021LHMS08032) and the Scientific Research Project of Inner Mongolia Minzu University (NMDYB20038).
Conflict of interest
The authors declare that the research was conducted in the absence of any commercial or financial relationships that could be construed as a potential conflict of interest.
Publisher’s note
All claims expressed in this article are solely those of the authors and do not necessarily represent those of their affiliated organizations, or those of the publisher, the editors and the reviewers. Any product that may be evaluated in this article, or claim that may be made by its manufacturer, is not guaranteed or endorsed by the publisher.
Abbreviations
DLPFC, Dorsal Lateral Prefrontal Cortex; PFC, Prefrontal Cortex; PBMCs, Peripheral Blood Mononuclear Cells (PBMCs); EM, ectron Microscope; EVs, Extracellular vesicles; ILVs, Intraluminal Vesicles; MVEs, multivesicular bodies; RLPs, Retrovirus-like particles.
References
Abels, E. R., and Breakefield, X. O. (2016). Introduction to extracellular vesicles: Biogenesis, RNA cargo selection, content, release, and uptake. Cell. Mol. Neurobiol. 36, 301–312. doi:10.1007/s10571-016-0366-z
Akers, J. C., Gonda, D., Kim, R., Carter, B. S., and Chen, C. C. (2013). Biogenesis of extracellular vesicles (EV): Exosomes, microvesicles, retrovirus-like vesicles, and apoptotic bodies. J. Neurooncol. 113, 1–11. doi:10.1007/s11060-013-1084-8
Akhter, R. (2018). Circular RNA and Alzheimer's disease. Adv. Exp. Med. Biol. 1087, 239–243. doi:10.1007/978-981-13-1426-1_19
Alvarez-Erviti, L., Seow, Y., Yin, H., Betts, C., Lakhal, S., and Wood, M. J. (2011). Delivery of siRNA to the mouse brain by systemic injection of targeted exosomes. Nat. Biotechnol. 29, 341–345. doi:10.1038/nbt.1807
Bai, Y., Zhang, Y., Han, B., Yang, L., Chen, X., Huang, R., et al. (2018). Circular RNA DLGAP4 ameliorates ischemic stroke outcomes by targeting miR-143 to regulate endothelial-mesenchymal transition associated with blood-brain barrier integrity. J. Neurosci. 38, 32–50. doi:10.1523/JNEUROSCI.1348-17.2017
Bieda, K., Hoffmann, A., and Boller, K. (2001). Phenotypic heterogeneity of human endogenous retrovirus particles produced by teratocarcinoma cell lines. J. Gen. Virol. 82, 591–596. doi:10.1099/0022-1317-82-3-591
Bronson, D. L., Fraley, E. E., Fogh, J., and Kalter, S. S. (1979). Induction of retrovirus particles in human testicular tumor (Tera-1) cell cultures: An electron microscopic study. Nat. Rev. Rheumatol. 63, 337–339.
Buzas, E. I., György, B., Nagy, G., Falus, A., and Gay, S. (2014). Emerging role of extracellular vesicles in inflammatory diseases. Nat. Rev. Rheumatol. 10, 356–364. doi:10.1038/nrrheum.2014.19
Caruso, S., and Poon, I. K. H. (2018). Apoptotic cell-derived extracellular vesicles: More than just debris. Front. Immunol. 9, 1486. doi:10.3389/fimmu.2018.01486
Chen, L. L. (2020). The expanding regulatory mechanisms and cellular functions of circular RNAs. Nat. Rev. Mol. Cell. Biol. 21, 475–490. doi:10.1038/s41580-020-0243-y
Cheng, L., Doecke, J. D., Sharples, R. A., Villemagne, V. L., Fowler, C. J., Rembach, A., et al. (2015). Prognostic serum miRNA biomarkers associated with Alzheimer's disease shows concordance with neuropsychological and neuroimaging assessment. Mol. Psychiatry 20, 1188–1196. doi:10.1038/mp.2014.127
Chivet, M., Javalet, C., Hemming, F., Pernet-Gallay, K., Laulagnier, K., Fraboulet, S., et al. (2013). Exosomes as a novel way of interneuronal communication. Biochem. Soc. Trans. 41, 241–244. doi:10.1042/BST20120266
Cocquerelle, C., Mascrez, B., Hetuin, D., and Bailleul, B. (1993). Mis-splicing yields circular RNA molecules. FASEB J. 7, 155–160. doi:10.1096/fasebj.7.1.7678559
Colombo, M., Raposo, G., and Thery, C. (2014). Biogenesis, secretion, and intercellular interactions of exosomes and other extracellular vesicles. Annu. Rev. Cell. Dev. Biol. 30, 255–289. doi:10.1146/annurev-cellbio-101512-122326
Cui, X., Niu, W., Kong, L., He, M., Jiang, K., Chen, S., et al. (2016). hsa_circRNA_103636: potential novel diagnostic and therapeutic biomarker in Major depressive disorder. Biomark. Med. 10, 943–952. doi:10.2217/bmm-2016-0130
Deolindo, P., Evans-Osses, I., and Ramirez, M. I. (2013). Microvesicles and exosomes as vehicles between protozoan and host cell communication. Biochem. Soc. Trans. 41, 252–257. doi:10.1042/bst20120217
Dewannieux, M., Blaise, S., and Heidmann, T. (2005). Identification of a functional envelope protein from the HERV-K family of human endogenous retroviruses. J. Virol. 79, 15573–15577. doi:10.1128/JVI.79.24.15573-15577.2005
Dickens, A. M., Tovar, Y. R. L. B., Yoo, S. W., Trout, A. L., Bae, M., Kanmogne, M., et al. (2017). Astrocyte-shed extracellular vesicles regulate the peripheral leukocyte response to inflammatory brain lesions. Sci. Signal. 10, eaai7696. doi:10.1126/scisignal.aai7696
Enuka, Y., Lauriola, M., Feldman, M. E., Sas-Chen, A., Ulitsky, I., and Yarden, Y. (2016). Circular RNAs are long-lived and display only minimal early alterations in response to a growth factor. Nucleic Acids Res. 44, 1370–1383. doi:10.1093/nar/gkv1367
Errichelli, L., Dini Modigliani, S., Laneve, P., Colantoni, A., Legnini, I., Capauto, D., et al. (2017). FUS affects circular RNA expression in murine embryonic stem cell-derived motor neurons. Nat. Commun. 8, 14741. doi:10.1038/ncomms14741
Ershova, E. S., Jestkova, E. M., Martynov, A. V., Shmarina, G. V., Umriukhin, P. E., Bravve, L. V., et al. (2019). Accumulation of circulating cell-free CpG-enriched ribosomal DNA fragments on the background of high endonuclease activity of blood plasma in schizophrenic patients. Int. J. Genomics 2019, 8390585. doi:10.1155/2019/8390585
Floris, G., Zhang, L., Follesa, P., and Sun, T. (2017). Regulatory role of circular RNAs and neurological disorders. Mol. Neurobiol. 54, 5156–5165. doi:10.1007/s12035-016-0055-4
Gassama, Y., and Favereaux, A. (2021). Emerging roles of extracellular vesicles in the central nervous system: Physiology, pathology, and therapeutic perspectives. Front. Cell. Neurosci. 15, 626043. doi:10.3389/fncel.2021.626043
Gong, G. H., An, F. M., Wang, Y., Bian, M., Wang, D., and Wei, C. X. (2018). Comprehensive circular RNA profiling reveals the regulatory role of the CircRNA-0067835/miR-155 pathway in temporal lobe epilepsy. Cell. Physiol. biochem. 51, 1399–1409. doi:10.1159/000495589
Han, B., Chao, J., and Yao, H. (2018). Circular RNA and its mechanisms in disease: From the bench to the clinic. Pharmacol. Ther. 187, 31–44. doi:10.1016/j.pharmthera.2018.01.010
Hansen, T. B., Jensen, T. I., Clausen, B. H., Bramsen, J. B., Finsen, B., Damgaard, C. K., et al. (2013). Natural RNA circles function as efficient microRNA sponges. Nature 495, 384–388. doi:10.1038/nature11993
Huang, J. L., Qin, M. C., Zhou, Y., Xu, Z. H., Yang, S. M., Zhang, F., et al. (2018). Comprehensive analysis of differentially expressed profiles of Alzheimer's disease associated circular RNAs in an Alzheimer's disease mouse model. Aging (Albany NY) 10, 253–265. doi:10.18632/aging.101387
Kalishwaralal, K., Kwon, W. Y., and Park, K. S. (2019). Exosomes for non-invasive cancer monitoring. Biotechnol. J. 14, e1800430. doi:10.1002/biot.201800430
Kang, T., Atukorala, I., and Mathivanan, S. (2021). Biogenesis of extracellular vesicles. Subcell. Biochem. 97, 19–43. doi:10.1007/978-3-030-67171-6_2
Kumar, L., ShamsuzzamaJadiya, P., Haque, R., Shukla, S., and Nazir, A. (2018). Functional characterization of novel circular RNA molecule, circzip-2 and its synthesizing gene zip-2 in C. elegans model of Parkinson's disease. Mol. Neurobiol. 55, 6914–6926. doi:10.1007/s12035-018-0903-5
Li, J., Lin, H., Sun, Z., Kong, G., Yan, X., Wang, Y., et al. (2018). High-throughput data of circular RNA profiles in human temporal cortex tissue reveals novel insights into temporal lobe epilepsy. Cell. Physiol. biochem. 45, 677–691. doi:10.1159/000487161
Li, T., Sun, X., and Chen, L. (2020a). Exosome circ_0044516 promotes prostate cancer cell proliferation and metastasis as a potential biomarker. J. Cell. Biochem. 121, 2118–2126. doi:10.1002/jcb.28239
Li, Y., Zhao, J., Yu, S., Wang, Z., He, X., Su, Y., et al. (2019). Extracellular vesicles long RNA sequencing reveals abundant mRNA, circRNA, and lncRNA in human blood as potential biomarkers for cancer diagnosis. Clin. Chem. 65, 798–808. doi:10.1373/clinchem.2018.301291
Li, Z., Liu, S., Li, X., Zhao, W., Li, J., and Xu, Y. (2020b). Circular RNA in schizophrenia and depression. Front. Psychiatry 11, 392. doi:10.3389/fpsyt.2020.00392
Liao, F., Zhu, L., Yang, J., Wu, X., Zhao, Z., Xu, B., et al. (2022). Whole transcriptome sequencing identified CircRNA profiles and the related networks in schizophrenia. J. Mol. Neurosci. 72, 1622–1635. doi:10.1007/s12031-022-02013-x
Liu, C., Yao, M. D., Li, C. P., Shan, K., Yang, H., Wang, J. J., et al. (2017). Silencing of circular RNA-znf609 ameliorates vascular endothelial dysfunction. Theranostics 7, 2863–2877. doi:10.7150/thno.19353
Lu, Z., Zhang, C., and Zhai, Z. (2005). Nucleoplasmin regulates chromatin condensation during apoptosis. Proc. Natl. Acad. Sci. U. S. A. 102, 2778–2783. doi:10.1073/pnas.0405374102
Lv, Y., Tan, J., Miao, Y., and Zhang, Q. (2019). The role of microvesicles and its active molecules in regulating cellular biology. J. Cell. Mol. Med. 23, 7894–7904. doi:10.1111/jcmm.14667
Mahmoudi, E., Fitzsimmons, C., Geaghan, M. P., Shannon Weickert, C., Atkins, J. R., Wang, X., et al. (2019). Circular RNA biogenesis is decreased in postmortem cortical gray matter in schizophrenia and may alter the bioavailability of associated miRNA. Neuropsychopharmacology 44, 1043–1054. doi:10.1038/s41386-019-0348-1
Mahmoudi, E., Green, M. J., and Cairns, M. J. (2021). Dysregulation of circRNA expression in the peripheral blood of individuals with schizophrenia and bipolar disorder. J. Mol. Med. 99, 981–991. doi:10.1007/s00109-021-02070-6
Mathieu, M., Martin-Jaular, L., Lavieu, G., and Thery, C. (2019). Specificities of secretion and uptake of exosomes and other extracellular vesicles for cell-to-cell communication. Nat. Cell. Biol. 21, 9–17. doi:10.1038/s41556-018-0250-9
Memczak, S., Jens, M., Elefsinioti, A., Torti, F., Krueger, J., Rybak, A., et al. (2013). Circular RNAs are a large class of animal RNAs with regulatory potency. Nature 495, 333–338. doi:10.1038/nature11928
Mueller-Lantzsch, N., Sauter, M., Weiskircher, A., Kramer, K., Best, B., Buck, M., et al. (1993). Human endogenous retroviral element K10 (HERV-K10) encodes a full-length gag homologous 73-kDa protein and a functional protease. AIDS Res. Hum. Retroviruses 9, 343–350. doi:10.1089/aid.1993.9.343
Mueser, K. T., and Mcgurk, S. R. (2004). Schizophrenia. Lancet 363, 2063–2072. doi:10.1016/S0140-6736(04)16458-1
Muller, N. (2018). Inflammation in schizophrenia: Pathogenetic aspects and therapeutic considerations. Schizophr. Bull. 44, 973–982. doi:10.1093/schbul/sby024
Mullins, R. J., Mustapic, M., Goetzl, E. J., and Kapogiannis, D. (2017). Exosomal biomarkers of brain insulin resistance associated with regional atrophy in Alzheimer's disease. Hum. Brain Mapp. 38, 1933–1940. doi:10.1002/hbm.23494
Osier, N., Motamedi, V., Edwards, K., Puccio, A., Diaz-Arrastia, R., Kenney, K., et al. (2018). Exosomes in acquired neurological disorders: New insights into pathophysiology and treatment. Mol. Neurobiol. 55, 9280–9293. doi:10.1007/s12035-018-1054-4
Pan, B., Qin, J., Liu, X., He, B., Wang, X., Pan, Y., et al. (2019). Identification of serum exosomal hsa-circ-0004771 as a novel diagnostic biomarker of colorectal cancer. Front. Genet. 10, 1096. doi:10.3389/fgene.2019.01096
Piwecka, M., Glazar, P., Hernandez-Miranda, L. R., Memczak, S., Wolf, S. A., Rybak-Wolf, A., et al. (2017). Loss of a mammalian circular RNA locus causes miRNA deregulation and affects brain function. Science 357, eaam8526. doi:10.1126/science.aam8526
Porro, C., Trotta, T., and Panaro, M. A. (2015). Microvesicles in the brain: Biomarker, messenger or mediator? J. Neuroimmunol. 288, 70–78. doi:10.1016/j.jneuroim.2015.09.006
Raposo, G., and Stoorvogel, W. (2013). Extracellular vesicles: Exosomes, microvesicles, and friends. J. Cell. Biol. 200, 373–383. doi:10.1083/jcb.201211138
Record, M., Silvente-Poirot, S., Poirot, M., and Wakelam, M. J. O. (2018). Extracellular vesicles: Lipids as key components of their biogenesis and functions. J. Lipid Res. 59, 1316–1324. doi:10.1194/jlr.E086173
Robbins, P. D., and Morelli, A. E. (2014). Regulation of immune responses by extracellular vesicles. Nat. Rev. Immunol. 14, 195–208. doi:10.1038/nri3622
Rufino-Ramos, D., Albuquerque, P. R., Carmona, V., Perfeito, R., Nobre, R. J., and Pereira De Almeida, L. (2017). Extracellular vesicles: Novel promising delivery systems for therapy of brain diseases. J. Control. Release 262, 247–258. doi:10.1016/j.jconrel.2017.07.001
Rybak-Wolf, A., Stottmeister, C., Glazar, P., Jens, M., Pino, N., Giusti, S., et al. (2015). Circular RNAs in the mammalian brain are highly abundant, conserved, and dynamically expressed. Mol. Cell. 58, 870–885. doi:10.1016/j.molcel.2015.03.027
Sáenz-Cuesta, M., Osorio-Querejeta, I., and Otaegui, D. (2014). Extracellular vesicles in multiple sclerosis: What are they telling us? Front. Cell. Neurosci. 8, 100. doi:10.3389/fncel.2014.00100
Salzman, J., Chen, R. E., Olsen, M. N., Wang, P. L., and Brown, P. O. (2013). Cell-type specific features of circular RNA expression. PLoS Genet. 9, e1003777. doi:10.1371/journal.pgen.1003777
Sanger, H. L., Klotz, G., Riesner, D., Gross, H. J., and Kleinschmidt, A. K. (1976). Viroids are single-stranded covalently closed circular RNA molecules existing as highly base-paired rod-like structures. Proc. Natl. Acad. Sci. U. S. A. 73, 3852–3856. doi:10.1073/pnas.73.11.3852
Seo, M. Y., and Rhee, K. (2018). Caspase-mediated cleavage of the centrosomal proteins during apoptosis. Cell. Death Dis. 9, 571. doi:10.1038/s41419-018-0632-8
Silverman, J. M., and Reiner, N. E. (2011). Exosomes and other microvesicles in infection biology: Organelles with unanticipated phenotypes. Cell. Microbiol. 13, 1–9. doi:10.1111/j.1462-5822.2010.01537.x
Singh, M., Dwibedy, S. L. L., Biswal, S. R., Muthuswamy, S., Kumar, A., and Kumar, S. (2022). Circular RNA: A novel and potential regulator in pathophysiology of schizophrenia. Metab. Brain Dis. 37, 1309–1316. doi:10.1007/s11011-022-00978-7
Stahl, P. D., and Raposo, G. (2019). Extracellular vesicles: Exosomes and microvesicles, integrators of homeostasis. Physiology 34, 169–177. doi:10.1152/physiol.00045.2018
Tan, G., Wang, L., Liu, Y., Zhang, H., Feng, W., and Liu, Z. (2021). The alterations of circular RNA expression in plasma exosomes from patients with schizophrenia. J. Cell. Physiol. 236, 458–467. doi:10.1002/jcp.29873
Van Beveren, N. J., Schwarz, E., Noll, R., Guest, P. C., Meijer, C., De Haan, L., et al. (2014). Evidence for disturbed insulin and growth hormone signaling as potential risk factors in the development of schizophrenia. Transl. Psychiatry 4, e430. doi:10.1038/tp.2014.52
Van Niel, G., D'angelo, G., and Raposo, G. (2018). Shedding light on the cell biology of extracellular vesicles. Nat. Rev. Mol. Cell. Biol. 19, 213–228. doi:10.1038/nrm.2017.125
Veno, M. T., Hansen, T. B., Veno, S. T., Clausen, B. H., Grebing, M., Finsen, B., et al. (2015). Spatio-temporal regulation of circular RNA expression during porcine embryonic brain development. Genome Biol. 16, 245. doi:10.1186/s13059-015-0801-3
Von Bartheld, C. S., and Altick, A. L. (2011). Multivesicular bodies in neurons: Distribution, protein content, and trafficking functions. Prog. Neurobiol. 93, 313–340. doi:10.1016/j.pneurobio.2011.01.003
Xu, X., Lai, Y., and Hua, Z. C. (2019). Apoptosis and apoptotic body: Disease message and therapeutic target potentials. Biosci. Rep. 39, BSR20180992. doi:10.1042/BSR20180992
Yao, G., Niu, W., Zhu, X., He, M., Kong, L., Chen, S., et al. (2019). hsa_circRNA_104597: a novel potential diagnostic and therapeutic biomarker for schizophrenia. Biomark. Med. 13, 331–340. doi:10.2217/bmm-2018-0447
Yuana, Y., Sturk, A., and Nieuwland, R. (2013). Extracellular vesicles in physiological and pathological conditions. Blood Rev. 27, 31–39. doi:10.1016/j.blre.2012.12.002
Zhang, G., and Yang, P. (2018). A novel cell-cell communication mechanism in the nervous system: Exosomes. J. Neurosci. Res. 96, 45–52. doi:10.1002/jnr.24113
Zhao, Y., and Yang, G. (2021). Potential of extracellular vesicles in the Parkinson's disease - pathological mediators and biomarkers. Neurochem. Int. 144, 104974. doi:10.1016/j.neuint.2021.104974
Zhuo, C. J., Hou, W. H., Jiang, D. G., Tian, H. J., Wang, L. N., Jia, F., et al. (2020). Circular RNAs in early brain development and their influence and clinical significance in neuropsychiatric disorders. Neural Regen. Res. 15, 817–823. doi:10.4103/1673-5374.268969
Keywords: schizophrenia, biomarkers, circular RNAs, extracellular vesicles, research progress
Citation: Guo C, Lv H, Bai Y, Guo M, Li P, Tong S and He K (2023) Circular RNAs in extracellular vesicles: Promising candidate biomarkers for schizophrenia. Front. Genet. 13:997322. doi: 10.3389/fgene.2022.997322
Received: 18 July 2022; Accepted: 05 December 2022;
Published: 06 January 2023.
Edited by:
Jinsong Tang, Zhejiang University, ChinaReviewed by:
Mengping Wei, Capital Medical University, ChinaTakanobu Tagawa, National Cancer Institute (NIH), United States
Copyright © 2023 Guo, Lv, Bai, Guo, Li, Tong and He. This is an open-access article distributed under the terms of the Creative Commons Attribution License (CC BY). The use, distribution or reproduction in other forums is permitted, provided the original author(s) and the copyright owner(s) are credited and that the original publication in this journal is cited, in accordance with accepted academic practice. No use, distribution or reproduction is permitted which does not comply with these terms.
*Correspondence: Kuanjun He, aGVrdWFuanVuNjY2QDEyNi5jb20=
†These authors have contributed equally to this work