- 1Reproductive Health Research Institute (RHRI), Santiago, Chile
- 2Fundación Médica San Cristóbal, Santiago, Chile
- 3Facultad de Medicina, Universidad San Sebastián, Santiago, Chile
- 4University of Nothe Dame, Sydney, NSW, Australia
- 5Facultad de Medicina Clínica Alemana, Universidad del Desarrollo, Santiago, Chile
- 6Tallahassee Community College, Tallahassee, FL, United States
Stress is known to be associated with adverse health outcomes. The COVID-19 pandemic and its associated lockdowns are examples of chronic stressors. Lockdown measures inadvertently caused significant psychological distress and became a powerful source of anxiety/stress, sleep disturbances, nutritional changes and weight gain. Stress is known to impact women's health specifically, through hypothalamic-pituitary-gonadal (HPG) axis dysfunction and resultant ovulatory dysfunction. Such dysfunction may manifest in menstrual irregularities and/or infertility due to hypothalamic hypogonadism. Here, we review the key physiological mediators of stress and associated ovulatory dysfunction. The kisspeptinergic system is comprised of sets of neurons located in the hypothalamus, the rostral periventricular region of the third ventricle (RP3V) and the arcuate nucleus (ARC). This system links nutrition, reproductive signals and stress. It plays a key role in the function of the HPG axis. During chronic stress, the kisspeptinergic system affects the HPG axis, GnRH pulsatility, and, therefore, ovulation. Leptin, insulin and corticotrophin-releasing hormone (CRH) are thought to be additional key modulators in the behavioral responses to chronic stress and may contribute to stress-related ovulatory dysfunction. This mini-review also summarizes and appraises the available evidence on the negative impact of chronic stress as a result of the COVID-19 pandemic lockdowns. It proposes physiological mechanisms to explain the observed effects on women's reproductive health and well-being. The review suggests areas for future research.
Introduction
Ovulation is the result of the coordinated action of the endocrine, paracrine, and autocrine systems. Any disruption in the delicately coordinated interaction between the components of the hypothalamic-pituitary-ovarian axis may lead to ovulatory dysfunction (1). Persistent irregularities in the ovulatory cycle can be associated with stress, as well as with, endocrine, gynecological, autoimmune, nutritional, genetic, and iatrogenic disorders (2). Despite regular menses generally being considered an indicator of ovulation, they can, in fact, be associated with anovulation (3). Therefore, monitoring for regular ovulation, not just regular menstruation, is key when analyzing women's health.
Stress has many adverse health effects (4). Unfortunately, the relative lack of objective markers for chronic stress, means that identifying individuals suffering with chronic stress is very challenging clinically. In women, phenotypic markers of chronic stress include menstrual irregularities, amenorrhea, and/or infertility due to hypothalamic hypogonadism (5). Previous studies of the relationship between stress and menstrual cycle have yielded conflicting results. Some have found stress is associated with longer cycles, others with shorter cycles, and still others have found no association of stress and cycle length (6). It is interesting to note that the menstrual cycle changes observed with stress are sometimes similar to those experienced by women in the perimenopause.
The COVID-19 pandemic, and its lockdowns, have caused psychological distress, resulting in populations living under conditions of chronic stress (7–13). Lockdowns have been characterized by the development of negative lifestyles and their consequent metabolic changes (7). During the COVID-19 pandemic, women have been found to have a higher incidence of anxiety and depression disorders (14). This mini-review describes how the kisspeptinergic system integrates women's response to stress through its impact on energy balance and reproduction. Understanding such integration reveals how the stress associated with the COVID-19 pandemic may affect women's ovulatory cycles. This review evaluates the clinical evidence on this topic thus far and suggests areas for future research.
Stress And Ovulatory Dysfunction
Ovulatory dysfunction is a group of disorders with variable clinical presentations that occasionally have serious long-term adverse effects. According to the World Health Organization (WHO), ovulation disorders are the main cause of infertility (82). These disorders fall into three categories: Group I ovulation disorders encompass hypothalamic insufficiency. Group II disorders involve HPO axis dysfunction and Group III constitutes ovarian insufficiency (15).
Group I ovulatory disorders include functional hypothalamic amenorrhea (FHA) (15–17). FHA is recognized as a sentinel indicator of chronic stress (4). FHA can also be triggered by excessive exercise or weight loss (16). In FHA, the final common pathway is activation of the limbic-hypothalamic-pituitary-adrenal axis (18) which then reduces the central gonadotropin-releasing hormone (GnRH) drive (19, 20). Stress and the resulting hormonal changes could trigger undernutrition or overnutrition, depending on fuel availability, attitudes toward food, and dietary behaviors such as binging, purging, overeating, or restricting. Reversal of functional hypothalamic amenorrhea includes restoration of ovulatory ovarian function and fertility (5).
Dysfunction of the HPO axis (Group II) constitutes 85% of ovulation disorders (15). Stress can trigger such dysfunction (4). It has been reported that prolonged or chronic stress in rats and human females can block, inhibit, or delay the preovulatory LH surge and thus disrupt the estrous or menstrual cycle (21). The endocrine system, including most specifically the hypothalamic-pituitary-adrenal (HPA) axis, and the immune system contribute to the development of these disorders (22–26).
Physiology Of Stress
Stress primarily activates two systems: The sympathetic nervous system (SNS) and the Hypothalamic-Pituitary-Adrenal Axis (HPA). The activation of the SNS causes the release of Epinephrine and Norepinephrine. The activation of the HPA axis triggers a hormonal cascade in which corticotropin releasing hormone (CRH) is released by the hypothalamus, adrenocorticotropic releasing hormone (ACTH) by the anterior pituitary and finally, glucocorticoids by the adrenal gland. This results in an increase in the level of cortisol (27). Cortisol is released in order to increase glucose levels, which are needed to adequately respond to stressful situations. In order to achieve these levels of circulating glucose, cortisol promotes gluconeogenesis in the liver, the mobilization of amino acids from muscles and an increased lipolysis in the adipocytes (28). There is a strong inter-relationship between activation of the hypothalamo-pituitary-adrenal axis and energy homeostasis. Stress and glucocorticoids act to control both food intake and energy expenditure.
Food Intake And Metabolic Signals
Under adequate nutritional conditions, the presence of metabolic signals such as insulin and leptin will activate anorexigenic neurons, as the POMC (pro-opiomelanocortin) neurons that release α-MSH (alpha-melanocyte stimulating hormone), causing satiety. On the other hand, insulin and leptin will inhibit the orexigenic neurons, which release NPY (neuropeptide Y) and AgRP (Agouti-related peptide), causing hunger (29). In overweight and obese individuals, high levels of leptin and insulin cause a state of resistance to both hormones, which, through positive feedback mechanisms, further increases their levels (30). Leptin and insulin resistance thus results in aberrant feedback signaling, causing the orexigenic neurons to release NPY and AgRP. This means the individual feels hungry, with the brain thinking more nutrition is needed, despite actually having excessive energy storage. These peptides are released into the arcuate nucleus of hypothalamus where reproductive signals are also sensed (31). The link between nutrition and reproductive signals is the kisspeptinergic system.
The Kisspeptinergic System
The kisspeptinergic system consists of two populations of neurons in the hypothalamus; the rostral periventricular region of the third ventricle (RP3V) (also known as the preoptic area); and the arcuate nucleus (ARC) (also known as the infundibular nucleus). Both neuronal groups produce the neuropeptide kisspeptin, which plays a critical role in the function of the hypothalamic-pituitary-gonadal (HPG) axis (32). From these two areas, kisspeptinergic neurons release kisspeptin to GnRH neurons, which have kisspeptin receptors (Kiss1R). This stimulates GnRH neurons to release GnRH (33) (Figure 1).
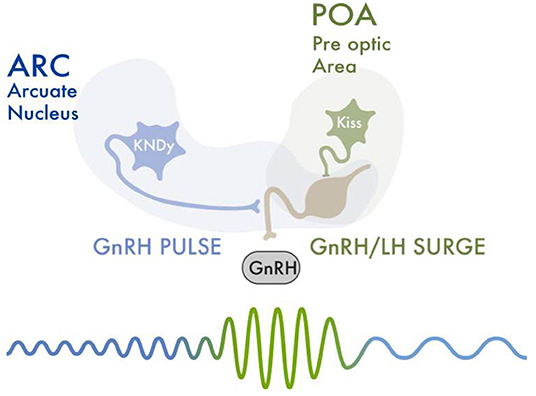
Figure 1. An increase in FSH levels leads to recruitment and development of ovarian follicles. Selected follicles produce rising estradiol levels. Estradiol and inhibin exert a negative feedback upon the HPG axis, thus decreasing FSH levels. Estradiol also causes a negative feedback upon the KissARC neurons. Kisspeptin-neurokinin B-dynorphin (KNDy) neurons present in the hypothalamus's arcuate nucleus (ARC), co-express neurokinin B (NKB) and dynorphin (Dy) and are essential for GnRH pulse generation and secretion. Low frequency hypothalamic GnRH pulses lead to a release of FSH and LH from the anterior hypophysis. One of the selected ovarian follicles becomes dominant, and secretes increasingly high levels of estradiol. This rapid and sustained increase in estradiol values gives the required signal to activate the KissRP3V/POA neurons. This activation triggers the GnRH pulsatility and release, necessary for the LH/FSH surge. The LH surge is initiated, which causes follicular luteinization and an initial progesterone rise. Progesterone maintains the LH peak and is necessary for follicular rupture and adequate ovulation. After ovulation, estradiol levels abruptly decrease. This “turns off” the KissRP3V/POA neurons, ending the LH/FSH surge.
Kisspeptinergic neurons located in the arcuate nucleus (KissARC) are regulated mainly by metabolic inputs such as insulin, leptin and ghrelin (31). Kisspeptinergic neurons located in the anteroventral periventricular nucleus in the preoptic area (KissRP3V/POA) are regulated mainly by reproductive signals such as estradiol, testosterone and progesterone (34). Arcuate kisspeptin expression is similar in both sexes, whereas kisspeptinergic expression in the preoptic area is greater in females (35). When estradiol concentration is elevated, kisspeptin mRNA expression is increased in KissRP3V neurons and decreased in the ARC nucleus (34). On the other hand, selective deletion of the classical progesterone receptor neurons in kisspeptinergic neurons prevents the LH surge. This suggests estrogens and progesterones act synergistically in kisspeptinergic neurons to modulate gonadotrophin release (36). The relationship between testosterone and the kisspeptinergic nuclei is not well established. In mammals, it has been shown that high levels of testosterone during prenatal development decrease the size of the preoptic kisspeptinergic nucleus area (34).
Kisspeptinergic System As The Pacemaker Of The Menstrual Cycle
GnRH is released in a pulsatile pattern throughout all of the menstrual cycle, but the frequency and amplitude of its pulses differs with cycle phase. During the periovulatory period, there is an increase in the frequency and amplitude of GnRH pulses. Kisspeptinergic neurons induce such changes in the pattern of GnRH release (33). The increase in GnRH is generated by the activation of the KissRP3V/POA. These neurons respond to the increasing estradiol levels produced by the dominant follicle that occur around the periovulatory period (37). The concentration of estradiol that is produced by recruited follicles during the early follicular phase increases the pattern of secretion of kisspeptin by the KissARC. Higher levels of estradiol are later produced by the dominant follicle and increase kisspeptin release by the KissRP3V/POA (38). Such positive feedback of estradiol on kisspeptin release therefore increases the amplitude and frequency of GnRH production and secretion. This causes the LH surge. After ovulation, during the luteal phase, estradiol and progesterone modulate GnRH pulsatility by acting upon the KissARC (39).
Stress And The Kisspeptinergic System
Under stress conditions, increased cortisol has an indirect inhibitory effect on KissARC neurons (40). This effect is mediated by the pro-opiomelanocortin and cocaine and amphetamine-regulated transcript (POMC/CART) neurons, located in the arcuate nucleus. These neurons, depending upon the stimuli they receive, secrete α-MSH. Alpha-MSH stimulates the KissARC, or β-endorphins, which inhibit the KissARC. Under stress conditions, these neurons detect CRH and cortisol. CRH and cortisol stimulate the production of β-endorphins over the production of α-MSH (16, 41). Beta-endorphins exert an inhibitory effect on KissARC neurons. Additionally, the deficit of α-MSH is sensed as an orexigenic stimulus (42). Another mechanism by which stress affects the kisspeptinergic system is through the increased expression and activity of gonadotropin-inhibitory hormone/RFamide-related peptides (GnIH/RFRP-3) (37, 43). GnIH/RFRP-3 is a peptide hormone that acts in the hypothalamus and pituitary gland. GnIH/RFRP-3 suppresses the synthesis and release of GnRH and gonadotropins (44). CRH, cortisol and GnIH inhibit the activity of Kiss ARC neurons, Kiss RP3V/POA neurons and GnRH neurons (45).
CRH is secreted by neurons in the anterior portion of the paraventricular nucleus of the hypothalamus. Under stress conditions, additional CRH-producing neurons are activated. This activation is greater in females than in males (46). This level of CRH expression in females has recently been associated with increased levels of anxiety (47). Data from non-human animal models also reveal extensive sex differences in CRH functions ranging from its presynaptic regulation to its postsynaptic efficacy (48). For example, females have greater CRH receptor turnover, post activation, than males. It has also been shown that at the locus coeruleus, which is involved in stress regulation and arousal, females have a greater number of neurons and dendritic projections (48–50). These differences make females more vulnerable to stress conditions (51). CRH is recognized as a key modulator of behavioral responses to stress. Therefore, sex differences in CRH processing may partly explain sex differences in responses to stress (48).
Stress, Cortisol And Appetite-Related Hormones
Glucocorticoids released during stressful conditions act on the hypothalamus, increasing the sensation of hunger. This leads to an increase in appetite and food intake, especially intake of foods high in saturated fat and carbohydrates (52). When glucocorticoid levels remain high over time, such persistent eating changes can cause weight gain (53, 54). This mechanism is not fully elucidated. However, it has been suggested that during stress, the expression of POMC (an anorexigenic stimulus) is inhibited, and the expression of NPY and AgRP (orexigenic stimuli) is increased (55, 56). Individuals under chronic stress conditions also have higher levels of leptin, insulin, glucose and ghrelin (57).
Sex is an important factor determining plasma leptin concentration. Women have markedly higher leptin concentrations than men (58). Elevated glucocorticoids, as found under stress conditions, affect leptin and insulin function and sensitivity. This contributes to the development of a leptin-insulin resistant state (59). Furthermore, in chronic stress situations, the release of LH and FSH is inhibited in both overweight and normal weight women. This contributes to ovulation inhibition (60, 61). An imbalance in leptin and insulin will also influence the kisspeptinergic system, thus affecting ovulatory function (Figure 2).
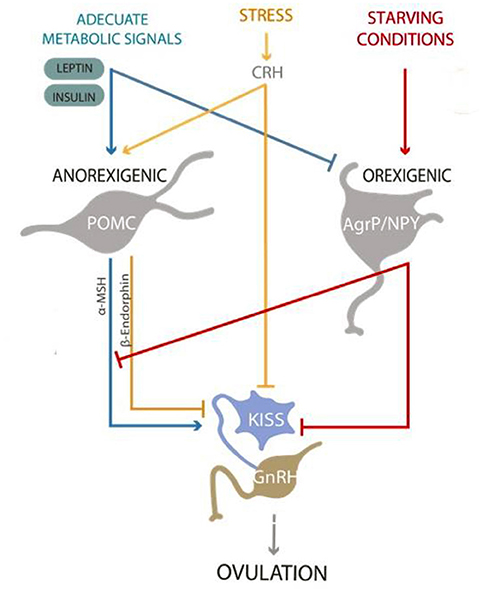
Figure 2. Kisspeptinergic neurons from the “preoptic area” and “arcuate nucleus” release kisspeptin to stimulate GnRH neurons to release GnRH. Under proper nutritional conditions, the presence of metabolic signals such as insulin and leptin will activate anorexigenic neurons, as the POMC neurons that release α-MSH, causing satiety. On the other hand, insulin and leptin will inhibit the orexigenic neurons, which release NPY and AgRP, causing hunger. Proper metabolic signals will stimulate the release of kisspeptin and promote ovulation. On the contrary, signs of starvation and/or stress will inhibit the release of kisspeptin, affecting ovulation and the reproductive process.
Functional Hypothalamic Amenorrhea
Weight loss and weight gain, excessive physical exercise, and chronic stress induce an anovulatory state which is called “functional hypothalamic amenorrhea” (FHA). This condition is one of the main causes of secondary amenorrhea. It occurs when GnRH pulsatility is affected by a decreased activity of the KissARC neurons. This decreases the release of FSH and LH, generating a state of anovulation and hypoestrogenism (16, 61). The mechanisms underlying the pathophysiology of FHA are not fully understood. However, kisspeptin, NPY, ghrelin, leptin and, corticotropin-releasing hormone (CRH) are thought to play important roles in the physiological regulation of pulsatile GnRH secretion and thus are likely to be involved in the pathophysiology of FHA (62). As mentioned above, kisspeptin can directly stimulate GnRH secretion from the arcuate nucleus of the hypothalamus. The importance of the suppression of the kisspeptinergic system in FHA is further demonstrated by the fact that acute administration of kisspeptin to women with FHA potently stimulates gonadotropin release and ultimately restores ovulation (63).
Stress And Lifestyle During Covid-19 Pandemic
The SARS-CoV-2 pandemic has affected millions of people worldwide. Many countries have adopted lockdowns or quarantines as strategies to help minimize the spread of the disease and the collapse of healthcare systems (64). The COVID-19 pandemic, and its lockdowns, have caused psychological distress, with populations living under conditions of chronic stress (7–13). It is important to note that chronic stress is a prolonged and constant feeling of stress that can negatively affect our health if it goes untreated and that state of emotional suffering associated with stressors and demands that are difficult to cope with in daily life lead to a psychological distress.
In the US, more than half university students reported moderate to severe anxiety symptoms during the pandemic (14). Severe anxiety symptoms were associated with increased hunger, emotional overeating and decreased enjoyment of food (14). Furthermore, obese people have reported, an excessive desire to eat during the pandemic (65). The lockdowns have caused so many changes in nutritional habits, sleep patterns, and physical activity routines that in the United States, people refer to the “Quarantine 15”. This phrase refers to the 15 pounds (6.8 kg) of weight that many Americans have gained during the lockdown (66). Individuals who reported changes in their eating behaviors during the pandemic also reported concurrent increases in depression (67). Stress is associated with an increase in high calorie food intake (68). This association is particularly strong in those who emotionally overeat and weaker or absent in those who have greater cognitive flexibility. Promoting cognitive flexibility and helping to prevent emotional overeating could help decrease the intake of high calorie foods during stressful conditions, such as the COVID-19 pandemic (68). Women are more vulnerable than men to developing anxiety (69–73). They are diagnosed twice as often as men and this prevalence increases with age and with the gradual decrease in estradiol secretion at menopause (74, 75). Therefore, tools to help prevent anxiety and pathological eating behaviors are like to particularly help women.
The Lockdown And The Menstrual Cycle
There is a growing, though nascent, body of evidence appraising the impact of the COVID-19 pandemic and its impact on women's reproductive health. Whilst one cross-sectional study of 125 women did not find that the pandemic altered menstrual cycle characteristics, several other larger-scale studies have linked altered menses with stress associated with COVID-19 pandemic. The smallest of these studies included 263 participants with an average age of 26.3 ± 6.9 (18–45) (76). The authors found that menses duration and heaviness decreased in a statistically significant fashion during the COVID-19 pandemic. However, the clinical significance of such changes is unclear. Period time only decreased from 6.3 to 5.9 days and pads per day changed from 3.7 to 3.2. A larger cross-sectional study of 952 female healthcare workers in Turkey found that COVID-19 pandemic-induced anxiety, perceived stress, and depressive symptoms, were associated with increased menstrual cycle irregularity (77). Women with regular menstrual cycles for more than 1 year before the beginning of the pandemic were included in the study. During the COVID-19 pandemic, 71% of participants had regular menstrual cycles, and 23% had irregular menstrual cycles. This was a significant change given that all recruited women had regular menstrual cycles for at least 1 year. Covid stress scale scores (CSS) were significantly higher in women with irregular cycles than women with regular cycles. Depression, anxiety, and stress scores were likewise significantly higher in women with irregular cycles. This study was limited by the self-selecting nature of survey responders and by its reliance on women -recall of cycle characteristics. However, as the authors note, over 75% of their respondents used a period tracking application on a smart device which improves accuracy of women's self-reported data. A further observational study of over 1,000 women corroborated such findings (78). This study used a social media and text survey to appraise the effects of the COVID-19 pandemic on women. All study participants reported typical signs of chronic stress such as a significant increase in low mood, poor appetite, binge eating, poor concentration, anxiety, poor sleep, loneliness and excess alcohol use. Women also reported a median 2 kg increase in self-reported body weight. Forty-six percent of participants reported a change in their menstrual cycle since the beginning of the pandemic and 53% described a worsening of premenstrual symptoms. Indeed, one third of participants reported new dysmenorrhea during the pandemic. Interestingly, whilst median cycle length and days of bleeding were unchanged, total cycle variability was increased.
The largest study of over 18,000 mobile app users similarly found that stress was reported in nearly half of participants during the COVID-19 pandemic (79). Interestingly, whilst a number of participants recorded more anovulatory cycles (7.7%) or cycles of abnormal length (19.5%) during the pandemic, a number of women actually recorded fewer anovulatory (9.6%) or abnormal length cycles (19.6%). The authors suggest that this may reflect that the COVID-19 pandemic likely affected women with different sociodemographic characteristics differently. For example, app-users in the study were typically from high-income countries (USA and Great Britain) and had high education levels. Therefore, a number of these women may have started working from home, rather than commuting. Studies have shown that women who began working from home, rather than commuting, may have had an increased opportunity to exercise or eat healthily, given the reduced commute time (80). Overall, these studies suggest there is an association between the COVID-19 pandemic-induced anxiety and increased prevalence of menstrual cycle irregularities in women. However, they also highlight that the COVID-19 pandemic measures did not affect all women equally.
Future Directions And Perspectives
The evidence evaluating the impact of the COVID-19 pandemic on women's reproductive health is still nascent and the consequences for women's reproductive health are just emerging. The medium- and longer-term effects of the pandemic remain to be seen. Even as lockdown-related stress recedes, chronic stress as a result of other factors (e.g., financial stressors) related to the pandemic may remain. The studies appraised in this mini-review were largely conducted at the beginning of the COVID-19 pandemic. It will be important to consider how stress levels develop and change at later stages of the pandemic and during post-pandemic recovery, as well as how such stress levels may influence women's menstrual cycles, reproductive health and well-being.
The studies were also limited in a number of other ways. Firstly, all the studies are based on self-reported personal data. Such self-reporting may have inaccuracies. In particular, pre-pandemic menstrual markers were often collected retrospectively and so were subject to recall bias. This also prevented rigorous controls for any changes in women's socio-economic and educational levels pre vs. during pandemic. Furthermore, a number of studies did not consider the effect of SARS-CoV-2 exposure or infection. This could present a confounding physiological factor. This is likely particularly important for studies that focused on female healthcare workers (77). Furthermore, many women in some studies were from a high socioeconomic group. This limits the general applicability of such work. For example, one study found that women with high levels of education (e.g., a PhD degree) had higher levels of increased perceived stress (48.9%) compared with women with a high school degree or lower (40.3%) (79).
The most significant limitation of these studies however was the lack of clinical laboratory data to determine objective measures of e.g., stress hormones and their impact on ovulation. For example, perceived stress may differ from physiologically high levels of stress. Clarifying whether women who feel more stressed show higher levels of stress hormones would be interesting. Furthermore, it is known that in chronic stress, the acute stress response may actually be blunted (81). Might this result in counter-intuitive impacts on women's ovulatory cycles?
Only one study used an additional biomarker (basal body temperature) in an attempt to record anovulatory cycles directly (79). Other papers focused on menses length and length of menstrual cycle. Total menstrual cycle length could be unchanged, but luteal and/or follicular phase, specifically might be impacted. As discussed in the introduction, ovulatory dysfunction can occur even when menstruation remains regular. More studies that track ovulatory function directly, through progesterone measurements, cervical fluid recordings or basal body temperature are needed. These studies could offer greater clarity and insight into women's health during times of high stress.
One strength of these studies was that the majority of papers did exclude women using hormonal contraceptives (76, 77, 79). This would have made it easier to observe physiological responses to stress on the menstrual cycle. It would be interesting to observe how hormonal contraception might impact perceived stress and physiological response.
Other areas for further research could involve whether times of chronic stress impact all women equally. For example, Sadler's work suggests that women who emotionally overeat may respond differently to stress (68). It would be interesting to further investigate this link and to elucidate whether its origins are genetic or social or both. Such work could allow women who are particularly vulnerable to stress to be identified and helped earlier. Finally, future work could look at how to best manage ovulatory dysfunction associated with chronic stress. For example, how might therapies target stress perception and management (e.g., cognitive behavioral therapy) or physiological markers of ovulatory dysfunction (e.g., hypoestrogenism in the case of FHA)?
Conclusion
During this time, a number of women have exhibited changes in their menstrual cycles. Many women have reported a worsening of premenstrual symptoms. This highlights the link between mental status and the reproductive axis. Monitoring their cycles more closely may allow women to identify alterations in their hormonal balance which might confirm or even indicate their stress levels. This mini-review has presented evidence that the COVID-19 pandemic has negatively affected women's reproductive health through the possible ovulatory dysfunction. Further work should focus on using biomarkers to better evaluate the nature of such dysfunction. Discerning which women are likely to be most at risk and benefit most from targeted therapies (e.g., cognitive behavioral therapy) may offer great help in the future. Even as the pandemic recedes, it is important to remember that women experience periods of acute and chronic stress across the world due to other factors. These can include war, famine and displacement. We hope that findings during the COVID-19 pandemic may allow us to give a better healthcare to women in the future.
Author Contributions
PV and JM: writing, review, and revision of the manuscript. HS and YB: bibliographic search and writing. SM: review and revision of the manuscript. All authors contributed to the article and approved the submitted version.
Conflict of Interest
The authors declare that the research was conducted in the absence of any commercial or financial relationships that could be construed as a potential conflict of interest.
Publisher's Note
All claims expressed in this article are solely those of the authors and do not necessarily represent those of their affiliated organizations, or those of the publisher, the editors and the reviewers. Any product that may be evaluated in this article, or claim that may be made by its manufacturer, is not guaranteed or endorsed by the publisher.
References
1. Vigil P, Lyon C, Flores B, Rioseco H, Serrano F. Ovulation, a sign of health. Linacre Q. (2017) 84:343–55. doi: 10.1080/00243639.2017.1394053
2. Vigil P, Ceric F, Cortés ME, Klaus H. Usefulness of monitoring fertility from menarche. J Pediatr Adolesc Gynecol. (2006) 19:173–79. doi: 10.1016/j.jpag.2006.02.003
3. Malcolm CE, Cumming DC. Does anovulation exist in eumenorrheic women? Obstet Gynecol. (2003) 102:317–18. doi: 10.1097/00006250-200308000-00019
4. Schneiderman N, Ironson G, Siegel SD. Stress and health: psychological, behavioral, and biological determinants. Annu Rev Clin Psychol. (2005) 1:607–28. doi: 10.1146/annurev.clinpsy.1.102803.144141
5. Prokai D, Berga SL. Neuroprotection via reduction in stress: altered menstrual patterns as a marker for stress and implications for long-term neurologic health in women. Int J Mol Sci. (2016) 17:2–9. doi: 10.3390/ijms17122147
6. Barsom SH, Mansfield PK, Koch PB, Gierach G, West SG. Association between psychological stress and menstrual cycle characteristics in perimenopausal women. Women's Health Issues. (2004) 14:235–41. doi: 10.1016/j.whi.2004.07.006
7. Droit-Volet S, Gil S, Martinelli N, Andant N, Clinchamps M, Parreira L, et al. Time and COVID-19 stress in the lockdown situation: time free, ≪dying≫ of boredom and sadness. PLoS ONE. (2020) 15:1–15. doi: 10.1371/journal.pone.0236465
8. Rehman U, Shahnawaz MG, Khan NH, Kharshiing KD, Khursheed M, Gupta K, et al. (2021). Depression, anxiety and stress among Indians in times of COVID-19 lockdown. Commun Mental Health J. 57:42–8. doi: 10.1007/s10597-020-00664-x
9. Dagnino P, Anguita V, Escobar K, Cifuentes S. Psychological effects of social isolation due to quarantine in Chile: an exploratory study. Front Psychiatry. (2020) 11:1–13. doi: 10.3389/fpsyt.2020.591142
10. Ramalho SM, Trovisqueira A, de Lourdes M, Gonçalves S, Ribeiro I, Vaz AR, et al. (2021). The impact of COVID-19 lockdown on disordered eating behaviors: the mediation role of psychological distress. Eat Weight Disord. 27:179–88. doi: 10.21203/rs.3.rs-98867/v1
11. Czepczor-Bernat K, Swami V, Modrzejewska A, Modrzejewska J. Covid-19-related stress and anxiety, body mass index, eating disorder symptomatology, and body image in women from poland: a cluster analysis approach. Nutrients. (2021) 13:1–12. doi: 10.3390/nu13041384
12. Stanton R, To QG, Khalesi S, Williams SL, Alley SJ, Thwaite TL, et al. Depression, anxiety and stress during covid-19: associations with changes in physical activity, sleep, tobacco and alcohol use in Australian adults. Int J Environ Res Public Health. (2020) 17:1–13. doi: 10.3390/ijerph17114065
13. Cherikh F, Frey S, Bel C, Attanasi G, Alifano M, Iannelli A. Behavioral food addiction during lockdown: time for awareness, time to prepare the aftermath. Obes Surg. 30:3585–87. doi: 10.1007/s11695-020-04649-3
14. Coakley KE, Le H, Silva SR, Wilks A. Anxiety is associated with appetitive traits in university students during the COVID-19 pandemic. Nutr J. (2021) 20:1–9. doi: 10.1186/s12937-021-00701-9
15. Mikhael S, Punjala-Patel A, Gavrilova-Jordan L. Hypothalamic-pituitary-ovarian axis disorders impacting female fertility. Biomedicines. (2019) 7:1–9. doi: 10.3390/biomedicines7010005
16. Meczekalski B, Katulski K, Czyzyk A, Podfigurna-Stopa A, MacIejewska-Jeske M. Functional hypothalamic amenorrhea and its influence on women's health. Acad Psychiatry. (2014) 37:1049–56. doi: 10.1007/s40618-014-0169-3
17. Gordon CM, Ackerman KE, Berga SL, Kaplan JR, Mastorakos G, Misra M, et al. Functional hypothalamic amenorrhea: an endocrine society clinical practice guideline. J Clin Endocrinol Metabol. (2017) 102:1413–39. doi: 10.1210/jc.2017-00131
18. Berga SL. Stress and reprodution: a tale of false dichotomy? Endocrinology. (2008) 149:867–68. doi: 10.1210/en.2008-0004
19. Reame NE, Sauder SE, Case GD, Kelch RP, Marshall JC. Pulsatile gonadotropin secretion in women with hypothalamic amenorrhea: evidence that reduced frequency of gonadotropin-releasing hormone secretion is the mechanism of persistent anovulation. J ical Endocrinol Metabol. (1985) 61:851–58. doi: 10.1210/jcem-61-5-851
20. Crowley WF, Filicori M, Spratt DI, Santoro NF. The Physiology of Gonadotropin-Releasing Hormone (GnRH) Secretion in Men and Women. Recent Prog Horm Res. (1985) 41:473–531. doi: 10.1016/B978-0-12-571141-8.50015-9
21. Kalantaridou SN, Makrigiannakis A, Zoumakis E, Chrousos GP. Stress and the female reproductive system. J Reprod Immunol. (2004) 62:61–8. doi: 10.1016/j.jri.2003.09.004
22. Eskandari F, Webster JI, Sternberg EM. Neural immune pathways and their connection to inflammatory diseases. Arthritis Res Ther. (2003) 5:251–65. doi: 10.1186/ar1002
23. Haddad JJ, Saadé NE, Safieh-Garabedian B. Cytokines and neuro-immune-endocrine interactions: a role for the hypothalamic-pituitary-adrenal revolving axis. J Neuroimmunol. (2002) 133:1–19. doi: 10.1016/S0165-5728(02)00357-0
24. Lundberg U. Stress hormones in health and illness: the roles of work and gender. Psychoneuroendocrinology. (2005) 30:1017–21. doi: 10.1016/j.psyneuen.2005.03.014
25. Anisman H, Merali Z. Cytokines, stress, and depressive illness. Brain Behav Immun. (2002) 16:513–24. doi: 10.1016/S0889-1591(02)00009-0
26. Padgett DA, Glaser R. How stress influences the immune response. Trends Immunol. (2003) 24:444–48. doi: 10.1016/S1471-4906(03)00173-X
27. Jaferi A, Bhatnagar S. Corticotropin-releasing hormone receptors in the medial prefrontal cortex regulate hypothalamic-pituitary-adrenal activity and anxiety-related behavior regardless of prior stress experience. Brain Res. (2007) 1186:212–23. doi: 10.1016/j.brainres.2007.07.100
28. Paredes S, Ribeiro L. Cortisol: the villain in metabolic syndrome? Rev Assoc Med Bras. (2014) 60:84–92. doi: 10.1590/1806-9282.60.01.017
29. Sohn JW. Network of hypothalamic neurons that control appetite. BMB Rep. (2015) 48:229–33. doi: 10.5483/BMBRep.2015.48.4.272
30. Morton GJ, Meek TH, Schwartz MW. Neurobiology of food intake in health and disease. Nat Rev Neurosci. (2014) 15:367–78. doi: 10.1038/nrn3745
31. Varela L, Horvath TL. Leptin and insulin pathways in POMC and AgRP neurons that modulate energy balance and glucose homeostasis. EMBO Rep. (2012) 13:1079–86. doi: 10.1038/embor.2012.174
32. Lehman MN, Hileman SM, Goodman RL. Neuroanatomy of the kisspeptin signaling system in mammals: comparative and developmental aspects. In: Kauffman AS, Smith JT, editors. Kisspeptin Signaling in Reproductive Biology Advances in Experimental Medicine and Biology. Springer Science+ Bussiness Media, LCC (2013). p. 27–62. doi: 10.1007/978-1-4614-6199-9_3
33. Matsuda F, Ohkura S, Magata F, Munetomo A, Chen J, Sato M, et al. Role of kisspeptin neurons as a gnrh surge generator: comparative aspects in rodents and non-rodent mammals. J Obstetrics Gynaecol Res. (2019) 45:2318–29. doi: 10.1111/jog.14124
34. Smith T, Dungan HM, Stoll EA, Gottsch ML, Braun RE, Eacker SM, et al. Differential regulation of KiSS-1 MRNA expression by sex steroids in the brain of the male mouse. Endocrinology. (2005) 146:2976–84. doi: 10.1210/en.2005-0323
35. Roseweir AK, Kauffman AS, Smith JT, Guerriero KA, Morgan K, Pielecka-Fortuna J, et al. Discovery of potent kisspeptin antagonists delineate physiological mechanisms of gonadotropin regulation. J Neurosci. (2009) 29:3920–29. doi: 10.1523/JNEUROSCI.5740-08.2009
36. Mittelman-Smith MA, Wong AM, Micevych PE. Estrogen and progesterone integration in an in vitro model of RP3V kisspeptin neurons. Neuroendocrinology. (2018) 106:101–15. doi: 10.1159/000471878
37. Iwasa T, Matsuzaki T, Yano K, Mayila Y, Irahara M. the roles of kisspeptin and gonadotropin inhibitory hormone in stress-induced reproductive disorders. Endocr J. (2018) 65:133–40. doi: 10.1507/endocrj.EJ18-0026
38. Tng EL. Kisspeptin signalling and its roles in humans. Singapore Med J. (2015) 56:649–56. doi: 10.11622/smedj.2015183
39. Clarkson J, Han SY, Piet R, McLennan T, Kane GM, Ng J, et al. Definition of the hypothalamic GnRH ||. Proc Natl Acad Sci USA. (2017) 114:E10216–23. doi: 10.1073/pnas.1713897114
40. Castellano JM, Roa J, Luque RM, Dieguez C, Aguilar E, Pinilla L, et al. KiSS-1/Kisspeptins and the metabolic control of reproduction: physiologic roles and putative physiopathological implications. Peptides. (2009) 30:139–45. doi: 10.1016/j.peptides.2008.06.007
41. Rivier C, Brownstein M, Spiess J, Rivier J, Vale W. In Vivo Corticotropin-releasing factor-induced secretion of adrenocorticotropin, beta-endorphin, and corticosterone. Endocrinology. (1982) 110:272–78. doi: 10.1210/endo-110-1-272
42. Duval F, González F, Rabia H Neurobiology of Stress. www.sonepsyn.cl (2010) 48:307–18. doi: 10.4067/S0717-92272010000500006
43. Iwasa T, Matsuzaki T, Yano K, Irahara M. Gonadotropin-inhibitory hormone plays roles in stress-induced reproductive dysfunction. Front Endocrinol. (2017) 8:62. doi: 10.3389/fendo.2017.00062
44. Ducret E, Anderson GM, Herbison AE. RFamide-related peptide-3, a mammalian gonadotropin-inhibitory hormone ortholog, regulates gonadotropin-releasing hormone neuron firing in the mouse. Endocrinology. (2009) 150:2799–804. doi: 10.1210/en.2008-1623
45. Putteeraj M, Soga T, Ubuka T, Parhar IS. A ‘Timed’ kiss is essential for reproduction: lessons from mammalian studies. Front Endocrinol. (2016) 7:1–10. doi: 10.3389/fendo.2016.00121
46. Dedic N, Chen A, Deussing JM. The CRF family of neuropeptides and their receptors - mediators of the central stress response. Curr Mol Pharmacol. (2017) 11:4–31. doi: 10.2174/1874467210666170302104053
47. Li K, Nakajima M, Ibañez-Tallon I, Heintz N. A cortical circuit for sexually dimorphic oxytocin-dependent anxiety behaviors. Cell. (2016) 167:60–72. doi: 10.1016/j.cell.2016.08.067
48. Bangasser DA, Wiersielis KR. Sex differences in stress responses: a critical role for corticotropin-releasing factor. Hormones. (2018) 17:5–13. doi: 10.1007/s42000-018-0002-z
49. Bangasser DA, Ordoñez E. Modulating chronic stress. Elife. (2020) 9:1–3. doi: 10.7554/eLife.63996
50. Bangasser DA, Wicks B. Sex-specific mechanisms for responding to stress. J Neurosci Res. (2017) 95:75–82. doi: 10.1002/jnr.23812
51. Hodes GE, Pfau ML, Purushothaman I, Ahn HF, Golden SA, Christoffel DJ, et al. Sex differences in nucleus accumbens transcriptome profiles associated with susceptibility versus resilience to subchronic variable stress. J Neurosci. (2015) 35:16362–76. doi: 10.1523/JNEUROSCI.1392-15.2015
52. Roberts CJ, Campbell IC, Troop N. Increases in weight during chronic stress are partially associated with a switch in food choice towards increased carbohydrate and saturated fat intake. Eur Eat Disord Rev. (2014) 22:77–82. doi: 10.1002/erv.2264
53. Sominsky L, Spencer SJ. Eating behavior and stress: a pathway to obesity. Front Psychol. (2014) 5:1–8. doi: 10.3389/fpsyg.2014.00434
54. Epel E, Lapidus R, Mc Ewen B, Brownell K. Stress may add bite to appetite in women: a laboratory study of stress-induced cortisol and eating behavior. Psychoneuroendocrinology. (2001) 26:37–49. doi: 10.1016/S0306-4530(00)00035-4
55. Demori I, Grasselli E. Stress-related weight gain: mechanisms involving feeding behavior, metabolism, gut microbiota and inflammation. J Nutr Food Sci. (2016) 6:1000457. doi: 10.4172/2155-9600.1000457
56. Kovačević S, Nestorov J, Matić G, Elaković I. Chronic stress combined with a fructose diet reduces hypothalamic insulin signaling and antioxidative defense in female rats. Neuroendocrinology. (2019) 108:278–90. doi: 10.1159/000496391
57. Chao AM, Jastreboff AM, White MA, Grilo CM, Sinha R. Stress, cortisol, and other appetite-related hormones: prospective prediction of 6-month changes in food cravings and weight. Obesity (Silver Spring). (2017) 25:713–20. doi: 10.1002/oby.21790
58. Somogyi V, Gyorffy A, Scalise TJ, Kiss DS, Goszleth G, Bartha T, et al. Endocrine factors in the hypothalamic regulation of food intake in females: a review of the physiological roles and interactions of ghrelin, leptin, thyroid hormones, oestrogen and insulin. Nutr Res Rev. (2011) 24:132–54. doi: 10.1017/S0954422411000035
59. Jequier E. Leptin signaling, adiposity and energy balance. Annals of NY Acad Sci. (2002) 967:379–88. doi: 10.1111/j.1749-6632.2002.tb04293.x
60. Schliep KC, Mumford SL, Vladutiu CJ, Ahrens KA, Perkins NJ, Sjaarda LA, et al. Perceived stress, reproductive hormones, and ovulatory function. Epidemiology. (2015) 26:177–84. doi: 10.1097/EDE.0000000000000238
61. Podfigurna A, Maciejewska-Jeske M, Meczekalski B, Genazzani AD. Kisspeptin and LH Pulsatility in patients with functional hypothalamic amenorrhea. Endocrine. (2020) 70:635–43. doi: 10.1007/s12020-020-02481-4
62. Marshall LA. Clinical evaluation of amenorrhea in active and athletic women. Clin Sports Med. (1994) 13:371–87. doi: 10.1016/S0278-5919(20)30336-7
63. Sills ES, Walsh AP. The GPR54-kisspeptin complex in reproductive biology: neuroendocrine significance and implications for ovulation induction and contraception. Neuro Endocrinol Lett. (2008) 29:846–51. Available online at: www.nel.edu.
64. Ghebreyesus TA,. (2020) WHO Director-general's opening remarks at the media briefing on COVID-19 - 11 March 2020. WHO Director General's Speeches, no March: 4. Available online at: https://wwwwhoint/dg/speeches/detail/who-director-general-s-opening-remarks-at-the-media-briefing-on-covid-19-−11-march- (2020).
65. Deger VB. Eating Behavior Changes of People with Obesity during the Covid-19 Pandemic. Diabetes Metabol Syndrome Obesity Targets Ther. (2021) 14:1987–97. doi: 10.2147/DMSO.S305782
66. Pearl RL. (2020). Weight Stigma and the ‘Quarantine 15.’ Obesity (Silver Spring) 28:1180–81. doi: 10.1002/oby.22850
67. McAtamney K, Mantzios M, Egan H, Wallis DJ. Emotional Eating during COVID-19 in the United Kingdom: Exploring the Roles of Alexithymia and Emotion Dysregulation. Appetite. (2021) 161:105120. doi: 10.1016/j.appet.2021.105120
68. Sadler JR, Thapaliya G, Jansen E, Aghababian AH, Smith KR, Carnell S. For Stress-Related Palatable Food Intake in U.S. Adults. Nutrients. (2021) 13:901. doi: 10.3390/nu13030901
69. Novotný JS, Gonzalez-Rivas JP, Kunzová Š, Skladaná M, Pospíšilová A, Polcrová A, et al. Risk Factors Underlying COVID-19 Lockdown-induced mental distress. Front Psychiatry. (2020) 11:1–11. doi: 10.3389/fpsyt.2020.603014
70. Risbroug B, Stein MB. Role of corticotropin releasing factor in anxiety disorders: a translational research perspective. Horm Behav. (2006) 50:550–61. doi: 10.1016/j.yhbeh.2006.06.019
71. Hariri AR, Drabant EM, Munoz KE, Kolachana BS, Mattay VS, Egan MF, et al. A susceptibility gene for affective disorders and the response of the human amygdala. Arch Gen Psychiatry. (2005) 62:146–52. doi: 10.1001/archpsyc.62.2.146
72. Palanza P. Animal models of anxiety and depression: how are females differents? Neurosci Biobehav Rev. (2001) 25:219–33. doi: 10.1016/S0149-7634(01)00010-0
73. Zimmerberg B, Farley MJ. Sex differences in anxiety behaviour in rats: role of gonadal hormones. Physiol Behav. (1993) 54:1119–24. doi: 10.1016/0031-9384(93)90335-D
74. Seeman MV. Psychopathology in women and men: focus on female hormones. Am J Psychiatry. (1997) 154:1641–47. doi: 10.1176/ajp.154.12.1641
75. Wittchen H-U. Generalized anxiety disorder: Prevalance burder, and cost ot society. Depress Anxiety. (2002) 62:162–71. doi: 10.1002/da.10065
76. Demir O, Hidayet S, Comba C. Triangle of COVID, anxiey and menstrual cycle. J Obstet Gynaecol. (2021) 41:1257–61. doi: 10.1080/01443615.2021.1907562
77. Takmaz T, Gundogmus I, Okten SB, Gunduz A. The impact of COVID-19-related mental health issues on menstrual cycle characteristics of female healthcare providers. J Obstetrics Gynaecol Res. (2021) 47:3241–49. doi: 10.1111/jog.14900
78. Phelan N, Behan LA, Owens L. The Impact of the COVID-19 Pandemic on Women's reproductive health. Front Endocrinol. (2021) 12:1–8. doi: 10.3389/fendo.2021.642755
79. Nguyen BT, Pang RD, Nelson AL, Pearson JT, Noccioli EB, Reissner HR, et al. Detecting variations in ovulation and menstruation during the COVID-19 pandemic, using real-world mobile app data. PLoS ONE. (2021) 16:1–12. doi: 10.1371/journal.pone.0258314
80. Knell G, Robertson MC, Dooley EE, Burford K, Mendez KS. Health behavior changes during Covid-19 pandemic and subsequent ‘stay-at-home’ orders. Int J Environ Res Public Health. (2020) 17:1–16. doi: 10.3390/ijerph17176268
81. Metz S, Duesenberg M, Hellmann-Regen J, Wolf OT, Roepke S, Otte C, et al. Blunted salivary cortisol response to psychosocial stress in women with posttraumatic stress disorder. J Psychiatr Res. (2020) 130:112–19. doi: 10.1016/j.jpsychires.2020.07.014
Keywords: menstrual cycle, kisspeptin, obesity, stress, anxiety, ovulation
Citation: Vigil P, Meléndez J, Soto H, Petkovic G, Bernal YA and Molina S (2022) Chronic Stress and Ovulatory Dysfunction: Implications in Times of COVID-19. Front. Glob. Womens Health 3:866104. doi: 10.3389/fgwh.2022.866104
Received: 30 January 2022; Accepted: 04 April 2022;
Published: 23 May 2022.
Edited by:
Geetha Desai, National Institute of Mental Health and Neurosciences (NIMHANS), IndiaReviewed by:
Elizabeth Thomas, Monash Alfred Psychiatry Research Centre, AustraliaCopyright © 2022 Vigil, Meléndez, Soto, Petkovic, Bernal and Molina. This is an open-access article distributed under the terms of the Creative Commons Attribution License (CC BY). The use, distribution or reproduction in other forums is permitted, provided the original author(s) and the copyright owner(s) are credited and that the original publication in this journal is cited, in accordance with accepted academic practice. No use, distribution or reproduction is permitted which does not comply with these terms.
*Correspondence: Pilar Vigil, cGlsYXJ2aWdpbEByaHJpLmNs