- 1Division of Basic and Translational Research, Department of Obstetrics and Gynecology, The University of Texas Medical Branch at Galveston, Galveston, TX, United States
- 2Department of Biochemistry and Molecular Biology, College of Medicine, University of the Philippines Manila, Manila, Philippines
Approximately 40% of cases of spontaneous preterm birth (sPTB) are associated with ascending intrauterine infections. The cervix serves as a physical and immunological gatekeeper, preventing the ascent of microorganisms from the vagina to the amniotic cavity. The cervix undergoes remodeling during pregnancy. It remains firm and closed from the start until the late third trimester of pregnancy and then dilates and effaces to accommodate the passage of the fetus during delivery. Remodeling proceeds appropriately and timely to maintain the pregnancy until term delivery. However, risk factors, such as acute and chronic infection and local inflammation in the cervix, may compromise cervical integrity and result in premature remodeling, predisposing to sPTB. Previous clinical studies have established bacterial (i.e., chlamydia, gonorrhea, mycoplasma, etc.) and viral infections (i.e., herpesviruses and human papillomaviruses) as risk factors of PTB. However, the exact mechanism leading to PTB is still unknown. This review focuses on: (1) the epidemiology of cervical infections in pregnant patients; (2) cellular mechanisms that may explain the association of cervical infections to premature cervical ripening and PTB; (3) endogenous defense mechanisms of the cervix that protect the uterine cavity from infection and inflammation; and (4) potential inflammatory biomarkers associated with cervical infection that can serve as prognostic markers for premature cervical ripening and PTB. This review will provide mechanistic insights on cervical functions to assist in managing cervical infections during pregnancy.
Introduction
Preterm birth (PTB) is defined as live birth before the completion of 37 weeks of pregnancy (1). It remains a global public health concern, affecting almost 15 million babies annually (2). It is also the leading cause of death in children younger than 5 years of age. Preterm neonates have a greater risk of developing neonatal morbidities, such as respiratory distress syndrome, bronchopulmonary dysplasia, necrotizing enterocolitis, sepsis, and infections (3). Studies have shown that prematurity may also cause a greater risk of developing comorbidities in adulthood, such as cardiometabolic, respiratory, and neuropsychiatric disorders (3–5).
PTB is now recognized as a syndrome associated with multiple pathologic mechanisms, including infection, vascular disorders, uterine overdistension, breakdown in maternal-fetal tolerance, and cervical disease (6). PTB is also associated with socioeconomic, lifestyle, and environmental factors (7–9). Previous studies have shown the association of advanced maternal age, smoking, drinking alcohol, illegal drug use, domestic violence, physical abuse, and exposure to environmental pollutants (7–10). PTB can be medically indicated in women who undergo preterm delivery due to pathologic conditions, such as preeclampsia or intrauterine growth restriction, or spontaneous when the cause is unclear (11, 12). PTB can also be due to preterm premature rupture of membranes (PPROM). However, most PTBs are due to spontaneous PTB with intact membranes (13, 14). It is estimated that 25–40% of PTBs are caused by intrauterine infections (14, 15). Most intrauterine infections during pregnancy are caused by bacteria ascending from the vagina and the cervix (16, 17). How the cervix is compromised to cause the ascent of an infectious agent is still not fully understood.
The cervix plays an essential role in protecting the developing fetus in the intra-amniotic cavity and helps to maintain the pregnancy until term delivery. The cervix remains firm and closed throughout pregnancy and undergoes cervical ripening and dilation during labor and delivery (18, 19). This process is called cervical remodeling and is divided into four distinct but overlapping phases: softening, ripening, dilation, and postpartum cervical repair (20). Cervical remodeling is associated with increased vascular permeability, production of inflammatory cytokines and collagen-degrading enzymes, leukocyte infiltration, and protease activation, which will further degrade the collagen in the cervix (21–26). This will weaken the cervix resulting in the disappearance of its morphological features to allow effacement of the uterus, which will facilitate labor and childbirth.
The cervix also serves as a barrier preventing the ascent of microorganisms from the vaginal canal to the uterine cavity. The mucus produced by cervical epithelia serves as a barrier to prevent infection (27–29). It also protects the cells against chemical and mechanical insults during pregnancy and parturition (30). Cytokines and chemokines secreted by cervical cells recruit and stimulate inflammatory cells and antimicrobial factors to kill invading pathogens (21, 31, 32). These functions of the cervix are partly regulated by the junction proteins that seal off the intercellular space and ensure apical and basolateral polarity. In mouse studies, tight junction proteins, such as claudin 1 and 2 and desmoglein, exhibit temporal changes during pregnancy. These proteins are upregulated during cervical ripening to ensure barrier formation in the cervix, which will prevent infection and other insults in the cervix and the reproductive tract (18, 33).
A compromise in the integrity of the cervical epithelial cell barrier makes the underlying cervical cells more susceptible to infection, which can promote inflammation and predispose women to preterm labor (PTL) (34). Decrease or degradation of these tight junctions might contribute to the pathogenesis of infection mediated PTB (33, 35). The other factors that can alter the integrity of the cervical epithelial barrier and induce premature cervical remodeling include microRNAs, the biomechanical properties of the cervix, and the cervicovaginal metabolome and microbiome (33, 34, 36–38). Conditions that compromise the integrity of the cervix, such as cervical insufficiency, short cervix, cervical trauma, and damage to the cervix from previous surgical treatments for cervical dysplasia, also contribute to PTL and PTB (39–44).
To summarize the evidence on the role of infection and inflammation in the cervix in the pathogenesis of PTB, we conducted this review, which focused on: (1) the epidemiology of cervical infections in pregnant patients; (2) cellular mechanisms that may explain the association of cervical infections to premature cervical ripening and PTB; (3) endogenous defense mechanisms of the cervix to protect the uterine cavity from infection and inflammation; and (4) potential inflammatory biomarkers associated with cervical infection that can serve as prognostic markers for premature cervical ripening and PTB. This review will provide mechanistic insights on cervical functions to assist in the management of cervical infections during pregnancy.
Cervical Infections Promote Preterm Birth
Infection and inflammation in the cervix appear to play a role in pregnancy and parturition. Cervical inflammation, with or without sexually transmitted disease, was significantly associated with PTB (45). We have summarized the current literature on bacterial and viral infections in the cervix during pregnancy and their association with maternal and fetal outcomes (Figure 1).
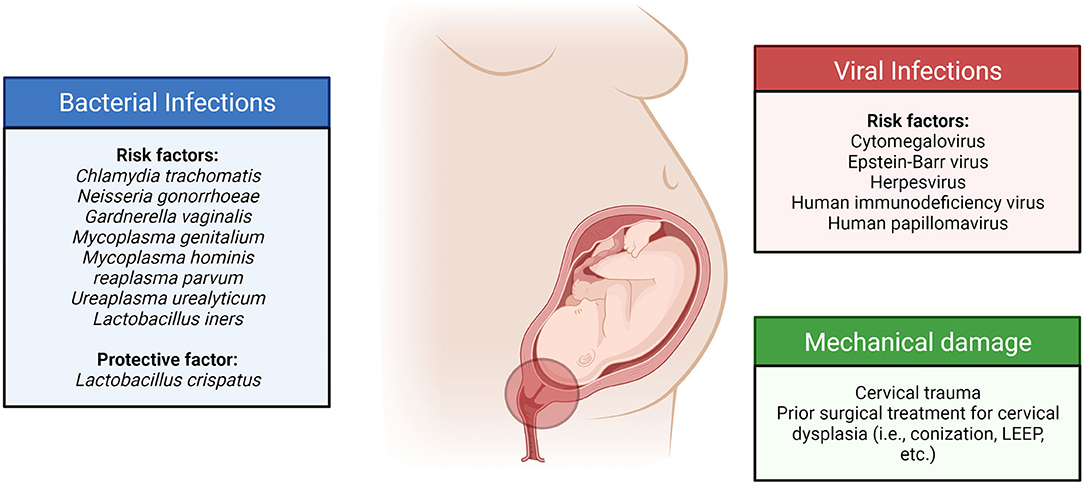
Figure 1. Cervical infections and their sequelae are associated with an increased risk of adverse pregnancy outcomes.
Bacterial Infections
Chronic cervical infections with bacteria were associated with high rates of recurrent threatened pregnancy loss, PPROM, and PTB. Ureaplasma urealyticum was the most isolated microorganism in the cervix of patients who experienced preterm delivery, with a prevalence of 34.5%, followed by Enterococcus spp. (27.6%) and Mycoplasma hominis (17.2%). On the other hand, U. parvum (85.48%) was the most prevalent pathogen detectable by PCR in the cervix, followed by Chlamydia trachomatis (8.0%) (46). Another study also showed that endocervical infection with Ureaplasma spp. was significantly associated with PTB (47).
The prevalence of C. trachomatis cervicitis in women with PTL was significantly higher in patients with PTBs than in women with term deliveries (48). Another study showed that cervicitis among pregnant women was commonly caused by C. trachomatis. Antimicrobial treatment reduced the incidence of preterm delivery in these patients, confirming an infectious etiology (49). In a case-control study in Iran involving 75 women with PTBs and 75 women with term deliveries, no significant association was found between C. trachomatis infection of the cervix and PTB (50). Patients with cervical C. trachomatis infection treated with antibiotics had lower rates of pPROM, PTB, and small-for-gestational-age infants compared to those who did not receive treatment (51, 52).
Among pregnant patients with PPROM, the presence of the abundant cervical microorganism Gardnerella vaginalis was related to microbial invasion of the amniotic cavity (MIAC) (with: 65% vs. without: 44%) (53). A study by Bartkeviciene et al. showed that Ureaplasma infections were associated with PPROM, placental inflammation, and newborn respiratory distress syndrome (54). A case-control study involving 94 pregnant Korean women used machine learning tools to predict PTB based on the bacterial risk score of cervicovaginal fluid (CVF). The PTB risk was low when the Lactobacillus iners ratio was high. A moderate and high risk was classified when the L. iners ratio was low, and the U. parvum ratio was high (55).
There were also observed temporal differences in the risk of PTB from cervicovaginal infections. A previous study showed that cervicovaginal infection in the second trimester, when the cervix is neither open nor short, did not significantly raise the rate of PTB. On the other hand, a third-trimester infection significantly increased the risk of PTB (RR: 2.1, p = 0.05). When this infection was coupled with premature cervical ripening, the risk of PTB was even more increased (RR: 14.0, p = 0.001) (56).
Genital infections during pregnancy are usually polymicrobial. Aside from infections with a single bacterial species, these microorganisms' type, composition, and diversity can affect the cervix and pregnancy outcomes. Abnormal vaginal flora, including M. hominis, was associated with cervical shortening. This compromises the cervix and exposes the intrauterine cavity to the pathogens in the cervicovaginal area, increasing PTB risk (57). In a previous microbiome study involving pregnant women of predominantly Caucasian ethnicity, CST III (L. iners-dominated) was also associated with extreme cervical shortening (OR: 6.4, 95% CI: 1.32–31.03) (58). Pregnant women with a cervicovaginal community characterized by increased facultative anaerobes and decreased Lactobacillus species (CST IV) are associated with short cervix and PTB (59). However, the presence of L. crispatus-dominated cervical microbiota in women with PPROM was associated with a lower risk of intra-amniotic complications and early-onset sepsis of the newborn (60). This lowered risk of complication from PPROM can be attributed to the antimicrobial activity of L. crispatus and other probiotics against bacterial vaginosis and urinary tract infection pathogens (61, 62).
In summary, these data showed the association of cervical infection with increased risk PTB. Moreover, antibiotic treatment of cervical infections resulted in better pregnancy outcomes. Some studies showed an association between single-species cervical infection, such as C. trachomatis, Enterococcus spp., G. vaginalis, M. hominis, U. parvum, and U. realyticum, and PTB. However, other studies also showed the importance of polymicrobial infection, bacterial composition, and diversity in increasing the risk of PTB. Cervical microbiota dominated by facultative anaerobes and with decreased Lactobacillus species were more predisposed to PTB.
Viral Infections
Viral infections in the cervix are also documented to impact pregnancy outcomes. Systemic infections, such as HIV, were also associated with cervical inflammation and prematurity. In a previous study involving pregnant women living with HIV-1 infection (PWLWH), 12% of them delivered preterm. PWLWH had a higher prevalence of L. iners dominant and mixed anaerobes groups, which were associated with increased pro-inflammatory cytokine concentrations (such as IL-1b) in the CVF (63). Epstein-Barr virus (17.2%) was the most prevalent pathogen detectable by PCR in the cervix of patients with PTB, followed by human herpesvirus 6 (8.0%) (46). In a study involving Kenyan women, cervical cytomegalovirus (CMV) infection was associated with higher cervical IL-6 and TNF-a. However, this did not significantly increase PTBs before 34 weeks of gestation (64). Genital herpes infection can cause blistering and ulceration of the cervix. This can disrupt the cervical epithelial barrier and promote ascending bacterial infections during pregnancy (65). A study involving 662,913 mother-newborn pairs showed that genital herpes during the first or second trimester was associated with more than double the risk of PTB (66).
Human papillomavirus (HPV) infection was also prevalent among pregnant patients. The estimated HPV prevalence in pregnant women varied from 9.58 to 46.67%, with a summary estimate of 16.82% (95% CI: 16.21–17.47) (67). The prevalence varied based on study region, age, and HPV type. Previous reports have shown that HPV infections are associated with an increased risk of adverse pregnancy outcomes (68–71). HPV infection is associated with a 1.5-times increased odds of PTB, 1.96-times increased odds of PPROM, 1.91-times increased odds of low birth weight, and 2.23-times increased odds of fetal death (70). A retrospective study involving 2,153 pregnant women showed an HPV positivity rate of 38.5%. HPV infection (OR: 2.07, 95% CI: 1.03–4.14) was associated with PPROM. HPV infection was also associated with newborn septicemia, respiratory distress syndrome, neonatal intensive care unit admission, and low birth weight (68). A retrospective population-based study showed that HPV infections before or during pregnancy were associated with PTB [adjusted odds ratio (aOR): 1.19, 95% CI: 1.01–1.42], PPROM (aOR: 1.52, 95% CI: 1.18–1.96), premature rupture of membranes (aOR: 1.24, 95% CI: 1.08–1.4), and neonatal mortality (aOR: 2.69, 95% CI: 1.25–5.78) (71).
Lack of intervention during chronic HPV infections can result in cervical intraepithelial neoplasia (CIN) and cervical cancer. Based on a previous study from the Scottish HPV archive, HPV-associated high-grade cervical diseases (CIN2 and CIN3) but not high-risk HPV infection or low-grade cervical disease (CIN1) were associated with PTB (OR: 1.843, 95% CI: 1.101–3.083) (72). Cervical surgeries that are usually done to treat chronic infections in the cervix were shown to be associated with adverse pregnancy outcomes. Maternal history of cervical conization and/or loop electrical excision procedure (LEEP) increases the risk of preterm delivery, irrespective of concurrent maternal HPV positivity (73). Another study showed that previous treatment for CIN from chronic HPV infection was associated with even more significant risks for PTB and PPROM and was also associated with PROM, neonatal mortality, and maternal and neonatal infectious complications (71).
A meta-analysis showed an overall higher risk of PTB associated with excisional procedures (RR: 1.87, 95% CI: 1.64–2.12) than ablative procedures (RR: 1.35, 95% CI: 1.20–1.52) in the cervix. The risk of PTB was higher for more radical excisional techniques, such as cold knife conization (RR 2.70, 95% CI 2.14–3.40), laser conization (RR: 2.11, 95% CI: 1.26–3.54), and large loop excision of the transformation zone (RR: 1.58, 95% CI: 1.37–1.81) (74). In summary, these results show that viral infections in the cervix, complications resulting from such conditions, and associated surgical procedures to treat them increased PTB risk.
Infection, Inflammation, and Cervical Remodeling
Cervical infection and inflammation may damage the cervix and hasten the cervical remodeling process during pregnancy. This aberrant timing of cervical ripening can increase the risk of PTB. Previous clinical, animal, and in vitro studies suggested several potential mechanisms on how infections can compromise the integrity of the cervix (Figure 2). Cervical canal infection with Ureaplasma, Mycoplasma, and Escherichia coli in pregnant women with singleton pregnancies was associated with cervical changes, which could be easily detected by the Bishop score (a pre-labor scoring system based on a digital cervical exam of the patient, which utilizes cervical dilation, position, effacement, consistency of the cervix, and fetal station to assist in predicting whether induction of labor will be required) and ultrasound assessment of cervical length (75, 76). However, another study showed no significant association between vaginitis, cervicitis, and cervical length (77).
Limited animal studies showed the potential mechanisms of how bacterial and viral infections can promote cervical ripening in the cervix. Sierra et al. reported that colonization of the cervicovaginal space with Gardnerella vaginalis significantly increased IL-6 levels in the cervicovaginal space. It also increased the gene expression of IL-1β, IL-8, and IL-10 in the cervix. Soluble E-cadherin, a molecular marker of cervical epithelial barrier disruption, and Tff-1, a molecule involved in cervical remodeling, were also increased with G. vaginalis infection. It also altered the cervical biomechanics, as shown by the significant decrease in tissue modulus of cervices colonized by G. vaginalis compared to uninfected cervices (78). This indicates that colonization hastens the cervical softening process.
McGee et al. reported that cervical HSV-2 infection promoted cervical remodeling in a mouse model of pregnancy. HSV-2 induced collagen remodeling and increased hyaluronic acid synthesis. HSV-2 also altered the responsiveness of the cervix to pregnancy hormones by changing the expression of estrogen and progesterone receptors in the cervical epithelium, which promoted premature cervical ripening and increased the risk of PTB (79). Cervical viral infections can also make the cervix more susceptible to bacterial infections that are more pathogenic and can cause PTB. Murine gammaherpesvirus 68 infections resulted in a lower expression of antimicrobial peptides (AMPs) and lower levels of pro-inflammatory cytokines in the cervix compared to pregnant mice not exposed to the virus (80).
Our previous in vitro studies have shown that infection and inflammation can cause cell-specific changes that contribute to cervical damage and cervical remodeling during pregnancy. These include inflammation, epithelial to mesenchymal transition (EMT), cell death (apoptosis, necrosis, and autophagy), and senescence. LPS and TNF-a increased the levels of inflammatory cytokines (IL-6 and IL-8) and MMP-9 in ectocervical and endocervical epithelial cells in in vitro monoculture and co-cultured in a microfluidic organ-on-a-chip of the cervical epithelial layer (34, 81). This increase in collagenolytic enzymes and pro-inflammatory cytokines can disrupt the cervical epithelial barrier and promote cervical ripening (20, 82, 83). Apoptosis and necrosis in the resident cervical cell types may contribute to cervical tissue remodeling during pregnancy. Human cervical stromal biopsies from pregnant women in active labor have shown higher apoptotic nuclei than those from cervices not in labor (84). Infection and inflammation also increased apoptosis and senescence in cervical epithelial cells (81). Similarly, sterile inflammation from oxidative stress also promoted an increased inflammatory response, apoptosis, senescence, and autophagy in cervical epithelial and stromal cells (85).
We have also shown that infection and inflammation can promote EMT in cervical epithelial cells (81, 86, 87). EMT was shown to be involved in the cervical changes that happen during parturition. Human and animal studies showed an increase in the expression of mesenchymal markers, such as vimentin and N-cadherin, and a decrease in epithelial markers, such as cytokeratin and E-cadherin, in the cervix during parturition (88, 89). EMT is often associated with an inflammatory milieu, primarily to remodel the affected area; however, a non-reversible state of EMT induced in response to infection or other risk exposures may cause persistent inflammation and cervical matrix degradation.
Aside from infection and inflammation, chemical and mechanical damage to the cervix can also promote inflammation resulting in premature cervical ripening. A previous animal study showed that pre-gestational cervical excision plus inflammation-induced PTL significantly increased the expression levels of collagen type IV alpha 1, collagen type V alpha 1, and MMP-14. This increase may promote premature cervical remodeling and PTB (90). A clinical study also showed that vaginal or cervical infections only demonstrated an increased risk for PTB in patients with a short cervix before 28 weeks or dilated cervix before 37 weeks of pregnancy (56). A recent study showed that damage to the cervical epithelium induced by a common spermicide, nonoxynol-9, promoted ascending infections with U. parvum, upregulated the production of pro-inflammatory cytokines, and increased PTB rates in mice (30). Altogether, these data show that an intact cervix can effectively prevent ascending infections in pregnancy. Unless the cervix is compromised by previous surgical procedures, chemical exposure, or overt infection, it can continue its role in protecting the fetus until term delivery.
Endogenous Defense Mechanisms of the Cervix Against Infections During Pregnancy
The cervix has endogenous defense mechanisms to fight off infections during pregnancy. These serve as the first line of defense and help maintain the cervix's barrier function during pregnancy. The cervical epithelial layers form a barrier through its junction proteins that seal off intercellular spaces and block the bacterial invasion of the cervix (18, 33). The cervix also produces AMPs that can kill bacteria. In a previous study in the United Kingdom, cervical fluid elafin or human β-defensin-1 protein levels were not associated with PTB (91). Another study showed that low β-defensin-2 protein levels (aOR: 1.40, 95% CI: 0.83–2.34) had slightly elevated but not significantly increased odds of sPTB (92). Another study showed that cervical gene delivery of HBD3 may be a potential candidate to boost innate immunity in the cervix and prevent E. coli ascending infection related PTB and its associated neonatal consequences (93).
As mentioned above, the cervical mucus plug (CMP) also plays a vital role in deterring bacteria from the vaginal canal trying to get past the cervix to invade the uterine cavity. The CMP contains large amounts of AMPs, such as secretory leukoprotease inhibitor (SLPI), lysozyme, lactoferrin, calprotectin, α-defensin human neutrophil peptides (HNPs) 1–3 and human β-defensin (27). Previous studies have shown that cervical mucus has good antibacterial properties. It can completely inhibit pathogenic bacteria such as Staphylococcus saprophyticus, E. coli, Pseudomonas aeruginosa, Enterococcus faecium, Streptococcus agalactiae, S. pyogenes, and S. aureus (94–96).
The CMP also performs essential innate and adaptive immune functions. CMP proteins can also activate the innate immune response of the cervix to kill bacteria trying to invade the cervix (97). Hence, a compromise in the barrier properties of the cervical mucus can predispose pregnant patients to PTB. Critchfield et al. reported that women at high risk for PTB have mucus that is more extensible and permeable and forms weaker gels than cervical mucus from women at low risk for PTB (28).
The cervix increases pro-inflammatory cytokine production to help fight off infection. Pregnant women with bacterial vaginosis or cervicitis have higher levels of IL-1β, IL-6, and IL-8 in their cervical mucus compared to patients with normal pregnancies (98–100). Bacterial and viral infections can also elicit a type 1 T-helper lymphocyte response in the cervix. This regulates cytotoxic T lymphocytes, which are in part responsible for clearing infections in the genital tract (101). Massive inflammation in the cervix may be detrimental since this can damage the cervical epithelial barrier (34, 37). However, the cervix also modulates the inflammatory response to an infection via secreting anti-inflammatory cytokines. Dubicke et al. showed that the anti-inflammatory cytokine IL-4 was significantly higher in the preterm subgroup with bacterial infection than in the non-infected group (21).
The cervix also harbors immune cells that can destroy pathogenic bacteria and viruses. Some of the primary immune cells in the cervix include macrophages, dendritic cells (DCs), and other lymphocyte lineage-negative cells (CD3, CD20, CD56, and CD16) (102). These immune cells are activated by bacteria and viruses that infect the cervix. Prakash et al. reported that women with cervicitis had increased recruitment of macrophages in their cervical epithelium (103). Endocervical secretions of patients with chlamydial cervicitis had higher CD4+, CD8+, and DC phenotypes (104). This shows that the cervix mounts a cell-mediated immune response to C. trachomatis. Persistent HSV-2 infection results in the formation of lymphoid clusters of CD4+ and CD8+ T-cells, B-cells, DCs, and macrophages in the cervix (105). These lymphoid cells persist for years, even after viral clearance, and confer lasting protection against reinfection (106). In summary, these physical, chemical, and immunological defense mechanisms in the cervix may help prevent the pathologic effects of bacterial and viral infections in the cervix. These mechanisms enable the cervix to perform its barrier function to protect the developing fetus in the uterine cavity.
Potential Cervical Biomarkers of Adverse Pregnancy Outcomes
The type of cervical infection and the specific cellular and immunologic responses of the cervix against infections can be used as biomarkers for diagnosing infections and prognosticating pregnancy outcomes (98, 100). Previous studies have shown some potential biomarkers associated with cervical infections that may be useful in predicting adverse pregnancy outcomes. In pregnancies with PTL, both intra-amniotic infection and sterile inflammation were associated with elevated concentrations of IL-6 in the cervical fluid (intra-amniotic infection: median 587 pg/mL; sterile intra-amniotic inflammation, median: 590 pg/mL; without inflammation, median: 136 pg/mL) (107). IL-6 and IL-8 in cervical secretions can also predict PTL caused by intra-amniotic infection or chorioamnionitis (108). Kim et al. showed that IL-8 and MCP-1 in the CVF were significantly increased in patients with intra-amniotic infections and/or inflammation (55).
Macrophage inflammatory protein (MIP)-1β levels in CVF were significantly higher in patients with imminent sPTB than those with term deliveries (55, 109). However, another study showed that the concentration of MIP-1α in the cervical fluid was not elevated in patients with PTL who had intra-amniotic infections. However, elevated MIP-1α levels in amniotic fluid may be valuable markers for intra-amniotic infection in women with PTL (107). The utility of amniotic fluid markers is limited, as many perinatal clinical settings around the globe do not perform amniocentesis for diagnostic purposes.
In women with PTL, elevated CVF levels of C5a (a complement-activated product) and IGFBP-1 (a transport protein for insulin-like growth factor 1) were significantly associated with IAI and PTB at <34 weeks of gestation, while those of C3a were associated with IAI but not PTB. Compared to amniotic fluid white blood cells, these biomarkers have similar or better diagnostic performance (110). In a study involving 35 women with PTB in the United Kingdom, IL-8 and IL-1β in the cervical fluid were lower in women who delivered preterm than those who had term delivery (91).
In a retrospective study of 99 women with PPROM, the use of protein-antibody microarray technology identified biomarkers in the CVF that can identify MIAC in women with PPROM. These biomarkers include IL-8, lipocalin-2, MIP-1α, MMP-9, and TIMP-1 (111). The protein concentrations of IgGFc-binding protein (FcγBP), which is a core mucus protein with anti-inflammatory properties, in the cervical fluid were also elevated in the presence of intra-amniotic infection in patients with PPROM (presence: 345 ng/mL vs. absence: 60 ng/mL) (112). This increase in FcγBP may help prevent massive inflammation from intra-amniotic infections.
AMPs and metabolites in the CVF may also serve as biomarkers for PTL and PTB. The cervicovaginal level of trappin-2, an AMP and a serine protease inhibitor, was found to be elevated in women who delivered preterm (113). A metabolomic study showed that high acetate levels in the CVF of women with PTL were predictive of preterm delivery and delivery within 2 weeks of presentation with PTL (109). Moreover, the predictive accuracy of acetate levels in CVF for PTB in symptomatic pregnant women was comparable to cervical length and fetal fibronectin (114). These data showed the potential use of cervical samples in developing biomarkers for stratifying pregnant patients at greater risk of PTB.
Clinical Implications
Cervical infections remain underdiagnosed among the pregnant population because patients are generally asymptomatic. This results in a chronic subclinical infection extending to the upper genital tract and a greater risk of adverse pregnancy outcomes. Currently, screening tests for cervical infections are not routinely done in most hospitals. The gold standard for diagnosing cervical infections is the nucleic acid amplification test. However, this is not available in most hospitals, especially in developing countries. This results in many pregnant women not being diagnosed with cervical infections. A previous study involving 1,876 pregnant women in Japan noted that 84% of pregnant women with gonococcal cervicitis may have been missed in hospitals that do not routinely perform the screening test for gonococcal cervicitis (115). These pregnant women who are not diagnosed with cervical infection are also not given the proper management that can help in preventing ascending infections during pregnancy.
Aside from the challenges in diagnosis, there are still a lot of uncertainties in managing cervical infections during pregnancy. The CDC has recommendations for managing common cervical infections during pregnancy, such as chlamydia, gonococcal, and M. genitalium infection (116). However, more studies are needed to manage non-chlamydial and non-gonococcal cervicitis, because these were shown to have an association with adverse pregnancy outcomes. The limited available data showed that antibiotics directed toward C. trachomatis and Neisseria gonorrhoeae are ineffective against non-chlamydial and non-gonococcal cervicitis (117).
Several cervical infections can easily be prevented by patient education and vaccination. The acquisition of many STIs is based on individual sexual behavior. Educating patients on safe sex practices can help prevent cervical infections (118). Vaccines are already available for HPV infection. Increasing the knowledge and awareness of patients regarding the HPV vaccination and improving its coverage in women may help prevent HPV infections during pregnancy.
Conclusion
This review summarized the existing evidence on the association of cervical infection and inflammation and PTB. Multitudes of studies have reported several biomarkers in response to infection that are pro-inflammatory that can cause derangement in the cervical environment. However, the cervix may withstand exogenous insults due to multiple barriers (mechanical barriers: tight junctions that seal the cervical epithelial layer and the CMP that blocks invading pathogens from passing through to the uterine cavity; chemical barriers: production of AMPs and anti-inflammatory cytokines that can fight off infection and temporize inflammation; and immunologic barriers: innate and adaptive immune responses in the cervix) that protect the cervix and balance the impact of infection and inflammation.
However, overt infections with a high load of pathogenic bacteria and viruses may overcome these barriers, compromise the barrier function of the cervix, and eventually increase the risk of PTB. Infection disrupts the integrity of the cervical epithelial barriers by promoting cell death and senescence, EMT, and decreasing AMP production. When the cervical epithelial cell layer is damaged, microorganisms can access and infect the stromal layer and cause collagen remodeling and degradation. Infections have been shown to promote collagen degradation by increasing the levels of collagenolytic enzymes and decreasing tissue inhibitors of matrix metalloproteinases. Cervical infection can also promote an inflammatory response, immune cell activation, and alteration of estrogen and progesterone receptors. These events will weaken the cervix and allow microorganisms to invade the uterine cavity, infect the developing fetus, and cause PTB.
Author Contributions
OT and RM conceived the review, contributed to analysis and interpretation of available literature, and prepared the manuscript. Both authors contributed to the article and approved the submitted version.
Funding
OT is an MD-PhD trainee in the MD-PhD in Molecular Medicine Program, supported by the Philippine Council for Health Research and Development, Department of Science and Technology, Republic of the Philippines, and administered through the University of the Philippines Manila. This study was supported by 5R01HD100729-03 (NIH/NICHD) to RM.
Conflict of Interest
The authors declare that the research was conducted in the absence of any commercial or financial relationships that could be construed as a potential conflict of interest.
Publisher's Note
All claims expressed in this article are solely those of the authors and do not necessarily represent those of their affiliated organizations, or those of the publisher, the editors and the reviewers. Any product that may be evaluated in this article, or claim that may be made by its manufacturer, is not guaranteed or endorsed by the publisher.
References
1. March March of Dimes PMNCH Save the Children W. Born too soon: the global action report on preterm birth. In: Howson CP, Kinney MV, Lawn JE, editors. World Health Organization. Vol. 25. Geneva: World Health Organization (2004).
2. Chawanpaiboon S, Vogel JP, Moller AB, Lumbiganon P, Petzold M, Hogan D, et al. Global, regional, and national estimates of levels of preterm birth in 2014: a systematic review and modelling analysis. Lancet Glob Health. (2019) 7:e37–46. doi: 10.1016/S2214-109X(18)30451-0
3. Crump C. Preterm birth and mortality in adulthood: a systematic review. J Perinatol. (2020) 40:833–43. doi: 10.1038/s41372-019-0563-y
4. Crump C, Howell EA, Stroustrup A, McLaughlin MA, Sundquist J, Sundquist K. Association of preterm birth with risk of ischemic heart disease in adulthood. JAMA Pediatr. (2019) 173:736–43. doi: 10.1001/jamapediatrics.2019.1327
5. Luu TM, Katz SL, Leeson P, Thébaud B, Nuyt A-M. Preterm birth: risk factor for early-onset chronic diseases. Can Med Assoc J. (2016) 188:736–46. doi: 10.1503/cmaj.150450
6. Romero R, Dey SK, Fisher SJ. Preterm labor: one syndrome, many causes. Science. (2014) 345:760–5. doi: 10.1126/science.1251816
7. Ciancimino L, Laganà AS, Chiofalo B, Granese R, Grasso R, Triolo O. Would it be too late? A retrospective case-control analysis to evaluate maternal-fetal outcomes in advanced maternal age. Arch Gynecol Obstetr. (2014) 290:1109–14. doi: 10.1007/s00404-014-3367-5
8. Ferguson KK, Chin HB. Environmental chemicals and preterm birth: biological mechanisms and the state of the science. Curr Epidemiol Rep. (2017) 4:56–71. doi: 10.1007/s40471-017-0099-7
9. Kumar S, Sharma S, Thaker R. Occupational, environmental, and lifestyle factors and their contribution to preterm birth - an overview. Indian J Occup Environ Med. (2017) 21:9–17. doi: 10.4103/ijoem.IJOEM_155_16
10. Donovan BM, Spracklen CN, Schweizer ML, Ryckman KK, Saftlas AF. Intimate partner violence during pregnancy and the risk for adverse infant outcomes: a systematic review and meta-analysis. BJOG Int J Obstet Gynaecol. (2016) 123:1289–99. doi: 10.1111/1471-0528.13928
11. Menon R. Spontaneous preterm birth, a clinical dilemma: etiologic, pathophysiologic and genetic heterogeneities and racial disparity. Acta Obstet Gynecol Scand. (2008) 87:590–600. doi: 10.1080/00016340802005126
12. Tucker JM, Goldenberg RL, Davis RO, Copper RL, Winkler CL, Hauth JC. Etiologies of preterm birth in an indigent population: is prevention a logical expectation? Obstet Gynecol. (1991) 77:343–7.
13. di Renzo GC, Roura LC. Guidelines for the management of spontaneous preterm labor. J Perinat Med. (2006) 34:359–66. doi: 10.1515/JPM.2006.073
14. Goldenberg RL, Culhane JF, Iams JD, Romero R. Epidemiology and causes of preterm birth. Lancet. (2008) 371:75–84. doi: 10.1016/S0140-6736(08)60074-4
15. Lamont RF. Infection in the prediction and antibiotics in the prevention of spontaneous preterm labour and preterm birth. BJOG Int J Obstetr Gynaecol. (2003) 110(Suppl.):71–5. doi: 10.1046/j.1471-0528.2003.00034.x
16. Chan MY, Smith MA. Infections in pregnancy. Compreh Toxicol. (2018) 232–49. doi: 10.1016/B978-0-12-801238-3.64293-9
17. Goldenberg RL, Hauth JC, Andrews WW. Intrauterine infection and preterm delivery. N Engl J Med. (2000) 342:1500–7. doi: 10.1056/NEJM200005183422007
18. Read CP, Word RA, Ruscheinsky MA, Timmons BC, Mahendroo MS. Cervical remodeling during pregnancy and parturition: molecular characterization of the softening phase in mice. Reproduction. (2007) 134:327–40. doi: 10.1530/REP-07-0032
19. Word RA, Li XH, Hnat M, Carrick K. Dynamics of cervical remodeling during pregnancy and parturition: mechanisms and current concepts. Semin Reprod Med. (2007) 25:69–79. doi: 10.1055/s-2006-956777
20. Sennstrom MB. Human cervical ripening, an inflammatory process mediated by cytokines. Mol Hum Reprod. (2000) 6:375–81. doi: 10.1093/molehr/6.4.375
21. Dubicke A, Fransson E, Centini G, Andersson E, Byström B, Malmström A, et al. Pro-inflammatory and anti-inflammatory cytokines in human preterm and term cervical ripening. J Reprod Immunol. (2010) 84:176–85. doi: 10.1016/j.jri.2009.12.004
22. Dubicke A, Ekman-Ordeberg G, Mazurek P, Miller L, Yellon SM. Density of stromal cells and macrophages associated with collagen remodeling in the human cervix in preterm and term birth. Reprod Sci. (2016) 23:595–603. doi: 10.1177/1933719115616497
23. Iwahashi M, Muragaki Y, Ooshima A, Umesaki N. Decreased type I collagen expression in human uterine cervix during pregnancy. J Clin Endocrinol Metab. (2003) 88:2231–5. doi: 10.1210/jc.2002-021213
24. Myers DA. The recruitment and activation of leukocytes into the immune cervix: further support that cervical remodeling involves an immune and inflammatory mechanism. Biol Reprod. (2012) 87:1–2. doi: 10.1095/biolreprod.112.105049
25. Stygar D, Wang H, Vladic YS, Ekman G, Eriksson H, Sahlin L. Increased level of matrix metalloproteinases 2 and 9 in the ripening process of the human cervix. Biol Reprod. (2002) 67:889–94. doi: 10.1095/biolreprod.102.005116
26. Vink J, Mourad M. The pathophysiology of human premature cervical remodeling resulting in spontaneous preterm birth: where are we now? Semin Perinatol. (2017) 41:427–37. doi: 10.1053/j.semperi.2017.07.014
27. Becher N, Waldorf KA, Hein M, Uldbjerg N. The cervical mucus plug: structured review of the literature. Acta Obstet Gynecol Scand. (2009) 88:502–13. doi: 10.1080/00016340902852898
28. Critchfield AS, Yao G, Jaishankar A, Friedlander RS, Lieleg O, Doyle PS, et al. Cervical mucus properties stratify risk for preterm birth. PLoS ONE. (2013) 8:2–8. doi: 10.1371/journal.pone.0069528
29. Hansen LK, Becher N, Bastholm S, Glavind J, Ramsing M, Kim CJ, et al. The cervical mucus plug inhibits, but does not block, the passage of ascending bacteria from the vagina during pregnancy. Acta Obstet Gynecol Scand. (2014) 93:102–8. doi: 10.1111/aogs.12296
30. Pavlidis I, Spiller OB, Sammut Demarco G, MacPherson H, Howie SEM, Norman JE, et al. Cervical epithelial damage promotes Ureaplasma parvum ascending infection, intrauterine inflammation and preterm birth induction in mice. Nat Commun. (2020) 11:1–12. doi: 10.1038/s41467-019-14089-y
31. Frew L, Stock SJ. Antimicrobial peptides and pregnancy. Reproduction. (2011) 141:725–35. doi: 10.1530/REP-10-0537
32. Yellon SM. Immunobiology of cervix ripening. Front Immunol. (2020) 10:31645. doi: 10.3389/fimmu.2019.03156
33. Timmons B, Akins M, Mahendroo M. Cervical remodeling during pregnancy and parturition. Trends Endocrinol Metab. (2010) 21:353–61. doi: 10.1016/j.tem.2010.01.011
34. Nold C, Anton L, Brown A, Elovitz M. Inflammation promotes a cytokine response and disrupts the cervical epithelial barrier: a possible mechanism of premature cervical remodeling and preterm birth. Am J Obstetr Gynecol. (2012) 206:208.e1–7. doi: 10.1016/j.ajog.2011.12.036
35. Holt R, Timmons BC, Akgul Y, Akins ML, Mahendroo M. The molecular mechanisms of cervical ripening differ between term and preterm birth. Endocrinology. (2011) 152:1036–46. doi: 10.1210/en.2010-1105
36. Anton L, DeVine A, Sierra L-J, Brown AG, Elovitz MA. miR-143 and miR-145 disrupt the cervical epithelial barrier through dysregulation of cell adhesion, apoptosis and proliferation. Sci Rep. (2017) 7:1–15. doi: 10.1038/s41598-017-03217-7
37. Anton L, Sierra LJ, de Vine A, Barila G, Heiser L, Brown AG, et al. Common cervicovaginal microbial supernatants alter cervical epithelial function: Mechanisms by which lactobacillus crispatus contributes to cervical health. Front Microbiol. (2018) 9:2181. doi: 10.3389/fmicb.2018.02181
38. Yoshida K, Jayyosi C, Lee N, Mahendroo M, Myers KM. Mechanics of cervical remodelling: insights from rodent models of pregnancy. Interface Focus. (2019) 9:20190026. doi: 10.1098/rsfs.2019.0026
39. Bevis KS, Biggio JR. Cervical conization and the risk of preterm delivery. Am J Obstet Gynecol. (2011) 205:19–27. doi: 10.1016/j.ajog.2011.01.003
40. Cho GJ, Ouh Y-T, Kim LY, Lee T-S, Park GU, Ahn KH, et al. Cerclage is associated with the increased risk of preterm birth in women who had cervical conization. BMC Pregn Childb. (2018) 18:277. doi: 10.1186/s12884-018-1765-6
41. Gomez R, Romero R, Nien JK, Chaiworapongsa T, Medina L, Kim M, et al. A short cervix in women with preterm labor and intact membranes : a risk factor for microbial invasion of the amniotic cavity. Am J Obstet Gynecol. (2005) 192:678–89. doi: 10.1016/j.ajog.2004.10.624
42. Kindinger LM, Kyrgiou M, MacIntyre DA, Cacciatore S, Yulia A, Cook J, et al. Preterm Birth Prevention Post-Conization: A Model of Cervical Length Screening with Targeted Cerclage. PLoS One. (2016) 11:e0163793–e0163793. doi: 10.1371/journal.pone.0163793
43. Slager J, Lynne S. Assessment of cervical length and the relationship between short cervix and preterm birth. J Midwif Womens Health. (2012) 57(Suppl. 1):S4–11. doi: 10.1111/j.1542-2011.2012.00209.x
44. Sundtoft I, Langhoff-Roos J, Sandager P, Sommer S, Uldbjerg N. Cervical collagen is reduced in non-pregnant women with a history of cervical insufficiency and a short cervix. Acta Obstet Gynecol Scand. (2017) 96:984–90. doi: 10.1111/aogs.13143
45. Ravindran J, Richardson BA, Kinuthia J, Unger JA, Drake AL, Osborn L, et al. Chlamydia, gonorrhea, and incident HIV infection during pregnancy predict preterm birth despite treatment. J Infect Dis. (2021) 224:2085–93. doi: 10.1093/infdis/jiab277
46. Barinov SV, Tirskaya YI, Kadsyna TV, Lazareva OV, Medyannikova IV, Tshulovski YI. Pregnancy and delivery in women with a high risk of infection in pregnancy. J MaternFetal Neonat Med. (2020). doi: 10.1080/14767058.2020.1781810. [Epub ahead of print].
47. González Bosquet E, Gené A, Ferrer I, Borrás M, Lailla JM. Value of endocervical Ureaplasma species colonization as a marker of preterm delivery. Gynecol Obstet Invest. (2006) 61:119–23. doi: 10.1159/000089457
48. Mohamed SS, Rabei NH, Faisal MM, Amin AS. Association between chlamydia trachomatis cervicitis and preterm labor. Egypt J Hosp Med. (2017) 68:1342–9. doi: 10.12816/0039672
49. Begum S, Sagawa T, Fujimoto S. Screening for bacterial vaginosis and cervicitis aimed at preventing premature delivery. J Obstetr Gynaecol Res. (1997) 23:103–10. doi: 10.1111/j.1447-0756.1997.tb00814.x
50. Ahmadi SJ, Farhadifar F, Sharami SRY, Zare S, Rezaei M, Soofizadeh N, et al. The role of Chlamydia trachomatis in preterm delivery: a case-control study in Besat Hospital, Sanandaj, Iran (2018-2019). Iran J Microbiol. (2020) 12:325–30. doi: 10.18502/ijm.v12i4.3936
51. Cohen I, Veille JC, Calkins BM. Improved pregnancy outcome following successful treatment of chlamydial infection. JAMA. (1990) 263:3160–3. doi: 10.1001/jama.1990.03440230056032
52. Ryan GMJ, Abdella TN, McNeeley SG, Baselski VS, Drummond DE. Chlamydia trachomatis infection in pregnancy and effect of treatment on outcome. Am J Obstet Gynecol. (1990) 162:34–9. doi: 10.1016/0002-9378(90)90815-O
53. Kacerovsky M, Pliskova L, Bolehovska R, Lesko D, Gerychova R, Janku P, et al. Cervical Gardnerella vaginalis in women with preterm prelabor rupture of membranes. PLoS ONE. (2021) 16:e0245937. doi: 10.1371/journal.pone.0245937
54. Bartkeviciene D, Opolskiene G, Bartkeviciute A, Arlauskiene A, Lauzikiene D, Zakareviciene J, et al. The impact of Ureaplasma infections on pregnancy complications. Libyan J Med. (2020) 15:1812821. doi: 10.1080/19932820.2020.1812821
55. Kim SA, Park KH, Lee SM, Kim YM, Hong S. Inflammatory proteins in the amniotic fluid, plasma, and cervicovaginal fluid for the prediction of intra-amniotic infection/inflammation and imminent preterm birth in preterm labor. Am J Perinatol. (2020). doi: 10.1055/s-0040-1718575. [Epub ahead of print].
56. Chambers S, Pons JC, Richard A, Chiesa M, Bouyer J, Papiernik E. Vaginal infections, cervical ripening and preterm delivery. Eur J Obstet Gynecol Reprod Biol. (1991) 38:103–8. doi: 10.1016/0028-2243(91)90185-N
57. Donders GG, van Calsteren C, Bellen G, Reybrouck R, van den Bosch T, Riphagen I, et al. Association between abnormal vaginal flora and cervical length as risk factors for preterm birth. Ultrasound Obstetr Gynecol. (2010). doi: 10.1002/uog.7568. [Epub ahead of print].
58. di Paola M, Seravalli V, Paccosi S, Linari C, Parenti A, de Filippo C, et al. Identification of vaginal microbial communities associated with extreme cervical shortening in pregnant women. J Clin Med. (2020) 9:3621. doi: 10.3390/jcm9113621
59. Gerson KD, McCarthy C, Elovitz MA, Ravel J, Sammel MD, Burris HH. Cervicovaginal microbial communities deficient in Lactobacillus species are associated with second trimester short cervix. Am J Obstetr Gynecol. (2020) 222:491.e1–8. doi: 10.1016/j.ajog.2019.11.1283
60. Kacerovsky M, Pliskova L, Bolehovska R, Gerychova R, Janku P, Matlak P, et al. Lactobacilli-dominated cervical microbiota in women with preterm prelabor rupture of membranes. Pediatr Res. (2020) 87:952–60. doi: 10.1038/s41390-019-0692-1
61. Atassi F, Pho Viet Ahn DL, Lievin-Le Moal V. Diverse expression of antimicrobial activities against bacterial vaginosis and urinary tract infection pathogens by cervicovaginal microbiota strains of Lactobacillus gasseri and Lactobacillus crispatus. Front Microbiol. (2019) 10:2900. doi: 10.3389/fmicb.2019.02900
62. Wang S, Wang Q, Yang E, Yan L, Li T, Zhuang H. Antimicrobial compounds produced by vaginal Lactobacillus crispatus are able to strongly inhibit Candida albicans growth, hyphal formation and regulate virulence-related gene expressions. Front Microbiol. (2017) 8:564. doi: 10.3389/fmicb.2017.00564
63. Short C-ES, Brown RG, Quinlan R, Lee YS, Smith A, Marchesi JR, et al. Lactobacillus-depleted vaginal microbiota in pregnant women living with HIV-1 infection are associated with increased local inflammation and preterm birth. Front Cell Infect Microbiol. (2021) 10:919. doi: 10.3389/fcimb.2020.596917
64. Begnel ER, Drake AL, Kinuthia J, Matemo D, Huang M-L, Ásbjörnsdóttir KH, et al. Cervical cytomegalovirus reactivation, cytokines and spontaneous preterm birth in Kenyan women. Clin Exp Immunol. (2021) 203:472–9. doi: 10.1111/cei.13558
65. Straface G, Selmin A, Zanardo V, de Santis M, Ercoli A, Scambia G. Herpes simplex virus infection in pregnancy. Infect Dis Obstet Gynecol. (2012) 2012:385697. doi: 10.1155/2012/385697
66. Li D-K, Raebel MA, Cheetham TC, Hansen C, Avalos L, Chen H, et al. Genital herpes and its treatment in relation to preterm delivery. Am J Epidemiol. (2014) 180:1109–17. doi: 10.1093/aje/kwu242
67. Liu P, Xu L, Sun Y, Wang Z. The prevalence and risk of human papillomavirus infection in pregnant women. Epidemiol Infect. (2014) 142:1567–78. doi: 10.1017/S0950268814000636
68. Caballero A, Dudley D, Ferguson J, Pettit K, Boyle A. Maternal human papillomavirus and preterm premature rupture of membranes: a retrospective cohort study. J Womens Health. (2019) 28:606–11. doi: 10.1089/jwh.2018.7043
69. Martínez-Leal B, Ivette Álvarez-Banderas K, Sánchez-Dávila H, Dávila-Rodríguez I, Cortés-Gutiérrez EI. Human papillomavirus as a single infection in pregnant women from Northeastern Mexico: cross-sectional study TT. Ijrm. (2020) 18:129–34. doi: 10.18502/ijrm.v18i2.6433
70. Niyibizi J, Zanré N, Mayrand M-H, Trottier H. Association between maternal human papillomavirus infection and adverse pregnancy outcomes: systematic review and meta-analysis. J Infect Dis. (2020) 221:1925–37. doi: 10.1093/infdis/jiaa054
71. Wiik J, Nilsson S, Kärrberg C, Strander B, Jacobsson B, Sengpiel V. Associations of treated and untreated human papillomavirus infection with preterm delivery and neonatal mortality: a Swedish population-based study. PLoS Med. (2021) 18:e1003641. doi: 10.1371/journal.pmed.1003641
72. Aldhous MC, Bhatia R, Pollock R, Vragkos D, Cuschieri K, Cubie HA, et al. HPV infection and pre-term birth: a data-linkage study using Scottish Health Data [version 1; peer review: 3 approved]. Wellcome Open Res. (2019). 4:48. doi: 10.12688/wellcomeopenres.15140.1
73. Wittmaack A, Dudley D, Boyle A. Maternal history of cervical surgery and preterm delivery: a retrospective cohort study. J Womens Health. (2019) 28:1538–42. doi: 10.1089/jwh.2018.7457
74. Kyrgiou M, Athanasiou A, Kalliala IEJ, Paraskevaidi M, Mitra A, Martin-Hirsch PP, et al. Obstetric outcomes after conservative treatment for cervical intraepithelial lesions and early invasive disease. Cochrane Database Syst Rev. (2017) 11:CD012847. doi: 10.1002/14651858.CD012847
76. Mešić Ð*ogić L, Lučić N, Mićić D, Omeragić F, HodŽić E, Fazlagić S, et al. Correlation between cervical infection and preterm labor. Medicinski Glasnik. (2017) 14:91–7. doi: 10.17392/886-16
77. Boomgaard JJ, Dekker KS, van Rensburg E, van den Berg C, Niemand I, Bam RH, et al. Vaginitis, cervicitis, and cervical length in pregnancy. Am J Obstet Gynecol. (1999) 181:964–7. doi: 10.1016/S0002-9378(99)70333-9
78. Sierra L-J, Brown AG, Barilá GO, Anton L, Barnum CE, Shetye SS, et al. Colonization of the cervicovaginal space with Gardnerella vaginalis leads to local inflammation and cervical remodeling in pregnant mice. PLoS ONE. (2018) 13:e0191524. doi: 10.1371/journal.pone.0191524
79. McGee D, Smith A, Poncil S, Patterson A, Bernstein AI, Racicot K. Cervical HSV-2 infection causes cervical remodeling and increases risk for ascending infection and preterm birth. PLoS ONE. (2017) 12:1–15. doi: 10.1371/journal.pone.0188645
80. Racicot K, Cardenas I, Wunsche V, Aldo P, Guller S, Means RE, et al. Viral infection of the pregnant cervix predisposes to ascending bacterial infection. J Immunol. (2013) 191:934–41. doi: 10.4049/jimmunol.1300661
81. Tantengco OAG, Richardson LS, Medina PMB, Han A, Menon R. Organ-on-chip of the cervical epithelial layer: a platform to study normal and pathological cellular remodeling of the cervix. FASEB J. (2021) 35:e21463. doi: 10.1096/fj.202002590RRR
82. Choi SJ, Jung KL, Oh SY, Kim JH, Roh CR. Cervicovaginal matrix metalloproteinase-9 and cervical ripening in human term parturition. Eur J Obstetr Gynecol Reprod Biol. (2009) 142:43–7. doi: 10.1016/j.ejogrb.2008.08.009
83. Kelly RW. Inflammatory mediators and cervical ripening. J Reprod Immunol. (2002) 57:217–. doi: 10.1016/S0165-0378(02)00007-4
84. Allaire AD, D'Andrea N, Truong P, McMahon MJ, Lessey BA. Cervical stroma apoptosis in pregnancy. Obstet Gynecol. (2001) 97:399–403. doi: 10.1097/00006250-200103000-00015
85. Tantengco OAG, Vink J, Medina PMB, Menon R. Oxidative stress promotes cellular damages in the cervix: implications for normal and pathologic cervical function in human pregnancy. Biol Reprod. (2021) 105:204–16. doi: 10.1093/biolre/ioab058
86. Tantengco OAG, Richardson LS, Menon R. Effects of a gestational level of estradiol on cellular transition, migration, and inflammation in cervical epithelial and stromal cells. Am J Reprod Immunol. (2021) 85:e13370. doi: 10.1111/aji.13370
87. Tantengco OAG, Richardson LS, Vink J, Kechichian T, Medina PMB, Pyles RB, et al. Progesterone alters human cervical epithelial and stromal cell transition and migration: Implications in cervical remodeling during pregnancy and parturition. Mol Cell Endocrinol. (2021) 529:111276. doi: 10.1016/j.mce.2021.111276
88. Gordon J, Mowa CN. Mechanobiology of mice cervix: expression profile of mechano-related molecules during pregnancy. Cell Tissue Res. (2019) 376:443–56. doi: 10.1007/s00441-018-02983-8
89. Hassan SS, Romero R, Tarca AL, Nhan-Chang C-L, Vaisbuch E, Erez O, et al. The transcriptome of cervical ripening in human pregnancy before the onset of labor at term: identification of novel molecular functions involved in this process. J Matern Fetal Neonat Med. (2009) 22:1183–93. doi: 10.3109/14767050903353216
90. Jeong HC, Kim HY, Kim HY, Wang E-J, Ahn KH, Oh M-J, et al. Changes in gene expression of cervical collagens, metalloproteinases, and tissue inhibitors of metalloproteinases after partial cervical excision-induced preterm labor in mice. PLoS ONE. (2021) 16:e0250108. doi: 10.1371/journal.pone.0250108
91. Manning R, James CP, Smith MC, Innes BA, Stamp E, Peebles D, et al. Predictive value of cervical cytokine, antimicrobial and microflora levels for pre-term birth in high-risk women. Sci Rep. (2019) 9:11246. doi: 10.1038/s41598-019-47756-7
92. Burris HH, Riis VM, Schmidt I, Gerson KD, Brown A, Elovitz MA. Maternal stress, low cervicovaginal β-defensin, and spontaneous preterm birth. Am J Obstetr Gynecol MFM. (2020) 2:100092. doi: 10.1016/j.ajogmf.2020.100092
93. Suff N, Karda R, Diaz JA, Ng J, Baruteau J, Perocheau D, et al. Cervical gene delivery of the antimicrobial peptide, Human β-Defensin (HBD)-3, in a mouse model of ascending infection-related preterm birth. Front Immunol. (2020) 11:106. doi: 10.3389/fimmu.2020.00106
94. Hein M, Helmig RB, Schønheyder HC, Ganz T, Uldbjerg N. An in vitro study of antibacterial properties of the cervical mucus plug in pregnancy. Am J Obstet Gynecol. (2001) 185:586–92. doi: 10.1067/mob.2001.116685
95. Hein M, Valore EV, Helmig RB, Uldbjerg N, Ganz T. Antimicrobial factors in the cervical mucus plug. Am J Obstetr Gynecol. (2002) 187:137–44. doi: 10.1067/mob.2002.123034
96. Helmig R, Uldbjerg N, Ohlsson K. Secretory leukocyte protease inhibitor in the cervical mucus and in the fetal membranes. Eur J Obstet Gynecol Reprod Biol. (1995) 59:95–101. doi: 10.1016/0028-2243(94)02023-8
97. Vornhagen J, Quach P, Santana-Ufret V, Alishetti V, Brokaw A, Armistead B, et al. Human cervical mucus plugs exhibit insufficiencies in antimicrobial activity towards group B Streptococcus. J Infect Dis. (2018) 217:1626–36. doi: 10.1093/infdis/jiy076
98. Dolgushin II, Telesheva LF, Savochkina AI, Markina OV. [Anti-inflammatory cytokines of cervical secretions and the blood serum in women with genital infections]. Zhurnal Mikrobiologii, Epidemiologii i Immunobiologii. (2004) 4:43–6.
99. Mattsby-Baltzer I, Platz-Christensen JJ, Hosseini N, Rosén P. IL-1beta, IL-6, TNFalpha, fetal fibronectin, and endotoxin in the lower genital tract of pregnant women with bacterial vaginosis. Acta Obstet Gynecol Scand. (1998) 77:701–6. doi: 10.1034/j.1600-0412.1998.770701.x
100. Sawada M, Otsuki K, Mitsukawa K, Yakuwa K, Nagatsuka M, Okai T. Cervical inflammatory cytokines and other markers in the cervical mucus of pregnant women with lower genital tract infection. Int J Gynaecol Obstet. (2006) 92:117–21. doi: 10.1016/j.ijgo.2005.10.004
101. Campos ACC, Murta EFC, Michelin MA, Reis C. Evaluation of cytokines in endocervical secretion and vaginal ph from women with bacterial vaginosis or human papillomavirus. ISRN Obstet Gynecol. (2012) 2012:342075. doi: 10.5402/2012/342075
102. de Tomasi JB, Opata MM, Mowa CN. Immunity in the cervix: interphase between immune and cervical epithelial cells. J Immunol Res. (2019) 2019:7693183. doi: 10.1155/2019/7693183
103. Prakash M, Patterson S, Kapembwa MS. Macrophages are increased in cervical epithelium of women with cervicitis. Sex Transm Infect. (2001) 77:366–9. doi: 10.1136/sti.77.5.366
104. Mittal A, Rastogi S, Reddy BS, Verma S, Salhan S, Gupta E. Enhanced immunocompetent cells in chlamydial cervicitis. J Reprod Med. (2004) 49:671–7.
105. Tang VA, Rosenthal KL. Intravaginal infection with herpes simplex virus type-2 (HSV-2) generates a functional effector memory T cell population that persists in the murine genital tract. J Reprod Immunol. (2010) 87:39–44. doi: 10.1016/j.jri.2010.06.155
106. Zhu J, Hladik F, Woodward A, Klock A, Peng T, Johnston C, et al. Persistence of HIV-1 receptor-positive cells after HSV-2 reactivation is a potential mechanism for increased HIV-1 acquisition. Nat Med. (2009) 15:886–92. doi: 10.1038/nm.2006
107. Stranik J, Kacerovsky M, Andrys C, Soucek O, Bolehovska R, Holeckova M, et al. Intra-amniotic infection and sterile intra-amniotic inflammation are associated with elevated concentrations of cervical fluid interleukin-6 in women with spontaneous preterm labor with intact membranes. J Matern Fetal Neonat Med. (2021) 1–9. doi: 10.1080/14767058.2020.1869932. [Epub ahead of print].
108. Rizzo G, Capponi A, Angelini E, Romanini C. Ultrasonographic and biochemical markers of preterm labor. J Matern Fetal Invest. (1998) 8:150–5.
109. Amabebe E, Reynolds S, Stern VL, Parker JL, Stafford GP, Paley MN, et al. Identifying metabolite markers for preterm birth in cervicovaginal fluid by magnetic resonance spectroscopy. Metabolomics. (2016) 12:67. doi: 10.1007/s11306-016-0985-x
110. Hong S, Jung YM, Lee YE, Park Y, Kim YM, Park KH. Complement and other immune-related factors in cervicovaginal fluid associated with intra-amniotic infection/inflammation and spontaneous preterm delivery in women with preterm labor. Arch Gynecol Obstet. (2020) 301:1431–9. doi: 10.1007/s00404-020-05560-z
111. Lee SM, Park KH, Hong S, Kim YM, Park YH, Lee YE, et al. Identification of cultivable bacteria in amniotic fluid using cervicovaginal fluid protein microarray in preterm premature rupture of membranes. Reprod Sci. (2020) 27:1008–17. doi: 10.1007/s43032-020-00143-4
112. Stranik J, Kacerovsky M, Soucek O, Kolackova M, Musilova I, Pliskova L, et al. IgGFc-binding protein in pregnancies complicated by spontaneous preterm delivery: a retrospective cohort study. Sci Rep. (2021) 11:6107. doi: 10.1038/s41598-021-85473-2
113. Negi D, Guleria K, Tyagi V, Dev Banerjee B, Suneja A. Evaluation of trappin-2 in cervicovaginal secretions as predictor of spontaneous preterm birth in asymptomatic high-risk women: nested case-control study. Int J Gynecol Obstetr. (2021) 154:56–61. doi: 10.1002/ijgo.13512
114. Amabebe E, Reynolds S, Stern V, Stafford G, Paley M, Anumba DOC. cervicovaginal fluid acetate: a metabolite marker of preterm birth in symptomatic pregnant women. Front Med. (2016) 3:48. doi: 10.3389/fmed.2016.00048
115. Suzuki S, Hoshi S, Sekizawa A, Sagara Y, Tanaka M, Kinoshita K, et al. Current status of Neisseria gonorrhoeae cervicitis in pregnant women in Japan. PLoS ONE. (2019) 14:e0211595. doi: 10.1371/journal.pone.0211595
116. Workowski KA, Bachmann LH, Chan PA, Johnston CM, Muzny CA, Park I, et al. Sexually transmitted infections treatment guidelines, 2021. MMWR. Recommend Rep. (2021) 70:1–187. doi: 10.15585/mmwr.rr7004a1
117. Marrazzo JM, Martin DH. Management of women with cervicitis. Clin Infect Dis. (2007) 44(Suppl. 3):S102–10. doi: 10.1086/511423
Keywords: biomarker, cervix, cervical remodeling, pregnancy, parturition
Citation: Tantengco OAG and Menon R (2022) Breaking Down the Barrier: The Role of Cervical Infection and Inflammation in Preterm Birth. Front. Glob. Womens Health 2:777643. doi: 10.3389/fgwh.2021.777643
Received: 15 September 2021; Accepted: 27 December 2021;
Published: 18 January 2022.
Edited by:
Dilly O. C. Anumba, The University of Sheffield, United KingdomReviewed by:
Antonio Simone Laganà, University of Insubria, ItalyKatherine E. A. Semrau, Ariadne Labs, United States
Copyright © 2022 Tantengco and Menon. This is an open-access article distributed under the terms of the Creative Commons Attribution License (CC BY). The use, distribution or reproduction in other forums is permitted, provided the original author(s) and the copyright owner(s) are credited and that the original publication in this journal is cited, in accordance with accepted academic practice. No use, distribution or reproduction is permitted which does not comply with these terms.
*Correspondence: Ramkumar Menon, cmEybWVub25AdXRtYi5lZHU=